Changes in 24(S)-Hydroxycholesterol Are Associated with Cognitive Performance in Early Huntington’s Disease: Data from the TRACK and ENROLL HD Cohorts
Abstract
Background:
There is evidence for dysregulated cholesterol homeostasis in Huntington’s disease (HD). The brain-specific cholesterol metabolite 24(S)-hydroxycholesterol (24(S)-OHC) is decreased in manifest HD. 24(S)-OHC is an endogenous positive allosteric modulator (PAM) of the N-methyl-D-aspartate (NMDA) receptor, suggesting lower 24(S)-OHC may contribute to NMDA receptor hypofunction in HD. We hypothesized changes in 24(S)-OHC would be associated with cognitive impairment in early HD.
Objective:
To determine the interactions between oxysterols (24(S)-OHC, 25-OHC, and 27-OHC) at the NMDA receptor, the plasma levels of these oxysterols, and how these levels relate to cognitive performance.
Methods:
An in vitro competition assay was used to evaluate interactions at the NMDA receptor, liquid chromatography coupled tandem mass spectrometry (LC-MS/MS) was used to measure plasma 24(S)-OHC, 25-OHC, and 27-OHC levels, and correlation analyses investigated their relationship to performance on cognitive endpoints in TRACK and ENROLL-HD (NCT01574053).
Results:
In vitro, 25-OHC and 27-OHC attenuated the PAM activity of 24(S)-OHC on the NMDA receptor. Lower plasma 24(S)-OHC levels and 24(S)/25-OHC ratios were detected in participants with early HD. Moderate and consistent associations were detected between plasma 24(S)/25-OHC ratio and performance on Stroop color naming, symbol digit modality, Trails A/B, and emotion recognition. Little association was observed between the ratio and psychiatric or motor endpoints, suggesting specificity for the relationship to cognitive performance.
Conclusions:
Our findings support growing evidence for dysregulated CNS cholesterol homeostasis in HD, demonstrate a relationship between changes in oxysterols and cognitive performance in HD, and propose that NMDA receptor hypofunction may contribute to cognitive impairment in HD.
INTRODUCTION
Cholesterol plays a critical role in many aspects of human and animal physiology, including being a core component of cell membranes and myelin (the majority of brain cholesterol is found within myelin sheaths), as well as acting as a precursor to produce bile acids, steroid hormones, and vitamin D.1 Peripheral cholesterol levels are determined by a balance of endogenously produced cholesterol via the mevalonate and HMG-COA-reductase pathways, along with dietary cholesterol. Conversely, central nervous system (CNS) cholesterol levels are governed by local synthesis of cholesterol in astrocytes, which is distributed throughout the CNS via transporters including APOE and ABCA1.2 Cholesterol homeostasis in the periphery is critical for maintaining many cellular processes, and dysregulation of cholesterol can lead to several pathological conditions including heart disease and atherosclerosis. In the CNS, dysregulation of cholesterol homeostasis has been implicated in a wide range of neurodegenerative disorders, including Huntington’s disease (HD)3 and Alzheimer’s disease.4
Oxidated cholesterol metabolites (oxysterols; including 24S-, 25-, and 27-hydroxycholesterol (HC)), have garnered increased interest in recent years as these metabolites have been shown to influence a wide range of physiological processes. Cholesterol is produced locally within the CNS and is unable to cross the blood-brain barrier. To maintain cholesterol homeostasis within the brain, cholesterol is converted by the brain specific enzyme 24-hydroxycholesterolase (CYP46A1) to 24(S)–OHC, which can cross the blood-brain barrier and enable the removal of cholesterol from the CNS to the periphery. This makes 24(S)-OHC a unique peripheral biomarker, as plasma levels of this metabolite reflect cholesterol dynamics within the CNS. While 24(S)-OHC is critically involved in maintaining cholesterol homeostasis in the CNS, it has also been implicated in cell viability, transcriptional regulation that includes nuclear liver X receptors (LXRs) α and β and SREBP, and may influence the activity of amyloid precursor protein.5 25-OHC, produced via the activity of cholesterol 25-hydroxylase (CH25H), is synthesized by macrophages, dendritic cells, and microglia in response to inflammatory stimuli.6 27-OHC, produced by CYP27A1 primarily in the liver, can serve as a ligand for steroid receptors including LXRα/β, has been implicated in the activity of estrogen receptors ER α/β, and may play a role in cancer, osteoporosis, and Alzheimer’s disease.7–9
Oxysterols have also been shown to directly influence the activity of N-methyl-D-aspartate (NMDA) receptors, which are ionotropic glutamatergic receptors that play a critical role in excitatoryneurotransmission, synaptic plasticity, and cognition. 24(S)-OHC was initially shown to be a positive allosteric modulator (PAM) of NMDA receptors, while 25-OHC and 27-OHC had little/no effect on NMDA receptor currents.10 Importantly, Linsenbardt et al. (2014) demonstrated that 25-OHC was able to compete with 24(S)-OHC at the NMDA receptor and decrease the PAM activity in a concentration-dependent manner.11 These findings raise the intriguing possibility that changes in 24(S)-OHC, via altered cholesterol metabolism in the CNS, along with alterations in 25-OHC mediated by inflammatory processes, may simultaneously influence NMDA receptor activity.
Several lines of pre-clinical and clinical evidence have emerged in the past decade that suggest HD is associated with dysregulated cholesterol metabolism/homeostasis in the brain. Key genes regulating cholesterol synthesis (HMGCoAred, Cyp51, and 7dhcred) were decreased in peripheral fibroblasts and post-mortem striatal/cortical tissue from HD patients compared to controls and fibroblasts from HD patients had decreased cholesterol synthesis.12 Multiple mouse models of HD (R6/2, YAC128, and Q175) have reductions in genes regulating cholesterol synthesis, cholesterol precursors (lanosterol, lathosterol), cholesterol content, cholesterol synthesis, and cholesterol efflux in the brain.12–15 Mutant Huntington (mHTT) has also been shown to interact with the sterol response element binding protein (SREBP2) that is critical for cholesterol synthesis when cellular cholesterol levels are low.3,12,16 Levels of 24(S)-OHC have also been shown to be decreased in post-mortem brain of late-stage HD patients,17 in plasma from early stage (HD stage 1–2) patients,18,19 and in all the primary rodent models of HD.15 These findings across in vitro models, preclinical models, and human participants, consistently support an important role for cholesterol dysfunction in HD.
Cognitive impairment is a prominent feature of HD that often emerges well before the motor diagnosis and can be the most burdensome aspect of HD for patients and their families.20 NMDA receptors are critical for synaptic plasticity, which is thought to underlie various forms of learning and memory.21 Although a myriad of pathological processes associated with HD likely contribute to cognitive impairment, the consistently observed decreases in 24(S)-OHC, and the established role for 24(S)-OHC in modulating NMDAR receptors, suggest that NMDA receptor hypofunction could play a role in the cognitive aspect of HD. However, to date, there have been limited reports of the association between levels of 24(S)-OHC and cognitive performance in HD19 and no reports examining the ratio of 24(S)-OHC to related oxysterols and cognitive performance in HD. Here, we initially demonstrate the ability of 25-OHC and 27-OHC to attenuate the positive allosteric modulation by 24(S)-OHC on the NMDA receptor in vitro, highlighting the potential importance of these oxysterols. Then, we describe findings from plasma samples taken from two independent registries: TRACK-HD22 and ENROLL-HD.23 TRACK-HD and ENROLL-HD were CHDI-sponsored studies that collected data and biospecimens from control, pre-manifest HD, and manifest HD participants, but differed in study duration, number of participants, and the specific components of the cognitive and clinical testing batteries. TRACK-HD was a small, longitudinal dataset that we used to generate the hypotheses that 24(S)-OHC and the ratios of 24(S)-OHC to 25-OHC and to 27-OHC in plasma would correlate with cognitive impairment in HD. We then tested these hypotheses in the larger dataset from ENROLL-HD. In both TRACK-HD and ENROLL-HD, we found a decrease in plasma 24(S)-OHC in early stage HD (HD stages 1– 2) and extended these observations to show moderate and consistent associations between levels of 24(S)-OHC and the ratio of 24(S)-OHC to other oxysterols with performance on several cognitive tasks, primarily in the executive function domains. These data support a hypothesis that changes in cholesterol homeostasis in HD may drive changes in NMDA receptor function and contribute to cognitive dysfunction in HD.
METHODS
Electrophysiology
The oxysterol competition assay was conducted similarly to previously described.24 Whole-cell patch-clamp recordings were performed using Syncropatch 384i automated platform (Nanion, Munich, Germany) with a HEK cell line expressing recombinant human GluN1/GluN2A NMDA receptor subunits (GenBank acc. Num. – GluN1: NP_015566.1; GluN2A: NP_001127880; RRID: CVCL_C9E4) under the control of a tetracycline inducible expression system. The recordings were performed using multi-hole high resistance chips (2– 3MΩ) at a holding potential of – 80 mV. Currents were leak corrected and sampled at 5 kHz. Extracellular solution contained (in mM) 140 NaCl, 4 KCl, 5 CaCl,2 10 HEPES, 5 glucose, pH 7.4 (adjusted with NaOH) and 0.2% DMSO and 0.01% Kolliphor EL. Internal solution contained (in mM) 120 CsF, 10 EGTA, 10 NaCl, 10 HEPES, 4 NaATP, 2 MgCl,2 pH 7.2 (adjusted with CsOH). A fast application protocol was used, in which 1 μM glutamate (subsaturating) and 100 μM glycine (saturating) were applied and rapidly removed from each well two times to demonstrate reproducibility of the NMDA receptor current. Next, the oxysterols 25- or 27-OHC were added to wells at either 0, 3, 30, 300 or 3000 nM for a 60-s preincubation, and then co-agonists were rapidly applied twice. Then, a solution containing either 25-OHC or 27-OHC and 24(S)-OHC was added to each well for a 60-s preincubation, and then NMDA receptor currents were evoked twice with co-agonists. 24(S)-OHC was evaluated at 1 nM to 3000 nM, half-log increments. Wells then underwent a washout period and co-agonist was applied once, and then finally a saturating concentration of agonist (100 μM glutamate/100 μM glycine) was applied to determine maximal current amplitude. There were between 2 and 8 wells per condition. Data collection and analysis were performed on PatchControl384/Datacontrol384 v.1.9 (Nanion, Munich, Germany).
Participants
Data used in this work were generously provided by the participants in the TRACK-HD and ENROLL-HD studies and made available by CHDI Foundation, Inc. TRACK-HD was a multi-center observational, longitudinal study HD with annual assessments of cognitive, motor, oculomotor, neuropsychiatric, and MRI measures over a period of 4 years in control, pre-manifest HD, and manifest HD participants.22 For a detailed description of each of the tasks analyzed in TRACK-HD, see.25 ENROLL-HD (NCT01574053) is an ongoing global clinical research platform designed to facilitate clinical research in HD. Core datasets are collected annually from all research participants as part of this multi-center longitudinal observational study. Data are monitored for quality and accuracy using a risk-based monitoring approach. All sites are required to obtain and maintain local ethical approval. For ENROLL-HD, plasma samples were available from a single timepoint, and our analyses represented a snapshot in time. For both TRACK-HD and ENROLL-HD, data is not available regarding the fed or fasting status for participants at the point of plasma sample collection. Data from both studies represent a matching plasma sample and clinical/cognitive evaluation (i.e., performance and plasma data were collected at the same time). We used TRACK-HD as a hypothesis-generating study and ENROLL-HD as a hypothesis-testing study.
