Load-Dependent Interference of Deep Brain Stimulation of the Subthalamic Nucleus with Switching from Automatic to Controlled Processing During Random Number Generation in Parkinson’s Disease
Abstract
Background: Deep brain stimulation of the subthalamic nucleus (STN DBS) ameliorates the motor symptoms of Parkinson’s disease (PD). However, some aspects of executive control are impaired with STN DBS.
Objective: We tested the prediction that (i) STN DBS interferes with switching from automatic to controlled processing during fast-paced random number generation (RNG) (ii) STN DBS-induced cognitive control changes are load-dependent.
Methods: Fifteen PD patients with bilateral STN DBS performed paced-RNG, under three levels of cognitive load synchronised with a pacing stimulus presented at 1, 0.5 and 0.33 Hz (faster rates require greater cognitive control), with DBS on or off. Measures of output randomness were calculated. Countscore 1 (CS1) indicates habitual counting in steps of one (CS1). Countscore 2 (CS2) indicates a more controlled strategy of counting in twos.
Results: The fastest rate was associated with an increased CS1 score with STN DBS on compared to off. At the slowest rate, patients had higher CS2 scores with DBS off than on, such that the differences between CS1 and CS2 scores disappeared.
Conclusions: We provide evidence for a load-dependent effect of STN DBS on paced RNG in PD. Patients could switch to more controlled RNG strategies during conditions of low cognitive load at slower rates only when the STN stimulators were off, but when STN stimulation was on, they engaged in more automatic habitual counting under increased cognitive load. These findings are consistent with the proposal that the STN implements a switch signal from the medial frontal cortex which enables a shift from automatic to controlled processing.
INTRODUCTION
Levodopa therapy is effective at controlling the motor symptoms of Parkinson’s disease but after about five years most patients experience debilitating side effects including dyskinesias, on-off fluctuations and end of dose deterioration. The complications associated with long-term levodopa therapy have led to the use of surgical interventions such as deep brain stimulation of the subthalamic nucleus (STN DBS). STN DBS has been demonstrated as clinically effective in improving the motor symptoms of PD as well as some levodopa-induced side effects [1, 2]. Other than a deficit in verbal fluency, STN DBS does not appear to produce any major adverse effects on general cognitive ability in PD [3–5]. However, when using DBS on versus off methodology, several studies have documented specific deficits in cognitive control in PD patients induced by STN stimulation. PD patients have shown deficits in cognitive control on a diverse range of tasks requiring suppression of pre-potent or habitual responses. These tasks include the Stroop [6, 7], Go/No-Go Reaction Time tasks with high target frequency and enhanced pre-potency of responses [8–10], the stop signal reaction time task [11, 12], fast-paced random number generation [13], the Simon task [14] and during probabilistic decision-making under conflict [15–17]. Furthermore, these STN DBS-induced deficits in performance of phonemic verbal fluency, the Stroop interference and fast-paced RNG tasks have been shown to be associated with significant alteration of activation in key frontal and subcortical areas [13, 18, 19]. Therefore, STN DBS can result in an impaired ability to inhibit automatic, pre-potent responses which is associated with altered activation in specific fronto-striatal regions.
One executive process that is critical to adaptive behaviour is the ability to switch between automatic/habitual and controlled/goal-directed processing [20] in a timely and efficient manner. Typically, automatic habitual processing would be employed when executing a well-learned behaviour that requires little attention. However, when engaging in a behaviour that is attentionally-demanding, such as learning a new behaviour or deliberately trying to override a well-learned habitual behaviour, goal-directed controlled processing would be used. From recordings of neuronal activity in primates, it has been proposed that the STN implements a switch signal from the pre-SMA which enables such a shift from automatic to controlled processing [21]. Consequently, high frequency stimulation of the STN during STN DBS in PD may interfere with the ability to switch between automatic and controlled processing when a situation/task demands it.
Random number generation (RNG) is a useful paradigm for investigating the ability to switch between automatic and controlled processing. RNG is an attention-demanding task that involves a number of executive processes. Participants are asked to imagine drawing a number (1–9 inclusive) at random from a hat, say it out loud, place the number back in the hat and then draw another one. In order to achieve a random sequence of numbers, the participant must suppress the habitual tendency to count in series and employ a controlled response selection strategy instead. When the habitual counting strategy is in use, healthy participants tend to count up or down in ones (CS1). This is somewhat replaced with a tendency to count in twos (CS2) when attempting to implement a controlled strategy. Researchers have examined the effect of transcranial magnetic stimulation (TMS) over the left and right dorsolateral prefrontal cortex (DLPFC) and the medial pre-frontal cortex during RNG in healthy volunteers [22]. Repetitive TMS delivered over the left DLPFC led to an increased CS1 score and a decreased CS2 score, thus increasing the automatic habitual counting bias and interfering with controlled processing. From this the ‘network modulation model of RNG’ was developed. This model proposes that suppression of a habitual counting response during RNG is achieved via a modulatory and inhibitory influence of the left DLPFC over the left superior temporal cortex (STC). This prevents spreading activation in an ordered number associative network located in the STC. Numbers in the STC network are ordered in series due to a lifetime of exposure to this pattern of counting. PD patients who show under-activation of the DLPFC during goal-directed willed actions [23], also have impaired performance on RNG tasks marked by higher CS1 scores relative to healthy controls. This bias became differentially greater at faster rates of RNG and therefore increased cognitive load [24, 25].
The aim of the present study was to investigate the effect of STN DBS on paced RNG in PD to specifically test two hypotheses generated from previous research. First, because STN DBS reduced frontal activation and altered pallidal-frontal and pallidal-temporal coupling during fast-paced RNG [13], PD patients should perform RNG even more poorly with stimulation on compared to stimulation off. This will be reflected by increased habitual counting and reduced controlled processing i.e. increased CS1 and decreased CS2. Thus, STN stimulation should reduce the patients’ ability to switch from automatic to controlled processing during RNG resulting in a less random performance with stimulation on as compared to off. Second, owing to the attention-demanding and limited capacity nature of controlled processing, the presence or absence of cognitive control deficits with STN DBS on should be load-dependent, as suggested by the results of previous studies [8, 26]. This prediction was tested by manipulating cognitive load during paced RNG, through increasing the rate of the RNG pacing stimulus; as previously shown, a faster rate increases cognitive load [25, 27–29]. If the impact of STN DBS on cognitive control is load-dependent, then STN stimulation would be predicted to specifically increase non-randomness of the output only at the fastest rate of paced RNG.
METHODS
Sample: Fifteen (7 male, 12 right-handed) individuals with a diagnosis of idiopathic PD aged between 44 and 66 (M = 57.1, SD = 6.4) participated. All patients had undergone surgery for bilateral DBS of the STN, and were recruited from the Functional Neurosurgery Unit, UCL Institute of Neurology and the National Hospital for Neurology and Neurosurgery. All patients suffered from akinetic-rigid symptoms and were treated by bilateral DBS of the STN for at least 3 months (M = 16.00 months, range 3 to 60 months). Prior to surgery, all patients met Parkinson’s Disease Society Brain Bank diagnostic criteria for PD [30], and were screened for absence of dementia and major psychiatric illness. Disease duration ranged from 8 to 21 years (M = 14.9, SD = 14.2). Stage of illness and severity of the motor symptoms of PD was rated by a neurologist while patients were off their usual medication and when they had their DBS switched both on and off, using the Hoehn & Yahr [31] scale and the motor section of the Unified Parkinson’s Disease Rating Scale [32]. All patients were in the moderate to severe stages of the disease, with scores on the H&Y scale of 2.5 to 4 (M = 3.2, SD = 0.5) and UPDRS motor scores ranging between 24 and 84 (M = 49.2, SD = 15.5) with their stimulators switched off. All patients were non-demented as demonstrated by scores >26 on the Mini-Mental State Examination (MMSE) [33]. Patients were also screened for clinical depression on the Beck Depression Inventory (BDI) [34] and none were clinically depressed. Patients were tested on their usual medication. The majority (10/11) were treated with levodopa (Sinemet, Madopar) and the mean levodopa equivalent daily dose was 821.4 milligrams (S.D. = 337.3). In addition, 7 patients were receiving dopaminergic and or anticholinergic medication including entacapone (n = 1), cabergoline (n = 6), pergolide (n = 1), ropinirole (n = 1). Post-surgical MRI confirmed correct positioning of at least one of the contacts of the implanted electrodes in or near the STN target in all patients. Detailed information about the sample and the DBS parameters is presented in Table 1.
