The leaf extract of Ribes nigrum L. is a potent stimulator of the endothelial formation of NO in cultured endothelial cells and porcine coronary artery rings
Abstract
BACKGROUND: Endothelial dysfunction is a major hallmark of most types of cardiovascular diseases. Numerous plant extracts have been shown to cause endothelium-dependent relaxations by increasing the endothelial formation of the potent vasoprotective factor, nitric oxide (NO).
OBJECTIVE: The ability of different Ribes nigrum L. extracts (Grossulariaceae) to induce endothelium-dependent relaxation by stimulating the endothelial formation of NO was assesssed.
METHODS: Ribes nigrum extracts were prepared from buds, berries and leaves by extraction (Acetone:H2O:Acetic Acid; 70/28/2 (v/v/v)) and lyophilized after acetone evaporation. The ability of the extracts to stimulate the endothelial formation of NO was assessed using cultured endothelial cells and isolated porcine coronary artery rings.
RESULTS: The Ribes nigrum leaf extract increased to a greater extent than the bud and the berry extracts the formation of NO, and up-regulated eNOS mRNA expression in cultured endothelial cells (the stimulatory effects amounted to 197±9%, 134±6% and 118±5%, respectively). The leaf extract induced greater relaxations of isolated coronary arteries with endothelium than the bud and the berry extracts whereas no such effects were observed in rings without endothelium. Relaxations to the leaf extract were minimally affected by indomethacin and by inhibitors of endothelium-dependent hyperpolarization response, and markedly reduced by NG-nitro-L-arginine.
CONCLUSIONS: The present findings indicate that the Ribes nigrum leaf extract is a more potent inducer of the endothelial formation of NO than the bud and the berry extracts.
1Introduction
Cardiovascular diseases (CVD) are one of the leading causes of mortality in Western countries. The major CVD risk factors include gender, smoking habit, hypertension, dyslipidaemia, insulin resistance, diabetes and hypertension. Over the last decades, a large number of pre-clinical and clinical studies have highlighted the key role of oxidative stress in the development of CVD and atherosclerosis [1]. Numerous studies have indicated a clear association between increased oxidative stress and endothelial dysfunction, which promote both functional and morphological vascular abnormalities [2]. Under physiological conditions, endothelial cells produce both vasorelaxant and vasoconstrictor factors, the equilibrium of which contributes to the control of vascular homeostasis. Nitric oxide (NO) produced by endothelial NO synthase (eNOS) plays a key role to inhibit vascular tone following its diffusion from endothelial cells to the underlying smooth muscle where it activates soluble guanylyl cyclase leading to the formation of cyclic 3’,5’-guanosine monophosphate (cGMP). However, in case of endothelial dysfunction, eNOS is often uncoupled leading to the generation of ROS (mainly superoxide anions) instead of NO [3]. In addition through a direct chemical interaction with superoxide anions, the bioavailability of NO can be significantly reduced leading to increased vascular tone and, hence, promoting the development of hypertension. Moreover, the pro-oxidant peroxynitrite resulting from the chemical interaction between NO and superoxide anions further promotes the imbalance between protective and deleterious factors. Clinical studies have shown an impaired endothelial function as measured by flow-mediated dilatation (FMD) in patients with established coronary artery disease or with coronary risk factors (diabetes, smoking) in both the coronary circulation and the peripheral circulation [4].
Of interest, pharmacological interventions with antioxidant agents such as dietary polyphenols, especially flavanols, flavones, flavan-3-ols, anthocyanins and combination of them [5, 6], have been shown to improve and/or restore the endothelial function and therefore, to reduce CVD risks. The mechanisms through which fruit and vegetable polyphenols may reduce CVD risks are not completely clear and they seem to be multiple [7]. Indeed, in humans, regular intake of food enriched in polyphenols such as black chocolate [8], green tea [9] and red wine in moderate amounts [10] has been shown to improve endothelial function with a concomitant reduction of arterial blood pressure. In vitro studies have also revealed that polyphenols contained in such foods are able to stimulate the expression and the activation of eNOS leading to increased generation of NO [11]. The dietary polyphenols seem also to have an effect on lipid profile of plasma by reducing low density lipoprotein oxidation (LDLs) [7].
Among berries, blackcurrant (Ribes nigrum L., Grossulariaceae), a dark-pigmented fruit native to central and northern Europe and northern Asia, exhibits one of the highest amount of phenolic compounds, especially anthocyanins. Other major phenolics present in blackcurrant include flavonols, procyanidins and several phenolic acids [12]. Only a very small portion of the berries is consumed fresh whereas the large remaining portion is processed for industrial use like in fruit juices and liquors. Blackcurrant has received much attention in recent years due to its superior antioxidant activity compared with many other kinds of fruits like blueberry and cranberry [12]. Besides antioxidant and anti-inflammatory properties [13, 14], Edirisinghe and colleagues [15] have shown that a blackcurrant juice concentrate is able to activate eNOS via the PI-3-kinase/Akt pathway in endothelial cells. Moreover, several studies have shown that blackcurrant juices are able to cause endothelium-dependent relaxations in rat aorta rings by increasing NO formation, in part, via the activation of histamine H1-receptors in endothelial cells [16–18]. The ability of blackcurrant products to activate the endothelial NO formation could be linked to their high level of anthocyanidins [19]. In humans, Zhu et al. [20] have shown that intake of 320 mg of anthocyanins purified from either bilberry (Vaccinium myrtillus) or blackcurrant (Ribes nigrum) improved endothelium-dependent vasodilatation in hypercholesterolemic individuals both after a short-term period (2 h after ingestion) and after a long-term period (intake every day during 12 weeks). In contrast, a consumption of a 20% blackcurrant juice drink (250 mL) did not significantly affect vascular function in the postprandial phase [21].
Except the work of Zhu et al. [20], most studies have evaluated the biological effect of blackcurrant juices. The possibility that blackcurrant extracts from berries and also those from leaves or buds are able to increase the endothelial formation of NO has not been investigated. However, blackcurrant leaves have been used as a traditional medicine notably for the treatment of rheumatic diseases in Europe [13], and blackcurrant buds and leaves for the production of essential oils. Moreover, our previous study [14, 22] has indicated that blackcurrant leaves have higher levels of antioxidants compared with buds and berries. In these previous works, the content of flavonoids of blackcurrant extracts were determined by HPLC-DAD analyses [22] and the antioxidant capacities were analyzed by various in vitro assays (i.e. for ORAC measurement, capacity was greatly higher in leaf extract than in bud (70%) and berry (16%) extracts [14].
Therefore, the aim of the present study was to evaluate in vitro the ability of blackcurrant (Ribes nigrum) extracts of leaves, buds and berries to stimulate the endothelial formation of the vasoprotective factor NO in both cultured cells and isolated coronary arteries.
