Strawberry physiological and biochemical responses to chilling and freezing stress and application of alleviating factors as countermeasures
Abstract
BACKGROUND:
Strawberry is usually cultivated in unheated glasshouses or outdoors, suffering many times by low temperatures during the winter period, with the danger of plant losses.
OBJECTIVE:
To investigate the efficacy of various alleviating agents against chilling and freezing stress.
METHODS:
Strawberry plants were foliarly treated with various alleviating substances, i.e. glycine betaine, trehalose, salicylic acid and proline and exposed to 5 °C (chilling treatment) for three weeks followed by a three hour exposure to – 10 °C (freezing treatment), while control plants were growing under 20 °C. At the end of each period polyamine and carbohydrate concentration, antioxidant enzymes activities, total phenols and proline concentration, chlorophyll concentration, lipid peroxidation, electrolyte leakage and H2O2 production in the leaves were measured.
RESULTS:
All applied products alleviated chilling stress, inducing lower electrolyte leakage, but higher sucrose and fructose concentration in the leaves compared to control. Under freezing temperature, salicylic acid proved to be the most efficient based on the lowest leaf damage observed, followed by trehalose and proline.
CONCLUSIONS:
Salicylic acid was the best alleviation agent based on leaf damage evaluation, by inducing low chlorophyll a degradation, low superoxide dismoutase, peroxidase and polyphenol oxidase activities as well as low putrescine concentration.
1Introduction
Extreme environmental conditions adversely affect plant growth and development. Drought, salinity and temperature extremes are the major determinants of plant survival and productivity [1]. As the changes of ambient temperature occur more rapidly and sharply than water shortage, salinity and nutrient imbalances, it is obvious that temperature extremes aggravate the adverse effects of the other stresses.
Strawberry is one of the most popular berry species and its fruit are highly appreciated by consumers all over the world. Although strawberry is mostly cultivated indoors, it is often exposed to low winter temperatures, as most greenhouses are not regularly heated. Similarly, strawberry plants are exposed to temperature extremes during the winter months in areas where it is grown as perennial.
Chilling (0–15 °C) and freezing (<0 °C) stresses are considered as the most devastating temperature stress factors. Chilling induces cell dehydration, leading to membrane lipid peroxidation, alterations of membrane fluidity and at the end loss of membrane integrity and selectivity [2]. Cold stress may affect chlorophyll concentration and chloroplast development and functions [3]. Furthermore, it creates severe imbalance between absorption and dissipation of light energy, causing an over-reduction of the electron transport chain, increasing the photosynthetic electron flux to O2, leading to the production of excess reactive oxygen species (ROS) and to oxidative damages [4].
ROS production triggers the counter-measures mechanism of the plant, including the activation of antioxidant enzymes such as catalase (CAT), peroxidase (POD), superoxide dismutase (SOD), glutathione reductase (GR), ascorbate peroxidase (APOX), polyphenol-oxidase (PPO) etc. as well as the production of antioxidant molecules such as ascorbic acid, phenolic compounds etc [3–5].
Low temperature stress have similar effects as drought stress, because water freezing creates a shortage of liquid water. Under such conditions some plants have evolved mechanisms to withstand water shortage under freezing temperatures. Upon exposure to low, non-freezing, non-lethal suboptimal temperatures, plant starts the synthesis of various molecules, among which cryo- or osmo-protectans have a major role [5]. The so-called compatible solutes are low molecular weight, highly soluble compounds, protecting cell integrity by participating in osmotic adjustment, detoxification of reactive oxygen species, protection of membrane function, and stabilization of enzymes/proteins. Some of them are nitrogenous compounds (proline, glycine betaine), soluble sugars (sucrose, raffinose, trehalose) as well as sugar alcohols (sorbitol, mannitol) [1]. Similarly, polyamines (PAs), low molecular, aliphatic nitrogenous compounds, have been found to increase under cold stress in cold tolerant genotypes, without their role being clearly defined till now [6, 7].
In order to increase plant tolerance to abiotic stresses, various cultural managements have been employed, among which is the external application of cryo-protective compounds. Glycine betaine, proline and trehalose are osmo-protectants which have been found to be effective against cold stress in various plant species [3, 4, 8]. Salicylic acid is another compound, a simple phenolic one (ortho-hydroxy benzoic acid), which has been used to induce abiotic stress tolerance in various crops [9].
The aim of the present trial was to investigate the efficacy of various stress mitigating agents on strawberry plants exposed to short term chilling and freezing stress, based on plant injury index and physiological and biochemical parameters involved in plant growth and abiotic tolerance.
2Materials and methods
2.1Plant material
Commercial fresh strawberry plants cv. Camarosa were purchased and used as plant material. Plants of similar development were planted in 5 L pots filled with a mixture of peat:perlite:garden soil at a ratio of 1 : 1:3. Plants were grown in a greenhouse from October till mid January when they attained enough foliage. In early January plants were fertilized with a water-soluble fertilizer (21-21-21 plus micronutrients) applying 2 g per plant along with iron (as iron chelate, FeEDDHA) at a dose rate of 1 g per plant too.
In the middle of January (plants were at the growth stage 15–17, i.e. 5 to 7 true leaves unfolded- based on BBCH crop growth stage scale) plants were sprayed till run off with the following substances: trehalose at a concentration of 20 mM (purchased from Cargill, Minnesota, USA), salicylic acid at a concentration of 2 mM (Sigma Co), which was first diluted in a small volume of ethanol, proline at a concentration of 20 mM (Sigma Co.) and glycine betaine at a dose rate of 2.5 g L–1 (applied as commercial product Bluestim WP, 50% w/w glycine betaine, a product of Verdera, Finland). Substances concentrations were selected based on the literature or the commercial product label. Control plants were sprayed with distilled water. Spraying solution pH ranged from 7.0 to 7.9 and the mean spray volume applied per plant was 18.81 mL.
Plants remained in the greenhouse for one week and later on, at least 12 plants from each treatment were transferred into a growth chamber under 5±1 °C (chilling treatment), while 12 different plants, from control treatment only, were transferred into a growth chamber under 20±1 °C. Plants remained under chilling and room temperature for 3 weeks, when the sampling event took place.
At the end of the three weeks period, different, previously non-sampled plants from each spray application, were transferred from the chilling treatment into a growth chamber under dark, where temperature was stepwise decreased by 2 °C per hour till – 10±1 °C (freezing treatment), in order to avoid sudden temperature shock of the plants. Strawberry plants remained under – 10 °C for 3 h, when the sampling event took place. The temperature was then gradually increased to room temperature levels and four days afterwards all treated plants were transferred to the greenhouse. Fifteen days after transferring to the greenhouse the freezing injury was assessed based on the following scale: 0, no symptom at all, 1, symptoms in less than 10% of the total leaf surface, 2, symptoms at 10–25% of the total leaf surface, 3, symptoms at 25–50% of the total leaf surface, 4, symptoms at more than 50% of the total leaf surface and 5, plants are dead. The freezing injury was assessed on at least 5 plants per treatment, subjected to the chilling and freezing procedure described above, without being sampled earlier.
2.2Sampling procedure
All healthy, fully expanded leaves (approximately 5–6 leaves per plant) were sampled from six plants per treatment in each sampling event (a total of 30–36 leaves per treatment). Part of the leaf sample was used immediately as fresh material (see below), while the rest was lyophilized and then ground into a fine powder by a centrifugal mill and the powder stored under –25±1 °C till further analyses.
2.3Chlorophyll measurement
Leaf discs 5.55 mm in diameter were excised from fresh strawberry leaves of approximately 15–20 mg per sample (2–3 disks per leaf, a total of approximately 15 disks per plant, were excised and part of them were used for the chlorophyll measurement – the rest were used for lipid peroxidation assay as indicated below). Chlorophylls were extracted with 5 mL of 80% (v/v) ethanol twice by continuous stirring and after centrifuging the absorbance of the supernatant was measured at 663 and 645 nm using a Helios γ spectrophotometer (Thermo Electron Corporation, Germany). Chlorophyll concentration was estimated using the equations described by Lichtenthaler [10] and expressed as mg chlorophyll per g fresh weight.
2.4Electrolyte leakage, hydrogen peroxide and lipid peroxidation based on thiobarbituric acid reacting substances (TBARS) assays
Leaf discs were excised from fresh strawberry leaves (approximately 5 per plant), immersed in 10 mL distilled water and electrolyte leakage was assessed according to the method of Bajji et al. [11] after a 3 h gentle agitation in an orbital shaker.
Lipid peroxidation was measured as described by Denaxa et al. [12], according to Chaparzadeh et al. [13] with some modifications. Briefly, 0.2 g of fresh leaf tissue was homogenized in 10 mL 0.25% w/v thiobarbituric acid diluted in 10% w/v trichloroacetic acid (TCA), using an Ultra-Turrax (IKA) and then incubated at 95 °C in a water bath for 30 min. The mixture was then cooled in an ice-bath and centrifuged at 4000 g for 10 min. The absorbance of the supernatant was measured at 532 nm and corrected for non-specific absorbance at 600 nm. TBARS were calculated using 155 mM–1 cm–1 as the molar extinction coefficient.
The H2O2 content was measured colorimetrically as described by Denaxa et al. [12], according to the method of Jana and Choudhuri [14]. H2O2 was extracted by homogenizing 150 mg fresh leaf tissue with 10 ml of phosphate buffer (50 mM, pH 6.5) using an Ultra Turrax (IKA T-25, IKA-Werke GmbH & Co, Germany) and the homogenate was then centrifuged at 4,000 g for 6 min. To determine H2O2 concentration, 3 ml of the supernatant reacted with 1 ml of 0.1% titanium sulfate in 20% (v/v) H2SO4. The mixture was then centrifuged at 4,000 g for 15 min and the intensity of the yellow color of the supernatant was measured at 410 nm. H2O2 concentration was calculated using the extinction coefficient 0.28μmol–1 cm–1.
2.5Phenolic compounds extraction, determination and quantification
Approximately 50 mg of freeze-dried leaf tissue was first extracted with 5 mL petroleum ether 40–60 °C in order to remove lipids and chlorophylls. The solution was centrifuged at 4000 g for 6 min and the supernatant was discarded. The pellet was extracted twice with 5 mL of 75% v/v ethanol in water, in a water bath at 45 °C for 30 min each time, with periodical stirring. After each time the solutions were centrifuged under the conditions described above and the two supernatants were combined. Total phenolic compounds, total o-diphenols, total flavanols and total flavonoids were determined as described by Roussos [15] and expressed as mg gallic acid equivalents, mg caffeic acid equivalents, mg catechin equivalents and mg caffeic acid equivalents respectively.
2.6Free polyamines (PAs) determination
Strawberry leaf samples (0.1 g) were extracted in 1 mL cold 5% (v/v) HClO4 for 1 h at 4 °C in the dark in the presence of 1, 6 hexane-diamine as internal standard. Samples were then centrifuged at 4,000 g for 15 min at 4 °C and 0.2 mL of the supernatant was used for the dansyl chloride derivatization of PAs. The derivatization procedure was conducted according to Sarjala and Kaunisto [16], with some modifications. Supernatant (0.2 mL) was reacted with 0.2 mL of saturated sodium carbonate followed by the addition of dansyl chloride (7.5 mg mL–1 acetone). The mixture was vortexed and let stand for 1 h in the dark in a water bath at 60 °C. In order to remove the excess dansyl chloride, 0.1 mL proline (100 mg mL–1 in water) was added and the mixture was incubated in the dark at room temperature for 15 min. Derivatized PAs were extracted with toluene by continuous vortexing for 1 min and the toluene fraction was dried under a stream of nitrogen. The dried residue was firstly dissolved in 0.1 mL tetrahydrofuran and 0.4 mL methanol was then added, filtered through a nylon syringe filter (0.2μm pore size) and stored in the freezer till analysis. PAs analysis was performed with an HP-1050 (Agilent, Santa Clara, California, United States) HPLC isocratic pump, on an Inertsil ODS-2 (GL Sciences, Japan) reverse phase column (240 x 4.3 mm, 5μm). The mobile phase consisted of 70% acetonitrile, 3% methanol and 27% water and the analysis was accomplished with a gradient flow rate: 0–7 min 0.5 mL min–1, 7.5–12 min 1.0 mL min–1 and at 12.5 min 1.5 mL min–1 at 30 °C. The PAs were detected with an HP 1046a fluorescence detector (Agilent, Santa Clara, California, United States), with λex adjusted at 365 nm and λem at 475 nm and quantified using 1, 2 hexane-diamine as internal standard and the corresponding relative response factors. Each sample was assayed twice and PA concentration was expressed as mg g–1 d.w.
