Greenhouse assays on the control of the bacterial canker of kiwifruit (Pseudomonas syringae pv. actinidiae)
Abstract
BACKGROUND: Pseudomonas syringae pv. actinidiae (Psa) is the etiologic agent of the bacterial canker of kiwifruit, the most severe disease of Actinidia spp. This pathogen was firstly recorded in Japan and in China. The initial occurrence in Italy dates back to 1992, but the most important outbreak was in 2008. From that year, Psa has spread worldwide with a devastating virulence causing substantial losses to kiwifruit production in China, Italy, New Zealand, Chile, France and Portugal.
OBJECTIVE: Screening the existing compounds with different mode of action for their efficacy in controlling Psa on Actinidia deliciosa (cv. Hayward) grown in controlled conditions.
METHODS: Products were grouped according to their active ingredients and mode of action in the following categories: Copper compounds, plant extracts, disinfectants, resistance inducers, filming agents and biological control agents (BCAs). The experiments were performed on potted A. deliciosa (cv Hayward) vines grown in controlled greenhouse conditions. Inoculation was experimentally performed by spraying each plant till run off with a suspension of a highly virulent, biovar 3 Psa strain. Disease control and phytotoxicity were monitored for 15 and 30 days after inoculation.
RESULTS: Copper compounds and resistance inducers (acibenzolar-S-methyl, Fosetyl-Al) showed the most promising results. However, few other compounds, such as some plant extracts and disinfectants (Verdeviva), provided some protection. Also biological control agents (BCAs), containing living microorganisms, partially controlled the disease.
CONCLUSION: Copper compounds and resistance inducers can be possibly combined to develop a more robust and effective control strategy in open field. In addition, BCAs seem interesting, particularly in specific phenological stages when other control methods cannot be used, although results require further validation.
1Introduction
Pseudomonas syringae pv. actinidiae (Psa) is a phytopathogenic, gram-negative bacterium causing the bacterial canker of kiwifruit. The disease affects the all economically important varieties of green-fleshed (A. deliciosa) and yellow-fleshed (A. chinensis) kiwifruit. Before 2008, the disease was reported in Japan [1], China [2], Korea [3] and Italy [4]. However, after 2008, the bacterial canker became a worldwide pandemic disease, threatening the kiwifruit industry in all countries where this crop is strategic such as Italy [5–7], France [8] New Zealand [9] and Chile [10].
The control of bacterial canker of kiwifruit may only rely on preventive methods, since there is no curative treatment known for Psa. Xenobiotic chemical formulates may be preventively applied to help containing the spread of the disease, but are not decisive, and must be accompanied by general measures to reduce inoculum through a good orchard hygiene, and an appropriate field management [11]. The current chemical control of Psa in the field is mainly dependent on spraying of copper-based compounds [12, 13]. The efficacy in the reduction of Psa epiphytic has been shown to vary according to the formulations of copper applied (sulphate or oxychloride) and the rate used [14]. Apart from the environmental concerns linked with copper application, copper compounds can have other limitations, such as bacterial resistance [15–17], phytotoxicity and persistence [18, 19]. Therefore, novel and reliable control strategies should rely on the combination of compounds with different mode action. A wide range of other protective compounds has been evaluated in the past [20], evindencing the effectiveness of some molecules, such as sterilizers (...) and filming agents (chitosan), in the control of bacterial canker. However, although these compounds may temporarily reduce epiphytic inoculum loads, in most cases they are not effective once the pathogen has entered the plant tissue. Integration of plant-induced resistance into the control program for Psa could provide systemic protection of kiwifruit vines ahead of infection risk events.
Based on greenhouse studies, acibenzolar-S-methyl (ASM) has been shown to be one of the most effective elicitors of plant defences, improving kiwifruit tolerance against Psa [21]. Indeed, Psa development can be effectively reduced by the application of resistance inducers priming the salicylic acid (SA) signalling pathway [22]. In addition, the use of BCAs could be useful for the control of Psa, but knowledge about their efficacy and reliability under a range of environmental conditions is still limited [23, 24].
The objective of this research was to screen, in greenhouse conditions, a selection of compounds with different mode of action to evaluate their efficacy in controlling Psa. Differently from previous research, all the different copper formulates were tested in order to provide the same amount of free copper ion.
2Material and method
2.1Experimental conditions and treatments
The experiments were performed on Actinidia deliciosa potted seedlings, grown in standard greenhouse conditions under natural light (relative humidity: 60%, temperature: 20–24°C). Plants were maintained with standard NPK fertilisation and irrigation. Treatments were applied as foliar sprays; concentration, timing and mode of the treatments are shown in Table 1.
The application was preventive (1–10 days before inoculation), except for disinfectants, which were tested before (1 day) and after infection (4-5 hours).
Water-treated plants and streptomycin sulphate (100 mg/l) were used as a negative and positive control, respectively. The products tested include traditional and new copper compounds, plant extracts, biological control agents, resistance inducers, disinfectants, filming agents. Traditional copper compounds (Bordeaux mixture and tribasic copper sulphate, copper oxychloride and hydroxide and copper oxide) were used at a dosage corresponding to about 50 g/100 L of Cu2 +.