2Materials and methods
2.1Reagents
β-Nicotinamide adenine dinucleotide phosphate (NADPH), N-(1-Naphthyl) ethylenediamine dihydrochloride (NED), sodium pyruvate, sodium nitrite, sulfanilamide, HAT medium supplement, 9,11-Dideoxy-11α,9α-epoxymethanoprostaglandin F2α (U46619), NG-Nitro-L-arginine (L-NAME), apamin, indomethacin, charybdotoxin, and in vitro toxicology assay kit were purchased from Sigma-Aldrich, nitrate reductase from Roche, Switzerland, RNeasy mini kit from Qiagen, Netherlands, GoTaq DNA polymerase, M-MLV Reverse Transcriptase (RNase H(-), Point Mutant enzyme) from Promega, USA. Dulbecco’s Modified Eagle’s Medium (DMEM), penicilin, streptomycin, fœtal bovine serum (FBS), 4-(2-hydroxyethyl)-1-piperazineethanesulfonic acid (HEPES), L-glutamine were obtained from Lonza, Switzerland, primers for β-actin and eNOS were synthetized and purchased from Invitrogen, and aceton, ethanol, orthophosphoric acid, acetic acid, D-glucose and salts from VWR.
2.2Sample preparation
Buds, leaves and berries of blackcurrant (Ribes nigrum ‘Noir de Bourgogne’, Grossulariaceae), two years old, were obtained from a bio-reserve in Bihain, Belgian Ardennes from the BIOGEM sprl, and were harvested respectively in March, June and July of 2009. The various explants were cut into pieces, frozen in liquid nitrogen, lyophilized and preserved at –20°C.
For preparing the extracts, 50 g of a frozen sample (berries, buds or leaves) were grinded with a few g of quartz and 500 mL of extraction solution containing acetone/water/acetic acid (70:28:2) [14]. The mixture was shaken for 1 h at 4°C and then centrifuged at 17,000 g for 15 min. The supernatant was collected, and the sample was again extracted with the same procedure as above but with a 15 min shaking period. After pooling of supernatants, acetone was evaporated and the residue was filtered and lyophilized before storage at –20°C. The extracts were so prepared and harvested in accordance to EMEA guidelines. The residue was diluted in culture medium for cell culture experiments, and in ethanol (50%) for vascular reactivity studies.
2.3Cell culture
The human endothelial-like EAhy926 cell line [23] was grown in DMEM (Biowithaker, Lonza) supplemented with 10% heat-inactivated FBS, 10μM HEPES solution, 5,000 units/L of penicillin and streptomycin, 1 mM sodium pyruvate, 2 mM L-glutamine and 2% HAT medium supplement. Cells were maintained at 37°C with 5% CO2 and treated when they had reached 75 percent of confluence.
2.12.4. 3-[4,5-dimethylthiazol-2-yl]-2,5-diphenyltetrazolium bromide viability assay
Toxicity of blackcurrant leaf extracts was tested using the cell growth determination kit MTT. The EAhy926 cell line was seeded in a 96-well plate at 5,000 cells/well and each treatment was done in replicate with increasing concentrations of the blackcurrant leaf extract (0 to 1 mg/mL) solubilized in complete DMEM or with complete DMEM as control. The cell density was assessed after 24 and 48 h incubation periods. Cell viability was evaluated as recommended by the manufacturer. The absorbance was measured using a microliter plate reader (Labsystems IEMS reader MF) at 570 nm. The background absorbance at 690 nm was subtracted.
2.5Determination of total nitrite content
Total nitrite accumulation in supernatant of EAhy926 cell culture was measured using the Griess method, adapted for 96-well plates [24]. Five hundred thousand cells were incubated in 6-well plates and exposed to a blackcurrant extract at different concentrations (0 to 1 mg/mL) for 24 h. In some experiments, cells were exposed to the leaf extract (0 to 1 mg/mL) for 5 min, 6 h, 12 h, 24 h and 48 h.
At the end of incubation, 100μL of the culture supernatant was mixed with 25μL of NADPH (93μg/mL final concentration) and 10μL of nitrate reductase (0.2 U) in a 96-well plate. After incubation at 25°C for 1.5 h, 100μL of Griess reagent (1 part 1% sulfanilamide and 1 part 0.1% NED in 5% ortho-phosphoric acid) was added. After 10 min incubation period, the absorbance at 540 nm was measured using a microliter plate reader (Thermo Scientific IEMS MF). Various concentrations of sodium nitrate (0 to 500μM) were used to obtain a calibration curve and all the results were expressed as μM of NaNO3.
2.6Semi-quantitative RT-PCR for eNOS mRNA analyses
The expression of eNOS mRNA was determined by semi-quantitative RT-PCR. EAhy926 cells were exposed to a blackcurrant extract at various concentrations (0 to 1 mg/mL) during 24 h or with the leaf extract at 250μg/mL for different times (5 min, 6 h, 12 h, 24 h and 48 h). After incubation, total RNA was isolated with the RNeasy mini kit as recommended by the supplier. RNA (1μg) was transcribed into cDNA by reverse transcription by M-MLV Reverse Transcriptase. For the PCR, 2.5μL of cDNA product of each sample was amplified on Gene Amp® PCR System 9700 (PE Applied BioSystems) with GoTaq polymerase using a primer pair specific to eNOS (forward primer: 5’-GCT GCG CCA GGC TCT CAC CTT C-3’, reverse primer: 5’-GGC TGC AGC CCT TTG CTC TCA A-3’, 35 cycles, 554 bp, annealing temperature: 55°C) and two primer pairs specific to β-actin ([forward primer: 5’-GGC ATC GTG ATG GAC TCC G-3’, reverse primer: 5’-GCT GGA AGG TGG ACA GCG A-3’, 23 cycles, 603 bp, annealing temperature: 68 °C] [forward primer: 5’- ATG GAT GAT GAT ATC GCC GCG -3’, reverse primer: 5’ - TCT CCA TGT CGT CCC AGT TG - 3’, 30 cycles, 248 bp, annealing temperature: 58°C]). The PCR amplified samples were visualized by electrophoresis using a 1.5% agarose gel in the presence of ethidium bromide. The quantification was carried out using the G: Box Imaging System (Syngene, Wesburg) and the GeneSnap and GeneTools softwares.
2.7Vascular reactivity study
Vascular reactivity studies were done using isolated porcine coronary arteries as described previously [25]. Pig hearts were collected from the local slaughterhouse (Holtzheim, France). The coronary artery was dissected and carefully cleaned of connective tissues before being cut into rings (3-4 mm length). Rings were suspended in organ baths containing oxygenated (95% O2 and 5% CO2) Krebs bicarbonate solution (mM: NaCl 119, KCl 4.7, KH2PO4 1.18, MgSO4 1.18, CaCl2 1.25, NaHCO3 25, and D-glucose 11, pH 7.4, 37°C) under a resting tension of 5g for the determination of changes in isometric tension. In some rings, the endothelium was removed mechanically by gently rubbing the lumen of the ring with forceps. Coronary artery rings were contracted with U46619, a stable thromboxane A2 agonist, before a concentration-relaxation curve to a blackcurrant extract was constructed by adding increasing concentrations of the extract in the organ bath. In some experiments, rings were exposed to various combinations of inhibitors for 30 min prior to the addition of U46619 (inhibitors tested: Indomethacin, apamin, L-NAME, charybdotoxin). Relaxations were expressed as relative responses, and 0% corresponds to the pre-contraction level to U46619 and 100% to be full relaxation of the U46619-induced contraction.
2.8Statistical analysis
Results are shown as mean±SD of n different experiments. The data were statistically analysed using an analysis of variance (ANOVA-1) to evaluate significant differences between groups. The difference was regarded as significant when P < 0.05.