2.7Carbohydrate determination
Carbohydrate extraction and determination was performed according to Tsafouros et al. [17]. For the HPLC analysis, a Waters 510 (Waters Corporation, Milford, Massachusetts, United States) isocratic pump was used at a flow rate of 0.6 mL min–1 of water, while the separation of the carbohydrates was achieved through a Hamilton HC-75 cation exchange column, calcium form (Ca++) (Hamilton, Bonaduz, Switzerland), equilibrated at 80 °C. Three carbohydrates were determined in strawberry leaves, thus sucrose, glucose and fructose and quantified using five-point authentic standards’ calibration curves. The final pellet was washed twice with 80% v/v ethanol in water and once with pure ethanol and let dry. Starch concentration was then estimated in the pellet after enzymatic hydrolysis according to Tsafouros et al. [17]. Briefly 1.0 mL of 0.5 N NaOH in water was added to the pellet and the solution remained for 20 min at room temperature in order to gelatinize starch. Afterwards, 0.55 mL of 2M acetic acid solution in water was added and centrifuged at 4000 g for 6 min. Part (0.5 mL) of the supernatant was withdrawn and transferred to another centrifuge tube into which 0.5 mL amyloglucosidase solution (Sigma Chemical Inc.)(approximately 4–5 mg enzyme in 25 mL of 0.2 M acetate buffer pH 4.5) was added, vortexed and placed in a waterbath at 55 °C for 1 h. The solution was then neutralized with 0.2 mL of 1.0 N NaOH, vortexed and 0.5 mL was reacted with 2 mL biosis reagent (http://www.biosis.com.gr) for 15 min in a water bath at 37 °C. The absorbance of the pink solution was measured at 510 nm against blank, while starch was quantified using a five-point calibration curve of original corn starch, treated as described above. Each sample was analyzed twice.
2.8Crude enzyme extraction
A crude extract was prepared according to the method of Denaxa et al. [12]. Fresh strawberry leaves (approximately 0.5 g fresh weight) were homogenized in an Ultra-Turrax at 4 °C in 10 mL cold 50 mM phosphate buffer pH 6.5 with 0.4 mM Na2EDTA, 5% glycerol, 1 mM ascorbic acid (for APOX extraction only) and 2% polyclar AT (w/v). The homogenate was centrifuged at 15000 g for 15 min at 4 °C. The supernatant was filtered through a Whatman No. 1 paper filter to remove floating particles and the filtered supernatant constituted the crude enzyme extract, which was held in an ice bath during the analyses.
2.9Enzyme assays
Peroxidase activity was measured according to the method of de Azevedo Neto et al. [18]. Polyphenol oxidase activity was measured according to the method of Flurkey and Jen [19]. One unit of POD or PPO enzyme activity was defined as the change of 0.01 of absorbance per minute within the linear portions of the curve. Catalase and APOX activities were measured according to the methods described by Ahmed et al. [20]. One unit of CAT activity was defined as the consumption of H2O2 per minute, using the extinction coefficient of 40 mM–1 cm–1. One unit of APX activity was defined as the consumption of ascorbic acid per minute, using the extinction coefficient of 2.8 mM–1 cm–1.
Superoxide dismutase was measured according to the method described by Giannopolitis and Ries [21] with slight modifications. A volume of 50μL of enzyme extract was added to 2.95 mL 0.2 M sodium carbonate buffer solution pH 10.2, containing 0.1 mM EDTA, 75μM nitroblue tetrazolium and 13 mM methionine with the reaction starting after the addition of 6μM riboflavin. Afterwards the reaction solution was placed under illumination (inside a circular fluorescent lamp of 30 W) for 15 min and the absorbance was measured at 560 nm. The blank consisted of the buffer solution plus riboflavin into which 100μL of extraction buffer (without polyclar AT) was added, placed under illumination for 15 min and the absorbance measured at 560 nm. The control consisted of the buffer solution plus riboflavin into which 100μL of extraction buffer (without polyclar AT) was added and the absorbance measured at 560 nm without illumination. The activity was expressed in units where 50% inhibition is equivalent to one unit of SOD activity.
Glutathione reductase activity measurement took place with modifications of the method described by Sairam and Srivastava [22] and Rios-Gonzalez et al. [23]. Briefly, 1 mmol EDTA, 1 mmol NADPH, 0.75 mmol DTNB (5,5 dithiobis-2-nitrobenzoic acid), and 0.1 mL of enzyme extract were added in a 0.1 M potassium phosphate buffer (pH 7.5), into which 1 mM glutathione disulfide (GSSG) was added in a final volume of 3 mL. The reaction started with the addition of GSSG and the change in absorbance at 412 nm was monitored for 5 min against blank (buffer plus GSSG). One unit of enzyme activity was defined as the change of absorbance per minute.
All assays were conducted at 25 °C. Protein content was determined by the dye-binding assay [24] and specific activity of each enzyme was expressed as units mg–1 protein unless otherwise stated. Each sample was assayed twice.
2.10Trial design and statistical analysis
The trial followed the completely randomized design with three replications of two plants each. Raw data were analyzed by one way Anova and significant differences between treatments under the same temperature, were determined using the Tukey HSD multiple range test at α= 0.05. In order to determine significant differences between treatments and control at 20 °C, Dunnett’s test was performed at α= 0.05.
Principal component analysis (PCA) based on physiological and phytochemical characteristics was used to evaluate the relative discriminative power of the principal components and to describe by a limited number of parameters either the treatments, under the same temperature compared to control, or the temperature effect, irrespective of the treatments applied. A heatmap based on variables’ correlations was constructed in order to investigate possible relationships. JMP 13.0 (SAS Institute, Cary, North Carolina, United States) statistical software was used for the analysis.
3Results
3.1Effect of temperature and alleviating products on chlorophyll concentration
There were not any significant changes regarding chlorophyll concentration among treatments under chilling temperature stress (Table 1). Nonetheless, chlorophyll a and total chlorophyll concentration (μg g–1 FW) was significantly decreased in control (C5), proline (PRO5) and trehalose (TREH5) treatments, compared to that detected in the leaves of plants grown under room temperature (C20). Chlorophyll b was significantly reduced under control condition at chilling temperature too, compared to those determined under room temperature. The ratio between chlorophyll a and b was found to be lower in PRO5 plants compared to that found in C20 plants.
Table 1
Effects of stress alleviating products and cold temperature on chlorophyll (Chl) concentration of strawberry leaves
Treatments | Chl a | Chl b | Total Chls | Chl a/Chl b | Chl a | Chl b | Total Chls | Chl a/Chl b |
mg g–1 FW | μg mm–2 | |||||||
C20 | 1.95 | 0.76 | 2.71 | 2.57 | 1.51 | 0.58 | 2.09 | 2.57 |
Temperature 5 °C | ||||||||
C5 | 1.24a * | 0.52a * | 1.76a * | 2.40a | 1.15a * | 0.48a | 1.63a | 2.40a |
GB5 | 1.58a | 0.60a | 2.18a | 2.63a | 1.47a | 0.56a | 2.03a | 2.63a |
PRO5 | 1.46a * | 0.64a | 2.10a * | 2.28a * | 1.26a | 0.55a | 1.82a | 2.28a * |
SA5 | 1.56a | 0.64a | 2.20a | 2.45a | 1.53a | 0.62a | 2.15a | 2.45a |
TREH5 | 1.50a * | 0.62a | 2.12a * | 2.42a | 1.47a | 0.60a | 2.07a | 2.42a |
Average | 1.47 | 0.60 | 2.07 | 2.44 | 1.38 | 0.56 | 1.94 | 2.44 |
Temperature – 10 °C | ||||||||
C-10 | 1.60ab | 0.61a | 2.21ab | 2.61bc | 1.60bc | 0.61a | 2.20b | 2.62b |
GB-10 | 1.54ab | 0.56a * | 2.10a * | 2.73c | 1.63bc | 0.60a | 2.23b | 2.73b |
PRO-10 | 1.50a * | 0.64ab | 2.14a | 2.35ab | 1.31a | 0.56a | 1.87a | 2.35a |
SA-10 | 1.81b | 0.77c | 2.58b | 2.34ab | 1.78c | 0.76b * | 2.53c | 2.34a |
TREH-10 | 1.69ab | 0.72bc | 2.41ab | 2.34a | 1.41ab | 0.60a | 2.01ab | 2.34a |
Average | 1.63 | 0.66 | 2.29 | 2.47 | 1.55 | 0.63 | 2.17 | 2.48a |
Mean values within the same column for the same temperature followed by different letters differ significantly based on Tukey HSD multiple range test at α= 0.05. Asterisks indicate significant difference of the specific treatment and control (control at 20 °C) based on Dunnett’s test at α= 0.05. Abbreviations: C20, control at 20 °C, C5, control at 5 °C, SA5, salicylic acid treated plants at 5 °C, PRO5, proline treated plants at 5 °C, GB5, glycine betaine treated plants at 5 °C, TREH5, trehalose treated plants at 5 °C, C-10, control at – 10 °C, SA-10, salicylic acid treated plants at – 10 °C, PRO-10, proline treated plants at – 10 °C, GB-10, glycine betaine treated plants at – 10 °C, TREH-10, trehalose treated plants at – 10 °C, FW, fresh weight.
Under freezing temperature though, significant differences were detected among treatments, as salicylic acid (SA-10) resulted in higher concentration of chlorophyll a than that found in proline (PRO-10) treated leaves. Salicylic acid resulted also in chlorophyll b concentration than that determined in glycine betaine (GB-10) and PRO-10 treated plants and control ones. Total chlorophyll concentration was higher in SA-10 plants compared to GB-10 and PRO-10 treated ones. Proline treated plants exposed to freezing temperatures presented lower chlorophyll a concentration compared to C20 plants, while chlorophyll b was lower in GB-10 plants compared to C20 plants. Proline treated plants exhibited lower total chlorophylls concentration upon exposure to freezing temperature compared to C20 plants, while the other treatments resulted in similar total chlorophylls concentration to those found in plants under room temperature.
3.2Effect of temperature and alleviating products on lipid peroxidation, H2O2 production and electrolyte leakage
Although there were not any significant differences among treatments, concerning TBARS production under cold stress (Fig. 1A), H2O2 concentration increased under SA5, PRO5 and TREH5 treatments compared to C5 (Fig. 1B). In all treatments, EL was lower than that determined in C5 (Fig. 1 C). TREH5 did not present any significant difference with C20 regarding TBARS production under cold stress, while EL was significantly higher under C5 compared to C20, which did not exhibit any difference from the alleviating treatments.
Fig. 1
Effect of temperature and alleviation factors on membrane lipid peroxidation (A), H2O2 (B) and electrolyte leakage (C) concentration in the leaves of strawberry plants. Different letters above each column under the same temperature denote statistically significant differences among treatments according to Tukey HSD test at α= 0.05. Asterisks denote statistically significant differences between specific treatment and control at 20 °C (C20) according to Dunnett’s test at α= 0.05. Abbreviations: C20, control at 20 °C, C, control, SA, salicylic acid treated plants, PRO, proline treated plants, GB, glycine betaine treated plants, TREH, trehalose treated plants.