2.2Plant inoculation and disease assessment
Pot-cultivated plants (with 5–8 leaves) and the Psa strain CFBP7286 were used in this study.
For the experiments, Psa strain was maintained on Luria Broth agar (1.5%) and incubated at 25±1°C for 24–48 hour. To prepare bacterial suspension, the plates were washed with MgSO4 (10 mM, pH 7) and cell density of Psa strain was adjusted to a turbidity of 0.1 absorbances at 600 nm, corresponding to 106 CFU mL–1. The plants were inoculated by spraying the abaxial surface of all the leaves until run-off with the bacterial suspension. The relative humidity was raised to approximately 100% for the first 72 h after inoculation and kept at 70±5% thereafter.
Symptoms were assessed by two independent observers 15 and 30 days after inoculation. All the experiments were repeated at least two times on independent replicates of five plants each.
The symptoms were rated using a Disease Index corresponding to the percentage of leaf area affected by necrotic spots, according to the following formula:
2.3Statistical analysis
The data are presented as the average efficacy (i. e., the percentage of DI reduction compared to the negative control) of each of independently performed experiment. Standard errors (S.E.) are shown.
3Results and Discussion
The traditional copper based compounds and the Oligal Cu significantly reduced the foliar symptoms, showing an efficacy of 50–80 %. Plants treated with Chelal Kubig showed symptoms of phytotoxicity with necrotic spots on leaves. Treatments with Glucocarrier+Glucoactivator and Labicuper showed a variable behaviour with general low efficacy (Fig. 1).
Biological control agents (BCAs) reduced the foliar symptoms. Increasing the timing between application and inoculation (from 1 to 2 days), the efficacy was increased by 40% (Fig. 2).
Preventive treatments with disinfectants were effective only in some of the experiments. In particular, Biobacter and Bioprotect showed the highest variability in effectiveness especially when applied 1 day prior to inoculation. Verdeviva applied at 4 hours after inoculation was the most effective (94.5%) compound, showing also the highest repeatability of results (Fig. 3).
Concerning the effect of resistance inducers, Bion and Fosetyl-Al showed an efficacy comparable to copper products when applied 7 days before inoculation. On the other hand, when applied at 3 days before inoculation, only Fosetyl-Al was effective. No satisfactory results were obtained with Prohexadione-Calcium (Regalis) (Fig. 4).
The last group of compound tested included formulates with different mode of action. In this group, poly-glucose-amine products such as Hendophyt PS (84% of efficacy) and Layer (81%) showed a high effectiveness. In addition, colloidal silver, applied as Agro Argentum, showed a high degree of control (74%). A lower, but significant effectiveness was showed by plant extracts (Xeda-Tim, Xeda-Cin) or acid clay (Ulmasud) (Fig. 5). Other products were ineffective and/or induced phytotoxicity (ex. Venturex) (Fig. 5).
4Conclusion
The greenhouse trials allowed to screen a large number of products, but, on the other hand, this experimental approach showed some limitations. Indeed, the use of young potted plants grown in conditions of high humidity boosted the phytotoxicity of some products, such as Venturex and Chelal Kubig, far beyond the levels commonly found in orchard conditions.
The highest and most reliable disease control was achieved with the use of traditional copper-based products (i.e. Bordeaux mixture and tribasic copper sulphate, copper oxychloride and hydroxide and copper oxide), and resistance inducers (Bion, Fosetyl-Al). Even though with different experimental approach (movement outside of inoculated plants and repeated treatments), our greenhouse results, were confirmed by Monchiero et al. [28], and currently different field trials rely on the use of these same products [25–28].
Concerning the resistance inducers, the lack of efficacy of Bion when applied at 3 days prior inoculation can be explained by the induction of salicylic acid-dependent plant defences, which need approximately 7 days to build up [22]. In our trials, Fosetyl-Al showed a higher efficacy than Bion, although with a high variability. Other studies showed that under low inoculum pressure, Fosetyl-Al may provide a significant level of protection, but under heavy disease pressure, the same compound may not provide a sufficient disease control [28, 31].
Biological control agents (BCAs) showed promising results, although further validation is required for the optimisation of the rate and timing application. Moreover, BCAs that need to have wide and stable colonization of the epiphytic niche to be effective, cannot be used alone to control the disease along the completely growing season when the environmental conditions limit bacterial growth. Indeed, they should be integrated in a complex control strategy possibly including resistance inducers and copper formulates.
Among the disinfectants, Verdeviva showed a good antimicrobial activity. However, its very low persistence makes it impractical for field use.
Filming agents such as Hendophyt, based on chitosan, or Layer, based on a mixture of amines and polyacrilic acid, showed promising results. These compounds may be used in an integrated strategy together with copper. Indeed, they may help to create a physical protective barrier able to prevent Psa penetration to the apoplast. A recent study indicates chitosan based products as a very promising option for the field control of Pseudomonas syringae pv. actinidiae [29].
