Inhibition of NF-κB pathway in LPS-stimulated THP-1 monocytes and COX-2 activity in vitro by berry pomace extracts from five Vaccinium species
Abstract
BACKGROUND:
Berry processing residues called pomaces are rich in polyphenols, sugars, organic acids, and minerals. Polyphenols are reported to reduce the risk of non-communicable diseases, including cardiovascular diseases, cancer, and diabetes mellitus, owing to their anti-inflammatory activity.
OBJECTIVE:
We aimed to assess the anti-inflammatory properties of five Vaccinium spp. berry pomace extracts using LPS-stimulated THP-1 monocytes and a COX-2 inhibition assay.
METHODS:
THP-1 monocytes were pre-incubated with chemically characterized bilberry, blueberry, American cranberry, bog cranberry, and lingonberry pomace extracts following LPS stimulation. NF-κB nuclear translocation was assessed by flow cytometry. TNF-α, MMP-9, IL-23, IL-10, IL-1β, CCL22, and IL-8 secretion was analyzed in cell culture supernatant by using Luminex assay and ELISA. The expression of IL-6, TNF-α, IL-10, IL-23, and TGF-β1 was assessed by qPCR, and COX-2 activity was determined by a fluorometric inhibition assay.
RESULTS:
All berry pomace extracts tested, except for lingonberry, inhibited LPS-induced NF-κB nuclear translocation. All five extracts significantly downregulated the expression of pro-inflammatory cytokines and inhibited COX-2 activity in vitro.
CONCLUSIONS:
Vaccinium spp. pomace extracts demonstrated anti-inflammatory properties in an LPS-stimulated THP-1 cell inflammation model and inhibited COX-2 activity. Thus, Vaccinium spp. berry pomace extracts could serve as a valuable source of anti-inflammatory compounds.
1Introduction
Fresh berries and processed berry products are a significant part of the human diet. The consumption of berries has increased during the past few years owing to the growing evidence for the beneficial health effects of the bioactive compounds in berries [1]. Furthermore, berries are exposed to a minimal level of pesticides during cultivation, which makes them an attractive source of healthy nutrients [2]. Berries and the residues from berry processing, called pomaces, are rich in a variety of phytochemicals, such as polyphenols, sugars, organic acids, and minerals [1, 3–6]. Polyphenols comprise a wide variety of compounds that are divided into several classes, such as hydroxybenzoic acids, hydroxycinnamic acids, anthocyanins, proanthocyanidins, flavonols, flavones, flavanols, flavanones, isoflavones, stilbenes, and lignans [7]. A substantial number of studies has shown that polyphenols reduce the risks of cardiovascular diseases, cancer, diabetes mellitus, neurodegeneration, and premature aging through their antioxidant and anti-inflammatory activity [7–10].
Inflammation is defined as the innate immune response to harmful stimuli such as pathogens, injury, and metabolic stress. It is believed to be a critical factor and unifying pathology for all non-communicable diseases [11, 12]. The mechanism of inflammation represents a chain of organized, dynamic responses that includes both cellular and vascular events with specific humoral secretions [13]. The inflammatory response is mediated by the innate immune system, which includes polymorphonuclear leukocytes and monocytes/macrophages, and the mediators released by these cells, such as cytokines, histamine, bradykinin, prostaglandins, leukotrienes, platelet activating factor, complement components, and inflammasomes [11]. It has been proposed that flavonoids exert anti-inflammatory activity through various mechanisms of action [14]. Polyphenol anti-inflammatory activities include the inhibition of enzymes associated with pro-inflammatory properties, such as cyclooxygenase-2 (COX-2), lipoxygenase (LOX), and inducible nitric oxide synthase (iNOS), nuclear factor kappa-light-chain-enhancer of activated B cells (NF-κB) and activating protein-1 (AP-1), the activation of phase-II antioxidant detoxifying enzymes, and the activation of mitogen activated protein kinase (MAPK), protein kinase-C, and nuclear factor erythroid 2-related factor (Nrf-2) [14].
NF-κB represents a family of inducible transcription factors that induce the expression of various pro-inflammatory molecules, including cytokines (IL-1, IL-6, IL-8, and chemokines), arachidonic acid metabolites, and NO [15, 16]. Therefore, the inhibition of NF-κB may be a useful strategy for the treatment of inflammatory disorders [15, 17]. COX-2 is a member of the COX enzyme family, which is responsible for the production of arachidonic acid metabolites such as prostaglandins (PGs) and thromboxane A2 (TXA2); the expression of proteins in this family is elicited by bacterial lipopolysaccharide (LPS) and pro-inflammatory cytokines. The ability of polyphenols to reduce the synthesis of prostaglandins and leukotrienes is considered to be one of the key mechanisms of their anti-inflammatory action [18].
Circulating monocytes are key players in the host defense against pathogens and the modulation of their function is therefore a suitable approach for anti-inflammatory treatment [19]. LPS-stimulated monocytes have been used in numerous studies to elucidate the anti-inflammatory activity of various compounds, including plant-derived polyphenols [18]. LPS, the major component of gram-negative bacterial cell walls, binds to the TLR4 receptor at the cell surface of monocytes and macrophages, prompting a downstream signaling cascade that activates MAPKs and NF-κB transcription factors. As a result, the production of a plethora of inflammatory cytokines, such as TNF-α, IL-1β, IL-6, IL-8, IL-10, and TGF-β, is induced [20].
Polyphenolic compounds, present widely in fruits, vegetables, and cereal grains, have been shown to modulate different inflammatory mediators, such as cytokines, peptides, and arachidonic acid [21]. Previously we have shown that high amounts of polyphenols could be extracted from Vaccinium spp. berry pomaces making them as an attractive source of bioactive compounds [22].
In the following studies of berry pomace extracts, the major constituents of five Vaccinium spp. berry pomace extracts, namely bilberry (Vaccinium myrtillus L.), highbush blueberry (Vaccinium corymbosum L.), American cranberry (Vaccinium macrocarpon Ait.), bog cranberry (Vaccinium oxycoccos L.), and lingonberry (Vaccinium vitis-idaea L.) were evaluated [23]. First, the total anthocyanin, procyanidin, and polyphenol contents in berry residues were measured by spectrophotometric pH-differential, 4-dimethylaminocinnamaldehyde, and Folin-Ciocalteu methods, respectively. Second, the identification and quantification of 37 separate compounds were performed by using ultra-performance liquid chromatography and liquid chromatography-time-of-flight mass spectroscopy [23]. Blueberry pomace extract contained the highest amount of anthocyanins, whereas bog cranberry pomace extract contained more procyanidins than the other Vaccinium spp. extracts [23].
The high polyphenol content in Vaccinium spp. berry pomace extracts prompted us to characterize the anti-inflammatory properties of these extracts on LPS-stimulated THP-1 monocytes and the enzyme COX-2. Berry processing pomaces with anti-inflammatory properties could serve as a valuable supplement to a healthy diet and as basis for an ecological food production system, supporting health and environmental sustainability [24].
The aim of the current study was to demonstrate the anti-inflammatory properties of previously chemically characterized berry pomace extracts in vitro by using an LPS-stimulated monocytic THP-1 cell line and a COX-2 inhibition assay as a proof-of-concept model.
2Materials and methods
2.1Cell culture
The human monocytic leukemia cell line THP-1 was obtained from the European Collection of Authenticated Cell Cultures (ECACC 88081201). THP-1 cells were propagated in RPMI-1640 culture medium (Sigma-Aldrich, MO, USA) supplemented with 10% fetal calf serum (FBS, Sigma-Aldrich), penicillin-streptomycin 100 U/mL-100μg/mL (ThermoFisher Scientific, MA, USA), and 25 mM HEPES (Sigma-Aldrich) (complete growth medium) in T25 tissue culture flasks (Sarstedt, Nümbrecht, Germany) at 37°C and 95% humidity. Cell morphology was observed by using a digital inverted microscope (AMG-Evos X1; AMG, WA, USA). THP-1 cells were subcultured when the cell concentration reached 8×105 cells/mL. Cells were stained with 0.4% Trypan blue solution (Sigma-Aldrich) for the assessment of viability and a viability of≥95% was considered acceptable for further experiments.
2.2Berry extracts
Five Vaccinium berry species – bilberries (extract 1), highbush blueberries (extract 2), American cranberries (extract 3), bog cranberries (extract 4) and lingonberries (extract 5) – were harvested; the berry residues were extracted and purified, and their chemical composition characterized as described in Klavins et al. [22], Muceniece et al. [23]. The extracts were dissolved in methanol to form 100 mg/mL stock solutions.