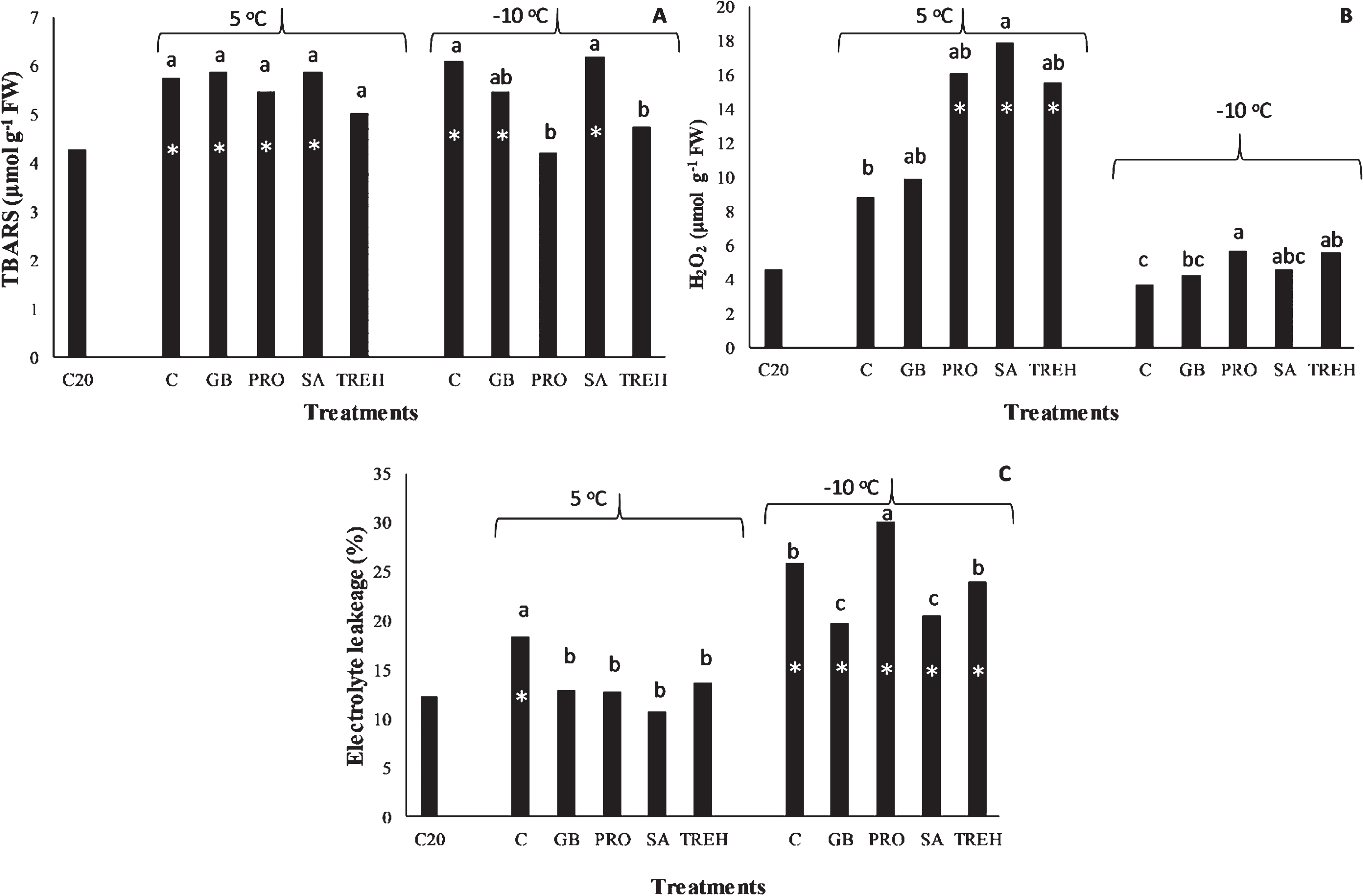
Under freezing stress though, PRO-10 and trehalose treated plants (TREH-10) presented lower TBARS production compared to C-10, without differing though with C20. Peculiarly enough, all treatments under freezing temperatures exhibited low H2O2 production, at the levels of C20, while EL was significantly higher than that determined at C20, with the highest being found under PRO-10 treatment and the lowest under GB-10 and SA-10 ones.
3.3Effect of temperature and alleviating products on antioxidant enzymes’ activity
There were significant differences among treatments concerning the antioxidant enzymes’ activity under chilling temperature, as indicated in Table 2. The SOD activity was highest in SA5 and TREH5 treated plants, higher than those measured in GB5 and PRO5 plants. POD was also highest in SA5 treated plants, with significant differences from all other treatments. SA5 treated plants exhibited also the highest PPO activity. SA5 plants presented also high GR activity, higher than those determined in C5 and TREH5 plants, as well as higher APOX activity than that measured in GB5, PRO5 and TREH5 plants, while GB5 plants presented the highest CAT activity. PRO5 plants exhibited lower SOD activity compared to C20 plants, while TREH5 plants presented significantly lower POD than the C20 plants. GR activity in all chilling stressed plants was higher than that determined in C20 plants. APOX activity was enhanced under chilling temperature in C5 and SA5 plants, while PPO activity was also enhanced in C5, PRO5, SA5 and TREH5 plants compared to those grown under room temperature. On the other hand, CAT was suppressed in C5 and PRO5, SA5 and TREH5 c plants compared to C20 ones.
Table 2
Effects of stress alleviating products and cold temperature on antioxidant enzyme activities (Units mg–1 protein) of strawberry leaves
Treatments | SOD | POD | GR | APOX | PPO | CAT |
C20 | 22.28 | 74.5 | 99.99 | 508.0 | 140.3 | 15.14 |
Temperature 5 °C | ||||||
C5 | 16.54bc | 36.99c | 217.4ab * | 651.6bc * | 415.5d * | 10.65ab * |
GB5 | 14.36b | 27.33b | 256.6bc * | 547.4a | 188.1a | 11.84b |
PRO5 | 10.40a * | 46.69 d | 231.6abc * | 542.7a | 206.4ab * | 8.74a * |
SA5 | 18.09c | 67.88 e | 267.0c * | 697.2c * | 313.4c * | 10.83ab * |
TREH5 | 18.17c | 13.86a * | 213.8a * | 585.0ab | 237.7b * | 8.92a * |
Average | 15.51 | 38.55 | 237.28 | 604.78 | 272.22 | 10.20 |
Temperature – 10 °C | ||||||
C-10 | 48.43b * | 328.5c * | 464.3c * | 872.2b * | 253.7ab * | 11.85a * |
GB-10 | 26.25a | 391.5c * | 436.6bc * | 729.1a * | 186.9a | 13.03a |
PRO-10 | 19.94a | 326.0c * | 490.1c * | 810.2ab * | 231.2a * | 12.89a |
SA-10 | 17.73a | 192.7b * | 310.0ab * | 794.2ab * | 187.8a | 13.56a |
TREH-10 | 41.01b * | 100.2a | 214.3a * | 733.7a * | 350.7b * | 14.50a |
Average | 30.67 | 267.78 | 383.06 | 787.88 | 242.06 | 13.17 |
Mean values within the same column for the same temperature followed by different letters differ significantly based on Tukey HSD multiple range test at α= 0.05. Asterisks indicate significant difference of the specific treatment and control (control at 20 °C) based on Dunnett’s test at α= 0.05. Abbreviations: C20, control at 20 °C, C5, control at 5 °C, SA5, salicylic acid treated plants at 5 °C, PRO5, proline treated plants at 5 °C, GB5, glycine betaine treated plants at 5 °C, TREH5, trehalose treated plants at 5 °C, C-10, control at – 10 °C, SA-10, salicylic acid treated plants at – 10 °C, PRO-10, proline treated plants at – 10 °C, GB-10, glycine betaine treated plants at – 10 °C, TREH-10, trehalose treated plants at – 10 °C.
Under freezing temperature, enzyme activities were significantly affected by the various treatments. C-10 and TREH-10 plants presented higher SOD activity compared to the other treatments, while TREH-10 plants exhibited the lowest POD and GR activities (without though significant difference with SA-10 regarding GR activity). APOX was enhanced in C-10 plants compared to plants treated with glycine betaine and trehalose. PPO activity was enhanced under TREH-10 treatment without significant difference from C-10 plants, while CAT was not affected by the treatments applied under freezing temperatures. All treatments exhibited significantly higher GR and APOX activities compared to C20. C-10 treatment presented higher SOD, POD and PPO and lower CAT activities, GB-10 higher POD, GR and APOX activities, PRO-10 higher POD. GR, APOX and PPO activities, SA-10 higher POD, GR and APOX acivities and TREH-10 higher SOD, GR, APOX and PPO activities than C20.
3.4Effect of temperature and alleviating products on phenolic compounds and proline concentration
There were not any significant differences among treatments under chilling temperature concerning the concentration of all phenolic compounds assayed and proline (Table 3). All treatments except for SA5 exhibited lower total phenolic compound concentration compared to control under room temperature (C20), which also presented higher total flavanol concentration than C5 and TREH5 treatments.
Table 3
Effects of stress alleviating products and cold temperature on phenolic compounds concentration of strawberry leaves
Treatments | Total phenols | Total o-diphenols | Total flavonoids | Total flavanols | Proline |
C20 | 93.62 | 21.55 | 31.03 | 142.10 | 1.03 |
Temperature 5 °C | |||||
C5 | 71.5a* | 20.92a | 25.60a | 101.44a* | 1.02a |
GB5 | 76.99a* | 21.97a | 29.95a | 124.53a | 0.79a |
PRO5 | 76.69a* | 20.89a | 25.99a | 110.53a | 1.46a |
SA5 | 81.86a | 19.91a | 28.72a | 129.29a | 0.90a |
TREH5 | 71.44a* | 20.83a | 26.24a | 98.26a* | 1.48a |
Average | 75.70 | 20.90 | 27.30 | 112.81 | 1.13 |
Temperature – 10 °C | |||||
C-10 | 75.96ab* | 24.05b | 32.68a | 133.05a | 1.85ab * |
GB-10 | 79.36b | 21.54ab | 32.45a | 135.73a | 1.19a |
PRO-10 | 64.34a* | 19.29a | 26.98a | 102.68a* | 2.19b * |
SA-10 | 68.8ab* | 21.10a | 27.77a | 104.23a* | 1.22a |
TREH-10 | 72.69ab* | 21.26a | 30.26a | 126.20a | 1.35a |
Average | 72.23 | 21.45 | 30.03 | 120.38 | 1.56 |
Mean values within the same column for the same temperature followed by different letters differ significantly based on Tukey HSD multiple range test at α= 0.05. Asterisks indicate significant difference of the specific treatment and control (control at 20 °C) based on Dunnett’s test at α= 0.05. Total phenols are expressed as mg equiv. gallic acid g–1 DW, total o-diphenols as mg equiv. caffeic acid g–1 DW, total flavanols and total flavonoids as mg equiv. catechin g–1 DW, proline as mg g–1 DW. Abbreviations: C20, control at 20 °C, C5, control at 5 °C, SA5, salicylic acid treated plants at 5 °C, PRO5, proline treated plants at 5 °C, GB5, glycine betaine treated plants at 5 °C, TREH5, trehalose treated plants at 5 °C, C-10, control at – 10 °C, SA-10, salicylic acid treated plants at – 10 °C, PRO-10, proline treated plants at – 10 °C, GB-10, glycine betaine treated plants at – 10 °C, TREH-10, trehalose treated plants at – 10 °C.
Significant differences were observed among treatments under freezing temperature concerning the concentration of total phenols and o-diphenols in the leaves. GB-10 treated plants exhibited the higher total phenol concentration than PRO-10 plants, while C-10 the higher total o-diphenols concentration compared to PRO-10, SA-10 and TREH-10 treated plants. All treatments apart from GB-10 exhibited significant differences with C20 regarding total phenols concentration, while only PRO-10 and SA-10 exhibited significantly lower total flavanol concentration than C20 among treatments. Proline concentration was found to be higher in PRO-10 plants, without though significant difference from C-10 plants, exhibiting significant difference from that detected in C20 plants.
3.5Effect of temperature and alleviating products on carbohydrate concentration
All the treatments with cryo-protective compounds resulted in higher sucrose, fructose and total sugars concentration than control (C5) (Table 4). TREH5 plants exhibited the highest leaf glucose concentration without though significant difference from GB5 plant, while there was not any difference regarding starch concentration. Sucrolysis index was found to be higher in SA5 treatment than TREH5, with significant difference from C20 too. Control plants grown under 5 °C presented lower sucrose, fructose and total sugars concentration than those grown under room temperature (C20).