For ENROLL-HD, analyzed data were from release PDS5, and special release SPS179-R2_ENR-EDC-2022-01-01. In ENROLL-HD, four participant groups were included: manifest/motor-manifest HD (CAG expansion≥36; clinical features that are diagnostic of HD); pre-manifest/pre-motor-manifest HD (CAG expansion≥36; not considered manifest by site investigator); genotype negative (first- or second-degree relatives of participants with HD who are negative for CAG expansion); and family controls (individuals not genetically related to participants with HD who are living alongside those with HD). In our analyses, we combined genotype-negative and family controls to create a single control group.
Plasma measurements
Plasma samples were analyzed by LC-MS/MS for concentration of 24S-, 25-, and 27-OHC. Assay calibrators and quality controls were made by spiking HC standards into charcoal-stripped human plasma as surrogate matrix. Stable isotope-labeled HCs were used as internal standards, and endogenous quality control (QC) samples were thawed and prepared alongside study specimens. The bioanalytical method underwent refinement over time as knowledge about oxysterol quantification evolved, both in terms of sample extraction and analysis. Our methods for plasma preparation evolved after we measured TRACK-HD samples. Samples from ENROLL-HD had an additional step where plasma hydrolysis using potassium hydroxide was introduced as the initial preparation step, followed by liquid-liquid extraction using methyl tert-butyl ether. The organic layer was nitrogen-dried and reconstituted in methanol prior to LC-MS/MS measurement.
Detection of 24S-, 25-, and 27-OHC was accomplished using a Sciex Qtrap 5500 or 6500 mass spectrometer (Sciex, Framingham, MA) coupled with an Eksigent Ekspert MicroLC200 microflow UPLC system (Waters, Milford, MA) in electrospray positive ion mode, monitoring for mass transitions of each analyte and its respective internal standard. The LC gradient was modified between TRACK-HD and ENROLL-HD samples to improve chromatographic resolution of the analytes. Sample concentrations were determined using the peak area ratio of analyte to internal standard and the least squares linear regression equation from the standard calibrationcurve.
Statistics
Electrophysiology
When analyzing the effect of 24(S)-OHC, 25-OHC, or 27-OHC alone, the percent current amplitude change was determined using the equation:
TRACK-HD and ENROLL-HD analyses
Samples below the lower limit of quantitation were not included in analyses of this analyte and were not used to calculate a ratio with that included the analyte. In TRACK-HD, year 1, nine participants had 25-OHC measurements below the level of quantitation (two control participants, five pre-manifest HD participants, and two manifest HD participants). In TRACK-HD, year 4, three participants had 25-OHC measurements below the level of quantitation (two control participants, one pre-manifest HD participant). In ENROLL-HD, 15 participants had unresolved 25-OHC measurements.
For both datasets, oxysterol concentrations that were 3-times the interquartile range were removed from the analysis. For TRACK-HD, outlier removal was done within year within oxysterol measurements; for ENROLL-HD, outlier removal was done within oxysterol measurements. In TRACK-HD, no outliers were detected in year 1, seven 25-OHC measurements and one 24(S)-OHC measurement were detected as outliers (three control participants, three pre- manifest HD participants, one manifest HD participant) in year 3, and one 27-OHC measurement was detected as an outlier (manifest HD participant) in year 4. In ENROLL-HD, two 27-OHC measurements were outliers (one control participant, one pre-manifest HD participant).
TRACK-HD is a longitudinal cohort, with data collected annually for 4 years, denoted as year 1, year 2, year 3, and year 4. Samples from year 2 were used to develop oxysterol measurement methods and were not used for statistical analysis. Years 1, 3, and 4 were used to analyze oxysterol concentrations in control, pre-manifest HD, and manifest HD groups, and the relationship of oxysterol concentrations to clinical assessments and performance on cognitive tests. Oxysterol measurements were compared between groups over years 1, 3, and 4 using a mixed model with repeated measure of year, random effect of subject, and Tukey post-hoc adjustment using R 4.2.1 with nlme and emmeans packages. ENROLL-HD participants had data and plasma samples collected at a single point in time. Oxysterol measurements were compared by one-way ANOVA with Tukey post-hoc comparison between all groups. Comparisons of interest between HD groups (pre-manifest HD vs manifest HD) were analyzed by t-test.
In TRACK-HD and ENROLL-HD, correlation analysis between oxysterol measurements and continuous variables of clinical features and measures of cognitive performance were analyzed by Pearson correlation using R 4.2.1 with psych package. Oxysterol concentrations were compared with mini-mental state exam (MMSE) performance, Unified Huntington Disease Rating Scale (UHDRS) Total Functional Capacity (TFC), and UHDRS Functional Assessment (FA) using binomial regression. Oxysterol concentrations were compared against UHDRS Total Motor Score (TMS) using zero-inflated Poisson model using R 4.2.1 with pscl package. In TRACK-HD, relationships were analyzed within year and p values were not adjusted for multiple comparisons. In ENROLL-HD, analyses of oxysterol measurements and clinical and cognitive measurements were adjusted for false-discovery rate (FDR). Across analyses, data were considered significant at a p < 0.05.
RESULTS
The presence of 25-OHC and 27-OHC attenuates the NMDA receptor PAM activity of 24(S)-OHC
It has been previously reported that 24(S)-OHC exhibits PAM activity at the NMDA receptor, while other oxysterol metabolites, such as 25-OHC and 27-OHC, do not significantly alter NMDA receptor activity.10 These results were replicated using an automated patch clamp platform. NMDA receptor currents were evoked with co-agonists glutamate (1 μM) and glycine (100 μM). 3 μM 24(S)HC significantly potentiated the agonist-evoked currents at the GluN1/GluN2A receptor subtype compared to 0.2% DMSO vehicle, while 3 μM 25-OHC or 27-OHC produced no modulatory effect (Kruskal-Wallis test, H(3) = 20.74; p < 0.0001; Fig. 1A). However, increasing concentrations of 25-OHC dose-dependently reduced the PAM activity of 24(S)-OHC. In the absence of 25-OHC, the 24(S)-OHC EC50 was 22 nM (95% CI 16-30 nM; n = 2– 6 wells per concentration), while in the presence of 3 μM 25-OHC, the EC50 was 1900 nM (170– 21000 nM; n = 5– 8 wells per concentration, Fig. 1B, C). While less robust, 27-OHC also dose-dependently reduced 24(S)-OHC PAM activity. In the absence of 27-OHC, the 24(S)-OHC EC50 was 9.3 nM (2.8– 31 nM; n = 4– 8 wells per concentration), while in the presence of 3 μM 27-OHC, the EC50 was 53 nM (13– 210 nM; n = 4– 7 wells per concentration, Fig. 1D). These results demonstrate a complex oxysterol interaction at GluN1/GluN2A NMDA receptors, in which 25-OHC and 27-OHC, reduce the PAM activity of 24(S)-OHC, while alone having no direct modulatory activity.
Fig. 1
The NMDA receptor PAM activity of 24(S)-OHC was negatively impacted by the presence of the oxysterols 25-OHC and 27-OHC. A) Summary of effect of 0.2% DMSO (n = 13 wells over two plates), 3 μM 24(S)-OHC (n = 6 wells over two plates), 3 μM 25-OHC (n = 4 wells), and 3 μM 27-OHC (n = 5 wells) on NMDA receptor currents at the GluN1/GluN2A subtype evoked by 1 μM glutamate/ 100 μM glycine (Dunn’s multiple comparisons test, **p < 0.01). B) Representative traces of NMDA receptor currents at GluN1/GluN2A evoked by a fast application of sub-saturating glutamate (1 μM) and saturating glycine (100 μM) during baseline (black), following the addition of 24(S)-OHC (green) or 25-OHC (orange), or with the addition of both 24(S)-OHC and 25-OHC (purple). C,D) Concentration-response relationship of 24(S)-OHC activity (1 nM to 3000 nM, half log increments) at the GluN1/GluN2A NMDA receptor subtype in the absence or presence of increasing concentrations of 25-OHC (C) or 27-OHC (D).
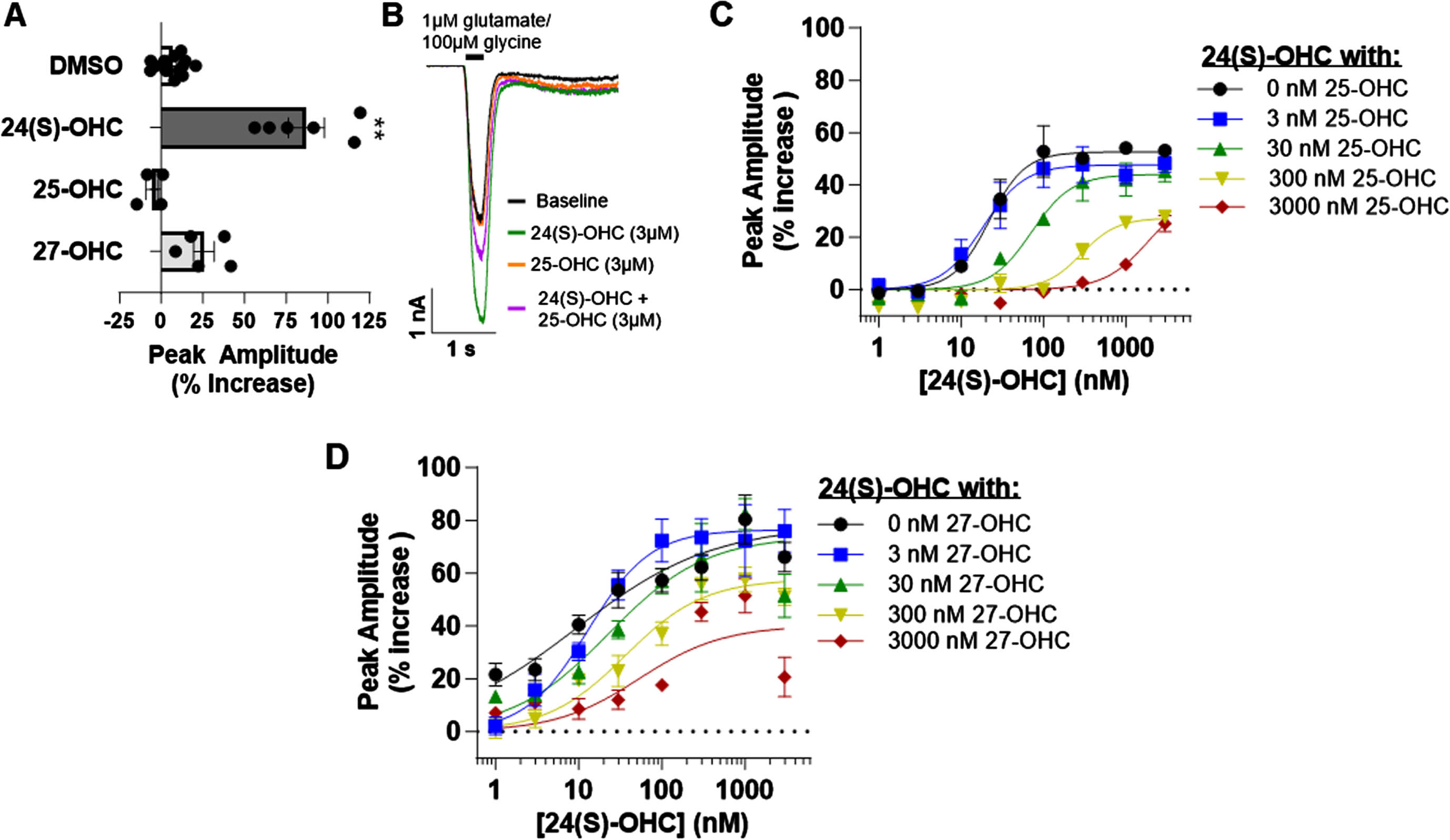
Participant characteristics
In the hypothesis-generating cohort, TRACK-HD, plasma samples were available from 44 control, 51 pre-manifest HD, and 45 manifest HD participants; all groups were ∼50% female (Table 1). CAG repeat number was comparable between pre-manifest HD and manifest HD participants. In the hypothesis-testing cohort, ENROLL-HD, plasma samples were available from 121 control (72 family control, 49 genotype negative control), 123 pre-manifest HD, and 149 manifest HD participants. Across both studies, age was roughly comparable (see Table 1).
