HSP90 and Its Novel Co-Chaperones, SGT1 and CHP-1, in Brain of Patients with Parkinson’s Disease and Dementia with Lewy Bodies
Abstract
Background:
Parkinson’s disease (PD) is a neurodegenerative disorder characterized by the presence of inclusions known as Lewy bodies in some brain regions. Lewy bodies consist of α-synuclein and many other proteins including chaperones.
Objective:
To learn more about the role of chaperone complexes in PD and a related disorder, i.e., dementia with Lewy bodies (DLB), in this work we analyzed the expression of HSP90 and its two quite recently identified co-chaperones, SGT1 and CHP-1, in selected brain regions from patients suffering from these diseases.
Methods:
To fulfill the aim of our study we used human material and applied immunohistochemistry, Western blot analysis and real time/quantitative PCR (RT-qPCR).
Results:
We have found that HSP90 mRNA level is higher in the temporal cortex of PD and in frontal cortex of DLB brains, even though level of protein does not change significantly. The mRNA level of SGT1 is higher in the frontal and temporal cortex of PD and in substantia nigra of DLB brains while no significant changes in the level of protein were noticed. Similarly, the mRNA level of CHP-1 was found to be higher in the frontal and temporal cortex of PD and in all examined regions i.e. substantia nigra, frontal and temporal cortex of DLB brains. In the case of CHP-1 the protein level was found to be higher in frontal cortex of PD and in all examined areas of DLB patients.
Conclusions:
Our data indicate that the level of HSP90, SGT1 and CHP-1 is upregulated in the majority of cases of PD and DLB, which suggests that the examined proteins might be involved in these pathologies.
INTRODUCTION
Parkinson’s disease (PD) is, after Alzheimer’s disease, the second, most common neurodegenerative disorder caused by a selective loss of dopaminergic neurons in the substantia nigra (SN) [1]. Neurodegeneration within the nigrostriatal system is a dominant, but not the only pathological process characterizing PD. The pathology of PD also includes changes in dopaminergic neurons of the mesocorticolimbic and hypothalamic dopaminergic systems. In the late stages of the disease, cholinergic (the basal nucleus of Meynert), serotonergic (raphe nuclei) and noradrenergic (locus coeruleus) systems are also affected. It has been shown that more than 80% of patients with PD present with pathology of the autonomic system within the central (changes in the brain stem and hypothalamus) and peripheral (changes in cardiac plexuses, intramural plexuses of the intestine and sympathetic ganglia) nervous system. Common symptoms of the autonomic system dysfunction are constipation, salivation, seborrhea, orthostatic hypotension, cardiac arrhythmia or dysphagia [2–8]. One of the hallmarks of PD is the presence of distinctive inclusion bodies known as Lewy bodies in brain stem neurons. Lewy bodies contain α-synuclein and many other proteins including ubiquitin, chaperones, etc. Lewy bodies are also a characteristic hallmark of dementia with Lewy bodies (DLB). DLB causes a progressive decline in mental abilities such as visual hallucinations, confusion, poor attention, visual-spatial problems and memory loss [9–11]. Signs of PD (parkinsonian symptoms), such as bradykinesia, rigid muscles, tremor or gait disorder are the most common manifestations in up to 70% of patients with DLB [12]. When present, these symptoms may be as severe in DLB as in PD [13].
Chaperones are involved in proper folding of their client proteins and may assist in the decision whether to refold a misfolded protein or to target it for degradation [14]. Importantly, in PD pathology, chaperones are capable of preventing α-synuclein misfolding, oligomerization and formation of aggregates as shown by studies performed in vitro and on animal models of PD [15]. Regarding DLB pathology, the transcriptome analysis in frontal cortex revealed upregulation of some chaperones when compared to healthy controls [16]. However, up to now, literature data concerning the localization/level and function of chaperone proteins in both diseases are very limited.
The best known/studied chaperone is HSP90. It is a ubiquitously expressed chaperone that plays a central role in folding and stabilization of its client proteins, and in preventing protein aggregation [17–19]. Some studies identified HSP90 as a predominant chaperone implicated in α-synuclein pathology since it was found to be the most abundant protein co-localizing with α-synuclein within Lewy bodies [20]. The activity of HSP90 is tightly regulated by many factors including co-chaperones. The list of HSP90 co-chaperones is long and still open. Among them are the best characterized p23 or Aha1, and many others including those identified quite recently such as SGT1 or CHP-1 [18].
SGT1 (Suppressor of G2 allele of skp1) has been identified in yeast and later found in other organisms including mammals [21, 22]. In yeast, the SGT1 protein is composed of three major domains, i.e., TPR (tetratricopeptide repeat), CS (present in CHORD-containing proteins and in SGT1) and SGS (SGT1 specific domain). The TPR domain is present in several other proteins and, importantly, may be responsible for the chaperone function of SGT1 [23]. Indeed, SGT1 was found to bind to HSP90 [24, 25]. Subsequent studies have revealed that SGT1 exhibits co-chaperone properties and that the C-terminal SGS domain is involved in the interaction with HSP90 [26, 27]. Regarding the possible role of SGT1 in neurodegenerative diseases, there is only one study describing its distribution in different brain areas of patients with Alzheimer disease [28].
CHP-1 (CHORD containing protein-1) is expressed in different tissues including postmitotic ones such as brain and skeletal muscle [29]. CHP-1 has been described as a regulator of centrosome duplication and as a protein involved in the maintenance of genomic stability in Drosophila and mouse [30]. Wu et al. [31] described the interaction of Morgana/CHP-1 with the molecular chaperone HSP90. Further studies of chaperone properties of CHP-1 have revealed that overexpression of this protein in NIH3T3 fibroblasts leads to increased stress resistance of these cells. Also, it has been found that upregulation of CHP-1 in response to transient global brain ischemia lasts longer in ischemia-resistant regions of the gerbil hippocampus than in vulnerable ones. These data suggest that CHP-1 may also be involved in protective mechanisms in vivo [32, 33].
Taking into account the potential involvement of HSP90 and other chaperones/co-chaperones in PD and DLB, in this work we analyzed the expression of HSP90 along with SGT1 and CHP-1, in selected brain regions of PD and DLB patients. For our studies we have chosen structures, which are mostly affected by these diseases, i.e., substantia nigra (SN) and the frontal and temporal cortex. We have performed the analyses on human material employing immunohistochemistry, Western blot and real time/quantitative PCR (RT-qPCR).
