Cardiac Involvement in LAMA2-Related Muscular Dystrophy and SELENON-Related Congenital Myopathy: A Case Series
Abstract
Background:
LAMA2-related muscular dystrophy (LAMA2-MD) and SELENON-related myopathy (SELENON-RM) are two rare neuromuscular diseases characterized by proximal and axial muscle weakness, scoliosis, spinal rigidity, low bone quality and respiratory impairment. Cardiac involvement has previously been described in retrospective studies and case reports, but large case series and prospective studies in unselected cohorts are lacking.
Objective:
The objective of this study is to conduct prevalence estimations, perform cardiac phenotyping, and provide recommendations for clinical care.
Methods:
In this case series including two time points, we conducted comprehensive assessments with electrocardiography (ECG) and transthoracic echocardiography (TTE). ECGs were systematically assessed for a large subset of variables. TTE included left and right ventricular ejection fraction (LVEF/RVEF) and left ventricular global longitudinal strain (GLS), the latter being a more early and sensitive marker of left ventricular dysfunction.
Results:
21 LAMA2-MD (M = 5; 20±14 years) and 10 SELENON-RM patients (M = 7; 18±12 years) were included. In most patients, QRS fragmentation and Q waves, markers of heterogeneous ventricular activation, were present both at baseline and at follow-up. GLS was abnormal (age specific in children, > –18% in adults) in 33% of LAMA2-MD and 43% of SELENON-RM patients at baseline. Reduced LVEF (<52% in males, <54% in females and <55% in pediatric population) was observed in three LAMA2-MD patients at baseline and in none of the SELENON-RM patients. GLS and LVEF did not change between baseline and follow-up. RVEF was normal in all patients.
Conclusion:
ECG abnormalities and abnormal GLS are prevalent in LAMA2-MD and SELENON-RM, yet abnormal LVEF was only seen in LAMA2-MD patients. One LAMA2-MD patient had a clinically relevant deterioration in LVEF during 1.5-year follow-up. We advise routine screening of all patients with LAMA2-MD or SELENON-RM with ECG and echocardiography at diagnosis, minimally every two years from second decade of life and if new cardiac signs arise.
LIST OF ABBREVIATIONS
ACE inhibitor | angiotensin-converting enzyme inhibitor |
ARB | angiotensin receptor blockers |
CMR | cardiac magnetic resonance imaging |
DMD | Duchenne muscular dystrophy |
ECG | electrocardiography |
EACVI | European association of cardiovascular imaging |
GLS | global longitudinal strain |
ICD | implantable cardio-defibrillator |
LAMA2-MD | LAMA2-related muscular dystrophy |
(i)LBBB | (incomplete) left bundle branch block |
LV | left ventricle |
LVEF | left ventricular ejection fraction |
MDC1A | merosin-deficient congenital muscular dystrophy type 1A |
MFM-20/32 | motor function measurement 20/32 |
PH | pulmonary hypertension |
(i)RBBB | (incomplete) right bundle branch block |
RV | right ventricle |
RVEF | right ventricular ejection fraction |
SELENON | SELENON(SEPN1)-related |
(SEPN1)-RM | congenital myopathy |
TDI | tissue doppler imaging |
TTE | transthoracic echocardiography |
2P | biplane |
3D | tridimensional |
3P | triplane |
INTRODUCTION
LAMA2-related muscular dystrophy (LAMA2-MD) and SELENON(SEPN1)-related congenital myopathy (SELENON-RM) are rare neuromuscular diseases characterized by slowly progressive axial and proximal muscle weakness, early-onset spinal rigidity, scoliosis, low bone quality and respiratory impairment [1–3]. LAMA2-MD and SELENON-RM are caused by pathologic variants in the LAMA2 and SELENON (SEPN1) genes, coding for the laminin alpha2 subunit and selenoprotein N, respectively. Currently, no curative treatment options exist for neither LAMA2-MD nor SELENON-RM, but promising preclinical studies are ongoing [4, 5]. Supportive care from rehabilitation and allied health care in combination with optimal respiratory, nutrition and orthopedic management is essential for preventing or treating severe complications in LAMA2-MD and SELENON-RM [6, 7]. Cardiac involvement in these two neuromuscular diseases has been reported in previous retrospective studies and case series/reports. Our recent scoping review showed that left ventricle (LV) systolic dysfunction and arrhythmia were most frequently seen in LAMA2-MD, while in SELENON-RM right ventricle (RV) dysfunction secondary to respiratory failure was most prevalent [8]. However, prevalence estimations and disease specific recommendations are not available for both neuromuscular diseases. Therefore, the general consensus statements on muscular dystrophies and congenital myopathies are accepted as alternatives for cardiac screening in LAMA2-MD and SELENON-RM patients [6, 7]. In these statements, screening every two years by a cardiologist is advised in all patients. By our case series including two time points in LAMA2-MD and SELENON-RM patients we aim to perform cardiac phenotyping, to do prevalence estimations on cardiac involvement, to assess cardiac disease progression, to optimize clinical care by providing disease specific cardiac screening recommendations and to do suggestions for the design of a future natural history study. In this manuscript, we jointly discuss LAMA2-MD and SELENON-RM since they are both ultrarare neuromuscular diseases. Further, they have similar clinical phenotypes, including distribution of muscle weakness, age of onset, bone complications, respiratory insufficiency and the prevalence of contractures, which made them fit in the same study protocol. Moreover, prospective natural history studies and cross-sectional studies on cardiac function of both muscle diseases were lacking [8]. Since promising new therapies are being developed, they both are in need of appropriate outcome measures in order to reach trial readiness [1]. Similar approaches have been applied in other muscle diseases, and are efficient and may lead to new insights by learning from the similarities and differences between twodiseases [9].
MATERIAL AND METHODS
Study design
This case series including two time points in adult and pediatric patients with LAMA2-MD or SELENON-RM is part of the LAST STRONG Study: a study on LAMA2-MD and SELENON-RM To Study Trial Readiness, Outcome measures and Natural history. A full overview on the LAST STRONG study protocol can be found elsewhere [1]. Cardiac examination took place at baseline and after one-year follow-up. A key summary of the cardiac results at baseline was already published elsewhere [2, 3]. The study has been approved by the medical ethical reviewing committee Region Arnhem – Nijmegen (NL64269.091.17; 2017-3911) and is registered at ClinicalTrials.gov(NCT04478981).
Study population
Patients were recruited non-selectively and consecutively in the period of August 2020 to June 2021. Inclusion criteria include a genetic confirmation of LAMA2-MD or SELENON-RM by two recessive (likely) pathologic variants in the LAMA2 gene, or typical clinical and histological alterations combined with genetic confirmation in a first degree relative. Exclusion criteria were an insufficient understanding of the Dutch language and the unwillingness of the patient or his/her legal representatives to provide written informed consent for participation in our study. All patients performed motor function measurement 20/32 (MFM-20/32) as part of the LAST STRONG Study. Spirometry (percentage predicted of forced expiratory volume in the first second (FEV1%)) was performed in patients≥5 years.
Cardiac assessment
Electrocardiography
Standard 12-lead electrocardiogram (ECG) recordings were conducted at baseline and at one-year follow-up. All ECGs were systematically assessed for heart rate, conduction times, Q, R, S amplitudes and were compared to normative values corrected for age and sex [10]. For the patients of 40 years or older, normative values of the group aged 22 to 39 years were used, since it concerns only a few patients who all were slightly older than 40 years, and no normal values for these parameters were available [10]. Heart rate, conduction times and Q, R, S amplitudes were considered normal when having a z-score between –2 to+2 [10]. All ECGs were assessed for rhythm disorders, heart axis, QRS fragmentation, (abnormal) Q waves, poor R wave progression, (incomplete) right or left bundle branch blocks ((i)RBBB or (i)LBBB) and low QRS voltages (microvoltages). QRS fragmentation, (abnormal) Q waves, poor R wave progression, (i)RBBB and (i)LBBB are markers of heterogeneous ventricular activation and are known to predict worse cardiac outcome in neuromuscular diseases [11–14]. QRS fragmentation was defined as the presence of an additional R-wave or notching in the nadir of the R-wave or S-wave, or the presence of more than one R’ without a typical bundle branch block [15]. Q waves were defined as the first negative deflection of the QRS complex. Poor R wave progression was established when R wave amplitude in lead V2 was≤to the R wave amplitude in V3 [16, 17]. For a complete bundle branch block, QRS duration needed to be ≥120 ms in adults, ≥100 ms in children aged 4 to 16 years and ≥90 ms in children below 4 years. For an incomplete bundle branch block, QRS duration needed to be between 110–120 ms in adults, between 90–100 ms in children aged 4 to 16 years, and between 80–90 ms in children aged less than 4 years [18]. An (i)RBBB was defined by an increase in QRS duration combined by rsr’, rsR’ or rSR’ in V1 or V2. An (i)LBBB was defined by an increase in QRS duration combined by a broad notched or slurred R wave (RR’) in leads I, aVL, V5 and V6 [18]. Low QRS voltages were defined as a QRS complex of <5 mm in I, II and II, or <10 mm in aVL, aVR, aVF and V1–V6 [19]. All ECGs were assessed by two assessors (KB and FU for pediatric patients; KB and FH for adults) and all the results were subsequently checked by two others (EB and RE). In case of disagreement, ECGs were discussed until consensus wasreached.