3Results
3.1Blackcurrant leaf extract stimulates the endothelial NO formation
A first series of experiments has evaluated the ability of blackcurrant extracts from either leaves, buds or berries to stimulate the formation of NO as assessed using the Griess method in cultured endothelial cells. Addition of a blackcurrant leaf extract to endothelial cells increased the formation of NO in a time and concentration-dependent manner up to 12 h (Table 1). Significantly increased levels of NO were observed at concentrations of or greater than 300μg/mL of the leaf extract after a 24 h incubation period (Table 1). In contrast to the blackcurrant leaf extract, no or only small effects were observed in response to the berry extract or the bud extract (data not shown).
The stimulatory effect of the leaf extract was observed as early as 5 min and persisted up to 48 h (Table 1).
3.2Blackcurrant extract stimulates eNOS mRNA expression
Semi quantitative RT-PCR was used to determine whether the eNOS gene expression is affected in endothelial cells after a 24 h incubation period with increasing concentrations of the blackcurrant leaf extract. The eNOS mRNA level was significantly increased in response of the leaf extract at 250μg/mL (197±9%, Fig. 1), and also, to some extent, with the berry extract and the bud extract (118±5% and 134±6%, respectively).
The eNOS mRNA level was also monitored over 48 h in cells incubated with 250μg/mL of the leaf extract (Fig. 2). The increased eNOS mRNA levels by the leaf extract was observed as early as 6 h, and this effect increased until 24 h and thereafter persisted up to 48 h (Fig. 2).
3.3Effect of blackcurrant leaf extract on endothelial cell viability
Experiments have also been performed to determine whether the blackcurrant leaf extract affects endothelial cells viability using the MTT assay. Exposure of endothelial cells to the leaf extract at concentrations up to 500μg/mL did not significantly affect cell viability up to a 48 h incubation period (Fig. 3). However at a concentration of 1000μg/mL, a significant reduction of cell viability was observed at 24 h and 48 h (Fig. 3).
3.4Blackcurrant extracts cause endothelium-dependent relaxations in coronary artery rings
Experiments were performed to determine the ability of blackcurrant extracts of either leaves, buds or berries to cause relaxations in porcine coronary artery rings with or without endothelium (Fig. 4). All three extracts induced concentration-dependent relaxations in rings with endothelium but only minor ones in those without endothelium (Fig. 4). In addition, the leave extract induced 100% relaxation at 0.03 mg/mL, and the bud and berry extracts cause about 95 and 87% relaxation, respectively, at 0.1 mg/mL.
The endothelium-dependent relaxation to the most active extract, the leaf extract, was further characterized using pharmacological inhibitors. Indomethacin (10μM, an inhibitor of cyclooxygenase) slightly shifted to the right the concentration-relaxation curve to the blackcurrant leaf extract (Fig. 5). In addition, the blackcurrant leaf extract-induced relaxation was further markedly inhibited by the addition of NG-nitro-L-arginine (a competitive inhibitor of NO synthase); the relaxation amounted to about 80% at 0.03 mg/mL (Fig. 5).
In contrast, addition of the combination of apamin+charybdotoxin (two inhibitors of endothelium-dependent hyperpolarization-mediated relaxation) did not significantly affect the blackcurrant leaf extract-induced relaxation in the presence of indomethacin (Fig. 6). The further addition of NG-nitro-L-arginine markedly reduced the leaf extract-induced relaxation to levels similar of those observed in rings without endothelium (Fig. 6). Altogether, these observations indicate that the leaf extract is a more potent inducer of endothelium-dependent relaxations than the bud extract and the berry extract, and that these relaxations involve predominantly NO and also, to some extent vasorelaxant prostanoids.
4Discussion
Several sources of polyphenols including red wines [26], grape juices, berry juices [27] and fruits such as acaí [28] have a potential to improve vascular health by various mechanisms including the induction of vasodilatation, scavenging of free radicals, reduction of vasoconstrictor and pro-inflammatory processes at adequate concentrations. However at higher concentrations, they may also induce opposite effects such as the induction of pro-constrictor and pro-oxidant effects [29].
The present study has investigated the effect of three different blackcurrant-derived extracts (leaf, bud and berry) on the endothelial formation of NO, a major vasoprotective factor, using two experimental vascular approaches: Cultured endothelial cells and isolated porcine coronary arteries. Previous investigations [22] have indicated that these blackcurrant extracts have high levels of antioxidants including anthocyanins and flavonols. Moreover, these extracts have been characterized to contain high levels of flavonoids such as flavonols, anthocyanins and flavanols, and that the leaf extract and the bud extract had a higher total content of polyphenols than the berry extract (Fig. 4).
Numerous in vitro studies have indicated that, at low concentrations (<10 mg/L), several polyphenols can induce endothelium-dependent relaxations whereas at high concentrations (> 30 mg/L) they can induce a direct relaxation of the vascular smooth muscle [30, 31].
The present study provides evidence that the blackcurrant leaf extract is able to up-regulate eNOS mRNA expression associated with an increased formation of NO in human endothelial cells (Fig. 1). Similar findings were also observed previously with other food products such as wines [32] and a strawberry aqueous extract [33]. The increased NO formation was observed 6 h after the incubation of endothelial cells with the blackcurrant leaf extract with a maximal response at 12 h. Xu and Huang [31] have observed a similar effect in response to icariin, a derivative of kaempferol 3,7-O-diglucoside. Thus, the present findings indicate that the blackcurrant leaf extract is able to cause an up-regulation of eNOS promoting a sustained and increased NO formation.
Such an increased endothelial formation of NO is well known to promote vascular protection and to have anti-atherosclerotic properties. The NO-mediated relaxation of blood vessels can be the consequence of an increased eNOS expression, eNOS activity and/or from activation of guanylyl cyclase leading to the accumulation of cyclic GMP [34]. This eNOS activation by blackcurrant leaf acetone extract was also shown by the team of Luzak [35]. This activation was combined with an increase of a particular ecto-ADPase, the CD39, and a modulation of the endothelial cell viability (HUVECs) in a time-and concentration-dependent manner.
Therefore, we have hypothesized that the formation of NO induced by blackcurrant extracts may be associated with its protective effect on the vascular system. For this purpose, we have investigated the effect of blackcurrant extracts on vascular reactivity using porcine coronary artery rings with either an intact endothelium or without endothelium (Fig. 4). The blackcurrant leaf extract caused full relaxation of precontracted coronary artery rings at a concentration of about 0.03 mg/mL, whereas the bud and berry extracts induced only about 95 and 87% relaxation at 0.1 mg/mL, respectively. In the case of a strawberry extract [36], a 70% relaxation was observed at a concentration of 1 mg/mL in aortic rings of New Zealand rabbit. Our findings on the effect of various extracts on vasorelaxtion (taken at 0.1 mg/mL) is largely correlated with antioxidant activity (1.0 for ORAC and DPPH assays and 0.981 for TEAC assay), as well as their contents in total polyphenols and flavonols (>0.8 for total phenolics and flavonols) (Fig. 4).