Table 1
Demographics of TRACK-HD registry cohort, which was used as a hypothesis-generating cohort, and ENROLL-HD registry cohort, which was used as a hypothesis-testing cohort
TRACK-HD | ENROLL-HD | |||||||
Control | Pre-manifest HD | Manifest HD | Family control | Genotype negative control | Combined controls | Pre-manifest HD | Manifest HD | |
N | 44 | 51 | 45 | 72 | 49 | 121 | 123 | 149 |
Age (y) | 45.3±10.3 | 42.2±9.4 | 49.1±9.5 | 56.7±12.7 | 46.7±12.9 | 52.6±13.7 | 42.5±11.6 | 52.3±12.2 |
Age at HD diagnosis (y) | – | – | – | n/a | n/a | n/a | 43.7±7.2 | 47.5±11.8 |
CAG | n/a | 43±2.4 | 43.5±2.4 | 19.1±3.2 | 20.4±3.9 | 19.6±3.5 | 42.1±2.8 | 43.8±3.8 |
CAG-Age-Product (CAP) | n/a | 81.7±8.3 | 99.7±13.7 | n/a | n/a | n/a | 75.8±14.8 | 106±17.2 |
Sex M:F (% F) | 21 : 23 (48% ) | 25 : 26 (51% ) | 23 : 22 (49% ) | 32 : 40 (56% ) | 19 : 30 (61% ) | 51 : 70 (58% ) | 37 : 86 (70% ) | 72 : 77 (52% ) |
Years of Education n (% total) | ||||||||
Primary | – | – | – | 3 | 3 | 6 (5.0% ) | 2 (1.6% ) | 13 (8.7% ) |
Lower secondary | – | – | – | 5 | 6 | 11 (9.0% ) | 6 (4.9% ) | 17 (11.4% ) |
Upper secondary | – | – | – | 14 | 12 | 26 (21.5% ) | 17 (13.8% ) | 34 (22.8% ) |
Occupational training | – | – | – | 18 | 8 | 26 (21.5% ) | 39 (31.7% ) | 32 (21.5% ) |
Bachelors | – | – | – | 25 | 17 | 42 (34.7% ) | 44 (35.8% ) | 42 (28.2% ) |
PhD/Doctorate | – | – | – | 6 | 2 | 8 (6.6% ) | 15 (12.2% ) | 10 (6.7% ) |
NA | – | – | – | 1 | 1 | 2 (1.7% ) | 0 (0% ) | 1 (0.7% ) |
TRACK-HD is a longitudinal study and demographics are from year 1, and the same participants were used for years 1, 3, and 4 in analyses. ENROLL-HD had two control groups: family controls who lived with HD participants and genotype negative controls, which were pooled for subsequent analyses as combined controls. CAG, number of CAG repeats; CAP, CAG-age-product.
Levels of 24(S)-OHC and the ratio of 24(S)-OHC to 25-OHC and 27-OHC are decreased in manifest HD
In TRACK-HD, individuals had plasma sample collection and cognitive and clinical assessments at annual visits over four years. To establish oxysterol analytical techniques, year 2 of the TRACK-HD registry was used for initial method development. Numerically, manifest HD participants had lower mean 24(S)-OHC levels compared to controls or pre-manifest HD, with no obvious differences detected in 25-OHC and 27-OHC (Supplementary Figure 1). This initial work was consistent with prior reports and provided confidence in the methodology to measure oxysterols in the remaining years of TRACK-HD.
The hypothesis-generating analyses focused on TRACK-HD samples and data from years 1, 3, and 4 and tested differences in oxysterol concentrations and ratios in a repeated-measures fashion to assess the impact of group (control, pre-manifest HD, and manifest HD) and year (1, 3, 4). In the TRACK-HD registry, mean concentrations of 24(S)-OHC and ratios of 24(S)/25-OHC and 24(S)/27-OHC were significantly lower in manifest HD participants compared to controls (Fig. 2A-C, Table 2). Between pre-manifest HD and manifest HD, 24(S)-OHC concentration and 24(S) ratios were numerically lower, but only reached statistical significance in the case of 24(S)/25-OHC. Group differences were not detected for 25-OHC, 27-OHC, or the 25/27-OHC ratio (Table 2, Supplementary Figure 2A-C).
Fig. 2
24(S)-OHC and 24(S)-OHC ratios were altered during manifest Huntington’s disease in independent registry cohorts. A-C) TRACK-HD cohort, which was used as a hypothesis-generating cohort (see Table 2 sample size); D-F) ENROLL-HD cohort, which was used as a hypothesis-testing cohort. Groups in both cohorts consisted of controls, pre-manifest HD (PreHD), and manifest HD (ManHD) participants. A and D) 24(S)-OHC concentrations; B, E) 24(S)/25-OHC ratio; C and F) 24(S)/27-OHC ratio. TRACK-HD data were compared by mixed model with repeated measure (year) with Tukey post-hoc test and shown as mean±standard error. ENROLL-HD data were compared by 1-way ANOVA with Tukey post hoc between all groups and shown as box and whiskers that span 5-95% of the data, with line at median and cross at the mean, and individual circles are shown for participants outside the 5-95% span. * p < 0.05, ** p < 0.01, *** p < 0.001, **** p < 0.0001.
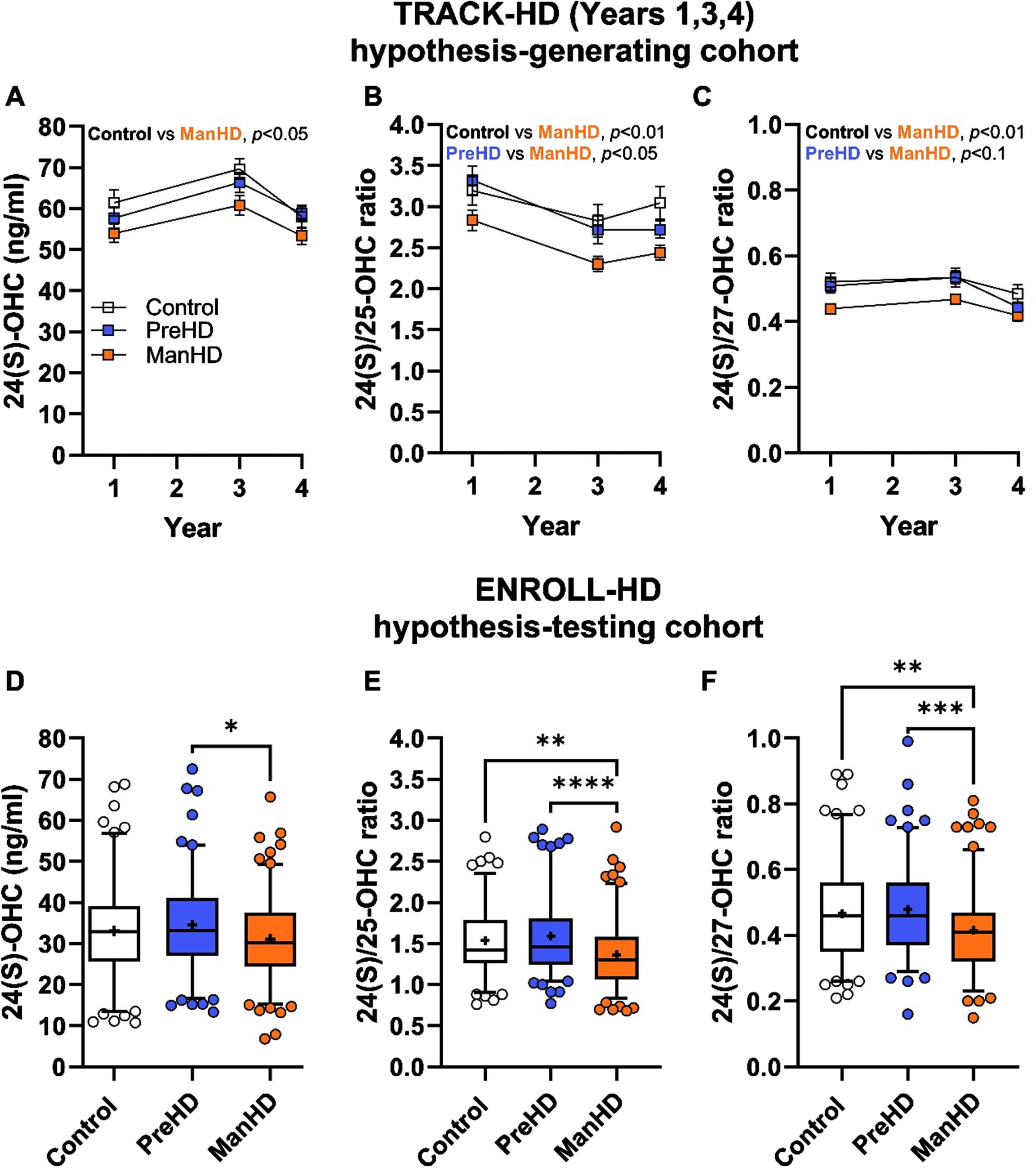
Table 2
Oxysterol concentration and ratios in TRACK-HD registry. Oxysterol concentrations and oxysterol ratios from TRACK-HD participants (control [Cntrl], pre-manifest HD [PreHD], and manifest HD [ManHD] participants) across three years (year 1 [Y1], year 3[Y3], and year 4[Y4]). Data are presented as LS means (95% confidence interval) with the number of samples measured within each group and year. In year 1, nine participants had 25-OHC measurements below the level of quantitation; in year 3 seven 25-OHC measurements and one 24(S)-OHC measurement were detected as outliers and removed; in year 4 three participants had 25-OHC measurements below the level of quantitation and one 27-OHC measurement was detected as an outlier. Data were compared by mixed model repeated measure (year) with Tukey post-hoc adjustment on p-values. No analytes had an interaction with group by year. Pairwise comparisons for group (control: Cntl, PreHD: pre-manifest HD, ManHD: manifest HD) and year (year 1: Y1, year 3: Y3, year 4: Y4) are shown, significant p-values are bolded. Extreme outliers were removed (see methods) from seven 25-OHC measurements and one 24(S)-OHC measurement (three control participants, three pre- manifest HD participants, one manifest HD participant) in year 3, and one 27-OHC measurement (manifest HD participant) in year 4
Year 1 | Year 3 | Year 4 | |||||||
Cntl | PreHD | ManHD | Cntl | PreHD | ManHD | Cntl | PreHD | ManHD | |
24(S)-OHC (ng/ml) | 61.4 (56.4– 66.4) n = 44 | 57.7 (53.0– 62.4) n = 51 | 54.0 (49.0– 58.9) n = 45 | 69.6 (64.6– 74.7) n = 44 | 66.6 (61.9– 71.3) n = 50 | 60.8 (55.8– 65.8) n = 45 | 58.1 (53.0– 63.1) n = 44 | 58.8 (54.2– 63.5) n = 51 | 53.6 (48.4– 58.4) n = 45 |
24(S)/25-OHC ratio | 3.2 (2.9– 3.5) n = 42 | 3.3 (3.0– 3.6) n = 46 | 2.8 (2.5– 3.1) n = 43 | 2.8 (2.5– 3.1) n = 41 | 2.7 (2.4– 3.0) n = 48 | 2.3 (2.0– 2.6) n = 44 | 3.1 (2.8– 3.4) n = 42 | 2.7 (2.5– 3.0) n = 50 | 2.4 (2.1– 2.7) n = 45 |