The study was approved by the Joint Ethics Committee of the Institute of Neurology and The National Hospital for Neurology and Neurosurgery. Informed consent was obtained prior to participation in the study from PD patients. Patients’ travelling expenses were reimbursed.
Design
A repeated measures design was used. Each participant was assessed on the RNG [22, 28, 29] task with DBS on and off, with the order counterbalanced across participants. For each stimulation condition, the RNG task was performed at three rates, with a visual (2×2 cm ‘flashing’ square, on screen time 200 ms) pacing stimulus presented once every 1, 2 or 3 seconds, (that is at 1, 0.5 or 0.33 Hz) with faster rates being more demanding. In each DBS condition, the participants completed the three RNG rates either in ascending or descending order of speed/difficulty, with the order counterbalanced across participants.
Procedure
During the RNG task a ‘hat’ analogy was used to explain the concept of randomness to the patients. Participants were asked to imagine pulling a number (1–9) from a hat, saying it out loud, which they were then to replace before drawing another one. Participants were asked to produce a sequence of 100 numbers in synchrony with the pacing stimulus, responding verbally. For each condition and rate, the total time taken to generate the 100 numbers was recorded. The numbers generated by the patients were recorded and subjected to offline analysis for randomness using a computer programme which compared the participants’ output to 10,000 random numbers generated by a computer [35].
Two measures of seriation, Countscore 1 (CS1) and Countscore 2 (CS2) were obtained. CS1 measures the tendency to count up or down in ones, e.g., 1-2-3-4-5-6-7-8-9 or 8-7-6. CS2 measures the tendency to count in steps of two, e.g. 2–4–6 or 8–6–4–2. CS1 is interpreted as the employment of a habitual counting strategy, ingrained over years of exposure to counting in series. The use of CS2 is taken as evidence of an alternative and more controlled counting strategy, whereby the participant attempts to avoid habitual counting in ones. These two Countscore measures show differential sensitivity to manipulations of cognitive load, such as performing paced RNG at faster rates or concurrently with a secondary task. In healthy participants, while the CS1 measure of automatic processing increases, the CS2 index of more controlled processing decreases with increases of cognitive load at faster rates and under dual task conditions [24, 28, 29].
Statistical analysis
The data were screened for outliers, which led to two participants in the initial sample of 17 being excluded as they had standardised residuals (z-scores) greater than 3. A series of repeated measures ANOVAs (analysis of variance) were employed. Three individual two-way ANOVAs at each rate with DBS condition and Type of Countscore as the within-subjects repeated measures factors were performed. These were followed by post-hoc paired t-tests to assess the direction of any significant main effects. If the processing strategy that patients employ on-stimulation during RNG is load-dependent, then one would predict a differential effect of DBS on the CS1 and CS2, which occurs at the fastest rate but not the two slower rates.
RESULTS
Patients obtained a significant clinical benefit from DBS (see Table 1). On medication, UPDRS scores were significantly lower with DBS On (M = 9.7, SD = 6.8) than with DBS Off (M = 18.92, SD = 7.56); t(11) = 5.56, p < 0.001. Significant clinical improvement was also seen off medication, with lower UPDRS scores when DBS was on (M = 18.46, SD = 13.05) than with DBS off (M = 40.11, SD = 17.81); t(12) = 4.97, p < 0.001. Therefore, bilateral STN DBS resulted in a significant improvement in the motor symptoms of PD, evident in every patient operated on, indicating that at least one of the contacts was correctly positioned close to the surgical target.
DBS condition had a significant effect on RNG processing strategies at the fastest 1 Hz rate
The two-way ANOVA at the fastest rate showed a significant main effect on Type of Countscore; F(1,13) = 13.89, p = 0.003. At the fastest rate of paced RNG, regardless of whether stimulation was on or off, participants consistently scored higher on CS1 than on CS2 (see Fig. 1). This indicates that there is a bias towards the use of automatic over controlled processing strategies during paced RNG at the fastest rate. Crucially, the analysis also revealed a significant DBS x Type of Countscore interaction; F(1,13) = 7.44, p = 0.017. A series of post-hoc paired t-tests clarified the nature of this effect. First, comparisons were made to determine if DBS condition had any effect on the Countscores individually. For CS1, participants scored significantly higher when DBS was on (M = 89.36, SD = 48.96) compared to when stimulation was off (M = 71.57, SD = 47.85); t(13) = 2.35, p = 0.035. DBS status had no effect on CS2 scores (p > 0.05). Subsequently the differences between CS1 and CS2 for each DBS status in isolation were compared. With DBS on, participants scored significantly higher on CS1 (M = 89.36, SD = 48.96) than on CS2 (M = 29.79, SD = 9.56); t(13) = 4.12, p < 001. Similarly, with DBS off participants scored more highly on CS1 (M = 71.57, SD = 47.85) than on CS2 (M = 33.64, SD = 10.06). However, the differences between CS1 and CS2 were significantly greater when stimulation was on (M = 59.57, SD = 54.1) than when stimulation was off (M = 37.93, SD = 48.02); t(13) = 2.78, p = 0.02. Therefore, three preliminary conclusions can be drawn. 1) At the fastest rate of paced RNG, participants adopted a more automatic habitual counting strategy and used a controlled processing strategy less when generating sequences of numbers. 2) At the fastest rate of paced RNG, the bias towards the use of automatic processing strategies was significantly magnified with stimulation on compared to off. 3) At the fastest rate of paced RNG, DBS status had no effect on the use of controlled processing strategies.
DBS condition did not have an effect on RNG processing strategies at the slower 0.5 Hz rate
If the effect of DBS on processing strategies is load-dependent, then it would be expected that significant main and interaction effects of DBS would only occur at the fastest rate, but not for the two slower rates. The results of the two-way repeated measures ANOVA with Countscore and DBS condition as within-subjects factors support this hypothesis. There is a similar pattern of numerical trends between the two faster 1 Hz and 0.5 Hz rates (See Fig. 2). CS2 was lower overall than CS1 and unaffected by DBS condition and CS1 was higher when stimulation was on compared to off. However, the interaction and both main effects were not significant (ps > 0.05). So, when cognitive demands of the RNG task are reduced and the rate at which the patients must produce random sequences of numbers slows by as little as one second, the effects of DBS on paced RNG become drastically weakened as differences between the use of controlled and automatic processing strategies begin to diminish.
DBS condition had no effect on automatic processing and was associated with the recovery of controlled processing at the slowest 0.33 Hz rate
A two-way repeated measures ANOVA with DBS condition and Type of Countscore as the within-subjects factors was performed. At the slowest rate, with DBS on, the trend for CS1 scores to exceed CS2 scores persisted and neither the interaction nor the two main effects were significant (ps > 0.05). However, paired t-tests revealed a significant increase in CS2 when STN stimulation was turned off. Patients scored higher on CS2 with stimulation off (M = 49.3, SD = 19.87) than on (M = 36.7, SD = 11.51); t(9) =−2.49, p = 0.035. In fact, CS2 levels increased to such an extent at the slowest rate with stimulation turned off that they matched CS1 scores with DBS off (mean score of 49). This may be interpreted as the switching to or the ‘recovery’ of controlled processing at the slowest rate with STN stimulation turned off. At the slowest rate of 0.33 Hz, patients had sufficient time to employ controlled processing strategies to a comparable level alongside the automatic processing strategies (see Fig. 3).
RNG performance was not influenced by the patients’ ability to keep up with the pacing stimulus
One potential caveat to the above conclusions is that participants’ choice of RNG strategy may be influenced by an impaired ability to keep up with the pacing stimulus, rather than any direct effect on automatic and controlled processing. To rule our this possibility, a 2×3 repeated measures ANOVA was conducted on the time taken to generate 100 numbers during RNG with DBS status as one factor (on vs. off) and rate of pacing stimulus (1 vs. 0.5 vs. 0.33 Hz) as the other factor. As expected, there was a significant main effect of pacing stimulus rate on time taken to generate 100 numbers; F(2,14) = 83.52, p < 0.001. However, while the mean time taken to generate 100 numbers was slightly quicker with DBS on than with DBS off across all three pacing stimulus rates, there was no significant main effect of DBS or significant interaction between pacing stimulus rate and DBS status (ps > 0.05), (see Fig. 4). Therefore, the rate at which participants generated numbers was consistent with each pacing stimulus rate, ruling out the possibility that DBS status influenced the patients’ ability to keep up with the pacing stimulus.