In conclusion, even though the use of copper formulates is still the most reliable control strategy, several other tested compounds showed a good activity against Psa, providing alternative or complementary control methods. Indeed, a number of these compounds may support or complement copper compounds in those phenological stages where copper may have phytotoxic effect or can lead to residues in fruits. In addition, the reduction of copper application and its combination with other bactericides may help in minimizing the risk of the development of copper resistance in Psa [17, 30].
Acknowledgments
The research was supported Regione Emilia-Romagna for the CRPV Project “Cancro batterico dell’actinidia (Pseudomonas syringae pv. actinidiae)” and by DROPSA Project “Strategies to develop effective, innovative and practical approaches to protect major European fruit crops from pests and pathogens”- FP7-KBBE-2013-7.
References
[1] | Takikawa Y , Serizawa S , Ichikawa T , Tsuyumu S , Goto M . Pseudomonas syringae pv, actinidiae pv. nov.: The causal bacterium of canker of kiwifruit in Japan. Annals of the Phytopathological Society of Japan. (1989) ;55: :437–44. |
[2] | Wang Z , Tang X , Liu S . Identification of the pathogenic bacterium for bacterial canker on Actinidia in Sichuan. Journal of Southwest Agricultural University (1992) . http://en.cnki.com.cn/Article_en/CJFDTOTAL-XNND199206007.htm. |
[3] | Koh YJ , Cha BJ , Chung HJ , Lee DH . Outbreak and spread of bacterial canker in kiwifruit. Korean Journal of Plant Pathology (1994) ;10: :68. |
[4] | Scortichini M . Occurrence of Pseudomonas syringae pv. actinidiae on kiwifruit in Italy. Plant Pathol (1994) ;43: :1035. |
[5] | Balestra GM , Mazzaglia A , Quattrucci A , Renzi M , Rossetti A . Current status of bacterial canker spread on kiwifruit in Italy. Australasian Plant Disease Notes (2009) ;4: :34. |
[6] | EPPO – Reporting Service. 201111:2009/215. |
[7] | Mazzaglia A , Renzi M , Taratufolo MC , Gallipoli L , Bernardino R , Ricci L , Quattrucci A , Rossetti A , Balestra MG . Cancro batterico dell’actinidia: Il punto della situazione in Italia. Frutticoltura (2010) ;9: :66. |
[8] | Vanneste JL , Poliakoff F , Audusseau C , Cornish DA , Paillard S , Rivoal C , Yu J . First report of Pseudomonas syringae pv. actinidiae, the causal agent of bacterial canker of kiwifruit in France. Plant Dis (2011) ;95: :1311. |
[9] | Everett KR , Taylor RK , Romberg MK , ReesGeorge J , Fullerton RA , Vanneste JL , Manning MA . First report of Pseudomonas syringae pv. actinidiae causing kiwifruit bacterial canker in New Zealand. Australasian Plant Disease Notes (2011) ;6: :67. |
[10] | EPPO. First report of Pseudomonas syringae pv. actinidiae in Chile. EPPO Reporting Service. 3:2011;2055. |
[11] | Donati I , Buriani G , Cellini A , Mauri S , Costa G , Spinelli F . New insights on the bacterial canker of kiwifruit (Pseudomonas syringae pv. actinidiae). Journal of Berry Research. 4 (2014) :53–67. |
[12] | Balestra GM , Varvaro L . Pseudomonas syringae pv. syringae Causal Agent of Disease on Floral Buds of Actinidia deliciosa (A. Chev) Liang et Ferguson in Italy. J Phytopathol (1997) ;145: :375. |
[13] | João PS , Cabral . The antibacterial action of cupric ions in Pseudomonas syringae. FEMS Microbiology Letters (1991) ;79: 2–3:303–8. |
[14] | Balestra GM , Bovo M . Effectiveness of copper compounds in the control of bacterial diseases on kiwifruit plants. No 9-03. Acta Hort (2003) ;610: :399. |
[15] | Rogers JS , Clark E , Cirvilleri G , Lindow SE . Cloning and characterization of genes conferring copper resistance in epiphytic ice nucleation-active Pseudomonas syringae strains. Phytopathology (1994) :891–97. |
[16] | Vanneste JL Voyle MD. Genetic basis of copper resistance in New Zealand strains of Pseudomonas syringae. New Zealand Plant Protection (2003) ;56: :109–12. |