MATERIALS AND METHODS
Human material
Human brain tissue from 3-4 patients with PD (age 80–84), DLB (age 74–80) and controls (age 60–77) from the Brain Bank of the Division of Neuropathology, Neuromed Campus, Kepler University Hospital, were analyzed. PD and DLB patients were diagnosed on the basis of medical history, review of signs and symptoms, neurological and physical examination, and post mortem neuropathological analysis (Supplementary Tables 1–3) [34–40]. Following the Austrian legal procedures, every patient who dies in a hospital can undergo autopsy for diagnostic and/or scientific purposes. Based on the request from the Ethical Committee of the State of Upper Austria upon hospital admission, patients sign a written consent for scientific evaluation of their brain after death. Established diagnostic criteria were used to assign brains to normal or disease groups as already described [13, 41, 42]. Briefly, after removal from the skull, the brain hemispheres are separated from each other by a mid-sagittal cut. One hemisphere is fixed for one week in 4% formaldehyde. Brain regions were then cut and embedded in paraffin. The other fresh hemisphere is cut into 1 cm thick coronal slabs which are immediately frozen at –80°C. For immunohistochemical analysis, formalin-fixed, paraffin-embedded sections of substantia nigra (SN), frontal cortex (FC) and temporal cortex (TC) of 5μm thickness were used. For Western blot and RT-qPCR analysis, samples from the frozen tissue were obtained by using a special trepan.
Preparation of tissue lysates
Frozen tissue from cerebral cortex (frontal and temporal) and substantia nigra, derived from human adult brain diagnosed with PD (mean age – 82 years) and corresponding controls with no signs of neurological or psychiatric disorders was homogenized 4 times for 20 s at 6000 rpm using a Polytron (Qiagen) in a solution containing 50 mM Tris pH 7.5, 1 mM EDTA, 2 mM DTT, 150 mM NaCl and protease inhibitors. The homogenate was then centrifuged at 20000×g for 45 min and protein concentration in the supernatant fraction was estimated by the Bradford’s procedure with bovine serum albumin as a standard. 30μg of protein from the supernatant fraction was when subjected to SDS–PAGE and Western blot.
Immunohistochemical staining
For staining of human formalin-fixed, paraffin-embedded sections the following antibodies were used: mouse monoclonal antibodies against HSP90 (Santa Cruz) diluted 1:200, rabbit polyclonal antibodies against SGT1 (Proteintech) diluted 1:200 and rabbit polyclonal against CHP-1 (Abcam) diluted 1:200. All steps were performed using UltraVision Quanto Detection System DAB (Thermo Scientific) according to manufacturer’s protocol.
Shortly, sections were preincubated with methanol/H2O2 to block endogenous peroxidases, washed with phosphate buffered saline and incubated with a given primary antibody. Thereafter, the sections were processed according to the avidin–biotin method (Vectastain-peroxidase kit; Vector, Burlingame, CA), and the reaction product was visualized with 3,3′-diaminobenzidine (DAB). Sections were then counterstained with Nissl and analyzed using a bright field microscope (Nikon Eclipse E 400). In all cases control sections were included in which the primary or secondary antibodies were omitted. No immunopositive reaction was observed for any control section (data not shown).
Quantitative analysis
Analysis of immunopositive cell numbers stained against HSP90, SGT1, and CHP-1 were performed in substantia nigra and in frontal and temporal cortex of three PD and three DLB patients and three corresponding control subjects. Three to five microphotographs from each structure were collected and analyses using the ImageJ software in order to determine the cell number in the region of interest (ROI) calculated by the software. The same square area (720×960 pixels) of ROI was applied for all microphotographs. Number of all Nissl counterstained cells with visible blue nuclei defined as total cell number (TCN) has been counted within the frame of ROI, as well as the immunopositive cell number (ICN) within the same ROI. The ratio of ICN to TCN obtained for PD/DLB was then divided by the ratio of ICN to TCN obtained for control and presented in %.
SDS-PAGE, Western blotting and densitometric analysis
Gel electrophoresis with 12% (w/v) polyacrylamide containing 0.1% SDS was performed by the method of Laemmli [43]. The levels of HSP90, SGT1 and CHP-1 were studied using mouse monoclonal antibody against HSP90 (BD Biosciences) diluted 1:2000, rabbit polyclonal antibody against SGT1 (Proteintech) diluted 1:4000, rabbit polyclonal antibody against CHP-1 (Thermo Scientific) diluted 1:2000 and mouse monoclonal antibody against β-actin conjugated with horseradish peroxidase (Sigma) diluted 1:10 000. In the case of HSP90, SGT1 and CHP-1, the blots were allowed to react with secondary anti-mouse (Jackson ImmunoResearch) or anti-rabbit (Millipore) antibodies conjugated to horseradish peroxidase diluted 1:10 000 and developed with the ECL chemiluminescence kit (BioRad) followed by exposure against an X-ray film. The intensities of protein bands on immunoblots were quantified using the Ingenius densitometer and the Gene Tools Software (Syngene) with β-actin as a reference protein.
Real time/quantitative PCR (RT-qPCR)
Total RNA was isolated from brain sample (300μg) using the RNeasy kit (Qiagen), RNA concentration was measured using nanodrop equipment (Eppendorf). RNA (1μg) was then reverse-transcribed using M-MLV Reverse Transcriptase and random nonamers (Sigma–Aldrich). PCR reaction for SGT1 and CHP-1 was performed using TaqMan Gene Expression Assay kit (Applied Biosystems) and for HSP90 using SYBR Green and 7500 Fast real-time PCR System (Applied Biosystems). The results were analyzed by quantification with a relative standard curve and normalized to GAPDH and 18 S mRNA. The sequences of primers are shown in (Supplementary Table 4).
Statistical analysis
Results of quantitative analysis of cell numbers, results of Western blots and RT-qPCR are presented as means±SEM. Differences in mean values were tested by Student’s t-test. The level of statistical significance was set at p≤0.05.
RESULTS
To learn more about the involvement of chaperones/co-chaperones in PD pathology and relative disorders such as DLB, in this work we analyzed the expression of two quite recently described HSP90 co-chaperones, SGT1 and CHP-1, in brain structures of patients suffering from these diseases and of healthy control subjects. For our studies we have chosen structures which are mainly affected by these diseases, i.e., substantia nigra (SN), and the frontal (FC) and temporal cortex (TC). To confirm Lewy body pathology brain sections were stained for α-synuclein using anti-phospho-α-synuclein (Ser 129). Examples of such staining are shown in (Supplementary Figure 1).
