Acute Effects of 1-methyl-4-phenyl-1,2,3,6-tetrahydropyridine (MPTP) or Paraquat on Core Temperature in C57BL/6J Mice
Abstract
Background:
MPTP and paraquat are two compounds that have been used to model Parkinson’s disease in mice. Previous studies in two non-traditional strains of mice have shown that a single dose of MPTP can induce changes in body temperature, while the effects of paraquat have not been examined. Examination of body temperature is important since small fluctuations in an animal’s core temperature can significantly affect drug metabolism, and if significant enough can even culminate in an animal’s death.
Objective:
To determine how external heating can alter the survival of C57BL/6J mice following MPTP administration.
Methods:
In this study, we examine the effects of MPTP (4×20 mg/kg, 2 hours apart) and paraquat (2×10 mg/kg/week for 3 weeks) on core temperature of C57BL/6J mice. Correlations of purine and catecholamine levels were also done in mice treated with MPTP.
Results:
We find that MPTP induces a significant hypothermia in C57BL/6J mice that reduces their core temperature below the limit of fatal hypothermia. Unlike MPTP, paraquat did not induce a significant hypothermia. Placement of animals on heating pads significantly abrogates the loss of core temperature. In both heated and non-heated conditions, mice treated with MPTP showed a significant depletion of ATP within 2 hours of administration in both striatum and SN that started to recover 2 hours after MPTP administration was complete. Striatal DA and DOPAC are significantly reduced starting 4–6 hours after MPTP.
Conclusions:
The fatal hypothermic effects of MPTP can be abrogated through use of external heating.
INTRODUCTION
Animal models of Parkinson’s disease (PD) fall into two broad categories. These are: 1) models induced by exogenous administration of substances, i.e. 1-methyl-4-phenyl-1,2,3,6-tetrahydropyridine or paraquat, and 2) models generated through manipulation of the genome. In the former, substances are administered peripherally and through various mechanisms are metabolized until they effect a neurodegenerative action.
In terms of exogenous models, the most widely used compound for induction of dopamine insufficiency to mimic that seen in PD is the systemic administration of the tertiary amine, 1-methyl–4-phenyl-1,2,3,6-tetrahydropyridine (MPTP). This agent was identified following the study of young adult heroin addicts who unknowingly injected the synthetic heroin, 1-methyl-4-phenyl-4-propionoxypiperidine (MPPP) that contained the contaminant, MPTP; after which they presented with symptoms indistinguishable from those of later stage PD patients [1, 2].
Another compound that has been used to induce experimental parkinsonism is 1,1′ di methyl-4,4′-bipyridium dichloride (paraquat, PQ). PQ is a commonly used non-selective herbicide that has been epidemiological linked to Parkinson’s disease [3, 4]. Experimentally, administration of PQ by systemic or direct injection into the CNS induces a relatively specific lesion in the SNpc that results in a small but significant dopaminergic neuron loss [5–9] (although note [10]). The relative small loss of DA neurons over a more protracted period of time appears to allow for plasticity in the system such that despite neuron loss in the SN, there is no apparent loss of dopamine in the striatum [11–13].
Paraquat can cross the blood brain barrier [14], but unlike MPTP, acts as a direct redox cycling agent that reduces molecular oxygen to its cationic radical that then further reduces molecular oxygen to its superoxide radical [15–17]. In addition to its direct effect on membrane phospholipids, PQ also actively transports into neurons via neutral amino acid transporters [18] where is damages mitochondria leading to secondary oxidative stress [19, 20] and eventual cell death through a BAK-dependent mechanism [21].
Since MPTP and PQ are generally administered via the periphery, either through direct injection or dietary means, one must also be aware of peripheral effects that could synergize with the CNS effects. Previous reports have shown that MPTP and/or PQ can induce cardiac [22, 23], lung [24] and kidney [25, 26] toxicity and alterations in muscle function [27]. In addition to these agent’s abilities to damage organ systems, we, and other have observed that peripheral administration of these chemicals appears to induce changes in the animal’s core body temperature [28–30].
Maintenance of body temperature is a combinatorial function of heat generation and heat loss; and small fluctuations in animal core temperature can have significant effects in physiological systems (cardiovascular, endocrine, nervous system) that can culminate in an animal’s death [31]; each of which has been reported with MPTP [32] and paraquat [24].
In this paper, we examine the effect of administering MPTP (acute administration of 4×20 mg/kg spaced two hours apart) and paraquat (2×10 mg/kg/week×3 weeks) on the core temperature in C57BL/6J mice. We find that acute MPTP treatment induces a rapid and lasting hypothermia that can be abrogated by increasing the environmental ambient temperature. The loss of body temperature correlates with a significant loss of ATP and dopamine in the striatum and ATP in the substantia nigra. Paraquat, like MPTP, also induces a transient hypothermia; however, unlike MPTP, animal’s treated with paraquat return to normal temperature soon after administration.
MATERIALS AND METHODS
Ethics statement
All of the experimental procedures in the animals were performed in accordance with the NIH Guide for the Care and Use of Laboratory Animals and all protocols, were approved by the St Jude Children’s Research Hospital IACUC (protocol 364). Experiments were carried out in accordance with The Code of Ethics of the World Medical Association (Declaration of Helsinki) for animal experiments.
Animals
16–20 week old male C57BL/6J mice (Jackson Labs, Bar Harbor, ME) mice were used in this study. Mice were housed 5 to a polycarbonate cage within the vivarium at St. Jude Children’s Research Hospital and were maintained on a 12:12 hour light:dark cycle. The animals were provided with corn cob bedding, ad libitum food (LabDiet 5013, Purina) and water.
Measurement of cage temperature
To determine the temperature of the animal’s environment, iButton ® Temperature Data Loggers (QA Supplies, LLC, Norfolk, VA) were placed in each cage and temperature was determined at 1 hour intervals. Over the course of the experimental procedure, the ambient temperature of the cages in the holding room was 23.2°C (73.8±0.10 °F), never fluctuating more than ±5% . Twenty-four hours prior to administration of MPTP or paraquat, mice were transferred from their holding room to caging maintained within a vented fume hood that had an empirically determined ambient temperature of 21.2 ± 0.1°C (70.2°F), which is within the optimal housing range for mice [33]. One half of the cages in the vented hood were maintained on heating pads set to “medium” which were empirically determined to be 35.1 ± 1.0°C (95.2 ± 2.0°F). When cages were placed on the heating pads the ambient cage temperature was empirically determined to be 24.6±0.3 °C (76.3±0.6 °F).
The other one-half of the mice were housed in cages seated on heating pads that were turned off. Mice were allowed 24 hours of acclimation in this new environment prior to administration of MPTP or paraquat.
MPTP treatment
C57BL/6J mice were administered either vehicle [0.9% sterile saline (Sal)] or MPTP (Catalog# M103, Sigma, St. Louis, MO) using the acute protocol (4×20 mg/kg MPTP-HCl ip injections at 2-h intervals, equivalent to 16.8 mg/kg free base) [34]. One-half of the mice injected with MPTP were maintained on heating pads and one-half of the mice were housed in cages seated on heating pads that were turned off. We have empirically observed that mice become hypokinetic and exhibit piloerection and wet dog shakes following administration of MPTP. Additionally, it has also been well documented that certain strains of mice, including C57BL/6J, can suffer from a non-predictable death (cause unknown, although speculated to be cardiac in nature) within 24 hours of its administration [35]. However, since there is no clear biomarker available that will allow one to determine if any particular mouse will succumb to MPTP toxicity, and almost all mice injected using the acute MPTP paradigm appear (at some time during the 8 hours of the injection period) moribund, an a priori determination of a humane endpoint during the injection protocol for euthanasia could not be made. However, prior to the start of the procedure, the protocol called for humane sacrifice within 24 hours of the final injection.
Paraquat treatment
1,1′ di methyl-4,4′-bipyridium dichloride (paraquat, PQ) (Catalog #36541 Sigma-St. Louis, MO) was dissolved in sterile saline to a final concentration of 20 mg/ml. Each animal was given a total of 60 mg/kg of PQ, using a dosage regimen of 10 mg/kg×2 per week for 3 weeks. One-half of the mice injected with PQ were maintained on heating pads and the other one-half of the mice were housed in cages seated on heating pads that were turned off. Like, MPTP, an a priori determination of a humane endpoint during the injection protocol for euthanasia could not be made. However, prior to the start of the procedure, the protocol called for humane sacrifice within 24 hours of the final injection.
Measurement of core temperature
Seven days prior to treatment with MPTP or paraquat, mice were anesthetized with inhaled isofluorane. Once deep tendon reflexes were absent, a 2 cm2 area of fur was removed from the intrascapular region and the exposed skin was cleaned with alternating iodine and 70% ethanol. The skin was then incised and a 10 g syringe preloaded with a temperature transponder with a programmable Radio Frequency Identification (RFID) signal (IPTT-300, BioMedic Data Systems, Inc., Seaford, DE) was placed subcutaneously and the transponder was expelled. Once the transponder was in place, the needle was removed and the skin was closed using a drop of n-butyl cyanoacrylate glue (Vet Bond ®, Fisher Scientific). Once the glue had set, the mouse was allowed to recover from the isofluorane anesthesia and placed into their home cage.