Echocardiography
Conventional transthoracic echocardiography (TTE) with speckle tracking and tissue doppler imaging (TDI) was performed by European association of cardiovascular imaging (EACVI) TTE certified sonographers using commercially available ultrasound systems (Affiniti70 General, Philips Healthcare, Best, the Netherlands for adult participants; or Vivid E9 or Vivid E95, GE Healthcare Ultrasound, Horten, Norway for pediatric participants) [20]. Offline analysis was performed using dedicated software (AGFA Enterprise Imaging Cardiology version 8.1.2, AGFA HealthCare, Mortsel, Belgium). Strain analyses were performed offline using vendor-independent software (2D Cardiac Performance Imaging, TomTec Imaging Systems, Munich, Germany). All measurements were done according to the EACVI recommendations for cardiac chamber quantification. Left ventricular ejection fraction (LVEF) was considered decreased if <52% in males, <54% in females and <55% in the pediatric population [21, 22]. A LVEF of up to 40% is considered as mildly abnormal, of 30–40% as moderately abnormal and <30% as severely abnormal [22]. A deterioration of LVEF between baseline and follow-up was defined as a minimal decrease of 5% in LVEF, and was considered clinically relevant if in addition there was a decrease in LVEF category (i.e. normal, mildly abnormal, moderately abnormal and severely abnormal) [23]. LVEF was determined through several methods in accordance with routine clinical care, with decreasing accuracy: 3D ejection fraction, triplane, biplane, Teichholz B mode, Teichholz M mode and eyeball. Since it was not feasible to acquire 3D LVEF in all patients due to artefacts caused by thorax deformities among others, the LVEF method with the highest accuracy available in both visits was used for statistical analysis. In case the same method could not be performed at follow-up, the best alternative method at follow-up was used instead. Global longitudinal strain (GLS) is an early marker of left ventricular (LV) dysfunction and was considered abnormal if below –18% (i.e., > –18%) in adults and were age specific in the pediatric population [24, 25]. GLS was measured at baseline in 3D or, in the absence of the 3D method, in triplane (3P) or from apical four-chamber views (A4C). If possible the same methods were used atfollow-up.
Statistical analysis
Descriptive statistics were used to summarize our data in IBM SPSS Statistics 25.0.0.1 for Windows (SPSS, Inc., Chicago, IL). Values are mean±SD, unless otherwise stated. Spearman’s correlation was used to assess correlations between number of leads with QRS fragmentation, number of leads with Q waves, LVEF, GLS, MFM-20/32, FEV1% and age. A Spearman’s correlation was considered as moderate (r = 0.40–0.59), strong (r = 0.60–0.80) or very strong (0.80–1.0) [26]. A paired sample t-test was used to compare LVEF, GLS, the number of leads with QRS fragmentation and the number of leads with Q waves between baseline and follow-up. An independent sample t-test was performed to compare the number of leads with QRS fragmentation, the number of leads with Q waves, LVEF and GLS between ambulant and non-ambulant patients. An independent sample t-test was further used to compare LVEF, GLS, MFM-20/32, FEV1% and age between patients with and without QRS fragmentation, Q waves, poor R wave progression, (in) complete right bundle branch block and sinus arrhythmia. A Chi-square test was used to compared the presence of QRS fragmentation and Q waves between ambulant and non-ambulant patients. In cases of missing data, patients were excluded from this specific sub analysis. Differences were consi-dered significant if p < 0.05. Due to the descriptive character of our study, we did not perform correction for multiple testing.
Data availability
Anonymized data not published within this article will be made available by reasonable request from any qualified investigator.
RESULTS
Study population
Twenty-one LAMA2-MD patients (M = 5, F = 16) and 10 SELENON-RM patients (M = 7, F = 3) underwent ECG and echocardiography at our hospital at baseline and after one-year follow-up. The mean age at baseline was 20±14 years (range: 3 to 50 years) (LAMA2-MD) and 18±12 years (range: 3 to 42 years) (SELENON-RM). All patients had genetic confirmation before enrollment in the study [2]. Eight (38%) LAMA2-MD patients and eight (80%) SELENON-RM patients were ambulant. The mean MFM-20/32 total score was 42% ±29 in LAMA2-MD patients and 71% ±15 in SELENON-RM patients. The mean FEV1% was 56% ±24 in LAMA2-MD patients and 42% ±15 in SELENON-RM patients. During the study, one LAMA2-MD patient (patient 15, nine years) died due to post anoxic encephalopathy after cardiopulmonary arrest following asphyxiation. One LAMA2-MD patient (patient 17, 31 years) declined further cardiac follow-up at our hospital due to the high perceived burden of participating in the LAST SRONG study combined with personal circumstances. The echocardiographic data at baseline of one SELENON-RM patient (patient 10, 11 years) was lost due to technical problems. At baseline, three LAMA2-MD patients used cardiac medication, including beta blockers and ACE inhibitors. These medications were initiated before inclusion in this study by their own health care providers because of impaired cardiac function, one in childhood, one in adolescence and one in adulthood. Off note, the pediatric patient had cardiomyopathy with dilation of the left and right ventricle combined with severe insufficiency of the mitral valve, which improved after initiation of heart failure therapy with digoxin and captopril. Currently, her cardiac function is stable using metoprolol and lisinopril. None of the SELENON-RM patients used cardiac medication. An overview on key patients’ characteristics can be found in Table 1 and has been published elsewhere [2, 3]
Table 1
Overview on patients’ characteristics
Demographics | Baseline (mean±SD (range)) | After 1 year (mean±SD (range)) |
LAMA2-MD | ||
N of patients | 21 | 19 |
Age at examination (years) | 20±14 (3 to 50) | 21±15 (4 to 51) |
Males (n (%)) | 6/21 (29%) | 6/19 (32%) |
BMI – child (kg/m2) | 18.2±3.4 (13.9 to 23.7) | 19.0±3.9 (13.7 to 25.9) |
BMI – adult (kg/m2) | 20.0±3.9 (13.0 to 25.5) | 21.3±3.0 (16.9 to 25.4) |
Ambulant (%) | 8/21 (38%) | 8/19 (42%) |
Blood pressure (mmHg) | ||
13 years or older | 121±19/75±15 | 118±18/70±12 |
4 to 12 years | 93±7/57±4 | 100±11/61±5 |
3 years or younger | 78/48 | n.a. |
SELENON-RM | ||
N of participants | 10 | 10 |
Age at examination (years) | 18±12 (3 to 42) | 19±12 (4 to 43) |
Males (%) | 7/10 (30%) | 7/10 (30%) |
BMI – child (kg/m2) | 16.2±3.3 (12.6 to 20.8) | 16.4±3.8 (12.9 to 21.4) |
BMI – adult (kg/m2) | 23.0±3.0 (19.1 to 26.3) | 23.9±3.7 (20.0 to 28.6) |
N of ambulant patients (%) | 8/10 (80%) | 8/10 (80%) |
Blood pressure (mmHg) | ||
13 years or older | 128±24/81±18 | 127±23/81±19 |
4 to 12 years | 106±6/62±7 | 103±11/64±8 |
3 years or younger | 122/73 | n.a. |
LAMA2-MD = LAMA2-related muscular dystrophy; SELENON-RM = SELENON-related congenital myopathy; N = number; BMI = Body Mass Index; Ambulant was defined by being able to walk 10 meter without the need for support.
LAMA2-MD
Baseline
ECG was performed in 21 patients (Table 2, Supplementary Table 1). Heart frequency, heart axis, and ST-segment, P wave and T wave morphology were normal in all patients. QRS fragmentation was seen in 13/21 patients (Figs. 1–3). Poor R wave progression was found in 12/21 patients. Q waves were present in all patients (Figs. 1–3). Eight out of 21 patients had a sinus arrhythmia, but no arrhythmia was observed. Three patients had an iRBBB (Fig. 1). None of the patients met the criteria for low QRS voltages. We found a moderate correlation between the number of leads with Q waves and age (Spearman, –0.437, p < 0.05). There was no difference in MFM-20/32 score, LVEF, GLS and age between patients with and without QRS fragmentation, poor R wave progression and iRBBB. Furthermore, there was no difference in ambulant status between patients with and without QRS fragmentation and poor R wave progression. Patients with sinus arrythmia were significantly younger (10±3) than patients without sinus arrhythmia (26±15) (p < 0.001).