24(S)/27-OHC ratio | 0.52 (0.48– 0.57) n = 44 | 0.51 (0.47– 0.55) n = 51 | 0.44 (0.40– 0.48) n = 45 | 0.53 (0.49– 0.58) n = 44 | 0.54 (0.50– 0.58) n = 50 | 0.47 (0.42– 0.51) n = 45 | 0.48 (0.44– 0.53) n = 44 | 0.44 (0.40– 0.49) n = 51 | 0.42 (0.37– 0.46) n = 44 |
25-OHC (ng/ml) | 21.3 (18.8– 23.7) n = 42 | 20.1 (17.8– 22.4) n = 46 | 20.8 (18.4– 23.2) n = 43 | 27.1 (24.7– 29.6) n = 41 | 26.5 (24.2– 28.7) n = 48 | 28.3 (26.0– 30.7) n = 44 | 21.8 (19.4– 24.2) n = 42 | 22.9 (20.7– 25.2) n = 50 | 23.2 (20.9– 25.6) n = 45 |
27-OHC (ng/ml) | 123.0 (110.5– 135.4) n = 44 | 123.0 (111.5– 134.6) n = 51 | 129.2 (116.9– 141.5) n = 45 | 143.0 (130.6– 155.5) n = 44 | 130.5 (118.9– 142.0) n = 51 | 134.4 (122.1– 146.7) n = 45 | 130.4 (117.9– 142.8) n = 44 | 137.6 (126.1– 149.2) n = 51 | 133.6 (121.3– 146.1) n = 44 |
25/27-OHC ratio | 0.17 (0.16– 0.19) n = 42 | 0.16 (0.14– 0.17) n = 46 | 0.16 (0.14– 0.18) n = 43 | 0.20 (0.18– 0.21) n = 41 | 0.21 (0.20– 0.23) n = 48 | 0.21 (0.19– 0.23) n = 44 | 0.16 (0.15– 0.18) n = 42 | 0.17 (0.15– 0.18) n = 50 | 0.17 (0.16– 0.19) n = 45 |
Mixed model p values | Group pairwise comparison, p values | Year pairwise comparisons, p values | |||||||
Group | Year | Group*Year | Cntl-PreHD | Cntl-ManHD | PreHD-ManHD | Y1-Y3 | Y1-Y4 | Y3-Y4 | |
24(S)-OHC | 0.026 | <0.001 | 0.777 | 0.715 | 0.024 | 0.124 | <0.001 | 0.849 | <0.001 |
24(S)/25-OHC | 0.003 | <0.001 | 0.561 | 0.825 | 0.005 | 0.020 | <0.001 | 0.002 | 0.437 |
24(S)/27-OHC | 0.027 | <0.001 | 0.252 | 0.819 | 0.028 | 0.096 | 0.054 | <0.001 | <0.001 |
25-OHC | 0.607 | <0.001 | 0.818 | 0.979 | 0.745 | 0.606 | <0.001 | 0.113 | <0.001 |
27-OHC | 0.942 | 0.015 | 0.251 | 0.961 | 0.999 | 0.945 | 0.017 | 0.068 | 0.859 |
25/27-OHC | 0.860 | <0.001 | 0.206 | 0.983 | 0.871 | 0.938 | <0.001 | 0.843 | <0.001 |
Measurement of oxysterols and oxysterol ratios in the ENROLL-HD registry was conducted to replicate the results from TRACK-HD and extend them into a larger cohort of participants. ENROLL-HD replicated the findings in TRACK-HD, with decreased 24(S)-OHC plasma levels detected in manifest HD (Fig. 2D), as well as robust decreases in 24(S)/25-OHC ratio (Fig. 2E) and 24(S)/27-OHC ratio (Fig. 2F). Moreover, differences in 25-OHC, 27-OHC, and the 25/27-OHC ratio were not different between groups in ENROLL-HD (Supplementary Figure 2D–F).
24(S)-OHC to 25-OHC ratio is correlated with cognitive performance
At annual visits, TRACK-HD participants were subjected to a battery of tests evaluating cognitive performance, neuropsychiatric symptoms, motor symptoms, and brain volume measurements. Pearson correlation analysis was performed to test whether concentrations of 24(S)-OHC, 25-OHC, 27-OHC, or ratios of 24(S)/25-OHC, 24(S)/27-OHC, and 25/27-OHC suggested relationships between oxysterol metabolism and these assessments. Given the exploratory nature of the TRACK-HD hypothesis-generating cohort, adjustments for multiple comparisons were not made to p values.
Across all groups in TRACK-HD, cognitive performance was significantly correlated with 24(S)/25-OHC (Fig. 3A; Supplementary Table 1). Particularly moderate and consistent correlations were observed with performance on emotion recognition test (Fig. 3B) and Stroop word reading test (Fig. 3C). In both cognitive tests, increased statistical significance in later years appears to be driven by better test performance as opposed to changes in 24(S)/25-OHC. Similar results were observed when investigating 24(S)-OHC concentrations and 24(S)/27-OHC ratio (Supplementary Table 1), though these plasma measures were not as robust as 24(S)/25-OHC. Notably, 25-OHC and 27-OHC did not significantly correlate with cognitive endpoints. The ratio of 24(S)/25-OHC to clinician-measured UHDRS TFC approached or achieved statistical significance in years 1, 3, and 4, but did not relate to UHDRS TMS (Supplementary Figure 3A, Supplementary Table 2). No clear relationship was observed between neuropsychiatric measures on the Problem Behaviors Assessment or individual motor tests (i.e., paced tapping, circle tracing, Supplementary Figure 3B), suggesting the relationship of 24(S)/25-OHC was specific to cognitive endpoints.
Fig. 3
24(S)/25-OHC ratio correlated with better performance on cognitive tests in the hypothesis-generating TRACK-HD. A) Log-transformed p values from correlation analysis of cognitive tests and 24(S)/25-OHC ratios over years 1, 3, and 4 across all TRACK-HD participants. In year 4, Trail A, Trail B, and smell test were not performed. B) Performance on emotion recognition test was positively correlated to 24(S)/25-OHC ratio. C) Performance on Stroop word reading test was positively correlated with 24(S)/25-OHC ratio. Correlation analyses were performed across control, pre-manifest HD, and manifest HD participants in years 1 (orange), 3 (blue), and 4 (pink) and are shown with 95% confidence interval (dashed lines).
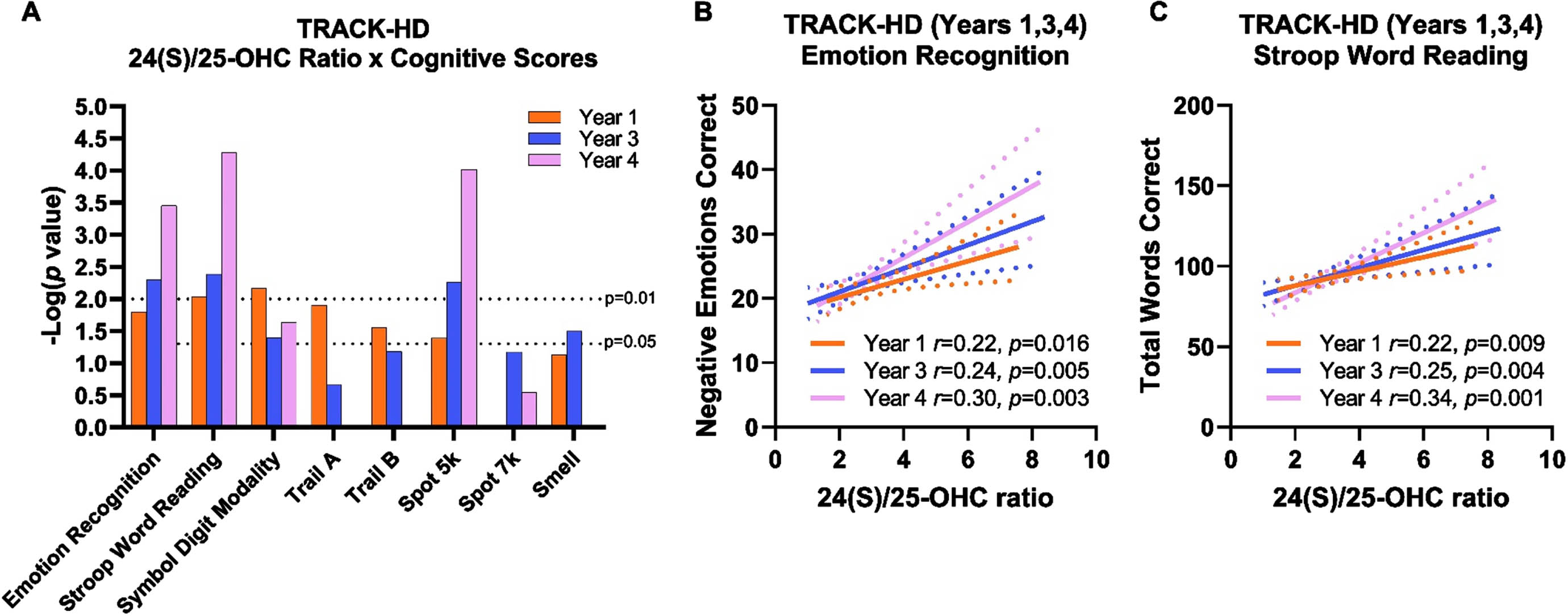
ENROLL-HD is a rich dataset that includes assessments that are comparable, but not identical, to TRACK-HD. We tested whether the relationships between 24(S)/25-OHC observed in TRACK-HD would be replicated in the larger ENROLL-HD registry. Pearson correlation analysis performed across all groups highlighted modest, but consistent, significant relationships between performance on cognitive tests and the ratio of 24(S)-OHC to 25-OHC (Fig. 4A, B). The concentration of 24(S)-OHC alone and the ratio of 24(S)-OHC to 27-OHC had similar trends in significance to 24(S)/25-OHC, albeit with lower r values and fewer comparisons reaching statistical significance. No significant correlations were observed with the concentrations of 25-OHC and 27-OHC (Supplementary Table 3). Following an FDR adjustment, the correlations between 24(S)/25-OHC and performance on the cognitive battery remained robustly, significantly related. To a lesser extent, performance on cognitive tests and plasma 24(S)-OHC and 24(S)/27-OHC were significantly related (Supplementary Figure 4, Supplementary Table 3). Comparable to the TRACK-HD registry, significant relationships were not observed between 25-OHC and 27-OHC to cognitive tests in ENROLL-HD (Supplementary Figure 4, Supplementary Table 3).