Core temperatures of the C57BL/6J mice were measured using a hand held reader (DAS-7066/7r, BioMedic Data Systems, Inc., Seaford, DE). For the MPTP study, animals were checked and temperatures were taken at baseline and 15, 30, and 60 and 120 minutes after each MPTP injection with an additional measurement of 180 and 240 minutes following the final (4th) injection (total of 600 minutes). For paraquat, animals were checked and temperatures were taken at baseline, 15, 30, 60, 120 and 24 hours after each injection through out the entire 3-week injection period.
Determination of purine and catecholamine levels in striatum and substantia nigra
At Time 0, 15 minutes after each injection of MPTP and 2 hours following the last injection of MPTP, C57BL/6J mice were rapidly decapitated. Brains were rapidly removed and placed in a brain matrix (Model BS-AL-5000C, Braintree Scientific, Braintree. MA) and sliced into 2 mm thick sections and placed on an ice-cooled plate. Striatum and substantia nigra were dissected using the following coordinates: SN (Bregma: −2.00- −4.00), striatum (Bregma: +0.00-+2.00 mm) [36]. HPLC-ECD was used to quantitate the levels of purines (adenosine (ADO), ATP, ADP and AMP) and catecholamines (dopamine (DA) and its metabolites (HVA and DOPAC), as previous described [37, 38]. Following chromatography, the signal from the electrochemical detector was recorded using a model SS420x integration device (Scientific Software Inc) and the retention time of each of the analytes were empirically determined. For both purines and catecholamines, a 4-point concentration curve (12.5, 25, 50 and 100 ng/ml) of external standards (Sigma) was generated and concentrations in tissues were quantified by comparing the peak areas of the sample chromatograms with the external standard chromatograms.
Statistics
Differences in mean core temperatures, as well as purine and catecholamine concentrations were analyzed by ANOVA using PRIZM software (GraphPad Software). If overall significance was found, individual comparisons were performed using Neumann-Keuls post-hoc comparisons.
RESULTS
Effect of external heat on animal environment
The ambient cage temperature in the procedure room at SJCRH that were not provided access to an external heat source was empirically determined to be 20.1±0.3°C (68.2±0.6 F). The ambient cage temperature in the procedure room at SJCRH that were provided access to an external heat source (35.1±1.0 °C,95.2±2.0°F) was empirically determined to be 24.6±0.3 °C (76.3±0.6 °F).
Effect of MPTP on core temperature
10 male C57BL/6J mice were administered 4 ip injections of MPTP (20 mg/kg) spaced 2 hours apart, while 10 C57BL/6J male C57BL/6J control animals were placed on heating pads and injected ip with 0.1 ml sterile 0.9% saline. Two temperature conditions were examined: those housed on heating pads (heat) and those housed at ambient temperature (no heat). Both groups had similar core temperatures at the start of the experiment (C57BL/6J with heat = 38.5±0.1°C (101.2±0.29 °F); C57BL/6J without heat = 38.3±0.1°C (100.8±0.21 °F), and (Table 1 and Fig. 1).
C57BL/6J mice housed in non-heated conditions (n = 5) that were administered an ip injection of MPTP showed a rapid 4.5% (35.7°C, 96.3°F) drop in core temperature that progressed to a loss of 10% (32.8°C, 90.9°F) at the conclusion of the first 2 hour inter-injection period. Following the second injection-at the 120-minute mark- the core temperature continued to fall, so that at the end of the 2nd inter-injection period, the core temperature of the C57BL/6J mice without parental heat support fell another 7.0% (29.2°C, 84.6°F). After the third injection-at the 360 minute mark- the core temperature continued to fall another 5.0% (26.9°C, 80.4°F), while 4 hours after the 4th injection, the core temperature of these C57BL6J mice fell another 10% (20.4°C, 68.8°F) for a total loss of 31% over the whole injection period. Twenty-four hours following the start of the injection, all of the mice (5 out of 5) that did not have additional access to an external heat source had died, likely due to the fact that they had surpassed the point of Fatal Hypothermic Temperature [31].
A second group of 5 male C57BL/6J mice were housed on heating pads and given the same dosing regimen of MPTP. These mice showed a rapid 2.8% drop in core temperature (36.9°C, 98.4°F) that progressed to a loss of 5.5% (35.3°C, 95.5°F) at the conclusion of the first 2-hour inter-injection period. Following the second injection-at the 120 minute mark- the core temperature continued a gradual fall, so that at the end of the 2nd inter-injection period, the core temperature of the C57BL/6J mice that had parental heat support fell another 10.8% (29.6°C, 85.3°F). After the third injection, the behavior of the core temperatures changed. Instead of continuing to fall, the core temperature of the mice rose by 3% (31.0°C, 87.8 °F), while 4 hours after the 4th injection, the core temperature continued to recovered (32.2°C, 90.0°F) so that the total loss of body temperature was only 17% over the whole injection period and by 6 hours the total loss was only 1.1% . Unlike the mice that did not have access to an external heat source, only 1 out of 5 of these animals died.
To determine the effect of animal handling and ip injections on body temperature with (n = 5) and without (n = 5) supplementary heat, C57BL/6J mice were administered 4 ip injections of 0.9% saline using the same average injection volume as the MPTP-treated mice (0.1 ml/injection). As can be seen in Fig. 1 and Table 1, the core temperature variance of these mice never varied more than 4% and at the end of the period had risen on average only 0.5% . Twenty-four hours after administration of ip saline, none of these male C57BL/6J mice had died.
Effect of paraquat on core temperature
10 male C57BL/6J mice were administered a total of 6 ip injections of paraquat (10 mg/kg) over a period of 3 weeks, with 2 injections/week spaced 2 days apart. A second group of 10 male C57BL/6J control animals were injected ip with 0.1 ml sterile 0.9% saline using the same injection calendar. Two temperature conditions were examined: those housed on heating pads (heat) and those housed at ambient temperature (no heat). Each groups had similar core temperatures at the start of the experiment (C57BL/6J with heat = 99.7±0.3 F (n = 5); C57BL/6J without heat = 100.5±0.2 F (n = 5), C57BL/6J+PQ+No Heat = 100.4 ±/2 (n = 5), and C57BL/6J + PQ + Heat = 99.6±0.3 (n = 5)) (Table 2 and Fig. 2).
Unlike C57BL/6J male mice administered MPTP, the first 4 injections of paraquat (weeks 1 and 2) did not induced hypothermia, but rather there seemed to be a small 1-2°C increase in core temperature. However, as can be seen in Table 2 and Fig. 2, this transient hyperthermia was observed in both mice that received PQ or saline; suggesting that this change was due to stress induced by the injection procedure and not the PQ itself. In the third week of injections, we did note that mice receiving PQ had a reduced core temperature compared to those receiving saline. Interestingly, animals given PQ without external heat support had a higher core body temperature 24 hours after PQ (Despite the lack of a significant change in body core temperature, we did observe that mice that were housed in cages with an external heat source did have lower core temperatures than those housed in cages without an external heat source (Fig. 2, red lines compared to green lines).
Effect of MPTP on purine levels in the striatum and substantia nigra
Given that we observed a significant decrease in core temperature in C57BL.6J mice following MPTP, we examined the concentration of purines, including adenosine (ADO), adenosine triphosphate (ATP), adenosine diphosphate (ADP) and adenosine monophosphate (AMP) in two regions of the brain (striatum and substantia nigra) that are known to be affected by this toxin.
Following MPTP, there was no change in adenosine, ADP or AMP in the striatum in animals with and without external heat (Fig. 3). We did find a significant drop in striatal ATP 15 minutes after the 2nd injection of MPTP (135 minutes after the 1st injection) that lasted until 2 hours after the final injection of MPTP, when ATP levels start to recover. In the SN, we observed a similar pattern of purine effect (no loss of ADO or ADP, significant loss of ATP, Fig. 4).
Examination of acute MPTP effects on dopamine, DOPAC and HVA in striatum revealed a significant loss of DA, 15 minutes after the 2nd injection (135 minutes after MPTP was first administered) in animals that had external heat while animals not exposed to an external heat source first showed significant changes after the 3rd injection (195 minutes after the initial injection of MPTP) (Fig. 5). A similar pattern of change was observed in the measurement of DOPAC, while significant effects on HVA were only noted after the 4th injection of MPTP. (Fig. 5). Although changes in DA were noted as early as 15 minutes after the second injection of MPTP, changes in DA turnover, calculated as (DOPAC+HVA)/DA were only observed in animals provided an external heat source starting 15 minutes after the 4th injection of MPTP (Fig. 5).