Table 2
Overview on electrocardiographic and echocardiographic features at baseline and at follow-up
Baseline | After 1 year | |
LAMA2-MD | ||
ECG | ||
Conduction times/heart rates | ||
Heart rate | 87±15 bpm (z-score 0.31) | 85±14 bpm (z-score 0.27) |
PR | 135±20 ms (z-score – 0.12) | 136±24 ms (z-score – 0.08) |
QRS | 92±10 ms (z-score 0.45) | 96±12 ms (z-score 0.61) |
QTc | 432±27 ms (z-score 0.13) | 432±22 ms (z-score 0.18) |
Peak values | ||
Q: II/III/V6 (z-score) | –0.31/–0.46/0.23 | –0.31/–0.51/–0.07 |
R: I/II/aVF/V1/V5/V6 | –0.04/–0.59/–0.58/0.90/0.08/–0.25 | 0.27/–0.51/–0.54/0.92/–0.03/–0.23 |
S: I/V1/V2/V6 | –0.62/0.47/0.59/0.02 | –0.49/0.48/0.56/–0.17 |
Other ECG characteristics | ||
QRS fragmentation | 13/21 | 15/19 |
Number of leads | 2.4±1.3 | 2.6±1.4 |
Q waves | 21/21 | 19/19 |
Number of leads | 3.7±1.9 | 3.6±1.8 |
Poor R wave progression | 12/21 | 12/19 |
iRBBB | 4/21 | 3/19 |
Sinus arrythmia | 8/21 | 7/19 |
Echocardiography | ||
LVEF | 59±7% | 57±10% |
Abnormal LVEF | 3/21 | 3/21 |
GLS | –20.2±4.0% | –21.4±4.8% |
Abnormal GLS | 4/12 | 3/13 |
SELENON-RM | ||
ECG | ||
Conduction times/heart rates | ||
Heart rate | 90±19 bpm (z-score 0.47) | 91±15 bpm (z-score 0.68) |
PR | 136±25 ms (z-score 0.47) | 138±27 ms (z-score –0.06) |
QRS | 87±10 (z-score –0.22) | 87.3±10 (z-score –0.37) |
QTc | 415±26 (z-score –0.31) | 423±15 (z-score –0.06) |
Peak values | ||
Q: II/III/V6 (z-score) | –0.45/–0.33/–0.30 | –0.47/–0.48/–0.30 |
R: I/II/aVF/V1/V5/V6 | –0.17/–0.71/–0.75/0.27/–0.16/–0.32 | 0.061/–0.73/–0.80/0.38/–0.50/–0.54 |
S: I/V1/V2/V6 | –0.19/–0.18/–0.17/–0.21 | –0.34/0.19/0.27/–0.25 |
Other ECG characteristics | ||
QRS fragmentation | 8/10 | 7/10 |
Number of leads | 2.3±1.4 | 1.8±1.3 |
Q waves | 7/10 | 8/10 |
Number of leads | 3±1.7 | 3.8±1.9 |
Poor R wave progression | 8/10 | 7/10 |
iRBBB | 2/10 | 2/10 |
Sinus arrythmia | 5/10 | 4/10 |
Echocardiography | ||
LVEF | 61±5% | 63±5% |
Abnormal LVEF | 0/9 | 0/10 |
GLS | –20.6±4.4% | –20.1±3.5% |
Abnormal GLS | 3/7 | 2/8 |
LAMA2-MD = LAMA2-related muscular dystrophy; SELENON-RM = SELENON-related congenital myopathy; ECG = electrocardiography; bpm = beats per minute; ms = milliseconds; iRBBB = incomplete right bundle branch block; LVEF = left ventricular ejection fraction; GLS = global longitudinal strain; all values are mean±SD unless otherwise stated.
Fig. 1
Electrocardiographic characteristics in a LAMA2-MD patient (patient 2, F, 8 years, baseline). QRS fragmentation in III, aVF and V1 (arrow); Q waves in I and aVL (open arrow); iRBBB (Rsr’ pattern in v1). iRBBB = incomplete right bundle branch block. LAMA2-MD = LAMA2-related muscular dystrophy.
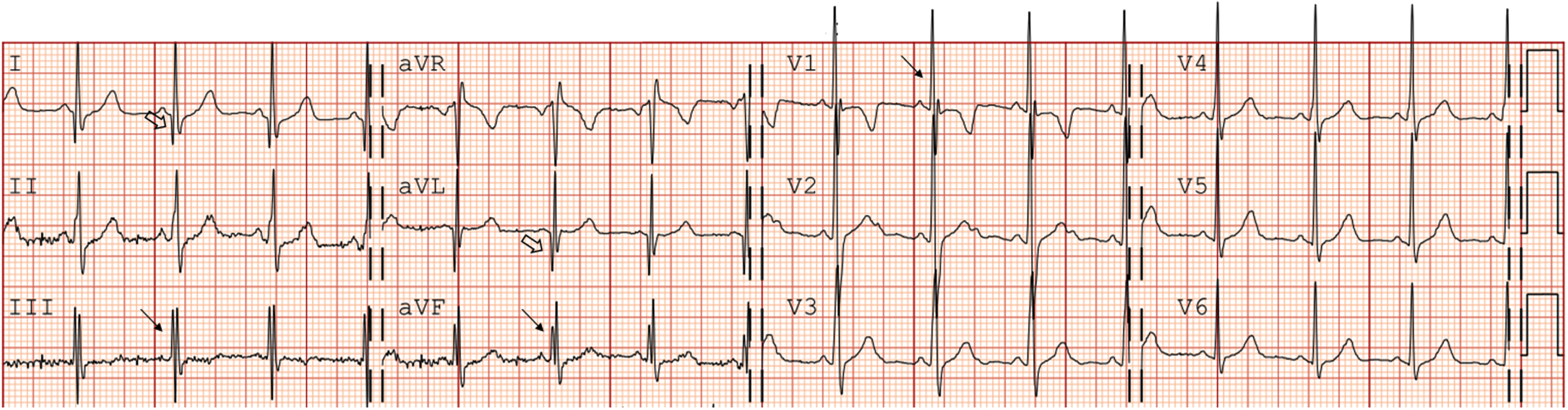
Fig. 2
Electrocardiographic changes in a LAMA2-MD patient (patient 9, male) between baseline (42 years) and follow-up (43 years). These electrocardiographic changes are accompanied by 14% decrease in LVEF. (A) ECG at baseline, showing QRS fragmentation in leads II and aVF (arrow), Q waves in leads I, aVL and V2 (open arrow) and anterior fascicular block. Heart rate is 57 beats per minute (z-score –0.87), PR is 145 ms (z-score –0.23), QRS is 109 ms (z-score 0.49) and QTc is 420 ms (z-score –0.29). (B) ECG at one-year follow-up showing QRS fragmentation in leads II, aVR, aVF, V1 and V3 (arrow), Q waves in leads I and aVL (open arrow), poor R wave progression and anterior fascicular block. Heart rate is 55 beats per minute (z-score –0.97), PR is 145 ms (–0.23), QRS is 130 ms (z-score 1.77) and QTc is 410 ms (z-score –0.57). LAMA2-MD = LAMA2-related muscular dystrophy; LVEF = left ventricular ejection fraction; ECG = electrocardiography.