A major finding of the present study indicates that the leaf extract was more potent inducer of endothelium-dependent relaxations than the bud extract and the berry extract. Consistent with the relaxation studies, the leaf extract induced the strongest NO formation and the expression of eNOS in endothelial cells. In a previous report [22], we have characterized the three blackcurrant extracts (leaf, bud and berry). After separation and HPLC analysis, quercetin and kampferol were most abundant in the leaf extract (the quercetin level was 1.6 and 10 times higher and that of kampferol 3.7 and 32 times in the leaf extract than in the bud and berry extracts, respectively). The group of Benito [37] has shown that quercetin increased eNOS activity and induced endothelium-dependent relaxations in aorta rings of hypertensive rats (Sprague-Dawley). Similar effects on eNOS activity were observed by Xu and Huang [31] with quercetin at 50μM. In contrast, Wallerath et al. [38] reported that quercetin (1 to 33μM) reduced eNOS mRNA expression in a concentration-dependent manner in EAhy926 cells after a 24 h incubation period. They suggested that the underlying mechanism involved an increased mRNA destabilization since the eNOS promoter activity was not affected by quercetin. Luzak et al. [35] showed no variation of an eNOS gene expression with their blackcurrant leaf extract but an increase of eNOS activation on HUVEC cells at 15μg EAG/mL. In addition, Xu et al. [39] have investigated the effect of 17 different flavonoids on porcine coronary artery rings with or without endothelium and they observed that kampferol was a relatively potent relaxing molecule whereas quercetin was a moderate one. Moreover, they observed that most flavonoids induced relaxations mainly through a direct action at the vascular smooth muscle. In addition, several anthocyanins such as delphinidin, an anthocyanin present in the leaf extract, might also contribute to the endothelium-dependent relaxation to the leaf extract. Stoclet et al. [40] have observed an endothelium-dependent relaxation to delphinidin with an EC50 of about 30μM.
The mechanism by which the leaf extract induced endothelium-dependent relaxation was investigated using several inhibitors (NG-nitro-L-arginine and/or the combination of apamin+charybdotoxin). The data indicate that the leaf extract induced a pronounced endothelium-dependent relaxation involving NO and also to some extent vasorelaxant prostanoids. These observations are in agreement with previous ones showing that several polyphenol-rich sources (i.e; lingonberries [27] and red wine [26]) induced redox-sensitive endothelium-dependent relaxations mostly involving the formation of NO- and, often also, to some extent, EDH.
Despite the apparently beneficial health effects of flavonoids, several studies have also indicated an increased mutagenicity and genotoxicity in several bacterial and mammalian experiments. This effect may be due to their pro-oxidant activity. In the present study, the blackcurrant leaf extract did not induce cytotoxic response in endothelial cells except at very high concentrations (more than 500μg of extract/mL, Fig. 6).
Many challenges still lie ahead. Although some groups showed dose response effects in cell culture [35], with stronger effects at nutritionally relevant concentrations, at present, it is not possible to conduct in vivo dose-response studies with polyphenols due to the ignorance of their absorption, distribution, metabolism and excretion. Actually, biomarker used to show the efficiency of polyphenols is not adequate because they are influenced by polyphenols. The outcomes are more important than those due to antioxidant activity only [41]. Another issue to consider is the complexity of food compounds and the fact that they are ingested over long period, widely different behavior of drugs [42].
In conclusion, the present investigations suggest that the blackcurrant leaf extract is a strong in vitro inducer of endothelium-dependent relaxations. The vasorelaxant effect is caused predominantly by the stimulation of the endothelial formation of NO and possibly also to some extent vasolorelaxant prostanoids.
Acknowledgments
J.T. gratefully acknowledges the FNR Luxembourg, the memberships of CEDEVIT A.S.B.L (Liège) and the memberships of CREDEC, especially Mme Ooms for her help on cell culture.
References
[1] | Schnabel R , Blankerberg S . Oxidative stress in cardiovascular disease successful translation from bench to bedside? Circulation. (2007) ;116: :1338. |
[2] | Su Y , Liu XM , Sun YM , Jin HB , Fu R , Wang YY , et al. The relationship between endothelial dysfunction and oxidative stress in diabetes and prediabetes. Int J Clin Pract. (2008) ;62: :877. |
[3] | Landmesser U , Dikalov S , Price SR , McCann L , Fukai T , Holland SM , et al. Oxidation of tetrahydrobiopterin leads to uncoupling of endothelial cell nitric oxide synthase in hypertension. J Clin Invest. (2003) ;111: :1201. |
[4] | Celermajer DS , Sorensen KE , Bull C , Robinson J , Deanfield JE . Endothelium-dependent dilation in the systemic arteries of asymptomatic subjects relates to coronary risk factors and their interaction. J Am Coll Cardiol. (1994) ;24: :1468. |
[5] | McCullough ML , Peterson JJ , Patel R , Jacques PF , Shah R , Dwyer JT . Flavonoid intake and cardiovascular disease mortality in a prospective cohort of US adults. Am J Clin Nutr. (2012) ;95: :454. |
[6] | Cassidy A , Mukamal KJ , Liu L , Franz M , Eliassen AH , Rimm EB . High anthocyanin intake is associated with a reduced risk of myocardial infraction in young and middle-aged women. Circulation. (2013) ;127: :188. |
[7] | Giamperi F , Forbes-Hernandez T , Gasparrini M , Alvarez-Suarez JM , Afrin S , Bompadre S , et al. Strawberry as a health promoter: An evidence based review. Food Funct. (2015) ;6: :1386. |
[8] | Hooper L , Kay C , Abdelhamid A , Kroon PA , Cohn JS , Rimm EB , et al. Effects of chocolate, cocoa, and flavan-3-ols on cardiovascular health: A systematic review and meta-analysis of randomized trials. Am J Clin Nutr. (2012) ;95: :740. |
[9] | Ras RT , Zock PL , Draijer R . Tea consumption enhances endothelial-dependent vasodilation; a meta-analysis. PLoS ONE. (2011) ;6: (3):e16974. |
[10] | Karatzi K , Papamichael C , Karatzis E , Papaioannou TG , Voidonilola PT , Vamvakou GD , et al. Postprandial improvement of endothelial function by red wine and olive oil antioxidants: A synergistic effect of components of the Mediterranean diet. J Am Coll Nutr. (2008) ;27: :448. |
[11] | Schini-Kerth VB , Auger C , Kim JH , Etienne-Selloum N , Chayaigneau T . Nutritional improvement of the endothelial control of vascular tone by polyphenols: Role of NO and EDHF. European J Physiol. (2010) ;459: :853. |
[12] | Borges G , Degeneve A , Mullen W , Crozier A . Identification of flavonoids and phenolic antioxidants in black currants, blueberries, raspberries, red currants, and cranberries. J Agric Food Chem. (2010) ;58: :3901. |