DISCUSSION
In this study we examined the effect of two chemicals, MPTP and paraquat, that have been used to induce experimental parkinsonism on core body temperature in C57BL/6J mice. We find that ip injection of MPTP has a rapid and significant hypothermic effect on body temperature that ultimately can prove fatal if no external heat source is supplied to mitigate this change. Unlike MPTP, we do not observe these largehypothermic effects following ip administration of paraquat.
Previous studies that have examined body temperature following one injection of high doses of MPTP have shown this compound induces transient hyperthermia followed by a profound hypothermia in both MPTP-sensitive ddY [39] and MPTP-resistant CD-1 mice, while C57BL/6N mice only showed a hypothermic response [28, 40]. A similar response was seen following administration of MPP+, with the caveat that this also induced hyperthermia in C57BL/6N mice [28, 40]. This suggests changes in body temperature are independent of the central dopaminergic effects of MPTP since MPP+, the neurotoxic metabolite of MPTP cannot cross the blood-brain-barrier [41]. In this study, we examined the core temperature of another strain of mouse (C57BL/6J) widely used in MPTP and other models of Parkinson’s disease [42]. Since the neurotoxic effects of this compound are only seen with repeated administration, we determined body temperature for 10 hours, a period that spans the entire MPTP administration period in our “acute” administration protocol [43]. In this study, we find that repeated injection of MPTP induces a 31% loss of core body temperature. This hypothermia is slightly more profound that other studies that have examined this variable. Satoh et al. [39] used several concentrations of MPTP in ddY mice and showed no more than a 5% loss of core temperature. Freyaldenhoven et al. [28] using single injections of 50 mg/kg MPTP or 12.5 mg/kg MPP+ reported a maximum loss of 28% in body temperature in 6 month C57BL/6N and a 17% loss in CD-1 mice given MPTP. What is interesting is that it appears that MPP+ is the critical mediator of temperature change since mice pretreated with Deprenyl, a MAO-B inhibitor that inhibits conversion of MPTP to MPP+ significantly reduces the hypothermia[28].
Studies examining the hypothermic effects of peripherally administered MPP+suggest the hypothermia is not directly mediated through a central CNS mechanism, despite the finding that labeled MPTP can bind in the hypothalamus [44], a primary site of central temperature regulation [45]. In the periphery, MPTP is converted to MPP+ by several mechanisms including MAO-B [46], mitochondrion-targeted human cytochrome P450 2D6 (CYP2D6) [47] and rat liver cytochromes P-450IIB1 and P-450IA1 [48]. Once converted to MPP+, this radical can be taken into cells preferentially through the dopamine transporter (DAT), although other have suggested alternative methods for cellular uptake including transport through the organic cation transporter, known in mouse as Orct3 (Slc22a1). Once in cells, MPP+ interferes with NADH-ubiquinone oxidoreductase (complex I) of the mitochondrial electron transport chain [50, 51] leading to a reduction in cellular ATP [52], a depletion of catecholaminergic stores [53–55] and increase in cellular adenosine [56]; all of which may contribute to induction of cell death.
To examine if maintenance of body temperature affected purine levels in the brain, both at resting and after MPTP, we used a novel HPLC-ECD detection method that allows simultaneous detection of adenosine, ATP, ADP and AMP from brain tissue [37]. As expected, based on the physiological effects of MPTP on Complex I, we observed a significant drop in ATP starting 135 minutes after the initial administration of MPTP. The timing of this slightly slower than that reported by Chan et al. [57] who reported a significant 20% drop in ATP in the striatum and ventral mesencephalon within 6 minutes, although they did give a dose 2x that of this study. We also find a greater loss of ATP than previously reported (approximately 48% compared to 20%), which could be due to the effects of the multiple adminstrations of MPTP versus that of a single injection; further supported by our observation that ATP levels start to recover within 2 hours of the last injection of MPTP.
Like cellular ATP, we also noted a drop in striatal DA occurring 2–4 hours after the start of MPTP administration. This significant loss occurs at the same time as we observed a significant loss of ATP.
Loss of cellular energy (ATP) can reduce the animal’s ability to maintain its cellular metabolism and maintain temperature homeostasis as well as maintain critical function of organ systems [58]. The maintenance of core body temperature occurs through a balance between heat generation and heat loss. When heat loss is greater than generation, hypothermia occurs. Since generation of body heat requires energy consumption, including induction of shivering and active vasoconstriction, loss of ATP would be expected to allow for a hypothermic response. Given that there appears to be little-to-no expression in skeletal muscle of the dopamine transporter and organic cation transporter 3 [59] or extraneuronal monoamine transporter [60], the effects of peripheral MPTP administration on muscle may be an indirect effects of changes occurring in the CNS. This is supported by studies that have shown that CNS injection of the dopamine antagonist, haloperidol [61], can induce a marked hypothermia.
One major observation we made in this study, and have noted in other experiments in our lab (Smeyne, unpublished observations) was that mice that received MPTP but were not provided with an external heat source did not survive more than 24 hours after the completion of the MPTP injection regimen. As noted earlier, C57BL/6J mice in this study had a ∼31% loss of body temperature that ultimately matched the animal’s cage temperature, and this temperature was slightly greater than the reported Fatal Hypothermic Temperature (FHT) of mice [31]. When access was given to an external heat source, these animals still became hypothermic, but the total body temperature loss was only ∼17% of its initial core temperature and significantly above the FHT. Since only 20% of these mice died, it appears that this 17% loss in body temperature was sufficiently tolerated by the C57BL/6J mice.
In conclusion, we examined the effect of MPTP and paraquat on core body temperature in C57BL/6J mice. Although both of these chemicals have been reported to induce experimental parkinsonism, only MPTP induced a hypothermic response that without external heat support proved fatal. Since MPTP and PQ work via different cellular mechanisms, it is likely that the peripheral hypothermia that is only seen in mice given MPTP is due its blockade of Complex I and subsequent downstream effects, while PQ, which affects cells via a direct lipid redox mechanism [62], does not affect cellular metabolism and thus has little effect core temperature.
CONFLICT OF INTEREST
The authors have no conflict of interest to report.
ACKNOWLEDGMENTS
The authors thank Nicole Lantz and Cara Davis Goodrum for their assistance in surgical placement of the temperature transponders. This work was supported by the American Lebanese Syrian Associated Charities (ALSAC).