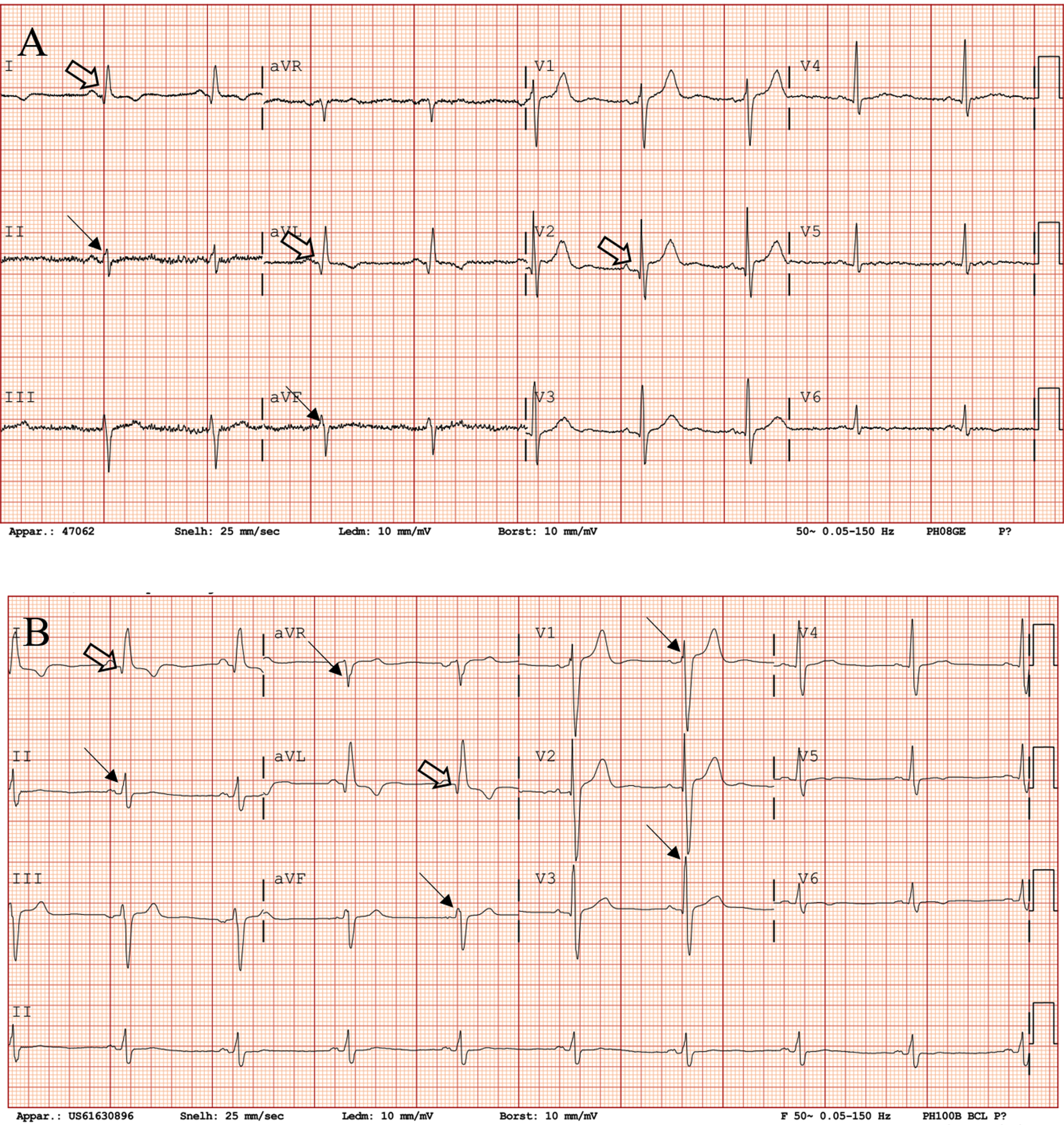
Fig. 3
QRS fragmentation and Q waves per lead in LAMA2-MD and SELENON-RM patients at baseline and follow-up. (A) number of LAMA2-MD patients with QRS fragmentation per lead at baseline; total n = 21. (B) number of LAMA2-MD patients with QRS fragmentation per lead at follow-up; total n = 19. (C) number of SELENON-RM patients with QRS fragmentation per lead at baseline; total n = 10. (D) number of SELENON-RM patients with QRS fragmentation per lead at follow-up; total n = 10. (E) number of LAMA2-MD patients with Q waves per lead at baseline; total n = 21. (F) number of LAMA2-MD patients with Q waves per lead at follow-up; total n = 19. (G) number of SELENON-RM patients with Q waves per lead at baseline; total n = 10. (H) number of SELENON-RM patients with Q waves per lead at follow-up; total n = 10. LAMA2-MD = LAMA2-related muscular dystrophy; SELENON-RM = SELENON-related congenital myopathy.
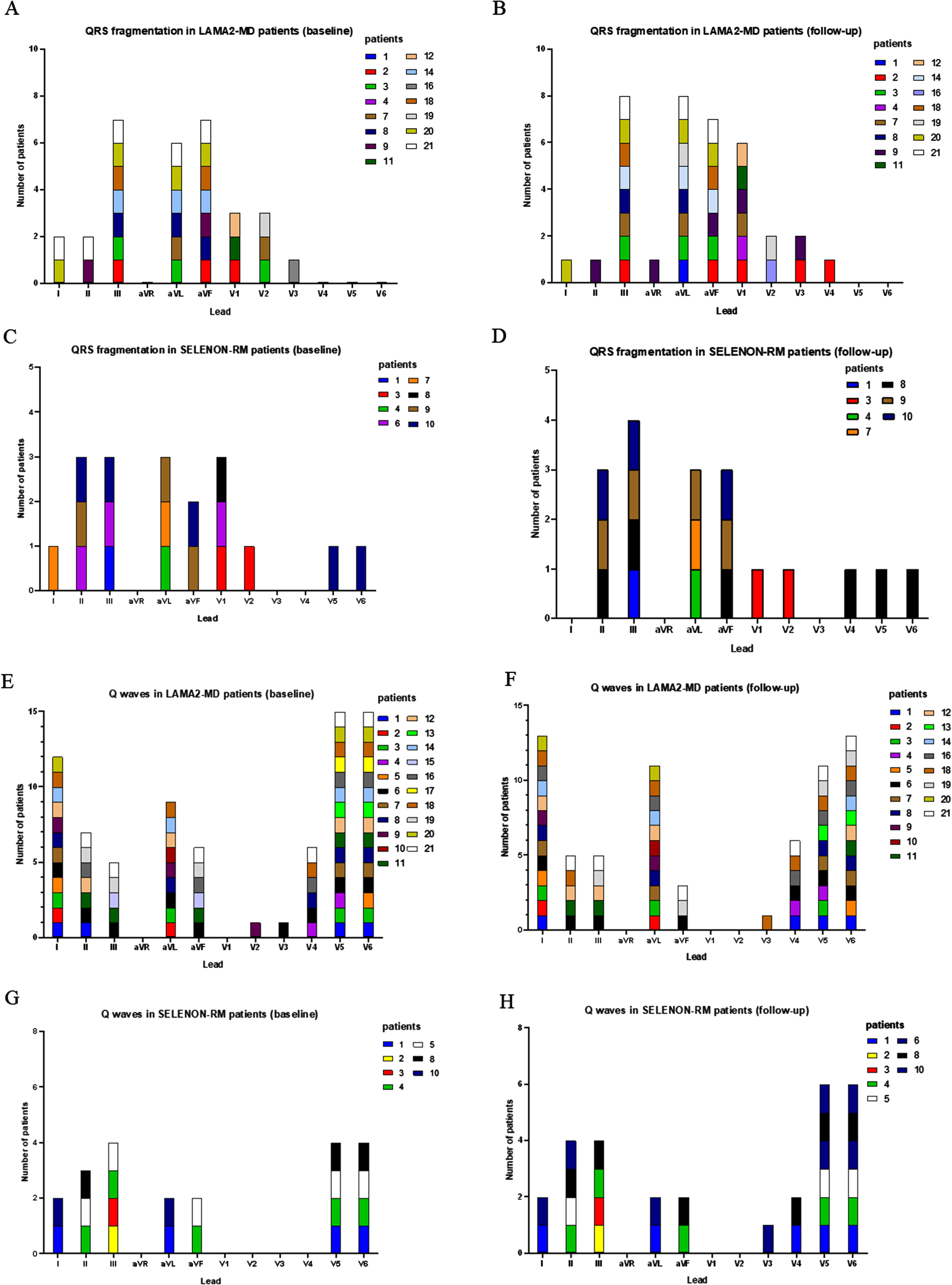
Echocardiography was performed in 21 patients, of whom 18 patients had normal LVEF (Fig. 4, Table 2, Supplementary Table 1). Three adult patients showed a mildly decreased LVEF (51% (F, 31 years), 44% (M, 42 years) and 40% (M, 21 years)). GLS was decreased in four patients (–12.4% (F, 31 years); –14.8% (M, 42 years); –17.7% (F, 13 years); –18,8% (M, 14 years)), of whom two also had a decreased LVEF. No significant abnormalities of the valves, right ventricle volume/function or atria were seen. There was a significant correlation between LVEF and GLS (Spearman, –0.755, p < 0.01) and between FEV1% and GLS (–0.648, p < 0.05). We found no correlation between LVEF and age, MFM-32, FEV1%, number of leads with QRS fragmentation or number of leads with Q waves, and no correlation between GLS and age or MFM-32. There was no difference in LVEF and GLS between ambulant and non-ambulant patients.
Fig. 4
LVEF and GLS at baseline and change in LVEF and GLS between baseline at follow-up. (A) LVEF against age in years at baseline. There was no significant correlation between LVEF and age in both LAMA2-MD and SELENON-RM, yet there was a tendency of LVEF to decrease with increasing age. (B) GLS (%) against age in years at baseline. There was a significant correlation between age and GLS in SELENON-RM (Spearman’s correlation, 0,821, p < 0.05), but not in LAMA2-MD. (C) Difference between baseline LVEF and LVEF at follow-up. A negative value implies an amelioration in LVEF, while a positive value implies a deterioration in LVEF. There was a significant correlation (Spearman’s correlation, 0.471, p < 0.05) in LAMA2-MD patients between change in LVEF and age. There was no significant correlation in SELENON-RM patients, yet there was a tendency for a deterioration of LVEF with age. (D) Difference between baseline GLS and GLS at follow-up. A negative value implies an deterioration in GLS, while a positive value implies a amelioration in GLS. There was no significant correlation between change in GLS from baseline to follow-up and age for both LAMA2-MD and SELENON-RM. LAMA2-MD = LAMA2-related muscular dystrophy; SELENON-RM = SELENON-related congenital myopathy; LVEF = left ventricular ejection fraction; GLS = global longitudinal strain.