Table 4
Effects of stress alleviating products and cold temperature on carbohydrate concentration (mg g–1 DW) and sucrolysis index (SI) of strawberry leaves
Treatments | Sucrose | Glucose | Fructose | Starch | Total sugars | SI |
C20 | 50.95 | 12.22 | 13.83 | 51.15 | 77.01 | 1.96 |
Temperature 5 °C | ||||||
C5 | 28.12a * | 8.95a | 6.14a * | 48.99a | 43.21a * | 1.88ab |
GB5 | 53.80b | 11.75ab | 16.20b | 47.32a | 81.77b | 1.92ab |
PRO5 | 53.52b | 7.81a | 16.33b | 49.11a | 77.66b | 2.24ab |
SA5 | 52.47b | 7.97a | 12.94b | 57.55a | 73.38b | 2.54b * |
TREH5 | 52.82b | 15.00b | 15.12b | 51.94a | 82.94b | 1.75a |
Average | 48.15 | 10.30 | 13.35 | 50.98 | 71.79 | 2.07 |
Temperature – 10 °C | ||||||
C-10 | 28.60a * | 11.05b | 24.57b * | 50.87a | 64.22a | 0.80a * |
GB-10 | 58.41b | 7.09a * | 19.06a * | 50.68a | 84.57a | 2.25b |
PRO-10 | 53.49b | 7.19a * | 25.45b * | 49.56a | 86.14a | 1.65ab |
SA-10 | 57.75b | 13.11b | 28.62b * | 58.31a | 99.49a | 1.36ab |
TREH-10 | 40.57b | 11.85b | 27.93b * | 42.24a | 80.37a | 1.03a |
Average | 47.76 | 10.06 | 25.13 | 50.33 | 82.96 | 1.42 |
Mean values within the same column for the same temperature followed by different letters differ significantly based on Tukey HSD multiple range test at α= 0.05. Asterisks indicate significant difference of the specific treatment and control (control at 20 °C) based on Dunnett’s test at α= 0.05. Abbreviations: C20, control at 20 °C, C5, control at 5 °C, SA5, salicylic acid treated plants at 5 °C, PRO5, proline treated plants at 5 °C, GB5, glycine betaine treated plants at 5 °C, TREH5, trehalose treated plants at 5 °C, C-10, control at – 10 °C, SA-10, salicylic acid treated plants at – 10 °C, PRO-10, proline treated plants at – 10 °C, GB-10, glycine betaine treated plants at – 10 °C, TREH-10, trehalose treated plants at – 10 °C.
Similarly, plants grown under freezing temperature, treated with the various cryo-protective compounds exhibited higher sucrose concentration than C-10 plants, while plants under GB-10 treatment presented the lowest glucose (along with PRO-10 treated plants) and fructose concentration. There were not any significant differences concerning starch and total sugars concentration, while C-10 and TREH-10 treated plants exhibited the lower sucrolysis index than GB-10 plants. All treatments under freezing temperatures resulted in higher fructose concentration than C20 one, while only C-10 treated plants presented lower sucrose concentration than C20 plants. Glucose was found to be in lower concentration in the leaves of GB-10 and PRO-10 treated plants compared to that determined in C20 plants.
3.6Effect of temperature and alleviating products on free polyamines concentration
There were not any significant differences among treatments concerning the concentration of free polyamines under chilling temperature, nor any differences compared to C20 (Table 5).
Table 5
Effects of stress alleviating products and cold temperature on polyamines concentration (μg g–1 DW) of strawberry leaves
Treatments | PUT | SPD | SPM | Total PAs |
C20 | 10.77 | 47.04 | 56.98 | 114.80 |
Temperature 5 °C | ||||
C5 | 7.82a | 27.86a | 101.93a | 137.62a |
GB5 | 11.15a | 30.64a | 63.60a | 105.40a |
PRO5 | 9.42a | 41.11a | 50.41a | 100.94a |
SA5 | 9.30a | 36.94a | 69.25a | 115.50a |
TREH5 | 5.39a | 39.59a | 66.23a | 111.21a |
Average | 8.62 | 35.23 | 70.28 | 114.13 |
Temperature – 10 °C | ||||
C-10 | 64.14a * | 96.59ab | 30.54ab | 191.27a |
GB-10 | 62.49a * | 52.24a | 25.40a | 140.13a |
PRO-10 | 89.15a * | 383.38d * | 80.27b | 552.81c * |
SA-10 | 58.45a * | 266.79c * | 65.27ab | 390.52bc * |
TREH-10 | 61.16a * | 183.64bc * | 65.73ab | 310.54ab * |
Average | 67.08 | 196.53 | 53.44 | 317.05 |
Mean values within the same column for the same temperature followed by different letters differ significantly based on Tukey HSD multiple range test at α= 0.05. Asterisks indicate significant difference of the specific treatment and control (control at 20 °C) based on Dunnett’s test at α= 0.05. Abbreviations: C20, control at 20 °C, C5, control at 5 °C, SA5, salicylic acid treated plants at 5 °C, PRO5, proline treated plants at 5 °C, GB5, glycine betaine treated plants at 5 °C, TREH5, trehalose treated plants at 5 °C, C-10, control at – 10 °C, SA-10, salicylic acid treated plants at – 10 °C, PRO-10, proline treated plants at – 10 °C, GB-10, glycine betaine treated plants at – 10 °C, TREH-10, trehalose treated plants at – 10 °C, PUT, putrescine, SPD, spermidine, SPM, spermine, PAs, polyamines.
Under freezing temperature though PRO-10 plants exhibited the highest spermidine (SPD) concentration. Similarly PRO-10 plants presented higher spermine (SPM) concentration than GB-10 plants, as well as higher total free polyamines (PAs) concentration than C-10, GB-10 and TREH-10 treated plants. All treatments presented significant differences with C20 concerning putrescine (PUT) concentration while PRO-10, SA-10 and TREH-10 treatments exhibited higher concentration of both SPD and total PAs compared to C20.
3.7Principal component analysis and correlations among parameters measured
The effects of the various treatments under chilling temperature are depicted in Fig. 2 after Principal Component Analysis in two components. It is clear that although the applications of the various products were not clearly distinguished among each other, they were placed between C5 and C20, indicating that they exhibited some kind of alleviating action under chilling temperature, triggering the plants to respond differently from control ones (C5), tending to have similar behavior to C20 plants.
Fig. 2
Bi-plot presentation of the principal component analysis and treatment classification based on the measured parameters in strawberry plants exposed to ambient and chilling temperatures. Abbreviations: C5, control at 5 °C, C20, control at 20 °C, Ods, o-diphenols, Chlr, chlorophyll ratio based on concentration, FLOIDS, total flavonoids, CAT, catalase, Chlrmm, chlorophyll ratio based on area, SOD, superoxide desmoutase, TPH, total phenols, FLANOLS, total flavanols, PUT, putrescine, POD, peroxidase, Chla, chlorophyll a, chls, total chlorophylls, chlb, chlorophyll b, SPD, spermidine, chlamm, chlorophyll a based on area, chlsmm, total chlorophylls based on area, GLUC, glucose, FRUCT, fructose, SUC, sucrose, SUGS, total soluble carbohydrates, chlbmm, chlorophyll b based on area, PRO, proline, SI, sucrolysis index, GR, glutathione reductase, PAs, total polyamines, SPM, spermine, TBARS, thiobarbituric reactive substances, APOX, ascorbate peroxidase, PPO, polyphenol oxidase, EL, electrolyte leakage.
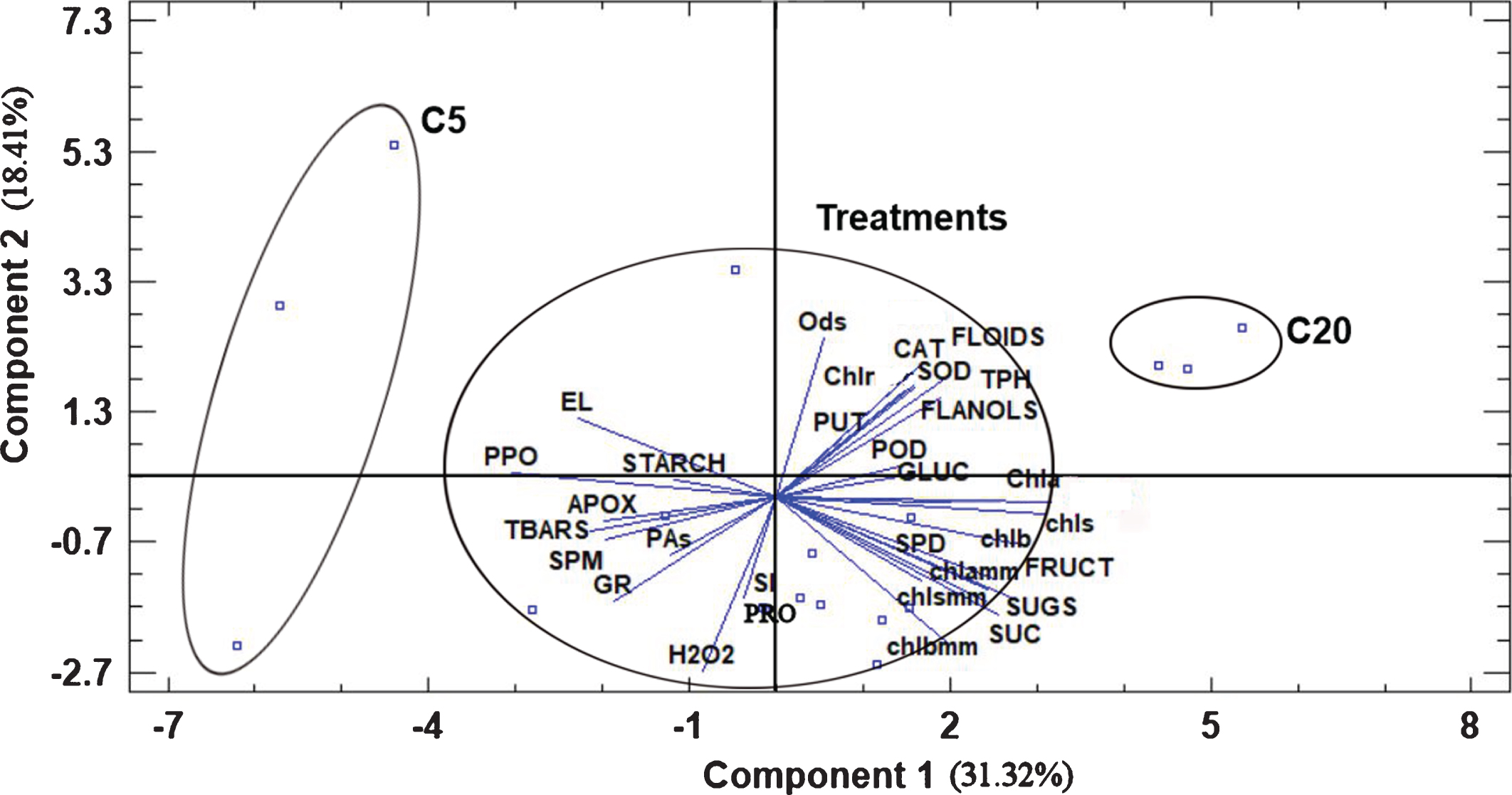
Nonetheless, treatments were clearly distinguished under freezing temperature. Plants grown under room temperature (C20) were placed in the positive side of principal component 2 (PC2) and on the negative side of PC1 (Fig. 3). Similarly, SA, PRO and TREH treated plants grown under freezing temperature were placed on the positive side of PC2, while GB-10 plants were placed together with control plants under freezing temperature, on the negative side of both PC1 and PC2.
