Berry fruit juices protect lymphocytes against DNA damage and ROS formation induced with heterocyclic aromatic amine PhIP
Abstract
BACKGROUND:
Heterocyclic aromatic amines are formed during thermal processing of meat and are known to be mutagenic and carcinogenic factors, while the consumption of fruit and vegetables decreases the risk of cancer.
OBJECTIVE:
The aim of the study was to investigate if berry fruit juices, a rich source of antioxidants (polyphenols, flavonoids, anthocyanins, ascorbate), may protect DNA from damages induced by the heterocyclic amine 2-amino-1-methyl-6-phenylimidazo(4, 5-b)pyridine (PhIP).
METHODS:
Lymphocytes isolated from healthy volunteers were treated with 25–100 μM PhIP in the presence or absence of pressed berry juice (0.1%) from: bilberry (Vaccinium myrtillus), chokeberry (Aronia melanocarpa), cranberry (Vaccinium oxycoccos), raspberry (Rubus idaeus), rosehips (Rosa rugosa), sea-buckthorn (Hippophae rhamnoides), Noni (Morinda citrifolia) and Goji (Lycium barbarum). Antioxidant power, polyphenols, flavonoids, anthocyanins and ascorbate concentration in juices were determined. DNA damage was measured using comet assay.
RESULTS:
Berry juices were not genotoxic and caused an overall significant decrease in oxidative stress and DNA damage induced by PhIP. The antioxidant properties depend on concentration of polyphenols in juice.
CONCLUSIONS:
Northern Hemisphere berries bear the negative effects of food mutagens. Berries as natural source of polyphenols should be recommended in daily diet for maintaining health.
Abbreviations
DCF | 2′, 7′-dichlorofluorescein |
DMSO | Dimethylsulfoxide |
EtBr | Ethidium bromide |
FBBB | Fast Blue BB N-(4-Amino-2,5-diethoxyphenyl)benzamide |
FBS | Fetal Bovine Serum |
FRAP | Ferric reducing antioxidant power assay |
GPX | Glutathione peroxidase |
GST | Glutathione S-transferase |
HCA | Heterocyclic aromatic amines |
NATs | N-acetyltransferases |
N-OH-PhIP | N-hydroxy-PhIP |
PBML | Human peripheral blood lymphocytes |
PhIP | 2-amino-1-methyl-6-phenylimidazo(4, 5-b)pyridine |
RFU | Relative fluorescence unit |
ROS | Reactive oxygen species |
RPMI | Roswell Park Memorial Institute Medium |
SULTs | Sulfotransferases |
UGT | UDP-glucuronosyltransferase |
1Introduction
Globally, cancer is one of the diseases that receives the most attention. Based on statistics of the National Cancer Institute (NCI), in 2016 it was estimated that more than 1.5 million new cases of cancer would be diagnosed and more than half a million people would die from cancer in the United States alone. The most common cancers in 2016 were breast, lung, prostate and colon. Diet, which is associated with about 35% of cases, is one of the primary determinants of the various types of cancer [1]. A particular relationship was observed in the case of consumption of red meat and processed meat products, as studies indicate that thermal processing of meat is a major cause of the creation of polycyclic aromatic hydrocarbons and heterocyclic aromatic amines (HCA). These chemical compounds are characterized by their mutagenic and carcinogenic properties [2–4] and are formed during thermal processing of high protein food, e.g. beef, poultry and fish [5]. Additionally, these compounds are present in the environment, including cigarette smoke, exhaust fumes, municipal sewage and river water samples [6]. More importantly, HCAs have been found in human tissues and body fluids, which indicates an accumulation of these toxic substances [4]. HCAs are classified as probable and possible human carcinogens by the International Agency for Research of Cancer (IARC). According to the European Prospective Investigation into Cancer and Nutrition (EPIC), PhIP (2-amino-1-methyl-6-phenylimidazo(4, 5-b)pyridine) is the most abundant heterocyclic amine present in processed meat in comparison to other HCAs [7]. On the other hand, a great deal of studies suggests that consumption of fruit and vegetables decreases the risk of cancer [8–14]. An adequate intake of fruit may hinder cancer growth also by reduction of the amount of damage introduced to DNA via oxidative stress either by enhancement of DNA repair processes [14], inhibition of pro-oxidative enzymes [15, 16], inhibition of angiogenesis by suppression of the growth factors signaling pathways [17] and induction of apoptosis in cancer cells [18, 19]. Grapefruit, cranberries, pomegranate, guava, pineapple and mango [20], green tea, red wine, blueberries, blackberries, red grapes, kiwi, watermelon, parsley, and spinach [14] have been proven to prevent carcinogenesis.
It has been shown that apple and grape seed juices prevent the formation of HCA [21], while sweet cherry, blueberry, and blackcurrant prevent HCA-induced DNA damage by modulation of the activity of phase I detoxification enzymes [22]. HCA genotoxicity is also inhibited by tea, soy and turmeric components [23–25], however, the wild fruits growing in Northern Hemisphere are less known for their chemopreventive activity.
The purpose of this study was to investigate the potentially protective effect of berry juices against the genotoxic action of heterocyclic amine PhIP on lymphocytes from healthy individuals. The total phenolic content, flavonoids, anthocyanins and vitamin C concentration, reduction power and antioxidant activity of juices from berry fruit were determined. The fruits of interest were chosen for their well-known health benefits: bilberry (Vaccinium myrtillus), cranberry (Vaccinium oxycoccos), chokeberry (Aronia melanocarpa), raspberry (Rubus idaeus), rosehips (Rosa rugosa), sea-buckthorn (Hippophae rhamnoides), Noni (Morinda citrifolia) and Goji (Lycium barbarum). Sea-buckthorn, rosehips and chokeberries were part of the diet of the previous generations, but nowadays they are not very popular ingredients and are restricted mainly to consumers who follow an ecological lifestyle. Sea-buckthorn, rosehips, chokeberries, raspberries, bilberries and cranberries are native to the Northern Hemisphere, therefore, we found it interesting to compare their properties to oriental fruit, namely Noni and Goji. Although rosehips, raspberries and sea-buckthorn are not considered as berries in the botanical classification, in colloquial speech all of the analyzed fruits are referred to in this way, so for simplicity the term berry is used.
2Materials and methods
2.1Cells
Human blood was provided by the Regional Blood Donation and Transfusion Center in Krakow. The blood was donated by three male, white, healthy donors aged 26, 34 and 36. Human peripheral blood lymphocytes (PBML) were isolated from heparinized blood using the gradient centrifugation method with Histopaque 1077 (Merck), according to the manufacturer’s protocol and stored frozen until the experiment at –80°C in 50% fetal bovine serum (FBS), 40% RPMI-1640 and 10% DMSO.
2.2Reagents
The following reagents were obtained from Merck (Sigma-Aldrich): Histopaque 1077; trypsin; ethidium bromide; FBBB, (+)-catechin, cyanidin-3-glucoside, quercetin; hydrogen peroxide; DMSO; Triton X-100; Trizma Base; 2′,7′-dichlorofluorescin diacetate.
RPMI 1640 was purchased from Cytogen GmbH; Fetal Bovine Serum (FBS) – Lonza; penicillin; streptomycin – Biomed; agarose-normal melting point (NMPA), agarose-low melting point (LMPA) – Gibco BRL; ethylenediaminetetraacetic acid (EDTA); propidium iodide – MP Biomedicals; 2,4,6-Tris(2-pyridyl)-s-triazine (TPTZ) – Fluka Analytical; PBS without (w/o) Ca2+ and Mg2+; AlCl3; KCl; NaCl; NaNO2; HCl; NaOH; methanol; sodium acetate trihydrate; ferrous sulfate heptahydrate – Avantor Performance Materials Poland S.A; iron (III) chloride hexahydrate (PARK Scientific); PhIP (CAS-No. 105650-23-5, 98% purity) – Toronto Research Chemicals Inc. PhIP was prepared in 1% DMSO and kept frozen (–20°C).
2.3Berry juices
Berry juices were obtained from commercial brands: Polska Róża and Oleofarm. Fruits were washed, milled, mashed, pressed and the juice was pasteurized. The juices were used in 0.1% dilution, as it was established in previous experiments that this provides the concentration of flavonoids in the in vitro culture comparable to the levels obtained in serum after dietary ingestion of 60 ml fruit juice [18, 26], however, 1% dilution was also tested to check if it is a critical concentration for cell viability.
2.4Cell culture and treatment
Before each experiment, lymphocytes were thawed in RPMI with 50% FBS and centrifuged at 1200 rpm at 4°C for 5 min. Subsequently, the cells were seeded in a 96-well plate at a density of 1×104 cells per well in RPMI medium with 10% FBS and incubated at 37°C in 5% CO2 for 30 minutes [27]. Subsequently, the cells were incubated in 25, 50, 75, 100 μM concentrations of PhIP, either with 0.1% berry juices for 1 hour. For the genoprotection investigation, the tested cells were treated with 100 μM PhIP together with 0.1% juices for 1 h. For the negative control samples the untreated cells were used, which were incubated in medium only. For the positive control samples the cells incubated in medium with 100 μM PhIP were used. The concentration of PhIP was chosen according to the obtained results which proved, that it will induce generation of DNA damage and ROS. All the experiments were run independently for lymphocytes from the three donors and all samples were run in duplicates.
2.5Total polyphenols content – Fast Blue BB assay
Polyphenol content was determined using Fast Blue BB (FBBB). This is an alternative method to Folin-Ciocalteu’s assay to quantify phenolic compounds through direct interaction of polyphenols with the FBBB reagent in an alkaline medium [28] without interference of ascorbic acid. A 0.2 ml aliquot of 0.1% Fast Blue BB reagent was added to 2 ml of samples, mixed for 1 min and 0.2 ml 5% NaOH was added. The reaction was allowed to proceed at room temperature for 90 min. The absorbance was measured with the LEDetect spectrophotometer (Labexim, Austria) at 420 nm. The results were expressed as quercetin equivalent weight [mg/ml].
2.6Vitamin C content with Tillman’s method
Tillman’s method is based on the reduction of 2, 6-dichlorophenolinodophenol (DCPIP, Tillman’s dye) by ascorbic acid. Because of the high coloration of berry fruit juices, the method was modified by an addition of an organic solvent (chloroform). 10 ml of the centrifuged juice was diluted with chloroform to 50 ml. 10 ml of the solution was titrated with 1 mM DCPIP until a slightly pink coloration lasting for at least 10 seconds was visible. Each sample was analyzed in triplicate. The 1 mg/ml ascorbic acid solution was used as a standard.
2.7Ferric reducing antioxidant power (FRAP) assay
The FRAP assay was adapted with minor modifications to evaluate the reducing power of the berry juices [29]. Briefly, the calibration curve was plotted using standard FeSO4 solution. FeSO4 solutions (0–1 mM) were mixed with FRAP 1 solution (300 mM acetate buffer, 10 mM TPTZ) and incubated (20 min, RT, dark). FRAP 2 solution (300 mM acetate buffer, 10 mM TPTZ, 20 mM FeCl3) was added to the 0.1% juices in ethanol. Subsequently, the mixture was allowed to stand for 20 minutes and the absorbance was measured at 593 nm using a Spectra Fluor Plus Tecan instrument (Tecan, Switzerland). Additionally, 0.1% vitamin C was used as a standard antioxidant solution. The juices analysis was performed in triplicate. The results were expressed as the equivalent of Fe2+ ion concentration and as the equivalent of ascorbic acid.
2.8Determination of total flavonoid content
For the evaluation of total flavonoid content, 0.3 ml of 5% NaNO2 was added to 1 ml of berry juice and after 5 min incubation, 0.3 ml 10% AlCl3 was added. Then 2 ml 1 M NaOH was added to the samples. Then the samples were diluted with 4 ml of distilled water. Absorbance was measured at 510 nm using LEDetect spectrophotometer (Labexim, Austria). The total flavonoid content was determined by a (+)-catechin standard curve and was expressed as catechin equivalents (CE) mg/ml [30].
2.9Determination of total anthocyanin content
The total anthocyanin content was determined by the pH differential method [31]. 0.2 ml of berry juice were diluted either with 0.8 ml 0.025 M KCl (pH 1.0) or with 0.8 ml 0.4 M CH3COONa·3H2O (pH 4.5) buffer solutions. Diluted samples were shaken and left in the dark for 15 min. Absorbance of each diluted sample was measured at both 520 nm and 700 nm at LEDetect spectrophotometer (Labexim, Austria) using water as a reference. Concentration was calculated as follows:
1 MW (molecular weight for cyanidin-3-glucoside) = 449.2 g/mol
2 DF (dilution factor) = 5
3 103 = factor for conversion from g to mg
4 ɛ= 26 900 molar extinction coefficient
5 l = path length in cm
The total anthocyanin content was expressed as cyanidin-3-glucoside equivalents in mg/ml.