Fig. 4
24(S)/25-OHC ratio correlated with better performance on cognitive tests in the hypothesis-testing ENROLL-HD. A) Pearson correlation analysis between 24(S)-OHC concentration, 24(S)/25-OHC ratio, 24(S)/27-OHC ratio, 25-OHC concentration, and 27-OHC concentration to cognitive tests, * p < 0.05 (prior to FDR adjustment). B) Log-transformed p values from cognitive tests and plasma 24(S)/25-OHC ratio; binomial regression (MMSE) and Pearson correlations (all other tests). C) Symbol digit modality was decreased in manifest HD (ManHD) compared to controls (Con) and pre-manifest HD (PreHD) participants. D) Performance on symbol digit modality test was positively correlated with 24(S)/25-OHC ratio. E) Stroop color naming performance was decreased in manifest HD (ManHD) compared to controls (Con) and pre-manifest HD (PreHD) participants. F) Performance on Stroop color naming test was positively correlated with 24(S)/25-OHC ratio. For A, B, D and F, correlation analysis was performed across control (white, Con), pre-manifest HD (blue, PreHD), and manifest HD (orange, ManHD) participants, reported p-value is FDR-adjusted. Correlations are shown with 95% confidence interval (dashed lines). For C and E, box and whiskers span 5– 95% of the data, with line at median and cross at the mean, and individual circles are shown for participants outside the 5-95% span; data were compared by 1-way ANOVA with Tukey post hoc between all groups. ns: not significant, **** p < 0.0001.
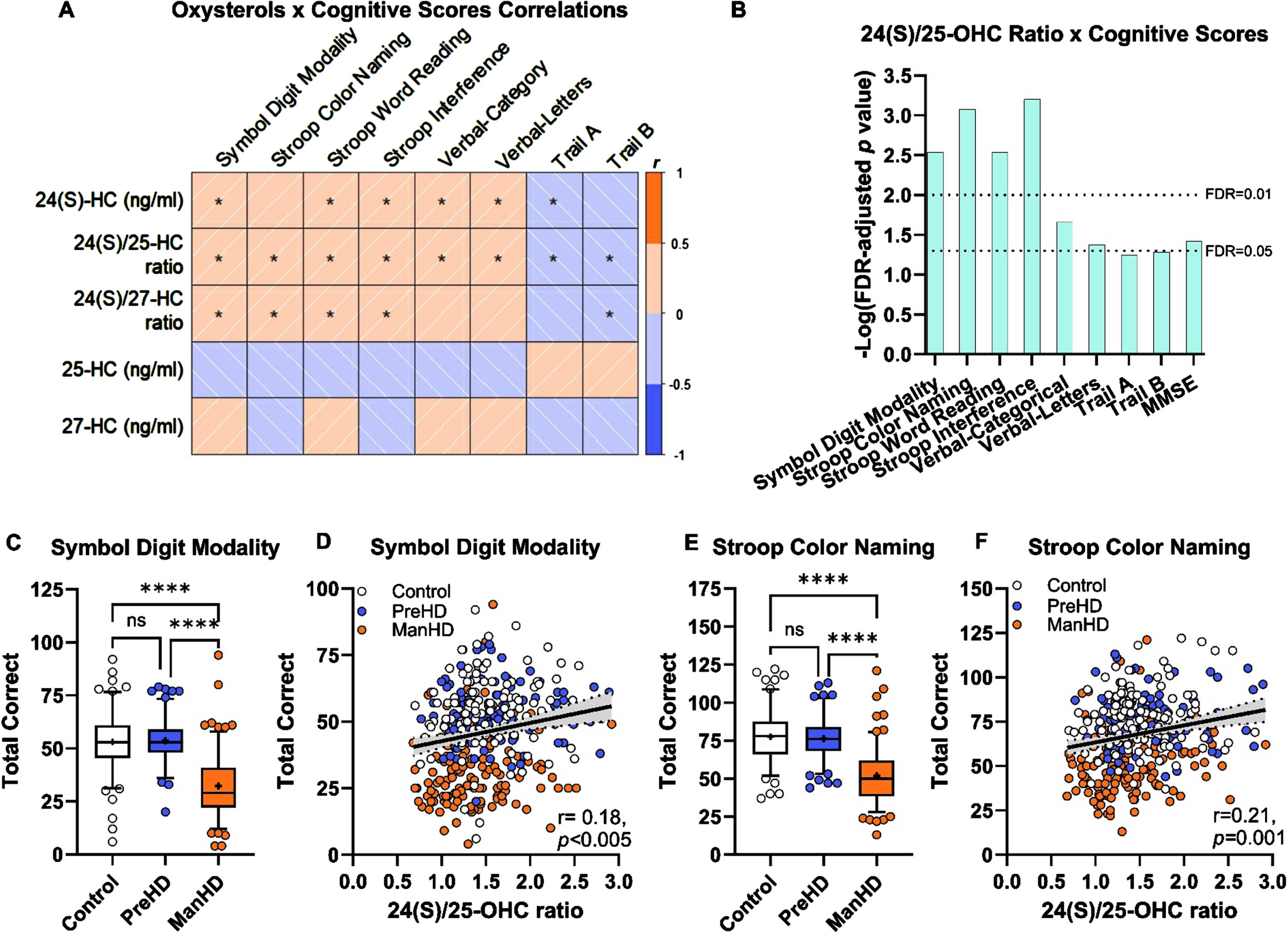
Symbol digit modality and Stroop color naming were selected to illustrate the relationship between 24(S)/25-OHC ratio and cognitive performance. In both symbol digit modality (Fig. 4C) and Stroop color naming (Fig. 4E) tests, control and pre-manifest HD individuals performed similarly, while individuals with manifest HD performed worse. Across the entire registry, increased 24(S)/25-OHC correlated to better performance on symbol digit modality (Fig. 4D) and Stroop color naming (Fig. 4F). In the ENROLL-HD hypothesis-testing cohort, 24(S)/25-OHC ratio correlates with better cognitive performance and this data replicated the findings in the TRACK-HD hypothesis-generating registry and underscored the specificity of 24(S)-OHC to cognitive performance, as compared to 25- or 27-OHC.
24(S)-OHC to 25-OHC ratios: Relationship to UHDRS
To investigate the relationships between oxysterols and the clinical features of HD, participants in pre-manifest HD and manifest HD groups from the ENROLL-HD registry were analyzed. Evaluations of UHDRS TFC, UHDRS FA, CAG-age-product (CAP), and UHDRS TMS were associated with 24(S)/25-OHC (Fig. 5A, Supplementary Table 4). To a lesser extent, these clinical features of HD were also related to 24(S)-OHC concentrations and the ratio of 24(S)-OHC to 27-OHC (Supplementary Figure 5). Individuals with lower TFC scores (indicating greater impairment) had lower 24(S)/25-OHC ratios (Fig. 5B). As anticipated, participants with TFC < 13 were overwhelmingly manifest HD participants. At a TFC of 13 (fully functioning), both pre-manifest and manifest HD were represented. When comparing individuals at TFC = 13, the 24(S)/25-OHC ratio was significantly lower in manifest HD individuals relative to pre-manifest participants (Fig. 5C), suggesting that a decrease in 24(S)/25-OHC ratio is detectable prior to a decrease in TFC score. A similar analysis of 24(S)/25-OHC ratio and FA score revealed that 24(S)/25-OHC was lower in individuals with decreasing function (Fig. 5D). Functional assessment scores below 25 were also predominantly observed in manifest HD participants. When comparing pre-manifest and manifest HD at intact function (score = 25), manifest HD participants again had significantly lower 24(S)/25-OHC ratio compared to pre-manifest HD participants (Fig. 5E).
Fig. 5
24(S)/25-OHC ratio correlated with better function on UHDRS scores and lower CAP scores in pre-manifest and manifest participants in the ENROLL-HD. A) Log-transformed p values from analyses of UHDRS and CAP scores with plasma 24(S)/25-OHC ratios; binomial regression (TFC, Functional), Pearson correlation (CAP), zero-inflated Poisson model (Motor score), with p values were adjusted for false-discovery rate (FDR). B) Total functional capacity (TFC) score and 24(S)/25-OHC ratio. C) 24(S)/25-OHC ratio in pre-manifest (PreHD) and manifest HD (ManHD) participants at TFC of 13. D) Functional assessment score and 24(S)/25-OHC ratio. E) 24(S)/25 ratio in pre-manifest (PreHD) and manifest HD (ManHD) at functional score of 25. F) Correlation of CAP with 24(S)/25-OHC ratio across pre-manifest HD (blue, PreHD), and manifest HD (orange, ManHD) participants, correlation is shown with 95% confidence interval (dashed lines), reported p value were FDR-adjusted. For C and E) unpaired t-test ** p < 0.01.