STN DBS improves the motor symptoms of PD but exacerbates cognitive control deficits
To examine the relationship between the therapeutic efficacy of STN DBS on motor symptoms and changes in the use of automatic and controlled processing, a series of Pearson’s correlation coefficients were calculated. Change scores for motor symptoms were calculated by subtracting UPDRS scores on medication/off stimulation from UPDRS scores with on medication/ on stimulation, such that larger UPDRS change scores reflected greater improvement in the motor symptoms with DBS on. For CS1 and CS2, change scores were calculated by subtracting scores with DBS on from DBS off, such that a smaller change score is indicative of a decrease in the use of either automatic or controlled processing and vice versa. When CS1 was combined together for all three rates, there was a significant negative correlation between CS1 change scores and UPDRS change scores, [r =−0.75, p = 0.012]. This indicates that greater improvement in the motor symptoms as a result of STN DBS on was associated with an increased use of automatic processing (see Fig. 5). Conversely, when CS2 was combined together for all three rates, there was a significant positive correlation between the CS2 change score and UPDRS change score [r = 0.64, p = 0.047]. This indicates that greater improvements in the motor symptoms when stimulation is switched on were associated with a decreased use of controlled processing (see Fig. 6). As such, there is a significant association between the simultaneous improvement of motor symptoms and increasing impairment of cognitive control with STN DBS.
Summary
Our results support the hypothesis that the effect of STN DBS on processing strategies employed during RNG is load-dependent. While STN DBS was effective in treating the motor symptoms of PD, it was also associated with deterioration of cognitive control during fast-paced RNG. In general, there was a tendency for PD patients to favour the use of automatic processing (habitual counting in ones) over controlled processing during paced RNG. Nevertheless, rate or load-dependent differences in controlled and automatic processing as a function of STN DBS were also observed. Stimulation in the vicinity of the STN significantly increased automatic processing reflected by increased habitual counting (CS1) relative to DBS off at the fastest rate only. In addition, at the slowest rate with stimulation off, greater use of controlled strategies as indexed by increased CS2 scores meant that differences between the use of controlled and automatic processing strategies disappeared altogether.
DISCUSSION
The present findings provide evidence for a load-dependent effect of STN DBS in PD on measures of randomness during paced RNG. In order to generate a random sequence of numbers, participants must inhibit the pre-potent tendency to habitually count in series and instead employ a controlled processing strategy to generate numbers randomly. On each trial, the paced RNG task demands the selection of one of nine competing responses in synchrony with the pacing stimulus. We have demonstrated that the existing bias in PD towards the selection of automatic processing strategies is significantly exacerbated when the STN stimulation is on at the fastest rate but not with DBS off or at the two slower rates of paced RNG. To test the hypothesis that STN DBS induces load-dependent deficits in cognitive control, we compared the use of automatic and controlled response strategies under differing levels of cognitive load during paced RNG by manipulating the rate of the pacing stimulus. With respect to automatic processing, the results demonstrated that STN DBS significantly magnified PD patients’ tendency to count in ones, such that they employed habitual counting strategies more when the stimulators were on compared to when the stimulators were off. Crucially, this effect was only significant at the fastest rate of paced RNG but not at the two slower rates. This indicates an inability to inhibit the prepotent and habitual response of counting in series under conditions of high cognitive load, probably because at the fastest rate, there is not sufficient time for patients to engage controlled processing strategies. Controlled processing is distinct from automatic processing by being attention and resource demanding [20]. Therefore, while an opposite and mirrored pattern of results for controlled compared to automatic responding may be expected, the former appears to be affected differently by cognitive load. The measure of the patients’ attempts to over-ride habitual counting in series (or controlled responding) by counting in twos (CS2), was lower than the measure of habitual/automatic counting (CS1), across almost all load and all DBS conditions. This confirms our previous findings in unoperated PD patients [24, 25] and suggests that, PD patients with or without STN DBS are less able to implement controlled processing strategies than automatic processing strategies in order to produce random sequences of numbers during RNG. In the present study, this was also the case, with the exception of one comparison at the slowest rate with DBS off. Only when the STN stimulation was switched off and patients performed RNG at the slowest rate (0.33 Hz), the measures of automatic and controlled processing equalised. This suggests that the slowest rate allowed sufficient time for the initiation of controlled processing strategies as readily as automatic strategies. However, even at this slowest rate, the patients were unable to effectively employ controlled responding with DBS on and were only able to engage in controlled responding at the slowest rate with DBS off. Our conclusions are two-fold; 1) the effect of STN DBS on switching from automatic to controlled processing during paced RNG is load-dependent and 2) automatic and controlled processing during paced RNG have different thresholds of load-dependency.
A potential limitation of this study is that it was conducted with patients on medication (and no off-medication control group), the effects of which could interfere with performance on tasks that involve impulse control. However, STN DBS allows for a significant reduction of dopaminergic medication and as such we might expect any deficits in cognitive control in this study to be less likely a result of medication and more likely to the effects of STN DBS [36].
These findings are in agreement with two previous studies that have revealed task-specific and load-dependent effects of STN DBS on the suppression of prepotent, automatic responses. STN DBS has been shown to reduce PD patients’ ability to inhibit prepotent responses on no trials of a Go/no-Go task with higher (83%) but not medium (50%) target frequency. In this study, the level of motor preparation and associated prepotency of the go response was higher in the former than the latter condition [8]. In the same study, the investigators found that STN DBS selectively impaired working memory performance on a high- but not a low-load spatial delayed response task. Similarly, it has been found that in PD, STN DBS induced impulsivity and faster reaction times [26]. This was accompanied by an inability to slow down responses to allow time for reflection during high but not low-conflict conditions of a probabilistic decision-making task. Therefore, our conclusions add weight to the emerging evidence that STN DBS interferes with the adaptive ability to switch from an automatic to a controlled processing mode of responding when a task demands it, and that this deficit is load-dependent. While STN DBS involves stimulation in the vicinity of the STN and likely involves stimulation of other adjacent neural tissue, nevertheless, from these results it may be inferred that the STN is involved in cognitive control.
STN connections to the frontal cortex are important for switching from habitual to controlled processing
It has been proposed that a critical function of the STN is switching between automatic and controlled response strategies [21]; the STN receives a switching signal from regions of the prefrontal cortex, including the pre-SMA. Single cell recordings in primates during oculomotor tasks that required such behavioural switching have revealed switch selective neurons in both the pre-SMA and the STN. Importantly, when a controlled response was required by the context, neurons in the pre-SMA fired before those in the STN, which in turn fired before response execution. Functional imaging in humans has corroborated these findings. The inferior frontal cortex and STN are both engaged when switches from a default ‘status quo’ decision are required. Decision difficulty was manipulated during a line-judgement task where the participant either had to accept a status quo decision or reject it [37]. When faced with a difficult decision, there is a tendency for healthy participants to favour the automatic, status-quo response over a controlled response. The tendency towards accepting the status quo decision, even if it was wrong, correlated positively with task difficulty. fMRI revealed activation of frontal circuits that run through the STN during trials when the STN was engaged and during high-difficulty trials where the default was rejected, or controlled responding was elicited.
The STN and frontal cortex are sufficiently interconnected to permit this switching function. Via the hyperdirect pathway, the STN receives projections from the SMA and DLPFC [38]. Of the five anatomically and functionally segregated fronto-striatal loops [39], the motor circuit between the putamen and the SMA and the associative circuit through the DLPFC and the dorsal caudate are of greatest relevance to the shifts from controlled to habitual processing and vice versa. The associative circuit and its across species homologues, has been proposed to play a critical role in goal-directed action in rats [40], non-human primates [41] and humans alike [42]. In human participants, changes in BOLD activation patterns have been demonstrated to move from associative to sensorimotor regions of the striatum during prolonged training and learning of motor tasks, as the initially controlled motor response becomes automatic and habitual with practice [43, 44]. Therefore, the control of goal-directed and habitual responding are mediated through segregated associative and motor fronto-striatal circuits respectively [42]. With imaging, researchers have established that in PD, STN DBS reduces activation in prefrontal (DLPFC, inferior frontal cortex) and anterior cingulate areas and alters pallidal-frontal/cingulate connectivity during fast-paced RNG at 1 Hz [13]. In light of these imaging results, the current findings suggest that the reduction of activity in the vicinity of the STN and increased output to the GPi with DBS results in a failure of implementing the ‘cognitive switching’ necessary for RNG task performance. As such patients employ automatic responding through habitual counting even though the task demands a controlled generation of numbers in a random fashion more so than with DBS off, with this stimulation effect being significant only at the fastest and most cognitively demanding rate.