2.10Evaluation of cell viability by double-staining fluorescence assay
For viability measurements in the presence of berry juices and PhIP, the differential fluorescence staining method was performed [27]. Cells were seeded at 2×104 cells/well in 96-well plates for 1 and 24 hours and 0.1% juice or 100 μM PhIP were added to the respective wells. The staining dye was prepared by mixing fluorescein diacetate in acetone (FDA, 5 mg/ml), ethidium bromide in PBS (EtBr, 200 μg/ml) and PBS w/o Ca2+ and Mg2+. Samples were mixed with FDA/EtBr and placed on microscope slides. The metabolically active cells are able to hydrolyze fluorescein diacetate to fluorescein, while loss of membrane integrity allows ethidium bromide to enter the cell and stain nucleic acids in red as it is shown in Fig. 1.
Fig.1
FDA/EtBr viability assay. Cell viability evaluation with the fluorescein diacetate and ethidium bromide differential staining method visualized in fluorescence microscope (magnification 100×). The green labelled cells are metabolically active and the red labelled cells are considered as dead.
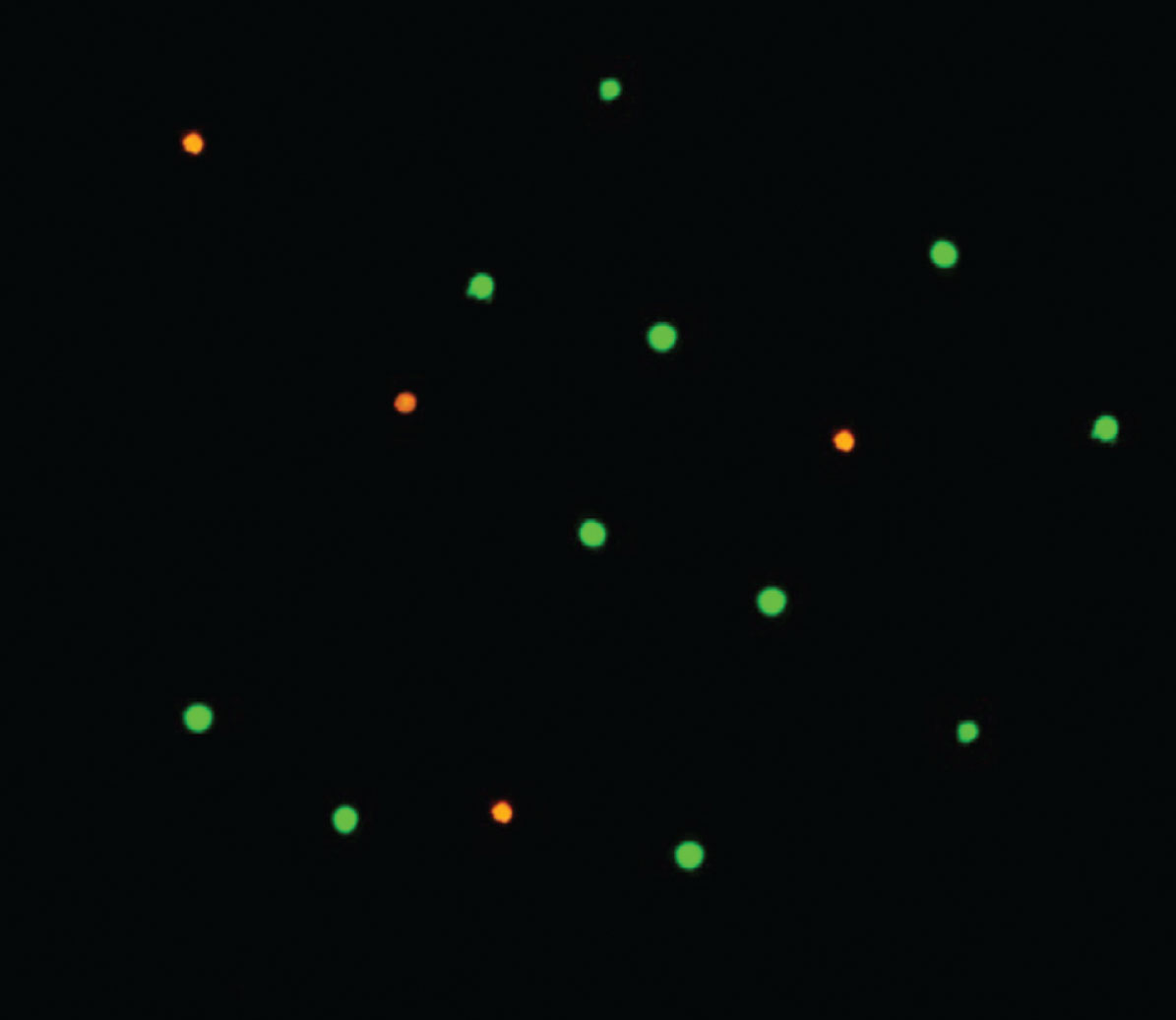
At least 100 randomly selected cells were analyzed per slide using a fluorescent inverted microscope Olympus IX50 (Olympus, Tokio, Japan).
2.11The comet assay – evaluation of DNA damage
The comet assay was performed under alkaline conditions according to the procedure described previously [27].
A pattern of DNA migration through the electrophoresis gel in fluorescent microscope resembles a comet: not damaged head and a tail of migrating fragments of damaged DNA as it is shown in Fig. 2. Comets were visualized using propidium iodine staining. The fluorescence intensity of the comet tail depends on the amount of DNA damage in the nucleus. A fluorescent microscope Olympus IX50 (Olympus, Tokio, Japan) equipped with a 515–560 nm excitation filter and a 590 nm barrier filter was used at a magnification of 200×. 100 randomly selected cells were analyzed per sample. All samples were proceeded in duplicates.
Fig.2
Comet assay. The comet assay visualization in fluorescence microscope in magnification of 200×. The fluorescence intensity of comet tail depends on the amount of DNA damage in nucleus. In the top picture: Nucleoids of not damaged cells; in the middle: Moderate DNA damage; in the bottom picture: Comets of severely damaged cells.
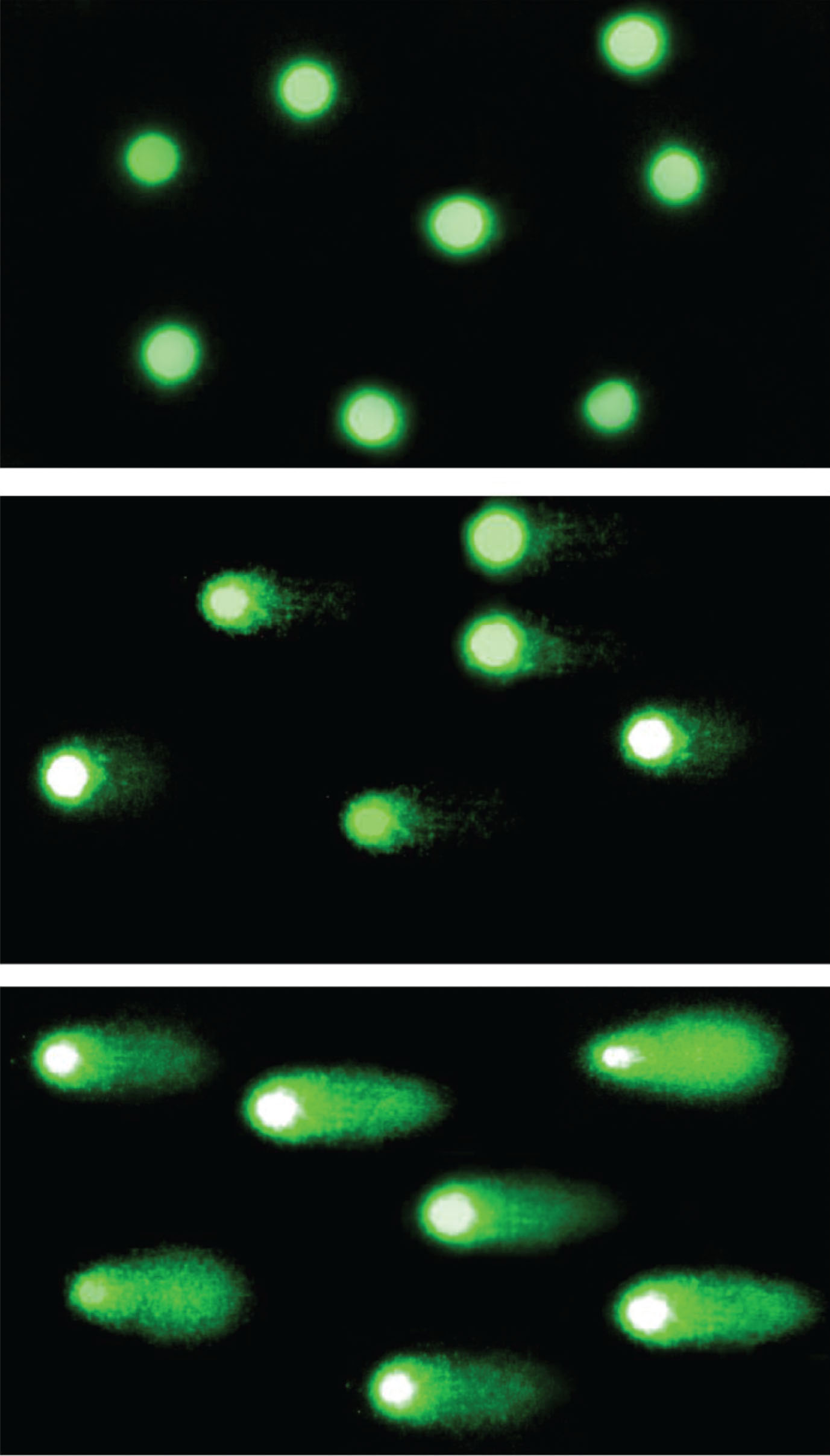
The images were analyzed in COMET ASSAY 2.6 software. The results from the three independent experiments were expressed as % DNA in the comet tail (TDC).
2.12Detection of reactive oxygen species by 2′,7′-dichlorodihydrofluorescein diacetate
Cells were seeded at 1×105 cells/ml in black 96-well plates, treated with the tested compounds for 1 hour and subsequently a cell-permeable fluorogenic oxidant-sensing probe, 2′,7′-dichlorodihydrofluorescein diacetate (DCFH-DA), was added at a final concentration of 25 μM [32]. For the positive control, 1 mM H2O2 was used. After 10 minutes the plate was centrifuged (3000 rpm, 5 min., RT) and the cell pellets were rinsed in PBS. The plate was read at 485/530 nm. All samples were run in triplicate and three independent experiments were performed.
3Statistical analysis
Statistical analysis was carried out with Statistica 9 (StatSoft, USA) software. The results obtained from lymphocytes donated by the donors did not differ significantly and they are expressed as a mean from three experiments. The statistical significance of differences between the experimental conditions was assessed by a one-way ANOVA test under the condition of positive homogeneity of variance in Levene’s test. The posteriori Tukey’s test was performed. A p value of 0.05 was considered as the cutoff for significance. The correlation analysis was performed with linear regression and the strength of correlation was established by Pearson’s correlation factors.
4Results
4.1Total phenolic content, flavonoids, anthocyanins and vitamin C concentration and reducing power of juices
The results of measured concentrations of phenolic compounds, flavonoids, anthocyanins and vitamin C as well as the reducing power of the berries’ juices are collected in Table 1.
Table 1
The antioxidant power (FRAP), polyphenols, flavonoids, anthocyanins and ascorbic acid concentrations in berry fruit juices. The results are expressed as mean±SD (N = 3)
Berry juice | FRAP [eq.Vit.C] | Polyphenols [mg/ml] | Flavonoids [mg/ml] | Anthocyanins [mg/ml] | Vitamin C [mg/100ml] |
Bilberry Vaccinium myrtillus | 0.44±0.005 | 12.90±2.01 | 2.22±0.23 | 6.23±0.03 | 3.8±1.2 |
Chokeberry Aronia melanocarpa | 0.50±0.004 | 14.18±1.05 | 3.5±0.32 | 8.75±0.54 | 10.6±2.2 |
Cranberry Vaccinium oxycoccos | 0.07±0.001 | 1.71±0.06 | 0.69±0.21 | 0.29±0.02 | 9.2±0.4 |
Raspberry Rubus idaeus | 0.11±0.001 | 3.85±1.22 | 1.60±0.32 | 1.63±0.07 | 28.9±0.6 |
Rose hips Rosa rugosa | 1.88±0.004 | 20.36±1.38 | 6.44±0.97 | 9.93±0.95 | 268.3±15.7 |
Sea-buckthorn Hippophae rhamnoides | 0.25±0.013 | 7.28±0.29 | 3.35±1.08 | 0.21±0.01 | 48.1±7.2 |
Goji Lycium barbarum | 0.37±0.016 | 11.07±1.05 | 4.00±1.23 | 0.24±0.01 | 2.9±0.2 |
Noni Morinda citrifolia | 0.08±0.002 | 2.02±0.02 | 1.01±0.04 | 0.23±0.01 | 2.1±0.1 |
Vitamin C 0.1% | 1 | – | – | – | 100 |
SD – standard deviation.