2.3Cell viability assay
Berry pomace extract stock solutions were diluted with complete growth medium to obtain the desired extract concentrations. To assess the effect of Vaccinium sp. berry pomace extracts on THP-1 cells, the lactate dehydrogenase assay (LDH Cytotoxicity Kit I (fluorometric), Promokine, Heidelberg, Germany) and ViaCount assay (Merck Millipore, MA, USA) were used in accordance with the manufacturers’ recommendations. THP-1 cells (5×104) in 100μL complete growth medium/well were seeded in 96-well cell cultivation plates (Sarstedt, Nümbrecht, Germany). Berry pomace extracts were tested at concentrations of 0.04 mg/mL, 0.2 mg/mL, and 1 mg/mL. The experimental controls comprised high control, containing 10μL cell lysis solution/well, and low control, containing 100μL complete growth medium without cells. THP-1 cells were incubated with test substances for 1.5 h. After incubation, 5μL of the supernatant from each well was transferred into a new 96-well flat-bottomed white plate (PerkinElmer Inc. Waltham, MA, USA) and 95μL of LDH reaction mix was added to each well. After incubation for 10 min, the fluorescence (excitation/emission wavelengths of 535/587 nm) was measured by using a Tecan Infinite M200 Pro microplate reader (Switzerland). Three biological replicates of the experiment were performed and each measurement was conducted in triplicate.
After incubation with the treatments, THP-1 cells were aspirated from the plate and stained with ViaCount reagent. Cells were analyzed by using a Guava EasyCyte 8HT flow cytometer and ExpressPro software (Merck Millipore, MA, USA). Three biological replicates of the experiment were performed and each measurement was conducted in triplicate.
2.4Analysis of anti-inflammatory effect of berry pomace extract on LPS-stimulated THP-1 cells
THP-1 cells were seeded in a 24-well cell cultivation plate (Sarstedt, Nümbrecht, Germany) at a density of 1×105 cells/well in 0.5 mL of complete growth medium and incubated with berry pomace extracts as described earlier. Subsequently, the inflammatory response in THP-1 cells was induced by the addition of 1μg/mL (final concentration) of bacterial LPS (Sigma-Aldrich) to the control wells and berry pomace extract-containing wells. After the addition of LPS, the samples were incubated for 1 h at 37°C and 95% humidity. THP-1 cells and supernatants were then collected and used for further analysis.
2.5Flow cytometric analysis of NF-κB nuclear translocation
After incubation with berry pomace extracts and/or 1μg/mL LPS, THP-1 cells were resuspended by pipetting and collected in 1.5 mL microcentrifuge tubes (Sarstedt, Nümbrecht, Germany). The collected samples were centrifuged at 1500 rpm (183 g) for 5 min, the supernatant was removed, and the cells were fixed with 0.1 mL Cytofix fixation buffer solution (BD Bioscience, CA, USA) for 10 min at 37°C. The cells were then washed in wash buffer (BD Bioscience, CA, USA), centrifuged at 1500 rpm (183 g) for 5 min, and the supernatant was removed. The cells were permeabilized for 10 min at room temperature of 23 °C in 0.1 mL perm/wash buffer solution, containing saponin and FBS, in accordance with the manufacturer’s recommendations (BD Bioscience, CA, USA). After permeabilization, the cells were washed and incubated with 0.5μg (5μg/mL) of human Fc blockTM reagent that blocks non-specific antibody binding to Fc receptors on THP-1 cell surface (BD Bioscience, CA, USA) for 10 min at room temperature. Next, 20μL of PE Mouse anti-NF-κB p65 (pS529) antibody (BD Bioscience, CA, USA) was added to the samples and incubated for 15 min at room temperature in the dark. Samples were centrifuged at 2000 rpm (326 g) for 3 min, washed with 1 mL of 1% FBS (Sigma-Aldrich) in phosphate-buffered saline (PBS, Sigma-Aldrich), and the nuclei were stained with 5μM DRAQ5 fluorescent probe solution (ThermoFisher Scientific) for 15 min at room temperature in the dark. Flow cytometry data were acquired by using a Guava EasyCyte 8HT flow cytometer and analyzed using ExpressPro software (Merck Millipore, MA, USA). The effect of berry pomace extract and LPS on NF-κB nuclear translocation was studied in a minimum of three biological replicates.
2.6IL-8 ELISA
IL-8 secretion in berry pomace extract and LPS-stimulated THP-1 cells was analyzed by using the human IL-8/CXCL8 DuoSet ELISA kit in accordance with the manufacturer’s recommendations (R&D Systems, MN, USA). The supernatant was collected from THP-1 cells treated with 1, 0.2, and 0.04 mg/mL berry pomace extracts and/or 1μg/mL LPS. Briefly, 96-well plates were coated with capture antibodies overnight. The cell culture supernatant was diluted two-fold with the 1% bovine serum albumin (Sigma-Aldrich) in PBS and incubated for 2 h at room temperature. The supernatant was aspirated and the plates were washed three times with wash buffer (0.05% Tween 20 in PBS). After the washing step, the samples were incubated with biotinylated detection antibodies for 2 h. After rigorous washing, a streptavidin-horseradish peroxidase conjugate was added to the plates. After incubation for 20 min, the plates were washed again and the substrate solution was applied. The reaction was stopped by the addition of 2 N sulfuric acid and the absorbance at 450 nm was measured by using a Tecan Infinite 200 Pro microplate reader and i-control software (Tecan Trading AG, Männedorf, Switzerland). IL-8 secretion in berry pomace extract- and LPS-treated cells was studied in a minimum of three biological replicates.
2.7Cytokine secretion analysis by Luminex
A Magnetic Luminex Assay panel of nine cytokines and chemokines, namely CCL22, CXCL8/IL-8, IL-10, IL-6, TNF-α, CXCL10/IP10, IL-1β, IL-23, and MMP-9, was used for analysis of cell culture supernatants from THP-1 monocytes treated with 0.2 and 1 mg/mL berry pomace extracts and/or 1μg/mL LPS in accordance with the manufacturer’s instructions (R&D Systems). Briefly, cell culture supernatants were diluted two-fold and incubated with antibody-coated microparticles for 2 h at room temperature. After a wash step, the samples were incubated with biotinylated antibodies. After another wash step, a streptavidin-phycoerythrin conjugate was added. After the final wash step, the microparticles were resuspended in assay buffer and analyzed by using the Luminex 200 detection platform. Luminex data were analyzed by using GraphPad Prism software (GraphPad Software Inc., USA). The cytokine secretion patterns of berry pomace extract- and LPS-treated cells were studied in three biological replicates.
2.8RNA extraction
THP-1 cells treated with 1 or 0.2 mg/mL berry pomace extracts and LPS were lysed and RNA was extracted with QIAzol lysis reagent (Qiagen, Hilden, Germany) in accordance with the manufacturer’s instructions. In brief, 0.1 ml of chloroform (Sigma-Aldrich) was added to the cell lysates and vortexed for 15 s, samples were then incubated for 5 min at room temperature and centrifuged for 15 min at 12 000 g at 4°C. The aqueous phase was transferred to a new tube and 0.25 ml of isopropanol was added. Samples were incubated for 10 min at room temperature and centrifuged for 10 min at 12 000 g at 4°C. Isopropanol was discarded and RNA pellet was resuspended in 0.5 ml of 75 % ethanol and centrifuged for 7 min at 7500 g at 8°C. Ethanol was discarded and pellet was air-dried for 10 min and then resuspended in RNA-free water. The concentration and purity of RNA were determined by using a Tecan Infinite M200 Pro microplate reader and i-control software. The RNA concentration was normalized to 500 ng/μL for all samples.
2.9Real-time RT-PCR analysis
cDNA was synthesized from extracted RNA by using the FIREScript RT cDNA Synthesis Kit in accordance with the manufacturer’s instructions. Real-time RT-PCR was performed for quantitative gene expression analysis using SYBR Green fluorescent dye in accordance with the manufacturer’s instructions. All reagents were obtained from Solis Biodyne (Tartu, Estonia). The Ct values were normalized to the average Ct value of the housekeeping gene, GAPDH. The relative gene expression of the extract/LPS or LPS-treated samples was expressed as the log2-fold-change (-ΔΔCt) and compared with the expression in untreated samples (negative control), for which the relative gene expression was set to zero. The fold-change in gene expression was calculated by using the ΔΔCt method [25].
The following primer sets were used:
IL-6: Forward: 5’-GACAAACAAATTCGGTACATCC-3’,
Reverse: 5’-GCAAGTCTCCTCATTGAATCC-3’;
TGF-β1: Forward: 5’- ACTGCAAGTGGACATCAACG-3’,
Reverse: 5’- TGCGGAAGTCAATGTACAGC-3’;
TNF-α: Forward: 5’- CCCAGGCAGTCAGATCATCTTC-3’,
Reverse: 5’- AGCTGCCCCTCAGCTTGA-3’;
IL-10: Forward: 5’- CCTGCCTAACATGCTTCGAG-3’,
Reverse: 5’- CAACCCAGGTAACCCTTAAAGTC-3’;
IL-23: Forward: 5’- GCTGTAATGCTGCTGTTGCT-3’,
Reverse: 5’- CTTCTCTTAGATCCATGTGTCCC-3’.
2.10COX-2 inhibition assay
The extracts were dissolved to a concentration 100 mg/mL in methanol and then diluted with 0.1 M PBS, pH 7.0, to obtain desired concentrations of 0.04, 0.2, and 1mg/mL. The highest methanol concentration (1%) was used as the reagent control. Celecoxib (Sigma-Aldrich) was used as a positive reference compound at concentration 0.45μM. Measurements of the inhibitory effects of berry pomace extract on COX-2 and the calculations were performed in accordance with the kit instructions (BioVision Catalog #K547-100; BioVision Inc., Milpitas, CA, USA). The celecoxib concentration was chosen in accordance with the manufacturer’s suggestion of the IC50. The extracts were tested in triplicate and the experiments were repeated three times. Samples without extracts and celecoxib were considered the 0% inhibition control. The fluorescence of the samples was measured at excitation/emission wavelengths of 535/587 nm in kinetic mode, for 5– 30 min at 25°C and protected from light, by using an Infinite 200 PRO microplate reader and i-control software (Tecan Trading AG, Switzerland).