[17] | Masami N , Masao G , Katsumi A , Tadaaki H . Nucleotide sequence and organization of copper resistance genes from Pseudomonas syringae pv. actinidiae. European Journal of Plant Pathology (2004) ;110: (2), 223–6. |
[18] | Lamb DT , Naidua R , Minga H , Megharaja M . Copper phytotoxicity in native and agronomical plant species. Ecotoxicology and Environmental Safety (2012) ;85: (1), 23–9. |
[19] | Serizawa S , Ichikawa T , Takikawa Y , Tsuyumu S , Goto M . Occurence of bacterial canker of kiwifruit in Japan: Description of symptoms, isolation of the pathogen and screening of bactericides. Annals of the Phytopathological Society of Japan (1989) ;55: :427–36. |
[20] | Gaskin RE , Manktelow DW , Cook S , May WA , van Leeuwen RM . Effects of canopy density on spray deposition in kiwifruit. New Zealand Plant Protection (2013) ;66: :194. |
[21] | Reglinski T , Vanneste J , Wurms K , Gould E , Spinelli F , Rikkerink E . Using fundamental knowledge of induced resistance to develop control strategies for bacterial canker of kiwifruit caused by Pseudomonas syringae pv. actinidiae. Frontiers in Plant Science (2013) ;4: :1. |
[22] | Cellini A , Fiorentini L , Buriani G , Yu J , Donati I , Cornish DA , Novak B , Costa G , Vanneste JL , Spinelli F . Elicitors of the salicylic acid pathway reduce incidence of bacterial canker of kiwifruit caused by Pseudomonas syringae pv. actinidae. Annals of Applied Biology (2014) ;165: :441–53. |
[23] | Stewart A , Hill R , Stark C . Desktop evaluation on commercially available microbial-based products for control or suppression of Pseudomonas syringae pv. actinidiae. Bio-Protection Research Centre – Report No 1, 2011. |
[24] | Kiwifruit Vine Health. Innovation Field Trials. 2012. |
[25] | Tosi L , Tacconi G , Spinelli F , Posenato G , Bertaiola F , Giacopini A . Efficacy of some products against the bacterial canker of kiwifruit caused by Pseudomonas syringae pv. actinidiae. Atti Giornate Fitopatologiche (2014) ;2: :157–62. |
[26] | Pizzinat A , Giordani L , Asteggiano L , Nari L , Giraudo M , Pavarino A , Bevilacqua A , Spinelli F , Morone C , Vittone G . Control of bacterial kiwifruit vine disease in Piedmont. Atti Giornate Fitopatologiche (2014) ;2: :163–72. |
[27] | Antoniacci L , Bugiani R , Rossi R , Cavazza F , Franceschelli F , Scannavini M . Efficacy of natural and synthetic products for the control of bacterial canker of kiwifruit. Atti Giornate Fitopatologiche (2014) ;2: :173–80. |
[28] | Monchiero M , Gullino ML , Pugliese M , Spadaro D , Garibaldi A . Efficacy of different chemical and biological products in the control of Pseudomonas syringae pv. actinidiae on kiwifruit. Australasian Plant Pathology (2015) ;44: (1), 13–23. |
[29] | Scortichini M . Field efficacy of chitosan to control Pseudomonas syringae pv. actinidiae, the causal agent of kiwifruit bacterial canker. European Journal Plant Pathology (2014) ;140: :887–92. |
[30] | Marcelletti S , Ferrante P , Petriccione M , Firrao G , Scortichini M . Pseudomonas syringae pv. actinidiae draft genomes comparison reveal strain-specific features involved in adaptation and virulence to Actinidia species. PLoS ONE (2011) ;6: :e27297. |
[31] | Brown S , Koike ST , Ochoa OE , Laemmlen F , Michelmore RW . Insensitivity to the fungicide fosetyl-aluminum in California isolates of the lettuce downy mildew pathogen, Bremia lactucae. Plant Disease (2004) ;88: :502–8. |
Figures and Tables
Fig.1
Efficacy of copper treatments on bacterial canker development in Actinidia deliciosa. Spray treatments were performed 1 day before inoculation. Data refer 30 days after inoculation. Data are expressed as percentage of efficacy, calculated as the reduction of disease incidence and severity compared to untreated control. The average of all the independently performed experiments±standard error (S.E.) is shown.