Fig. 3
Bi-plot presentation of the principal component analysis and treatment classification based on the measured parameters in strawberry plants exposed to ambient and freezing temperatures. Abbreviations: C-10, control at – 10 °C, C20, control at 20 °C, SA-10, salicylic acid treated plants at – 10 °C, PRO-10, proline treated plants at – 10 °C, GB-10, glycine betaine treated plants at – 10 °C, TREH-10, trehalose treated plants at – 10 °C, Ods, o-diphenols, Chlr, chlorophyll ratio based on concentration, FLOIDS, total flavonoids, CAT, catalase, Chlrmm, chlorophyll ratio based on area, SOD, superoxide desmoutase, TPH, total phenols, FLANOLS, total flavanols, PUT, putrescine, POD, peroxidase, Chla, chlorophyll a, chls, total chlorophylls, chlb, chlorophyll b, SPD, spermidine, chlamm, chlorophyll a based on area, chlsmm, total chlorophylls based on area, GLUC, glucose, FRUCT, fructose, SUC, sucrose, SUGS, total soluble carbohydrates, chlbmm, chlorophyll b based on area, PRO, proline, SI, sucrolysis index, GR, glutathione reductase, PAs, total polyamines, SPM, spermine, TBARS, thiobarbituric reactive substances, APOX, ascorbate peroxidase, PPO, polyphenol oxidase, EL, electrolyte leakage.
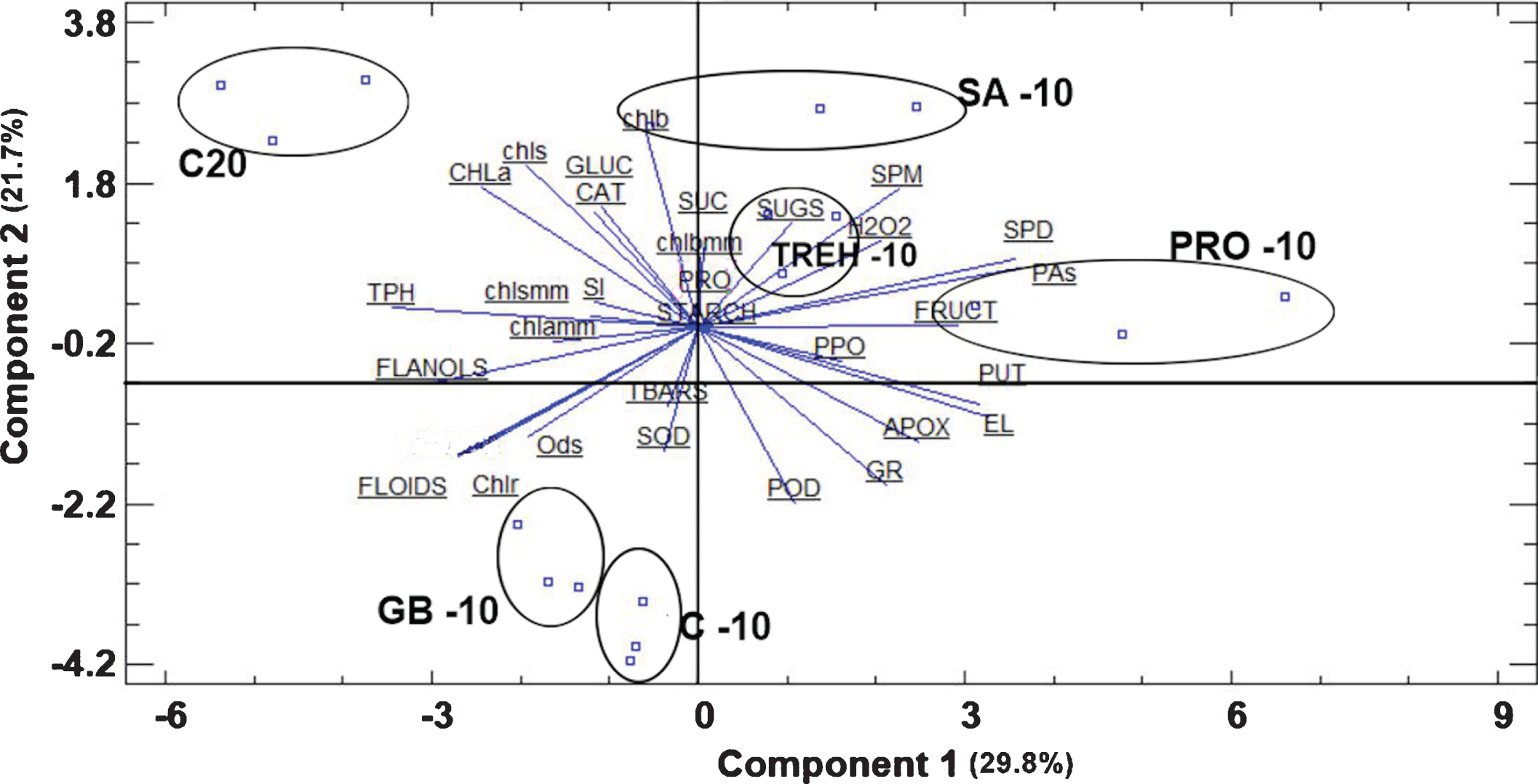
Temperature, irrespective of the treatment applied, had a significant, definite effect on the plants, as is clearly shown in Fig. 4. Plants grown under room temperature are located on the negative side of PC2 and on the positive of PC1, exhibiting high concentration of total flavanols. Plants grown under chilling temperature are located on the negative side of PC1, presenting high H2O2 concentration and sucrolysis index values, on the opposite side of plants grown under freezing temperature, which were characterized by high APOX, POD, SOD, CAT and GR activity, FLOIDS, fructose, putrescine, spermidine, total polyamines and PRO concentration.
Fig. 4
Bi-plot presentation of the principal component analysis and temperature classification based on the measured parameters in strawberry plants exposed to ambient, chilling and freezing temperatures. Abbreviations: C5, control at 5 °C, C-10, control at – 10 °C, T5, temperature 5 °C, T-10, temperature – 10 °C, T20, temperature 20 °C, Ods, o-diphenols, Chlr, chlorophyll ratio based on concentration, FLOIDS, total flavonoids, CAT, catalase, Chlrmm, chlorophyll ratio based on area, SOD, superoxide desmoutase, TPH, total phenols, FLANOLS, total flavanols, PUT, putrescine, POD, peroxidase, Chla, chlorophyll a, chls, total chlorophylls, chlb, chlorophyll b, SPD, spermidine, chlamm, chlorophyll a based on area, chlsmm, total chlorophylls based on area, GLUC, glucose, FRUCT, fructose, SUC, sucrose, SUGS, total soluble carbohydrates, chlbmm, chlorophyll b based on area, PRO, proline, SI, sucrolysis index, GR, glutathione reductase, PAs, total polyamines, SPM, spermine, TBARS, thiobarbituric reactive substances, APOX, ascorbate peroxidase, PPO, polyphenol oxidase, EL, electrolyte leakage.
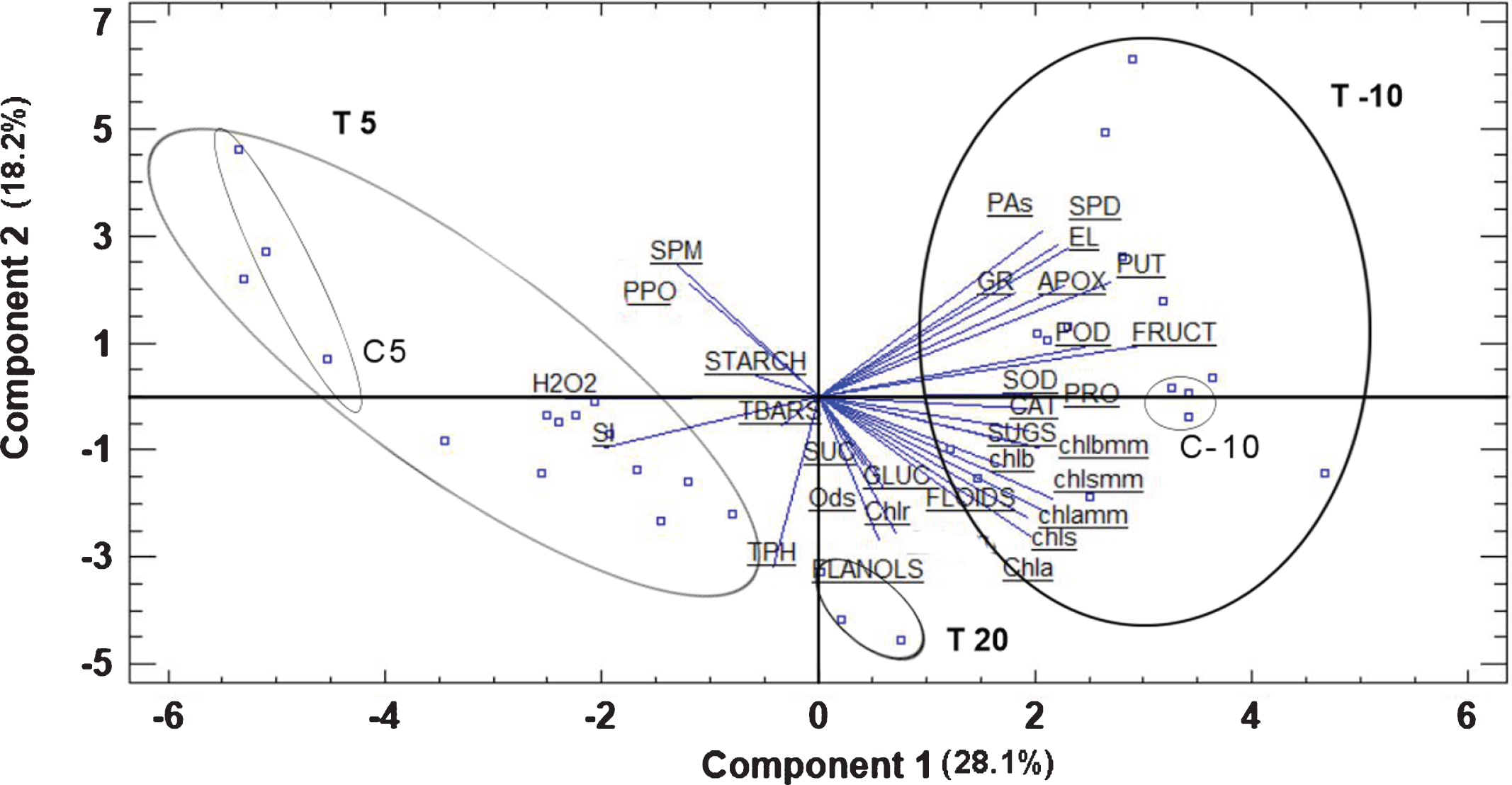
The correlation analysis is shown in Fig. 5 as a heatmap. It can be seen that POD, CAT, SOD and PUT exhibited a negative correlation with H2O2, while APOX and GR exhibited a positive correlation. Total polyamines, SPD and PUT presented strong positive relationship with EL.
Fig. 5
Heatmap presentation of the correlation analysis among the measured variables. Abbreviations: APOX, ascorbate peroxidase, CAT, catalase, Chla, chlorophyll a, chl a mm, chlorophyll a based on area, chl b, chlorophyll b, chl b mm, chlorophyll b based on area, Chlr, chlorophyll ratio, chls, total chlorophylls, chlsmm, total chlorophylls based on area, EL, electrolyte leakage, FLANOLS, total flavanols, FLOIDS, total flavonoids, FRUCT, fructose, GLUC, glucose, GR, glutathione reductase, Ods, o-diphenols, PAs, total polyamines, POD, peroxidase, PPO, polyphenol oxidase, PRO, proline, PUT, putrescine, SI, sucrolysis index, SOD, superoxide desmoutase, SPD, spermidine, SPM, spermine, SUC, sucrose, SUGS, total soluble carbohydrates, TBARS, thiobarbituric reactive substances, TPH, total phenols.