2.11Statistical analysis
The data are presented as mean±standard deviation (SD) (n≥3). Statistical analyses were performed by one-way analysis of variance (ANOVA) followed by Dunnett’s multiple comparison test. Significant differences between the groups were accepted for P values of≤0.05. The statistical analyses were computed by using GraphPad Prism 7 software (GraphPad Software Inc., San Diego, CA, USA), and statistical significance was expressed as * P < 0.05, ** P < 0.01, and *** P < 0.001.
3Results
3.1The effect of Vaccinium spp. extracts on THP-1 viability
The effect of 0.04, 0.2, and 1 mg/mL berry pomace extract on THP-1 cell viability was tested to find the optimal concentration for the measurement of the inhibition of NF-κB nuclear translocation in LPS-stimulated human monocytic THP-1 cells.
The results from the fluorescent LDH cytotoxicity assay, which measure the release of LDH from damaged cells, demonstrated that none of the five extracts were cytotoxic to THP-1 cells at concentrations of 0.04, 0.2, and 1 mg/mL (data not shown). To confirm this, a flow cytometry-based cytotoxicity assay (ViaCount) was performed. The ViaCount assay data showed that there was a slight decrease in cell viability at the highest extract concentration (1 mg/mL) for bog cranberry pomace extract (to 87% viability) and lingonberry extract (to 95% viability); however, this was considered acceptable for further experiments (Fig. 1).
Fig. 1
THP-1 cell viability after incubation with bilberry, blueberry, American cranberry, bog cranberry, and lingonberry pomace extracts. A – cytotoxicity (ViaCount) assay results (n = 3), B – viability of THP-1 cells after incubation with 1 mg/mL berry pomace extracts in ViaCount flow cytometry assay. Filled histogram – cells without extract, gray lines – bilberry (1), blueberry (2), American cranberry (3), lingonberry (4), and dotted line – bog cranberry extract (5). Histogram marker shows cytotoxic events with bog cranberry extract. Representative data are shown. Statistical significance was expressed as *P < 0.05.
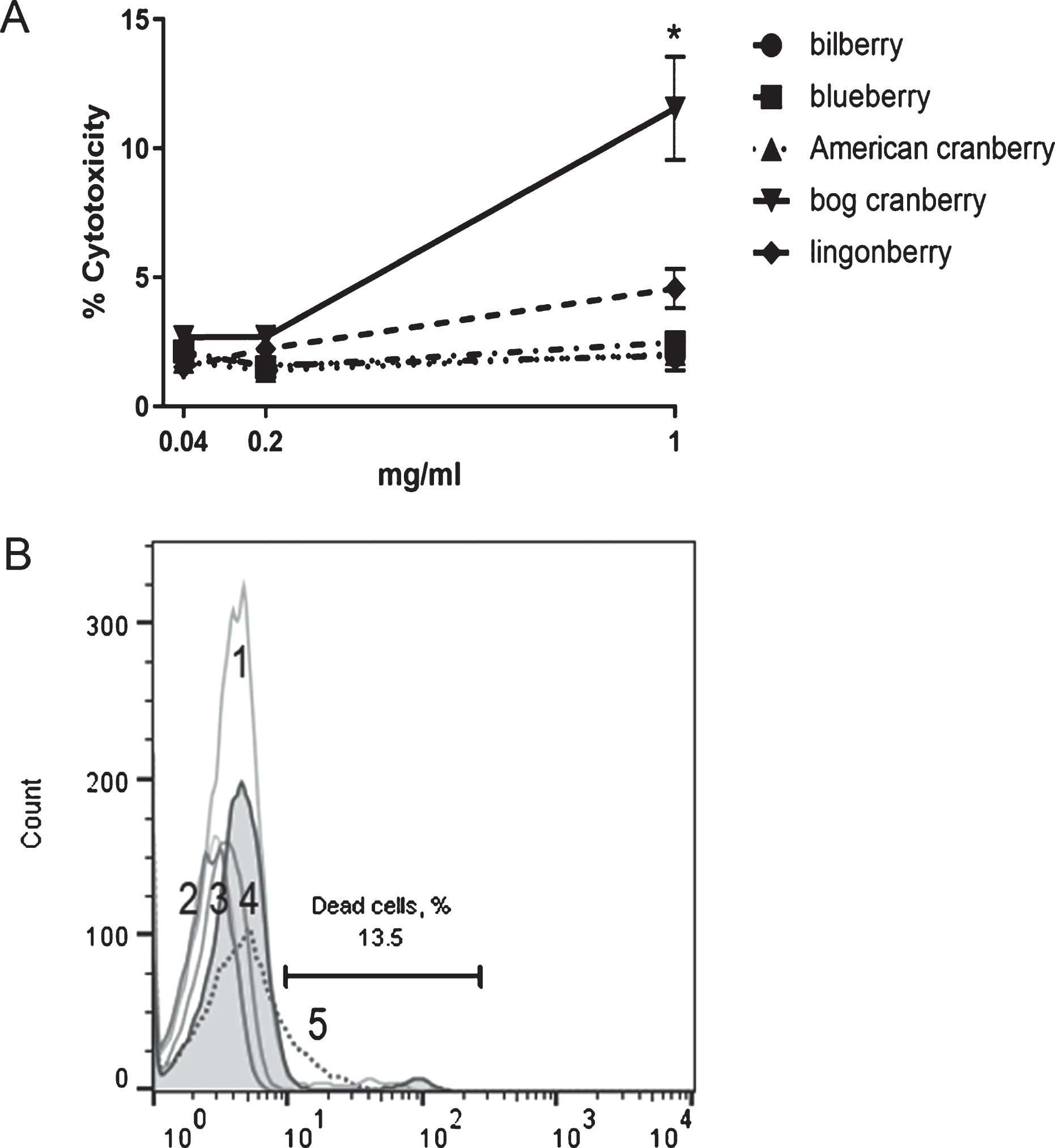
3.2Vaccinium spp. extracts inhibited LPS-induced NF-κB nuclear translocation in THP-1 cells
The effect of Vaccinium spp. berry pomace extracts on NF-κB nuclear translocation in LPS-stimulated THP-1 cells was analyzed by using flow cytometry. Based on the literature data and CCK-8 viability test results (data not shown), 1μg/mL was selected as the optimal LPS concentration for THP-1 stimulation experiments [26]. The optimal LPS incubation time was 1 h; at this time point, NF-κB nuclear translocation was induced in the greatest number of cells (Fig. 2).
Fig. 2
The dynamics of NF-κB nuclear translocation in LPS-stimulated THP-1 cells. A – the percentage of THP-1 cells with NF-κB nuclear translocation after LPS stimulus (THP-1 neg is the unstained control) (n = 3). B – flow cytometry results of anti-NF-κB p65 PE and DRAQ5 co-staining in unstimulated THP-1 cells (left) and LPS-stimulated cells (right). Representative data are shown. C – flow cytometry analysis of NF-κB nuclear translocation dynamics in THP-1 cells after stimulation with LPS. The filled histogram shows the unstained cell control, the black line shows NF-κB p65 PE staining in LPS-stimulated THP-1 cells, and the gray line shows NF-κB p65 PE staining in unstimulated THP-1 cells. Representative data are shown. Statistical significance was expressed as **P < 0.01 and ***P < 0.001 compared with the response of unstimulated THP-1 cells.