REFERENCES
1 | Langston JW, Ballard P, Tetrud JW, Irwin I (1983) Chromic parkinsonism in humans due to a product of merperidine-analog synthesis Science 219: 979 980 |
2 | Kopin IJ (1987) MPTP: An industrial chemical and contaminant of illicit ntics stimulates a new era in research on Parkinson’s disease Environ Health Perspect 75: 45 51 arco |
3 | Tanner CM, Kamel F, Ross GW, Hoppin JA, Goldman SM, Korell M, Marras C, Bhudhikanok GS, Kasten M, Chade AR, Comyns K, Richards MB, Meng C, Priestley B, Fernandez HH, Cambi F, Umbach DM, Blair A, Sandler DP, Langston JW (2011) Rotenone, paraquat, and Parkinson’s disease Environ Health Perspect 119: 866 872 |
4 | Costello S, Cockburn M, Bronstein J, Zhang X, Ritz B (2009) Parkinson’s disease and residential exposure to maneb and paraquat from agricultural applications in the central valley of California Am J Epidemiol 169: 919 926 |
5 | Liou HH, Chen RC, Tsai YF, Chen WP, Chang YC, Tsai MC (1996) Effects of paraquat on the substantia nigra of the wistar rats: Neurochemical, histological, and behavioral studies Toxicol Appl Pharmacol 137: 34 41 |
6 | Peng J, Mao XO, Stevenson FF, Hsu M, Andersen JK (2004) The herbicide paraquat induces dopaminergic nigral apoptosis through sustained activation of the JNK pathway J Biol Chem 279: 32626 32632 Epub 32004 May, 32620 |
7 | Yin L, Lu L, Prasad K, Richfield EK, Unger EL, Xu J, Jones BC (2011) Genetic-based, differential susceptibility to paraquat neurotoxicity in mice Neurotoxicol Teratol 33: 415 421 |
8 | Jiao Y, Lu L, Williams RW, Smeyne RJ (2012) Genetic dissection of strain dependent paraquat-induced neurodegeneration in the substantia nigra pars compacta PLoS One 7: e29447 |
9 | Corasaniti MT, Bagetta G, Rodino P, Gratteri S, Nistico G (1992) Neurotoxic effects induced by intracerebral and systemic injection of paraquat in rats Hum Exp Toxicol 11: 535 539 |
10 | Minnema DJ, Travis KZ, Breckenridge CB, Sturgess NC, Butt M, Wolf JC, Zadory D, Beck MJ, Mathews JM, Tisdel MO, Cook AR, Botham PA, Smith LL (2014) Dietary administration of paraquat for 13 weeks does not result in a loss of dopaminergic neurons in the substantia nigra of C57BL/6J mice Regul Toxicol Pharmacol 68: 250 258 |
11 | Woolley DE, Gietzen DW, Gee SJ, Magdalou J, Hammock BD (1989) Does paraquat (PQ) mimic MPP+ toxicity? Proc West Pharmacol Soc 32: 191 193 |
12 | Breckenridge CB, Sturgess NC, Butt M, Wolf JC, Zadory D, Beck M, Mathews JM, Tisdel MO, Minnema D, Travis KZ, Cook AR, Botham PA, Smith LL (2013) Pharmacokinetic, neurochemical, stereological and neuropathological studies on the potential effects of paraquat in the substantia nigra pars compacta and striatum of male C57BL/6J mice Neurotoxicology 37: 1 14 |
13 | Ethell DW, Fei Q (2009) Parkinson-linked genes and toxins that affect neuronal cell death through the Bcl-2 family Antioxid Redox Signal 11: 529 540 |
14 | Corasaniti MT, Defilippo R, Rodino P, Nappi G, Nistico G (1991) Evidence that paraquat is able to cross the blood-brain barrier to a different extent in rats of various age Funct Neurol 6: 385 391 |
15 | Ramachandiran S, Hansen JM, Jones DP, Richardson JR, Miller GW (2007) Divergent mechanisms of paraquat, MPP+, and rotenone toxicity: Oxidation of thioredoxin and caspase-3 activation Toxicol Sci 95: 163 171 |
16 | Peter B, Wartena M, Kampinga HH, Konings AW (1992) Role of lipid peroxidation and DNA damage in paraquat toxicity and the interaction of paraquat with ionizing radiation Biochem Pharmacol 43: 705 715 |
17 | Yamada K, Fukushima T (1993) Mechanism of cytotoxicity of paraquat. II. Organ specificity of paraquat-stimulated lipid peroxidation in the inner membrane of mitochondria Exp Toxicol Pathol 45: 375 380 |
18 | Shimizu K, Ohtaki K, Matsubara K, Aoyama K, Uezono T, Saito O, Suno M, Ogawa K, Hayase N, Kimura K, Shiono H (2001) Carrier-mediated processes in blood–brain barrier penetration and neural uptake of paraquat Brain Res 906: 135 142 |
19 | Cocheme HM, Murphy MP (2008) Complex I is the major site of mitochondrial superoxide production by paraquat J Biol Chem 283: 1786 1798 |
20 | Fukushima T, Yamada K, Hojo N, Isobe A, Shiwaku K, Yamane Y (1994) Mechanism of cytotoxicity of paraquat. III. The effects of acute paraquat exposure on the electron transport system in rat mitochondria Exp Toxicol Pathol 46: 437 441 |
21 | Fei Q, McCormack AL, Di Monte DA, Ethell DW (2008) Paraquat neurotoxicity is mediated by a Bak-dependent mechanism J Biol Chem 283: 3357 3364 |
22 | Takatsu H, Nishida H, Matsuo H, Watanabe S, Nagashima K, Wada H, Noda T, Nishigaki K, Fujiwara H (2000) Cardiac sympathetic denervation from the early stage of Parkinson’s disease: Clinical and experimental studies with radiolabeled MIBG J Nucl Med 41: 71 77 |
23 | Wilson JS, Shearer DT, Adelakun AK, Carpentier RG (1991) Mechanisms of the inotropic actions of MPTP and MPP+ on isolated atria of rat Toxicol Appl Pharmacol 111: 49 57 |
24 | Dinis-Oliveira RJ, Duarte JA, Sanchez-Navarro A, Remiao F, Bastos ML, Carvalho F (2008) Paraquat poisonings: Mechanisms of lung toxicity, clinical features, and treatment Crit Rev Toxicol 38: 13 71 |
25 | Purser DA, Rose MS (1979) The toxicity and renal handling of paraquat in cynomolgus monkeys Toxicology 15: 31 41 |
26 | Webb DB (1983) Nephrotoxicity of paraquat in the sheep and the associated reduction in paraquat secretion Toxicol Appl Pharmacol 68: 282 289 |
27 | Tabata N, Morita M, Mimasaka S, Funayama M, Hagiwara T, Abe M (1999) Paraquat myopathy: Report on two suicide cases Forensic Sci Int 100: 117 126 |
28 | Freyaldenhoven TE, Ali SF, Hart RW (1995) MPTP- and MPP(+)-induced effects on body temperature exhibit age- and strain-dependence in mice Brain Res 688: 161 170 |
29 | Satoh N, Yonezawa A, Tadano T, Kisara K, Arai Y, Kinemuchi H (1987) Central hypothermic effects of some analogues of 1-methyl-4-phenyl-1,2,3,6-tetrahydropyridine (MPTP) and 1-methyl-4-phenylpyridinium ion (MPP+) Neurosci Lett 80: 100 105 |
30 | Cagen SZ, Janoff AS, Bus JS, Gibson JE (1976) Effect of paraquat (methyl viologen) on liver function in mice J Pharmacol Exp Ther 198: 222 228 |
31 | Vlach KD, Boles JW, Stiles BG (2000) Telemetric evaluation of body temperature and physical activity as predictors of mortality in a murine model of staphylococcal enterotoxic shock Comp Med 50: 160 166 |
32 | Przedborski S, Jackson-Lewis V, Naini AB, Jakowec M, Petzinger G, Miller R, Akram M (2001) The parkinsonian toxin 1-methyl-4-phenyl-1,2,3,6-tetrahydropyridine (MPTP): A technical review of its utility and safety J Neurochem 76: 1265 1274 |