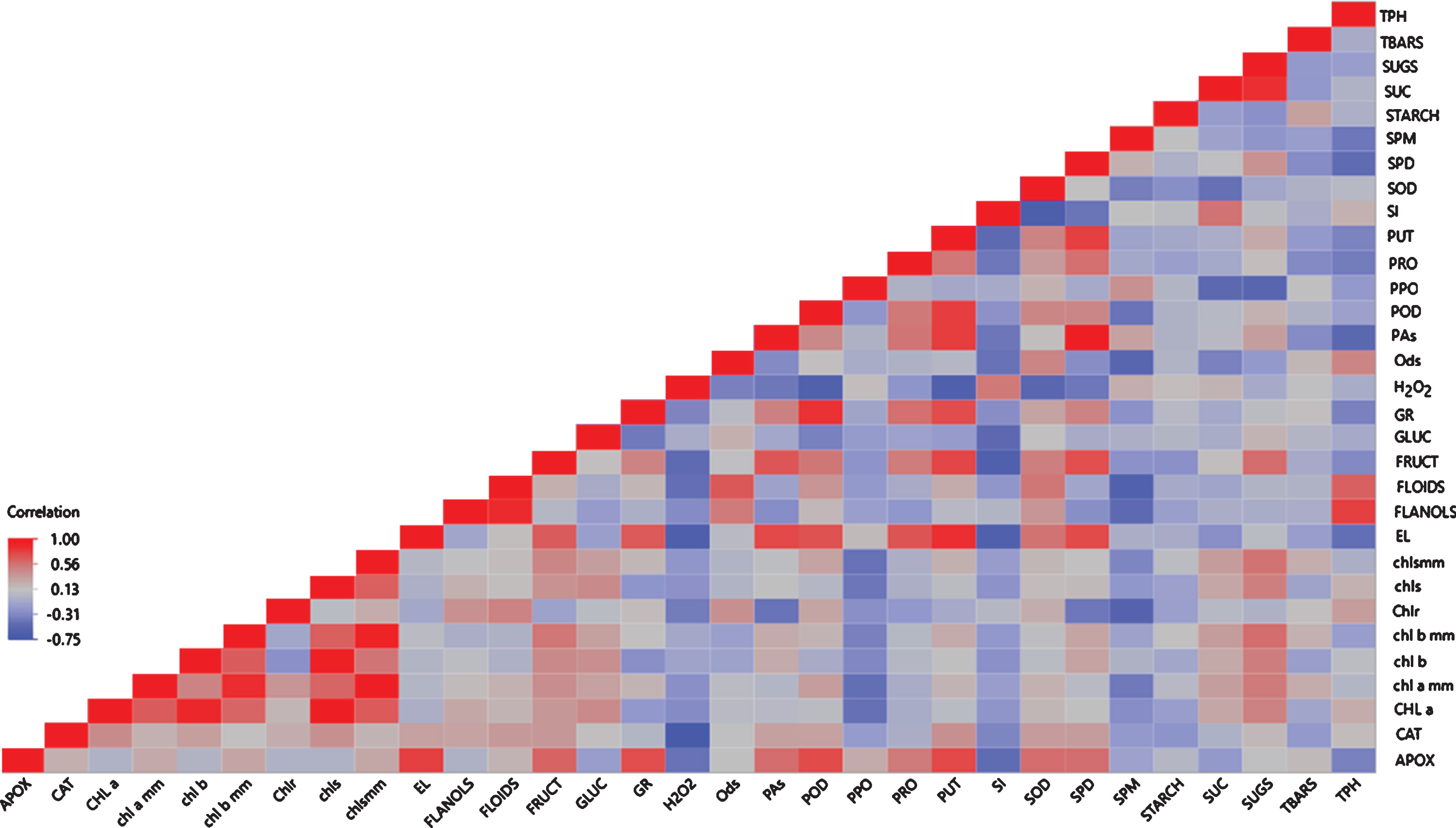
3.8Severity of symptoms
Leaf damage was quite obvious in all plants, irrespective of the treatment employed, as can be seen in Fig. 6. The distribution of damage though was different among treatments, with the majority (60%) of control plants and of GB-10 plants presenting symptoms on more than 50% of the leaf area. On the other hand, SA-10 plants presented the least symptoms, with the majority of them (approximately 85%) exhibiting symptoms on less than 25% of the leaf area. The mean leaf area damage differed significantly among treatments, as C-10 presented a mean value of 3.6 while SA-10 a mean value of 1.9, significantly lower than that of C-10.
Fig. 6
Leaf damage classification percentage of plants exposed to – 10 °C under the effect of the various alleviating treatments. Means at the top of the bars indicate the mean leaf damage based on the scale denoted at the right side of the graph. Means followed by the same letter do not differ significantly based on Tukey HSD multiple range test at α= 0.05. Abbreviations: C-10, control at – 10 °C, GB-10, glycine betaine treated plants at – 10 °C, PRO-10, proline treated plants at – 10 °C, SA-10, salicylic acid treated plants at – 10 °C, TREH-10, trehalose treated plants at – 10 °C. Numbers in the legend box at the right indicate severity symptoms based on the following scale: 0, no symptom at all, 1, symptoms in less than 10% of the total leaf surface, 2, symptoms at 10– 25% of the total leaf surface, 3, symptoms at 25– 50% of the total leaf surface, 4, symptoms at more than 50% of the total leaf surface and 5, plants are dead.
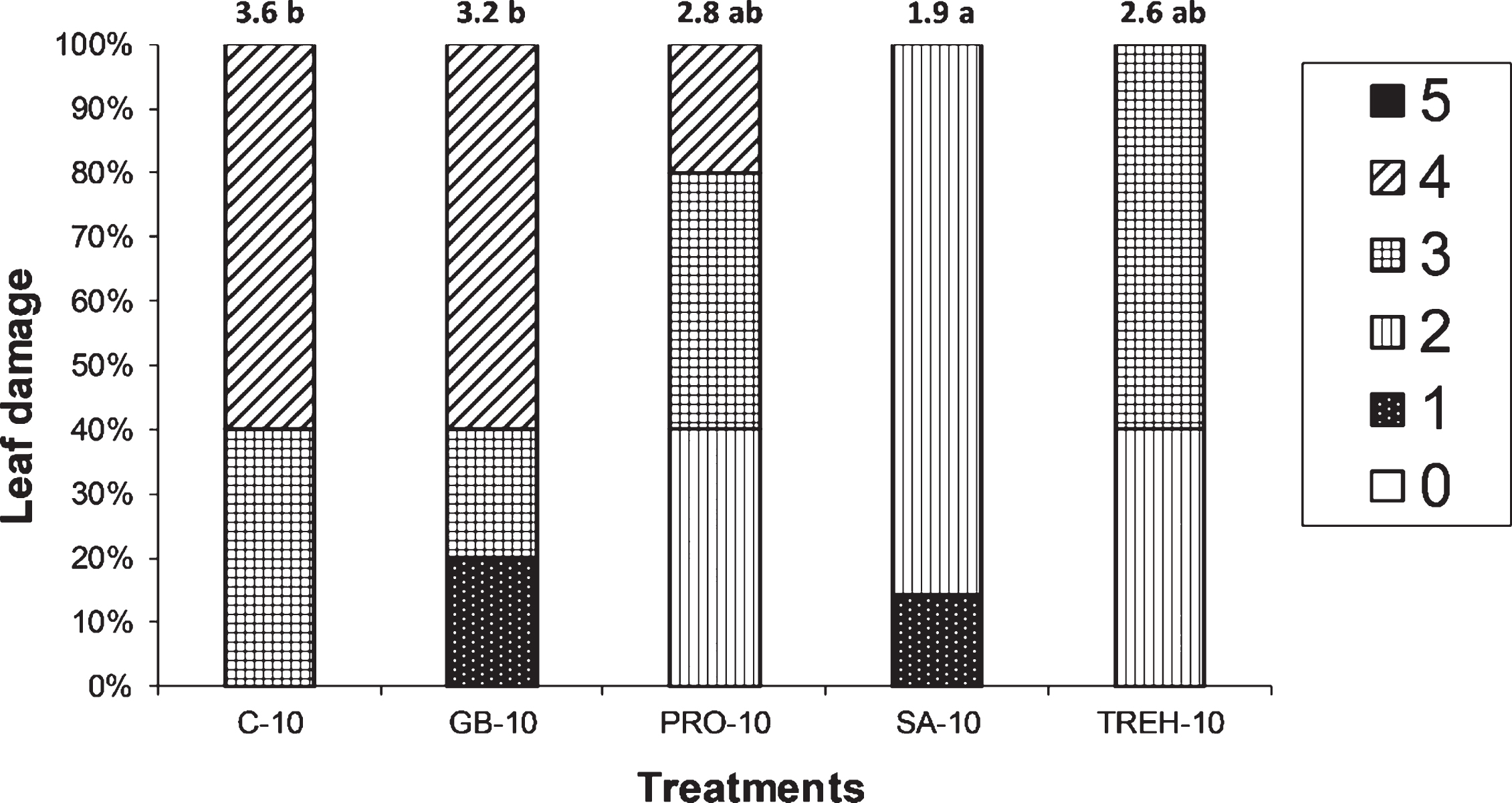
4Discussion
The effects of cold and freeze stress were quite obvious, both on severity symptoms assessed and on biochemical changes induced, as indicated by the PCA depicted in Fig. 4.
Decreases in chlorophyll concentration under cold conditions have been previously reported [25, 26], indicating a reduced chlorophyll synthesis rate and/or an increased breakdown [27]. This chlorophyll loss could possibly lead to a reduced photosynthetic rate, justifying partly the decrease of carbohydrate concentration detected.
Proline is an effective osmo-protectand and cryo-protective agent in many species [33, 36]. In the present experiment freezing induced an accumulation of proline in control plants in accordance with the literature [33], but based on the severity symptoms it seems that it does not have a significant role in freeze protection of strawberry plants.
Since phenolic compounds confer to the antioxidant potential of the cells, the observed decrease in their concentration under cold and freeze stress could be the result of their contribution to the antioxidant defense system of the plant [28], justified by the negative correlation of electrolyte leakage with phenol concentration. Similar decreases in the concentration of phenolic compounds have been reported in grapevine leaves grown under cold stress [29– 31], while there are many reports of phenolic compounds concentration increasing with cold stress [32– 34]. Phenolic compound accumulation under cold stress has been reported also in petunia [35], without though exhibiting any relationship with chilling tolerance. These discrepancies could be due to the genetic material used, to the stress conditions imposed as well as to the time of sampling, since the increase of phenolic compounds could be an early response to stress, while their decrease could indicate their active participation to the antioxidant defense system under continuous stress, contributing to cell redox homeostasis. Beyond this point though, as the stress conditions continue, phenolic compound concentration could be depleted, contributing thus no more to the antioxidant defense mechanism of the plant.
On the other hand, though, the increased PPO activity determined under both cold and freeze stress, could have triggered phenolic compound catabolism, as has been reported in peach fruit under cold storage [37]. According to Ortega-García and Peragón [38] the induction of PPO expression has been related to plant tolerance against stress, and this was confirmed also by Hashempour et al. [39] working with olive under cold stress. The present findings indicate that in the antioxidant defense arsenal, besides the phenolic compounds, the antioxidant enzymes may also confer protection. Cold and freeze stress resulted in increased antioxidant enzyme activities, apart from CAT activity, which decreased, similar to the results reported with other plants subjected to cold stress [31, 32, 40– 42]. CAT activity exhibited a negative correlation with H2O2 indicating their close relation, as CAT is among the enzymes detoxifying cells from H2O2. Nonetheless, H2O2 can be also neutralized through the activity of the Asada-Halliwell scavenging cycle, through the upregulation of the activity of APOX, GR, monodehydroascorbate reductase (MDHAR) and dehydroascorbate reductase (DHAR). APOX catalyzes the reaction between ascorbic acid and H2O2, and GR mediates the regeneration of ascorbic acid, with APOX exhibiting higher affinity towards H2O2 compared to CAT, being thus a more efficient H2O2 scavenger [43]. In the present experiment APOX activity increased under both cold and freeze stress, but it seemed to be unable to control H2O2 concentration increase, under cold stress. On the other hand, H2O2 concentration decreased under freezing temperature, which coincided with increased APOX, POD (another efficient H2O2 scavenger), GR and SOD activities, as has been reported in many other plants subjected to cold stress [32, 40]. All these enzymes exhibited a negative correlation with H2O2 concentration (shown in the heatmap), indicative of the effective control achieved [32]. It seems thus that a single enzyme is not enough to control H2O2 rise and prevent membrane damage. Instead, an orchistrated increase of the activities of H2O2 scavenging enzymes is needed, in order to retain H2O2 concentration at low levels and protect cellular stability. In the end, this effective antioxidant mechanism inhibited extensive lipid peroxidation and resulted in a negative correlation between electrolyte leakage and H2O2 concentration.