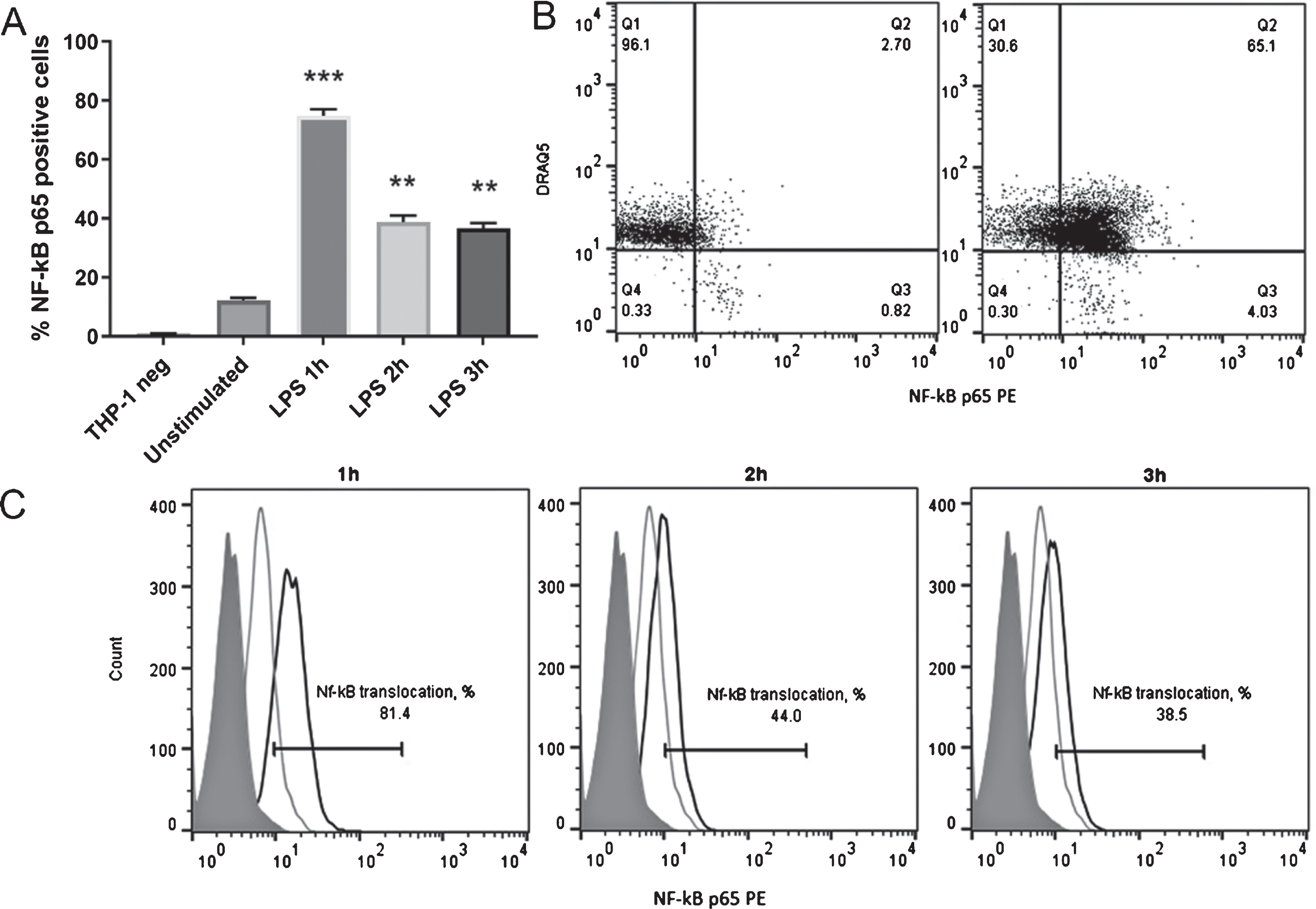
In LPS-induced (positively stained) THP-1 cells, NF-κB nuclear translocation could be detected in an average of 75% of cells, and varied between 60% and 85% of cells in all experiments (Fig. 2). NF-κB nuclear translocation was confirmed by nuclei co-staining with DRAQ5 (Fig. 2). In unstimulated (negative) cells, NF-κB nuclear translocation was always below 10% (Fig. 2), which confirmed the validity of the method for NF-κB induction.
The addition of the extract 2 (blueberry) inhibited LPS-induced NF-κB nuclear translocation in a concentration-dependent manner; for extracts 1, 3, and 4 (bilberry, American cranberry, and bog cranberry, respectively) the NF-κB translocation was inhibited only at the highest concentration 1 mg/mL (Fig. 3). For extracts 1 to 4, the highest NF-κB inhibition was observed at 1 mg/mL and was comparable with the negative (unstimulated) control cell level (0% – 10% NF-κB-positive cells, p < 0.001). Extract 5 (lingonberry) did not result in statistically significant inhibition of NF-κB nuclear translocation in LPS-stimulated THP-1 cells owing to the high variability between biological replicates (Fig. 3).
Fig. 3
The effect of five Vaccinium spp. berry pomace extracts on NF-κB nuclear translocation in LPS-stimulated cells. Negative indicates unstimulated THP-1 cells and positive indicates LPS-stimulated THP-1 cells. Statistical significance was expressed as *P < 0.05, **P < 0.01, and ***P < 0.001 compared with the response of LPS-stimulated THP-1 cells.
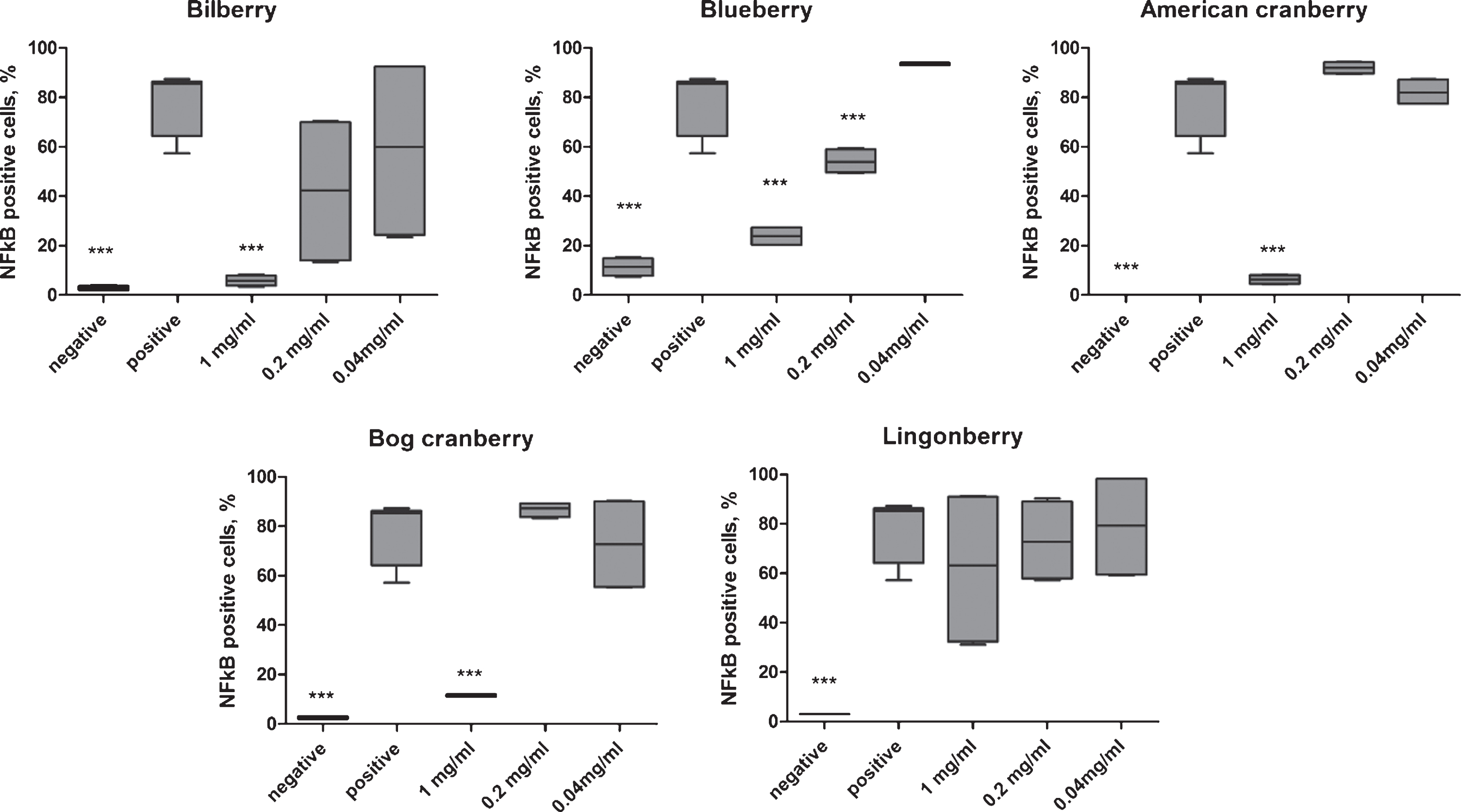
3.3The effect of Vaccinium spp. extracts on the secretory profile of LPS-stimulated THP-1 cells
The addition of LPS to THP-1 cells induced the translocation of the NF-κB complex to the nucleus and the subsequent activation of pro-inflammatory cytokine gene transcription. We analyzed IL-8 secretion in LPS-stimulated THP-1 cell culture supernatant at 1 h after stimulation by ELISA and TNF-α, MMP-9, IL-23, IL-10, IL-1β, CCL22 secretion by Luminex assay. Untreated THP-1 cells (negative) secreted 50 pg/mL IL-8. In LPS-treated THP-1 cells, IL-8 secretion increased up to 100 pg/mL. All berry pomace extracts significantly (p < 0.01, p < 0.001) inhibited IL-8 secretion at 0.04, 0.2, and 1 mg/mL compared with LPS-stimulated cells (positive control; Fig. 4). However, the inhibition of IL-8 secretion was lower for extract 4 (bog cranberry) at concentration 0.04 mg/mL (p < 0.01). At all concentrations tested, extracts 1, 2, 3, and 5 inhibited IL-8 secretion in LPS-stimulated THP-1 cells to the level of unstimulated cells (p < 0.001) (Fig. 4).
Fig. 4
Effect of Vaccinium spp. berry pomace extracts on IL-8 secretion in LPS-stimulated THP-1 monocytes. Negative indicates the IL-8 level in unstimulated THP-1 cells. LPS indicates the IL-8 level in LPS-stimulated THP-1 cells. The statistical significance of the effect of the extract is denoted as *P < 0.05, **P < 0.01, and ***P < 0.001 compared with the response of LPS-stimulated THP-1 cells.