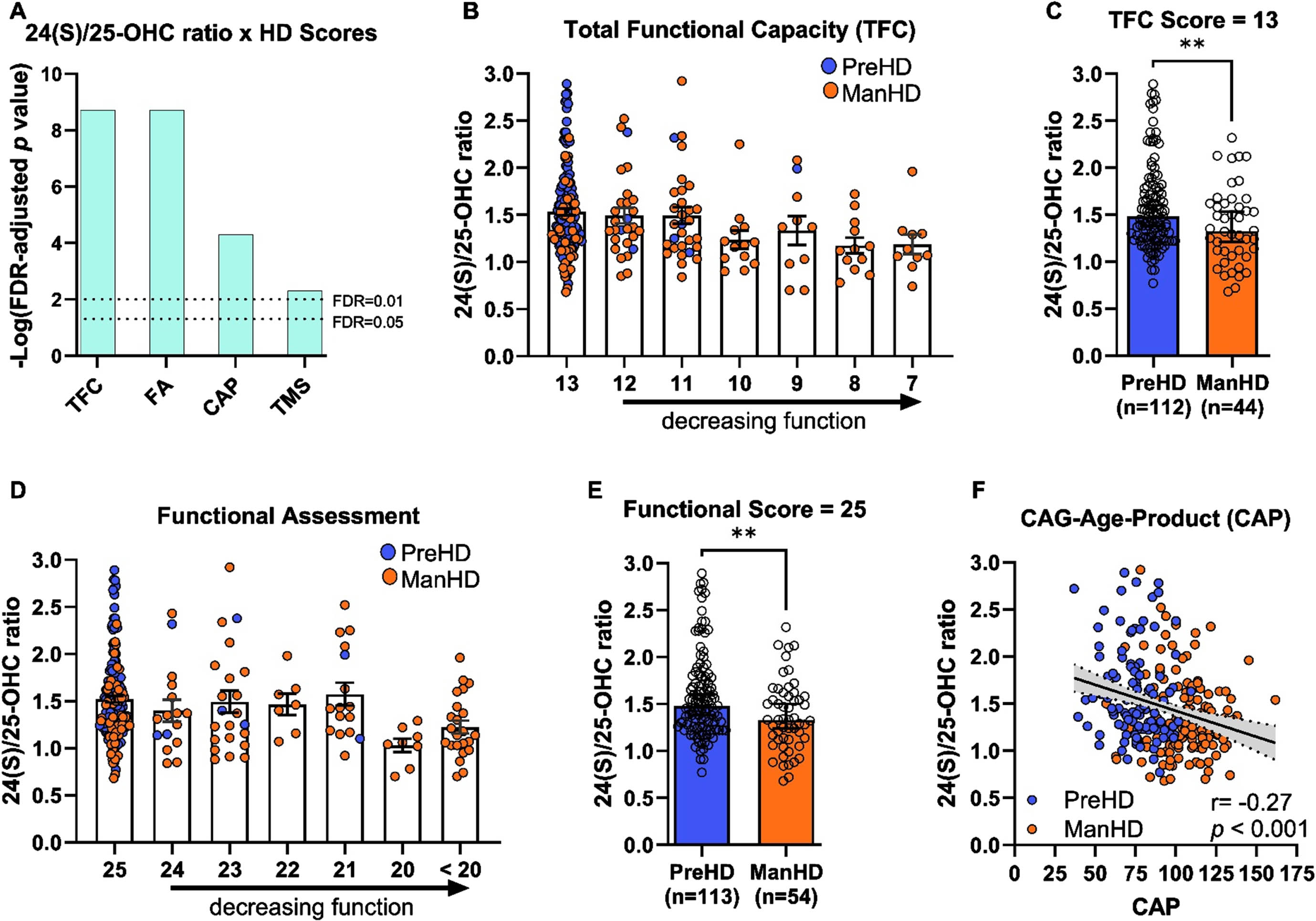
While relationships between 24(S)/25-OHC ratio and TMS were not clearly detected in the TRACK-HD registry (Supplementary Figure 3, Supplementary Table 2), the ENROLL-HD registry indicated a relationship between overall motor score and 24(S) ratios (Fig. 5A, Supplementary Figure 6), albeit with less statistical significance compared to other HD scores. The zero-inflated Poisson model used to assess the relationship can be interpreted as the probability that the participant will have a score of ‘0’ on the total motor score test. Participants with pre-manifest HD had little or no impaired motor function (i.e., lower TMS scores), while those with manifest HD had impaired motor scores (Supplementary Figure 6A). When pre-manifest HD and manifest HD participants with lower TMS scores (1–10) were compared, there was no difference between the two groups (Supplementary Figure 6B). Thus, the significance detected between motor score and 24(S)/25-OHC ratio is likely reflective of group-driven differences, with pre-manifest HD participants contributing to the zero scores and manifest HD participants having motor scores. To probe into this relationship further, the relationship between oxysterols and oxysterol ratios was compared to motor sub scores (Supplementary Figure 7C) and these data did not support a robust relationship between 24(S)-OHC or its ratios to performance on motor tests.
Total motor score, TFC, and FA scales rely on clinician assessment for HD progression, while CAG-age-product (CAP) is a calculated score to estimate transition from pre-manifest HD to manifest HD. CAP incorporates genetic information and the individual’s age, whereby transition from pre-manifest HD to manifest HD occurs at a CAP score of 100. CAP scores were negatively correlated with 24(S)/25-OHC ratio, suggesting that lower 24(S)/25-OHC ratios occur in participants closer to transition to manifest HD or that have already transitioned to manifest HD (Fig. 5F). Collectively, HD progression, as assessed by CAP and clinically by UHDRS scoring, is associated with decreased 24(S)/25-OHC ratio and this association is strongest in measures of function (TFC and functional assessment). Moreover, these data suggests that decreases in 24(S)/25-OHC ratio are observed in manifest HD individuals prior to decreasing function as measured by TFC and functional assessment scales.
Decreases in 24(S)/25-OHC are specific to cognitive and functional assessments
To test whether the relationship of 24(S)/25-OHC was specific to cognitive and UHDRS assessments, oxysterol ratios were compared with short form health survey (SF-12) self-scoring in ENROLL-HD. Manifest HD participants had lower mental and physical component scores on SF-12 compared to control participants (Fig. 6A, C). Significant correlations were not detected between 24(S)/25-OHC and SF-12 mental component (Fig. 6B), SF-12 physical component (Fig. 6D) or SF-12 component scores (Supplementary Figure 7). These data further support the specificity of 24(S)/25-OHC as a biomarker for changes in performance on cognitive tests in participants in the ENROLL-HD registry.
Fig. 6
24(S)/25-OHC ratio did not correlate with SF-12 scores in ENROLL-HD. A) SF-12 mental component scores were lower in manifest HD participants compared to controls. B) Correlation between SF-12 mental component and 24(S)/25-OHC ratio was not significant. C) SF-12 physical component was lower in manifest HD (ManHD) compared to control (Con) and pre-manifest HD (PreHD) participants. D) Correlation between SF-12 physical component and 24(S)/25-OHC ratio was not significant. For A and D, box and whiskers span 5-95% of the data, with line at median and cross at the mean, and individual circles are shown for participants outside the 5-95% span; data were compared by 1-way ANOVA with Tukey post hoc between all groups. ns: not significant, * p < 0.05, ** p < 0.01, *** p < 0.001, **** p < 0.0001 For D and F, correlation analysis was performed across control (white, Con), pre-manifest HD (blue, PreHD), and manifest HD (orange, ManHD) participants.
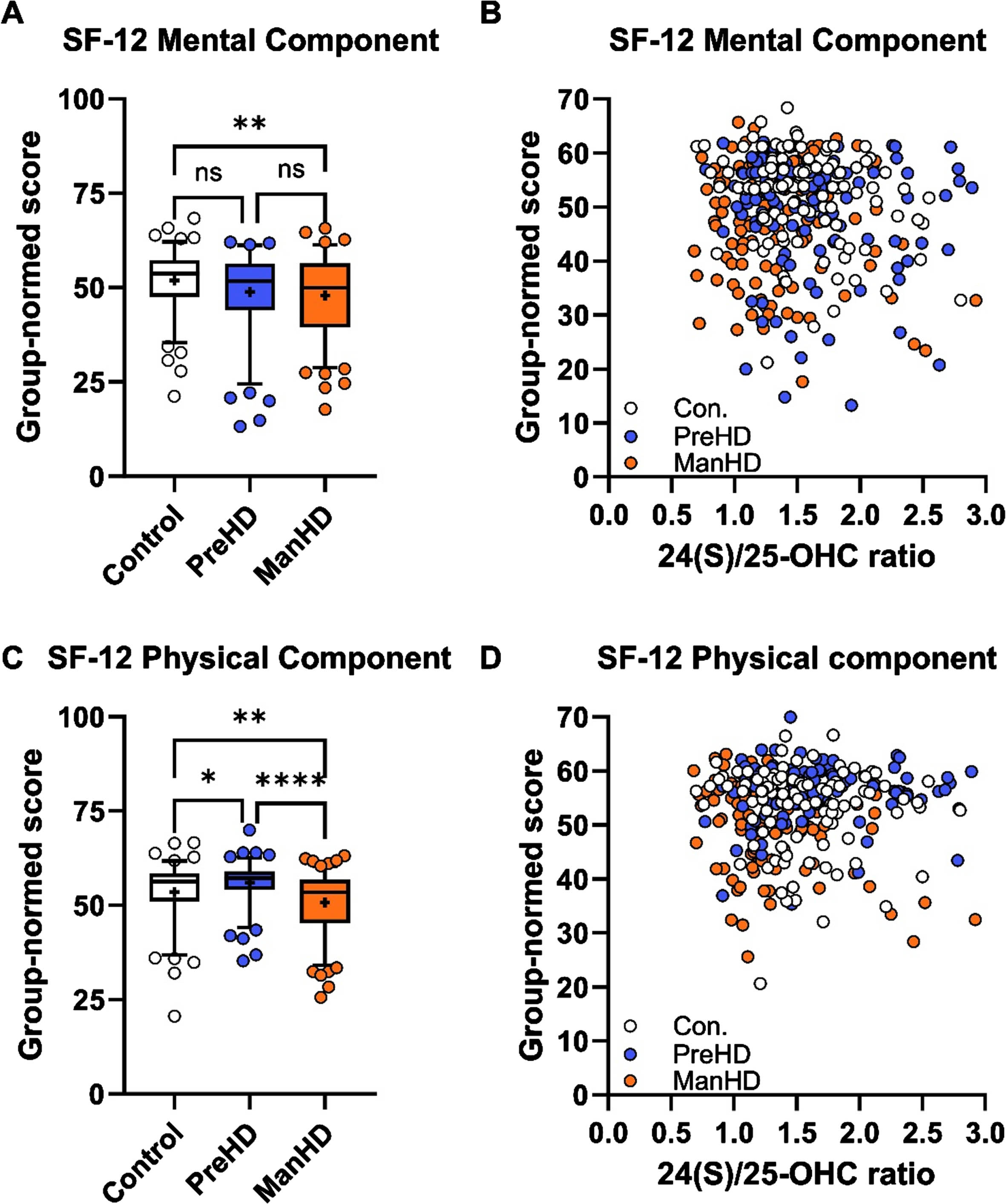
DISCUSSION
In our current study, we demonstrate that 24(S)-OHC is a NMDA receptor PAM, consistent with previously published results.10,11 We also show that 25-OHC and 27-OHC have no NMDA receptor PAM activity alone but can potently compete with, and decrease the PAM activity of, 24(S)-OHC. This finding underscores the importance of characterizing a complete panel of oxysterols when probing for the potential association of these metabolites with NMDA receptor-related behaviors. For example, lower levels of 24(S)-OHC may suggest lower NMDA receptor tone in certain populations but increased levels of 25-OHC, known to be influenced by inflammation and a hallmark of many pathological states, may also drive NMDA receptor hypofunction independent of changes in absolute levels of 24(S)-OHC. For our analyses, we focused on the ratios of 24S- to 25- and 27-OHC to reflect this important interaction. Our results demonstrate that higher plasma concentrations of 24(S)-OHC and higher ratios of 24S- to 25- and 27-OHC are positively related with better performance on cognitive tests and better functional assessments in early HD in two independent cohorts.
Our approach leveraged the TRACK-HD cohort to generate hypotheses and refine our oxysterol analytical method, as measuring these cholesterol metabolites can present many technical challenges.26,27 Samples from year two in TRACK-HD were utilized to develop methods that detected concentrations of 24(S)-OHC that were largely consistent with previously reported oxysterol levels in HD18,28 and provided reliable and consistent measurement of the related oxysterols 25-OHC and 27-OHC. The resulting comprehensive oxysterol panel was then used in hypothesis-generating studies of the relationships of oxysterols to cognitive, neuropsychiatric, and clinical assessments in years one, three, and four of TRACK-HD, which suggested 24(S)-OHC and its ratios to 25- and 27-OHC were associated with deteriorations in cognitive performance during early manifest HD. We tested whether this result would replicate in ENROLL-HD, which had a greater number of participants and a more extensive battery of cognitive, clinical, and psychometric tests. Collectively, the refined methodology for oxysterol measurement, initial hypothesis-generating work in TRACK-HD, and subsequent hypothesis-testing work in ENROLL-HD allowed for a robust investigation into plasma biomarkers for HD and their association with various cognitive and clinical aspects of the disease.