We noted the highest content of phenolic compounds in rose hip (Rosa rugosa), chokeberry (Aronia melanocarpa), sea-buckthorn (Hippophae rhamnoides) and goji berry juices (Lycium barbarum) along with the highest ferric reducing potentials also observed for these juices. Importantly, Rosa rugosa fruit had the highest total content of polyphenols, the highest concentration of flavonoids, anthocyanins and ascorbic acid as well as the highest reducing power. The highest ratio of antocyanins to total phenolic compounds was found in chokeberry juice (over 61%), then in rosehips, bilberries and raspberries juice (48%, 48% and 42%). The lowest anthocyanins to total phenolic ratio was found in Goji and sea-buckthorn (2.1% and 2.8%). The highest flavonoid to phenolic ratio was found in Noni however this juice was characterized by lowest phenolic content among studied juices (2 mg/ml).
There was a strong positive correlation found between the antioxidant activity of the fruit juices and total phenolic content (y = 0.0774x–0.2476; R2 = 0.738; p = 0.0051) (Fig. 3) The outstanding point is representing rosehip juice, which revealed higher antioxidant capacities than would be implied only by the flavonoid content. After exclusion of rosehip juice point from the analysis, the correlation was stronger (R2 = 0.9947; p = 0.0003). There was a significant correlation between FRAP and flavonoids and anthocyanins concentration (y = 2.7744x + 1.5681, R2 = 0.7698, p = 0.0002 and y = 5.3907x+0.9455, R2 = 0.5917, p = 0.0031 respectively, shown in Fig. 3).
Fig.3
The effect of phenolic compounds in berry juices on FRAP. The correlation between antioxidant power of berry juices measured with FRAP method and concentration of polyphenols (p = 0.0051), flavonoids (p = 0.0002) and anthocyanins (p = 0.0031). The regression after exclusion of rosehips outlier is also shown for polyphenols (p = 0.0000).
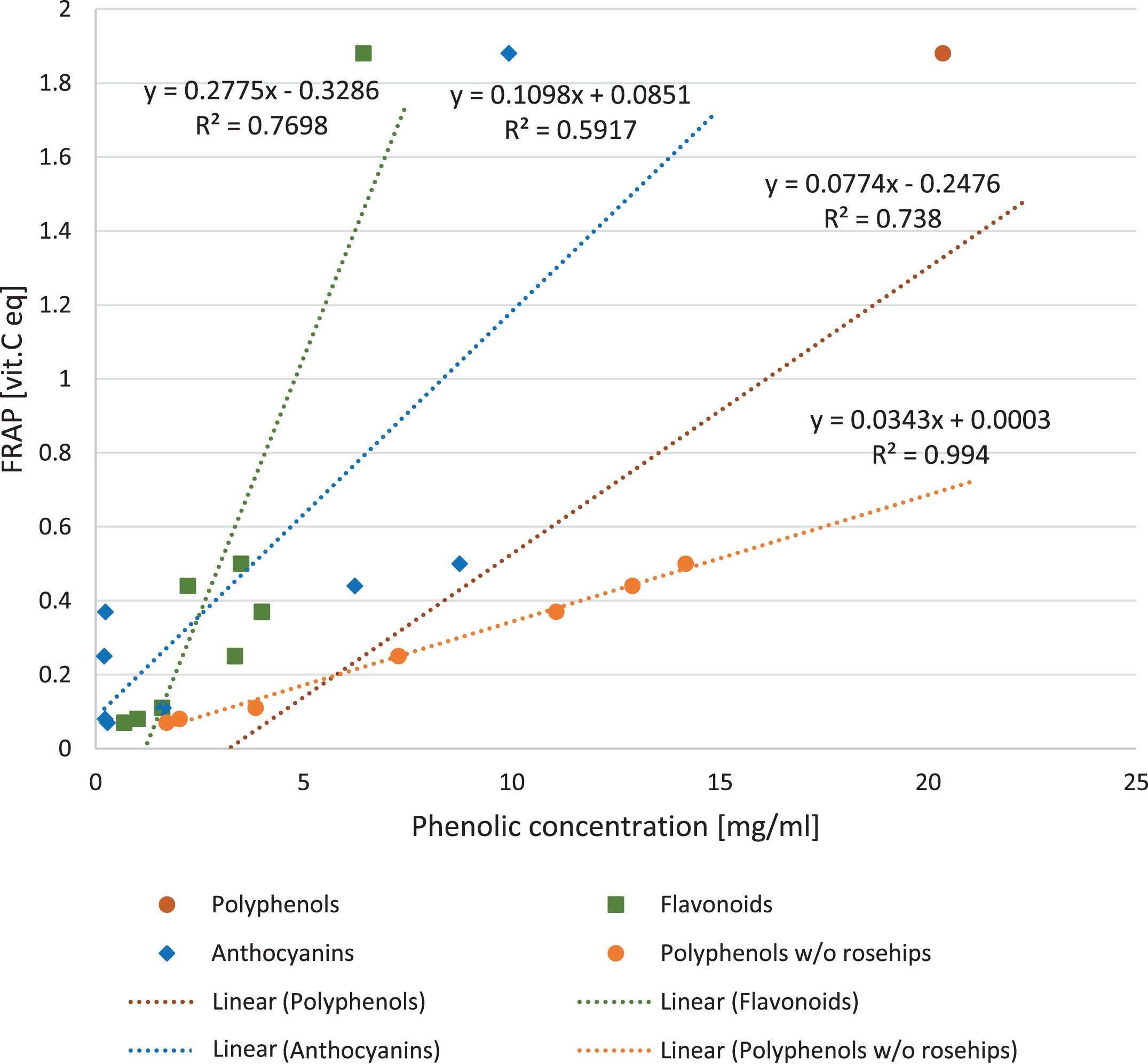
After excluding the outlier of rosehip juice, which was also characterized by very high ascorbic acid concentration, there was no longer a significant correlation between the ascorbic acid content and the antioxidant power of the juices (y =–17.572x + 19.655; R2 = 0.0335, p = 0.7669). If rosehips juice was included in the analysis, the correlation between FRAP and ascorbic acid concentration was very strong (y = 142.81x–19.313, R2 = 0.879; p = 0.0289) as shown in Fig. 4.
Fig.4
The relation between vitamin C concentration in berry juices and FRAP. The correlation between antioxidant power measured with FRAP and concentration of ascorbic acid in berry juices (p = 0.0051).
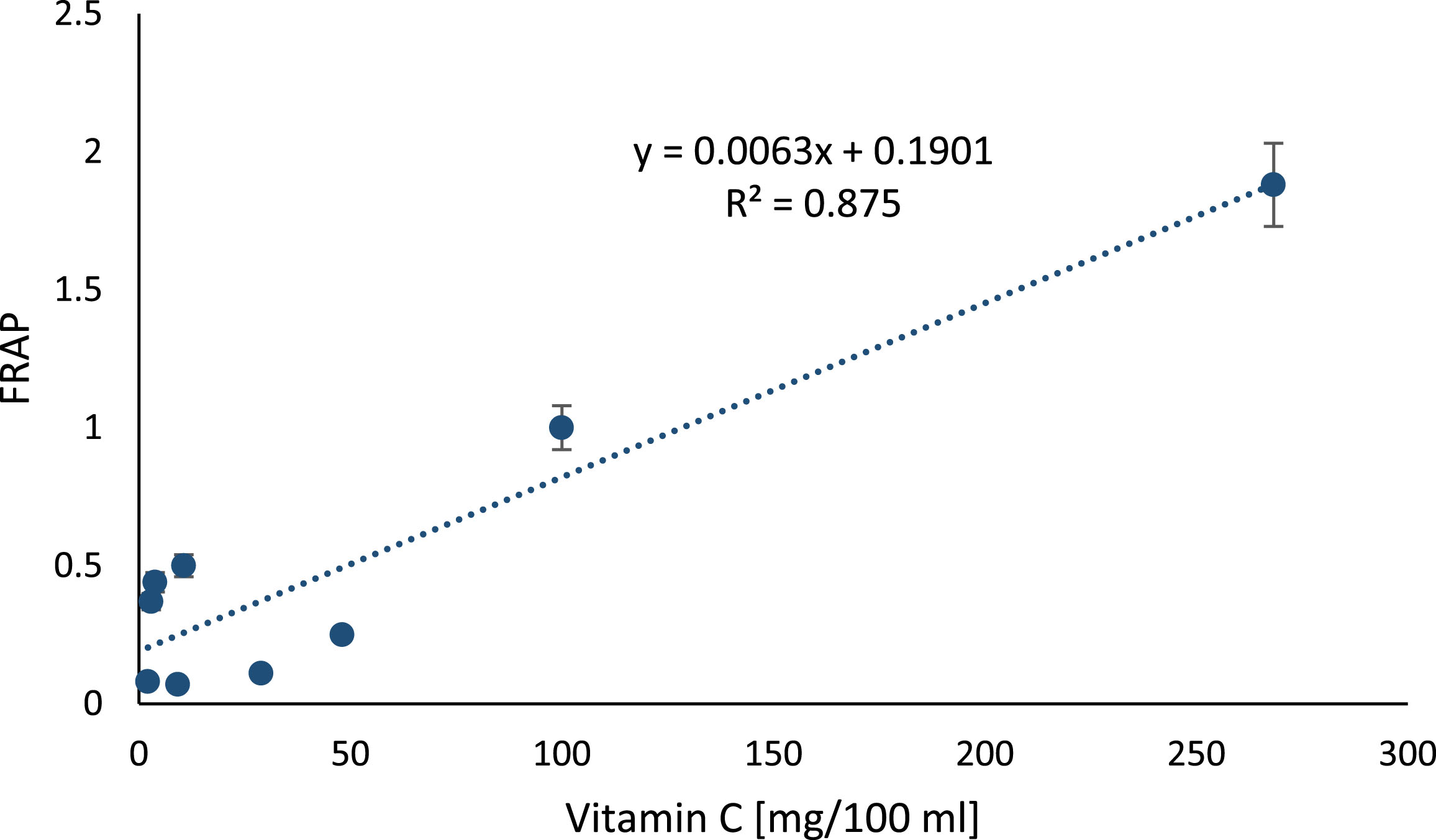
4.2Determination of cell viability
To assess the viability of lymphocytes treated with juices at concentrations of 1% 0.1% for 1 and 24 h, a differential double-staining fluorescence assay was performed. The results obtained in all experiments on lymphocytes donated by different donors did not differ significantly.
The obtained data suggests that juices at concentrations of 1% and 0.1% are not toxic to lymphocytes after 1 h and 24 h exposure. As shown in Table 2, when compared to the control, from 91 up to 98% of cells survived a brief exposure to 0.1% juices. After 24 hours we observed a decrease in cell viability for of all the tested juices as well as the control. The viability of cells exposed to 1% juice was significantly lower than exposed to 0.1% (p < 0.05). Based on these results, further experiments were performed using 0.1% berry juices.
Table 2
Viability of lymphocytes exposed to berry juices in concentration of 1% and 0.1% during 1 h and 24 h incubations
Juice | 1% 1 h | SD | 1% 24 h | SD | 0.1% 1 h | SD | 0.1% 24 h | SD |
Control | 94.7 | 4.1 | 84 | 6.5 | 98 | 1.7 | 90 | 3.8 |
Bilberry | 87.7 | 6.3 | 52* | 7.5 | 95 | 2.7 | 83 | 4.2 |
Chokeberry | 88 | 9.1 | 83 | 7.5 | 94 | 3.9 | 83 | 2 |
Cranberry | 91.3 | 3.3 | 89 | 0.5 | 96 | 1.4 | 83 | 1.3 |
Raspberry | 87.7 | 4.9 | 81 | 16 | 97 | 2.1 | 80 | 12 |
Rosehips | 86.3 | 7.6 | 67* | 10 | 95 | 3.2 | 83 | 5.7 |
Sea-buckthorn | 83.7 | 8.2 | 78 | 7.5 | 91 | 3.5 | 85 | 2.4 |
Noni | 90.7 | 4.6 | 87 | 1.5 | 95 | 1.9 | 83 | 1 |
Goji | 89.7 | 4.6 | 77 | 0 | 91 | 1 | 78* | 2.5 |
SD – standard deviation. *p < 0.05 in comparison to control sample.
