Wild Andean blackberry (Rubus glaucus Benth) and Andean blueberry (Vaccinium floribundum Kunth) from the Highlands of Ecuador: Nutritional composition and protective effect on human dermal fibroblasts against cytotoxic oxidative damage
Abstract
BACKGROUND:
Andean blackberries (Rubus glaucus Benth) and Andean blueberries (Vaccinium floribundum Kunth) are wild berries consumed and commercialised by the indigenous people of the Andean regions of Ecuador.
OBJECTIVE:
This study aims to determine the chemical composition and the ability of A. blackberries and A. blueberries to protect human dermal fibroblast (HDFa) against cytotoxic oxidative damage.
METHODS:
Total phenolic, flavonoid, anthocyanins and tannins content were determined spectrophotometrically, while vitamin C and carotenes were determined by HPLC. Total antioxidant capacity was determined by the Ferric Reducing Antioxidant Power (FRAP) assay, the hydrogen peroxide scavenging activity and the DPPH and superoxide radical scavenging capacity. HDFa was pre-treated with A. blackberries or A. blueberries crude extract, subjected to a model of oxidative stress using the stressor 2,2’-azobis(2-amidinopropane) dihydrochloride (AAPH) and the markers of cell cytotoxic damage (intracellular ROS levels, catalase, superoxide dismutase and glutathione activities, lipid peroxidation, protein oxidation and ATP and nitrite levels) were determined.
RESULTS:
A. blueberries showed significantly high values of total phenolic, flavonoid, anthocyanin, lutein tannin content, whilst A. blackberries showed the highest values of vitamin C and β-carotene. After HDFa was pre-incubated with crude extracts of A. blackberries and A. blueberries, the markers of oxidative damage were significantly improved compared with the stressed cells group. In all cases, crude extract of A. blueberries showed a higher protective effect compared to A. blackberries.
CONCLUSION:
A. blackberry and A. blueberry attenuated the oxidative damage in HDFa showing that both fruits may represent a relevant source of bioactive compounds with promising benefits for human health.
1Introduction
Numerous epidemiological studies draw attention to the high correlation between a diet based on fruit and vegetables and lower incidences of different chronic pathologies, such as diabetes, cardiovascular disease, neurodegenerative diseases and cancer. The beneficial effect of this dietary pattern has been principally associated with the contribution of different bioactive compounds, such as flavonoids, anthocyanins and vitamin C [1]. Red fruits, especially berries, have been widely studied for their important beneficial effects on health. Berries are a group of interest because of their high content of vitamin C, folates, anthocyanins and ellagitannins, as well as other phenolic compounds that contribute to their high antioxidant capacity and beneficial effects on human health [2–4].
There are accumulating studies indicating the benefits of consuming berries in counteracting the pathophysiological processes that predispose people to chronic pathologies, which justify the studies on this fruit group worldwide [3–5]. Recently, South American berries have been an object of increased interest due to their chemical composition and potential health benefits [6, 7]. The Andean blackberry and Andean blueberry are wild berries that grow in isolation, scattered or in groups with other species in the high inter-Andean valleys of Ecuador. They are collected, consumed and commercialised by the indigenous people of these regions and by the Ecuadorian population in general in the form of jams, wine and boiled drinks such as “Colada morada” [7, 8]. Few studies have been carried out regarding Andean berries; for example in Ecuador, there are only a few reports that describe their chemical composition and biological effects [9–12]. Thus, the aim of this study was to investigate the chemical composition of two of the most representative wild berries from the Highlands of Ecuador and their capacity to protect human dermal fibroblasts against oxidative damage as an indicator of their possible health benefits.
2Materials and methods
2.1Fruit collection
The ripe, wild Andean blackberries (Rubus glaucus Benth) and Andean blueberries (Vaccinium floribundum Kunth) (Fig. 1) were collected in the Highlands of Ecuador, located at an altitude surpassing 3000 metres above sea level in the provinces of Tungurahua, Pichincha and Cotopaxi. The berries were collected on two different occasions between September and December 2016 and the specimens were identified by specialists at the Jardín Botánico de Quito, Ecuador, using the reference samples found in the herbarium of this centre. The edible parts of the fruits were chopped and freeze-dried; after that, samples were ground to a fine powder and stored at −20°C until analysis.
Fig. 1
Whole and halved Andean blackberry (Rubus glaucus Benth) (A) and Andean blueberry (Vaccinium floribundum Kunth) (B) fruits.
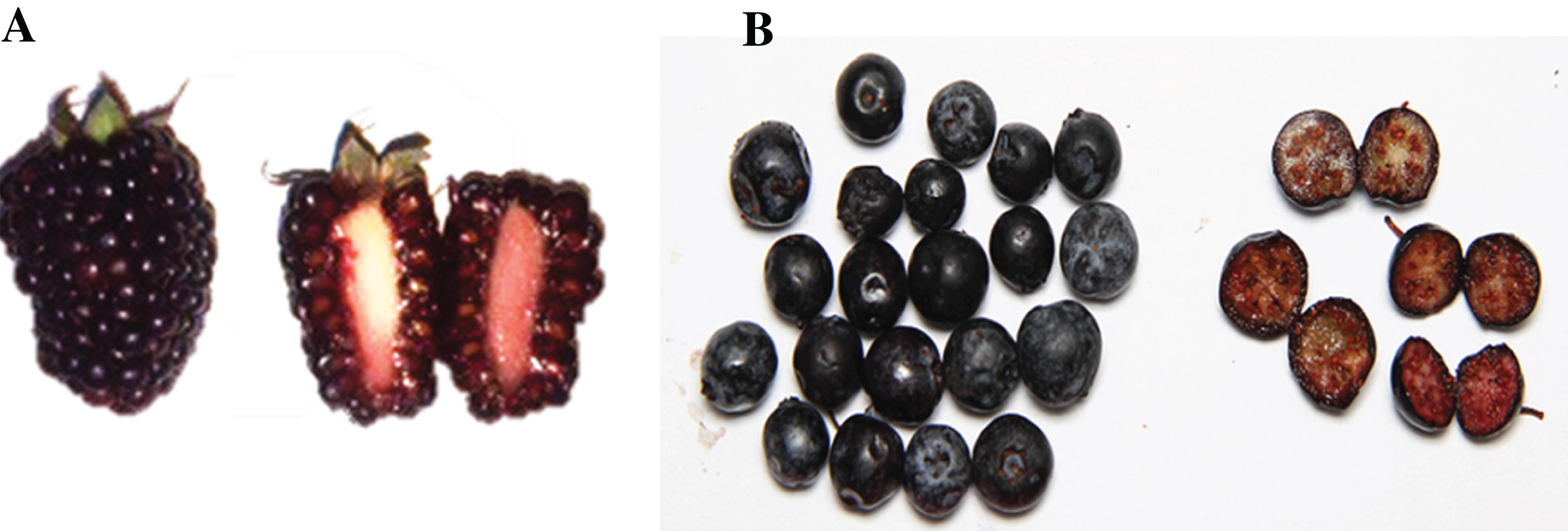
2.2Spectrophotometric determination of total phenolic content (TPC), total flavonoid content (TFC), total anthocyanins content (ACYs) and total tannins content (TTC)
A hydroalcoholic extract was obtained using a methanol-water solution (80:20, v/v) according to a previously described method [13]. The extract was used for the studies of total antioxidant capacity (TAC), total polyphenols, flavonoid and anthocyanin content.