33 | Speakman JR, Keijer J (2012) Not so hot: Optimal housing temperatures for mice to mimic the thermal environment of humans Mol Metab 2: 5 9 |
34 | Smeyne M, Goloubeva O, Smeyne RJ (2001) Strain–dependent susceptibility to MPTP and MPP+-induced Parkinsonism is determined by glia Glia 74: 73 80 |
35 | Jackson-Lewis V, Przedborski S (2007) Protocol for the MPTP mouse model of Parkinson’s disease Nat Protoc 2: 141 151 |
36 | Paxinos G, Franklin KBJ (2001) The Mouse Brain in Stereotaxic Coordinates Academic Press San Diego |
37 | Pani AK, Jiao Y, Sample KJ, Smeyne RJ (2014) Neurochemical measurement of adenosine in discrete brain regions of five strains of inbred mice PLoS One 9: e92422 |
38 | Gerecke KM, Jiao Y, Pani A, Pagala V, Smeyne RJ (2010) Exercise protects against MPTP-induced neurotoxicity in mice Brain Res 1341: 72 83 |
39 | Satoh N, Yonezawa A, Tadano T, Kisara K, Arai Y, Kinemuchi H (1987) Acute effects of a parkinsonism-inducing neurotoxin, 1-methyl-4-phenyl-1,2,3,6-tetrahydropyridine (MPTP) on mouse body temperature Life Sci 41: 1415 1424 |
40 | Freyaldenhoven TE, Ali SF (1997) Role of heat shock proteins in MPTP-induced neurotoxicity Annals of the New York Academy of Sciences 825: 167 178 |
41 | Riachi NJ, LaManna JC, Harik SI (1989) Entry of 1-methyl-4-phenyl-1,2,3,6-tetrahydropyridine into the rat brain J Pharmacol Exp Ther 249: 744 748 |
42 | Hamre K, Tharp R, Poon K, Xiong X, Smeyne; RJ (1999) Differential strain susceptibility following 1-methyl-4-phenyl-1,2,3,6-tetrahydropyridine (MPTP) administration acts in an autosomal dominant fashion: Quantitative analysis in seven strains of Mus musculus Brain Res 828: 91 103 |
43 | Smeyne M, Boyd J, Raviie Shepherd K, Jiao Y, Pond BB, Hatler M, Wolf R, Henderson C, Smeyne RJ (2007) GSTpi expression mediates dopaminergic neuron sensitivity in experimental parkinsonism Proc Natl Acad Sci U S A 104: 1977 1982 |
44 | Sandyk R, Iacono RP, Kay SR (1990) The hypothalamus in MPTP-induced parkinsonism Ital J Neurol Sci 11: 367 372 |
45 | Hissa R (1990) Central control of body temperature A review. Arctic Med Res 49: 3 15 |
46 | Ransom BR, Kunis DM, Irwin I, Langston JW (1987) Astrocytes convert the parkinsonism inducing neurotoxin, MPTP, to its active metabolite, MPP+ Neurosci Lett 75: 323 328 |
47 | Bajpai P, Sangar MC, Singh S, Tang W, Bansal S, Chowdhury G, Cheng Q, Fang JK, Martin MV, Guengerich FP, Avadhani NG (2013) Metabolism of 1-methyl-4-phenyl-1,2,3,6-tetrahydropyridine by mitochondrion-targeted cytochrome P450 2D6: Implications in Parkinson disease J Biol Chem 288: 4436 4451 |
48 | Ottoboni S, Carlson TJ, Trager WF, Castagnoli K, Castagnoli N Jr (1990) Studies on the cytochrome P-450 catalyzed ring alpha-carbon oxidation of the nigrostriatal toxin 1-methyl-4-phenyl-1,2,3,6-tetrahydropyridine (MPTP) Chem Res Toxicol 3: 423 427 |
49 | Zwart R, Verhaagh S, Buitelaar M, Popp-Snijders C, Barlow DP (2001) Impaired activity of the extraneuronal monoamine transporter system known as uptake-2 in Orct3/Slc22a3-deficient mice Mol Cell Biol 21: 4188 4196 |
50 | Suzuki K, Mizuno Y, Yoshida M (1990) Effects of 1-methyl-4-phenyl-1,2,3,6-tetrahydropyridine (MPTP)-like compounds on mitochondrial respiration Adv Neurol 53: 215 218 |
51 | Nicklas WJ, Vyas I, Heikkila RE (1985) Inhibition of NADH-linked oxidation in brain mitochondria by 1-methyl-4-phenyl-pyridine, a metabolite of the neurotoxin, 1-methyl-4-phenyl-1,2,5,6-tetrahydropyridine Life Sci 36: 2503 2508 |
52 | Kutty RK, Santostasi G, Horng J, Krishna G (1991) MPTP-induced ATP depletion and cell death in neuroblastoma X glioma hybrid NG 108-15 cells: Protection by glucose and sensitization by tetraphenylborate Toxicol Appl Pharmacol 107: 377 388 |
53 | Fuller RW, Hemrick-Luecke SK (1986) Depletion of norepinephrine in mouse heart by 1-methyl-4-phenyl-1,2,3,6-tetrahydropyridine (MPTP) mimicked by 1-methyl-4-phenylpyridinium (MPP+) and not blocked by deprenyl Life Sci 39: 1645 1650 |
54 | Fuller RW, Hemrick-Luecke SK, Kindt MV, Heikkila RE (1988) Different effects of monoamine oxidase inhibition on MPTP depletion of heart and brain catecholamines in mice Life Sci 42: 263 271 |
55 | Przedborski S, Vila M (2003) The 1-methyl-4-phenyl-1,2,3,6-tetrahydropyridine mouse model: A tool to explore the pathogenesis of Parkinson’s disease Ann N Y Acad Sci 991: 189 198 |
56 | Fredholm BB, Johansson S, Wang YQ (2011) Adenosine and the regulation of metabolism and body temperature Adv Pharmacol 61: 77 94 |
57 | Chan P, DeLanney LE, Irwin I, Langston JW, Di Monte D (1991) Rapid ATP loss caused by 1-methyl-4-phenyl-1,2,3,6-tetrahydropyridine in mouse brain J Neurochem 57: 348 351 |
58 | Boutilier RG (2001) Mechanisms of cell survival in hypoxia and hypothermia J Exp Biol 204: 3171 3181 |
59 | Duan H, Wang J (2010) Selective transport of monoamine neurotransmitters by human plasma membrane monoamine transporter and organic cation transporter 3 J Pharmacol Exp Ther 335: 743 753 |
60 | Haag C, Berkels R, Grundemann D, Lazar A, Taubert D, Schomig E (2004) The localisation of the extraneuronal monoamine transporter (EMT) in rat brain J Neurochem 88: 291 297 |
61 | Vybiral S, Jansky L (1989) The role of dopaminergic pathways in thermoregulation in the rabbit Neuropharmacology 28: 15 20 |
62 | Bonneh-Barkay D, Reaney SH, Langston WJ, Di Monte DA (2005) Redox cycling of the herbicide paraquat in microglial cultures Mol Brain Res 134: 52 56 |
Figures and Tables
Fig.1
Effect of MPTP on Core Temperature in C57BL/6J mice. In C57BL/6J mice administered acute MPTP-HCl (4×20 mg/kg, each injection spaced 2 hours apart) without heat support (black lines), there is a rapid hypothermic effect that is progressive throughout the entire measurement period of 600 minutes. When heat support is provided, MPTP is still hypothermic, although the effect is significantly modified (blue lines). Administration of saline with supplemental heat (red) or without supplemental heat (green) has no effect on the core temperature of C57BL/6J mice.
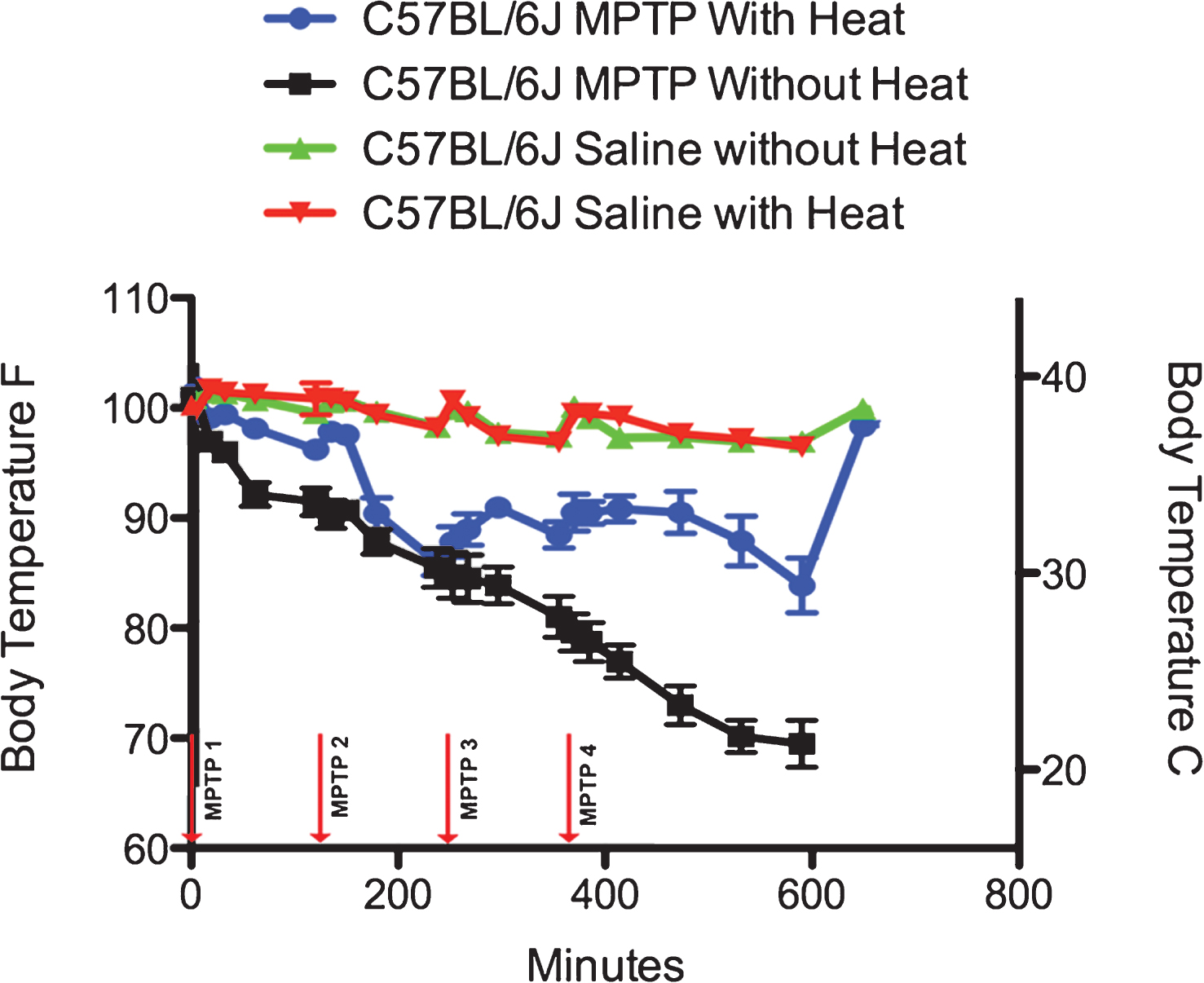
Fig.2
Effect of Paraquat on Core Temperature in C57BL/6J mice. Paraquat (2 injections of 10 mg/kg PQ/week spaced 48 hours apart for 3 weeks) administered to C57BL/6J mice has no significant effect on the core temperature of mice throughout the entire injection period. One does note, however, a slight hyperthermia that is observed 15 minutes after administration of paraquat (increase of 3%) starting after the 2nd paraquat injection that recedes within 30 minutes of administration.