As to HSP90 we have found that the level of mRNA is upregulated in the temporal cortex of PD and in the frontal cortex of DLB (Fig. 1C, Supplementary Figure 2C, and Table 1). On the other hand, no statistically significant changes in protein level are observed in any analyzed structures between control and PD or DLB patients. Furthermore, the number of cells expressing HSP90 does not change significantly between control and PD patients in all analyzed structures (Fig. 1A).
Fig.1
Presence of HSP90 in the substantia nigra, frontal and temporal cortex. (A) Representative immunohistochemical images from the control and PD group are shown. Scale bar is 60μm. Lower panel presents the analysis of HSP90 positive neurons. (B) Western blot (upper panel) and densitometric analysis of HSP90 level (lower panel). (C) RT-qPCR of HSP90 mRNA level. In (B) and (C) samples from 3 control and 3 PD patients were analyzed. White and blue bars represent control and PD cases, respectively. Error bars indicate mean±SEM; **p < 0.01.
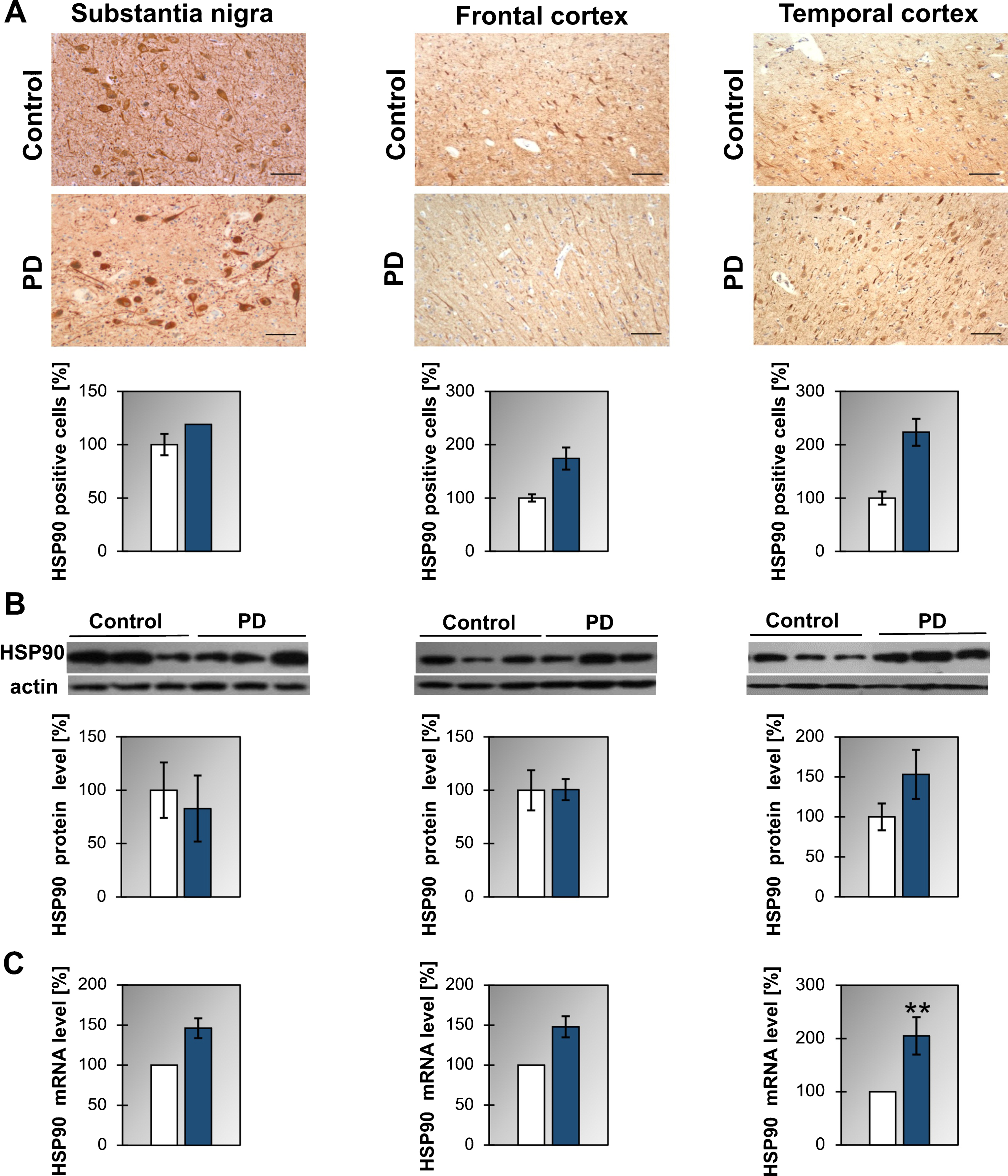
Table 1
Protein and mRNA levels of HSP90, SGT1 and CHP-1 in Parkinson’s disease and in dementia with Lewy bodies
Parkinson’s disease | |||||
HSP90 | |||||
SN | FC | TC | |||
Protein | mRNA | Protein | mRNA | Protein | mRNA |
NC | NC | NC | NC | NC | ↑ |
SGT1 | |||||
NC | NC | NC | ↑ | NC | ↑ |
CHP-1 | |||||
NC | NC | ↑ | ↑ | NC | ↑ |
Dementia with Lewy bodies | |||||
HSP90 | |||||
NC | NC | NC | ↑ | NC | NC |
SGT1 | |||||
NC | ↑ | NC | NC | NC | NC |
CHP-1 | |||||
↑ | ↑ | ↑ | ↑ | ↑ | ↑ |
SN, substantia nigra; FC, frontal cortex; TC, temporal cortex; ↑ and NC - statistically significant and non-significant changes, respectively, in comparison to controls.
Regarding SGT1 we have found that the level of mRNA is upregulated in temporal and frontal cortex of PD and in the substantia nigra of DLB patients (Fig. 2C, Supplementary Figure 3C and Table 1) while the level of the SGT1 protein did not change (Fig. 2B, Supplementary Figure 3A). Interestingly, the number of cells expressing SGT1 was significantly higher in the frontal cortex of PD patients (Fig. 2A).
Fig.2
Presence of SGT1 in the substantia nigra, frontal and temporal cortex. (A) Representative immunohistochemical images from the control and PD group are shown. Scale bar is 60μm. Lower panel presents the analysis of SGT1 positive neurons. (B) Western blot (upper panel) and densitometric analysis of SGT1 level (lower panel). (C) RT-qPCR of SGT1 mRNA level. In (B) and (C) samples from 3-4 control and 3-4 PD patients were analyzed. White and orange bars represent control and PD cases, respectively. Error bars indicate mean±SEM; *p < 0.05.