Polyamines are low aliphatic molecules, which have been found to participate in abiotic stress alleviation in many plants. In the present experiment, polyamine concentration was not greatly changed under cold stress, but it significantly increased under freezing temperature, especially that of PUT and SPD. Increased PUT levels under chilling stress have been reported in many plants [7, 44], while SPD external application has conferred tolerance to cold in rice [45].
External application of alleviating factors proved to have a significant effect on alleviating the stress response of strawberry plants. Based on the PCA of the data derived when the plants were exposed to chilling stress, it was evident that all treatments alleviated stress, as all treatments were placed between plants grown at 20 °C and plants under chilling conditions. Under freezing temperature SA, PRO and TREH seemed to alleviate stress symptoms-responses more effectively than GB, as indicated by the corresponding PCA graph. SA treatment resulted in increased chlorophyll concentration in the leaves, followed by TREH. Similarly, increased chlorophyll concentration under cold, after TREH treatment compared to control, has been observed in pepper [46] as well as in other species under drought stress [47, 48]. SA, on the other hand, has been found to increase chlorophyll concentration in strawberry leaves under stress [49, 50] and to induce tolerance to cold [51], as was also found in the present research. SA increased also H2O2 concentration in the leaves under cold stress, without though increasing electrolyte leakage. A similar increase of H2O2 concentration and induction of freezing tolerance has been reported in wheat [52], indicating a close relation between SA application, H2O2 production and induction of tolerance. It could be possible that the triggering of H2O2 production after SA application under chilling conditions, may act as a messenger for the up-regulation of the antioxidant defense mechanism (mainly of antioxidant enzymes activities), was observed in SA-10 treated plants.
Alleviating products had a significant effect on the enzymatic antioxidant mechanism of the plants too. Variations on the effects of exogenously applied alleviating products have been reported, concerning antioxidant enzyme activities [47, 53– 57] as has been found in the present experiment. Similarly, a great variation of the activities of these enzymes during the exposure to stress has been observed, with inconsistent changes in time [53, 58]. In most cases though, the applied products resulted in increased enzyme activities [46, 56, 59] and as a result in increased protection under various abiotic stresses. In the present experiment though, plants treated with the alleviating products, exhibited a significant reduction of antioxidant enzyme activities. Nonetheless, they induced significant frost protection, as indicated by the lower symptom’s index, suggesting that in strawberry the specific alleviating factors may trigger different protection mechanisms against cold and freezing stress, other than antioxidant enzymes. This is also supported by the PCA of the freezing experiment, where the majority of these enzymes fall out of the areas denoting each specific alleviation substance.
Phenolic compounds are a large group of compounds, which may serve as non-enzymatic antioxidant molecules. In the present experiment, the application of the alleviating products did not induce, in most cases, any significant changes in the concentration of these compounds, revealing the minor role played by them in protection against cold and freeze damage. Similar results have been reported in strawberry under salt stress after SA application [57] and in olive under drought stress after GB application [12]. Nonetheless, several studies indicate that the application of ameliorating products induce the accumulation of phenolic compounds as part of their protective mechanism [60–62]. It is obvious thus, that the efficacy of either the applied ameliorating substance or of phenolic metabolism in conferring protection is influenced by many factors and it cannot be defined under a universal approach.
Sugars are also molecules which serve as osmo-protectants and therefore can confer, to some degree, cold protection. In the present experiment, all applied alleviation products resulted in increased sucrose and fructose concentration under chilling stress and of sucrose under freezing stress. Glycine betaine application resulted in similar results and conferred cold stress protection in peach fruits by enhancing sugar metabolism [62], while increased activity of sucrose synthase under cold stress has been reported by Kaur et al. [63] in chickpea seedlings, conferring tolerance. Salicylic acid external application improved chilling tolerance in sacha inchi (Plukenetia volubilis) by increasing soluble sugars concentration [64], while PRO and TREH application confer also tolerance to abiotic stresses through increased concentration of soluble sugar [36, 65]. Thus part of the amelioration action of the alleviating products could be based on their effect on inducing soluble sugars accumulation under cold stress in strawberry leaves, acting as compatible solutes as well as components of the ROS scavenging system, preventing thus cellular damage, sustaining cellular structure, function and integrity. PRO application resulted also in increased proline concentration in the leaves, supporting even further its amelioration action through the accumulation of compatible solutes (sugars and proline). It was also evident that GB-10 plants exhibited low concentration of PRO, which may be an indication that the presence of an osmo-protectant (in this case glycine betaine) may render the accumulation of another osmo-protectant (i.e. proline) unnecessary, as has been also reported by Shahid et al. [66] in strawberry plants exposed to cold, under the effect of GB. The same authors suggested that in strawberry the low levels of PRO in the leaves, may indicate low cold damage, supporting the findings of the present experiment in SA treated plants, which exhibited low PRO concentration as well as low frost damage.
Polyamines are also molecules of high biological significance under abiotic stress. Under chilling stress, none of the external applications had a significant effect on polyamines concentration. Nonetheless, under freezing stress, PRO and SA induced a significant increase of SPD. Similar increase after PRO and SA application in polyamines concentration have been reported in other species too [67, 68], while SPD accumulation has been correlated with reduced chilling injury and multiple stress tolerance, by enhancing antioxidant defenses, reducing thus oxidative damage [69–71].
From the present findings, it was obvious that alleviating products had a significant protective action under chilling stress, as indicated by the PCA depicted in Fig. 2, where all alleviation treatments were located between temperature 20 °C and 5 °C, indicating a trend of mitigating cold stress responses. On the other hand, alleviating product efficacy was clearly distinguished under freezing stress, as GB treatment was not able to confer tolerance, located close to control treatment under –10 °C (C-10). Primarily SA and TREH and secondary PRO seemed to be more efficient in mitigating the adverse effects of freezing stress, as they were located closer to control plants under 20 °C.
In conclusion, all products offered significant protection under cold, whilst SA was the most effective in protecting strawberry leaves under freezing stress, mainly due to its effect on inducing soluble sugars accumulation and prevent chlorophyll degradation. Nonetheless, since the trial involved the use of only one cultivar, it is advisable to test more cultivars in the future in order to get a more spherical aspect of the reaction of strawberry plant against chilling and freezing stress and the efficacy of the various alleviating agents.
Funding
There was not any external funding for the present research, apart from that of the university annual budget.
Conflict of interest
The authors have no conflict of interest to report.
Acknowledgements
The authors would like to thank Georion SA, Ilia County, for providing the strawberry plants.
References
[1] | Ashraf M , Foolad M . Roles of glycine betaine and proline in improving plant abiotic stress resistance. Environ Exp Bot. (2007) ;59: (2):206–16. |
[2] | Shin H , Min K , Arora R . Exogenous salicylic acid improves freezing tolerance of spinach (Spinacia oleracea L.) leaves. Cryobiology. (2018) ;81: :192–200. |
[3] | Ding F , Wang R . Amelioration of postharvest chilling stress by trehalose in pepper. Scientia Hortic. (2018) ;232: :52–6. |
[4] | Kumar V , Yadav SK . Proline and betaine provide protection to antioxidant and methylglyoxal detoxification systems during cold stress in Camellia sinensis (L.) O. Kuntze. Acta Physiologiae Plant. (2009) ;31: (2):261–9. |
[5] | Zhang Y , Tang H-R , Luo Y , Hou Y-X . Responses of antioxidant enzymes and compounds in strawberry (Fragaria×ananassa ‘Toyonaka’) to cold stress. New Zealand J Crop Hortic Sci. (2009) ;37: (4):383–90. |
[6] | Nayyar H , Chander S . Protective effects of polyamines against oxidative stress induced by water and cold stress in chickpea. J Agron Crop Sci. (2004) ;190: (5):355–65. |
[7] | Wang Y , Wang G , Zheng Y , Zheng Y , Li S , Shao J , et al. Polyamines are involved in chilling tolerance in tobacco (Nicotiana tabacum) seedlings. Plant Growth Regul. 2019:1-14. |
[8] | Rajashekar C , Zhou H , Marcum K , Prakash O . Glycine betaine accumulation and induction of cold tolerance in strawberry (Fragaria X ananassa Duch.) plants. Plant Sci. (1999) ;148: (2):175–83. |
[9] | Ahmad F , Singh A , Kamal A . Salicylic acid–mediated defense mechanisms to abiotic stress tolerance. Plant Signaling Molecules: Elsevier; 2019, p. 355-69. |
[10] | Lichtenthaler HK . [34] Chlorophylls and carotenoids: pigments of photosynthetic biomembranes. Methods in Enzymology. 148: Elsevier; 1987, p. 350-82. |
[11] | Bajji M , Kinet J-M , Lutts S . The use of the electrolyte leakage method for assessing cell membrane stability as a water stress tolerance test in durum wheat. Plant Growth Regul. (2002) ;36: (1):61–70. |
[12] | Denaxa N-K , Damvakaris T , Roussos PA . Antioxidant defense system in young olive plants against drought stress and mitigation of adverse effects through external application of alleviating products. Sci Hortic. (2020) ;259: :108812. https://doi.org/10.1016/j.scienta.2019.108812. |
[13] | Chaparzadeh N , D’Amico ML , Khavari-Nejad R-A , Izzo R , Navari-Izzo F . Antioxidative responses of Calendula officinalis under salinity conditions. Plant Physiol Bioch. (2004) ;42: (9):695–701. |
[14] | Jana S , Choudhuri MA . Glycolate metabolism of three submersed aquatic angiosperms: effect of heavy metals. Aquatic Bot. (1981) ;11: :67–77. |
[15] | Roussos PA . Phytochemicals and antioxidant capacity of orange (Citrus sinensis (l.) Osbeck cv. Salustiana) juice produced under organic and integrated farming system in Greece. Sci Hortic. (2011) ;129: (2):253–8. |
[16] | Sarjala T , Kaunisto S . Needle polyamine concentrations and potassium nutrition in Scots pine. Tree Physiol. (1993) ;13: (1):87–96. |
[17] | Tsafouros A , Frantzeskaki A , Assimakopoulou A , Roussos PA . Spatial and temporal changes of mineral nutrients and carbohydrates in cuttings of four stone fruit rootstocks and their contribution to rooting potential. Sci Hortic. (2019) ;253: :227–40. |