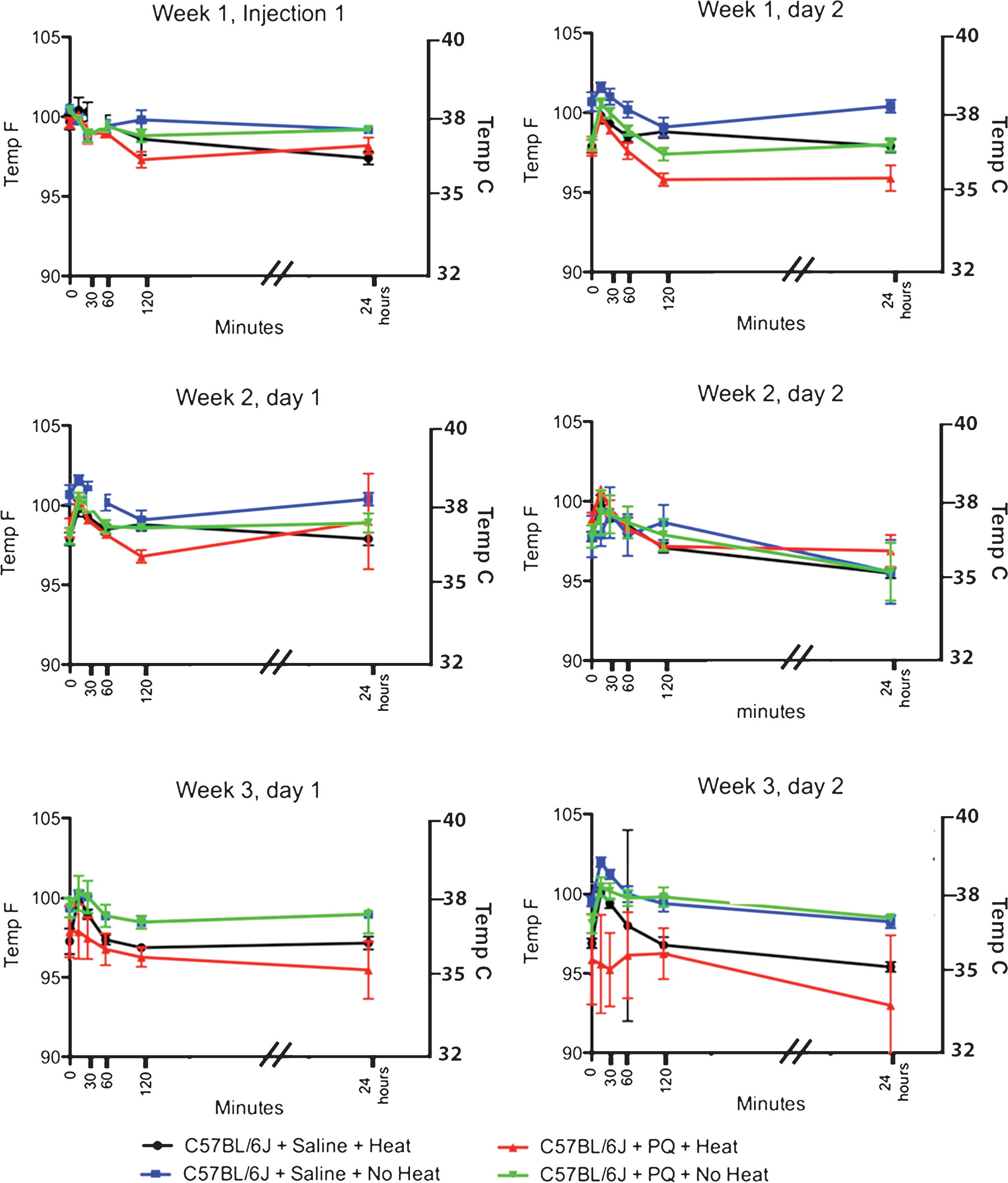
Fig.3
Effect of MPTP on purine levels in the striatum. Intraperitoneal administration of 4 injections of 20 mg/kg MPTP spaced 2 hours apart does not alter adenosine, ADP or AMP levels. Starting 15 minutes after the 2nd injection of MPTP (135 minutes after the first injection), there is a significant loss of ATP that is maintained until 2 hrs after the last injection, when this purine begins to recover to baseline levels. The MPTP-induced changes in ATP are not affected by the addition of an external heat source. * p≤0.05 compared to no heat controls; $ p≤0.05 compared to heat controls.
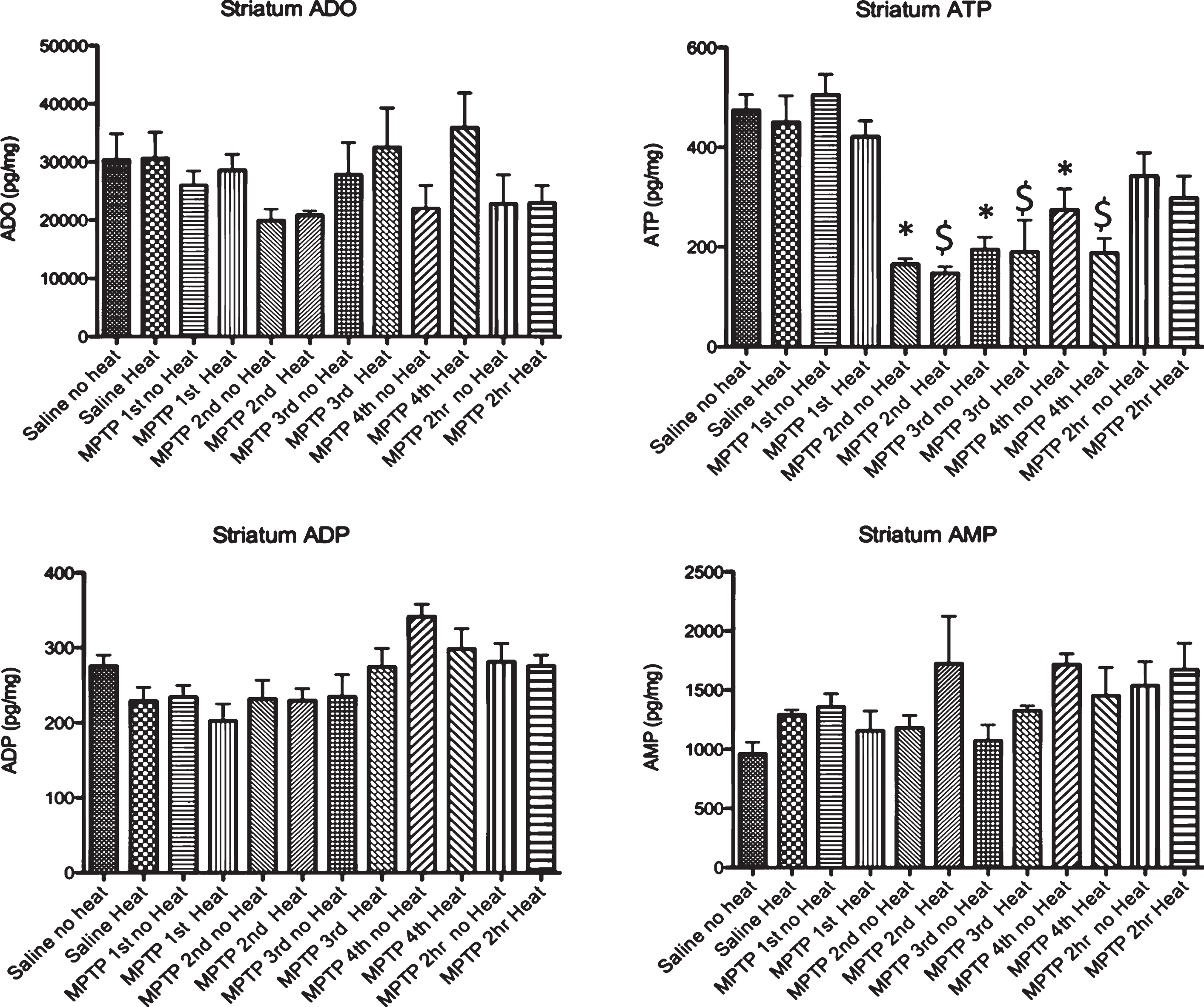
Fig.4
Effect of MPTP on purine levels in the substantia nigra. Intraperitoneal administration of 4 injections of 20 mg/kg MPTP spaced 2 hours apart does not alter adenosine, ADP or AMP levels. Starting 15 minutes after the 2nd injection of MPTP (135 minutes after the first injection), there is a significant loss of ATP that is maintained until 2 hrs after the last injection, when this purine begins to recover to baseline levels. The MPTP-induced changes in ATP are not affected by the addition of an external heat source. * p≤0.05 compared to no heat controls; $ p≤0.05 compared to heat controls.