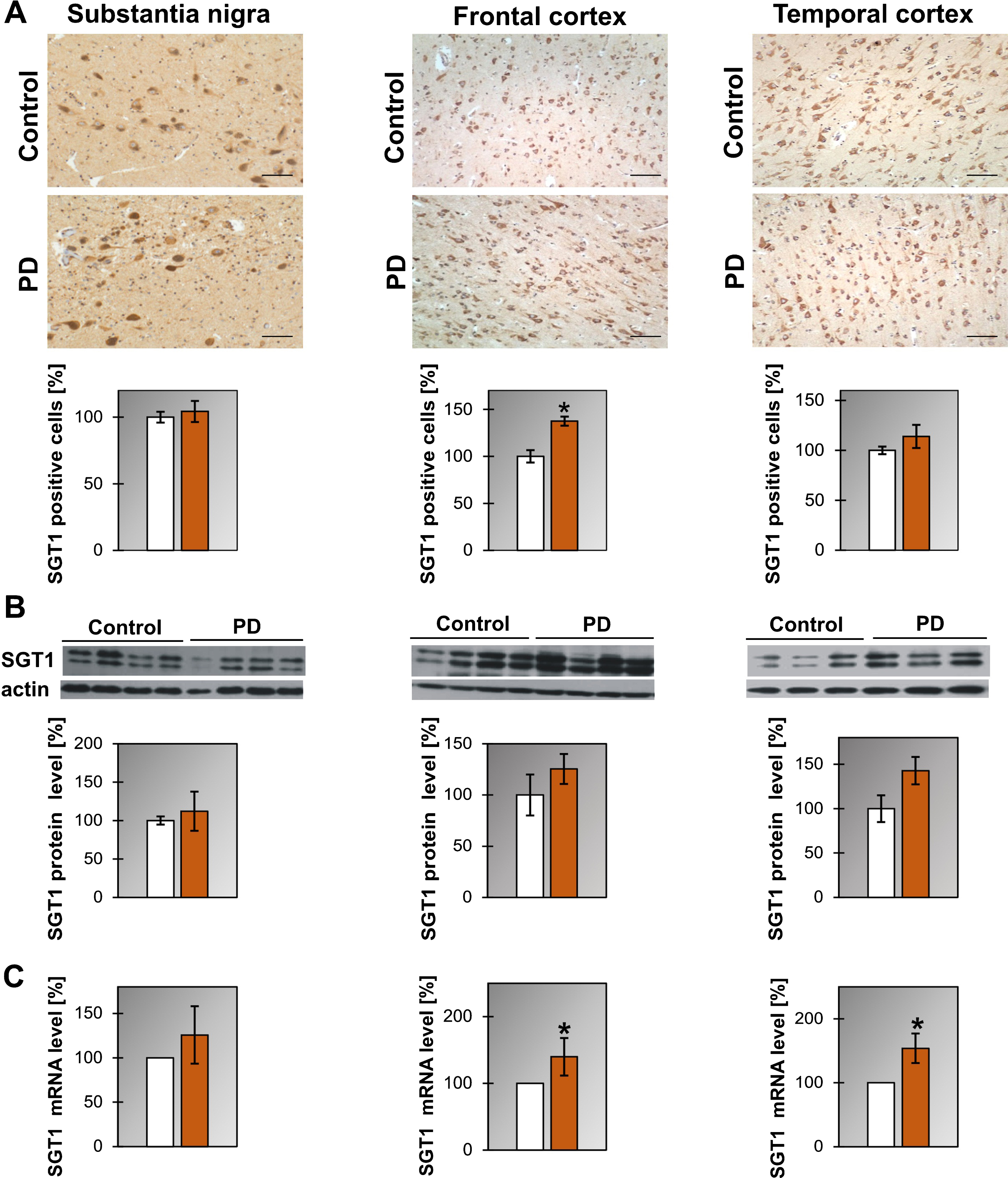
Similarly as it was found for SGT1 the level of mRNA for CHP-1 in PD patients was upregulated in the frontal and temporal cortex (Fig. 3C and Table 1) while the protein level increased only in the frontal cortex. Also, the number of cells expressing CHP-1 was significantly higher in the frontal cortex of PD patients (Fig. 3A). Concerning DLB, in all regions examined, the level of mRNA of CHP-1 was upregulated significantly when compared to controls (Supplementary Figure 4B and Table 1). Changes in CHP-1 protein level basically mirror the corresponding changes in mRNA, except for the frontal cortex (Supplementary Figure 4A and Table 1).
Fig.3
Presence of CHP-1 in the substantia nigra, frontal and temporal cortex. (A) Representative immunohistochemical images from the control and PD group are shown. Scale bar is 60μm. Lower panel presents the analysis of CHP-1 positive neurons. (B) Western blot (upper panel) and densitometric analysis of CHP-1 level (lower panel). (C) RT-qPCR of CHP-1 mRNA level. In (B) and (C) samples from 3 control and 3 PD patients were analyzed. White and yellow bars represent control and PD cases, respectively. Error bars indicate mean±SEM; *p < 0.05; ***p < 0.001.