TPC was determined using the Folin-Ciocalteu method [14] and results were expressed as milligrams of gallic acid equivalents (GAEq) per gram of fresh weight (FW) of fruits (mg GAEq/g FW). TFC was determined using the aluminium chloride method [15] and results were expressed as milligrams of catechin equivalents (CatEq) per gram of FW of fruits (mg CatEq/g FW), whilst ACYs content was determined using a modified pH differential method [16] and results were expressed as milligrams of Pg-3-gluc equivalents (PgEq) per gram of FW of fruits (mg PgEq/g FW).
TTC was determined directly from the lyophilized fruit in accordance with the standard 9648 ISO [17] and results were expressed as milligrams of tannic acid equivalents (TAEq) per gram of FW of fruits (mg TAEq/g FW).
2.3HPLC-DAD quantificacion of ascorbic acid
Ascorbic acid was determined as previously reported [13]. In short, 10 ml of the extraction solution (metaphosphoric acid and acetic acid, 73/84, p/v) was added to 0.5 g of freeze-dried fruit powder. The solution obtained was sonicated for 20 mins, filtered through a 0.45μm syringe filter and immediately analysed on an HPLC system. Analyses were performed using an HPLC system (Agilent Technologies Series 1260, Santa Clara, California, United States) consisting of a Quaternary Pump Agilent Technologies 1260 Infinity G1312B and a Diode Array Detector (DAD) Agilent Technologies 1260 Infinity G1315C set at absorbances of 245 nm. An Eclipse Plus C18 (5μm, 4.6×150 mm) was used as the stationary phase and elution was performed with 50 mM KH2PO4 (pH 2.5) at a flow rate of 1 ml/min for 20 min and the results were expressed as mg of vitamin C per 100 g of FW of fruits (mg Vit C/100 g FW).
2.4HPLC-DAD determination and quantification of carotenoid content
Carotenoid content was determined as previously reported [13]. Five grams of freeze-dried plant powder was added to 100 ml of chloroform, stirred for 24 hours in the dark at room temperature and filtered through a 0.45μm Minisart filter (PBI International). The carotenoid extract was dried in a vacuum and 3 grams of the dry residue was subjected to saponification by the reflux method using 100 ml of a KOH (5%) methanolic solution for 4 hours at 50 °C in the dark. After saponification, the extract was combined with 100 ml of petroleum ether, then 100 ml of distilled water was added and the organic layer was collected and dried in a vacuum in a rotary evaporator. The dried residue was dissolved in methanol-isopropanol (35:65, v/v), filtered through a 0.45μm syringe filter and analysed on an HPLC system. The HPLC-DAD system (Agilent Technologies Series 1260, Santa Clara, California, United States) consisted of a Quaternary Pump Agilent Technologies 1260 Infinity G1312B and a Diode Array Detector (DAD) Agilent Technologies 1260 Infinity G1315C set at an absorbance of 450 nm and equipped with a Eclipse Plus C18 (5μm, 4.6×250 mm) as the stationary phase. Elution was performed with methanol-isopropanol (35:65, v/v) in an isocratic gradient at a flow rate of 1 ml/min for 15 mins. β-carotene, lutein and lycopen contents were quantified using a calibration curve of the corresponding standard compound and the results were expressed as μg per 100 g of FW of fruits.
2.5Total antioxidant capacity (TAC) and superoxide radical (O2·)− and hydrogen peroxide (H2O2) scavenging activities
The TAC of hydroalcoholic extracts was determined using the Ferric Reducing Antioxidant Power (FRAP) assay [18] and the 2.2-diphenyl-1-picrylhydrazyl free radical method (DPPH) [19] and results were expressed as μmol of Trolox equivalents (TEq) per gram of fresh weight (FW) of fruits (μmol TEq per g FW) for both assays.
Superoxide radical (O2· -) scavenging capacities were determined based on the reduction of nitroblue tetrazolium into a purple-coloured diformazan [20] and the O2·− scavenging activity was expressed as mmol of α-tocopherol equivalents (αTEq) per g of FW of fruits (mmol αTEq/g FW). The capacity to scavenge hydrogen peroxide (H2O2) was determined according to the method previously described [21] and results were expressed as mmol of ascorbate equivalents (AscEq) per g of FW of fruits (mmol AscEq/g FW).
2.6Protective effect of crude extract of berries on human dermal fibroblasts against oxidative damage
2.6.1Cell line and treatments
Human Dermal Fibroblasts, adult (HDFa, ATCC® PCS-201-012trademark), were cultivated in 25cm2 flasks in Eagle’s Minimum Essential Medium (EMEM) supplemented with 10% fetal bovine serum, 2 mM of glutamine, and 1% penicillin-streptomycin antibiotics (100 IU/ml penicillin and 100μg/ml streptomycin) in a humidified atmosphere with 5% CO2 at 37°C and the medium was changed every 2-3 days. The hydroalcoholic extracts of both berries (Andean blackberry and Andean blueberry) were dried in a vacuum to eliminate total methanol and the resulting crude extracts (Andean blackberry, BlackCExt and Andean blueberry, BlueCExt) were resuspended in EMEM to achieve the final concentration of 100μg/ml. The stressor 2,2’-azobis(2-amidinopropane) dihydrochloride (AAPH) (10 mM) was used as an inductor of the oxidative damage in HDFa. Control cells were treated with EMEM only (Ctrl), Blackberry crude extract for 24 hrs (BlackCExt), Blueberry crude extract for 24 hrs (BlueCExt), AAPH (10 mM) for 24 hrs (AAPH) and BlackCExt or BlueCExt for 24 hrs and then with AAPH (10 mM) for 24 hrs (BlackCExt + AAPH or BlueCExt + AAPH). The effective dose/time of both BlackCExt and BlueCExt was determined in previous cytotoxicity assays using the MTT assay and represents the minimum concentration that showed protective effect against the citotoxic damage induced by the AAPH as compared to the control (P≤0.05) (data not shown). The cytotoxic concentration of AAPH (10 mM), used as oxidant, was also determined through preliminary cytotoxicity assays and was selected according to its capacity to decrease cell vitality (∼50 %) as compared to the control (P≤0.05).
2.6.2Cell viability
Cell viability was determined using the MTT assay [22]. After each treatment, cells were washed twice with a phosphate buffered saline solution (PBS) and incubated with a salt solution of MTT (3-[4,5-dimethylthiazole-2-yl]-2,5-diphenyltetrazolium bromide) at a concentration of 0.5 mg/ml for 2 hours at 37°C. Then the medium was removed, the crystals were dissolved in dimethyl sulfoxide (DMSO) and the optical density was read at 550 nm using a microplate reader (Synergy HT, Biotek, Winooski, VT, USA). Cell viability was expressed as the percentage of live cells as compared to controls.