[13] | Garbacki N , Tits M , Angenot L , Damas J . Inhibitory effects of proanthocyanidins from Ribes nigrum leaves on carrageenin acute inflammatory reactions induced in rats. BMC Pharmacol. (2004) ;4: :25. |
[14] | Tabart J , Franck T , Kevers C , Pincemail J , Serteyn D , Defraigne JO , et al. Antioxidant and anti-inflammatory activities of Ribes nigrum extracts. Food Chem. (2012) ;131: :1116. |
[15] | Edirisinghe I , Banaszewski K , Cappozzo J , McCarthy D , Burton-Freeman BM . Effect of black currant anthocyanins on the activation of endothelial nitric oxide synthase (eNOS) in vitro in human endothelial cells. J Agric Food Chem. (2011) ;59: :8616. |
[16] | Auger C , Pollet B , Arnold C , Marx C , Schini-Kerth VB . Great heterogeneity of commercial fruit juices to induce endothelium-dependent relaxations in isolated porcine coronary arteries: Role of the phenolic content and composition. J Med Food. (2015) ;18: :128. |
[17] | Nakamura Y , Matsumoto H , Todoki K . Endothelium-dependent vasorelaxation induced by blackcurrant concentrate in rat thoracic aorta. Jpn J Pharmacol. (2002) ;89: :29. |
[18] | Yonei Y , Iwabayashi M , Fujioka N , Nomoto K , Miyazaki R , Hibino S , et al. Evaluation of effects of cassis (Ribes nigrum L.) juice on human vascular function and gene expression using a microarray system. Anti-aging Med. (2009) ;6: :22. |
[19] | Speciale A , Virgili F , Saija A , Cimino F . Anthocyanins in vascular diseases, In Watson RR, Preedy VR, Zibadi S, editors. Polyphenols in Human Health and Diseases. Academic Press. (2014) ;2: :923. |
[20] | Zhu Y , Xia M , Yang Y , Liu F , Li Z , Hao Y , et al. Purified anthocyanin supplementation improves endothelial function via NO-cGMP activation in hypercholesterolemic individuals. Clin Chem. (2011) ;57: :1524. |
[21] | Jin Y , Alimbetov D , George T , Gordon MH , Lovegrove JA . A randomized trial to investigate the effects of acute consumption of blackcurrant juice drink on markers of vascular reactivity and bioavailability of anthocyanins in human subjects. Eur J Clin Nutr. (2011) ;65: :849. |
[22] | Tabart J , Kevers C , Evers D , Dommes J . Ascorbic acid, Phenolic acid, Flavonoid and Carotenoid profiles of selected extracts from Ribes nigrum. J Agric Food Chem. (2011) ;59: :6165. |
[23] | Edgell CIS , McDonald CC , Graham JB . Permanent cell line expressing human factor VIII-related antigen established by hybridization. Proc Natl Acad Sci USA. (1983) ;80: :3734. |
[24] | Bani D , Failli P , Bello MG , Thiemermann C , Bani Sacchi T , Bigazzi M , et al. Relaxin activates the L-Arginine-nitric oxide pathway vascular smooth muscle cells in culture. Hypert. (1998) ;31: :1240. |
[25] | Anselm E , Chataigneau M , Ndiaye M , Chataigneau T , Schini-Kerth VB . Grape juice causes endothelium-dependent relaxation via a redox-sensitive Src- and Akt-dependent activation of eNOS. Cardiovasc Res. (2007) ;73: :404. |
[26] | Ndiaye M , Chataigneau T , Andriantsitohaina R , Stoclet JC , Schini-Kerth VB . Red wine polyphenols cause endothelium-dependent EDHF-mediated relaxations in porcine coronary arteries via redox-sensitive mechanism. Biochem Biophys Res Commun. (2003) ;310: :371. |
[27] | Kivimäki AS , Ehlers PI , Turpeinen AM , Vapaatalo H , Korpela R . Lingonberry juice improves endothelium-dependent vasodilatation of mesenteric arteries in spontaneously hypertensive rats in a long-term intervention. J Funct Foods. (2011) ;3: :267. |
[28] | Rocha APM , Carvalho LCRM , Sousa MAV , Madeira SVF , Sousa PJC , Tano T , et al. Endothelium-dependent vasodilator effect of Euterpe oleracea Mart, (Açaí) extracts in mesenteric vascular bed of the rat. Vasc Pharmacol. (2007) ;46: :97. |
[29] | Andriantsitohaina R , Auger C , Chataigneau T , Etienne-Selloum N , Li H , Martínez MC , et al. Molecular mechanisms of the cardiovascular protective effects of polyphenols. Br J Nutr. (2012) ;108: :1532. |
[30] | Andriambeloson E , Magnier C , Haan-Archipoff G , Lobstein A , Anton R , Beretz A , et al. Natural dieatry polyphenolic compounds cause endothelium-dependant vasorelaxation in rat thoracic aorta. J Nutr. (1998) ;128: :2324. |
[31] | Xu HB , Huang ZQ . Icariin enhances endothelial nitric-oxide synthase expression on human endothelial cells in vitro. Vasc Pharmacol. (2007) ;47: :18. |
[32] | Wallerath T , Poleo D , Li H , Föstermann U . Red wine increases the expression of human endothelial nitric oxide synthase. J Am Coll Cardiol. (2003) ;41: :471. |
[33] | Soares VC , Varanda EA , Raddi MS . In vitro basal and metabolism-mediated cytotoxicity of flavonoids. Food Chem Toxicol. (2006) ;44: :835. |
[34] | Moncada M , Palmer RM , Higgs EA . Nitric oxide: Physiology, pathophysiology and pharmacology. Pharmacol Rev. (1991) ;43: :109. |
[35] | Luzak B , Boncler M , Rywaniak J , Dudzinska D , Rosalski M , Krajewska U , et al. Estract from Ribes nigrum leaves in vitro activates nitric oxide synthase (eNOS) and increases CD39 expression in human endothelial cells. J Physiol Biochem. (2014) ;70: :1007. |
[36] | Edirisinghe I , Burton-freeman B , Varelis P , Kappagoda T . Strawberry extract caused endothelium-dependent relaxation through the activation of PI3 kinase/Akt. J Agric Food Chem. (2008) ;56: :9383. |
[37] | Benito S , Lopez D , Saiz MP , Buxaderas S , Sanchez J , Puig-Parellada P , et al. A flavonoid-rich diet increases nitric oxide production in rat aorta. Br J Pharmacol. (2002) ;135: :910. |
[38] | Wallerath T , Li H , Gödtel-Ambrust U , Schwarz PM , Föstermann U . A blend of polyphenolic compounds explains the stimulatory effect of red wine on human endothelial NO synthase. Nitric Oxide. (2005) ;12: :97. |
[39] | Xu YC , Leung SWS , Yeung DKY , Hu LH , Chen GH , Che CM , et al. Structure-activity relationships of flavonoids for vascular relaxation in porcine coronary artery. Phytochem. (2007) ;68: :1179. |
[40] | Stoclet JC , Andriambeloson E , Andriantsitohaina R . Endothelial NO release caused by red wine polyphenols with specific structures. Exp Clin Cardiol. (2000) ;5: :24. |
[41] | Calder PC , Albers R , Antoine JM , Blum S , Bourdet-Sicard R , Ferns GA , et al. Inflammatory disease processes and interactions with nutrition. Br J Nutr. (2009) ;101: :S1. |
[42] | Chiva-Blanch G , Visioli F . Polyphenols and health: Moving beyond antioxidants. J Berry Res. (2012) ;2: :63. |
Figures and Tables
Fig.1
Effect of blackcurrant leaf extracts on eNOS mRNA expression in human endothelial EAHy926 cells (5×106 cells) after a 24 h of incubation period with various concentrations of the extracts (0 to 1 mg/mL). The results are expressed in % of expression and were normalized to β-actin mRNA expression taken as 100%. Data are shown as means±SD (n = 3). Significant differences at p < 0.05 are indicated by different numbers of *. Representative agarose gel showing the effect of 0, 250, 500 and 1000μg/mL of leaf extract on the expression level of eNOS and β-actin.