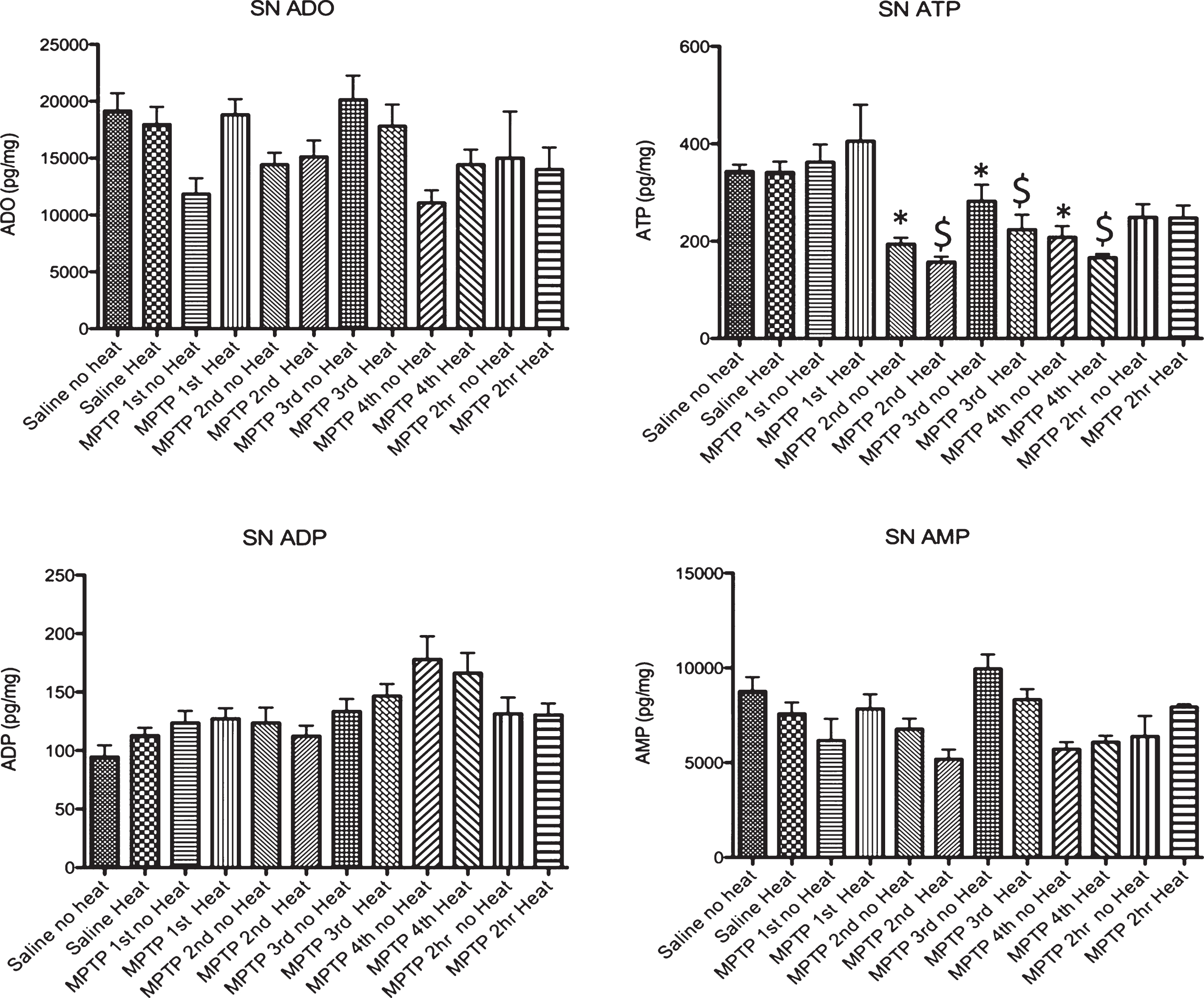
Fig.5
Effect of MPTP on dopamine DOPAC and HVA levels in striatum.. Intraperitoneal administration of 4 injections of 20 mg/kg MPTP spaced 2 hours apart induces a significant loss of dopamine 15 minutes after the 2nd injection of 20 mg/kg MPTP (135 minutes after the first injection) that is progressive after each subsequent injection. There is a reduction of DOPAC that is seen 15 minutes after the third injection (195 minutes after the first injection). No change in HVA is seen in animals not provided an external heat source, although a small but significant change is seen in animals in heated cages starting 15 minutes after the 4th injection of MPTP (495 minutes after the first injection). 15 minutes after the 4th injection of MPTP, animals in heated cages show an increase in dopamine turnover. * p≤0.05 compared to no heat controls; $ p≤0.05 compared to heat controls; # * p≤0.05 non heated cages compared to heated cages.
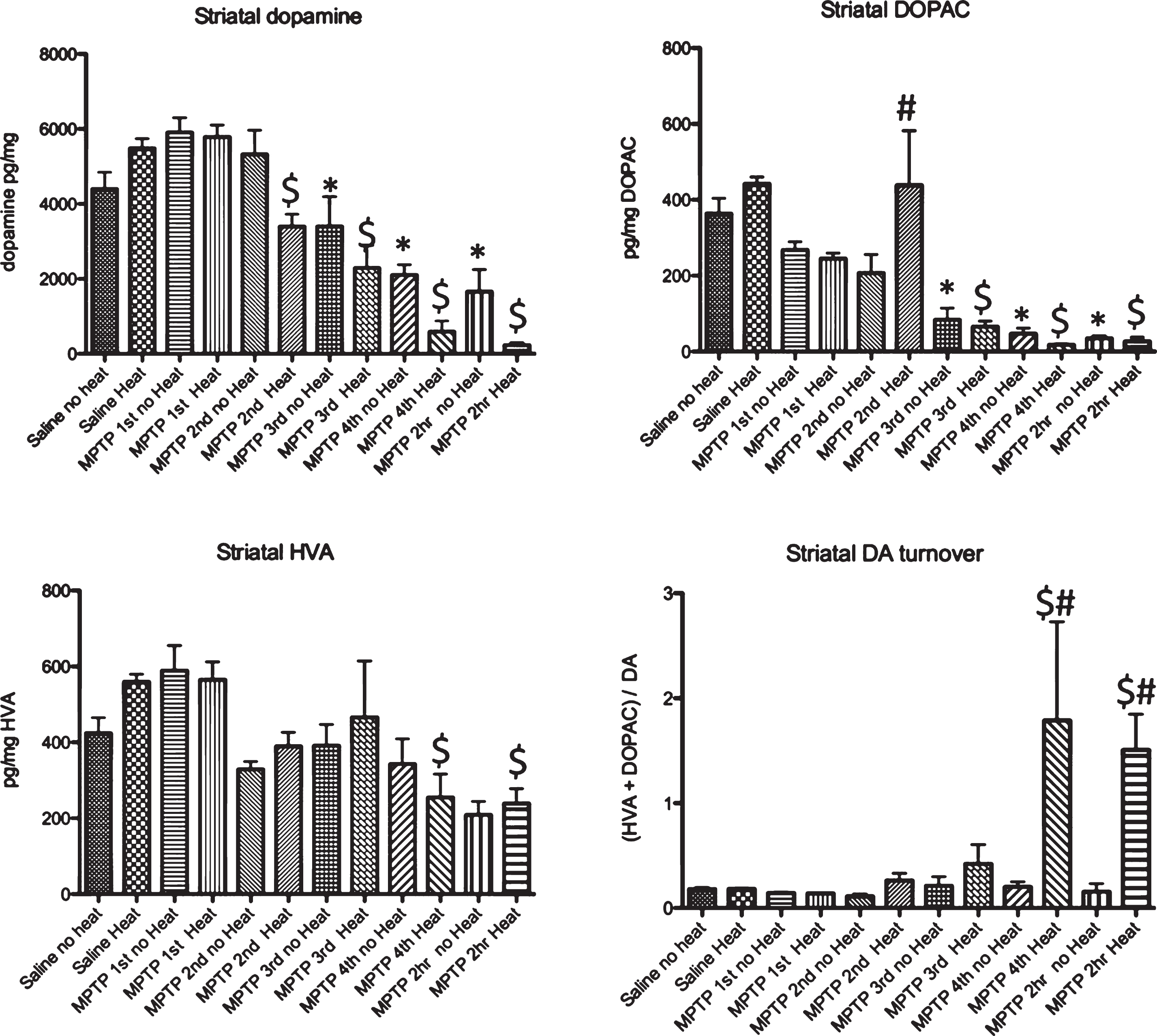
Table 1
Effect of Heat Supplementation on Core Temperature (°C) following MPTP in the Male C57BL/6J Mouse
Injection time | Cumulative minutes | C57BL/6J+ MPTP+No Heat | C57BL/6J+ MPTP+Heat | C57BL/6J+ saline+No Heat | C57BL/6J+ saline+Heat | Statistics |
Baseline (0 minutes) | 0 | 38.3 ±0.1 | 38.5±0.2 | 37.9±0.2 | 37.2±0.2 | NS |
Injection 1–15 minutes | 15 | 35.7±0.3 | 36.9±0.2 | 38.2±0.2 | 38.3±0.2 | 1, 2, 3,4, 5 |
Injection 1–30 minutes | 30 | 35.2±0.3 | 37.1±0.2 | 38.2±0.1 | 38.2±0.1 | 1,2,3 |
Injection 1–60 minutes | 60 | 33.1±0.6 | 36.4±0.3 | 37.8±0.2 | 38.1±0.3 | 1,2,3 |
Injection 1–120 minutes | 120 | 32.7±0.7 | 35.3±0.5 | 37.2±0.1 | 37.9±0.3 | 1,2,3 |
Injection 2–15 minutes | 135 | 32.1±0.7 | 36.2±0.4 | 37.7±0.2 | 37.7±0.4 | 1,2,3 |
Injection 2–30 minutes | 150 | 32.3±0.5 | 36.1±0.3 | 37.8±0.2 | 37.1±0.3 | 1,2,3 |
Injection 2–60 minutes | 180 | 30.7±0.6 | 32.1±0.8 | 37.2±0.2 | 36.4±0.3 | 2,3,4,5 |
Injection 2–120 minutes | 240 | 29.4±1.0 | 29.6±0.6 | 36.5±0.2 | 37.8±0.2 | 2,3,4,5 |
Injection 3–15 minutes | 255 | 29.0±1.2 | 30.7±0.8 | 37.4±0.2 | 37.0±0.2 | 2,3,4,5 |
Injection 3–30 minutes | 270 | 28.8±1.2 | 31.3±0.8 | 37.3±0.1 | 36.0±0.5 | 1,2,4,5 |
Injection 3–60 minutes | 300 | 28.5±0.9 | 32.4±0.5 | 36.2±0.3 | 35.7±0.3 | 1,2,3,4,5 |
Injection 3–120 minutes | 360 | 26.9±1.0 | 31.0±0.7 | 36.0±0.2 | 37.1±0.2 | 1,2,3,4,5 |
Injection 4–15 minutes | 375 | 26.1±0.9 | 32.2±0.9 | 37.5±0.2 | 37.1±0.3 | 1,2,3,4,5 |
Injection 4–30 minutes | 390 | 25.6±1.0 | 32.1±0.6 | 36.9±0.1 | 37.0±0.3 | 1,2,3,4,5 |
Injection 4–60 minutes | 420 | 24.6±0.8 | 32.3±0.6 | 35.9±0.1 | 36.1±0.1 | 1,2,3,4,5 |
Injection 4–120 minutes | 480 | 22.4±1.0 | 32.2±1.0 | 35.9±0.1 | 35.8±0.2 | 1,2,3 |
Injection 4–540 minutes | 540 | 21.1±0.8 | 30.7±1.3 | 35.7±0.4 | 35.5±0.0 | 1,2,3 |
Injection 4–600 minutes | 600 | 20.5±1.0 | 38.5±1.4 | 35.7±0.1 | 37.5±0.3 | 2,3,4,5 |