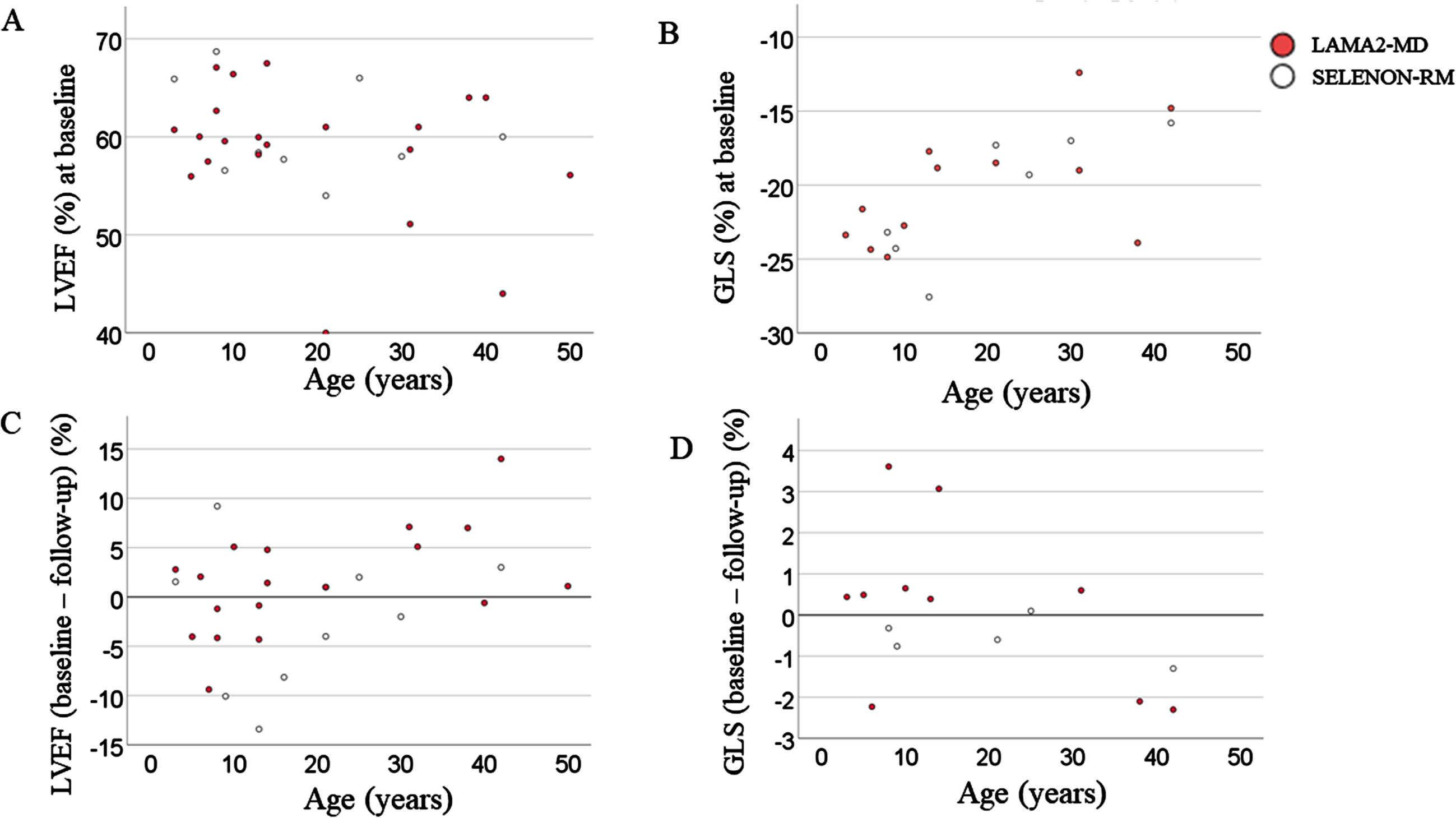
One-year follow-up
ECG was performed in 19 patients (Table 2, Supplementary Table 1). In one patient (patient 9) having a normal QRS duration at baseline (109 ms), had a striking increase in QRS duration to 130 ms (z-score 1.77) at follow-up. This was accompanied by a deterioration in LVEF from 44% to 30%. Two patients (patient 1 and patient 4) had new QRS fragmentation (Fig. 3). The number of leads with QRS fragmentation or Q waves per patient was not changed between baseline and follow-up. There was also no correlation between the number of leads having fragmented QRS complexes or Q waves and LVEF, GLS, MFM-20/32 or age. There is a significant difference in GLS between patients with (–19.8±4.2) and without (–26.6±2.8) poor R wave progression (p = 0.024). Similar to baseline, we found a significant difference in age between patients with (11±3) and without (27±16) sinus arrythmia (p = 0.022).
Echocardiography was performed in 19 patients (Fig. 4, Table 2, Supplementary Table 1). We found no difference in LVEF and GLS between baseline and follow-up. We found a moderate correlation between age and LVEF (Spearman, –0.494, p < 0.05). There was no correlation between LVEF and GLS, MFM-20/32 or FEV1, and no correlation between GLS and MFM-20/32 or FEV1.
SELENON-RM
Baseline
ECG was performed in 10 patients (Table 2, Supplementary Table 1). Heart rate, QRS conduction times, QTc times, and ST-segment, P wave and T wave morphology were normal in all patients. One patient had a right heart axis. QRS fragmentation was seen in eight patients. Poor R wave progression and Q waves were seen in seven patients. Five patients had a sinus arrhythmia and two an iRBBB. None of the patients met the criteria for low QRS voltages. We found no correlation between the number of leads with QRS fragmentation or the number of leads with Q waves, and LVEF, GLS, MFM or age. We found no difference in GLS, MFM-20/32, age or ambulant status between patients with and without QRS fragmentation. Furthermore, MFM-20/32 was decreased in patients with an iRBBB (49±8%) compared to patients without an iRBBB (79±6%) (p < 0.001). There was no difference in MFM-20/32, LVEF, GLS and age between patients with an without Q waves, poor R wave progression and sinusarrythmia.
Echocardiography was performed in nine patients, of whom all had normal LVEF (Fig. 4, Table 2, Supplementary Table 1). GLS could be measured in seven patients and was decreased in three patients (–17.3 (M, 21 years); –15.8 (M, 42 years); –17 (F, 30 years)). No significant abnormalities of the valves, right ventricle volume/function or atria were seen. We found a correlation between age and GLS (Spearman’s correlation, 0,821, p < 0.05) or FEV1 (Spearman’s correlation, 0,800, p < 0.01). There was also a correlation between GLS and FEV1 (Spearman’s correlation, 0,786, p < 0.05). We found no correlation between LVEF and age, GLS, MFM-32 or FEV1, and no correlation between GLS and MFM-32. There was no difference in LVEF and GLS between ambulant and non-ambulant patients.
One-year follow-up
ECG was performed in 10 patients (Table 2, Supplementary Table 1). QRS fragmentation disappeared in one pediatric (patient 8) and in one adult (patient 6) patient. New Q waves were seen in one patient. The number of leads with QRS fragmentation or Q waves per patient was not changed between baseline and follow-up. There was no differences in LVEF, GLS, MFM-20/32 or age between patients with and without fragmented QRS complexes, Q waves, iRBBB and sinus arrhythmia.
Echocardiography was performed in 10 patients, of whom all had normal LVEF (Fig. 4, Table 2, Supplementary Table 1). GLS could be measured in eight patients, of whom two patient had an abnormal GLS (–14.5% (M, 43 years), –18,1% (M, 12 years). Of one patient having an abnormal GLS (–17% (F, 31 years)) at baseline, the GLS could not be measured at follow-up due to poor image quality. Of the other patient having an abnormal GLS (–17.3%), the GLS increased to just above cut-off value at follow-up (–18.8% (M, 22 years)). There was no difference in LVEF and GLS between baseline and follow-up. We found a correlation between GLS and age (Spearman’s correlation, 0.857, p < 0.001) and LVEF (Spearman’s correlation, –0.833, p < 0.5). We found no correlation between LVEF and age, MFM-20/32 or FEV1%, and no correlation between GLS, and MFM-32 or FEV1%.
DISCUSSION
This case series on cardiac involvement in LAMA2-MD and SELENON-RM patients fills in an important knowledge gap on cardiac phenotyping, prevalence estimations and cardiac disease progression. The main findings include: 1) prevalent QRS fragmentation, 2) frequent Q waves, 3) poor R wave progression and 4) abnormal GLS in both neuromuscular diseases, while 5) decreased LVEF was only found in LAMA2-MD patients. This study enables us to optimize clinical care by providing disease specific cardiac recommendations on clinical care and research, which we will discuss below.