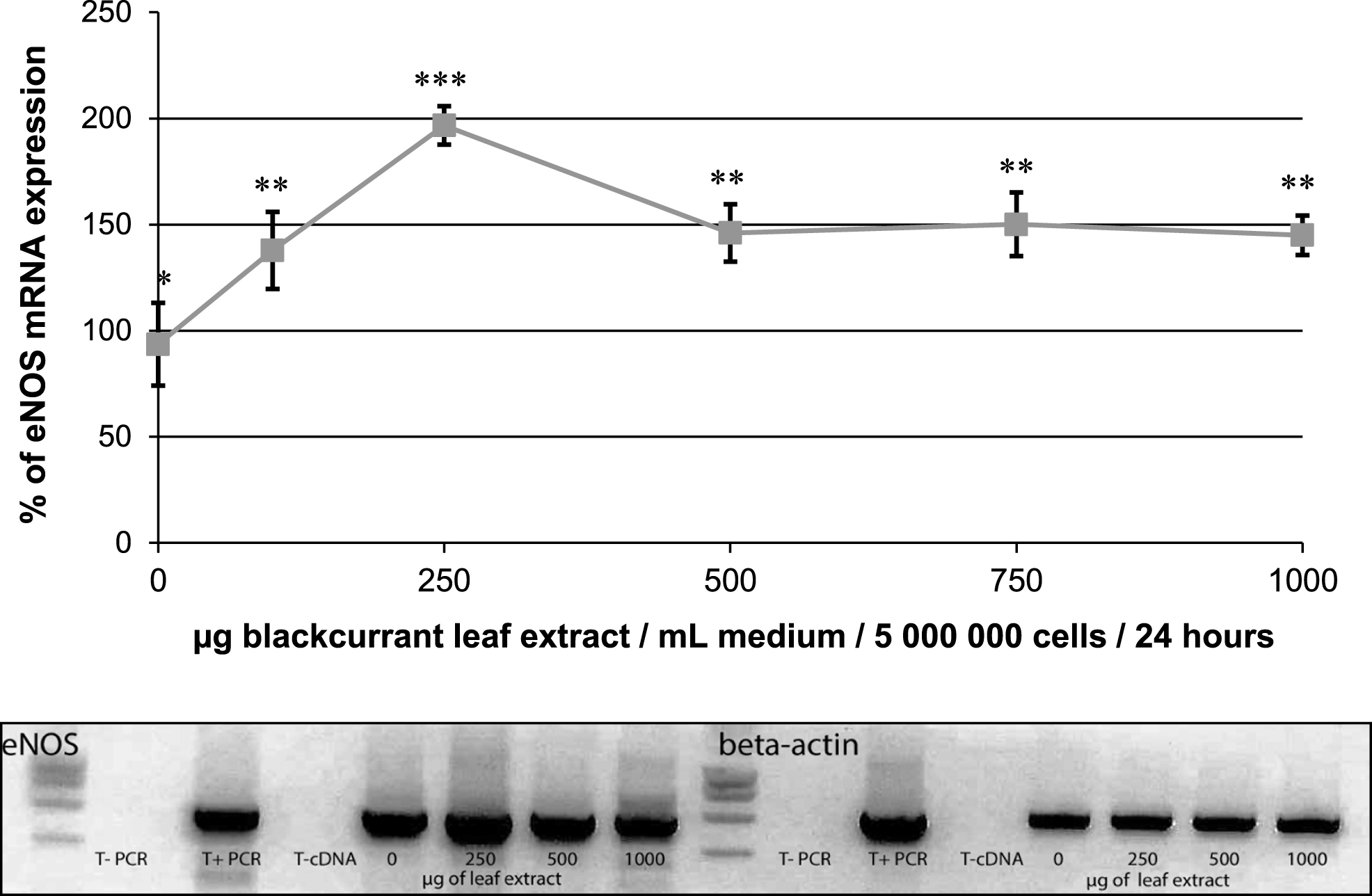
Fig.2
Time course of eNOS mRNA expression after incubation of 5×106 cells with 250μg/mL of leaf extract in the medium up to 48 h. The results are expressed in % of expression and are compared with β-actin mRNA expression taken as 100%. Data are shown as means±SD (n = 3). Significant differences at p < 0.05 (n = 3) are indicated by different numbers of *.
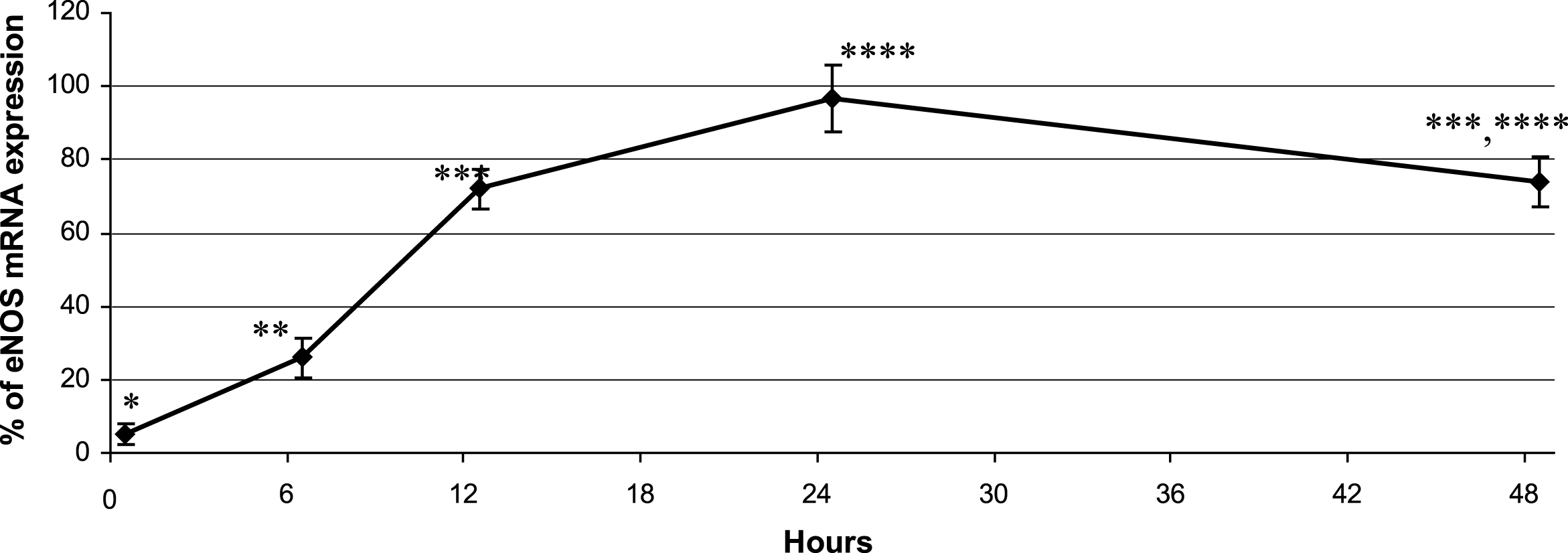
Fig.3
Evolution of endothelial cell viability during 48 h of incubation with different concentrations of leaf extract (0 to 1 mg/mL). Data are shown as means±SD (n = 6). Significant differences at p < 0.05 are indicated by * symbols, for 24 and 48 H of incubation time.