2.6.3Measurement of intracellular reactive oxygen species (ROS) levels
Intracellular ROS levels were determined using the 2′-7′-dichlorofluorescin diacetate (DCFH) kit (Merck, Germany) according to the manufacturer’s instructions. Cells were first treated with the different treatments previously described in section 2.7.1 and then incubated with 5μmol/l DCFH at 37°C for 30 mins under light protection. Fluorescence intensity was read at an excitation wavelength of 485 nm and an emission wavelength of 530 nm using a Microplate Reader | BioTek Instruments (Winooski, VT, USA) and results were expressed as arbitrary units of fluorescence intensity/μg cell protein.
2.6.4Determination of oxidative damage markers: catalase, superoxide dismutase and glutathione activities, lipid peroxidation and protein oxidation
Cells were lysed using the RIPA buffer and then stored at –80°C until analyses. Catalase activity (CAT) was determined spectrophotometrically at 240 nm [23], whilst superoxide dismutase (SOD) activity was determined at 540 nm on the basis of inhibition of the formation of NADH-phenazine methosulphate-nitroblue tetrazolium formazan by SOD [24] and results were expressed as units per milligram of protein per minute (U/mg prot/min) for both assays. Glutathione peroxidase (GPx), glutathione reductase (GR) and glutathione transferase (GST) [25] were also determined and results were expressed as nmol of NADPH oxidized per milligram of protein per minute (nmol of NADPH oxidized/mg protein/min) for GPx and GR and nmol CDNB-GSH conjugate/mg protein/min for GST. Lipid oxidation damage was determined in parallel using the thiobarbituric acid-reactive substances (TBARS) assay [26] and hydroperoxides levels [27] and the results were expressed as μM for both assays, whilst protein carbonyl levels were analysed with the 2,4-dinitrophenylhydrazine methods (DNPH method) [28] and results were expressed as nmol per milligram of protein (nmol /mg of protein).
2.6.5Measurement of cell adenosine triphosphate (ATP) levels
ATP was quantified from cells in culture using the ATP Cell Titer Glo® assay (Promega, Madison, WI, U.S.A.) according to the manufacturer’s instructions. After treatments, the medium was removed and cells were washed with 200μl of PBS. 100μl of PBS was added to each well and then 100μl of the Cell Titer Glo reagent was also added to each one. Cells were incubated with reagent according to the manufacturer’s instructions and 200μl of the supernatant was transferred to the black 24-well clear-bottom plate, luminescence was determined using a microplate reader (BioTek Instruments, Winooski, VT, USA) and results were expressed as fluorescence units×1000 cells.
2.6.6Measurement of nitrite levels
The nitrite levels were determined using the Griess Reagent Kit for nitrite quantitation (Thermo Fisher Scientific, USA) according to the manufacturer’s instructions. Cell supernatant (100μl) was combined with 100μl of the Griess reagent and the absorption of the coloured compound formed by the interaction. NO2 – / Griess reagent was determined at 550 nm using the microplate reader (BioTek InstrumentWinooski, VT, USA). NaNO2 (10–100μM) was used as standard and results were expressed as μM of NaNO2/105 cells.
2.7Statistical analysis
Statistical analyses were performed using IBM SPSS Statistic for Windows version 2.0. The samples were analysed in triplicate and results are reported as mean±standard deviation (SD). Data between different groups were analysed statistically using a one-way ANOVA and Tukey’s post hoc test; P≤0.05 was considered as significant and P≤0.01 highly significant.
3Results and discussion
3.1Chemical composition and total antioxidant capacity
The Andean blackberry (Rubus glaucus Benth) and Andean blueberry (Vaccinium floribundum Kunth) from the highlands of Ecuador were studied to determine their chemical composition and protective effects on human dermal fibroblast against oxidative damage. The contents of bioactive compounds in both fruit types are presented in Table 1. According to the results, the Andean blackberry and Andean blueberry are relevant natural sources of bioactive compounds with important antioxidant capacities. TPC and TFC in the Andean blackberry were within the range of values previously reported for Andean blackberries from Ecuador [11] and blackberries from other geographical regions [29, 30], but lower than those values reported in the United States [31]. However, compared to other berries, the values found in Andean blackberries were in line with those values previously reported in strawberries [2] and cherries [9, 32], whilst they were higher than those reported in raspberries [4]. The Andean blueberry showed significantly higher TPC and TFC values than in the Andean blackberry as well as in strawberries [2], blackberries [3] and raspberries [4], while the values were in line with those previously reported in blueberries from other geographical regions [31]. The phenolic profile in the Andean blackberry and Andean blueberry has been previously studied, wherby researchers noted the presence of several phenolic groups that have been associated with important healthy effects. In the Andean blackberry, phenolics acids, such as gallic and p-coumaric acid derivates as well as proanthocyanidins and quercetin and kaempferol derivates [11] have been reported, whilst the Andean blueberry proved to be an important source of gallic acid, hydroxybenzoic acid derivates (vanillic and p-hydrobenzoic acid), flavan-3-ols and proanthocyanidins (cathechin and epicatechin), flavonol derivates (quercetin and myricetin), as well as hydroxyciannamic acid derivates (chlorogenic, caffeic, ferulic and p-coumaric acids) [12]. These compounds have been related to important health beneifts via the use use of several biological models [33].
Table 1
Chemical composition of the Andean blackberry and Andean blueberry
Analyses | A. blackberry | A. blueberry |
---|---|---|
Total phenolic content (TPC) (mg GAE/g FW) | 2.66±0.31a | 9.25±1.41b |
Total flavonoid content (TFC) (mg CE/g FW) | 1.25±0.14a | 6.56±0.75b |
Total anthocyanins content (ACY) (mg PgEq/g FW) | 0.49±0.03a | 1.94±0.72b |
Total tannins content (TTC) (mg TAEq/g FW). | 0.95±0.09a | 4.19±0.85 |
Vitamin C (mg Vit C/100 g FW) | 92.92±11.51a | 45.94±6.75b |
Total carotenoids content (ug/100 g FW) | ||
β-carotene content | 91.79±2.06a | 70.63±2.01b |
Lutein content | 538.66±27.51a | 866.61±7.52b |
Data is mean±standard deviation. The samples were analysed in triplicate. Mean values in a column marked with different letters are significantly different for P < 0.05.
Within the most representative bioactive compounds in red fruits, anthocyanins represent a prominent group which has been related to important beneficial health effects, such as their capacity to stimulate several pathways associated with antioxidant responses, as well as with anti-inflammatory, antiproliferative, antitumoral, antithrombotic and antiatherosclerotic properties [33]. Similar to TPC and TFC, the ACYs content in Andean blackberries was within the range of values previously reported in blackberries from other geographical regions [3, 34] and other berries, such as in strawberries [35], Ecuadorian capuli cherries [36] and raspberries [4]. The Andean blueberry showed ACYs values that were significantly higher than those values reported in Andean blackberres as well as in strawberries [2], Ecuadorian capuli cherries [36] and raspberries [4], but the values were within the range of those previously reported in different Vaccinium genotypes [31, 37]. The ACYs content was also previously reported for both the Andean blackberry and blueberry, showing cyanidin and pelargonidin glycosides as the most representative anthocyanins in the Andean blackberry [11], whilst in the Andean blueberry only cyanidin has been reported [12].