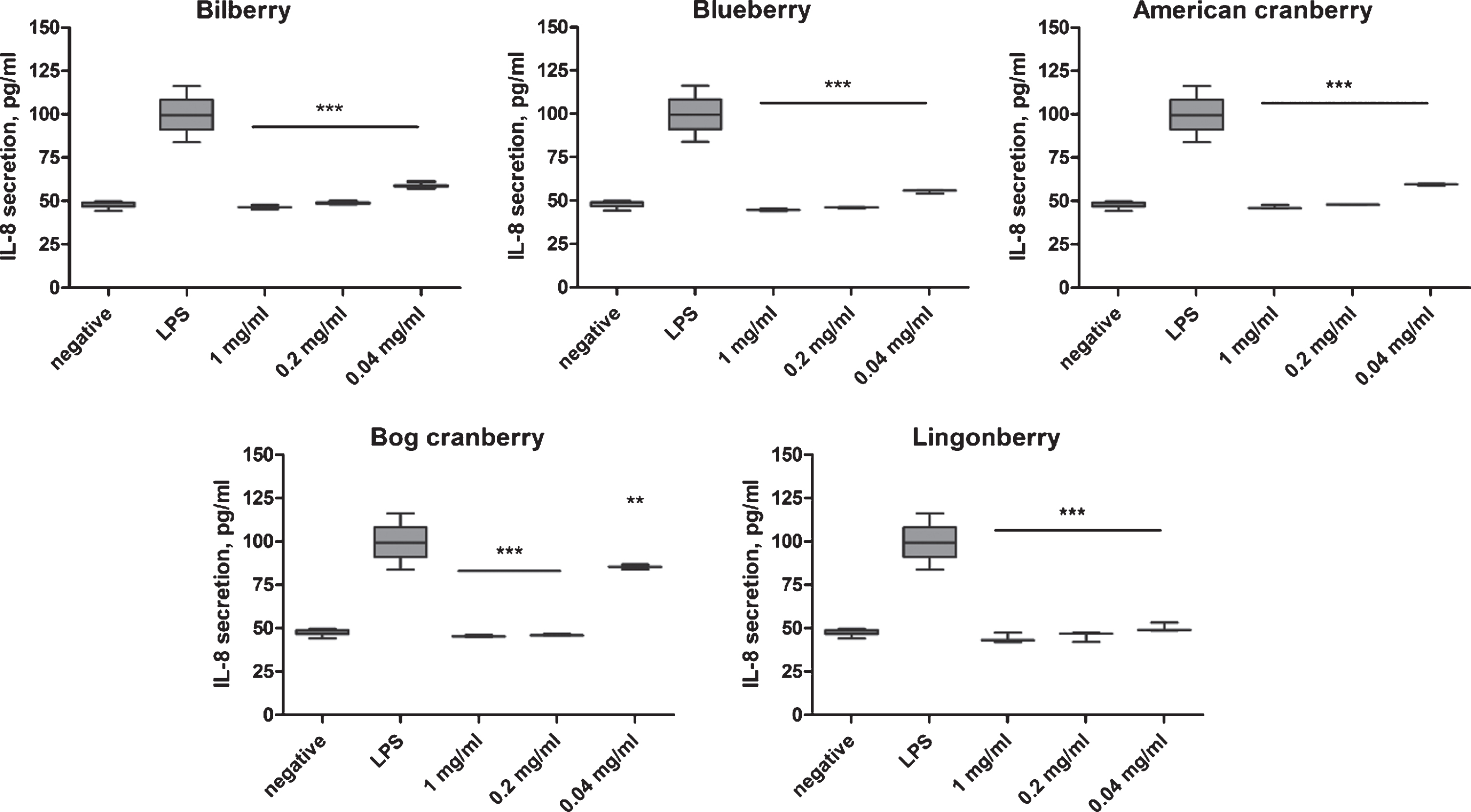
A broader analysis of cytokine secretion pattern, including TNF-α, MMP-9, IL-23, IL-10, IL-1β, and CCL22, of LPS-stimulated THP-1 cells was performed for at 0.2 and 1 mg/mL berry pomace extracts. After LPS stimulation, increased secretion of TNF-α, IL-23, MMP-9, and IL-1β was observed, with no induction of IL-10 and CCL-22 secretion (Fig. 5). In berry pomace extract-treated THP-1 cells stimulated with LPS, we observed a statistically significant inhibition of TNF-α, IL-1β, and IL-23 (Fig. 5). IL-10 secretion was decreased by extract 3 at 1 mg/mL and extract 5 at 0.2 mg/mL in the treated cell culture supernatants (Fig. 5). Interestingly, extracts 1 to 4 had opposing effects on MMP-9 secretion, depending on the concentration. At concentrations below 0.2 mg/mL, extracts 1 to 4 induced MMP-9 secretion; conversely, at 1 mg/mL extracts 1 to 4 downregulated MMP-9 secretion to the level of unstimulated cells (Fig. 5). However, treatment with extract 5 inhibited MMP-9 secretion at both concentrations. Collectively, our data showed a clear inhibitory effect on the LPS-induced pro-inflammatory cytokines, TNF-α, IL-8, IL-23, and IL-1β (Fig. 5).
Fig. 5
Effect of Vaccinium spp. berry pomace extracts on cytokine secretion in LPS-stimulated THP-1 monocytes as determined by Luminex assay. Extr 1 – bilberry, Extr 2 – blueberry, Extr 3 – American cranberry, Extr 4 – bog cranberry, Extr 5 – lingonberry. The statistical significance of the effect of the extract is denoted as *P < 0.05 and ***P < 0.001 compared with LPS-stimulated control cells (untreated with extract).
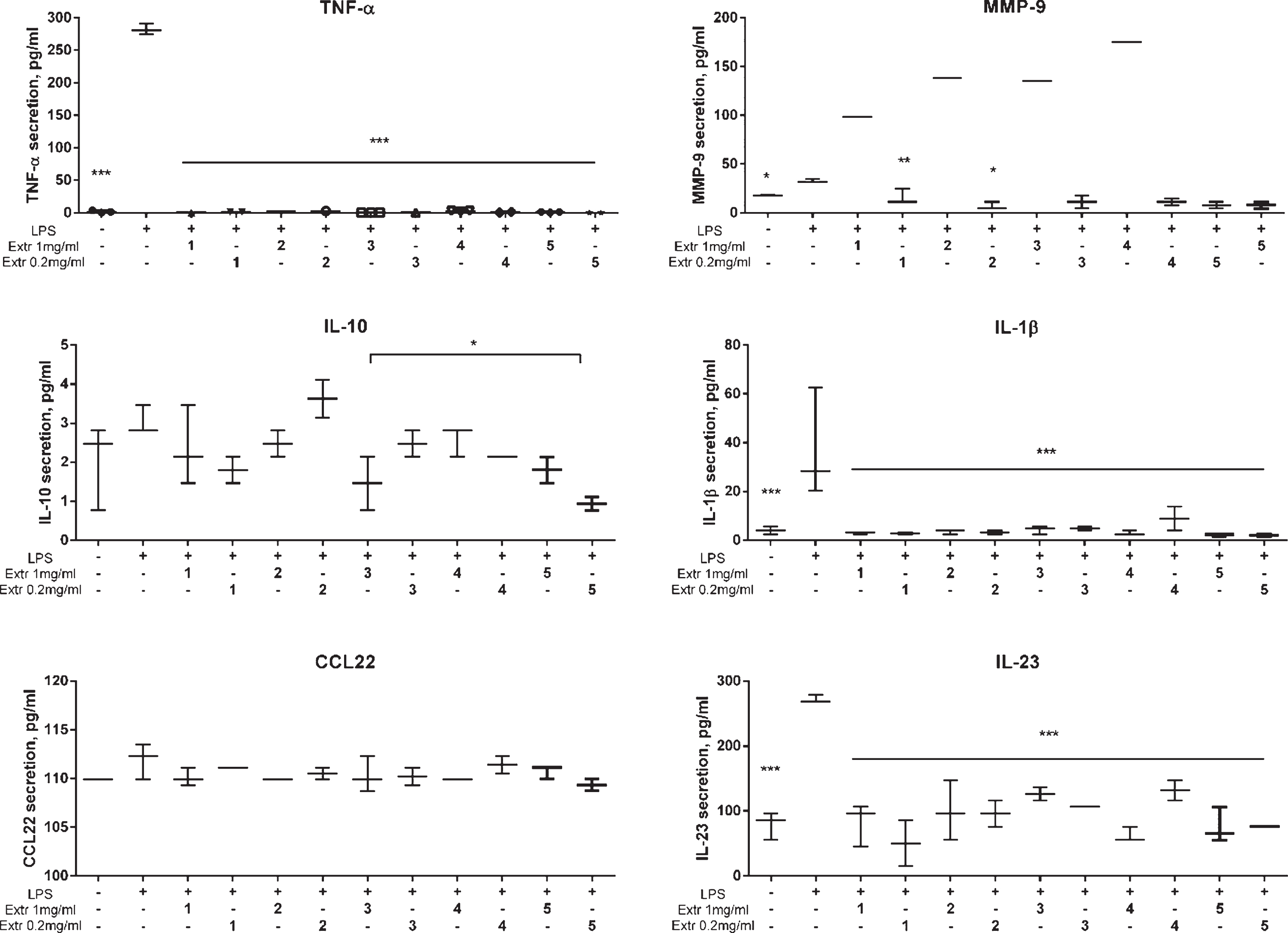
3.4The effect of Vaccinium sp. extracts on the expression of cytokine genes
The anti-inflammatory effect of Vaccinium spp. berry extracts on cytokine expression in LPS-stimulated THP-1 cells was studied at the transcript level. We performed real-time RT-PCR to analyze the expression of IL-6, TNF-α, IL-10, IL-23, and TGF-β1 in LPS-stimulated THP-1 cells with or without berry pomace extract treatment. Untreated samples (negative) were set as the baseline (level zero). The effects of the berry pomace extract were compared with the positive control (LPS-stimulated cells) (Fig. 6). LPS induced the expression of all genes except for TGF-β1. The analysis of cytokine secretion showed that LPS induced TNF-α and IL-23, whereas IL-10 was unchanged. The incubation of THP-1 cells with 0.02 and 1 mg/mL berry pomace extracts significantly downregulated the expression of IL-6, TNF-α, IL-10, and IL-23 compared with the expression in LPS-stimulated cells. For extract 2 (blueberry) the statistical significance of the downregulation of IL-6 expression at both concentrations could not be demonstrated as the threshold level was not reached. A potential explanation for this effect is that the absolute level of gene expression in the extract 2-treated samples was below the detection limit for the method used. Importantly, extract 2 (blueberry) inhibited the expression of TGF-β1, indicating that blueberry pomace extract could inhibit IL-6 and TGF-β1 expression at the transcript level.