Cardiac involvement in neuromuscular diseases
Cardiac involvement is a key symptom in several other neuromuscular diseases, including Duchenne muscular dystrophy (DMD), limb-girdle muscular dystrophies and myotonic dystrophy, and yearly routine cardiac screening is recommended [27]. Clinically relevant cardiac involvement most commonly consists of either cardiomyopathy, conduction defects or arrhythmias. The underlying pathophysiology of this cardiac involvement in other neuromuscular diseases includes myocardial fibrosis and scarring [27–29]. Our recent scoping review on LAMA2-MD and SELENON-RM patients showed cardiac involvement in all ages [8]. In LAMA2-MD, LV systolic dysfunction was prevalent, while in SELENON-RM RV dysfunction secondary to respiratory failure was frequently found. An autopsy study showed extensive interstitial fibrosis of the myocardium in one LAMA2-MD patient, which has not been confirmed in larger cohorts [30]. We found no autopsy studies in SELENON-RM patients [8]. Below we discuss the electrocardiographic and echocardiographic findings and their clinical relevance from case series in LAMA2-MD and SELENON-RM.
Electrocardiographic findings
QRS fragmentation was an outstanding electrocardiographic abnormality in our LAMA2-MD and SELENON-RM cohorts and might indicate myocardial involvement. A fragmented QRS complex is a marker of heterogenous myocardial electrical activation that can be caused by ventricular chamber dilatation, myocardial scarring and fibrosis which result in a slow and inhomogeneous myocardial conduction [31]. QRS fragmentation is a good predictor of mortality and cardiac events among patients with coronary artery disease or idiopathic dilated cardiomyopathy [15, 32]. QRS fragmentation was further associated with cardiomyopathy, a lower LVEF, more frequent arrhythmias and requirement of cardiac medical therapies or cardiac device intervention in other neuromuscular diseases [11, 33]. QRS fragmentation has also been described to be a predictor of sudden cardiac death in patients with coronary artery disease and also in nonischemic cardiomyopathy [34]. However, QRS fragmentation is a nonspecific finding and should only be interpreted in the presence of pertinent clinical evidence of myocardial fibrosis. We are not aware of any Dutch or Flemish patients that died of sudden cardiac death, which might partly be explained by the small cohorts combined with the lack of standardized clinical phenotyping (including cause of death) in national databases before the start of this study.
We additionally found frequent Q waves in the contiguous leads, while most of those waves were not fitting the criteria for abnormal Q waves. Abnormal Q waves can be a clue for different disease entities, for which age is an important predicting factor for the underlying pathophysiology [35]. In older patients (>40 years), pathologic Q waves are often a sign of myocardial infarction. Contrarily, in the younger patients, abnormal Q waves are mostly a sign of congenital heart disease or cardiomyopathy unrelated to myocardial infarction. In patients with DMD, abnormal Q waves were the most common electrocardiographic abnormality [12]. Abnormal Q waves were mostly at the anterolateral wall leads (I, aVL, V4–V6), but could also appear at the inferior wall leads (II, III, aVF). Further, abnormal Q waves were common in patients with severe myotonic dystrophy [36]. In LAMA2-MD and SELENON-RM patients, we mostly saw Q waves that did not fit our definition of ‘abnormal Q waves’. However, pronounced Q waves were often present in many leads, were in some patients remarkably deep (>2 mm), were seen in right-sided leads and were >25% of depth of the QRS complex which might indicate cardiac involvement (Fig. 1).
Poor R wave progression can be caused by multiple underlying pathophysiological mechanisms including anterior myocardial infarction, LV hypertrophy, RV hypertrophy or it can be a variant of normal [37]. In DMD, tall R waves and increased R/S amplitude in V1 are prevalent [38]. This is caused by decreased muscle mass of the LV due to fibrosis and scarring and results in a decreased leftward depolarization. Consequently, the overall depolarization becomes more rightward, which leads to a tall R wave in V1 and poor R wave progression. Poor R wave progression in LAMA2-MD and SELENON-RM might thus indicate myocardial involvement.
(i)RBBB was found in some LAMA2-MD and SELENON-RM patients. In the general population, especially in the pediatric, an (i)RBBB can be a benign finding [39, 40]. However, it can also represent underlying myocardial disease and can be a predictor of mortality in certain patient populations [40, 41]. (i)RBBB has previously been described in other neuromuscular diseases, including DMD and myotonic dystrophy [42]. In a French study, 34% of the DMD patients had a RBBB, which was a predictive factor associated with cardiac events [13]. An (i) RBBB in LAMA2-MD and SELENON-RM patients could thus reflect underlying cardiomyopathy. On the other hand, it can also be a benign finding, since it is mainly present in the pediatric population, sometimes spontaneously disappears and is not related with an abnormal cardiac function. Also sinus arrhythmia, which was frequently seen in our (pediatric) population, was considered as benign [43].
Echocardiographic findings
LVEF was abnormal in three (14%) LAMA2-MD patients, both at baseline and follow-up, and in none of the SELENON-RM patients, which is in line with the results of our scoping review [8]. No other underlying pathophysiology than cardiomyopathy as part of the LAMA2-MD disease spectrum was identified. RV dysfunction due to severe respiratory impairment was the most common cardiac feature previously described in SELENON-RM. Chronic respiratory failure causes pulmonary vasoconstriction, muscularization of non-muscular arterioles, increased media thickness, perivascular leakage and inflammatory infiltration, leading to pulmonary hypertension [44]. Contrarily to the literature, we did not observe right ventricle dysfunction [8]. This could be attributed to strict respiratory follow-up in our cohort and the prompt start of respiratory support. Strict respiratory follow-up as part of clinical care is advised to prevent cardiac complications.
Abnormal GLS was prevalent in both LAMA2-MD and SELENON-RM patients. GLS is an echocardiographic index of LV systolic function and is a more reproducible and sensitive method for the evaluation of LV function than left ventricular ejection fraction (LVEF) [45]. However, the value of GLS in neuromuscular diseases remains unclear. In DMD, GLS can detect subtle left ventricular systolic dysfunction before the decline of LVEF [46]. Early detection of cardiac dysfunction is crucial to initiate cardiac medication in time in order to reverse remodeling and improve prognosis. Both at baseline and at follow-up, in two out of four patients with an abnormal GLS, LVEF was abnormal. Moreover, the only LAMA2-MD patient having a clinically relevant decrease in LVEF between baseline and follow-up (patient 9), had a very abnormal GLS (–14.8%) at baseline. Although our cohort is too small and the follow-up period is too short to statistically support GLS as an early marker of cardiomyopathy in LAMA2-MD and SELENON-RM, both the literature on GLS in other neuromuscular diseases combined with the findings in our cohort point towards this direction.
We did not find a correlation between either LVEF or GLS and the motor function (MFM-20/32). Motor function will therefore not be taken into account for cardiac recommendations for clinical care.
Recommendation for clinical care
Based on the results of this case series, our recent scoping review and on the recommendations on cardiac care in other muscle diseases, we recommend cardiac screening with ECG and echocardiography with GLS in all LAMA2-MD and SELENON-RM patients by a cardiologist at diagnosis, at least every two years from second decade of life and if new cardiac signs arise. We opt for personal coordination between the parent(s) and treating physician for the frequency of cardiac screening in the interim period. This recommendation is based on several observations in this study, our recent scoping review and on natural history studies in other congenital muscular dystrophies and congenital myopathies. First, we found a decreased LVEF in several LAMA2-MD patients, and prevalent abnormal GLS in both LAMA2-MD and SELENON-RM patients. Second, our scoping review showed decreased LVEF in LAMA2-MD patients and RV failure in SELENON-RM patients [8]. Third, the literature in other neuromuscular diseases proposes GLS as early detector of decreased cardiac function [46, 47]. Fourth, QRS fragmentation and frequent Q waves can be a sign of underlying myocardial involvement. The screening interval is in line with the current clinical guidelines on congenital myopathies and congenital muscular dystrophies by Wang et al. [6, 7]. If abnormalities are detected on ECG and/or echocardiography, we recommend cardiac magnetic resonance imaging (CMR). Furthermore, in patients with abnormal LV function, we recommend initiating nonpharmacological and pharmacological interventions in line with the heart failure guidelines [48, 49]. Nonpharmacological interventions include treatment adherence and health maintenance behaviors, the latter inclu-ding restricting sodium intake, staying physically active, and getting vaccinations. Pharmacological interventions include renin-angiotensin system inhibition with angiotensin-converting enzyme (ACE) inhibitors or angiotensin receptor blockers (ARBs) as first step to reduce morbidity and mortality.
Recommendations for research
For future research, we propose long-term prospective cardiac follow-up in a large cohort of LAMA2-MD and SELENON-RM patients to further examine the predictive value of ECG abnormalities and abnormal GLS or LVEF and to optimize the recommendations for clinical care. This calls for international collaboration due to the rarity of these diseases. Further, we suggest myocardial tissue cha-racterization through CMR [50, 51]. CMR can assess the presence of myocardial inflammation, infiltration or fibrosis. The location of this cardiac involvement on MRI should subsequently be correlated to the leads on ECG with QRS fragmentation, Q waves and poor R waves progression. This is of particular interest in LAMA2-MD and SELENON-RM due to the ECG (QRS fragmentation, Q waves, poor R wave progression) and echocardiographic findings that indicate myocardial involvement. Further, CMR is a more sensitive technique than echocardiography for early diagnosis of cardiac involvement and for prediction of long-term outcomes [52].