In addition to ACYs, hydrolysable tannins have often been identified as active principles in plants [38]. TTC was significantly higher (P≤0.01) in Andean blueberries in comparison to the results reported here regarding Andean blackberries (Table 1). Although TTC has been reported by means of more sensitive methods than the one used in our study, when our results are compared with those, it was observed that in all cases, the values obtained in Andean blueberries were higher than those reported in strawberries and raspberries [2, 4], whilst the values obtained in Andean blackberries were in line with those previously reported in blackberries [3].
Red fruits are also known to be an important natural source of vitamin C. The total vitamin C content in both fruit types was determined by HPLC-DAD analysis. In this case, the vitamin C values in Andean blackberries were significantly higher (P≤0.01) than those found in Andean blueberries (Table 1). According to the results here exposed, the vitamin C content in Andean blackberries was higher than those values previously reported in other blackberry cultivars, strawberries, raspberries and cherries from Ecuador [2–4, 36]. Variations in vitamin C content in fruits have been associated with various factors, such as the degree of maturity and storage and climatic conditions (including temperature and radiation). Ascorbate is a major metabolite in plants, with an important function as an antioxidant that, associated with other antioxidants, protects plants against oxidative damage [39]. The high inter-Andean valleys of Ecuador are located at an altitude surpassing 3000 metres above sea level, with average temperatures of 6°C to 8°C during the day, which can drop to 0°C during the night [40, 41]. Therefore, we assume that the high vitamin C content in Andean blackberries could be associated with the critical environmental conditions to which the berries are exposed.
The carotenoid content in both berry types is present in Table 1. The results here exposed clearly show the differences in the carotenoid composition between both berry types. We found significantly higher carotenoid values (P≤0.01) in Andean blueberries than in Andean blackberries. Lutein was the most predominant carotenoid, which is in agreement with Marinova and Ribarova’s study [42]. However, our results differ with those previously reported by the aforementioned authors, as they reported that blackberries had higher carotenoid contents (440μg/100 g) than blueberries (290μg/100 g) [42]. In our opinion, this difference could be due to the differences in the ecosystems in which these plants grow. Andean blueberries grow at higher altitudes (3000 m above sea level) and are constantly exposed to high levels of radiation and low temperatures (between 6 and 8°C) [40], as compared to other blueberries which grow mostly in warmer periods (May to August) at lower altitudes. The Andean blueberry also presented a higher carotenoid content than other berries, such as capuli cherries from Ecuador [36], strawberries, raspberries, redcurrants and blackcurrants from Bulgaria [42] and other berries from different geografical regions [43]. Although berries are not considered to be a representative source of carotenoids and since data in literature about carotenoid content in berries is limited, we consider the results here exposed to be significant. Our results show that in the Andean berries here studied, the carotenoid content could be compared and equated with other berries and tropical frutis [42, 43]. These results could also support the hypothesis that the carotenoid content in Andean berries could contribute significantly to their biological properties, in addition to other predominant bioactive compounds, such as anthocyanins and vitamin C.
The TAC of hydroalcoholic extracts, as well as the capacity to scavenge and activity against O2·− and H2O2, was also determined (Fig. 2). According to the result here exposed, both hydroalcoholic extracts were efficient electron donors, being able to reduce Fe3 + to Fe2 + as well as to quench the DPPH·, H2O2 and O2·− radicals. In all the TAC assays, the results in Andean blueberries were significantly highly (P≤0.01) than those in Andean blackberries (Fig. 2A and B). Our results are in agreement with those previously reported by other authors [6, 7, 11]. On the other hand, both berry types had higher TAC values than other fruits from Ecuador [44] and other geographical regions [29, 30, 32, 45].
Fig. 2