In a highly influential neurocomputational model, it has been proposed that a critical function of the STN is to implement a global ‘hold your horses’ signal [26]. This ‘no go’ function is engaged during conditions of high conflict when a change in processing strategy is required to make an adaptive decision and temporarily delays responding to buy time for the selection of the optimal response. In a subsequent study from the same group, an increase in theta band activity over the medial prefrontal cortex was associated with raising the decision threshold for high conflict trials and that in PD, STN DBS reversed this relationship during a probabilistic decision-making task [16]. In fast-paced RNG, the selection of the controlled response, generating numbers in a random fashion, requires more time than the automatic response of habitual counting in series. Consequently, without the contribution of the STN to ‘buy time’ for this selection, performance is impaired with STN DBS on at the fastest rate of paced RNG when there is little time for such switching from automatic to controlled processing, as observed in the present results. Further support for the involvement of the STN in implementing a switch from automatic to controlled processing was provided in a study recording local field potentials from electrodes chronically implanted in the STN for DBS in 7 PD patients [45]. Relative to a control counting task, performance of RNG was associated with power increase in a narrow gamma band, which correlated with CS1 and number of repeated pairs (indices of the ability to suppress habitual counting and to engage in controlled processing) indicating that activity of the STN is directly modulated during RNG performance. Therefore, several lines of evidence indicate that switching between automatic and controlled processing depends on the integrity of the network between the STN, basal ganglia and prefrontal areas.
Clinical implications
STN DBS has been repeatedly demonstrated to be a highly effective treatment for the motor symptoms of PD. However, simultaneously and paradoxically, the executive dysfunction and cognitive control deficits associated with PD appear to be worsened during conditions of high conflict or high cognitive load when the STN stimulators are on, as supported by the present results and past studies [6, 7, 9, 10, 13, 14, 16, 26]. STN DBS has also been linked with emergence of impulse control disorders (ICDs) such as pathological gambling or shopping [46, 47], although others have reported improvement of ICDs with STN DBS in PD [5, 48]. Increased impulsivity and magnification of deficits in cognitive control with STN DBS may also contribute to the increased risk of suicides documented in a minority of cases following STN DBS surgery [49]. Disruption of the STN with DBS could have other as yet unknown implications for the ability of PD patients to exert cognitive control in making adaptive decisions during conditions of high-conflict in daily life, instead causing them to revert to automatic/status quo responses even though these may be sub-optimal. Future research would be well placed to examine the potential link between cognitive control impairments and psychiatric outcomes following this surgical intervention which nevertheless is very effective in controlling the motor symptoms of PD.
CONFLICT OF INTEREST
The authors have no conflict of interest to report.
ACKNOWLEDGMENTS
This work was funded by a R01 grant number NS40862-02 from the National Institutes of Health (PI: MJ). Leonora Wilkinson was funded by a Career Development Fellowship from Parkinson’s UK. We would like to thank the patients for their participation.
REFERENCES
1 | Limousin P, Pollak P, Benazzouz A, Hoffmann D, Broussolle E, Perret JE, Benabid AL (1995) Bilateral subthalamic nucleus stimulation for severe Parkinson’s disease Mov Disord 10: 672 674 |
2 | Krack P, Benazzouz A, Pollak P, Limousin P, Piallat B, Hoffmann D, Xie J, Benabid AL (1998) Treatment of tremor in Parkinson’s disease by subthalamic nucleus stimulation Mov Disord 13: 907 914 |
3 | Pillon B, Ardouin C, Damier P, Krack P, Houeto JL, Klinger H, Bonnet AM, Pollak P, Benabid AL, Agid Y (2000) Neuropsychological changes between “off” and “on” STN or GPi stimulation in Parkinson’s disease Neurology 55: 411 418 |
4 | Parsons TD, Rogers SA, Braaten AJ, Woods SP, Troster AI (2006) Cognitive sequelae of subthalamic nucleus deep brain stimulation in Parkinson’s disease: A meta-analysis Lancet Neurol 5: 578 588 |
5 | Smeding HM, Speelman JD, Koning-Haanstra M, Schuurman PR, Nijssen P, van Laar T, Schmand B (2006) Neuropsychological effects of bilateral STN stimulation in Parkinson disease: A controlled study Neurology 66: 1830 1836 |
6 | Jahanshahi M, Ardouin CM, Brown RG, Rothwell JC, Obeso J, Albanese A, Rodriguez-Oroz MC, Moro E, Benabid AL, Pollak P, Limousin-Dowsey P (2000) The impact of deep brain stimulation on executive function in Parkinson’s disease Brain 123: Pt 6 1142 1154 |
7 | Witt K, Daniels C, Herzog J, Lorenz D, Volkmann J, Reiff J, Mehdorn M, Deuschl G, Krack P (2006) Differential effects of L-dopa and subthalamic stimulation on depressive symptoms and hedonic tone in Parkinson’s disease J Neuropsychiatry Clin Neurosci 18: 397 401 |
8 | Hershey T, Revilla FJ, Wernle A, Gibson PS, Dowling JL, Perlmutter JS (2004) Stimulation of STN impairs aspects of cognitive control in PD Neurology 62: 1110 1114 |
9 | Hershey T, Campbell MC, Videen TO, Lugar HM, Weaver PM, Hartlein J, Karimi M, Tabbal SD, Perlmutter JS (2010) Mapping Go-No-Go performance within the subthalamic nucleus region Brain 133: 3625 3634 |
10 | Ballanger B, van Eimeren T, Moro E, Lozano AM, Hamani C, Boulinguez P, Pellecchia G, Houle S, Poon YY, Lang AE, Strafella AP (2009) Stimulation of the subthalamic nucleus and impulsivity: Release your horses Ann Neurol 66: 817 824 |
11 | Ray NJ, Jenkinson N, Brittain J, Holland P, Joint C, Nandi D, Bain PG, Yousif N, Green A, Stein JS, Aziz TZ (2009) The role of the subthalamic nucleus in response inhibition: Evidence from deep brain stimulation for Parkinson’s disease Neuropsychologia 47: 2828 2834 |
12 | Obeso I, Wilkinson L, Rodriguez-Oroz MC, Obeso JA, Jahanshahi M (2013) Bilateral stimulation of the subthalamic nucleus has differential effects on reactive and proactive inhibition and conflict-induced slowing in Parkinson’s disease Exp Brain Res 226: 451 462 |
13 | Thobois S, Hotton GR, Pinto S, Wilkinson L, Limousin-Dowsey P, Brooks DJ, Jahanshahi M (2007) STN stimulation alters pallidal-frontal coupling during response selection under competition J Cereb Blood Flow Metab 27: 1173 1184 |
14 | Wylie SA, Ridderinkhof KR, Elias WJ, Frysinger RC, Bashore TR, Downs KE, van Wouwe NC, van den Wildenberg WP (2010) Subthalamic nucleus stimulation influences expression and suppression of impulsive behaviour in Parkinson’s disease Brain 133: 3611 3624 |
15 | Frank MJ (2006) Hold your horses: A dynamic computational role for the subthalamic nucleus in decision making Neural Netw 19: 1120 1136 |
16 | Cavanagh JF, Wiecki TV, Cohen MX, Figueroa CM, Samanta J, Sherman SJ, Frank MJ (2011) Subthalamic nucleus stimulation reverses mediofrontal influence over decision threshold Nat Neurosci 14: 1462 1467 |