The cells were also exposed to 100 μM PhIP; their viability did not fall below 98% and statistical analysis did not reveal significant differences between PhIP-treated cells and the control sample after 1 h incubation (Student t-test, α= 5%). However, the viability of lymphocytes after 24 h exposure to 100 μM PhIP significantly decreased, therefore, the experiments with PhIP were carried out with 1 h incubation.
4.3DNA damage
Results of the comet assay used for the assessment of the DNA damage level in individual lymphocytes after exposure to different concentrations of PhIP are presented in Fig. 5.
Fig.5
Genotoxic effect of PhIP on lymphocytes. DNA damage measured with comet assay in lymphocytes exposed to different concentrations of heterocyclic amine PhIP (0–100 μM). The results are shown as the percentage of Tail DNA Content (TDC) in comparison to control sample (exposed to DMSO as PhIP solvent).
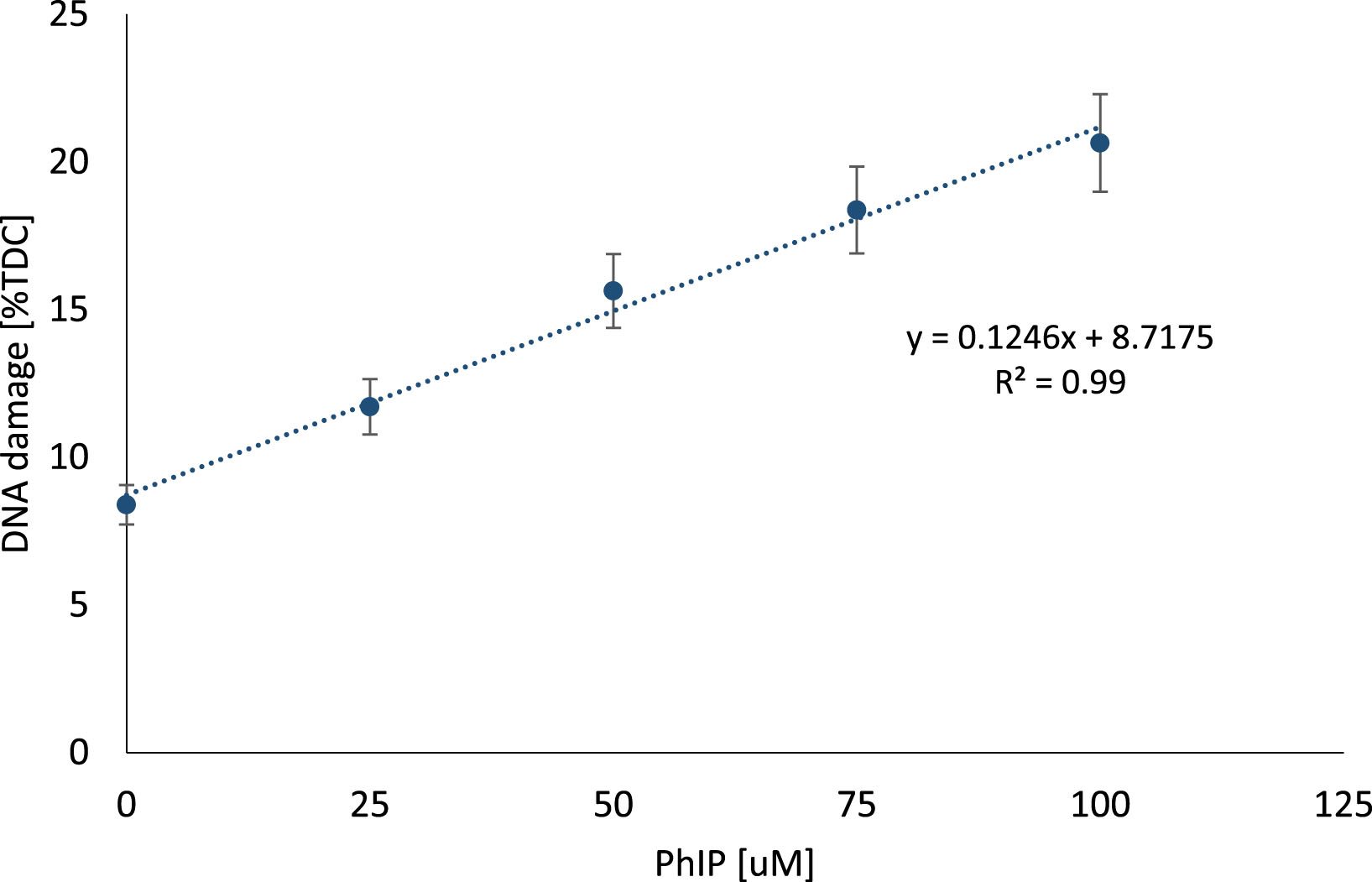
The results indicate that PhIP induces DNA damage in a dose-dependent manner (y = 37.134x + 29.62; R2 = 0.99). At higher concentrations of 75 and 100 μM, the strongest potential of PhIP to induce DNA strand breaks was observed. Based on these results, further experiments were performed using PhIP at a concentration of 100 μM to evaluate the possible protective effect of berry juices more clearly. Since DMSO had no significant influence on PhIP-induced DNA damage (p > 0.05), the samples treated with DMSO were considered as control sample.
To evaluate the influence of the tested berry fruit juices on DNA damage induced by PhIP, lymphocytes were simultaneously exposed to 0.1% berry juices and 100 μM PhIP for 1 hour. The level of DNA fragmentation in lymphocytes was reduced significantly in comparison to the cells exposed only to PhIP (Fig. 6).
Fig.6
The effect of berry juices on DNA damage induced by PhIP. DNA damage measured with comet assay in lymphocytes exposed for 1 hour to different 0.1% berry juices alone and to 0.1% juices and 100 μM PhIP simultaneously. The results are shown as the percentage of Tail DNA Content (TDC) in comparison to control sample. 100 μM PhIP was used as a positive control. *Asterisks indicate the significant difference between cell treated with juices and PhIP in comparison to cells treated with PhIP only. **Double asterisks denotes the significant difference between cells treated with juice and PhIP in compare to juice only.
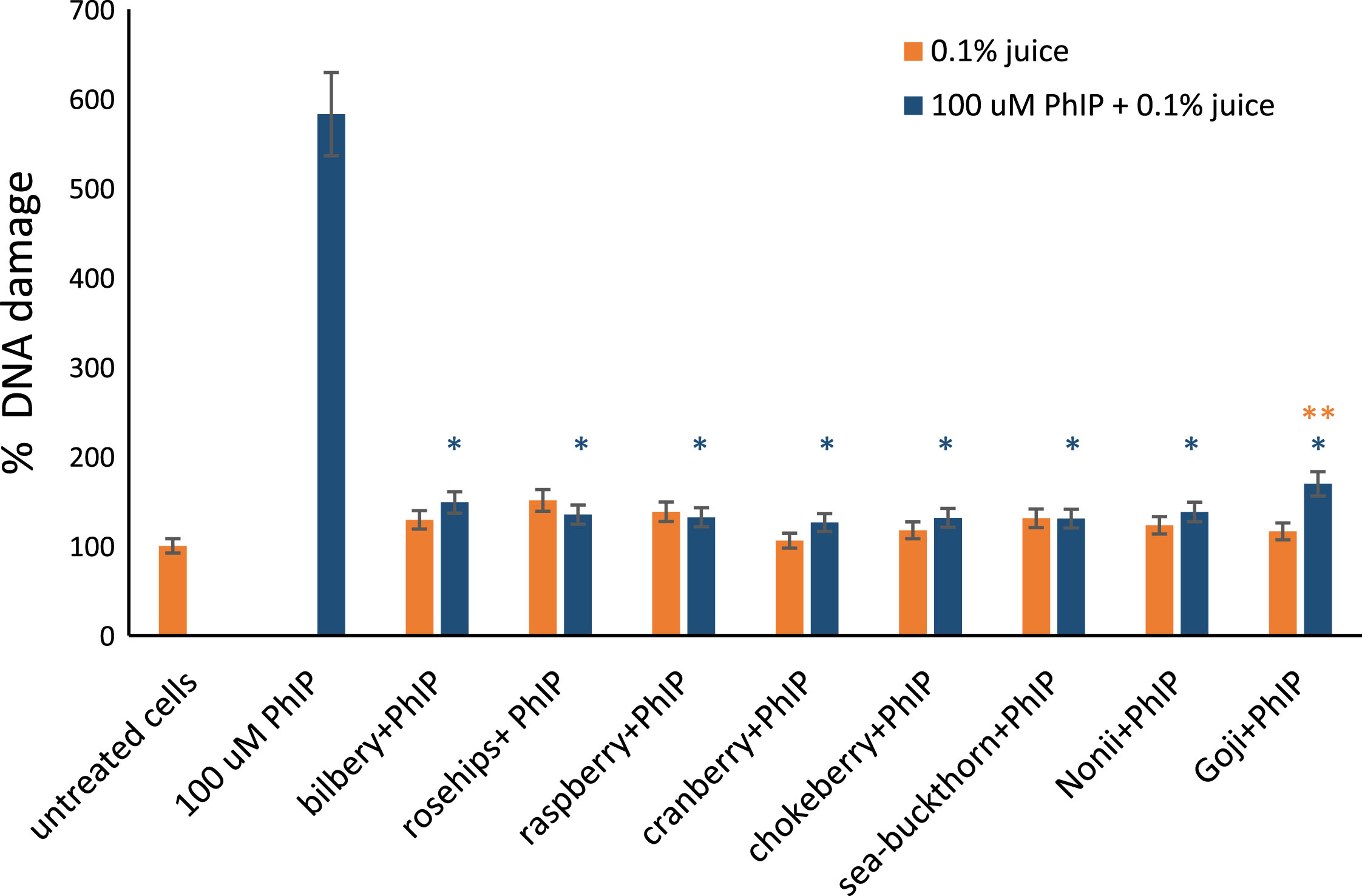
In comparison to the group treated with berry juices alone and cells treated with berry juices and 100 μM PhIP there was no significant difference in DNA damage, thus berries efficiently protect DNA against PhIP-induced damage. The exception was Goji juice and PhIP treated sample, where the increase of DNA damage level was observed in comparison to lymphocytes treated with Goji juice only (p = 0.01).
4.4ROS formation
Intracellular ROS level was quantified using 2′,7′-dichlorofluorescein diacetate (DCFH-DA) fluorescent dye. DCFH-DA is oxidized into a highly fluorescing compound, 2′,7′-dichlorofluorescein (DCF) at presence of ROS, and the fluorescence intensity correlates with the amount of ROS generated within the lymphocytes in density 1×105 cells/ml.
The results revealed dose-dependent correlation between PhIP concentration and ROS formation (y = 0.044x + 5.22; R2 = 0.9418). The strongest ROS release was observed in lymphocytes after 1-hour incubation with 100 μM PhIP concentration (Fig. 7).
Fig.7
ROS formation in lymphocytes exposed to PhIP. Reactive oxygen species formation measured using DCFH-DA probe in lymphocytes at a density of 1×105 cells/ml exposed to different concentrations of heterocyclic amine PhIP (0–100 μM). The results are shown as a percentage of control sample. ROS level is expressed in relative fluorescence unit.