Total antioxidant capacity (FRAP and DPPH assay) (A) and O2·- and H2O2 radical scavenging activities (B) of the Andean blackberry and Andean blueberry crude extracts. Results are reported as mean±SD of three experiments. **P≤0.01, significant differences compared to the Andean blackberry. Superoxide radical (O2·-) scavenging capacities were expressed as mmol of α-tocopherol equivalents (αTEq) per g of FW (mmol αTEq/g FW), whilst the capacity to scavenge hydrogen peroxide (H2O2) was expressed as mmol of ascorbate equivalents (AscEq) per g of FW (mmol AscEq/g FW).
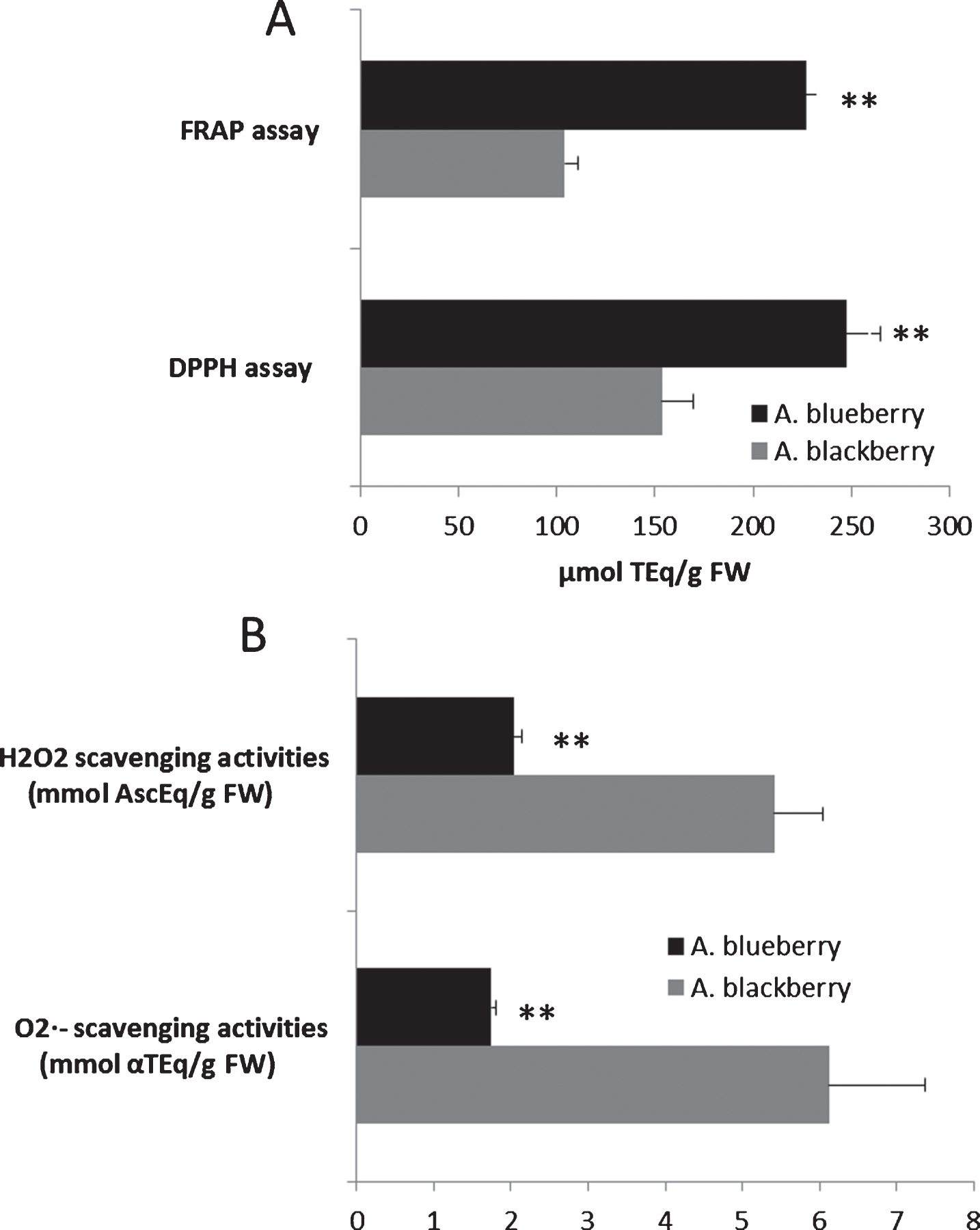
3.2Protective effect againts oxidative damage in HDFa
Berry fruits have been reported as an important natural source of bioactive compounds with important health effects [2–4]. However, while the properties related to the health and chemical composition of berries that are commonly consumed in North America and Europe have been widely studied, in the case of the Andean berries, as in Ecuador, they are still generally unknown. There are few reports about the chemical composition of Andean berries [11, 12], while studies related to its healthy effects are even scarcer [10, 36]. Here, to the best of our knowledge, we present the first report about the protective effect of two of the most representative Ecuadorian berries against the oxidative damage using an in vitro cellular model of human dermal fibroblasts (HDFa). The protective effect of both BlackCExt and BlueCExt was evidenced when HDFa cells were incubated with BlackCExt or BlueCExt for 24 hrs before inducing the oxidative damage with AAPH for 24 hrs. Pre-incubation with BlackCExt or BlueCExt was able to increase cell vitality compared to cells treated with AAPH (P≤0.01), where BlueCExt showed a more protective effect compared to BlackCExt (P≤0.05) (data not shown).
The pre-incubation with BlackCExt and BlueCExt was able to significantly reduce the intracellular ROS levels compared with the AAPH-stressed cells (Fig. 3A), where BlueCExt significantly reduced the intracellular ROS levels compared to BlackCExt (P≤0.05) and respect to the cells treated with AAPH (P≤0.01). Cellular ATP levels were also measured to determine mitochondrial damage in cells (Fig. 3B). Similar to the above results, the treatment with AAPH significantly decreased (P≤0.01) the ATP levels with respect to the control cells, which showed significant damage to mitochondrial functionality. However, when cells were incubated with BlackCExt or BlueCExt, these levels were significantly improved (P≤0.01) compared with the AAPH stressed cells, where BlueCExt once again showed the best protection capacity compared to BlackCExt (P≤0.05). Our results are in agreement with those previously reported that demonstrated the protective effect of several berry extract and honey against mitochondrial oxidative damage mediated in HDFa [9, 13].
Fig. 3
Intracellular ROS (A), ATP (B) and NO levels (C) in HDFa cells. Cont (Control cells), treatment with Andean blackberry and Andean blueberry crude extracts (BlackCExt or BlueCExt), treatment with the stressor AAPH (AAPH), and pre-treatment with BlackCExt or BlueCExt and then stressed with AAPH (BlackCExt + AAPH; BlueCExt + AAPH). Results are reported as mean±SD of three experiments. *P≤0.05, **P≤0.01, significant differences compared to control; #P≤0.05, # #P≤0.01, significant differences between AAPH and pre-treatment with BlackCExt or BlueCExt and $P≤0.05, $$ P≤0.01, significant differences between BlackCExt or BlueCExt pre-treatment.
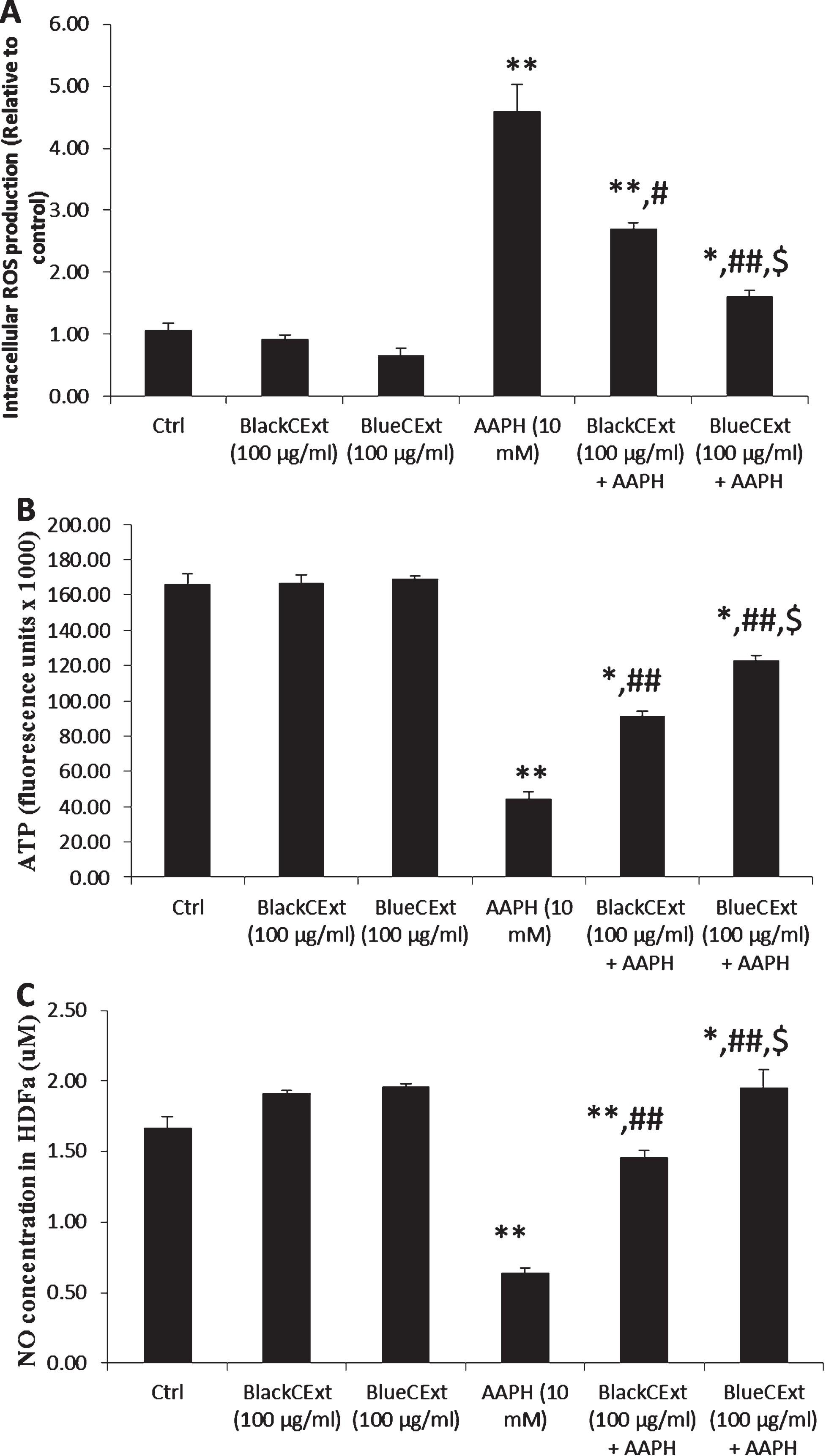
BlackCExt and BlueCExt were also able to protect macromolecules and the antioxidant enzymes against the oxidative damage in HDFa cells (Table 2). When HDFa cells were pre-incubated with BlackCExt or BlueCExt, the oxidative damage in lipids and proteins was significantly lower (P≤0.05) when compared with the AAPH-stressed cells. AAPH treatment also significantly affected the activity of the GPx, GST and GR antioxidant enzymes compared to the control (P≤0.05); however, their activity was significantly improved (P≤0.05) in comparison to the AAPH-stressed cells when HDFa cells were pre-incubated with BlackCExt or BlueCExt (Table 2). In all cases, BlueCExt showed the best protective effects compared with BlackCExt (P≤0.05). Our results are in agreement with those previously exposed that demonstrated the efficacy of berries against oxidative damage, highlighting their ability to exert direct antioxidant effects by scavenging reactive oxidant species and to improve antioxidant defenses [9, 13] through the activation the AMP-activated protein kinase signalling cascade, reinforcing the hypothesis about the direct effect of polyphenols in the molecular pathways related to the antioxidant response [46].
Table 2