17 | Coulthard EJ, Bogacz R, Javed S, Mooney LK, Murphy G, Keeley S, Whone AL (2012) Distinct roles of dopamine and subthalamic nucleus in learning and probabilistic decision making Brain 135: 3721 3734 |
18 | Schroeder U, Kuehler A, Lange KW, Haslinger B, Tronnier VM, Krause M, Pfister R, Boecker H, Ceballos-Baumann AO (2003) Subthalamic nucleus stimulation effects a frontotemporal network: A PET study Ann Neurol 54: 445 450 |
19 | Schroeder U, Kuehler A, Haslinger B, Erhard P, Fogel W, Tronnier VM, Lange KW, Boecker H, Ceballos-Baumann AO (2002) Subthalamic nucleus stimulation affects striato-anterior cingulate cortex circuit in a response conflict task: A PET study Brain 125: 1995 2004 |
20 | Shiffrin RM, Schneider W (1977) Controlled and automatic human information processing: II. Perceptual learning, automatic attending and a general theory Psychol Rev 84: 127 190 |
21 | Isoda M, Hikosaka O (2008) Role for subthalamic nucleus neurons in switching from automatic to controlled eye movement J Neurosci 28: 7209 7218 |
22 | Jahanshahi M, Profice P, Brown RG, Ridding MC, Dirnberger G, Rothwell JC (1998) The effects of transcranial magnetic stimulation over the dorsolateral prefrontal cortex on suppression of habitual counting during random number generation Brain 121: Pt 8 1533 1544 |
23 | Jahanshahi M, Jenkins IH, Brown RG, Marsden CD, Passingham RE, Brooks DJ (1995) Self-initiated versus externally triggered movements. I. An investigation using measurement of regional cerebral blood flow with PET and movement-related potentials in normal and Parkinson’s disease subjects Brain 118: Pt 4 913 933 |
24 | Brown RG, Soliveri P, Jahanshahi M (1998) Executive processes in Parkinson’s disease–random number generation and response suppression Neuropsychologia 36: 1355 1362 |
25 | Dirnberger G, Frith CD, Jahanshahi M (2005) Executive dysfunction in Parkinson’s disease is associated with altered pallidal-frontal processing Neuroimage 25: 588 599 |
26 | Frank MJ, Samanta J, Moustafa AA, Sherman SJ (2007) Hold your horses: Impulsivity, deep brain stimulation, and medication in parkinsonism Science 318: 1309 1312 |
27 | Baddeley AD (1966) The capacity for generating information by randomization Q J Exp Psychol 18: 119 129 |
28 | Jahanshahi M, Dirnberger G, Fuller R, Frith CD (2000) The role of the dorsolateral prefrontal cortex in random number generation: A study with positron emission tomography Neuroimage 12: 713 725 |
29 | Jahanshahi M, Saleem T, Ho AK, Dirnberger G, Fuller R (2006) Random number generation as an index of controlled processing Neuropsychology 20: 391 399 |
30 | Hughes AJ, Daniel SE, Kilford L, Lees AJ (1992) Accuracy of clinical-diagnosis of idiopathic Parkinson’s disease - a clinicopathological study of 100 cases J Neurol Neurosurg Psychiatry 55: 181 184 |
31 | Hoehn MM, Yahr MD (1967) Parkinsonism - onset progression and mortality Neurology 17: 427 442 |
32 | Fahn S, Elton R, Members of the UPDRS, Development, Committee (1987) Unified Parkinson’s Disease Rating Scale. In Recent Developments in Parkinson’s Disease, Fahn S MC, Calne DB, Goldstein M, ed. Macmillan Healthcare Information, Florham Park, NJ, pp. 153-163 293-304 |
33 | Folstein MF, Folstein SE, McHugh PR (1975) Mini-Mental State - practical method for grading cognitive state of patients for clinician J Psychiatr Res 12: 189 198 |
34 | Beck AT, Erbaugh J, Ward CH, Mock J, Mendelsohn M (1961) An inventory for measuring depression Arch Gen Psychiatry 4: 561 |
35 | Sprott JC (1991) Routines and Examples in Cambridge University Press, Cambridge Numerical Recipes BASIC |
36 | Castrioto A, Funkiewiez A, Debu B, Cools R, Lhommee E, Ardouin C, Fraix V, Chabardes S, Robbins TW, Pollak P, Krack P (2015) Iowa gambling task impairment in Parkinson’s disease can be normalised by reduction of dopaminergic medication after subthalamic stimulation J Neurol Neurosurg Psychiatry 86: 186 190 |
37 | Fleming SM, Thomas CL, Dolan RJ (2010) Overcoming status quo bias in the human brain Proc Natl Acad Sci U S A 107: 6005 6009 |
38 | Nambu A, Tokuno H, Takada M (2002) Functional significance of the cortico-subthalamo-pallidal ‘hyperdirect’ pathway Neurosci Res 43: 111 117 |
39 | Alexander GE, DeLong MR, Strick PL (1986) Parallel organization of functionally segregated circuits linking basal ganglia and cortex Annu Rev Neurosci 9: 357 381 |
40 | Yin HH, Ostlund SB, Balleine BW (2008) Reward-guided learning beyond dopamine in the nucleus accumbens: The integrative functions of cortico-basal ganglia networks Eur J Neurosci 28: 1437 1448 |
41 | Miyachi S, Hikosaka O, Lu X (2002) Differential activation of monkey striatal neurons in the early and late stages of procedural learning Exp Brain Res 146: 122 126 |
42 | Balleine BW, O’Doherty JP (2010) Human and rodent homologies in action control: Corticostriatal determinants of goal-directed and habitual action Neuropsychopharmacology 35: 48 69 |
43 | Jueptner M, Frith CD, Brooks DJ, Frackowiak RS, Passingham RE (1997) Anatomy of motor learning. II. Subcortical structures and learning by trial and error J Neurophysiol 77: 1325 1337 |
44 | Lehericy S, Benali H, Van de Moortele PF, Pelegrini-Issac M, Waechter T, Ugurbil K, Doyon J (2005) Distinct basal ganglia territories are engaged in early and advanced motor sequence learning Proc Natl Acad Sci U S A 102: 12566 12571 |
45 | Anzak A, Gaynor L, Beigi M, Limousin P, Hariz M, Zrinzo L, Foltynie T, Brown P, Jahanshahi M (2011) A gamma band specific role of the subthalamic nucleus in switching during verbal fluency tasks in Parkinson’s disease Exp Neurol 232: 136 142 |
46 | Lim SY, O’Sullivan SS, Kotschet K, Gallagher DA, Lacey C, Lawrence AD, Lees AJ, O’Sullivan DJ, Peppard RF, Rodrigues JP, Schrag A, Silberstein P, Tisch S, Evans AH (2009) Dopamine dysregulation syndrome, impulse control disorders and punding after deep brain stimulation surgery for Parkinson’s disease J Clin Neurosci 16: 1148 1152 |
47 | Halbig TD, Tse W, Frisina PG, Baker BR, Hollander E, Shapiro H, Tagliati M, Koller WC, Olanow CW (2009) Subthalamic deep brain stimulation and impulse control in Parkinson’s disease Eur J Neurol 16: 493 497 |
48 | Ardouin C, Voon V, Worbe Y, Abouazar N, Czernecki V, Hosseini H, Pelissolo A, Moro E, Lhommee E, Lang AE, Agid Y, Benabid AL, Pollak P, Mallet L, Krack P (2006) Pathological gambling in Parkinson’s disease improves on chronic subthalamic nucleus stimulation Mov Disord 21: 1941 1946 |
49 | Voon V, Krack P, Lang AE, Lozano AM, Dujardin K, Schupbach M, D’Ambrosia J, Thobois S, Tamma F, Herzog J, Speelman JD, Samanta J, Kubu C, Rossignol H, Poon YY, Saint-Cyr JA, Ardouin C, Moro E (2008) A multicentre study on suicide outcomes following subthalamic stimulation for Parkinson’s disease Brain 131: 2720 2728 |
Figures and Tables
Fig.1
Mean countscore 1 (CS1) and countscore 2 (CS2) at the fastest rate (1 Hz) of paced random number generation, with deep brain stimulation (DBS) of the subthalamic nucleus on versus off. Error bars are standard error. An asterisk indicates the comparison between on and off stimulation was significant.