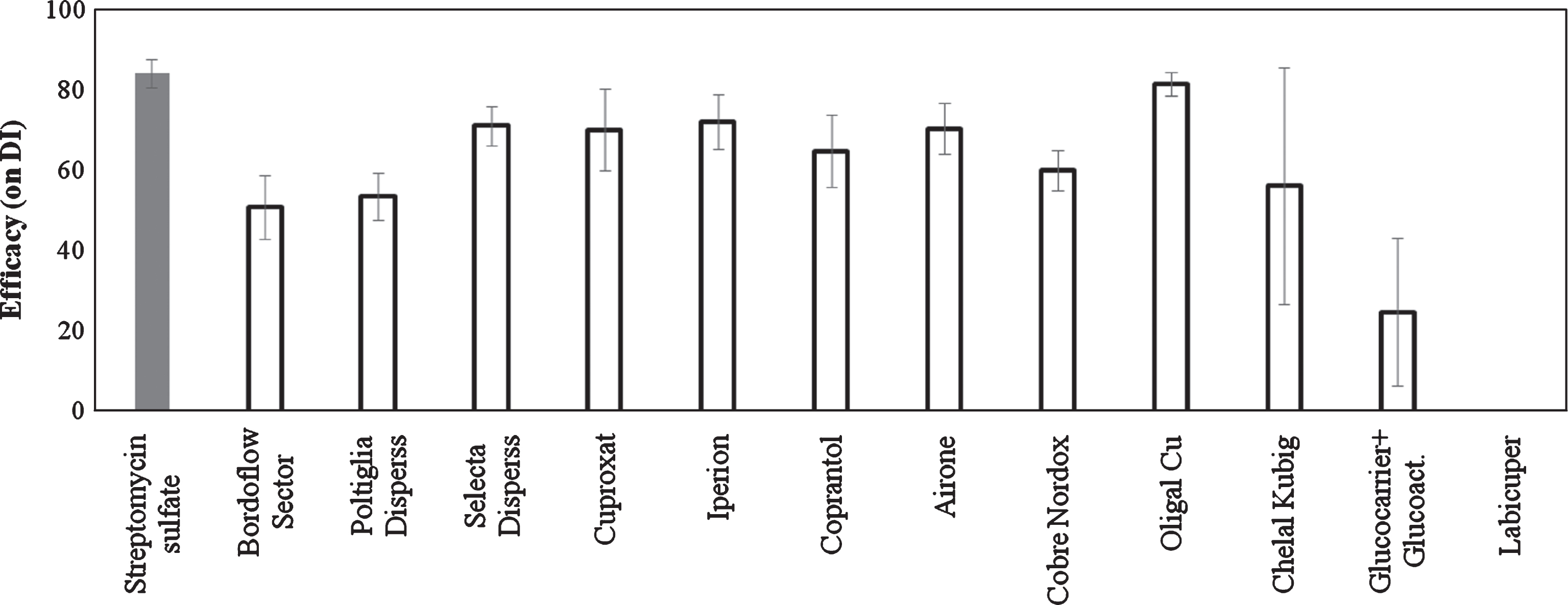
Fig.2
Efficacy of Biological Control Agents (BCAs) on bacterial canker development in Actinidia deliciosa. Spray treatments were performed 1 or 2 days before inoculation. Data refer 30 days after inoculation. Data are expressed as percentage of efficacy, calculated as the reduction of disease incidence and severity compared to untreated control. The average of all the independently performed experiments±standard error (S.E.) is shown.
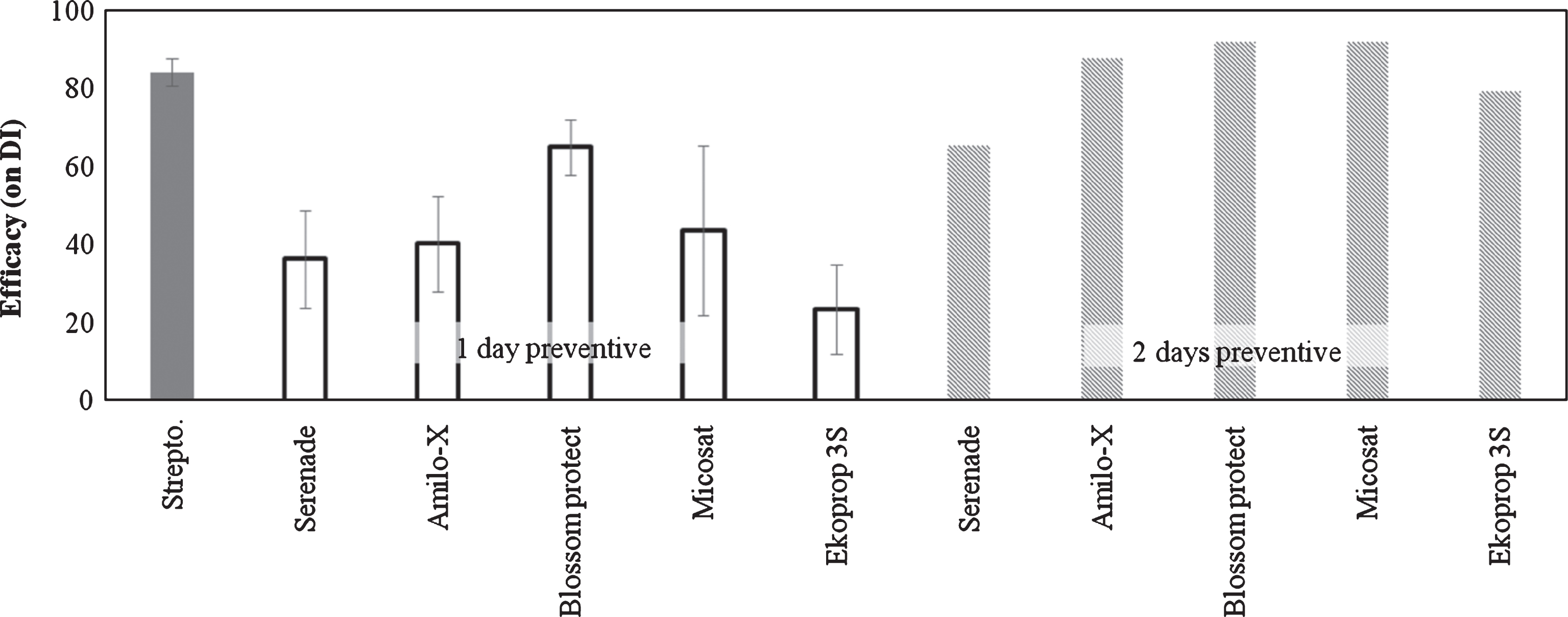
Fig.3
Efficacy of disinfectant products on bacterial canker development in Actinidia deliciosa. Spray treatments were performed 1 day before inoculation (A) or 3-4 hours after inoculation time (B). Data refer 30 days after inoculation. Data are expressed as percentage of efficacy, calculated as the reduction of disease incidence and severity compared to untreated control. The average of all the independently performed experiments±standard error (S.E.) is shown.