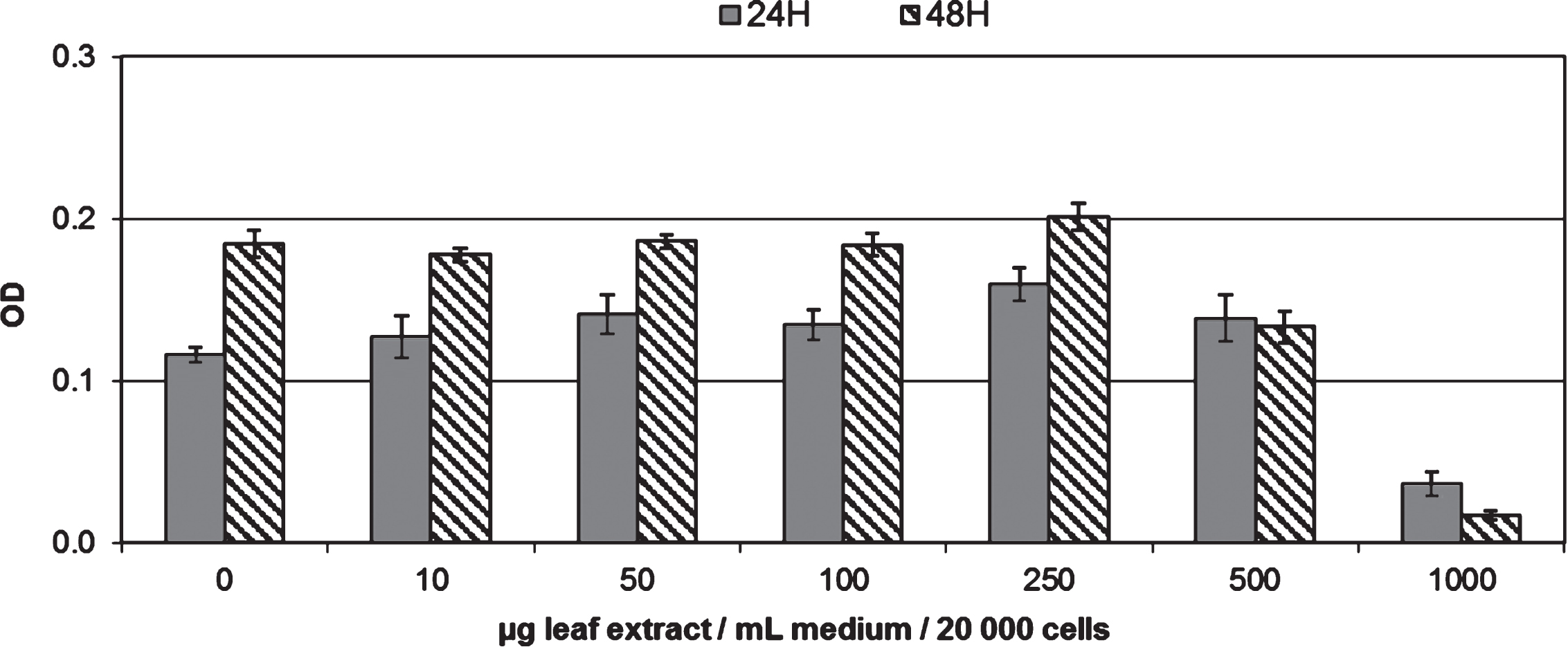
Fig.4
Characterisation of the relaxation induced by increasing concentrations of blackcurrant berry, bud or leaf extracts (0.0001 to 0.1 mg/mL) in porcine coronary artery rings with either an intact endothelium or without endothelium and precontracted with U46619. Data are shown as means±SD of 5 different experiments. The table annexed to the figure shows previous results published by our team reporting the content of various phenolic compounds [22] and the antioxidant capacity of extracts using three methods (TEAC, DPPH and ORAC assays, [14]). A correlation has been made between these results and the endothelium-dependent vasorelaxation results at 0.1 mg/mL of extract.
![Characterisation of the relaxation induced by increasing concentrations of blackcurrant berry, bud or leaf extracts (0.0001 to 0.1 mg/mL) in porcine coronary artery rings with either an intact endothelium or without endothelium and precontracted with U46619. Data are shown as means±SD of 5 different experiments. The table annexed to the figure shows previous results published by our team reporting the content of various phenolic compounds [22] and the antioxidant capacity of extracts using three methods (TEAC, DPPH and ORAC assays, [14]). A correlation has been made between these results and the endothelium-dependent vasorelaxation results at 0.1 mg/mL of extract.](https://content.iospress.com:443/media/jbr/2016/6-3/jbr-6-3-jbr129/jbr-6-jbr129-g004.jpg)
Fig.5
Characterisation of the relaxation induced by increasing concentrations of blackcurrant leaf extract (0.0001 to 0.03 mg/mL) in porcine coronary artery rings, precontracted with U46619. Rings with an intact endothelium were incubated with NG-nitro-L-arginine (100μM). All experiments were performed in the presence of Indomethacin (10μM), except the control. Data are shown as mean±SD of two independent experiments.
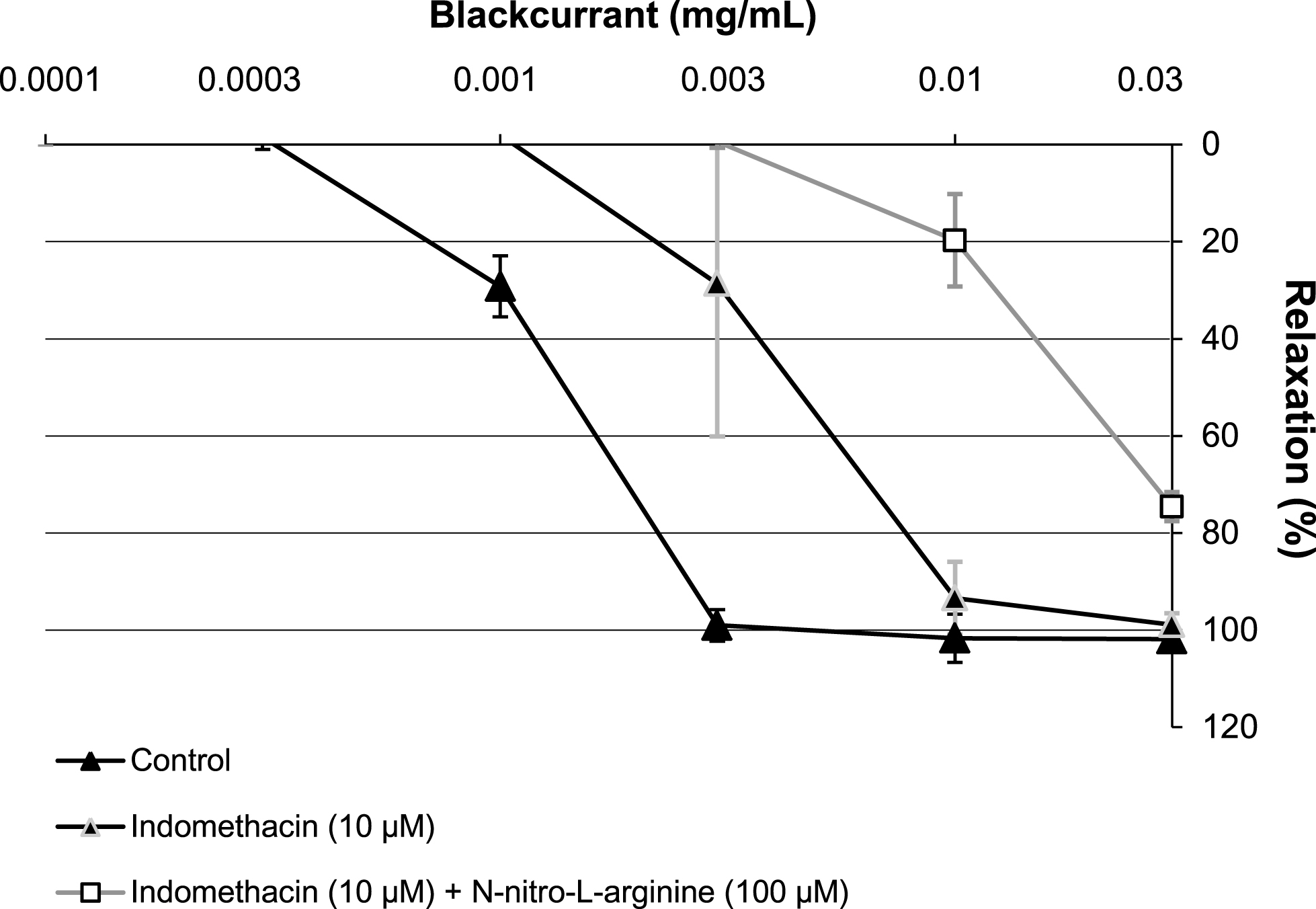
Fig.6
Characterisation of the relaxation induced by increasing concentrations of blackcurrant leaf extract (0.0001 to 0.03 mg/mL) in porcine coronary artery rings, precontracted with U46619. Rings with an intact endothelium were incubated with various inhibitors: NG-nitro-L-arginine (100μM) and/or apamin+charybdotoxin (0.1μM each). All experiments were performed in the presence of indomethacin (10μM). Data are shown as mean±SD of two independent experiments.