Fig. 6
Effect of Vaccinium spp. berry pomace extract on the expression of cytokine genes in LPS-stimulated THP-1 monocytes. Extr 1 – bilberry, Extr 2 – blueberry, Extr 3 – American cranberry, Extr 4 – bog cranberry, Extr 5 – lingonberry. LPS indicates LPS-stimulated THP-1 cells. Statistical significance is denoted as *P < 0.05, ***P < 0.001 compared with LPS-stimulated control cells.
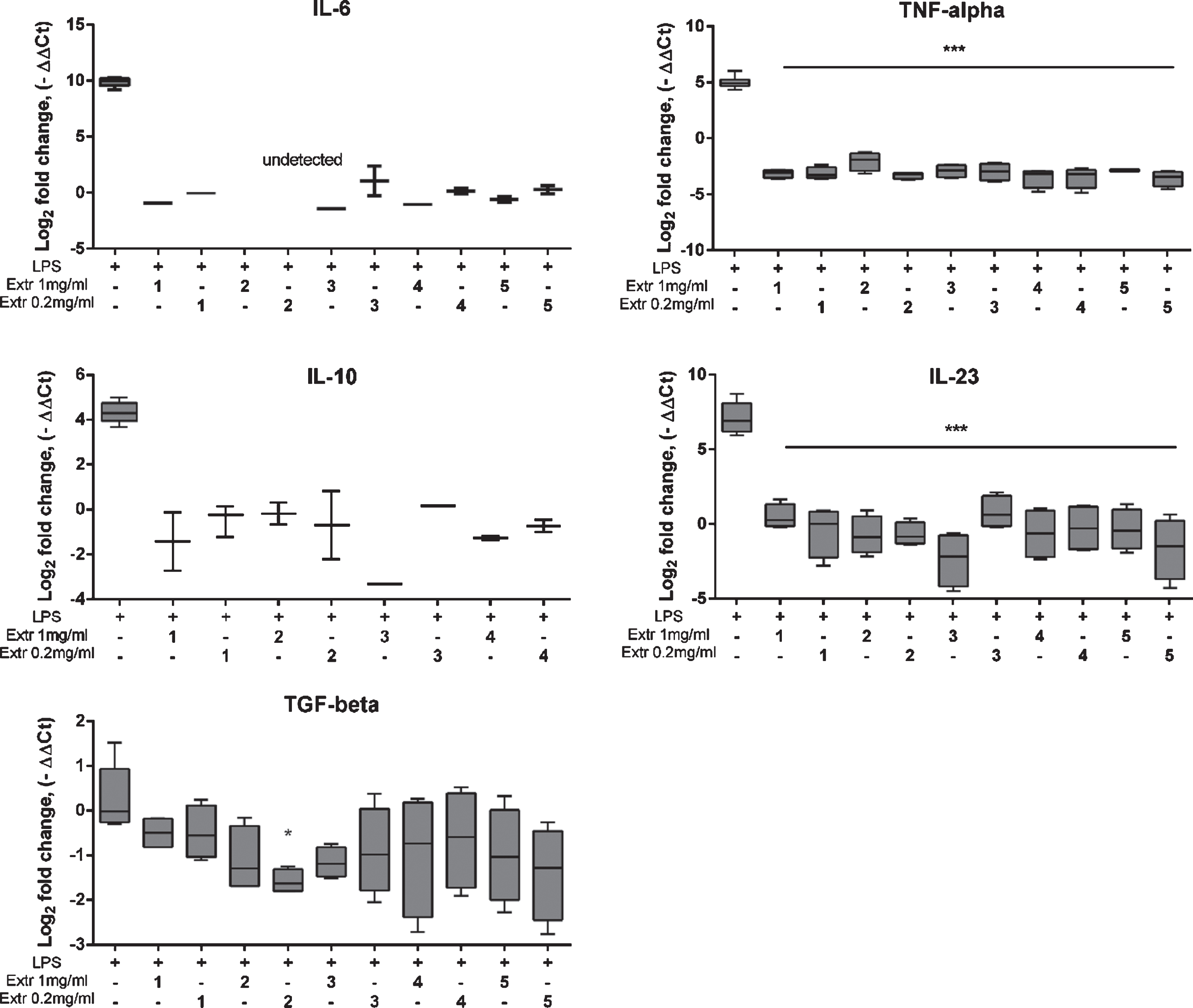
3.5Berry pomace extract effect on COX-2 inhibition
The inhibitory effect of bilberry (Extr 1), blueberry (Extr 2), American cranberry (Extr 3), bog cranberry (Extr 4), and lingonberry (Extr 5) pomace extracts on COX-2 was tested by using a fluorometric enzyme inhibition assay. All extracts resulted in concentration-dependent inhibitory effects on COX-2 (Fig. 7). At 1 mg/mL, more than 50% inhibition occurred, which was higher than (Extr 2 to 4) or equal to (Extr 5) the celecoxib-treated control. Only extract 2 (blueberry) exhibited 50% COX-2 inhibition at 0.2 mg/mL. The inhibitory effect of extract 1 (bilberry) was below 30% at all concentrations tested.
Fig. 7
Effect of Vaccinium spp. berry pomace extract effects on COX-2 enzymatic activity. Extr 1 – bilberry, Extr 2 – blueberry, Extr 3 – American cranberry, Extr 4 – bog cranberry, Extr 5 – lingonberry. Enzyme activity without extracts and celecoxib is taken as 0% inhibition. Statistical significance is denoted as ***P < 0.001 compared with enzyme activity without extract or celecoxib.