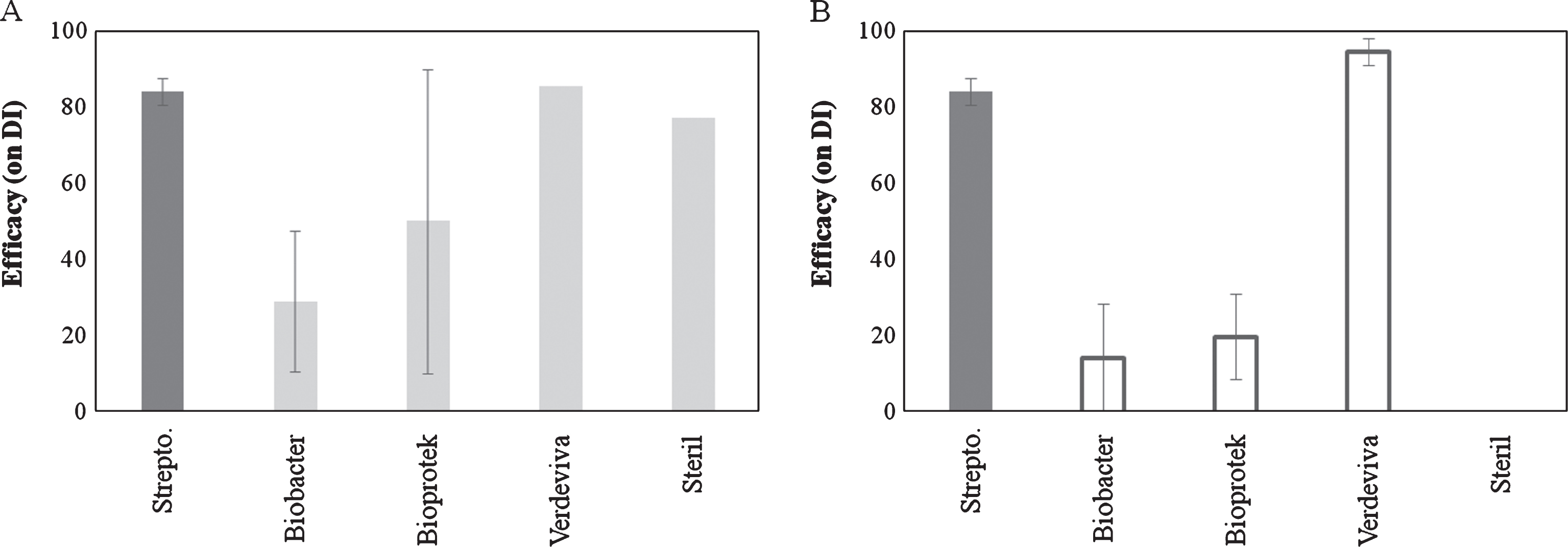
Fig.4
Efficacy of resistance inducers on bacterial canker development in Actinidia deliciosa. Spray treatments were performed 7day before inoculation (A) or 3 day before inoculation (B). Data refer 30 days after inoculation. Data are expressed as percentage of efficacy, calculated as the reduction of disease incidence and severity compared to untreated control. The average of all the independently performed experiments±standard error (S.E.) is shown.