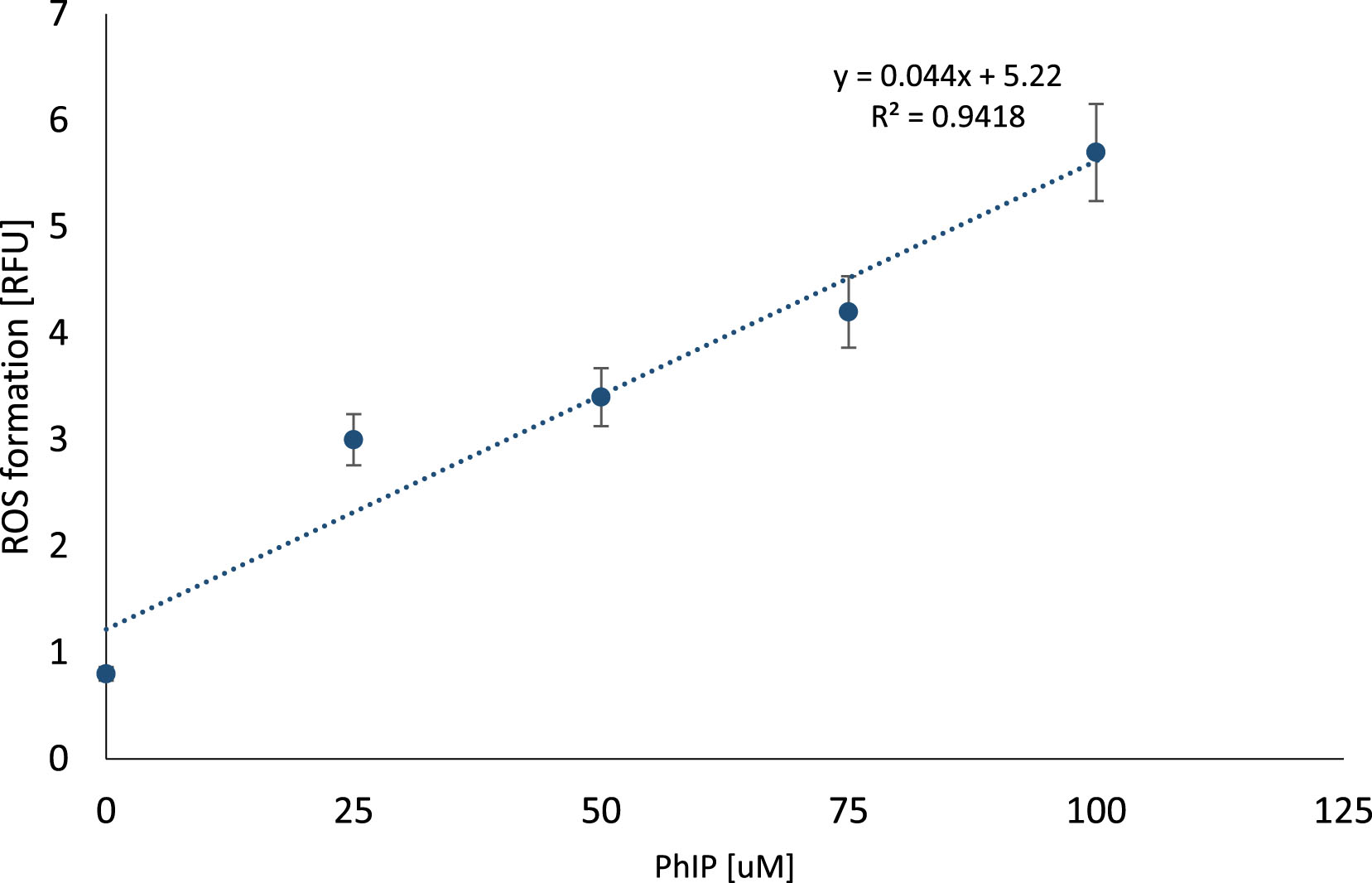
Consequently, ROS measurement was performed for lymphocytes exposed to 0.1% berry juices. In comparison to untreated cells, we noted that juices from the tested berries did not significantly increase ROS production in lymphocytes, with the exception of cranberries (p = 0.03) and Noni juices (p = 0.0002). The ROS formation was negatively correlated with polyphenols concentration in berry juices as shown in Fig. 8 (y =–0.0826x + 6.4073; R2 = 0.4603; p = 0.0399) and FRAP (y = –0.8853x + 6.0595; R2 = 0.4298; p = 0.04839).
Fig.8
The relation between polyphenols in berry juices and ROS formation in lymphocytes. The correlation between reactive oxygen species formation in lymphocytes treated with berry juices. ROS generation was measured using DCFH-DA probe in lymphocytes at a density of 1×105 cells/ml exposed for 1 hour to different 0.1% berry juices. ROS level is expressed in relative fluorescence unit.
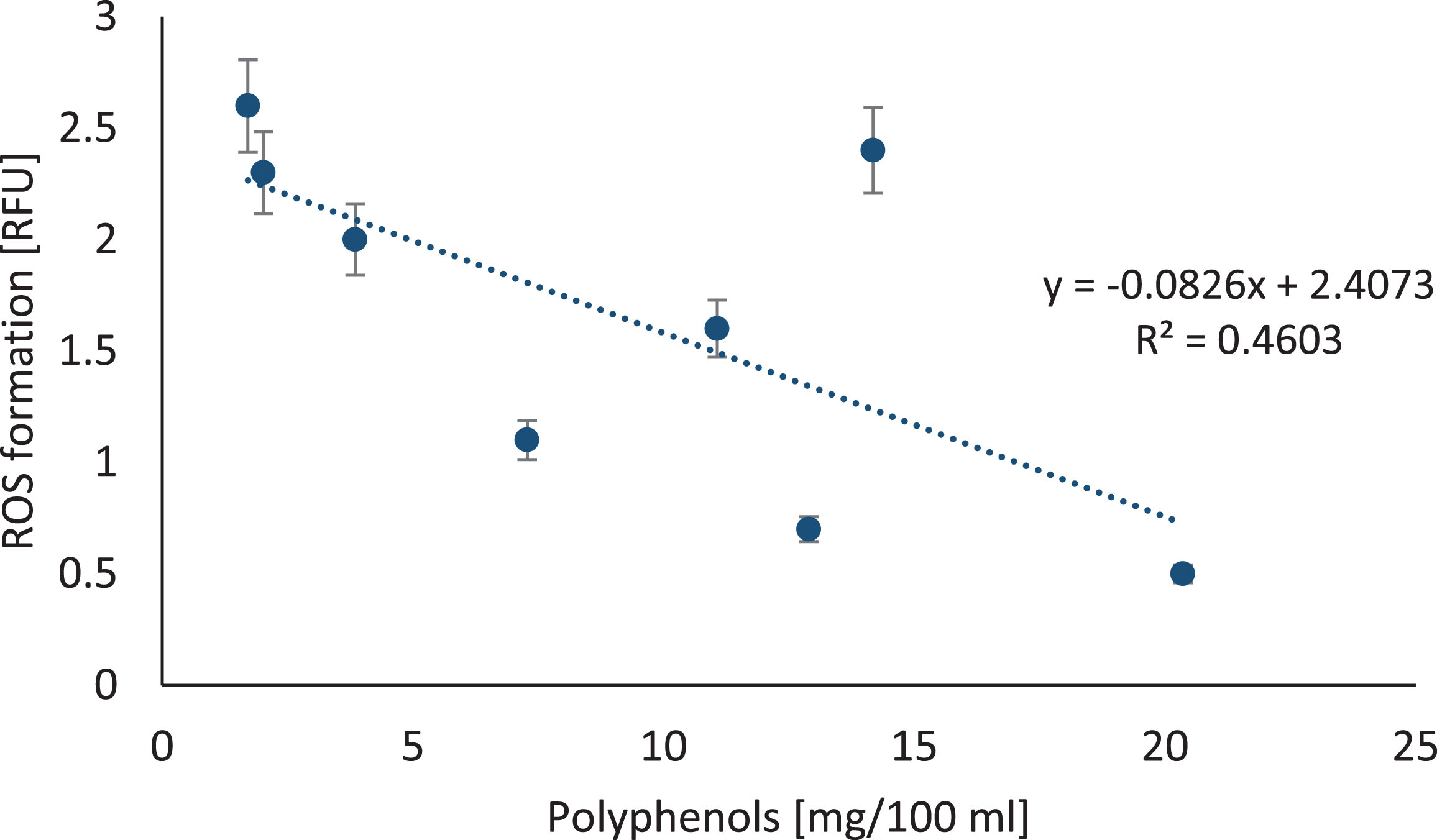
Finally, the ability of juices to decrease ROS generated by PhIP was evaluated. Therefore, the lymphocytes were simultaneously treated with 100 μM PhIP and 0.1% berry fruit juices. The results are shown in Fig. 9, including cells treated with 100 μM PhIP only, as a positive control.
Fig.9
The effect of berry juices on ROS induced in lymphocytes by PhIP. Reactive oxygen species formation measured using DCFH-DA probe in lymphocytes at a density of 1×105 cells/ml exposed for 1 hour to different 0.1% berry juices alone and to 0.1% juices and 100 μM aromatic amine PhIP simultaneously. The results are shown as a percentage of negative control sample. 100 μM PhIP was used a positive control. *Asterisks indicate the significant differences between cell treated with juices and PhIP in comparison to cells treated with PhIP only. **Double asterisks denotes the significant difference between cells treated with juice and PhIP in comparison to juice only. # Hashes indicate significant differences between the cells treated with juice only to untreated cells.
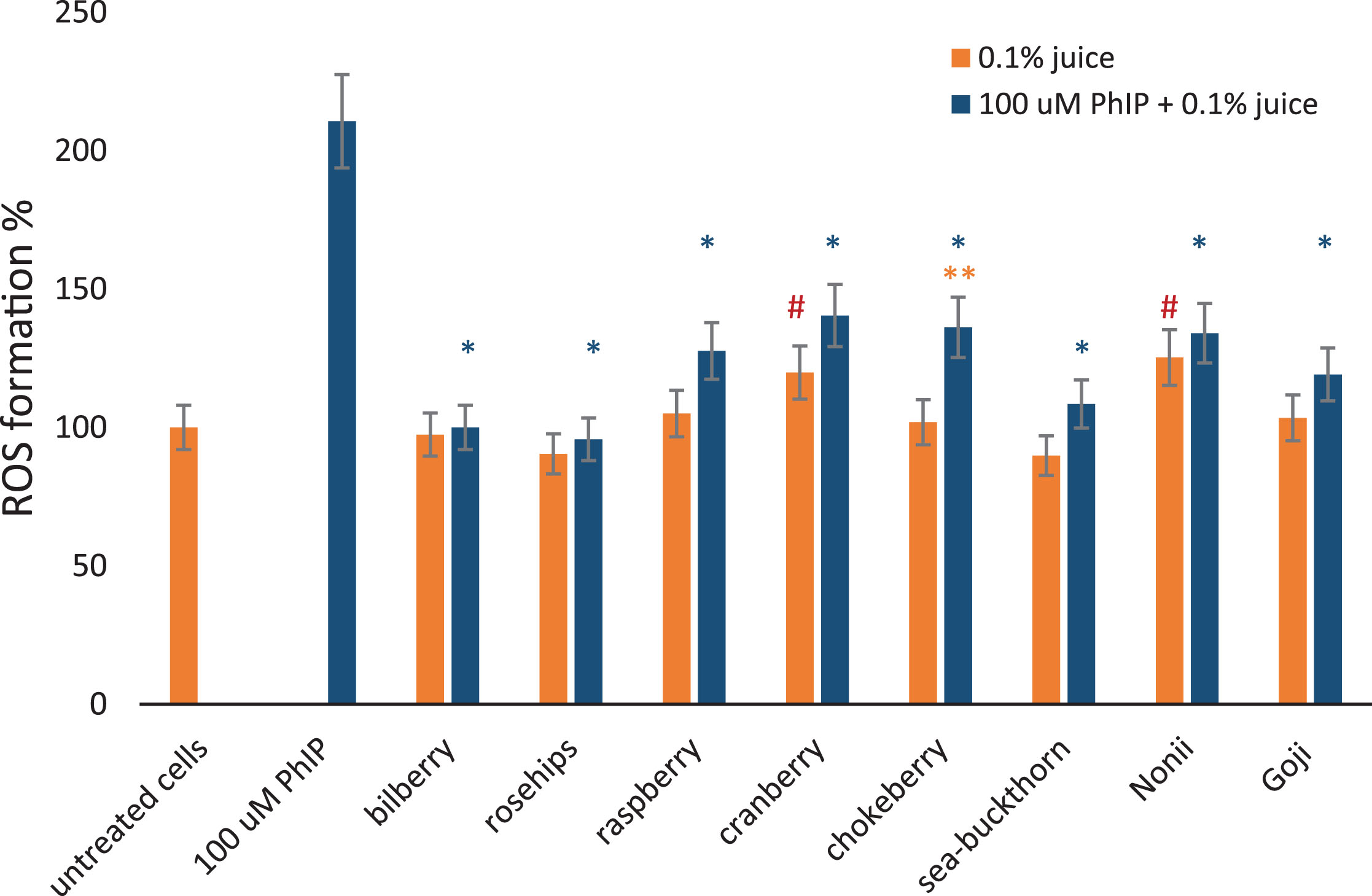
We noted that all of the investigated berry fruit juices reduced oxidative stress caused by 100 μM PhIP in lymphocytes from healthy individuals.