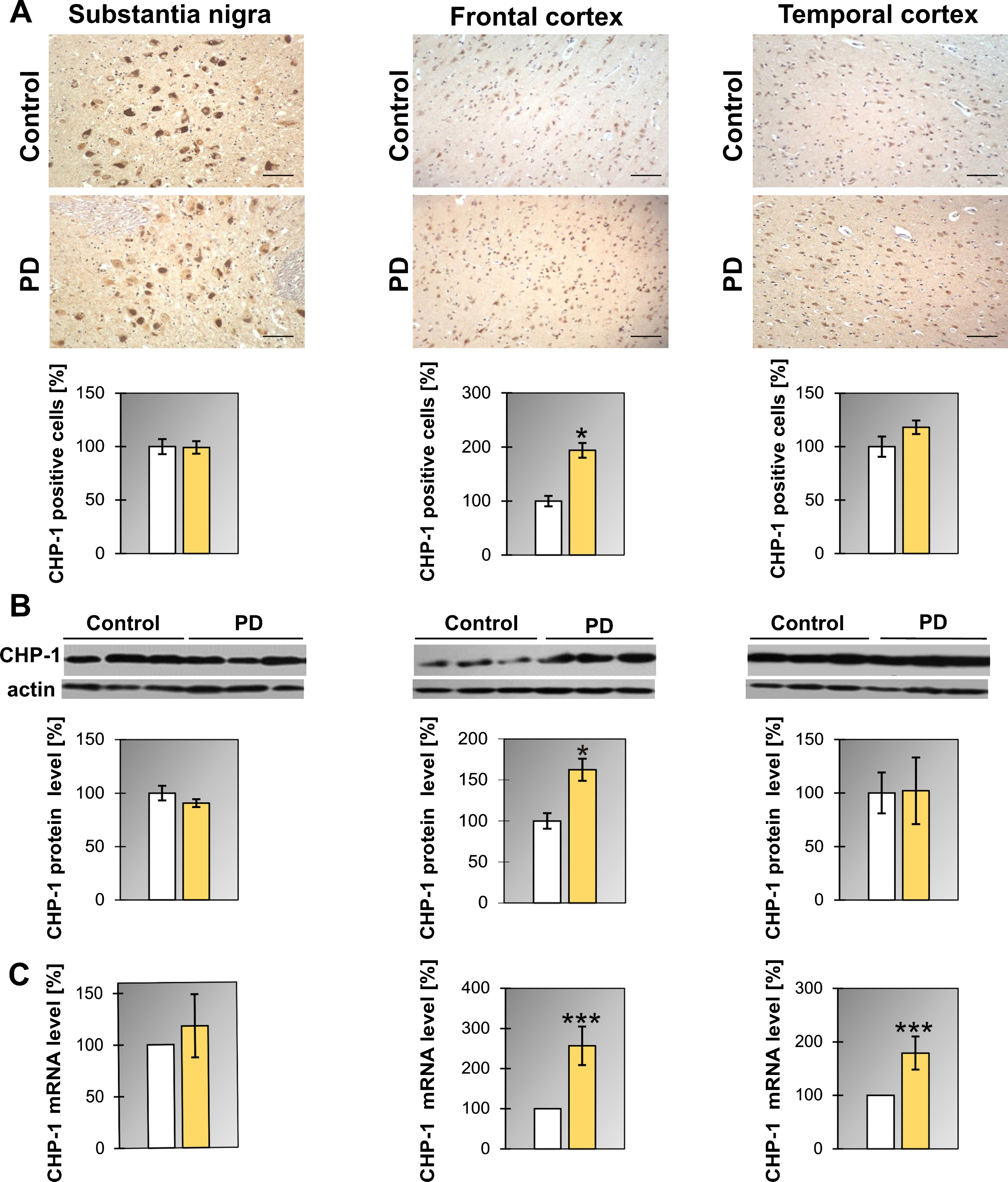
All these data show that in both PD and DLB brain the expression of HSP90, SGT1 and CHP-1 seems to be increased. Interestingly, the most pronounced changes are observed for mRNA and protein level of CHP-1 in all examined regions in DLB. To learn more about the possible influence of HSP90, SGT1 and CHP-1 on α-synuclein processing/functioning in the cell co-localization of these proteins with Lewy bodies in the substantia nigra was assessed. The results of immunohistochemical staining are shown in (Fig. 4). As it can be seen in this figure HSP90 and CHP-1 are found in Lewy bodies of PD and DLB brain while SGT1 is not. This suggests that CHP-1, but not SGT1, associates with aggregated/phosphorylated α-synuclein.
Fig.4
Localization of HSP90, SGT1 and CHP-1 in Lewy bodies in the substantia nigra of PD (upper panel) and DLB brain (lower panel). Scale bar is 50μm.
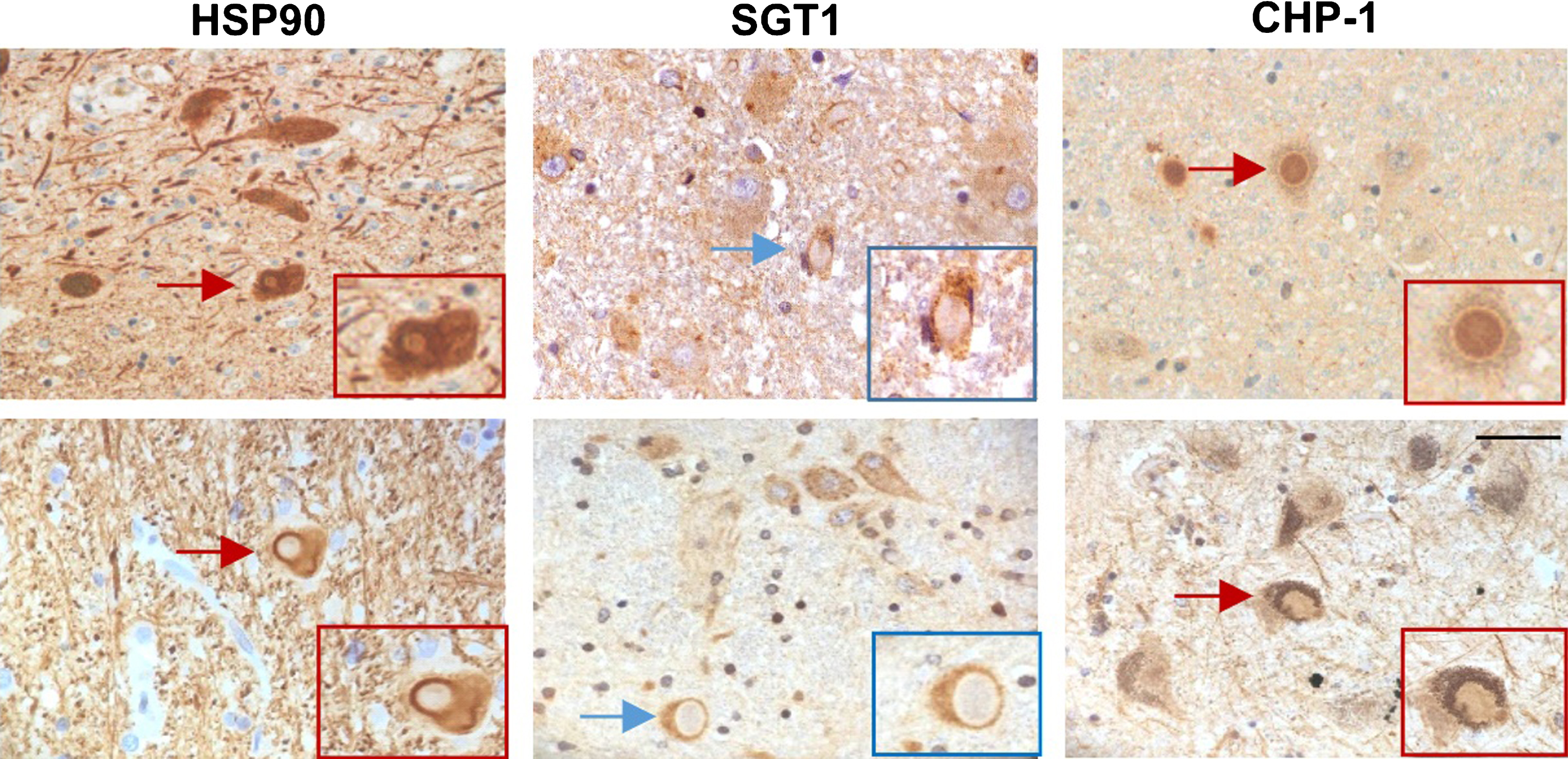
DISCUSSION
In the present work we have analyzed the expression of HSP90 and its two co-chaperones, SGT1 and CHP-1, in selected brain regions of PD and DLB patients. We have found that in both PD and DLB brain the expression of HSP90, SGT1 and CHP-1 seems to be increased. More pronounced changes are observed for the CHP-1 protein, particularly in the DLB cases.