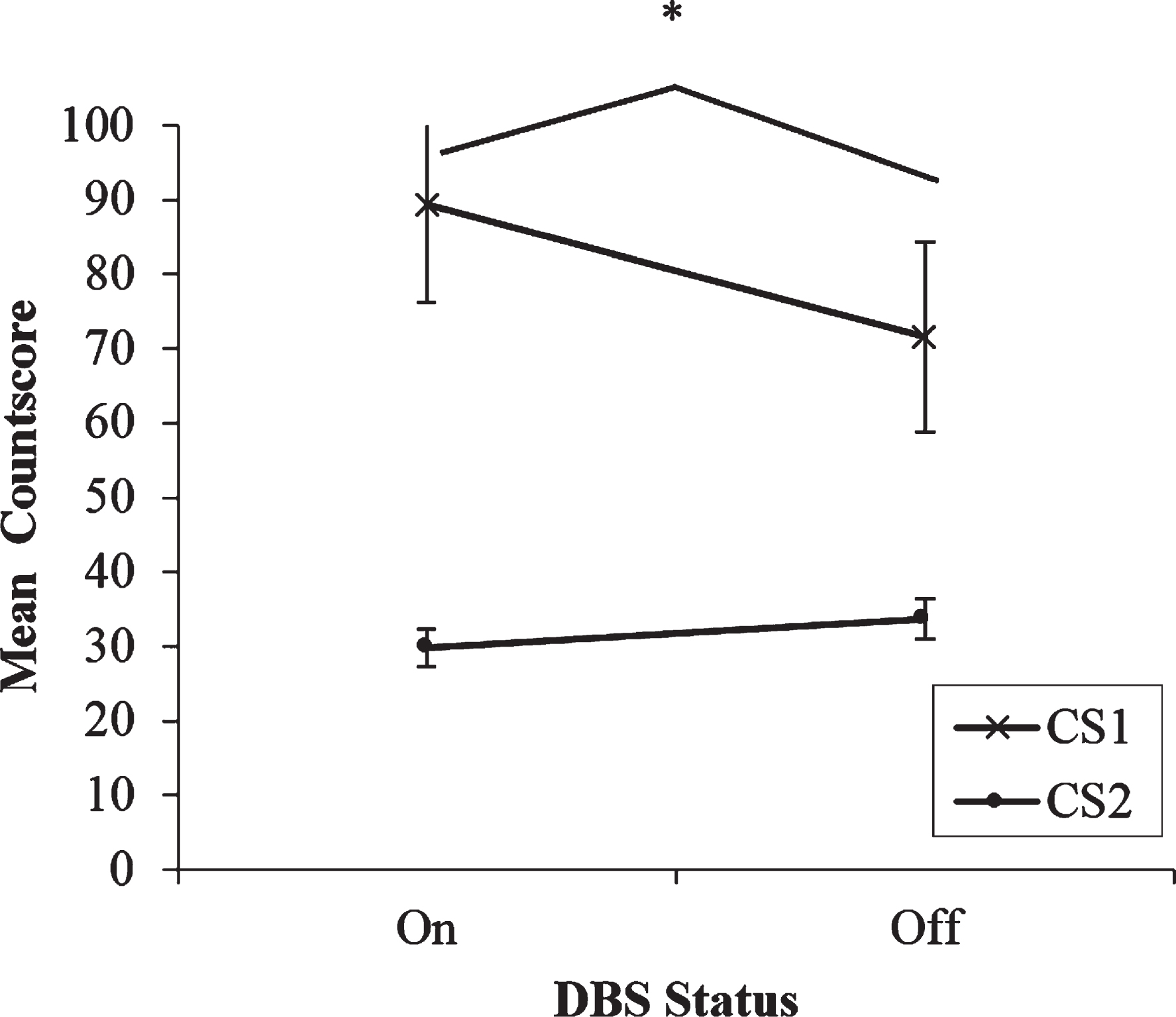
Fig.2
Mean countscore 1 (CS1) and countscore 2 (CS2) at the middle rate (0.5 Hz) of paced random number generation, with deep brain stimulation (DBS) of the subthalamic nucleus on versus off. Error bars are standard error.
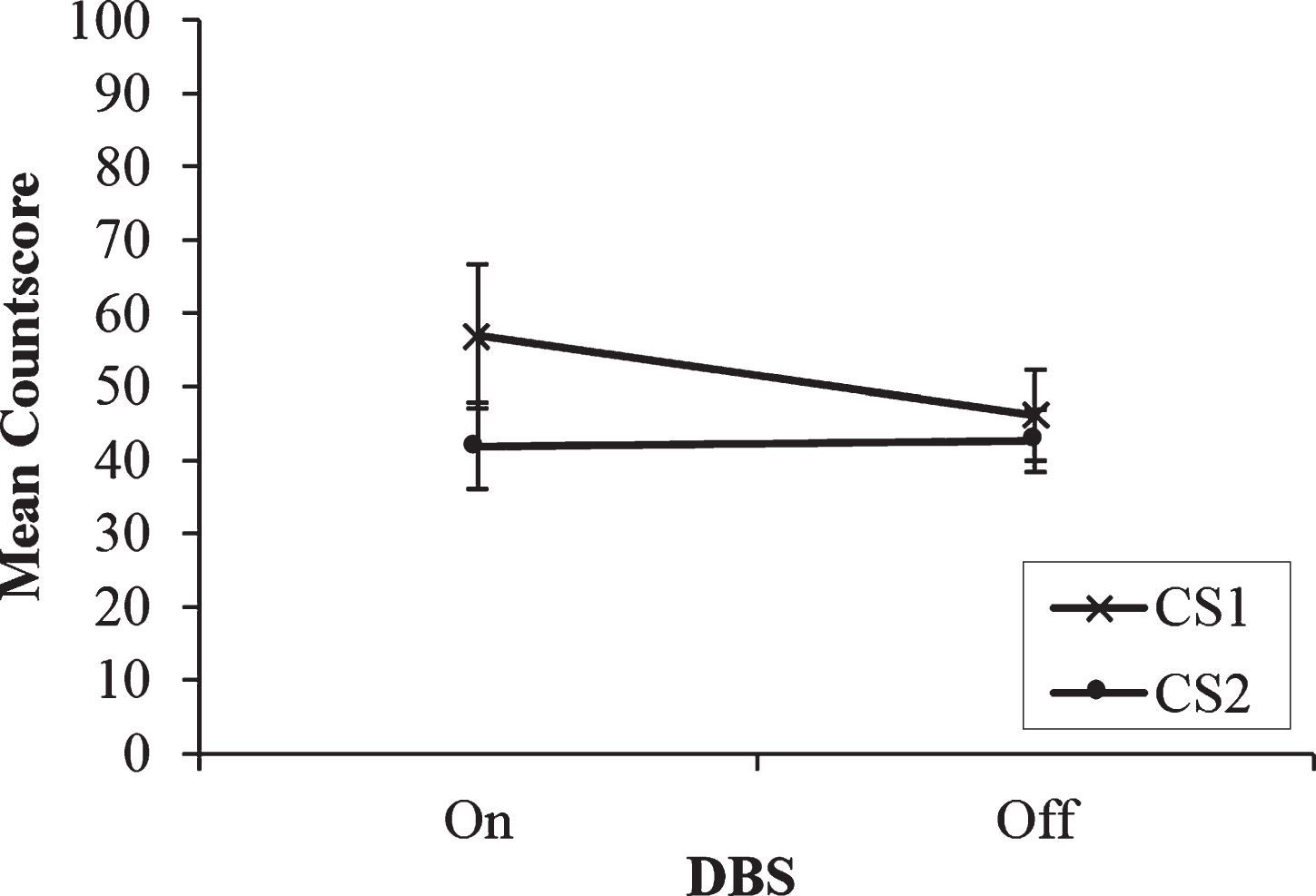
Fig.3
Mean countscore 1 (CS1) and countscore 2 (CS2) at the slowest rate (0.33 Hz) of paced random number generation, with deep brain stimulation (DBS) of the subthalamic nucleus on versus off. Error bars are standard error. An asterisk indicates the comparison between on and off stimulation was significant.