5Discussion
The results of this study demonstrate that PhIP leads to DNA damage induction in healthy lymphocytes in a dose-dependent manner, as it is consistent with previous reports [33–35]. Regardless of the notion that PhIP is one of the most abundant genotoxic amines in the human diet [36], the main aim of our study was to establish the effect of berry juices against the harmful effect of PhIP on healthy lymphocytes.
Fruit juices, that can be directly consumed as part of the human diet, instead of isolated phytochemicals, were chosen as the subject of this study because they presumably exhibit greater health benefits due to the synergic effect of polyphenols, vitamins and other nutrients [37, 38]. It is important to highlight that the antioxidant potential of whole dietary products should be tested, due to the unique complexity of phytochemicals contained in them. In our diet we do not consume just a mixture of carotenoids, polyphenols, vitamins, minerals, amines and other constituents. The active components function in comprehensive and balanced systems that are characterized by a broad spectrum of synergic actions, and it is these systems – not the isolated compounds – that we consume in plant food. The incubation of cells in a juice concentration of 0.1% is comparable to the polyphenol concentration in serum achieved by consumption of 60 ml of juice [18, 26] and, as has been shown, higher concentrations may reduce cell viability and increase DNA damage. However, fruits may differ in phytochemicals’ concentrations between different cultivars and due to weather, region, harvest time and stage of ripeness [39–42]. Then research using separated compounds might be helpful to understand the mechanism of observed phenomena. However, it must be stated that the study is always a simplified model whereas complex juices with the variety of phytochemical composition faces the diversity of reactions of different tissues and inter-individual variability resulting from genetic polymorphism, hormonal status and metabolic challenges. Therefore, in vitro studies are an introduction helping to evaluate possible results of in vivo research.
In the first part of experiments, we investigated whether the tested juices contain high amounts of antioxidant compounds. The studied juices differed in anthocyanins and flavonoid content. Chokeberries’, rosehips’ and bilberries’ phytochemical composition was characterized by a prevalence of anthocyanins, which is consistent with published data [43–48]. The lowest anthocyanins to total phenolic ratio was found in Goji and sea-buckthorn, which was characterized by high flavonoid content – comparable to other studies [40, 45, 49–51]. The highest flavonoid to phenolic ratio was found in Noni, however, this juice was characterized by the lowest phenolic content among studied juices, which differs from other studies [39, 52]. Possibly Noni was harvested before ripening to enable long-term transport what might explain the differences [39].
Especially high concentrations of polyphenols in Rosa rugosa juice were noted, as well as the concentrations of flavonoids and anthocyanins were the highest among studied juices [41, 43, 44, 53]. We confirmed that pasteurized juice pressed from rosehips also contains high amounts of vitamin C, over 260 mg/100 ml, while fresh fruit content was found to vary from 400 to 2500 mg/100 g [54–56]. Moreover, in this study, a significant anti-radical activity and genoprotective properties of rosehips were confirmed [32].
The bilberry (Vaccinium myrtillus) grows in North America, Europe, Asia and Africa; it is also known as wild blueberries or European blueberries to distinguish it from American blueberries (Vaccinium cyanococcus). We confirmed that main polyphenols in bilberries are anthocyanins [45–47, 53]. Bilberries pasteurized juice contained less vitamin C than fresh fruit (3,8 mg/100 ml versus 18 mg/100 g [57]). Different species of blueberries (Vaccinium) demonstrate anticarcinogenic action, especially due to their antioxidant properties [47, 53, 57–60].
Likewise, chokeberry juice revealed antioxidant and anti-inflammatory activity due to its free radical scavenging [45, 48, 61]. It was observed that chokeberry juice enhanced the tumor-promoting action of carcinogenic N-nitrosodiethylamine in rat liver [62], and in our study we also observed an effect on ROS formation in human lymphocytes incubated with PhIP, which may indicate paths to new studies on the usage of chokeberries in supportive therapy.
We confirmed that the juice of sea-buckthorn (Hippophae rhamnoides) contains flavonoids as the dominant polyphenols fraction [45, 51, 63], however, the process of pressing and pasteurization of juice causes the loss of vitamin C down to 48 mg/100 ml, while in fresh fruit it is reported at level of 114 to 1550 mg/100 g). Sea-buckthorn juice acts as a genoprotective agent and its antioxidative properties have also been confirmed [45, 51, 64].
Cranberries (Vaccinium oxycoccos), the European swamp cranberry juice, contained the prevalence of flavonoids, however, the concentration of polyphenols was much lower than reported previously [46, 47, 65, 66]. Although, some studies suggested that cranberries have genoprotective abilities [20, 67], in contrast we observed a slight ROS generation capacity of cranberry juice. Nevertheless, it did not interfere with the protective action of cranberries against PhIP-induced DNA damage.
Raspberries (Rubus idaeus) contain flavonoids and anthocyanins at a comparable level to cranberries [42, 45, 68]. Moreover, raspberries have proven their antioxidant action [42, 68].
Morinda citrifolia (commonly known as Noni fruit) is often used in the folk medicine of the Indian. The anticarcinogenic and antimutagenic effects of Noni juice were proved in Drosophila, as well as in humans [70]. However, in our study we did not confirm the reports of high phenolic content in Noni [69, 71]. Noni pressed and pasteurized juice was characterized by very low concentration of polyphenols compounds, like flavonoids and anthocyanins, and it revealed weak genoprotective abilities against PhIP induced damage. Moreover, Noni also generated ROS and some DNA damage in lymphocytes by itself. The low polyphenols content and low genoprotective capacity of Noni may indicate that the fruits were harvested before full ripening [39, 41].
Goji berry (Lycium barbarum) is the traditional Chinese medicinal plant which is valued for its abundance of bioactive like anthocyanins [72]. It was demonstrated that Goji berries decrease levels of PhIP-induced DNA damage [73–75], however, in our study Goji showed the weakest protective abilities of all of the studied fruits, although, it has high flavonoid content. The possible explanation could be a presence of some additional chemicals in juice, which block the positive effect of polyphenols. More accurate chemical analyzes are needed to explain this observation.
The present results confirm the well-established genotoxic effect of PhIP [76]. More importantly, we have shown here that berries native to the Northern Hemisphere act as antioxidants and protective agents and all tested crude berry juices are able to reduce PhIP-induced DNA damage. The protective effect may be obtained by direct antioxidant action, but also other mechanisms are possible, e.g. activation of detoxification enzymes [22, 77, 78]. The analysis of anthocyanins and flavonoids concentrations shows that both groups have a contribution to antioxidant properties of berry juices.
The antioxidant action may also arise from the vitamin C content in fruit juices, however, as it was observed in other studies, polyphenols contribute substantially to the antioxidant effect, while ascorbic acid makes a minor contribution to the total antioxidant capacity [53]. Nevertheless, juices contain less vitamin C than fresh fruit as it is partially lost during processing – juices exhibit strong antioxidant power correlated with polyphenols concentration.
We observed a dose-dependent increase of ROS formation by PhIP. Previous reports have shown that PhIP can lead to the production of ROS and DNA adduct formation in cancer cell lines [79, 80] as well as in normal cell lines (MCF-10A, normal breast epithelial cells) [34]. We have shown that antioxidant-rich berry juices can almost totally inhibit oxidative stress induced by PhIP.
6Conclusions
Our results clearly show that PhIP is responsible for DNA damage and ROS formation in non-transformed cells. Furthermore, as nontoxic agents, berry fruit juices rich in polyphenols display a great ability to reduce DNA damage and ROS formation induced by PhIP.
Due to the fact that both groups of tested samples (heterocyclic aromatic amines (PhIP) and berries) are present in our diet, the results obtained in this study provide an up-to-date understanding of the role of the interaction between components ingested on a daily basis. Testing the neutralizing capacity of fruit polyphenols towards HCAs can serve as a new strategy in the field of chemoprevention of a wide variety of cancers. Furthermore, as naturally occurring antioxidants, berries are effective in reducing the amount of free radicals generated in normal cells, which provides protection against oxidative stress. The results show that berries native to the Northern Hemisphere have strong genoprotective abilities and that the phenolic compounds are responsible for the antioxidant action of berry fruit. It is extremely important to emphasize the importance of polyphenols in maintaining health, because usually neither the nutrition data, nor any recommended dietary allowances documents contain the information on necessity of consuming phenolic compounds. The classical dietetics focuses solely on ensuring the fulfillment of the nutritional demand for vitamins, microelements and energetic components. This can lead to the illusory impression that supplementation of analytical ingredients can fulfill all of the nutritional needs. While polyphenols, especially flavonoids, seem to have important influence on human wellness and their only dietary source are plants.
Current nutrition recommendations state that a plant-based diet is considered the most beneficial for humans [81, 82], while processed meat products are proven to increase cancer risk [83, 84]. Moreover, the high ratio of vegetable and fruit intake to meat consumption is confirmed as one of the most important factors in the context of cancer development [8, 13]. The benefits of eating fruit cannot be overestimated [14, 85-86] and the more types of fruit we eat, the more benefits for our health [87]. Therefore, berries, as natural source of polyphenols, should be recommended in the daily diet.
Funding
The authors report no funding.
Conflict of interest
The authors have no conflict of interest to report.
Acknowledgments
This study was supported by the Statutory Scientific Fund of Andrzej Frycz Modrzewski Krakow University. Faculty of Biochemistry, Biophysics and Biotechnology of Jagiellonian University is a partner of the Leading National Research Center (KNOW) supported by the Ministry of Science and Higher Education.