The presence of molecular chaperones, HSP90, HSP70, HSP60, HSP40, and HSP27, in inclusions characteristic for PD and DLB, has been previously shown [20, 44, 45]. Moreover, regarding HSP90, the published results reveal an elevated level of HSP90 in Lewy bodies of PD patients and a considerable extent of co-localization of HSP90 with ubiquitinated α-synuclein [20]. In vitro experiments also show that HSP90 can both abolish α-synuclein binding to vesicles and promote fibril formation in an ATP-dependent manner [46]. More recent in vitro studies indicate that HSP90 interaction with the A53T mutant of α-synuclein prevents its aggregation [47] and that deletion of Hsp82 in yeast enhances α-synuclein toxicity, specifically, by increasing the level of reactive oxygen species [48]. However, Putcha et al. [49] showed that specific HSP90 inhibition prevents α-synuclein oligomerization and toxicity in the H4 neuroglioma cell line. Thus, the involvement of HSP90 in PD and DLB pathology, although extensively studied, is still obscure.
Our results for the first time describe the expression of SGT1 and CHP-1, HSP90 co-chaperones, in PD and DLB brains. They show, in general, that in the frontal and temporal cortex of PD brain, expression of these co-chaperones is increased while for DLB the expression of SGT1 did not change. Interestingly, in the substantia nigra of PD brains there is no significant change in expression of the examined proteins while in the same structure of DLB brain the change in expression of SGT1 and CHP-1 is more evident, particularly for CHP-1. Whereas Lewy bodies are found mainly within the brainstem in the case of PD brain, their widespread distribution through almost all brain areas is a characteristic feature of DLB [50]. Lewy bodies are found in the frontal cortex, midbrain and brainstem nuclei, dorsal efferent nucleus of the vagus, basal forebrain nuclei, and limbic cortical regions at a relatively high percentage than in PD [51]. It is possible that the upregulation of chaperone/co-chaperone mRNA and protein levels, particularly for CHP-1 in patients with DLB, as described in the present work, is associated with a greater number of Lewy bodies, the pathological structures of which chaperones constitute one of the components. A higher level of CHP-1 may represent a mechanism through which co-chaperone activity mitigates cellular toxicity associated with abnormal α-synuclein aggregation. Interestingly, SGT1 seems not to interact with aggregated/phosphorylated α-synuclein present in Lewy bodies which suggests a different function of these two co-chaperones in synucleinopathies.
Altogether, little is known about chaperone complexes and their role in preventing α-synuclein aggregation in PD and DLB. Thus, further studies concerning identification and function of chaperones and their regulators, such as SGT1, CHP-1 and others, using animal and cellular models of these diseases could help to elucidate the mechanisms of synucleinopathy and develop effective therapies.
CONFLICT OF INTEREST
The authors have no conflict of interest to report.
ACKNOWLEDGMENTS
Authors would like to thank Prof. W. Leśniak for a critical reading of the manuscript and B. Sobiak for help with RT-qPCR. This work has been supported by the European Union’s Horizon 2020 research and innovation programme under the Marie Sklodowska-Curie grant agreement no 665735 (Bio4Med) and by the funding from Polish Ministry of Science and Higher Education within 2016–2020 funds for the implementation of international projects (agreement no 3548/H2020/COFUND/2016/2) and by NCN grant 2014/15/B/NZ4/05041 and by statutory funds from the Nencki Institute of Experimental Biology PAS. The research subject was carried out with the use of CePT infrastructure financed by the European Union – the European Regional development Fund within the Operational Programme “Innovative Economy” for 2007–2013.
SUPPLEMENTARY MATERIAL
[1] The supplementary material is available in the electronic version of this article: http://dx.doi.org/10.3233/JPD-181443.