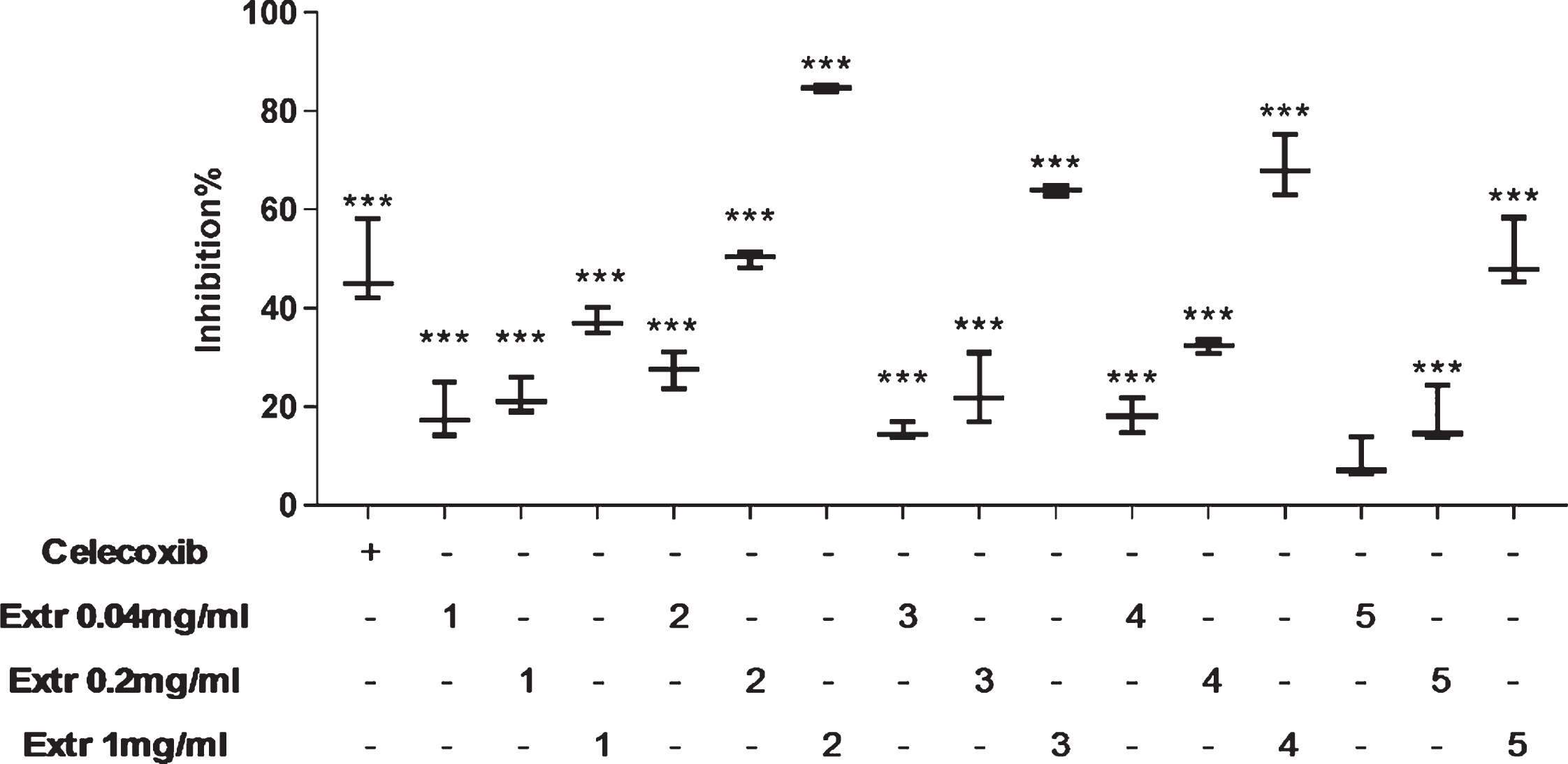
4Discussion
Vaccinium spp. berries are consumed and used widely in food production throughout the world, including Northern Europe and Baltic countries [27, 28]. Approximately 30% of the berry content, mainly skin and seeds, are left over as a presscake after berry juice processing and subsequently discarded as waste. Berry skins are rich in bioactive compounds, such as the anthocyanins that give the blue/purple color to berries [4]. Therefore, in view of the sustainable use of forest and agricultural resources, the identification of methods for bioactive compound extraction from pomace and implementation in healthy food supplements is a high priority. Previously, we showed that berry pomace extracts from Vaccinium spp. wild berries (bilberry, blueberry, American cranberry, bog cranberry, and lingonberry) contained high amounts of polyphenols, anthocyanins, and procyanidins [22, 23]. In the current study, we aimed to characterize the anti-inflammatory properties of the same five Vaccinium spp. berry pomace extracts by using the well-established in vitro inflammation model of LPS-stimulated human THP-1 monocytes [29]. The anti-inflammatory properties of five Vaccinium spp. berry pomace extracts were assessed in LPS-stimulated and berry pomace extract-pre-treated THP-1 cells through multiple measurements: NF-κB nuclear translocation activity; cytokine release; cytokine gene expression analysis; and the COX-2 enzyme inhibition assay.
First, we examined the cytotoxicity of the berry pomace extracts and found out that the extracts do not considerably alter the viability of THP-1 cells at concentrations of 1 mg/mL and below. Next, our data showed that bilberry, blueberry, American cranberry, and bog cranberry pomace extracts inhibited LPS-induced NF-κB nuclear translocation to the level of residual NF-κB nuclear translocation in unstimulated THP-1 cells (Fig. 3). Unexpectedly, blueberry pomace extract showed inhibitory effects, even at the lowest concentration tested (0.04 mg/mL) (Fig. 3). The finding that bilberry and blueberry extracts inhibited NF-κB nuclear translocation was in line with previous studies that used an LPS-induced human monocytic U937-3xκB-Luc cell model [30, 31]. Additionally, it has been shown that strawberry and Lonicera caerulea L. extracts protect human dermal fibroblasts and rodent macrophages against LPS induced inflammation by inhibiting NF-κB signaling pathways [32–34]. It is of interest that lingonberry pomace extract did not show a significant inhibitory effect on NF-κB translocation in LPS-stimulated THP-1 cells.
As found in previous studies, our results showed that LPS-stimulated THP-1 cells secreted pro-inflammatory cytokines, including TNF-α, IL-1β, IL-8, IL-23, and MMP-9 [35–37]. Unlike the previous studies that measured cytokine expression 3, 6, and 18 h after LPS induction [29, 36], we found that LPS stimulation for 1 h resulted in a sufficiently high level of cytokine secretion for detection using Luminex assay and conventional ELISA. This approach can be used to demonstrate that Vaccinium spp. berry pomace extracts have an early-preventive anti-inflammatory effect.
Despite the differences in Vaccinium spp. berry pomace extract content [22, 23] (Supplement 1), the inhibitory effect on the secretion of pro-inflammatory cytokines TNF-α, IL-1β, IL-8, IL-23, and MMP-9 from LPS-stimulated THP-1 cells was almost similar (Figs. 4 and 5). The inhibition of anti-inflammatory cytokine IL-10 secretion by American cranberry, bog cranberry, and lingonberry pomace extracts was significantly different from LPS-stimulated THP-1 cells; however, the level of IL-10 was low in general and the meaning of this effect is ambiguous. In addition, lingonberry pomace extract inhibited MMP-9 secretion at both concentrations tested, whereas extracts 1 to 4 exerted inhibitory effects only at the lowest concentration. MMP-9 expression is reported to be associated with the development of cardiovascular diseases [38]. Interestingly, it was found that cranberry juice consumption decreased MMP-9 plasma concentrations in abdominally obese men, which favors the hypothesis that the consumption of flavonoid-rich foods may have cardioprotective effects [39]. In addition, at concentration of 1 mg/mL, blueberry, American cranberry, and bog cranberry pomace extracts resulted in more than 50% inhibition of COX-2 enzymatic activity, whereas lingonberry extract showed only approximately 50% inhibition (Fig. 7). Notably, the data from the in vitro inflammatory model and COX-2 inhibition assay did not allow us to conclude that there was a major difference in the anti-inflammatory effects between the berry pomace extracts of these Vaccinium spp. Nevertheless, to the best of our knowledge, we have presented the first demonstration the anti-inflammatory effects of five Vaccinium spp. berry pomace extracts analyzed simultaneously in vitro. Comparable anti-inflammatory effects of blueberry and cranberry extracts were also found in an in vivo model of mouse paw edema [40]. Nonetheless, some differences between the analyzed Vaccinium spp. berry pomace extracts were observed in the effects on cytokines at the transcript level (Fig. 6). For example, the inhibitory effect of blueberry (extract 2) on IL-6 transcript was so great that transcript level was below the RT-PCR assay detection limit. As blueberry pomace extract showed strong inhibitory activity on NF-κB nuclear translocation and COX-2 activity (Figs. 3 and 7), this may suggest that the anti-inflammatory potential of blueberry pomace extract is greater than that of the other Vaccinium spp. extracts. However, further research is needed to expand knowledge of the anti-inflammatory properties of Vaccinium spp. berry pomace extracts.
5Conclusion
Bilberry (Vaccinium myrtillus L.), highbush blueberry (Vaccinium corymbosum L.), American cranberry (Vaccinium macrocarpon Ait.), bog cranberry (Vaccinium oxycoccos L.), and lingonberry (Vaccinium vitis-idaea L.) berry pomace extracts exerted anti-inflammatory properties in an LPS-stimulated THP-1 cell inflammation model, as shown by the inhibition of NF-κB nuclear translocation and the downregulation of the expression of pro-inflammatory cytokines TNF-α, IL-1β, IL-6, IL-8, IL-23, and MMP-9. In addition, Vaccinium spp. pomace extracts inhibited COX-2 enzymatic activity. Therefore, Vaccinium spp. berry pomace extracts may be a valuable source of bioactive compounds for healthy nutrition to prevent non-communicable diseases caused by inflammation.
Funding
European Regional Development Fund within the project No. 1.1.1.1/16/A/047 “Genus Vaccinium berry processing using “green” technologies and innovative, pharmacologically characterized biopharmaceutical products”.
Conflict of interest
Authors declare no conflict of interest.
Acknowledgments
This work has been supported by the European Regional Development Fund within the project No. 1.1.1.1/16/A/047 “Genus Vaccinium berry processing using “green” technologies and innovative, pharmacologically characterized biopharmaceutical products”.
Supplementary material
[1] The supplementary material is available in the electronic version of this article: https://dx.doi.org/10.3233/JBR-190485.