Strength and limitations
Major strengths of our study include the standardized and prospective follow-up in an unselected cohort of LAMA2-MD and SELENON-RM patients, with great variability in age and disease severity, using the most sensitive echocardiographic functions. This enables us to do prevalence estimations, a more detailed cardiac phenotyping and consequently disease specific recommendations. Further, the method of ECG assessment, performed by four (pediatric) cardiologists (FH, EB, RE and FU) with special focus on cardiac involvement in neuromuscular diseases and a PhD candidate in (pediatric) neurology (KB), improves the validity of the study. Finally, the majority of the Dutch and Dutch-speaking Belgium patients were included, with only minimal loss from follow-up [2]. However, the number of patients in this study is low, which is inherent to rare neuromuscular diseases. In LAMA2-MD relatively more woman, while in SELENON-RM relatively more men are included. Further, a one-year follow-up period is too short in these slowly progressive neuromuscular diseases in order to assess cardiac disease progression and to determine risk factors for developing cardiac dysfunction on the long-term. We acknowledge that a one-year follow-up period is too short to have expected any significant changes between the two assessments. A major strength of the design of our study includes the possibility to extend the follow-up period provided that financial resources are available. This will enable us to further adapt our recommendations on the frequency of cardiac follow-up. Finally, due to severe thorax deformities, image quality of the echocardiography was not always sufficient to perform 3D LVEF and 3D GLS, resulting in missing data in generally the most severely affected patients.
CONCLUSION
This study shows cardiac involvement in a subset of LAMA2-MD and SELENON-RM patients, irrespective of motor function. This stresses the need for routine cardiac screening as part of clinical care in these patients.
ACKNOWLEDGEMENTS
We thank all patients and their relatives for participation in our study. We thank Marit Boxum and Daniëlle Franken for their help in contacting patients and requesting medical data from other hospitals. We thank the technicians, especially Jacquelien van den Dungen-Toet, for performing echocardiography and assisting us with the analysis. Several authors of this publication are members of the Radboudumc Neuromuscular Center (Radboud-NMD), Netherlands Neuromuscular Center (NL-NMD) and European Reference Network for rare neuromuscular diseases (EURO-NMD).
FUNDING
The study is financially supported by a compe-titively awarded, peer-reviewed grant from Stichting Spieren voor Spieren, Stichting Stofwisselkracht, and the Stichting Voor Sara, The Netherlands.
CONFLICT OF INTEREST
The authors have no conflict of interest to report.
ETHICS APPROVAL AND CONSENT TO PARTICIPATE
This study was registered at clinicaltrials.gov (NCT04478981). This study was approved by the medical ethical reviewing committee of Region Arnhem-Nijmegen (NL-number NL64269.091.17, dossier number 2017-3911; date of approval of last amendment: 8 July 2020). From all patients, or in case of children their parent or legal guardian, informed consent has been obtained for participating in our study.
DATA AVAILABILITY
The data that support the findings of this study are available within the article and supplementary material, and from the corresponding author, upon reasonable request.
AUTHOR CONTRIBUTIONS
KB: study concept design, inclusion of patients, (co-) performing all medical examinations, collection of all data, assessment and critical analysis of electrocardiography and echocardiography, performing all (statistical) analyses, and manuscript writing and revision. FvdH, RN, FU: study concept design, collection of all data, assessment and critical analysis of electrocardiography and echocardiography, supervision of KB, and critical revision of manuscript. RE, EB: assessment and critical analysis of electrocardiography and echocardiography, and critical revision of manuscript. JG, BE, CE, NV: study concept design, supervision of KB, and critical revision of manuscript. All authors read and approved the manuscript.
SUPPLEMENTARY MATERIAL
[1] The supplementary material is available in the electronic version of this article: https://dx.doi.org/10.3233/JND-230190.
REFERENCES
[1] | Bouman K , Groothuis JT , Doorduin J , van Alfen N , Udink Ten Cate FEA , van den Heuvel FMA , et al. Natural history, outcome measures and trial readiness in LAMA2-related muscular dystrophy and SELENON-related myopathy in children and adults: protocol of the LAST STRONG study. BMC Neurol. (2021) ;21: (1):313. |
[2] | Bouman K , Groothuis JT , Doorduin J , van Alfen N , Udink Ten Cate FEA , van den Heuvel FMA , et al. LAMA2-Related Muscular Dystrophy Across the Life Span: A Cross-sectional Study. Neurol Genet. (2023) ;9: (5):e200089. |
[3] | Bouman K , Groothuis JT , Doorduin J , van Alfen N , Udink Ten Cate FEA , van den Heuvel FMA , et al. SELENON-Related Myopathy Across the Life Span, a Cross-Sectional Study for Preparing Trial Readiness. J Neuromuscul Dis. (2023) ;10: (6):1055–74. |
[4] | Barraza-Flores P , Bates CR , Oliveira-Santos A , Burkin DJ . Laminin and Integrin in LAMA2-Related Congenital Muscular Dystrophy: From Disease to Therapeutics. Front Mol Neurosci. (2020) ;13: :1. |
[5] | Filipe A , Chernorudskiy A , Arbogast S , Varone E , Villar-Quiles R-N , Pozzer D , et al. Defective endoplasmic reticulum-mitochondria contacts and bioenergetics in SEPN1-related myopathy. Cell Death & Differentiation. (2021) ;28: (1):123–38. |
[6] | Wang CH , Bonnemann CG , Rutkowski A , Sejersen T , Bellini J , Battista V , et al. Consensus statement on standard of care for congenital muscular dystrophies. J Child Neurol. (2010) ;25: (12):1559–81. |
[7] | Wang CH , Dowling JJ , North K , Schroth MK , Sejersen T , Shapiro F , et al. Consensus statement on standard of care for congenital myopathies. J Child Neurol. (2012) ;27: (3):363–82. |
[8] | Bouman K , Gubbels M , van den Heuvel FMA , Groothuis JT , Erasmus CE , Nijveldt R , et al. Cardiac involvement in two rare neuromuscular diseases: LAMA2-related muscular dystrophy and SELENON-related myopathy. Neuromuscular Disorders. (2022) ;32: (8):635–42. |
[9] | Park JJH , Siden E , Zoratti MJ , Dron L , Harari O , Singer J , et al. Systematic review of basket trials, umbrella trials, and platform trials: A landscape analysis of master protocols. Trials. (2019) ;20: (1):572. |
[10] | Bratincsák A , Kimata C , Limm-Chan BN , Vincent KP , Williams MR , Perry JC . Electrocardiogram Standards for Children and Young Adults Using Z-Scores. Circ Arrhythm Electrophysiol. (2020) ;13: (8):e008253. |
[11] | Cho M-J , Lee J-W , Lee J , Shin YB , Lee HD . Relationship Between Fragmented QRS Complexes and Cardiac Status in Duchenne Muscular Dystrophy: Multimodal Validation Using Echocardiography, Magnetic Resonance Imaging, and Holter Monitoring. Pediatric Cardiology. (2017) ;38: (5):1042–8. |
[12] | Takami Y , Takeshima Y , Awano H , Okizuka Y , Yagi M , Matsuo M . High Incidence of Electrocardiogram Abnormalities in Young Patients With Duchenne Muscular Dystrophy. Pediatric Neurology. (2008) ;39: (6):399–403. |
[13] | Fayssoil A , Ben Yaou R , Ogna A , Chaffaut C , Leturcq F , Nardi O , et al. Left bundle branch block in Duchenne muscular dystrophy: Prevalence, genetic relationship and prognosis. PLoS One. (2018) ;13: (1):e0190518. |
[14] | Tang L , Shao S , Wang C . Electrocardiographic features of children with Duchenne muscular dystrophy. Orphanet Journal of Rare Diseases. (2022) ;17: (1):320. |
[15] | Pietrasik G , Zaręba W . QRS fragmentation: Diagnostic and prognostic significance. Cardiol J. (2012) ;19: (2):114–21. |
[16] | DePace NL , Colby J , Hakki AH , Manno B , Horowitz LN , Iskandrian AS . Poor R wave progression in the precordial leads: Clinical implications for the diagnosis of myocardial infarction. J Am Coll Cardiol. (1983) ;2: (6):1073–9. |
[17] | Brunet Garcia L , Hajra A , Field E , Wacher J , Walsh H , Norrish G , et al. Cardiac Manifestations of Myotonic Dystrophy in a Pediatric Cohort. Front Pediatr. (2022) ;10: :910660. |