REFERENCES
[1] | Lan D , Wang W , Zhuang J , Zhao Z ((2015) ) Proteasome inhibitor-induced autophagy in PC12 cells overexpressing A53T mutant α-synuclein. Mol Med Rep 11: , 1655–1660. |
[2] | Ziemssen T , Reichmann H ((2007) ) Non-motor dysfunction in Parkinson’s disease. Parkinsonism Relat Disord 13: , 323–332. |
[3] | Bohnen NI , Albin RL ((2011) ) The cholinergic system and Parkinson disease. Behav Brain Res 221: , 564–573. |
[4] | Przedborski S ((2017) ) The two-century journey of Parkinson disease research. Nat Rev Neurosci 18: , 251–259. |
[5] | Surmeier DJ , Obeso JA , Halliday GM ((2017) ) Selective neuronal vulnerability in Parkinson disease. Nat Rev Neurosci 18: , 101–113. |
[6] | Poewe W , Seppi K , Tanner CM , Halliday GM , Brundin P , Volkmann J , Schrag AE , Lang AE ((2017) ) Parkinson disease. Nat Rev Dis Primers 3: , 17013. |
[7] | Dickson DW ((2018) ) Neuropathology of Parkinson disease. Relat Disord 46: (Suppl 1), S30–S33. |
[8] | Zeng XS , Geng WS , Jia JJ , Chen L , Zhang PP ((2018) ) Cellular and molecular basis of neurodegeneration in Parkinson disease. Front Aging Neurosci 10: , 109. |
[9] | Collerton D , Burn D , McKeith I , O’Brien J ((2003) ) Systematic review and meta-analysis show that dementia with Lewy bodies is a visual-perceptual and attentional-executive dementia. Dement Geriatr Cogn Disord 16: , 229–237. |
[10] | Cagnin A , Bussè C , Gardini S , Jelcic N , Guzzo C , Gnoato F , Mitolo M , Ermani M , Caffarra P ((2015) ) Clinical and cognitive phenotype of mild cognitive impairment evolving to dementia with Lewy dodies. Extra 5: , 442–449. |
[11] | Kemp J , Philippi N , Phillipps C , Demuynck C , Albasser T , Martin-Hunyadi C , Schmidt-Mutter C , Cretin B , Blanc F ((2017) ) Cognitive profile in prodromal dementia with Lewy bodies. Alzheimers Res Ther 9: , 19. |
[12] | Burn DJ , Rowan EN , Allan LM , Molloy S , O’Brien JT , McKeith IG ((2006) ) Motor subtype and cognitive decline in Parkinson’s disease, Parkinson’s disease with dementia, and dementia with Lewy bodies. J Neurol Neurosurg Psychiatry 77: , 585–589. |
[13] | McKeith IG , Galasko D , Kosaka K , Perry EK , Dickson DW , Hansen LA , Salmon DP , Lowe J , Mirra SS , Byrne EJ , Lennox G , Quinn NP , Edwardson JA , Ince PG , Bergeron C , Burns A , Miller BI , Lovestone S , Collerton D , Jansen EN , Ballard C , De Vos RA , Wilcock GK , Jellinger KA , Perry RH ((1996) ) Consensus guidelines for the clinical and pathologic diagnosis of dementia with Lewy bodies (DLB): Report of the consortium on DLB International Workshop. Neurology 47: , 1113–1124. |
[14] | Wickner S , Maurizi MR , Gottesman S ((1999) ) Posttranslational quality control: Folding, refolding, and degrading proteins. Science 286: , 1888–1893. |
[15] | Lackie RE , Maciejewski A , Ostapchenko VG , Marques-Lopes J , Choy WY , Duennwald ML , Prado VF , Prado MAM ((2017) ) The Hsp70/Hsp90 chaperone machinery in neurodegenerative diseases. Front Neurosci 11: , 254. |
[16] | Santpere G , Garcia-Esparcia P , Andres-Benito P , Lorente-Galdos B , Navarro A , Ferrer I ((2018) ) Transcriptional network analysis in frontal cortex in Lewy body diseases with focus on dementia with Lewy bodies. Brain Pathol 28: , 315–333. |
[17] | Picard D ((2002) ) Heat-shock protein 90, a chaperone for folding and regulation. Cell Mol Life Sci 59: , 1640–1648. |
[18] | McClellan AJ , Xia Y , Deutschbauer AM , Davis RW , Gerstein M , Frydman J ((2007) ) Diverse cellular functions of the Hsp90 molecular chaperone uncovered using systems approaches. Cell 131: , 121–135. |
[19] | Schopf FH , Biebl MM , Buchner J ((2017) ) The HSP90 chaperone machinery. Nat Rev Mol Cell Biol 18: , 345–360. |
[20] | Uryu K , Richter-Landsberg C , Welch W , Sun E , Goldbaum O , Norris EH , Pham CT , Yazawa I , Hilburger K , Micsenyi M , Giasson BI , Bonini NM , Lee VM , Trojanowski JQ ((2006) ) Convergence of heat shock protein 90 with ubiquitin in filamentous alpha-synuclein inclusions of alpha-synucleinopathies. Am J Pathol 168: , 947–961. |
[21] | Kitagawa K , Skowyra D , Elledge SJ , Harper JW , Hieter P ((1999) ) SGT1 encodes an essential component of the yeast kinetochore assembly pathway and a novel subunit of the SCF ubiquitin ligase complex. Mol Cell 4: , 21–33. |
[22] | Spiechowicz M , Filipek A ((2005) ) The expression and function of Sgt1 protein in eukaryotic cells. Neurobiol Exp 65: , 161–165. |
[23] | Blatch GL , Lässle M ((1999) ) The tetratricopeptide repeat: A Structural motif mediating protein-protein interactions. Bioessays 21: , 932–939. |
[24] | Takahashi A , Casais C , Ichimura K , Shirasu K ((2003) ) HSP90 interacts with RAR1 and SGT1 and is essential for RPS2-mediated disease resistance in Arabidopsis. Proc Nat Acad Sci U S A 100: , 11777–11782. |
[25] | Lee YT , Jacob J , Michowski W , Nowotny M , Kuznicki J , Chazin WJ ((2004) ) Human Sgt1 binds HSP90 through the CHORD-Sgt1 domain and not the tetratricopeptide repeat domain. J Biol Chem 279: , 16511–16517. |
[26] | Spiechowicz M , Zylicz A , Bieganowski P , Kuznicki J , Filipek A ((2007) ) Hsp70 is a new target of Sgt1—an interaction modulated by S100A6. Biochem Biophys Res Commun 357: , 1148–1153. |
[27] | Zabka M , Leśniak W , Prus W , Kuźnicki J , Filipek A ((2008) ) Sgt1 has co-chaperone properties and is up-regulated by heat shock. Biochem Biophys Res Commun 370: , 179–183. |
[28] | Spiechowicz M , Bernstein HG , Dobrowolny H , Leśniak W , Mawrin C , Bogerts B , Kuźnicki J , Filipek A ((2006) ) Density of Sgt1-immunopositive neurons is decreased in the cerebral cortex of Alzheimer’s disease brain. Neurochem Int 49: , 487–493. |
[29] | Brancaccio MN , Menini N , Bongioanni D , Ferretti R , De Acetis M , Silengo L , Tarone G ((2003) ) Chp-1 and melusin, two CHORD containing proteins in vertebrates. FEBS Lett 551: , 47–52. |