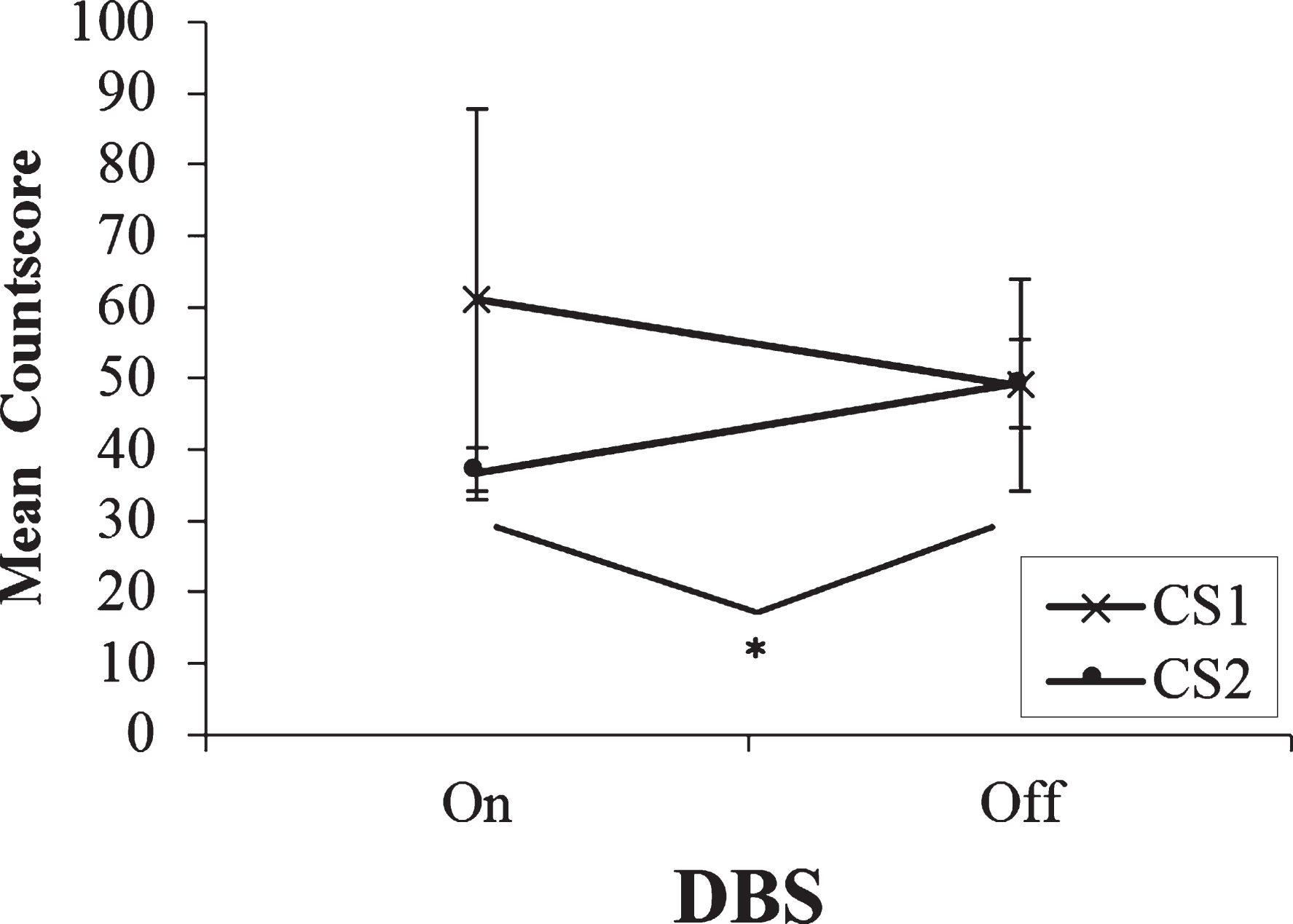
Fig.4
Mean total time taken to generate 100 numbers during paced random number generation with deep brain stimulation (DBS) of the subthalamic nucleus on or off and at the three rates of the pacing stimulus. Error bars are standard error.
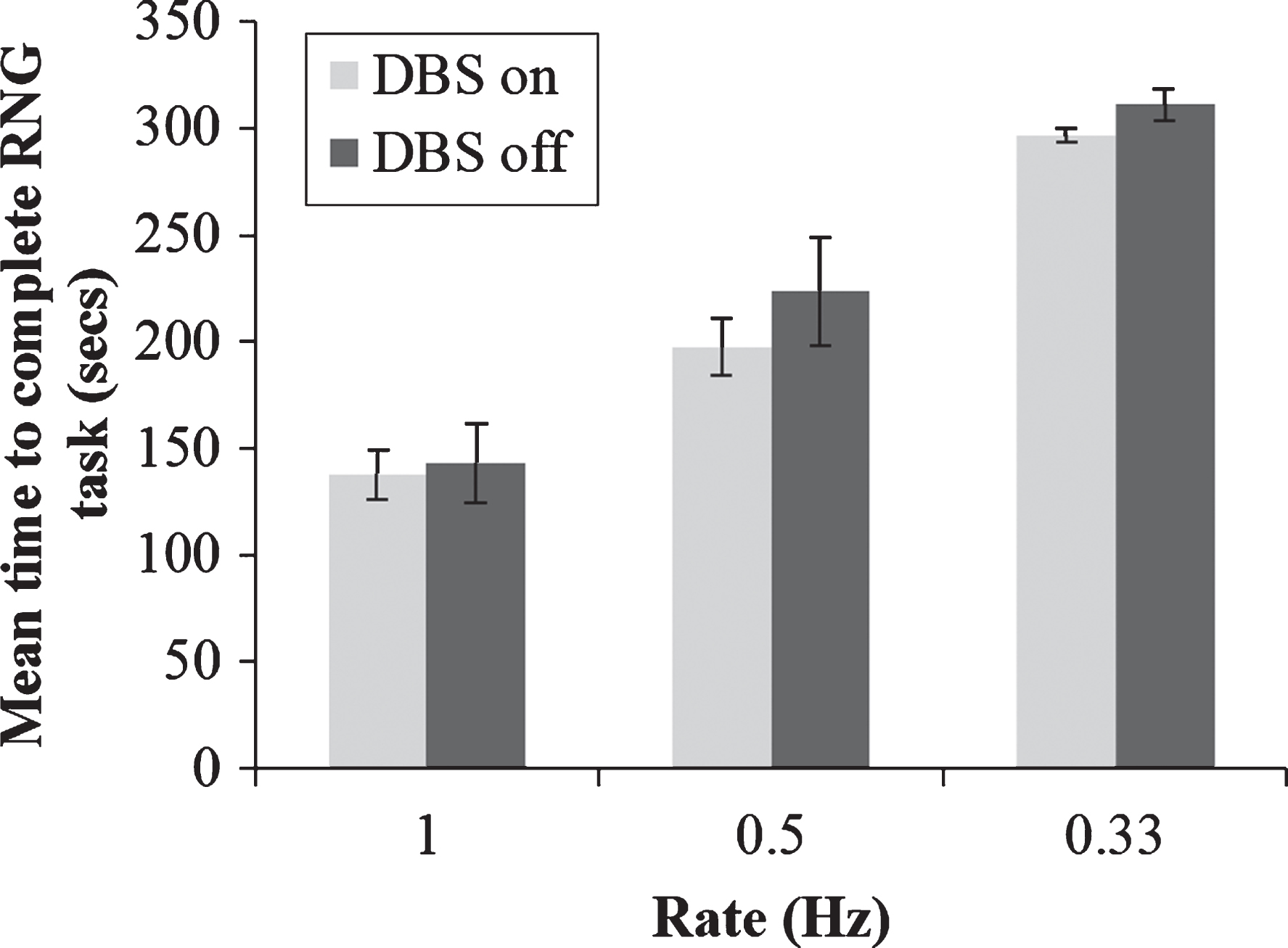
Fig.5
Scatterplot illustrating the negative correlation between change scores of the Unified Parkinson’s Disease Rating Scale (UPDRS) on versus off subthalamic stimulation and CS1 change scores, such that improvement in the motor symptoms was associated with increased use of CS1 during paced random number generation.