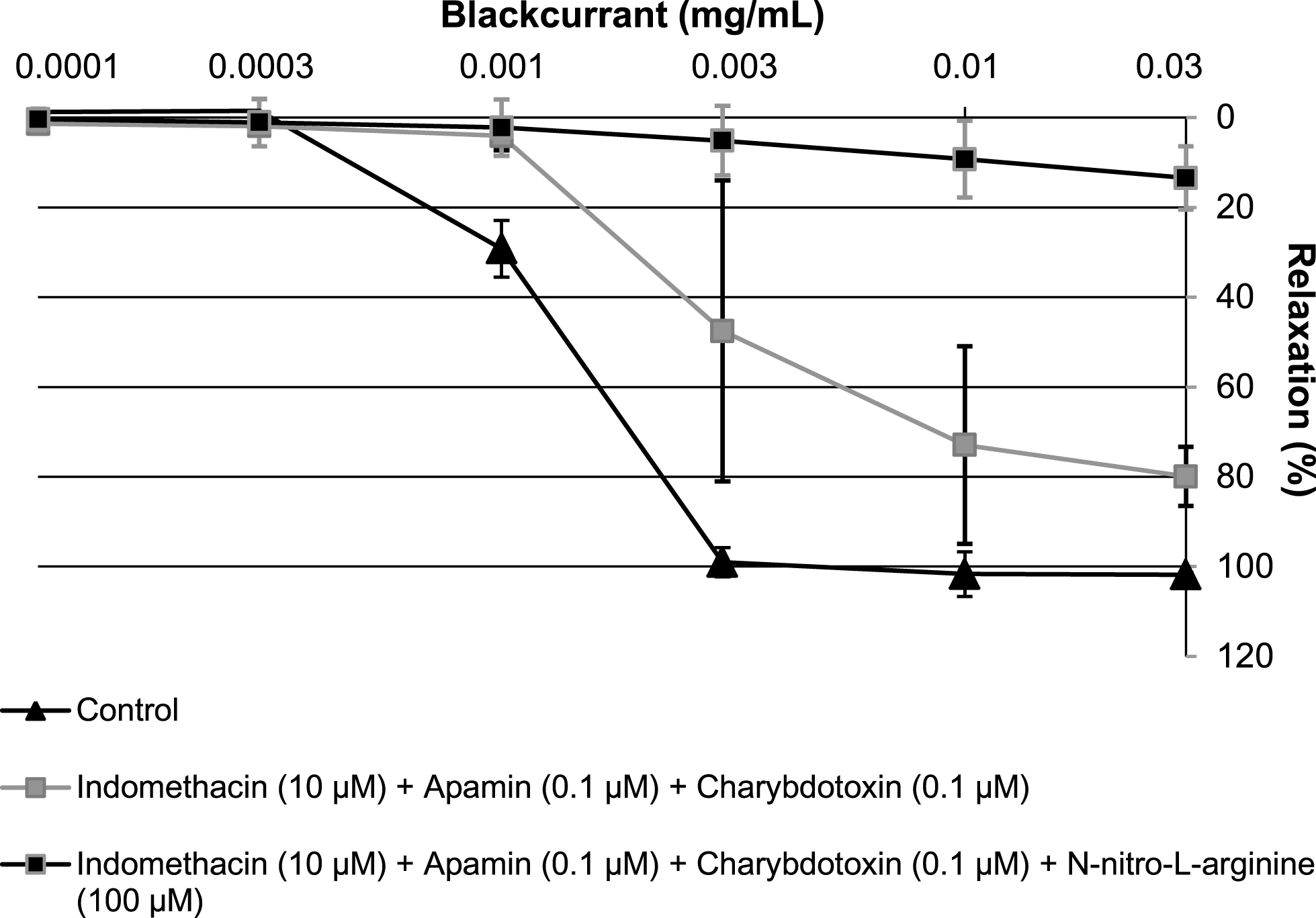
Table 1
Effect of leaf extract on NO formation in EAHy926 cells. 5 × 105 cells were treated with various concentrations of leaf extract (0 to 1 mg/mL). After 5 min, 6 h, 12 h, 24 h and 48 h, NO content was assessed by the Griess method. Data are shown as means±SD of six independent experiments. Significantly difference at p < 0.05 are indicated by a different number of * symbols during the kinetic, for each concentration tested (n = 6)
Conc. | Formation of NO (μM) | ||||||
5 min | 6 h | 12 h | 24 h | 48 h | |||
Control | Med. | 0±0 | 0±0 | 0±0 | 0±0 | 0±0** | |
Leaf extr. | 100 | μg/ml | 1.5±0.6 | 1.0±0.4 | 2.2±0.7 | 1.4±0.9 | 4.0±0.8** |
200 | μg/ml | 5.6±0.7 | 9.7±0.4 | 8.3±2.5 | 6.3±1.2 | 7.3±0.9** | |
300 | μg/ml | 2.1±1.8* | 12.8±0.8** | 11.4±0.9** | 4.2±1.2* | 6.8±1.5** | |
400 | μg/ml | 9.8±1.3* | 11.5±1.1* | 16.0±1.6** | 7.6±2.1* | 6.5±1.8** | |
500 | μg/ml | 9.4±3.7* | 10.0±1.7* | 23.5±2.2** | 7.6±1.7* | 6.3±1.4** | |
750 | μg/ml | 0±0 | 1.9±4.0* | 22.1±2.8** | 5.0±1.4* | 12.2±2.9*** | |
1000 | μg/ml | 0±0 | 9.6±7.1* | 3.0±7.9* | 7.2±1.4* | 31.9±6.5*** |