[30] | Ferretti R , Palumbo V , Di Savino A , Velasco S , Sbroggiò M , Sportoletti P , Micale L , Turco E , Silengo L , Palumbo G , Hirsch E , Teruya-Feldstein J , Bonaccorsi S , Pandolfi PP , Gatti M , Tarone G , Brancaccio M ((2010) ) Morgana/chp-1, a ROCK inhibitor involved in centrosome duplication and tumorigenesis. Dev Cell 18: , 486–495. |
[31] | Wu J , Luo S , Jiang H , Li H ((2005) ) Mammalian CHORD-containing protein 1 is a novel heat shock protein 90-interacting protein. FEBS Lett 579: , 421–426. |
[32] | Michowski W , Ferretti R , Wisniewska MB , Ambrozkiewicz M , Beresewicz M , Fusella F , Skibinska-Kijek A , Zablocka B , Brancaccio M , Tarone G , Kuznicki J ((2010) ) Morgana/CHP-1 is a novel chaperone able to protect cells from stress. Biochim Biophys Acta 1803: , 1043–1049. |
[33] | Ferretti R , Sbroggiò M , Di Savino A , Fusella F , Bertero A , Michowski W , Tarone G , Brancaccio M ((2011) ) Morgana and melusin: Two fairies chaeroning signal transduction. Cell Cycle 10: , 3678–3683 . |
[34] | Braak H , Braak E ((1991) ) Neuropathological stageing of Alzheimer-related changes. Acta Neuropathol 82: , 239–259. |
[35] | Mirra SS , Heyman A , McKeel D , Sumi SM , Crain BJ , Brownlee LM , Vogel FS , Hughes JP , van Belle G , Berg L ((1991) ) The Consortium to Establish a Registry for Alzheimer’s Disease (CERAD). Part II. Standardization of the neuropathologic assessment of Alzheimer’s disease. Neurology 41: , 479–486. |
[36] | Mirra SS , Hart MN , Terry RD ((1993) ) Making the diagnosis of Alzheimer’s disease. A primer for practicing pathologists. Arch Pathol Lab Med 117: , 132–144. |
[37] | Braak H , Del Tredici K , Rub U , de Vos RA , Jansen Steur EN , Braak E ((2003) ) Staging of brain pathology related to sporadic Parkinson’s disease. Neurobiol Aging 24: , 197–211. |
[38] | Hyman BT , Phelps CH , Beach TG , Bigio EH , Cairns NJ , Carrillo MC , Dickson DW , Duyckaerts C , Frosch MP , Masliah E , Mirra SS , Nelson PT , Schneider JA , Thal DR , Thies B , Trojanowski JQ , Vinters HV , Montine TJ ((2012) ) National Institute on Aging-Alzheimer’s Association guidelines for the neuropathologic assessment of Alzheimer’s disease. Alzheimers Dement 8: , 1–13. |
[39] | Montine TJ , Phelps CH , Beach TG , Bigio EH , Cairns NJ , Dickson DW , Duyckaerts C , Frosch MP , Masliah E , Mirra SS , Nelson PT , Schneider JA , Thal DR , Trojanowski JQ , Vinters HV , Hyman BT ((2012) ) National Institute on Aging-Alzheimer’s Association guidelines for the neuropathologic assessment of Alzheimer’s disease: A Practical approach. Acta Neuropathol 123: , 1–11. |
[40] | McKeith IG , Boeve BF , Dickson DW , Halliday G , Taylor JP , Weintraub D , Aarsland D , Galvin J , Attems J , Ballard CG , Bayston A , Beach TG , Blanc F , Bohnen N , Bonanni L , Bras J , Brundin P , Burn D , Chen-Plotkin A , Duda JE , El-Agnaf O , Feldman H , Ferman TJ , Ffytche D , Fujishiro H , Galasko D , Goldman JG , Gomperts SN , Graff-Radford NR , Honig LS , Iranzo A , Kantarci K , Kaufer D , Kukull W , Lee VMY , Leverenz JB , Lewis S , Lippa C , Lunde A , Masellis M , Masliah E , McLean P , Mollenhauer B , Montine TJ , Moreno E , Mori E , Murray M , O’Brien JT , Orimo S , Postuma RB , Ramaswamy S , Ross OA , Salmon DP , Singleton A , Taylor A , Thomas A , Tiraboschi P , Toledo JB , Trojanowski JQ , Tsuang D , Walker Z , Yamada M , Kosaka K ((2017) ) Diagnosis and management of dementia with Lewy bodies: Fourth Consensus Report of the DLB Consortium. Neurology 89: , 88–100. |
[41] | Harding AJ , Halliday GM ((1988) ) Simplified neuropathological diagnosis of dementia with Lewy bodies. Neuropathol Appl Neurobiol 24: , 195–201. |
[42] | Hurtig HI , Trojanowski JQ , Galvin J , Ewbank D , Schmidt ML , Lee VM , Clark CM , Glosser G , Stern MB , Gollomp SM , Arnold SE ((2000) ) Alpha synuclein cortical Lewy bodies correlate with dementia in Parkinson’s disease. Neurology 54: , 1916–1921. |
[43] | Laemmli UK ((1970) ) Cleavage of structural proteins during the assembly of the head of bacteriophage T4. Nature 227: , 680–685. |
[44] | McLean PJ , Kawamata H , Shariff S , Hewett J , Sharma N , Ueda K , Breakefield XO , Hyman BT ((2002) ) Torsin A and heat shock proteins act as molecular chaperones: Suppression of alpha-synuclein aggregation. J Neurochem 83: , 846–854. |
[45] | Leverenz JB , Umar I , Wang Q , Montine TJ , McMillan PJ , Tsuang DW , Jin J , Pan C , Shin J , Zhu D , Zhang J ((2007) ) Proteomic identification of novel proteins in cortical Lewy bodies. Brain Pathol 17: , 139–145. |
[46] | Falsone SF , Kungl AJ , Rek A , Cappai R , Zangger K ((2009) ) The molecular chaperone Hsp90 modulates intermediate steps of amyloid assembly of the Parkinson-related protein alpha-synuclein. J Biol Chem 284: , 31190–31199. |
[47] | Daturpalli S , Waudby CA , Meehan S , Jackson SE ((2013) ) Hsp90 inhibits alpha-synuclein aggregation by interacting with soluble oligomers. J Mol Biol 425: , 4614–4628. |
[48] | Liang J , Clark-Dixon C , Wang S , Flower TR , Williams-Hart T , Zweig R , Robinson LC , Tatchell K , Witt SN ((2008) ) Novel suppressors of alpha-synuclein toxicity identified using yeast. Hum Mol Genet 17: , 3784–3795. |
[49] | Putcha P , Danzer KM , Kranich LR , Scott A , Silinski M , Mabbett S , Hicks CD , Veal JM , Steed PM , Hyman BT , McLean PJ ((2010) ) Brain-permeable small-molecule inhibitors of Hsp90 prevent alpha-synuclein oligomer formation and rescue alpha-synuclein-induced toxicity. J Pharmacol Exp Ther 332: , 849–857. |
[50] | Beyer K , Domingo-Sàbat M , Ariza A ((2009) ) Molecular pathology of Lewy body diseases. Int J Mol Sci 10: , 724–745. |
[51] | Neef D , Walling AD ((2006) ) Dementia with Lewy bodies: An Emerging disease. Am Fam Physician 73: , 1223–1229. |