Across both TRACK-HD and ENROLL-HD, we observed similar decreases in 24(S)-OHC and the ratio of 24(S)-OHC to 25-OHC and 27-OHC in early-stage HD. Our findings of lower 24(S)-OHC in early-stage HD are consistent with those reported previously in independent cohorts of early stage HD patients18,19,28 as well as in later stages of the disease,17 making 24(S)-OHC one of the more reproducible biomarkers for CNS cholesterol dysfunction in HD. Additionally, our studies expand the work in 24(S)-OHC to incorporate its relationship to related oxysterols by incorporating concentrations of 25- and 27-OHC and testing how the ratios of these oxysterols relate to cognitive performance. Symbol digit modality test and Stroop word reading test are two of the most sensitive measures of cognitive decline during early HD29,30 and performance on these tests has been positively correlated with plasma 24(S)-OHC concentrations in the PREDICT-HD cohort.19 Our observations in TRACK-HD (years 1, 3, 4) and ENROLL-HD are the first to detect a positive relationship between the ratio of 24(S)/25-OHC ratio in plasma to performance on symbol digit modality test and Stroop word reading tests. Performance on Trail A and B suggested a similar, positive relationship with 24(S)/25-OHC ratio, although statistical significance was variable across years in TRACK-HD and did not achieve significance following FDR adjustment in ENROLL-HD. In TRACK-HD, the strongest relationship we observed was the positive correlation between 24(S)/25-OHC ratio and better performance on the emotional recognition test, suggesting this plasma biomarker may track with early cognitive deficits in HD. Indeed, impaired emotional recognition occurs prior to motor symptoms31 and is well documented in manifest HD.32 In ENROLL-HD, 24(S)/25-OHC ratio was positively associated with performance on additional tests, including Stroop tests (color naming, interference) and verbal tests, all of which were statistically significant after FDR adjustment. Collectively, the cognitive batteries in TRACK-HD and ENROLL-HD support the notion that increased 24(S) and 24(S) to 25- or 27-OHC ratios is associated with better performance on cognitive tests.
Beyond the extensive cognitive battery performed in TRACK-HD and ENROLL-HD, we tested whether plasma oxysterol levels were related to the functional and motor aspects of HD. UHDRS TFC is composite score that incorporates an individual’s ability to perform their work, manage finances, perform domestic chores and daily living tasks, and whether they require care, thus capturing cognitive and motor aspects of HD. In both TRACK-HD and ENROLL-HD, higher TFC scores were associated with higher levels of 24(S)-OHC and 24(S) ratios. In ENROLL-HD, higher FA scores, which relates to an individual’s independence, were also associated with higher 24(S)/25-OHC ratios. Calculation of the CAP score is an objective metric meant to predict a participants transition to manifest HD at a score of 100. Higher CAP scores, i.e., scores for participants that were nearing or had transitioned to manifest HD, were correlated with lower 24(S)/25-OHC ratios in the ENROLL-HD registry. Motor manifestations demarcate the transition from pre-manifest HD to early manifest HD and we did not observe a relationship between 24(S)/25-OHC with TMS in TRACK-HD; however, this relationship was significant in in ENROLL-HD. Importantly, across both datasets, we did not observe relationships with individual motor assessments. To inspect participants near the transition to manifest HD with more granularity, we compared pre-manifest and manifest HD participants that had full functional capacity and minor motor impairment (Fig. 5C, 5E, Supplementary Figure 7C) and these analyses collectively suggested there was no difference in 24(S)/25-OHC ratio at minor motor impairment, but significant decreases in 24(S)/25-OHC ratio in manifest HD despite intact TFC and functional scores. It is challenging to decouple the relationship between cognitive impairment and motor symptom onset, as manifest HD is characterized by the latter. Additionally, at the point of motor symptom onset, 90% of participants in ENROLL-HD were characterized with either mild cognitive impairment (84.2% ) or dementia (5.3% ).33 We propose that these data, combined with the weak associations between 24(S)/25-OHC ratio on motor and psychiatric endpoints, lack of association with components of the SF-12, and that the observed associations with TFC and overall function are driven primarily by effects on cognitive performance.
Our analyses of TRACK-HD and ENROLL-HD offer a unique opportunity to unite cholesterol dysregulation and the pathophysiology of cognitive impairment in early-stage HD. Although the precise mechanism(s) driving the changes in 24(S)-OHC remain unknown, changes in cholesterol synthesis via HMG CoA reductase,12 CYP46A1 (the primary enzyme responsible for converting cholesterol to 24(S)-OHC in the brain),17 and altered transcriptional regulation of cholesterol synthesis (SREBP)16 have all been implicated in cholesterol dysregulation in HD. Deficits in negative emotion recognition, Stroop task, and symbol digit modality are hallmark cognitive impairments observed in all stages of HD, including pre-manifest patients.32,34,35 Importantly, it has been demonstrated that NMDA receptors are critical for performance on each of these tasks. The non-competitive NMDA receptor open channel blocker ketamine impairs performance on emotion recognition, the Wisconsin Card Sorting task, and SDMT in healthy volunteers.36–38 Along with consistent involvement of NMDA receptors, the SDMT, Stroop, and emotion tasks also share a reliance on the prefrontal cortex, an area critical for executive function where NMDA receptors have been shown to play a vital role.39–41 Previous imaging studies have demonstrated that, while some pre-frontal degeneration is present in HD patients (especially later in the disease course), overall changes in prefrontal volume are proportional to overall brain atrophy.42 Thus, deficits observed in performance on these tasks in both the TRACK-HD and ENROLL-HD populations are unlikely to be driven solely by neurodegeneration/cell loss and suggest an alternative explanation for cognitive impairment on these tasks. Our data support the hypothesis that changes in cholesterol metabolism, potentially affecting NMDA receptor tone via reductions in the NMDA receptor PAM 24(S)-OHC, may contribute to cognitive impairment in HD.
The data presented here have limitations that are worth noting. First, TRACK-HD and ENROLL-HD are independent datasets and did not perform identical cognitive and clinical batteries, meaning that some of our specific findings were strong in one dataset but unable to be tested in the other (e.g., 24(S)/25-OHC ratio and performance emotional recognition test). However, there are some overlapping measures in TRACK-HD and ENROLL-HD, i.e., TFC, TMS, and several cognitive tests, and review of both datasets support the conclusions we have drawn. Second, our oxysterol assay evolved between measuring TRACK-HD and ENROLL-HD, and we were not able to directly compare oxysterol concentrations across the two cohorts. Use of the ratio of 24(S)-OHC to 25- and 27-OHC allowed for comparisons across the method evolution. Next, we are unable to make longitudinal inferences regarding 24(S)-OHC changes over the limited time course of TRACK-HD and the single timepoint studied in ENROLL-HD. Data from the four-year TRACK-HD study did not reflect progressive decreases in 24(S)-OHC in participants and we suspect that this short study duration was not sufficient for detecting statistically significant decreases in plasma oxysterols. Year 4 of the TRACK-HD dataset had stronger correlations (e.g., higher r and lower p values) than other years; however, this appears to be due to changes on cognitive test performance (including stable or better performance in control participants from year 1 to 4 and/or decreases in performance in manifest HD participants from year 1 to 4). ENROLL-HD was designed as a longitudinal study; however, only a single time point had plasma samples available for analysis. We compared the cross section of data available at this time point for analyses and did not adjust for age or education level, which others have used in longitudinal analyses of ENROLL.43 As more plasma samples become available in future years for ENROLL-HD, we plan to continue our analyses and adjust for additional factors of interest that may help us to gain longitudinal insight. Lastly, our investigation into plasma oxysterols and cognitive performance necessitated that we look across controls, pre-manifest HD, and early manifest HD participants to glean an understanding of how changes in plasma biomarkers could relate to performance on cognitive tests. As there is considerable inter-individual variability in plasma oxysterols, we are unable to provide a discrete cutoff for beneficial levels of 24(S)-OHC or 24(S)-OHC ratios. Despite these caveats, we believe these data contribute meaningfully to our understanding cholesterol dysregulation in HD, particularly as it relates to the cognitive aspects of the disease.
Given the moderate and consistent relationship between changes in 24(S)-OHC, the 24(S)/25-OHC ratio to cognitive performance in HD, and the known role of these cholesterol metabolites to influence the activity of NMDA receptors, one potential therapeutic approach to improve cognitive performance in HD may be to increase the activity of NMDA receptors. Our group has identified oxysterol-based molecules that are PAMs of the NMDA receptor.10,44–46 These NMDA receptor PAMs show pre-clinical efficacy in a range of behavioral models of NMDA receptor hypofunction, including PCP- induced social interaction deficits produced by subchronic administration of an NMDA receptor open channel blocker and age-related memory impairment. Dalzanemdor (SAGE-718),44–46 an NMDA receptor PAM that has entered clinical development, improved performance on the two-back test with trends towards improvement on the Groton Maze learning test in early stage HD participants in a small, open-label study incorporated into our multiple ascending dose clinical trial.47 As both tasks rely on prefrontal cortical networks48,49 and have evidence for an NMDA receptor-mediated component50,51 these early data are consistent with our hypotheses generated from TRACK-HD and ENROLL-HD and provide a conceptual foundation for our ongoing clinical trials in HD. Beyond NMDA receptor PAMs, other trials testing therapies focused on modulating cholesterol metabolism have initiated (CYP46A1 gene therapy; AskBio AB-1001) based on compelling pre-clinical data demonstrating that modulation of cholesterol synthesis/catabolism, and cholesterol replacement strategies, can ameliorate aspects of HD, including cognitive impairment.52 The pre-clinical benefits of the restoration of cholesterol homeostasis are likely to involve multiple mechanisms including, but not limited to, increases in 24(S)-OHC, decreases in neuroinflammation, and restoration of myelination, each of which may contribute to cognitive improvements observed in the models. While it is too early to know whether either of these efforts will ultimately be successful, cholesterol dysfunction is likely a contributing factor to HD pathology and represents a new avenue for therapeutic exploration.
ACKNOWLEDGMENTS
Biosamples and data used in this work were generously provided by the participants in the TRACK-HD and ENROLL-HD studies and made available by CHDI Foundation, Inc. ENROLL-HD is a clinical research platform and longitudinal observational study for Huntington’s disease families intended to accelerate progress towards therapeutics; it is sponsored by CHDI Foundation, a nonprofit biomedical research organization exclusively dedicated to collaboratively developing therapeutics for HD. ENROLL-HD would not be possible without the vital contribution of the research participants and their families (https://enroll-hd.org/enrollhd_documents/ENROLL-HD_AcknowledgementsListPDS6_v1.0_20230119.pdf). We thank Tatiana Kazdoba, Jason Johannesen, Kirsty Nahm for their contributions to the manuscript. We thank Al Robichaud, Jim Doherty, and Rebecca Hammond for their leadership and guidance throughout these experiments.
FUNDING
The authors have no funding to report.
CONFLICT OF INTEREST
Sarah M. Gray, Jing Dai, Anne C. Smith, Jacob T. Beckley, Michael C. Lewis, and Michael C. Quirk are employees of Sage Therapeutics, Inc. and hold stock or stock options. Negah Rahmati is a former employee of Sage Therapeutics, Inc. and may hold stock or stock options.
DATA AVAILABILITY
The data are not publicly available due to privacy restrictions. The data supporting the findings of this study are available on request from the corresponding author. Participant data requires a data use arrangement with CHDI Foundation Inc.
SUPPLEMENTARY MATERIAL
[1] The supplementary material is available in the electronic version of this article: https://dx.doi.org/10.3233/JHD-240030.