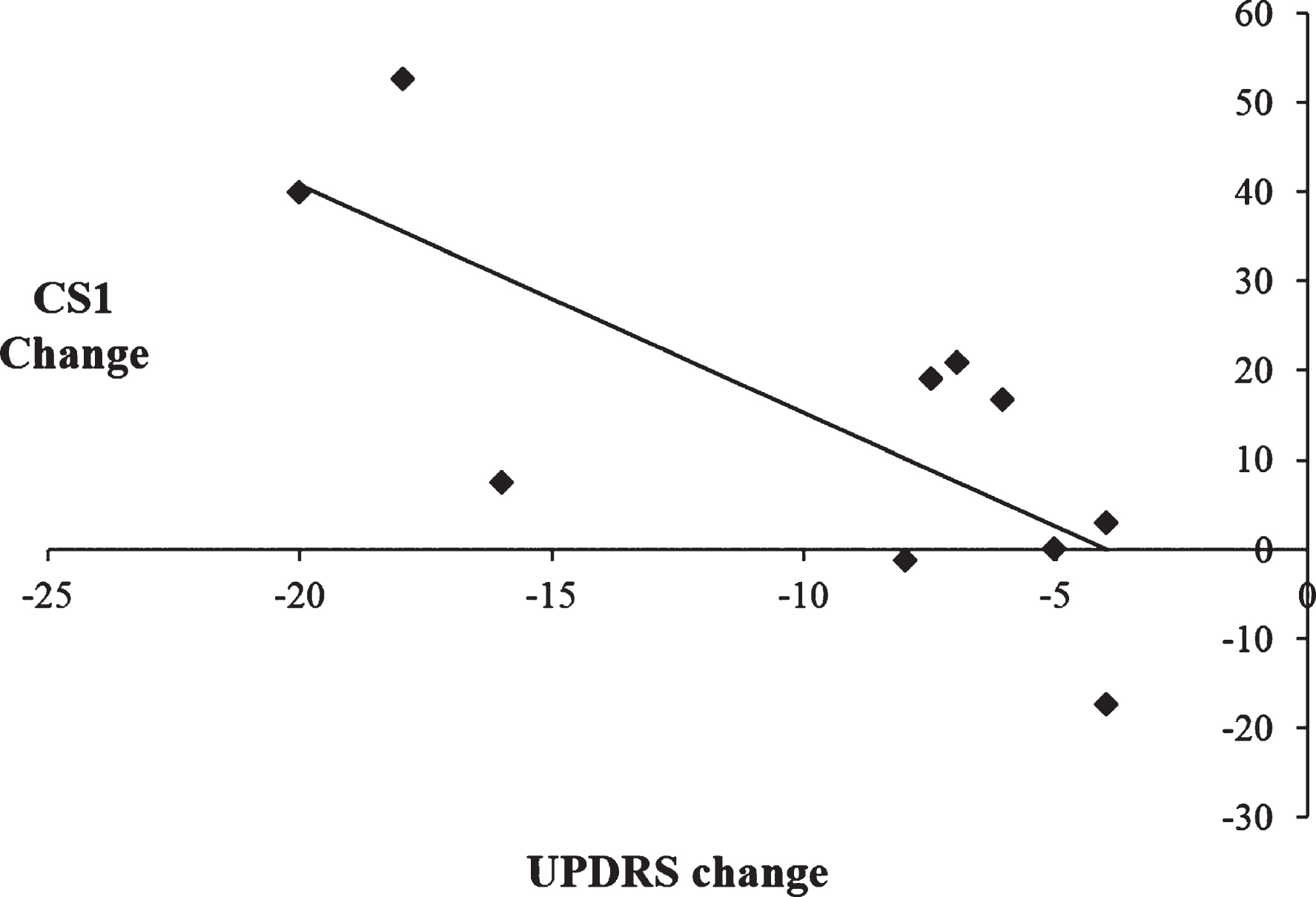
Fig.6
Scatterplot illustrating the positive correlation between the change scores of the Unified Parkinson’s Disease Rating Scale (UPDRS) on versus off stimulation and CS2 change scores with subthalamic nucleus stimulation, indicating that improvement in the motor symptoms was associated with decreased use of CS2 during paced random number generation.
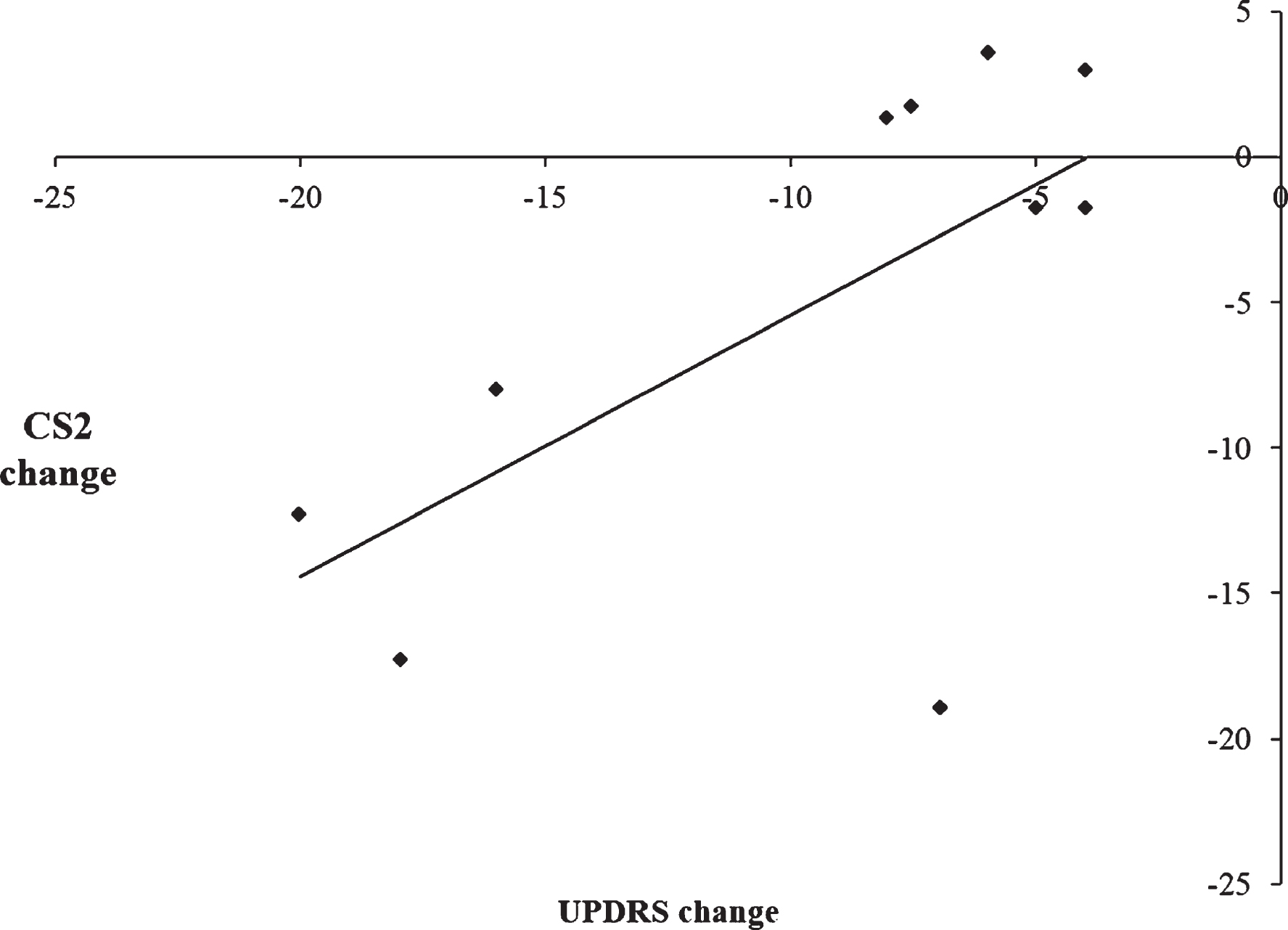
Table 1
Clinical characteristics and demographic information for patients with Parkinson’s disease
Parkinson’s disease (N = 15) | Mean (SD) | P | ||
Age (years) | 57.13 (6.40) | |||
Education (years) | 13.96 (2.52) | |||
Disease Duration (years) | 14.93 (4.18) | |||
Time since surgery (months) | 16 (15.69) | |||
Unified Parkinson’s Disease Rating Scale (off med, DBS on) (n = 14) | 18.46 (13.1) | <0.001 | ||
Unified Parkinson’s Disease Rating Scale (off med, DBS off) (n = 14) | 40.11 (17.81) | |||
Right (n = 15) | Voltage (V) | 3.02 (0.66) | ||
pulse width (ms) | 60 (0) | |||
Stimulation | frequency (Hz) | 136 (16.28) | ||
parameters | Left (n = 15) | Voltage (V) | 3.15 (0.62) | |
pulse width (ms) | 64 (10.56) | |||
frequency (Hz) | 139.67 (20.48) |