1: p≤0.05, C57BL/6J MPTP+no heat vs C57BL/6J MPTP+Heat. 2: p≤0.05, C57BL/6J MPTP+no heat vs C57BL/6J saline+no heat. 3: p≤0.05, C57BL/6J+MPTP+no heat vs C57BL/6J Saline+Heat. 4: p≤0.05, C57BL/6J MPTP Heat vs C57BL/6J saline+no heat. 5: p≤0.05, C57BL/6J MPTP Heat vs C57 Saline Heat. 6: p≤0.05, C57BL/6J saline+no heat vs C57BL/6J Saline+Heat.
Table 2
Effect of Heat Supplementation on Core Temperature (°C) following Paraquat in the C57BL/6J Mouse
C57BL/6J+ PQ+No Heat | C57BL/6J+ PQ+Heat | C57BL/6J+ saline+Heat | C57BL/6J+ saline+No Heat | Statistics | |
Baseline (injection 1) | 38.0±0.1 | 37.4±0.2 | 37.6±0.3 | 38.5±0.3 | NS |
15 minutes | 37.7±0.2 | 37.9±0.1 | 38.0±0.4 | 38.7±0.3 | NS |
30 minutes | 37.1±0.2 | 37.1±0.1 | 37.9±0.3 | 38.3±0.2 | 3,5 |
60 minutes | 37.4±03 | 37.4±0.1 | 37.5±0.3 | 37.5±0.3 | NS |
120 minutes | 37.1±0.4 | 36.4±0.3 | 37.0±0.6 | 37.8±0.3 | NS |
24 hours | 37.3±0.1 | 37.1±0.2 | 36.3±0.4 | 38.3±0.2 | 2,3,5,6 |
Baseline (injection 2) | 36.7±0.3 | 36.7±0.4 | 36.6±0.2 | 38.6±0.3 | 3,6 |
15 minutes | 38.1±0.2 | 37.8±0.1 | 37.7±0.3 | 38.7±0.3 | NS |
30 minutes | 37.8±0.2 | 37.3±0.1 | 37.4±0.1 | 38.4±0.3 | 3,6 |
60 minutes | 37.2±02 | 36.6±0.1 | 36.9±0.2 | 37.9±0.4 | 5 |
120 minutes | 36.3±0.2 | 35.6±0.2 | 37.1±0.2 | 37.8±0.3 | 3,4,5 |
24 hours | 36.7±0.2 | 35.9±0.2 | 36.6±0.3 | 38.0±0.2 | 3,5,6 |
Baseline (injection 3) | 36.7±0.3 | 37.1±0.4 | 36.3±0.4 | 36.8±0.4 | NS |
15 minutes | 37.9±0.3 | 38.2±0.1 | 38.4±0.2 | 38.6±0.2 | NS |
30 minutes | 37.8±0.3 | 37.3±0.1 | 38.2±0.1 | 38.5±0.3 | 5 |
60 minutes | 37.1±0.3 | 36.8±0.1 | 36.8±0.1 | 37.7±0.3 | NS |
120 minutes | 37.0±0.2 | 36.0±0.1 | 36.0±0.1 | 37.3±0.2 | 1,2,5,6 |
24 hours | 36.1±0.8 | 37.2±0.2 | 37.0±0.2 | 37.9±0.1 | NS |
Baseline (injection 4) | 37.0±0.2 | 37.1±0.2 | 36.6±0.2 | 37.6±0.3 | NS |
15 minutes | 38.2±0.4 | 38.2±0.1 | 37.8±0.2 | 38.8±0.2 | 6 |
30 minutes | 38.0±0.2 | 37.5±0.3 | 37.2±0.1 | 38.4±0.2 | 5,6 |
60 minutes | 37.6±0.2 | 36.8±0.1 | 36.9±0.3 | 37.4±0.2 | NS |
120 minutes | 37.0±0.6 | 36.2±0.4 | 36.1±0.2 | 37.2±0.2 | NS |
24 hours | 36.4±0.2 | 36.0±0.5 | 35.3±0.2 | 37.1±0.3 | 6 |
Baseline (injection 5) | 37.5±0.3 | 36.6±0.9 | 36.3±0.4 | 37.3±0.4 | NS |
15 minutes | 37.9±0.6 | 36.6±0.9 | 37.8±0.2 | 38.8±0.2 | NS |
30 minutes | 37.9±0.6 | 36.4±0.7 | 37.2±0.1 | 38.1±0.2 | NS |
60 minutes | 37.2±0.4 | 36.0±0.6 | 36.3±0.2 | 37.5±0.2 | NS |
120 minutes | 36.9±0.2 | 35.7±0.3 | 36.1±0.2 | 36.6±0.2 | 1 |
24 hours | 37.2±0.6 | 35.3±1.0 | 35.0±0.1 | 36.8±0.2 | NS |
Baseline (injection 6) | 36.8±0.4 | 35.5±1.6 | 36.1±0.1 | 37.5±0.2 | |
15 minutes | 38.0±0.4 | 35.3±1.8 | 37.8±0.1 | 38.9±0.2 | |
30 minutes | 37.9±0.3 | 35.2±1.3 | 37.5±0.3 | 38.5±0.2 | |
60 minutes | 37.6±0.3 | 35.7±1.5 | 36.7±0.3 | 37.5±0.3 | |
120 minutes | 37.7±0.4 | 35.7±0.9 | 36.0±0.3 | 37.5±0.3 | |
24 hours | 37.0±00 | 33.9±2.4 | 35.3±0.2 | 36.8±0.2 |
1: p≤0.05, C57BL/6J PQ+no heat vs C57BL/6J PQ+Heat. 2: p≤0.05, C57BL/6J PQ+no heat vs C57BL/6J saline+no heat. 3: p≤0.05, C57BL/6J+PQ+no heat vs C57BL/6J Saline+Heat. 4: p≤0.05, C57BL/6J PQ Heat vs C57BL/6J saline+no heat. 5: p≤0.05, C57BL/6J PQ Heat vs C57 Saline Heat. 6: p≤0.05, C57BL/6J saline+no heat vs C57BL/6J Saline+Heat.