REFERENCES
[1] | Schade DS , Shey L and Eaton RP. Cholesterol review: A metabolically important molecule. Endocrine Practice (2020) ; 26: : 1514–1523. |
[2] | Orth M and Bellosta S. Cholesterol: Its regulation and role in central nervous system disorders. Cholesterol (2012) ; 2012: : 292598. |
[3] | Karasinska JM and Hayden MR. Cholesterol metabolism in Huntington disease. Nat Rev Neurol (2011) ; 7: : 561–572. |
[4] | Dai L , Zou L , Meng L , et al. Cholesterol metabolism in neurodegenerative diseases: Molecular mechanisms and therapeutic targets. Mol Neurobiol (2021) ; 58: : 2183–2201. |
[5] | Sodero AO . 24S-hydroxycholesterol: Cellular effects and variations in brain diseases. J Neurochem (2021) ; 157: : 899–918. |
[6] | Odnoshivkina UG , Kuznetsova EA and Petrov AM. 25-hydroxycholesterol as a signaling molecule of the nervous system. Biochemistry (Mosc) (2022) ; 87: : 524–537. |
[7] | Ma L , Cho W and Nelson ER. Our evolving understanding of how 27-hydroxycholesterol influences cancer. Biochem Pharmacol (2022) ; 196: : 114621. |
[8] | Sandebring-Matton A , Goikolea J , Björkhem I , et al. 27-Hydroxycholesterol, cognition, and brain imaging markers in the FINGER randomized controlled trial. Alzheimers Res Therapy (2021) ; 13: : 56. |
[9] | Nelson ER , Wardell SE and McDonnell DP. The molecular mechanisms underlying the pharmacological actions of estrogens, SERMs and oxysterols: Implications for the treatment and prevention of osteoporosis. Bone (2013) ; 53: : 42–50. |
[10] | Paul SM , Doherty JJ , Robichaud AJ , et al. The major brain cholesterol metabolite 24(S)-hydroxycholesterol is a potent allosteric modulator of N-Methyl-D-aspartate receptors. J Neurosci (2013) ; 33: : 17290–17300. |
[11] | Linsenbardt AJ , Taylor A , Emnett CM , et al. Different oxysterols have opposing actions at N-methyl-d-aspartate receptors. Neuropharmacology (2014) ; 85: : 232–242. |
[12] | Valenza M , Rigamonti D , Goffredo D , et al. Dysfunction of the cholesterol biosynthetic pathway in Huntington’s disease. J Neurosci (2005) ; 25: : 9932–9939. |
[13] | Valenza M , Carroll JB , Leoni V , et al. Cholesterol biosynthesis pathway is disturbed in YAC128 mice and is modulated by huntingtin mutation. Hum Mol Genet (2007) ; 16: : 2187–2198. |
[14] | Shankaran M , Di Paolo E , Leoni V , et al. Early and brain region-specific decrease of de novo cholesterol biosynthesis in Huntington’s disease: A cross-validation study in Q175 knock-in mice. Neurobiol Dis (2017) ; 98: : 66–76. |
[15] | Valenza M , Leoni V , Karasinska JM , et al. Cholesterol defect is marked across multiple rodent models of Huntington’s disease and is manifest in astrocytes. J Neurosci (2010) ; 30: : 10844–10850. |
[16] | Di Pardo A , Monyror J , Morales LC , et al. Mutant huntingtin interacts with the sterol regulatory element-binding proteins and impairs their nuclear import. Hum Mol Genet (2020) ; 29: : 418–431. |
[17] | Kreilaus F , Spiro AS , McLean CA , et al. Evidence for altered cholesterol metabolism in Huntington’s disease post mortem brain tissue, Neuropathol Appl Neurobiol (2016) ; 42: : 535–546. |
[18] | Leoni V , Mariotti C , Tabrizi SJ , et al. Plasma 24S-hydroxycholesterol and caudate MRI in pre-manifest and early Huntington’s disease. Brain (2008) ; 131: : 2851–2859. |
[19] | Leoni V , Long JD , Mills JA , et al. Plasma 24S-hydroxycholesterol correlation with markers of Huntington disease progression. Neurobiol Dis (2013) ; 55: : 37–43. |
[20] | Paulsen JS . Cognitive impairment in Huntington disease: Diagnosis and treatment. Curr Neurol Neurosci Rep (2011) ; 11: : 474–483. |
[21] | Geoffroy C , Paoletti P and Mony L. Positive allosteric modulation of NMDA receptors: Mechanisms, physiological impact and therapeutic potential. J Physiol (2022) ; 600: : 233–259. |
[22] | Tabrizi SJ , Langbehn DR , Leavitt BR , et al. Biological and clinical manifestations of Huntington’s disease in the longitudinal TRACK-HD study: Cross-sectional analysis of baseline data. Lancet Neurol (2009) ; 8: : 791–801. |
[23] | Landwehrmeyer GB , Fitzer-Attas CJ , Giuliano JD , et al. Data analytics from Enroll-HD, a global clinical research platform for Huntington’s disease. Mov Disord Clin Pract (2017) ; 4: : 212–224. |
[24] | Tang W , Beckley JT , Zhang J , et al. Novel neuroactive steroids as positive allosteric modulators of NMDA receptors: Mechanism, site of action, and rescue pharmacology on GRIN variants associated with neurological conditions. Cell Mol Life Sci (2023) ; 80: : 42. |
[25] | Stout JC , Jones R , Labuschagne I , et al. Evaluation of longitudinal 12 and 24 month cognitive outcomes in premanifest and early Huntington’s disease. J Neurol Neurosurg Psychiatry (2012) ; 83: : 687–694. |
[26] | Lütjohann D , Björkhem I , Friedrichs S , et al. International descriptive and interventional survey for oxycholesterol determination by gas- and liquid-chromatographic methods. Biochimie (2018) ; 153: : 26–32. |
[27] | Griffiths WJ and Wang Y. Oxysterol research: A brief review. Biochem Soc Trans (2019) ; 47: : 517–526. |
[28] | Leoni V , Mariotti C , Nanetti L , et al. Whole body cholesterol metabolism is impaired in Huntington’s disease. Neurosci Lett (2011) ; 494: : 245–249. |
[29] | Tabrizi SJ , Reilmann R , Roos RA , et al. Potential endpoints for clinical trials in premanifest and early Huntington’s disease in the TRACK-HD study: Analysis of 24 month observational data. Lancet Neurol (2012) ; 11: : 42–53. |
[30] | Paulsen JS and Long JD. Onset of Huntington’s disease: Can it be purely cognitive?. Mov Disord (2014) ; 29: : 1342–1350. |
[31] | Bora E , Velakoulis D and Walterfang M. Social cognition in Huntington’s disease: A meta-analysis. Behav Brain Res (2016) ; 297: : 131–140. |
[32] | Henley SMD , Novak MJU , Frost C , et al. Emotion recognition in Huntington’s disease: A systematic review. NeurosciBiobehav Rev (2012) ; 36: : 237–253. |
[33] | Julayanont P , McFarland NR and Heilman KM. Mild cognitive impairment and dementia in motor manifest Huntington’s disease: Classification and prevalence. J Neurol Sci (2020) ; 408: : 116523. |
[34] | Snowden JS . The neuropsychology of Huntington’s disease. Arch Clin Neuropsychol (2017) ; 32: : 876–887. |
[35] | Sierra LA , Ullman CJ , Baselga-Garriga C , et al. Prevalence of neurocognitive disorder in Huntington’s disease using the Enroll-HD dataset. Front Neurol (2023) ; 14: : 1198145. |
[36] | Abel KM , Allin MPG , Kucharska-Pietura K , et al. Ketamine alters neural processing of facial emotion recognition in healthy men: An fMRI study. Neuroreport (2003) ; 387–391. |
[37] | Krystal JH , Bennett A , Abi-Saab D , et al. Dissociation of ketamine effects on rule acquisition and rule implementation: Possible relevance to NMDA receptor contributions to executive cognitive functions. Biol Psychiatry (2000) ; 47: : 137–143. |
[38] | Tucker AP , Kim YI , Nadeson R , et al. Investigation of the potentiation of the analgesic effects of fentanyl by ketamine in humans: A double-blinded, randomised, placebo controlled, crossover study of experimental pain[ISRCTN83088383]. BMC Anesthesiol (2005) ; 5: : 2. |
[39] | Dogan I , Saß C , Mirzazade S , et al. Neural correlates of impaired emotion processing in manifest Huntington’s disease. Soc Cogn Affect Neurosci (2014) ; 9: : 671–680. |
[40] | Silva PHR , Spedo CT , Barreira AA , et al. Symbol Digit Modalities Test adaptation for magnetic resonance imaging environment: A systematic review and meta-analysis. Mult Scler Relat Disord (2018) ; 20: : 136–143. |
[41] | Vendrell P , Junqué C , Pujol J , et al. The role of prefrontal regions in the Stroop task. Neuropsychologia (1995) ; 33: : 341–352. |
[42] | Aylward EH , Li Q , Stine OC , et al. Longitudinal change in basal ganglia volume in patients with Huntington’s disease. Neurology (1997) ; 48: : 394–399. |
[43] | Mills JA , Long JD , Mohan A , et al. Cognitive and motor norms for Huntington’s disease. Arch Clin Neuropsychol (2020) ; 35: : 671–682. |
[44] | La DS , Salituro FG , Martinez Botella G , et al. Neuroactive steroid N-Methyl-d-aspartate receptor positive allosteric modulators: Synthesis, SAR, and pharmacological activity. J Med Chem (2019) ; 62: : 7526–7542. |
[45] | Hill MD , Blanco M-J , Salituro FG , et al. SAGE-718: A first-in-class N-Methyl-d-Aspartate receptor positive allosteric modulator for the potential treatment of cognitive impairment. J Med Chem (2022) ; 65: : 9063–9075. |
[46] | Beckley JT , Aman TK , Ackley MA , et al. Pharmacological characterization of SAGE-718, a novel positive allosteric modulator of N-methyl-d-aspartate receptors. Br J Pharmacol (2024) ; 181: : 1028–1050. |
[47] | Koenig A , Lewis M , Wald J , et al. Dalzanemdor (SAGE-718), a novel, investigational N-methyl-D-aspartate receptor positive allosteric modulator: Safety, tolerability, and clinical pharmacology in randomized dose-finding studies in healthy participants and an open-label study in participants with Huntington’s disease. Clin Transl Sci (2024) ; 17: : e13852. |
[48] | Tsuchida A and Fellows LK. Lesion evidence that two distinct regions within prefrontal cortex are critical for n-Back performance in humans. J Cogn Neurosci (2009) ; 21: : 2263–2275. |
[49] | Pietrzak R , Cohen H and Snyder P. Spatial learning efficiency and error monitoring in normal aging: An investigation using a novel hidden maze learning test. Arch Clin Neuropsychol (2007) ; 22: : 235–245. |
[50] | Avellar M , Scoriels L , Madeira C , et al. The effect of D-serine administration on cognition and mood in older adults. Oncotarget (2016) ; 7: : 11881–11888. |
[51] | Forsyth JK , Bachman P , Mathalon DH , et al. Augmenting NMDA receptor signaling boosts experience-dependent neuroplasticity in the adult human brain. Proc Natl Acad Sci U S A (2015) ; 112: : 15331–15336. |
[52] | Valenza M , Birolini G and Cattaneo E. The translational potential of cholesterol-based therapies for neurological disease. Nat Rev Neurol (2023) ; 19: : 583–598. |