References
[1] | Anand P , Kunnumakara AB , Sundaram C , Harikumar KB , Tharakan ST , Lai O , Sung B , Aggarwal BB . Expert Review Cancer is a Preventable Disease that Requires Major Lifestyle Changes. Pharm Res. (2008) ;25: , 2097–2116. doi: 10.1007/s11095-008-9661-9 |
[2] | El-zein R , Etzel CJ , Lopez MS , Gu Y , Spitz MR , Strom SS . Human sensitivity to PhIP: A novel marker for prostate cancer risk. Mutat Res-Fund Mol M. (2006) ;601: :1–10. doi: 10.1016/j.mrfmmm.2006.05.023 |
[3] | Tavan E , Cayuela C , Antoine J , Trugnan G , Chaugier C , Cassand P . Effects of dairy products on heterocyclic aromatic amine-induced rat colon carcinogenesis. Carcinogenesis. (2002) ;23: , 477–483. doi: 10.1093/carcin/23.3.477 |
[4] | Nakagama M , Nakanishi M , Ochiai M . Modeling human colon cancer in rodents using a food-borne carcinogen, PhIP. Cancer Sci. (2005) ;96: , 627–636. doi: 10.1111/j.1349-7006.2005.00107.x |
[5] | Oz F , Kaban G , Kaya M . Effects of cooking methods and levels on formation of heterocyclic aromatic amines in chicken and fish with Oasis extraction method. Food Sci Technol. (2010) ;43: :1345–1350. doi: 10.1016/j.lwt.2010.04.014 |
[6] | Jamali M , Teng H , Zhang Y , Peng Z . Inhibitory Effect of Rosa rugosa Tea Extract on the Formation of Heterocyclic Amines in Meat Patties. Molecules. (2016) ;21: :173. doi: 10.3390/molecules21020173 |
[7] | Rohrmann S , Hermann S , Linseisen J . Heterocyclic aromatic amine intake increases colorectal adenoma risk: Findings from a prospective European cohort study. Am J Clin. (2009) ;89: :1418–1424. doi: 10.3945/ajcn.2008.26658 |
[8] | Kapiszewska M . A vegetable to meat consumption ratio as a relevant factor determining cancer preventive diet. The Mediterranean versus other European countries. Forum Nutr. (2006) ;59: :130–153. doi: 10.1159/000095211 |
[9] | Vanamala J . Food Systems Approach to Cancer Prevention. Crit Rev Food Sci Nutr. (2015) ;57: (12):2573–2588. doi: 10.1080/10408398.2015.1028023 |
[10] | Boeing H , Bechthold A , Bub A , Ellinger S , Haller D , Kroke A , Leschik-Bonnet E , Müller MJ , Oberritter H , Schulze M , Stehle P , Watzl B . Critical review: Vegetables and fruit in the prevention of chronic diseases. Eur J Nutr. (2012) ;51: (6):637–663. doi: 10.1007/s00394-012-0380-y |
[11] | Afrin S , Giampieri F , Gasparrini M , Forbes-Hernandez TY , Varela-López A , Quiles JL , Mezzetti B , Battino M . Chemopreventive and Therapeutic Effects of Edible Berries: A Focus on Colon Cancer Prevention and Treatment. Molecules. (2016) ;21: :169. doi: 10.3390/molecules21020169 |
[12] | Aqil F , Jeyabalan J , Kausarb H , Munagalaa R , Singhd IP , Gupta R . Lung cancer inhibitory activity of dietary berries and berry polyphenolics. J Berry Res. (2017) ;6: :105–114. doi: 10.3233/JBR-160120 |
[13] | de Carvalho AM , Carioca AA , Fisberg RM , Qi L , Marchioni DM . Joint association of fruit, vegetable, and heterocyclic amine intake with DNA damage levels in a general population. Nutrition. (2016) ;32: (2):260–4. doi: 10.1016/j.nut.2015.08.018 |
[14] | López-Romero D , Izquierdo-Vega JA , Morales-González JA , Madrigal-Bujaidar E , Chamorro-Cevallos G , Sánchez-Gutiérrez M , Betanzos-Cabrera G , Alvarez-Gonzalez I , Morales-González Á , Madrigal-Santillán E . Evidence of Some Natural Products with Antigenotoxic Effects. Part 2: Plants, Vegetables, and Natural Resin. Nutrients. (2018) ;10: :1954. doi: 10.3390/nu10121954 |
[15] | Wu S , Tian L . Diverse Phytochemicals and Bioactivities in the Ancient Fruit and Modern Functional Food Pomegranate (Punica granatum). Molecules. (2017) ;22: pii: E1606. doi: 10.3390/molecules22101606 |
[16] | Diaconeasa Z , Leopold L , Rugină D , Ayvaz H , Socaciu C . Antiproliferative and antioxidant properties of anthocyanin rich juices from blueberry and blackcurrant juice. Int J Mol Sci. (2015) ;16: , 2352–2365. doi: 10.3390/ijms16022352 |
[17] | Baba AB , Kowshik J , Krishnaraj J , Sophia J , Dixit M , Nagini S . Blueberry inhibits invasion and angiogenesis in 7,12-dimethylbenz [a]anthracene (DMBA)-induced oral squamous cell carcinogenesis in hamsters via suppression of TGF-β and NF-κB signaling pathways. J Nutr Biochem. (2016) ;35: , 37–47. doi: 10.1016/j.jnutbio.2016.06.002 |
[18] | Nguyen V , Tang J , Oroudjev E , Lee CJ , Marasigan C , Wilson L , Ayoub G . Cytotoxic effects of bilberry extract on MCF7-GFP-tubulin breast cancer cells. J Med Food. (2010) ;13: , 278–285. doi: 10.1089/jmf.2009.0053 |
[19] | Deng Y , Li Y , Yang F , Zeng A , Yang S , Luo Y , Zhang Y , Xie Y , Ye T , Xia Y , Yin W . The extract from Punica granatum (pomegranate) peel induces apoptosis and impairs metastasis in prostate cancer cells. Biomed Pharmacother. (2017) ;93: , 976–984. doi: 10.1016/j.biopha.2017.07.008 |
[20] | Izquierdo-Vega JA , Morales-González JA , SánchezGutiérrez M , Betanzos-Cabrera G , Sosa-Delgado SM , Sumaya-Martínez MT , Morales-González Á , Paniagua-Pérez R , Madrigal-Bujaidar E , Madrigal-Santillán E . Evidence of Some Natural Products with Antigenotoxic Effects. Part 1: Fruits and Polysaccharides. . Nutrients. (2017) ;9: (2):pii: E102. doi: 10.3390/nu9020102 |
[21] | Cheng KW , Wu Q , Zheng ZP , Peng X , Simon JE , Chen F , Wang M . Inhibitory effect of fruit extracts on the formation of heterocyclic amines. J Agric Food Chem.. (2007) ;55: (25):10359–65. doi: 10.1021/jf071820z |
[22] | Platt KL , Edenharder R , Aderhold S , Muckel E , Glatt H . Fruits and vegetables protect against the genotoxicity of heterocyclic aromatic amines activated by human xenobiotic-metabolizing enzymes expressed in immortal mammalian cells. Mutat Res. (2010) ;703: , 90–98. doi: 10.1016/j.mrgentox.2010.08.007 |
[23] | Zhao M , Wang P , Li D , Shang J , Hu X , Chen F . Protection against neo-formed contaminants (NFCs)-induced toxicity by phytochemicals. Food Chem Toxicol. (2017) ;108: , (Pt B):392–406. doi: 10.1016/j.fct.2017.01.023 |
[24] | Cierniak A , Łabno A , Winiarski M , Dembiński M , Budzyński P , Kalemba-Drożdż M . “Wpływ kurkuminy na uszkodzenia DNA indukowane mutagennym składnikiem żywności (heterocykliczną aminą aromatyczną) w limfocytach pacjentów z nowotworem jelita grubego”, Państwo i Społeczeństwo, Medycyna i Zdrowie Publiczne. (2017) ;(4), 23–40. doi: 10.31749/pismzp2017/19183 |
[25] | Cierniak A , Skubal M , Kalemba-Drożdż M . Czy galusan epigallokatechiny może być skutecznym polifenolem w terapii skojarzonej z etopozydem w leczeniu przewlekłej białaczki szpikowej? Państwo i Społeczeństwo, Medycyna i Zdrowie Publiczne. (2018) ;(3), s 9–28. doi: 10.31749/pismzp2018/20836 |
[26] | Hollman PC , van Trijp JM , Mengelers MJ , de Vries JH , Katan MB . Bioavailability of the dietary antioxidant flavonol quercetin in man. Cancer Lett. (1997) ;114: :139–140. doi: 10.1016/s0304-3835(97)04644-2 |
[27] | Kalemba-Drożdż M , CierniakA. Antioxidant and genoprotective properties of extracts from edible flowers. J Food Nutr Res. (2019) ;58: (1), 42–50. doi: 10.13140/RG.2.2.17195.49442/1 |
[28] | Medina MB . Simple and rapid method for the analysis of phenolic compounds in beverages and grains. J Agric Food Chem. (2011) ;59: (5):1565–71. doi: 10.1021/jf103711c |
[29] | Szeto YT , Chu WK , Benzie IF . Antioxidants in fruits and vegetables: a study of cellular availability and direct effects on human DNA. Biosci Biotechnol Biochem. (2006) ;70: (10):2551–5. doi: 10.1271/bbb.60224 |
[30] | Kim D , JeongSW, LeeCY. Antioxidant capacity of phenolic phytochemicals from various cultivars of plums. Food Chem. (2003) ;81: , 321–326. doi: 10.1016/S0308-8146(02)00423-5 |
[31] | AOAC No. 88.1269 Official Method 2005.02. Total monomeric anthocyanin pigment content of fruit juices, beverages, natural colorants, and wines, pH Differential method, First Action 2005. In: Horwitz,W. – Latimer,G. (Ed.): Official methods of analysis of AOAC International. 18th Ed. Gaithersburg : AOAC International, (2005) , pp. 1269–1278. |
[32] | Kalemba-Drożdż M , CierniakA. Wpływ róż na zdrowie – farmakologiczne i biochemiczne działanie ekstraktów z płatków Rosa rugosa i Rosa damascena. Współczesne kierunki w medycynie prewencyjnej. (red.) Anna Goździalska, Jerzy Jaśkiewicz. Kraków: Oficyna Wydawnicza AFM (2013) ;127–138. |
[33] | Kurzawa-Zegota M , Najafzadeh M , Baumgartner A , Andrson D . The protective effect of the flavonoids on food-mutagen-induced DNA damage in peripheral blood lymphocytes from colon cancer patients. Food Chem Toxicol. (2012) ;50: :124–129. doi: 10.1016/j.fct.2011.08.020 |
[34] | Jain A , Samykutty A , Jackson C , Browning D , Bollag WB , Thangaraju M , Takahashi S , Singh SR . Curcumin inhibits PhIP induced cytotoxicity in breast epithelial cells through multiple molecular targets. Cancer Lett. (2015) ;365: ,122–131. doi: 10.1016/j.canlet.2015.05.017 |
[35] | Pezdirc M , Zegura B , Filipic M . Genotoxity and induction od DNA damage responsive by food-borna heterocyclic aromatic amines in human hepatoma HepG2 cells. Food Chem Toxicol. (2013) ;59: , 386–394. doi: 10.1016/j.fct.2013.06.030 |
[36] | Richling E , Häring D , Herderich M , Schreier P . Determination of heterocyclic aromatic amines (HAA) in commercially available meat products and fish by high performance liquid chromatography– electrospray tandem mass spectrometry (HPLC–ESI-MS–MS). Chromatographia. (1998) ;48: , 258–262. doi: 10.1007/BF02467680 |
[37] | Lansky E , Harrison G , Froom P , Jiang W . Pomegranate (Punica granatum) pure chemical show possible synergistic inhibition of human PC-3 prostate cancer cell invasion across Matrigel. Investig. New Drugs. (2018) ;23: :121–122. doi: 10.1007/s10637-005-5856-7 |
[38] | Seeram NP , Adams LS , Henning SM , Niu Y , Zhang Y , Nair MG , Heber D . In vitro antiproliferative, apoptotic and antioxidant activities of punicalagin, ellagic acid and a total pomegranate tannin extract are enhanced in combination with other polyphenols as found in pomegranate juice. J. Nutr. Biochem. (2005) ;16: , 360–367. doi: 10.1016/j.jnutbio.2005.01.006 |
[39] | Singh DR , Singh S , Banu VS . Changes in Antioxidants and Minerals in Noni (Morinda citrifolia L.) Fruits during Development Process. Br J Pharm Res. (2016) ;10: (5):1–11, Article no.BJPR23836. doi: 10.9734/BJPR/2016/23836 |
[40] | Islam T , Xiaoming Yu , Badwal TS , Xu B . Comparative studies on phenolic profiles, antioxidant capacities and carotenoid contents of red goji berry (Lycium barbarum) and black goji berry (Lycium ruthenicum). Chem Cent J. (2017) ;11: :59. doi: 10.1186/s13065-017-0287-z |
[41] | Elmastaş M , Demir A , Genç N , Dölek Ü , Güneş M . Changes in flavonoid and phenolic acid contents in some Rosa species during ripening. Food Chem. (2017) ;235: :154–159. doi: 10.1016/j.foodchem.2017.05.004 |
[42] | Ponder A , Hallmann E . The effects of organic and conventional farm management and harvest time on the polyphenol content in different raspberry cultivars. Food Chem. (2019) ;301: :125295. doi: 10.1016/j.foodchem.2019.125295 |
[43] | Abací ZT , Zarífíkhosroshahí M , Kafkas E , Sevindik E . Characterization of bioactive compounds in rosehip species from East Anatolia region of Turkey. It J Food Sci. (2016) ;28: (2):314–325. |
[44] | Bhave A , Schulzova V , Chmelarova H , Mrnka L , Hajslova J . Assessment of rosehips based on the content of their biologically active compounds. J Food Drug Anal. (2017) ;25: (3):681–690. doi: 10.1016/j.jfda.2016.12.019 |