Biomarkers of oxidative damage in HDFa cells treated with both A. blackberry and A. blueberry crude extracts, the stressor AAPH and with A. blackberry and A. blueberry crude extracts and then with AAPH
Treatments | Catalase (U/mg prot/min) | SOD (U/mg prot/min) | GPx (nmol NADPH oxidized/ mg protein/min) | GR (nmol CDNB-GSH conjugate/mg protein/min) | GST (nmol CDNB-GSH conjugate/mg protein/min) | TBARS (μM) | Lipid hydroperoxides (μM) | Protein carbonyl (nmol/mg prot) |
---|---|---|---|---|---|---|---|---|
Ctrl | 38.24±0.61a | 64.43±3.64a | 243.62±12.64a | 241.52±16.32a | 506.20±22.21a | 4.12±0.76a | 57.22±4.01a | 0.56±0.21a |
BlackCExt (100μg/ml) | 36.14±0.42a | 65.31±4.41a | 247.93±21.53a | 248.88±9.21a | 510.42±20.54a | 4.06±0.38a | 59.02±3.22a | 0.51±0.12a |
BlueCExt (100μg/ml) | 47.43±0.74b | 74.21±3.71b | 291.52±16.31b | 288.21±12.83b | 583.21±18.76b | 4.04±0.32a | 57.67±6.32a | 0.58±0.18a |
AAPH (10 mM) | 10.95±0.21c | 22.73±2.51c | 97.13±4.61c | 114.84±10.47c | 226.83±16.42c | 7.96±0.98b | 106.84±6.18b | 2.58±0.86b |
BlackCExt (100μg/ml) + AAPH | 21.43±0.69d | 41.91±1.82d | 168.31±12.52d | 197.94±6.31d | 412.96±26.34d | 5.81±0.54c | 82.34±6.10c | 1.72±0.09c |
BlueCExt (100μg/ml) + AAPH | 30.64±0.96e | 56.74±4.74e | 206.33±11.77e | 228.66±13.50e | 478.55±17.47e | 5.96±0.61c | 68.84±4.51d | 0.83±0.41d |
Results are reported as mean±SD of three experiments. Columns belonging to the same set of data with different superscript letters are significantly different (P≤0.05).
The capacity of BlackCExt and BlueCExt to protect againt oxidative damage could also be associated with the capacity of certain bioactive compounds, such as polyphenols, to reduce intracellular ROS levels and to protect macromolecules, such as DNA, lipids and proteins, against oxidative damage [1]. Recently, it has been suggested that polyphenol activity could be linked to the modulation of different molecular pathways related to antioxidant response, such as the activation of the gene transcription of nuclear factor-erythroid 2-related factor 2 (Nrf2), regulating the response of antioxidant enzymes such as catalase and superoxide dismutase [47]. Polyphenols could also act indirectly on the activation of the AMPK/SIRT1(5’-adenosine monophosphate-activated protein kinase/sirtuin 1) signaling cascade, increasing cellular metabolism, mitochondrial biogenesis and functionality [5], and in this way justifying their protective effect against oxidative damage.
Since nitrite is a well-known mediator of normal tissue repair [48], the ability of BlackCExt and BlueCExt to induce nitrite production in HDFa was studied to determine their capacity to induce tissue repair (Fig. 3C). When HDFa cells were stressed with AAPH, the nitrite levels significantly decreased (P≤0.01) compared to control cells, indicating the possible damage to tissue repair that can cause oxidative stress. However, when HDFa were pre-incubated with both BlackCExt and BlueCExt, the nitrite levels were significantly higher compared to AAPH-stressed cells (P≤0.01), which allows us to infer the capacity of BlackCExt and BlueCExt to induce a repair of the dermal tissue affected by oxidative damage. The capacity of several steroidal glycosides from Easter lily (Lilium longiflorum Thunb.) bulbs or Manuka honey to promote dermal fibroblast migration in vitro through the stimulation of nitrite production has been previously reported [49, 50]. Therefore, our results could explain, to a certain degree, the possible topical use of both extracts to promote cellular migration and proliferation against tissue oxidative damage to promote skin tissue repair.
In conclusion, the results here exposed demonstrate that the Andean blackberry and Andean blueberry, similarly to others berries, represent an important dietary source of bioactive compounds, such as polyphenols, carotenes and vitamin C. To the best of our knowledge, this study is the first research data related to the ability of both the Andean blackberry and Andean blueberry to counteract oxidative stress in human dermal fibroblasts due to their capacity to efficiently reduce intracellular ROS levels and improve antioxidant defences and mitochondrial funtionability in AAPH-treated cells. BlueCExt showed a higher effect compared to BlackCExt, which could be, to a certain extent, due to the high content of bioactive compounds such as flavonoids and anthocyanins found in this fruit. The results obtained in this study may serve as a basis for future animal or human studies designed in order to confirm the potential of these fruits for the prevention of oxidative damage and skin injury and justify their use as a source of bioactive compounds with relevant health benefits.