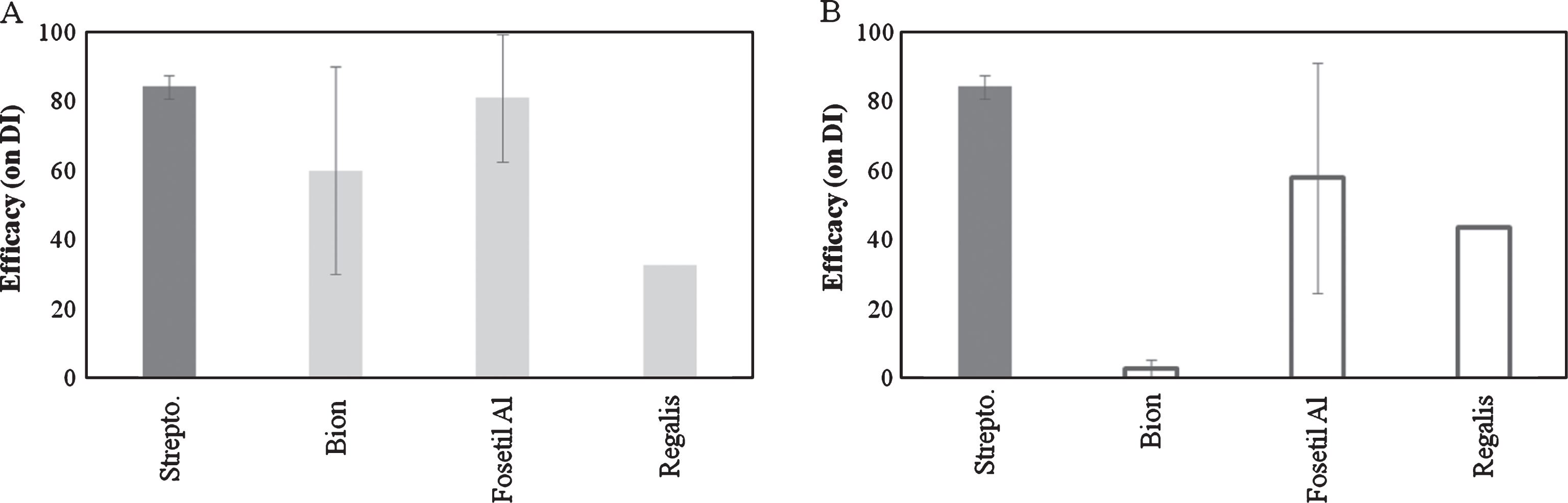
Fig.5
Control of bacterial canker development in Actinidia deliciosa. Spray treatments with various products were performed 1day before inoculation. Data refer 30 days after inoculation. Data are expressed as percentage of efficacy, calculated as the reduction of disease incidence and severity compared to untreated control. The average of all the independently performed experiments±standard error (S.E.) is shown.
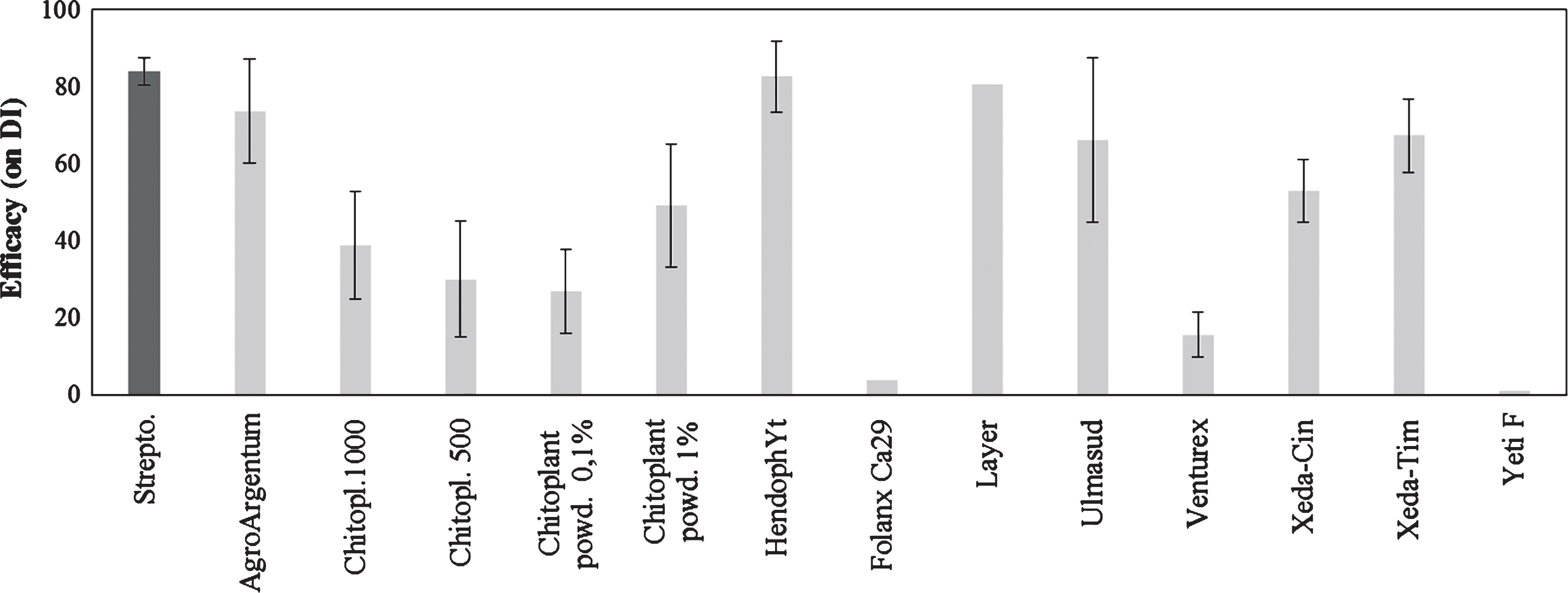
Table 1
Active ingredients, trade names, formulations and application rates of chemicals
Commercial Name | Active Ingredient | Company | a.i. % | Application Rate (100 l water) | n° Assay carried out |
Copper compounds 1 DBI* | |||||
Bordoflow Sector | Bordeaux mixture | Manica | 10 | 400 ml | 8 |
Poltiglia Disperss | Bordeaux mixture | Cerexagri – UPL | 20 | 250 g | 3 |
Selecta Disperss | Bordeaux mixture | Cerexagri – UPL | 20 | 250 g | 4 |
Cuproxat SDI | Tribasic copper sulphate | Nufarm | 27 | 250 g | 5 |
Iperion | Copper oxychloride | Isagro (Siapa) | 37.5 | 130 g | 5 |
Coprantol Hi Bio | Copper hydroxide | Syngenta | 32 | 150 g | 5 |
Airone Piú | Copper hydrox. + c. oxych. | Sumitomo | 14 + 14 | 170 g | 3 |
Cobre Nordox | Copper oxide | Massò | 75 | 67 g | 3 |
Oligal Cu | Copper nitrate | Timac Agro Italia | 11.9 | 50 ml | 2 |
Chelal Kubig | Copper chelated | BMS micro-nutrients | 8 | 100 ml | 3 |
Glucocarrier + Glucoact. | Copper glucoumate | FertireV | – | 200 g+ 1000 g | 2 |
Labicuper | Copper gluconate | Agricola Int. | 8 | 150 ml | 1 |
Disinfectants 1 DBI* or 4 HAI** | |||||
Biobacter Plus | Zinc sulphate, peracetic ac., performic ac., acetic ac. Hydrogen peroxide, carbamide peroxide | LG Italia | 3–15–10–15–35–5 | 200 ml | 7 |
Bioprotek AHC Plus | NPK | Dall’Agata | 10–10–0 | 300 ml | 5 |
Steril | Mixture of ammonium quaternary | LG Italia | – | 500 g | 2 |