[45] | Tian Y , Liimatainen J , Alanne AL , Lindstedt A , Liu P , Sinkkonen J , Kallio H , Yang B . Phenolic compounds extracted by acidic aqueous ethanol from berries and leaves of different berry plants. Food Chem. (2017) ;220: :266–281. doi: 10.1016/j.foodchem.2016.09.145 |
[46] | Díaz-García MC , Obón JM , Castellar MR , Collado J , Alacid M . Quantification by UHPLC of total individual polyphenols in fruit juices. Food Chem. (2013) Jun 1;138: (2-3):938–49. doi: 10.1016/j.foodchem.2012.11.061 |
[47] | Bujor OC , Le Bourvellec C , Volf I , Popa VI , Dufour C . Seasonal variations of the phenolic constituents in bilberry (Vaccinium myrtillus L.) leaves, stems and fruits, and their antioxidant activity. . Food Chem. (2016) Dec 15;213: :58–68. doi: 10.1016/j.foodchem.2016.06.042 |
[48] | Stanisavljević N , Samardžić J , Janković T , Šavikin K , Mojsin M , Topalović V , Stevanović M . Antioxidant and antiproliferative activity of chokeberry juice phenolics during in vitro simulated digestion in the presence of food matrix. Food Chem. (2015) May 15;175: :516–22. doi: 10.1016/j.foodchem.2014.12.009 |
[49] | Protti M , Gualandi I , Mandrioli R , Zappoli S , Tonelli D , Mercolini L . Analytical profiling of selected antioxidants and total antioxidant capacity of goji (Lycium s) berries. J Pharm Biomed Anal. (2017) Sep 5;143: :252–260. doi: 10.1016/j.jpba.2017.05.048 |
[50] | Zhang Q , Chen W , Zhao J , Xi W . Functional constituents and antioxidant activities of eight Chinese native goji genotypes. Food Chem. (2016) Jun 1;200: :230–6. doi: 10.1016/j.foodchem.2016.01.046 |
[51] | Fatima T , Kesari V , Watt I , Wishart D , Todd JF , Schroeder WR , Paliyath G , Krishna P . Metabolite profiling and expression analysis of flavonoid, vitamin C and tocopherol biosynthesis genes in the antioxidant-rich sea buckthorn (Hippophae rhamnoides L.). Phytochemistry. (2015) ;118: :181–91. doi: 10.1016/j.phytochem.2015.08.008 |
[52] | Lin YL , Chang YY , Yang DJ , Tzang BS , Chen YC . Beneficial effects of noni (Morinda citrifolia L.) juice on livers of high-fat dietary hamsters. Food Chem. (2013) ;140: (1-2):31–8. doi: 10.1016/j.foodchem.2013.02.035 |
[53] | Hidalgo GI , Almajano MP . Red Fruits: Extraction of Antioxidants, Phenolic Content, and Radical Scavenging Determination: A Review. Antioxidants. (2017) ;6:7: :1–27. doi: 10.3390/antiox6010007 |
[54] | Gu D , Yang Y , Bakri M , Chen Q , Xin X , Aisa HA . A LC/QTOF-MS/MS application to investigate chemical compositions in a fraction with protein tyrosine phosphatase 1B inhibitory activity from Rosa rugosa flowers. Phytochem Anal. (2013) ;24: , 661–670. doi: 10.1002/pca.2451 |
[55] | Cunja V , Mikulic-Petkovsek M , Weber N , Jakopic J , Zupan A , Veberic R , Stampar F , Schmitzer V . Fresh from the ornamental garden: hips of selected rose cultivars rich in phytonutrients. J Food Sci. (2016) ;81: :C369–379. doi: 10.1111/1750-3841.13220. |
[56] | Olech M , Nowak R , Pecio Ł , Łoś R , Malm A , Rzymowska J , Oleszek W . Multidirectional characterisation of chemical composition and health-promoting potential of Rosa rugosa hips. Nat Prod Res. (2017) ;31: :667–671. doi: 10.1080/14786419.2016.1180601 |
[57] | Baby B , Antony P , Vijayan R . Antioxidant and anticancer properties of berries. Crit Rev Food Sci Nutr. (2017) ;13: :1–17. doi: 10.1080/10408398.2017.1329198 |
[58] | Johnson SA , Arjmandi BH . Evidence for anti-cancer properties of blueberries: A mini-review. Anticancer Agents Med Chem. (2013) ;13: :1142–1148. |
[59] | Wang E , Liu Y , Xu C , Liu J . Antiproliferative and proapoptotic activities of anthocyanin and anthocyanidin extracts from blueberry fruits on B16-F10 melanoma cells. Food Nutr Res. (2017) ;61: :1325308. doi: 10.1080/16546628.2017.1325308 |
[60] | Katsube N , Iwashita K , Tsushida T , Yamaki K , Kobori M . Induction of apoptosis in cancer cells by Bilberry (Vaccinium myrtillus) and the anthocyanins. J Agric Food Chem. (2003) ;51: :68–75. doi: 10.1021/jf025781x |
[61] | Jurikova T , Mlcek J , Skrovankova S , Sumczynski D , Sochor J , Hlavacova I , Snopek L , Orsavova J . Fruits of Black Chokeberry Aronia melanocarpa in the Prevention of Chronic Diseases. Molecules. (2017) ;22: , pii: E944. doi: 10.3390/molecules22060944 |
[62] | Kujawska M , Kant P , Mayoral IH , Ignatowicz E , Sikora J , Oszmianski J , Czapski J , Jodynis-Liebert J . Effect of Chokeberry Juice on N-Nitrosodiethylamine-Induced Rat Liver Carcinogenesis. J Agric Food Chem. (2016) ;35: (4):317–331. doi: 10.1615/JEnvironPatholToxicolOncol.2016014030 |
[63] | Xu YJ , Kaur M , Dhillon RS , Tappia PS , Dhalla NS . Health benefits of sea buckthorn for the prevention of cardiovascular diseases. J Funct Foods. (2011) ;3: , 2–12. doi: 10.3389/fphar.2018.00232 |
[64] | Geetha S , Sai Ram M , Singh V , Ilavazhagan G , Sawhney RC . Anti-oxidant and immunomodulatory properties of seabuckthorn (Hippophae rhamnoides) – an in vitro study. J Ethnopharmacol. (2002) ;79: :373–378. doi: 10.1016/s0378-8741(01)00406-8 |
[65] | Jurikova T , Skrovankova S , Mlcek J , Balla S , Snopek L . Bioactive Compounds, Antioxidant Activity, and Biological Effects of European Cranberry (Vaccinium oxycoccos). Molecules. (2018) ;24: (1). pii: E24. doi: 10.3390/molecules24010024 |
[66] | Baranowska M , Bartoszek A . Antioxidant and antimicrobial properties of bioactive phytochemicals from cranberry. Postepy Hig Med Dosw (Online). (2016) ;70: (0):1460–1468. doi: 10.5604/17322693.1227896 |
[67] | Vu KD , Carlettini H , Bouvet J , Côté J , Doyon G , Sylvain J-F , Lacroix M . Effect of different cranberry extracts and juices during cranberry juice processing on the antiproliferative activity against two colon cancer cell lines. Food Chem. (2012) ;132: , 959–967. doi: 10.1016/j.foodchem.2011.11.078 |
[68] | Szymanowska U , Baraniak B , Bogucka-Kocka A . Antioxidant, Anti-Inflammatory, and Postulated Cytotoxic Activity of Phenolic and Anthocyanin-Rich Fractions from Polana Raspberry (Rubus idaeus L.) Fruit and Juice-In Vitro Study. Molecules. (2018) ;23: (7). pii: E1812. doi: 10.3390/molecules23071812 |
[69] | Franchi LP , Guimarães NN , De Andrade LR , De Andrade HH , Lehmann M , Dihl RR , Cunha KS . Antimutagenic and antirecombinagenic activities of Noni fruit juice in somatic cells of Drosophila melanogaster. An Acad Bras Cienc. (2013) ;85: , 585–594. doi: 10.1590/S0001-37652013000200008 |
[70] | Wang MY , Peng L , Jensen CJ , Deng S , West BJ . Noni juice reduces lipid peroxidation-derived DNA adducts in heavy smokers. Food Sci Nutr. (2013) ;1: :141–149. doi: 10.1002/fsn3.21 |
[71] | Wang MY , Su C . Cancer preventive effect of Morinda citrifolia (Noni). Ann N Y Acad Sci. (2001) ;952: :161–168. doi: 10.1111/j.1749-6632.2001.tb02737.x |
[72] | Donno D , Beccaro GL , Mellano MG , Cerutti AK , Bounous G . Goji berry fruit (Lycium s): Antioxidant compound fingerprint and bioactivity evaluation. J Funct Foods. (2015) ;18: :1070–1085. doi: 10.1016/j.jff.2014.05.020 |
[73] | Lin KH , Yang YY , Yang CM , Huang MY , Lo HF , Liu KC , Lin HS , Chao PY . Antioxidant activity of herbaceous plant extracts protect against hydrogen peroxide-induced DNA damage in human lymphocytes. BMC Res Notes. (2013) ;6: , 490. doi: 10.1186/1756-0500-6-490 |
[74] | Wawruszak A , Czerwonka A , Okła K , Rzeski W . Anticancer effect of ethanol Lycium barbarum (Goji berry) extract on human breast cancer T47D cell line. Nat Prod Res. (2016) ;30: :1993–1996. doi: 10.1080/14786419.2015.1101691 |
[75] | Lee YJ , Ahn Y , Kwon O , Lee MY , Lee CH , Lee S , Park T , Kwon SW , Kim JY . Dietary Wolfberry Extract Modifies Oxidative Stress by Controlling the Expression of Inflammatory mRNAs in Overweight and Hypercholesterolemic Subjects: A Randomized, Double-Blind, Placebo-Controlled Trial. J Agric Food Chem. (2017) ;65: , 309–316. doi: 10.1021/acs.jafc.6b04701 |
[76] | Gooderham NJ , Creton S , Lauber SN , Zhu H . Mechanisms of action of the carcinogenic heterocyclic amine PhIP. Toxicol Lett. (2007) ;168: , 269–277. doi: 10.1016/j.toxlet.2006.10.022 |
[77] | Hofmann T , Liegibel U , Winterhalter P , Bub A , Rechkemmer G , Pool-Zobel BL . Intervention with polyphenol-rich fruit juices results in an elevation of glutathione S-transferase P1 (hGSTP1) protein expression in human leucocytes of healthy volunteers. Mol Nutr Food Res. (2006) ;50: :1191–1200. doi: 10.1002/mnfr.200600177 |
[78] | Weisel T , Baum M , Eisenbrand G , Dietrich H , Will F , Stockis JP , Kulling S , Rüfer C , Johannes C , Janzowski C . An anthocyanin/polyphenolic-rich fruit juice reduces oxidative DNA damage and increases glutathione level in healthy probands. Biotechnol J. (2006) ;1: , 388–397. doi: 10.1002/biot.200600004 |
[79] | Lauber SN , Gooderham N J . The cooked meat derived genotoxic carcinogen 2-amino-3-methylimidazo [4, 5-b]pyridine has potent hormone-like activity: mechanistic support for a role in breast cancer. Cancer Res. (2007) ;67: , 9597–9602. doi: 10.1158/0008-5472.CAN-07-1661 |
[80] | Tang D , Liu JJ , Rundle A , Neslund-Dudas C , Savera AT , Bock CH , Nock NL , Yang JJ , Rybicki BA . Grilledmeatconsumption and PhIP-DNA adducts in prostate carcinogenesis. Cancer Epidemiol Biomarkers Prev. (2007) ;16: , 803–808. doi: 10.1158/1055-9965.EPI-06-0973 |
[81] | George VC , Dellaire G , Rupasinghe HP . Plant flavonoids in cancer chemoprevention: Role in genome stability. J Nutr Biochem. (2016) ;45: :1–14. doi: 10.1016/j.jnutbio.2016.11.007 |
[82] | Nelson ME , Hamm MW , Hu FB , Abrams SA , Griffin TS . Alignment of Healthy Dietary Patterns and Environmental Sustainability: A Systematic Review. AdvNutr. (2016) ;7: :1005–1025. doi: 10.3945/an.116.012567 |
[83] | Diallo A , Deschasaux M , Latino-Martel P , Hercberg S , Galan P , Fassier P , Allès B , Guéraud F , Pierre FH , Touvier M . Red and processed meat intake and cancer risk: Results from the prospective NutriNet-Santé cohort study. Int J Cancer. (2018) ;15;142: (2):230–237. doi: 10.1002/ijc.31046 |
[84] | Fretts AM , Howard BV , Siscovick DS , Best LG , Beresford SA , Mete M , Eilat-Adar S , Sotoodehnia N , Zhao J . Processed Meat, but Not Unprocessed Red Meat, Is Inversely Associated with Leukocyte Telomere Length in the Strong Heart Family Study. J Nutr. (2016) ;146: (10):2013–2018. doi: 10.3945/jn.116.234922 |
[85] | Rodriguez-Casado A . The Health Potential of Fruits and Vegetables Phytochemicals: Notable Examples. Crit Rev Food Sci Nutr. (2016) ;56: (7):1097–107. doi: 10.1080/10408398.2012.755149 |
[86] | Miller V , Mente A , Dehghan M , Rangarajan S , Zhang X , Swaminathan S , Dagenais G , Gupta R , Mohan V , Lear S , Bangdiwala SI , Schutte AE , Wentzel-Viljoen E , Avezum A , Altuntas Y , Yusoff K , Ismail N , Peer N , Chifamba J , Diaz R , Rahman O , Mohammadifard N , Lana F , Zatonska K , Wielgosz A , Yusufali A , Iqbal R , Lopez-Jaramillo P , Khatib R , Rosengren A , Kutty VR , Li W , Liu J , Liu X , Yin L , Teo K , Anand S , Yusuf S . Fruit, vegetable, and legume intake, and cardiovascular disease and deaths in 18 countries (PURE): A prospective cohort study. Lancet. (2017) ;390: , No. 10107, p2037–2049. doi: 10.1016/S0140-6736(17)32253-5 |
[87] | Lachat C . Dietary species richness as a measure of food biodiversity and nutritional quality of diets. Proc Natl Acad Sci USA. (2018) ;115: (1):127–132. doi: 10.1073/pnas.1709194115 |