Acknowledgments
This study was funded by Universidad de Las Américas (UDLA) (grant number VET.JMA.17.05), Quito, Ecuador. Access to plant genetic resources was granted on the basis of the “FRAMEWORK AGREEMENT FOR ACCESS TO PLANT GENETIC RESOURCES: MAE-DNB-CM-2017-0072” celebrated between the Ministry of the Environment (MAE), Ecuador and the Universidad de Las Américas (UDLA), Ecuador.
References
[1] | Baum JI , Howard LR , Prior RL , Lee SO . Effect of Aronia melanocarpa (Black Chokeberry) supplementation on the development of obesity in mice fed a high-fat diet. J Berry Res. (2016) ;6: (2):203–12. |
[2] | Giampieri F , Tulipani S , Alvarez-Suarez JM , Quiles JL , Mezzetti B , Battino M. The strawberry: Composition, nutritional quality, and impact on human health. Nutrition. (2012) ;28: (1):9–19. |
[3] | Kaume L , Howard LR , Devareddy L . The Blackberry Fruit: Areview on its composition and chemistry, metabolism and bioavailability, and health benefits. J Agric Food Chem. (2012) ;60: (23):5716–27. |
[4] | Zhang X , Sandhu A , Edirisinghe I , Burton-Freeman B . Anexploratory study of red raspberry (Rubus idaeus L.) (poly)phenols/metabolites in human biological samples. Food Funct. (2018) ;9: (2):806–18. |
[5] | Afrin S , Gasparrini M , Forbes-Hernandez TY , Reboredo-Rodriguez P , Mezzetti B , Varela-Lopez A , Giampieri F , Battino M. Promising health benefits of the strawberry: A focus on clinical studies. J Agric Food Chem. (2016) ;64: (22):4435–49. |
[6] | Schreckinger ME , Lotton J , Lila MA , de Mejia EG. Berries from South America: A comprehensive review on chemistry, health potential, and commercialization. J Med Food. (2010) ;13: (2):233–46. |
[7] | Carrillo-Perdomo E , Aller A , Cruz-Quintana SM , Giampieri F , Alvarez-Suarez JM . Andean berries from Ecuador: A review on botany, agronomy, chemistry and health potential. J Berry Res. (2015) ;5: (2):49–69. |
[8] | Ortiz J , Marín-Arroyo MR , Noriega-Domínguez MJ , Navarro M , Arozarena I. Color, phenolics, and antioxidant activity of blackberry (Rubus glaucus Benth.), blueberry (Vaccinium floribundum Kunth.), and apple wines from ecuador. J Food Sci. (2013) ;78: (7):C985–C93. |
[9] | Alvarez-Suarez JM , Giampieri F , Gasparrini M , Mazzoni L , Santos-Buelga C , Gonzáilez-Paramás AM , Forbes-Hernández TY , Afrin S , Páez-Watson T , Quiles JL , Battino M. The protective effect of acerola (Malpighia emarginata) against oxidative damage in human dermal fibroblasts through the improvement of antioxidant enzyme activity and mitochondrial functionality. Food Funct. (2017) ;8: (9):3250–8. |
[10] | Llivisaca S , Manzano P , Ruales J , Flores J , Mendoza J , Peralta E , Cevallos-Cevallos JM . Chemical, antimicrobial, and molecular characterization of mortiño (Vaccinium floribundum Kunth) fruits and leaves. Food Sci Nutr. (2018) . 10.1002/fsn3.638 |
[11] | Vasco C , Riihinen K , Ruales J , Kamal-Eldin A . Phenolic Compounds in Rosaceae Fruits from Ecuador. J Agric Food Chem. (2009) ;57: (4):1204–12. |
[12] | Vasco C , Riihinen K , Ruales J , Kamal-Eldin A . Chemical composition and phenolic compound profile of mortino (Vaccinium floribundum Kunth). J Agric Food Chem. (2009) ;57: (18):8274–81. |
[13] | Giampieri F , Alvarez-Suarez JM , Mazzoni L , Forbes-Hernandez TY , Gasparrini M , Gonzalez-Paramas AM , Santos-Buelga C , Quiles JL , Bompadre S , Mezzetti B , Battino M. An anthocyanin-rich strawberry extract protects against oxidative stress damage and improves mitochondrial functionality in human dermal fibroblasts exposed to an oxidizing agent. Food Funct. (2014) ;5: (8):1939–48. |
[14] | Slinkard K , Singleton VL . Totalphenol analysis: Automation and comparison with manual methods. J Enology Vitic. (1977) ;28: (1):49–55. |
[15] | Dewanto V , Wu X , Adom KK , Liu RH . Thermal processing enhances the nutritional value of tomatoes by increasing total antioxidant activity. J Agric Food Chem. (2002) ;50: (10):3010–4. |
[16] | Giusti MM , Wrolstad RE . Characterization and measurement of anthocyanins by UV-visible spectroscopy. Current protocols in food analytical chemistry: John Wiley & Sons, Inc.; (2001) . |
[17] | ISO. Norma Internacional para la Determination de Taninos. (1988) . |
[18] | Benzie IFF , Strain JJ . The Ferric Reducing Ability of Plasma (FRAP) as a measure of “Antioxidant Power”: The FRAP assay. Anal Biochem. (1996) ;239: (1):70–6. |
[19] | Prymont-Przyminska A , Zwolinska A , Sarniak A , Wlodarczyk A , Krol M , Nowak M , de Graft-Johnson J , Padula G , Bialasiewicz P , Markowski J , Rutkowski KP , Nowak D. Consumption of strawberries on a daily basis increases the non-urate 2,2-diphenyl-1-picrylhydrazyl (DPPH) radical scavenging activity of fasting plasma in healthy subjects. J Clin Biochem Nutr. (2014) ;55: (1):48–55. |
[20] | Gomes A , Fernandes E , Silva AMS , Santos CMM , Pinto DCGA , Cavaleiro JAS , Lima JL . 2-Styrylchromones: Novel strong scavengers of reactive oxygen and nitrogen species. Bioorganic Med Chem. (2007) ;15: (18):6027–36. |
[21] | Ruch RJ , Cheng S-J , Klaunig JE. Prevention of cytotoxicity and inhibition of intercellular communication by antioxidant catechins isolated from Chinese green tea. Carcinogenesis. (1989) ;10: (6):1003–8. |
[22] | Maines MD , Costa LG , Reed DJ , Sassa S , Sipes IG . Current protocols in toxicology. New York: John Wiley & Sons; (1998) . |
[23] | Aebi H . Catalase in vitro. Methods in Enzymol. (1984) ;105: :121–6. |
[24] | Kakkar P , Das B , Viswanathan PN . A modified spectrophotometric assay of superoxide dismutase. Indian J Biochem Biophys. (1984) ;21: (2):130–2. |
[25] | Yu WJ , Chang CC , Kuo TF , Tsai TC , Chang SJ . Toona sinensis Roem leaf extracts improve antioxidant activity in the liver of rats under oxidative stress. Food Chem Toxicol. (2012) ;50: (6):1860–5. |