References
[1] | Nile SH , Park SW . Edible berries: bioactive components and their effect on human health. Nutrition. (2014) ;30: (2):134–44. |
[2] | Laroze LE , Díaz-Reinoso B , Moure A , Zúñiga ME , Domínguez H . Extraction of antioxidants from several berries pressing wastes using conventional and supercritical solvents. Eur Food Res Technol. (2010) ;231: (5):669–77. |
[3] | Jurikova T , Skrovankova S , Mlcek J , Balla S , Snopek L . Bioactive Compounds, Antioxidant Activity, and Biological Effects of European Cranberry (Vaccinium oxycoccos). Molecules. (2018) ;24: (1). |
[4] | Skrovankova S , Sumczynski D , Mlcek J , Jurikova T , Sochor J . Bioactive Compounds and Antioxidant Activity in Different Types of Berries. Int J of Mol Sci. (2015) ;16: (10):24673–706. |
[5] | Struck S , Plaza M , Turner C , Rohm H . Berry pomace – a review of processing and chemical analysis of its polyphenols. Int J Food Sci Technol. (2016) ;51: (6):1305–18. |
[6] | Reque PM , Steffens RS , Silva AMD , Jablonski A , Flôres SH , Rios AdO , et al Characterization of blueberry fruits (Vaccinium spp.) and derived products. Food Sci Technol. (2014) ;34: :773–9. |
[7] | Olas B . Berry Phenolic Antioxidants – Implications for Human Health?. Front Pharmacol. (2018) ;9: (78). |
[8] | Chu Y-F , Liu RH . Cranberries inhibit LDL oxidation and induce LDL receptor expression in hepatocytes. Life Sci. (2005) ;77: (15):1892–901. |
[9] | Mazzoni L , Perez-Lopez P , Giampieri F , Alvarez-Suarez JM , Gasparrini M , Forbes-Hernandez TY , et al The genetic aspects of berries: from field to health. J Sci Food Agric. (2016) ;96: (2):365–71. |
[10] | Battino M , Forbes-Hernandez TY , Gasparrini M , Afrin S , Cianciosi D , Zhang J , et al Relevance of functional foods in the Mediterranean diet: the role of olive oil, berries and honey in the prevention of cancer and cardiovascular diseases. Crit Rev Food Sci Nutr. (2019) ;59: (6):893–920. |
[11] | Antonelli M , Kushner I . It’s time to redefine inflammation. The FASEB Journal. (2017) ;31: (5):1787–91. |
[12] | Hunter P . The inflammation theory of disease. EMBO reports. (2012) ;13: (11):968–70. |
[13] | Abdulkhaleq LA , Assi MA , Abdullah R , Zamri-Saad M , Taufiq-Yap YH , Hezmee MNM . The crucial roles of inflammatory mediators in inflammation: A review. Vet World. (2018) ;11: (5):627–35. |
[14] | Hussain T , Tan B , Yin Y , Blachier F , Tossou MCB , Rahu N . Oxidative Stress and Inflammation: What Polyphenols Can Do for Us? Oxid Med Cellular Longev. (2016) ;2016: :9. |
[15] | Liu T , Zhang L , Joo D , Sun S-C . NF-κB signaling in inflammation. Sig Transduct Target Ther. (2017) ;2: :17023. |
[16] | Sonar K , Purohit D . Therapeutic effect of flavonoids as anti-inflammatory agents. World Journal of Pharmacy and Pharmaceutic Sciences. (2016) ;5: (12). |
[17] | Rahman I , Biswas SK , Kirkham PA . Regulation of inflammation and redox signaling by dietary polyphenols. Biochem Pharmacol. (2006) ;72: (11):1439–52. |
[18] | Yahfoufi N , Alsadi N , Jambi M , Matar C . The Immunomodulatory and Anti-Inflammatory Role of Polyphenols. Nutrients. (2018) ;10: (11):1618. |
[19] | Chanput W , Mes J , Vreeburg RA , Savelkoul HF , Wichers HJ . Transcription profiles of LPS-stimulated THP-1 monocytes and macrophages: a tool to study inflammation modulating effects of food-derived compounds. Food Funct. (2010) ;1: (3):254–61. |
[20] | Tucureanu MM , Rebleanu D , Constantinescu CA , Deleanu M , Voicu G , Butoi E , et al Lipopolysaccharide-induced inflammation in monocytes/macrophages is blocked by liposomal delivery of G(i)-protein inhibitor. Int J Nanomedicine. (2017) ;13: :63–76. |
[21] | Ambriz-Pérez DL , Leyva-López N , Gutierrez-Grijalva EP , Heredia JB . Phenolic compounds: Natural alternative in inflammation treatment. A Review. Cogent Food & Agriculture. (2016) ;2: (1):1131412. |
[22] | Klavins L , Kviesis J , Nakurte I , Klavins M . Berry press residues as a valuable source of polyphenolics: Extraction optimisation and analysis. LWT. (2018) ;93: :583–91. |
[23] | Muceniece R , Klavins L , Kviesis J , Jekabsons K , Rembergs R , Saleniece K , Dzirkale Z , Saulite L , Riekstina U , Klavins M . Antioxidative, hypoglycaemic and hepatoprotective properties of five Vaccinium spp. berry pomace extracts. J Berry Res. (2019) ;9: (2):267–82. |
[24] | Willett W , Rockström J , Loken B , Springmann M , Lang T , Vermeulen S , et al Food in the Anthropocene: the EAT Lancet Commission on healthy diets from sustainable food systems. The Lancet. (2019) ;393: (10170):447–92. |
[25] | Livak KJ , Schmittgen TD . Analysis of relative gene expression data using real-time quantitative PCR and the ((2001) ;2: (-Delta Delta C(T)) Method. Methods. 25(4):402–8. |
[26] | Yang L , Guo H , Li Y , Meng X , Yan L , Dan Z , et al Oleoylethanolamide exerts anti-inflammatory effects on LPS-induced THP-1 cells by enhancing PPARα signaling and inhibiting the NF-κB and ERK1/2/AP-1/STAT3 pathways. Sci Rep. (2016) ;6: :34611. |
[27] | Michalska A , Łysiak G . Bioactive Compounds of Blueberries: Post-Harvest Factors Influencing the Nutritional Value of Products. Int J Mol Sci. (2015) ;16: (8):18642–63. |
[28] | Grivins M . Global and Local Wild Blueberry Supply Chains in Latvia. 2014. |
[29] | Chanput W , Mes JJ , Wichers HJ . THP-1 cell line: an in vitro cell model for immune modulation approach. Int Immunopharmacol. (2014) ;23: (1):37–45. |
[30] | Karlsen A , Retterstol L , Laake P , Paur I , Bohn SK , Sandvik L , et al Anthocyanins inhibit nuclear factor-kappaB activation in monocytes and reduce plasma concentrations of pro-inflammatory mediators in healthy adults. The Journal of nutrition. (2007) ;137: (8):1951–4. |
[31] | Ben Lagha A , LeBel G , Grenier D . Dual action of highbush blueberry proanthocyanidins on Aggregatibacter actinomycetemcomitans and the host inflammatory response. BMC Complement Altern Med. (2018) ;18: (1):10. |
[32] | Gasparrini M , Forbes-Hernandez TY , Giampieri F , Afrin S , Alvarez-Suarez JM , Mazzoni L , et al Anti-inflammatory effect of strawberry extract against LPS-induced stress in RAW 264.7 macrophages. Food Chem Toxicol. (2017) ;102: :1–10. |
[33] | Gasparrini M , Giampieri F , Forbes-Hernandez TY , Afrin S , Cianciosi D , Reboredo-Rodriguez P , et al Strawberry extracts efficiently counteract inflammatory stress induced by the endotoxin lipopolysaccharide in Human Dermal Fibroblast. Food Chem Toxicol. (2018) ;114: :128–40. |
[34] | Wu S , Yano S , Chen J , Hisanaga A , Sakao K , He X , et al Polyphenols from Lonicera caerulea L. Berry Inhibit LPS-Induced Inflammation through Dual Modulation of Inflammatory and Antioxidant Mediators. J Agric Food Chem. (2017) ;65: (25):5133–41. |
[35] | Chanput W , Krueyos N , Ritthiruangdej P . Anti-oxidative assays as markers for anti-inflammatory activity of flavonoids. Int Immunopharmacol. (2016) ;40: :170–5. |
[36] | do Nascimento GE , Winnischofer SMB , Ramirez MI , Iacomini M , Cordeiro LMC . The influence of sweet pepper pectin structural characteristics on cytokine secretion by THP-1 macrophages. Food Res Int. (2017) ;102: :588–94. |
[37] | Popēna I , ābols A , Saulīte L , Pleiko K , Zandberga E , Jēkabsons K , et al Effect of colorectal cancer-derived extracellular vesicles on the immunophenotype and cytokine secretion profile of monocytes and macrophages. Cell Commun Signal. (2018) ;16: (1):17. |
[38] | Yabluchanskiy A , Ma Y , Iyer RP , Hall ME , Lindsey ML . Matrix Metalloproteinase- Many Shades of Function in Cardiovascular Disease. Physiology. (2013) ;28: (6):391–403. |
[39] | Ruel G , Pomerleau S , Couture P , Lemieux S , Lamarche B , Couillard C . Plasma Matrix Metalloproteinase (MMP)-9 Levels Are Reduced following Low-Calorie Cranberry Juice Supplementation in Men. Journal of the American College of Nutrition. (2009) ;28: (6):694–701. |
[40] | Nardi G , Farias Januario A , Freire C , Megiolaro F , Schneider K , Perazzoli M , et al Anti-inflammatory activity of berry fruits in mice model of inflammation is based on oxidative stress modulation. Pharmacognosy Res. (2016) ;8: (5):42–9. |