[18] | Surawicz B , Childers R , Deal BJ , Gettes LS , Bailey JJ , Gorgels A , et al. AHA/ACCF/HRS recommendations for the standardization and interpretation of the electrocardiogram: Part III: Intraventricular conduction disturbances: A scientific statement from the American Heart Association Electrocardiography and Arrhythmias Committee, Council on Clinical Cardiology; the American College of Cardiology Foundation; and the Heart Rhythm Society. Endorsed by the International Society for Computerized Electrocardiology. J Am Coll Cardiol. (2009) ;53: (11):976–81. |
[19] | Madias JE . Low QRS voltage and its causes. J Electrocardiol. (2008) ;41: (6):498–500. |
[20] | (EACVI) EAoCI. EACVI Certification in Adult Transthoracic Echocardiography (TTE). Certification organised by the European Association of Cardiovascular Imaging (EACVI) https://www.escardio.org/Education/Career-Development/Certification/Adult-Transthoracic-Echo. |
[21] | Tissot C , Singh Y , Sekarski N . Echocardiographic Evaluation of Ventricular Function-For the Neonatologist and Pediatric Intensivist. Front Pediatr. (2018) ;6: :79. |
[22] | Kosaraju A , Goyal A , Grigorova Y , Makaryus AN . Left Ventricular Ejection Fraction. StatPearls. Treasure Island (FL): StatPearls Publishing.Copyright © 2022, StatPearls Publishing LLC.; (2022) . |
[23] | Pellikka PA , She L , Holly TA , Lin G , Varadarajan P , Pai RG , et al. Variability in Ejection Fraction Measured By Echocardiography, Gated Single-Photon Emission Computed Tomography, and Cardiac Magnetic Resonance in Patients With Coronary Artery Disease and Left Ventricular Dysfunction. JAMA Netw Open. (2018) ;1: (4):e181456. |
[24] | Truong VT , Phan HT , Pham KNP , Duong HNH , Ngo TNM , Palmer C , et al. Normal Ranges of Left Ventricular Strain by Three-Dimensional Speckle-Tracking Echocardiography in Adults: A Systematic Review and Meta-Analysis. J Am Soc Echocardiogr. (2019) ;32: (12):1586–97.e5. |
[25] | Levy PT , Machefsky A , Sanchez AA , Patel MD , Rogal S , Fowler S , et al. Reference Ranges of Left Ventricular Strain Measures by Two-Dimensional Speckle-Tracking Echocardiography in Children: A Systematic Review and Meta-Analysis. J Am Soc Echocardiogr. (2016) ;29: (3):209–25.e6. |
[26] | Spearman C . The Proof and Measurement of Association between Two Things. The American Journal of Psychology. (1904) ;15: (1):72–101. |
[27] | Feingold B , Mahle WT , Auerbach S , Clemens P , Domenighetti AA , Jefferies JL , et al. Management of Cardiac Involvement Associated With Neuromuscular Diseases: A Scientific Statement From the American Heart Association. Circulation. (2017) ;136: (13):e200–e31. |
[28] | Frankel KA , Rosser RJ . The pathology of the heart in progressive muscular dystrophy: Epimyocardial fibrosis. Hum Pathol. (1976) ;7: (4):375–86. |
[29] | Petri H , Ahtarovski KA , Vejlstrup N , Vissing J , Witting N , Køber L , Bundgaard H . Myocardial fibrosis in patients with myotonic dystrophy type A cardiovascular magnetic resonance study. J Cardiovasc Magn Reson. (2014) ;16: (1):59. |
[30] | Hissong E , Salvatore S , Tanji K , Lavi E . Congenital muscular dystrophy, cardiomyopathy, and peripheral neuropathy due to merosin deficiency: Peripheral nerve histology of cauda equina. Human Pathology: Case Reports. (2016) ;4: :4–8. |
[31] | de Bakker JM , van Capelle FJ , Janse MJ , Tasseron S , Vermeulen JT , de Jonge N , Lahpor JR . Slow conduction in the infarcted human heart ‘Zigzag’ course of activation. Circulation. (1993) ;88: (3):915–26. |
[32] | Sha J , Zhang S , Tang M , Chen K , Zhao X , Wang F . Fragmented QRS is associated with all-cause mortality and ventricular arrhythmias in patient with idiopathic dilated cardiomyopathy. Ann Noninvasive Electrocardiol. (2011) ;16: (3):270–5. |
[33] | Nikhanj A , Yogasundaram H , Kimber S , Siddiqi ZA , Oudit GY . Clinical utility of 12-lead electrocardiogram in evaluating heart disease in patients with muscular dystrophy: Assessment of left ventricular hypertrophy, conduction disease, and cardiomyopathy. Ann Noninvasive Electrocardiol. (2021) ;26: (6):e12876. |
[34] | Das MK , Zipes DP . Fragmented QRS: A predictor of mortality and sudden cardiac death. Heart Rhythm. (2009) ;6: (3 Suppl):S8–14. |
[35] | MacAlpin RN . Significance of abnormal Q waves in the electrocardiograms of adults less than 40 years old. Ann Noninvasive Electrocardiol. (2006) ;11: (3):203–10. |
[36] | Olofsson BO , Forsberg H , Andersson S , Bjerle P , Henriksson A , Wedin I . Electrocardiographic findings in myotonic dystrophy. Br Heart J. (1988) ;59: (1):47–52. |
[37] | Zema MJ , Kligfield P . ECG poor R-wave progression: Review and synthesis. Arch Intern Med. (1982) ;142: (6):1145–8. |
[38] | Rajdev A , Groh WJ . Arrhythmias in the muscular dystrophies. Card Electrophysiol Clin. (2015) ;7: (2):303–8. |
[39] | Floria M , Parteni N , Neagu AI , Sascau RA , Statescu C , Tanase DM . Incomplete right bundle branch block: Challenges in electrocardiogram diagnosis. Anatol J Cardiol. (2021) ;25: (6):380–4. |
[40] | Harkness WT , Hicks M . Right Bundle Branch Block. StatPearls. Treasure Island (FL): StatPearls Publishing. Copyright © 2022, StatPearls Publishing LLC.; (2022) . |
[41] | Rankinen J , Haataja P , Lyytikäinen LP , Huhtala H , Lehtimäki T , Kähönen M , et al. Long-term outcome of intraventricular conduction delays in the general population. Ann Noninvasive Electrocardiol. (2021) ;26: (1):e12788. |
[42] | Pelargonio G , Dello Russo A , Sanna T , De Martino G , Bellocci F . Myotonic dystrophy and the heart. Heart. (2002) ;88: (6):665–70. |
[43] | Lubocka P , Sabiniewicz R . Respiratory Sinus Arrhythmia in Children-Predictable or Random? Front Cardiovasc Med. (2021) ;8: :643846. |
[44] | Singh N , Dorfmüller P , Shlobin OA , Ventetuolo CE . Group 3 Pulmonary Hypertension: From Bench to Bedside. Circ Res. (2022) ;130: (9):1404–22. |
[45] | Karlsen S , Dahlslett T , Grenne B , Sjøli B , Smiseth O , Edvardsen T , Brunvand H . Global longitudinal strain is a more reproducible measure of left ventricular function than ejection fraction regardless of echocardiographic training. Cardiovasc Ultrasound. (2019) ;17: (1):18. |
[46] | Shehta M , Rayan MM , Fahmy NA , Onsy A , Bastawy I . Global longitudinal strain detects subtle left ventricular systolic dysfunction in Duchenne muscular dystrophy patients and carriers. Egypt Heart J. (2021) ;73: (1):91. |
[47] | Lima MSM , Villarraga HR , Abduch MCD , Lima MF , Cruz C , Sbano JCN , et al. Global Longitudinal Strain or Left Ventricular Twist and Torsion? Which Correlates Best with Ejection Fraction? Arq Bras Cardiol. (2017) ;109: (1):23–9. |
[48] | Heidenreich PA BB , Aguilar D , Allen LA , Byun JJ , Colvin MM , Deswal A , Drazner MH , Dunlay SM , Evers LR , Fang JC , Fedson SE , Fonarow GC , Hayek SS , Hernandez AF , Khazanie P , Kittleson MM , Lee CS , Link MS , Milano CA , Nnacheta LC , Sandhu AT , Stevenson LW , Vardeny O , Vest AR , Yancy CW . 2022 AHA/ACC/HFSA guideline for the management of heart failure: A report of the American College of Cardiology/American Heart Association Joint Committee on Clinical Practice Guidelines . Circulatione . (2022) ;145: :e895–e1032. doi: 101161/CIR0000000000001063. |
[49] | McDonagh TA , Metra M , Adamo M , Gardner RS , Baumbach A , Böhm M , et al. ESC Guidelines for the diagnosis and treatment of acute and chronic heart failure. Eur Heart J. (2021) ;42: (36):3599–726. |
[50] | Busse A , Rajagopal R , Yücel S , Beller E , Öner A , Streckenbach F , et al. Cardiac MRI-Update 2020. Radiologe. (2020) ;60: (Suppl 1):33–40. |
[51] | Gardner BI , Bingham SE , Allen MR , Blatter DD , Anderson JL . Cardiac magnetic resonance versus transthoracic echocardiography for the assessment of cardiac volumes and regional function after myocardial infarction: An intrasubject comparison using simultaneous intrasubject recordings. Cardiovasc Ultrasound. (2009) ;7: :38. |
[52] | Lamacie MM , Warman-Chardon J , Crean AM , Florian A , Wahbi K . The Added Value of Cardiac Magnetic Resonance in Muscular Dystrophies. J Neuromuscul Dis. (2019) ;6: (4):389–99. |