[26] | Ohkawa H , Ohishi N , Yagi K. Assay for lipid peroxides in animal tissues by thiobarbituric acid reaction. Analytical Biochem. (1979) ;95: (2):351–8. |
[27] | Jiang ZY , Hunt JV , Wolff SP . Ferrous ion oxidation in the presence of xylenol orange for detection of lipid hydroperoxide in low density lipoprotein. Analytical Biochem. (1992) ;202: (2):384–9. |
[28] | Levine RL , Garland D , Oliver CN , Amici A , Climent I , Lenz AG , Ahn BW , Shaltiel S , Stadtman ER . Determination of carbonyl content in oxidatively modified proteins. Methods Enzymol. (1990) ;186: :464–78. |
[29] | Araya M , Carvajal Y , Alvarez V , Orozco R , Rodriguez G. Polyphenol characterization of three varieties of Blackberry fruits (Rubus adenotrichos), cultivated in Costa Rica. J Berry Res. (2017) ;7: (2):97–107. |
[30] | Reyes-Carmona J , Yousef GG , Martínez-Peniche RA , Lila MA . Antioxidant capacity of fruit extracts of blackberry (Rubus sp.) produced in different climatic regions. J Food Sci. (2005) ;70: (7):S497–S503. |
[31] | Moyer RA , Hummer KE , Finn CE , Frei B , Wrolstad RE . Anthocyanins, phenolics, and antioxidant capacity in diverse small fruits: Vaccinium, Rubus, and Ribes. J Agric Food Chem. (2002) ;50: (3):519–25. |
[32] | Liu Y , Liu X , Zhong F , Tian R , Zhang K , Zhang X , Li T. Comparative study of phenolic compounds and antioxidant activity in different species of cherries. J Food Sci. (2011) ;76: (4):C633–C638. |
[33] | Del Rio D , Rodriguez-Mateos A , Spencer JPE , Tognolini M , Borges G , Crozier A. Dietary (poly)phenolics in human health: Structures, bioavailability, and evidence of protective effects against chronic diseases. Antioxid Redox Signal. (2013) ;18: (14):1818–92. |
[34] | Koca I , Karadeniz B. Antioxidant properties of blackberry and blueberry fruits grown in the black sea region of Turkey. Sci Hortic. (2009) ;121: (4):447–50. |
[35] | Giampieri F , Alvarez-Suarez JM , Mazzoni L , Forbes-Hernandez TY , Gasparrini M , Gonzàlez-Paramàs AM , Santos-Buelga C , Quiles JL , Bompadre S , Mezzetti B , Battino M. Polyphenol-rich strawberry extract protects human dermal fibroblasts against hydrogen peroxide oxidative damage and improves mitochondrial functionality. Molecules. (2014) ;19: (6):7798–816. |
[36] | Alvarez-Suarez JM , Carrillo-Perdomo E , Aller A , Giampieri F , Gasparrini M , González-Pérez L , Beltrán-Ayala P , Battino M. Antiinflammatory effect of Capuli cherry against LPS-induced cytotoxic damage in RAW 264.7 macrophages. Food Chem Toxicol. (2017) ;102: :46–52. |
[37] | Drózdz P , Iene V , Pyrzynska K. Phytochemical properties and antioxidant activities of extracts from wild blueberries and lingonberries. Plant Foods Hum Nutr. (2017) ;72: (4):360–4. |
[38] | Quideau S . Chemistry and biology of ellagitannins: An underestimated class of bioactive plant polyphenols. World Scientific; (2009) ;374: :298–319. |
[39] | Smirnoff N . The function and metabolism of ascorbic acid in plants. Ann Bot. (1996) ;78: (6):661–9. |
[40] | Hofstede R , Calles J , López V , Polanco R , Toores F , Ulloa J , . Los Paramos Andinos ¿Qué sabemos? Estado de conocimiento sobre el impacto del cambio climático en el ecosistema páramo. Quito, Ecuador: UICN, Quito, Ecuador; (2014) ;154. |
[41] | Khayyat M , Barati Z , Aminifard MH , Samadzadeh A. Changes in fruit maturity indices and growth pattern along the harvest season in seedless barberry under different altitude conditions. J Berry Res. (2018) ;8: (1):25–40. |
[42] | Marinova D , Ribarova F. HPLC determination of carotenoids in Bulgarian berries. J Food Comp Anal. (2007) ;20: (5):370–4. |
[43] | Khoo HE , Ismail A , Mohd-Esa N , Idris S. Carotenoid content of underutilized tropical fruits. Plant Foods Hum Nutr. (2008) ;63: (4):170–5. |
[44] | Vasco C , Ruales J , Kamal-Eldin A . Total phenolic compounds and antioxidant capacities of major fruits from Ecuador. Food Chem. (2008) ;111: (4):816–23. |
[45] | Carvalho MJ , Gouveia CS , Vieira AC , Pereira AC , Carvalho MA , Marques JC . Nutritional and phytochemical composition of Vaccinium padifolium Sm wild berries and radical scavenging activity. J Food Sci. (2017) ;82: (11):2554–61. |
[46] | Giampieri F , Alvarez-Suarez JM , Cordero MD , Gasparrini M , Forbes-Hernandez TY , Afrin S , Santos-Buelga C , Gonzalez-Paramas AM , Astolfi P , Rubini C , Zizzi A , Tulipani S , Quiles JL , Mezzetti B , Battino M. Strawberry consumption improves aging-associated impairments, mitochondrial biogenesis and functionality through the AMP-activated protein kinase signaling cascade. Food Chem. (2017) ;234: :464–71. |
[47] | Na H-K , Surh Y-J . Modulation of Nrf2-mediated antioxidant and detoxifying enzyme induction by the green tea polyphenol EGCG. Food Chem Toxicol. (2008) ;46: (4):1271–8. |
[48] | Frank S , Kämpfer H , Wetzler C , Pfeilschifter J. Nitric oxide drives skin repair: Novel functions of an established mediator. Kidney Int. (2002) ;61: (3):882–8. |
[49] | Esposito D , Munafo JP Jr , Lucibello T , Baldeon M , Komarnytsky S , Gianfagna TJ . Steroidal glycosides from the bulbs of Easter lily (Lilium longiflorum Thunb.) promote dermal fibroblast migration in vitro. J Ethnopharmacol. (2013) ;148: (2):433–40. |
[50] | Alvarez-Suarez JM , Giampieri F , Cordero M , Gasparrini M , Forbes-Hernández TY , Mazzoni L , Afrin S , Beltrán-Ayala P , Gonzalez-Paramás AM , Santos-Buelga C , Varela-Lopez A , Quiles JL , Battino M. Activation of AMPK/Nrf2 signalling by Manukahoney protects human dermal fibroblasts against oxidative damage by improving antioxidant response and mitochondrial function promoting wound healing. J Funct Foods. (2016) ;25: :38–49. |