Verdeviva | Electrolysed water | Industrie De Nora | – | 200–300 mg/L Free Available Chlorine | 4 |
Resistance inducers 3 or 7 DBI* | |||||
Bion WG | Acibenzolar-S-methyl | Syngenta | 50 | 20 g | 3 |
Fosetil Al | Aluminum tris (O-ethyl phosphonate) | Bayer | 80 | 250–500 g | 3 |
Regalis | Prohexadione Ca | Basf | 10 | 150 g | 3 |
Biological control agents 1 or 2 DBI* | |||||
Amylo-X | Bacillus amyloliquefaciens plantarum D747 | CBC Europe | 25 | 150 g | 7 |
Blossom protect | Aureobasidium pullulans DSM 14940 and DSM14941 | Manica | 25.8 + 25.8 | 1050 g buffer + 150 g yeast | 4 |
EKOprop3S | Glomus spp | Nufarm | 0.5 | 100 g | 3 |
Bacillus amyloliquefaciens, Streptomyces spp., | 1×106 CFU/g | ||||
Trichoderma harzianum | 5×105 CFU/g | ||||
MICOSAT F | Glomus coronatum GO01 and GU53, | Demetra | 40 | 800 g | 5 |
G.caledonium GM24, | |||||
G.intraradices GB67 and GG31, | |||||
G.mossae GP11 and GC11, | |||||
G. viscosum GC41 | |||||
Bacillus subtilis BA41, Streptomyces spp. SB 14, Pseudomonas spp. Saprophytic fungi: Trichoderma harzianum TH01, Trichoderma viride TV03. Yeast: Pichia pastoris PP 59 | 21.6 | ||||
Serenade Max | Bacillus subtilis (strain QST 713) | Bayer | 15.67 | 400 g | 7 |
Other 1DBF* | |||||
Agroargentum | Colloidal silver | Manna Italia | – | 200 ml | 3 |
BioYeti F | Organic fertilizers | Summerfruit | – | 2000 ml | 1 |
Chitoplant powder | Chitosan | Agritalia | 1 | 0.1–1 g | 3 |
Chitoplant solution | Chitosan | Agritalia | – | 500 or 1000 ml | 3 |
Folanx Ca29 | Calcium formate | Lanxess | 29 | 50 g | 1 |
Hendophyt PS | Biopolymers of polysaccharides | Iko-Hydro Srl | 4.5 | 200 g | 2 |
Layer | Mixture of amines, poliacrylic acids | LG Italia | – | 500 g | 1 |
Ulmasud | Acid clays | Nufarm | 70 | 500 g | 2 |
Venturex | Dodine | Agriphar | 35 | 120–140–200 ml | 4 |
Xeda – Cin | Cynnamic aldehyde extract | Xeda | 15 | 0.8 ml | 3 |
Xeda-TIM | Thymol extracts | Xeda | 15 | 0.8 or 1.2 ml | 5 |
*Days before inoculation. ** Hours after inoculation.