[18] | de Azevedo Neto AD , Prisco JT , Enéas-Filho J , de Abreu CEB , Gomes-Filho E . Effect of salt stress on antioxidative enzymes and lipid peroxidation in leaves and roots of salt-tolerant and salt-sensitive maize genotypes. Environ Exp Bot. (2006) ;56: (1):87–94. |
[19] | Flurkey WH , Jen JJ . Peroxidase and polyphenol oxidase activities in developing peaches. J Food Sci. (1978) ;43: (6):1826–8. |
[20] | Ahmed S , Nawata E , Hosokawa M , Domae Y , Sakuratani T . Alterations in photosynthesis and some antioxidant enzymatic activities of mungbean subjected to waterlogging. Plant Sci. (2002) ;163: (1):117–23. |
[21] | Giannopolitis CN , Ries SK . Superoxide dismutases: I. Occurrence in higher plants. Plant Physiol. (1977) ;59: (2):309–14. |
[22] | Sairam R , Srivastava G . Changes in antioxidant activity in sub-cellular fractions of tolerant and susceptible wheat genotypes in response to long term salt stress. Plant Sci. (2002) ;162: (6):897–904. |
[23] | Rios-Gonzalez K , Erdei L , Lips SH . The activity of antioxidant enzymes in maize and sunflower seedlings as affected by salinity and different nitrogen sources. Plant Sci. (2002) ;162: (6):923–30. |
[24] | Bradford MM . A rapid and sensitive method for the quantitation of microgram quantities of protein utilizing the principle of protein-dye binding. Anal Biochem. (1976) ;72: (1-2):248–54. |
[25] | Ding Z-T , Ma Q-P , Wang Y . The differences between two tea varieties in their response to natural cold conditions. J HorticSci Biotech. (2016) ;91: (5):506–13. |
[26] | Wani MA , Jan N , Qazi HA , Andrabi KI , John R . Cold stress induces biochemical changes, fatty acid profile, antioxidant system and gene expression in Capsella bursa pastoris L. Acta Physiol Plant. (2018) ;40: (9):167. |
[27] | Zhu X-C , Song F-B , Xu H-W . Arbuscular mycorrhizae improves low temperature stress in maize via alterations in host water status and photosynthesis. Plant Soil. (2010) ;331: (1-2):129–37. |
[28] | Oh M-M , Trick HN , Rajashekar C . Secondary metabolism and antioxidants are involved in environmental adaptation and stress tolerance in lettuce. J Plant Physiol. (2009) ;166: (2):180–91. |
[29] | Król A , Amarowicz R , Weidner S . The effects of cold stress on the phenolic compounds and antioxidant capacity of grapevine (Vitis vinifera L.) leaves. J Plant Physiol. (2015) ;189: :97–104. |
[30] | Amarowicz R , Weidner S , Wójtowicz I , Karmac M , Kosinska A , Rybarczyk A . Influence of low-temperature stress on changes in the composition of grapevine leaf phenolic compounds and their antioxidant properties. Funct. Plant Sci. Biot. (2010) ;4: :90–96. |
[31] | Posmyk MM , Bailly C , Szafrańska K , Janas KM , Corbineau F . Antioxidant enzymes and isoflavonoids in chilled soybean (Glycine max (L.) Merr.) seedlings. J Plant Physiol. (2005) ;162: (4):403–12. |
[32] | Jan N , Majeed U , Andrabi KI , John R . Cold stress modulates osmolytes and antioxidant system in Calendula officinalis. Acta Physiol Plant. (2018) ;40: (4):73. |
[33] | Esra K , İşlek C , Üstün AS . Effect of cold on protein, proline, phenolic compounds and chlorophyll content of two pepper (Capsicum annuum L.) varieties. Gazi Univ J Sci. (2010) ;23: (1):1–6. |
[34] | Rivero RM , Ruiz JM , Garcıa PC , Lopez-Lefebre LR , Sánchez E , Romero L . Resistance to cold and heat stress: accumulation of phenolic compounds in tomato and watermelon plants. Plant Sci. (2001) ;160: (2):315–21. |
[35] | Pennycooke JC , Cox S , Stushnoff C . Relationship of cold acclimation, total phenolic content and antioxidant capacity with chilling tolerance in petunia (Petunia×hybrida). Environ Exp Bot. (2005) ;53: (2):225–32. https://doi.org/10.1016/j.envexpbot.2004.04.002. |
[36] | Hayat S , Hayat Q , Alyemeni MN , Wani AS , Pichtel J , Ahmad A . Role of proline under changing environments: a review. Plant Sign Behav. (2012) ;7: (11):1456–66. |
[37] | Meng X , Han J , Wang Q , Tian S . Changes in physiology and quality of peach fruits treated by methyl jasmonate under low temperature stress. Food Chem. (2009) ;114: (3):1028–35. |
[38] | Ortega-García F , Peragón J . The response of phenylalanine ammonia-lyase, polyphenol oxidase and phenols to cold stress in the olive tree (Olea europaea L.cv. Picual). J Sci Food Agric. (2009) ;89: (9):1565–73. |
[39] | Hashempour A , Ghasemnezhad M , Ghazvini RF , Sohani MM . Olive (Olea europaea L.) freezing tolerance related to antioxidant enzymes activity during cold acclimation and non acclimation. Acta Physiol Plant. (2014) ;36: (12):3231–41. |
[40] | Fan J , Ren J , Zhu W , Amombo E , Fu J , Chen L . Antioxidant responses and gene expression in bermudagrass under cold stress. J Am Soc Hortic Sci. (2014) ;139: (6):699–705. |
[41] | Chakraborty A , Bhattacharjee S . Differential competence of redox-regulatory mechanism under extremes of temperature determines growth performances and cross tolerance in two indica rice cultivars. J Plant Physiol. (2015) ;176: :65–77. |
[42] | Zhang X , Ervin E , LaBranche A . Metabolic defense responses of seeded bermudagrass during acclimation to freezing stress. Crop Sci. (2006) ;46: (6):2598–605. |
[43] | Choudhary DK , Kasotia A , Jain S , Vaishnav A , Kumari S , Sharma KP , et al. Bacterial-mediated tolerance and resistance to plants under abiotic and biotic stresses. J Plant Growth Regul. (2016) ;35: (1):276–300. |
[44] | Shen W , Nada K , Tachibana S . Involvement of polyamines in the chilling tolerance of cucumber cultivars. Plant Physiol. (2000) ;124: (1):431–40. |
[45] | Fu Y , Zhang Z , Liu J , Chen M , Pan R , Hu W , et al. Seed priming with spermidine and trehalose enhances chilling tolerance of rice via different mechanisms. J Plant Growth Regul. 2019:1-11. |
[46] | Ding F , Wang R . Amelioration of postharvest chilling stress by trehalose in pepper. Sci Hortic. (2018) ;232: :52–56. |
[47] | Akram NA , Waseem M , Ameen R , Ashraf M . Trehalose pretreatment induces drought tolerance in radish (Raphanus sativus L.) plants: some key physio-biochemical traits. Acta Physiol Plant. (2016) ;38: (1):3. |
[48] | Akram NA , Noreen S , Noreen T , Ashraf M . Exogenous application of trehalose alters growth, physiology and nutrient composition in radish (Raphanus sativus L.) plants under water-deficit conditions. Brazil J Bot. (2015) ;38: (3):431–39. |
[49] | Karlidag H , Yildirim E , Turan M . Exogenous applications of salicylic acid affect quality and yield of strawberry grown under antifrost heated greenhouse conditions. J Plant Nutrand Soil Sci. (2009) ;172: (2):270–76. |
[50] | Karlidag H , Yildirim E , Turan M . Salicylic acid ameliorates the adverse effect of salt stress on strawberry. Scientia Agric. (2009) ;66: (2):180–87. |
[51] | Min K , Showman L , Perera A , Arora R . Salicylic acid-induced freezing tolerance in spinach (Spinacia oleracea L.) leaves explored through metabolite profiling. Environ Exp Bot. (2018) ;156: :214–27. |
[52] | Wang W , Wang X , Huang M , Cai J , Zhou Q , Dai T , et al. Hydrogen peroxide and abscisic acid mediate salicylic acid-induced freezing tolerance in wheat. Frontiers Plant Sci. (2018) ;9: :1137. |
[53] | Wang Y , Wen T , Huang Y , Guan Y , Hu J . Salicylic acid biosynthesis inhibitors increase chilling injury to maize (Zea mays L.) seedlings. Plant Growth Regul. (2018) ;86: (1):11–21. |
[54] | Kumar V , Yadav SK . Proline and betaine provide protection to antioxidant and methylglyoxal detoxification systems during cold stress in Camellia sinensis (L.) O. Kuntze. Acta Physiol Plant. (2009) ;31: (2):261–69. |
[55] | Aggarwal M , Sharma S , Kaur N , Pathania D , Bhandhari K , Kaushal N , et al. Exogenous proline application reduces phytotoxic effects of selenium by minimising oxidative stress and improves growth in bean (Phaseolus vulgaris L.) seedlings. Biol Trace Elem Res. (2011) ;140: (3):354–67. |
[56] | Mohammadrezakhani S , Hajilou J , Rezanejad F , Zaare-Nahandi F . Assessment of exogenous application of proline on antioxidant compounds in three Citrus species under low temperature stress. J Plant Interact. (2019) ;14: (1):347–58. |
[57] | Samadi S , Habibi G , Vaziri A . Effects of exogenous salicylic acid on antioxidative responses, phenolic metabolism and photochemical activity of strawberry under salt stress. Plant Physiol. (2019) ;9: (2):2685–94. |
[58] | Mustafa MA , Ali A , Seymour G , Tucker G . Delayed pericarp hardening of cold stored mangosteen (Garcinia mangostana L.) upon pre-treatment with the stress hormones methyl jasmonate and salicylic acid. Sci Hortic. (2018) ;230: :107–16. |
[59] | Yao W , Xu T , Farooq SU , Jin P , Zheng Y . Glycine betaine treatment alleviates chilling injury in zucchini fruit (Cucurbita pepo L.) by modulating antioxidant enzymes and membrane fatty acid metabolism. Postharv Biol Techn. (2018) ;144: :20–28. |
[60] | Ali Q , Ashraf M . Induction of drought tolerance in maize (Zea mays L.) due to exogenous application of trehalose: growth, photosynthesis, water relations and oxidative defence mechanism. J Agron Crop Sci. (2011) ;197: (4):258–71. |
[61] | Siboza XI , Bertling I , Odindo AO . Salicylic acid and methyl jasmonate improve chilling tolerance in cold-stored lemon fruit (Citrus limon). J Plant Physiol. (2014) ;171: (18):1722–31. |
[62] | Wang L , Shan T , Xie B , Ling C , Shao S , Jin P , et al. Glycine betaine reduces chilling injury in peach fruit by enhancing phenolic and sugar metabolisms. Food Chem. (2019) ;272: :530–38. |
[63] | Kaur S , Gupta A , Kaur N , Sandhu J , Gupta S . Antioxidative enzymes and sucrose synthase contribute to cold stress tolerance in chickpea. J Agron Crop Sci. (2009) ;195: (5):393–97. |
[64] | Luo Y , Su Z , Bi T , Cui X , Lan Q . Salicylic acid improves chilling tolerance by affecting antioxidant enzymes and osmoregulators in sacha inchi (Plukenetia volubilis). Brazil J Bot. (2014) ;37: (3):357–63. |
[65] | Abdallah M-S , Abdelgawad Z , El-Bassiouny H . Alleviation of the adverse effects of Salinity stress using trehalose in two rice varieties. South Afric J Bot. (2016) ;103: :275–82. |
[66] | Aras S , Eşitken A , editors. Effects of antifreeze proteins and glycine betaine on strawberry plants for resistance to cold temperature. Proc Intern Conf Agric Biotechn. 2013; pp. 29-30. |
[67] | Shahid MA , Balal RM , Pervez MA , Abbas T , Aqeel MA , Javaid MM , et al. Exogenous proline and proline-enriched Lolium perenne leaf extract protects against phytotoxic effects of nickel and salinity in Pisum sativum by altering polyamine metabolism in leaves. Turk J Bot. (2014) ;38: (5):914–26. |
[68] | Wei Y , Liu Z , Su Y , Liu D , Ye X . Effect of salicylic acid treatment on postharvest quality, antioxidant activities, and free polyamines of asparagus. J Food Sci. (2011) ;76: (2):S126–S32. |
[69] | Kramer GF , Wang CY . Correlation of reduced chilling injury with increased spermine and spermidine levels in zucchini squash. Physiol Plant. (1989) ;76: (4):479–84. |
[70] | Li Z , Zhou H , Peng Y , Zhang X , Ma X , Huang L , et al. Exogenously applied spermidine improves drought tolerance in creeping bentgrass associated with changes in antioxidant defense, endogenous polyamines and phytohormones. Plant Growth Regul. (2015) ;76: (1):71–82. |
[71] | Kasukabe Y , He L , Nada K , Misawa S , Ihara I , Tachibana S . Overexpression of spermidine synthase enhances tolerance to multiple environmental stresses and up-regulates the expression of various stress-regulated genes in transgenic Arabidopsis thaliana. Plant Cell Physiol. (2004) ;45: (6):712–22. |