Effect of cranberry (Vaccinium macrocarpon) oligosaccharides on the formation of advanced glycation end-products
Abstract
BACKGROUND: The formation and accumulation of advanced glycation end-products (AGEs) are implicated in several chronic human illnesses including type-2 diabetes, renal failure, and neurodegenerative diseases. The cranberry (Vaccinium macrocarpon) fruit has been previously reported to show anti-AGEs effects, attributed primarily to its phenolic constituents. However, there is lack of similar data on the non-phenolic constituents found in the cranberry fruit, in particular, its carbohydrate constituents. Herein, a chemically characterized oligosaccharide-enriched fraction purified from the cranberry fruit was evaluated for its potential anti-AGEs and free radical scavenging effects.
OBJECTIVE: The aim of this study was to evaluate the in vitro anti-AGEs and free radical scavenging effects of a chemically characterized oligosaccharide-enriched fraction purified from the North American cranberry (Vaccinium macrocarpon) fruit.
METHOD: The cranberry oligosaccharide-enriched fraction was purified from cranberry hull powder and characterized based on spectroscopic and spectrometric (NMR, MALDI-TOF-MS, and HPAEC-PAD) data. The oligosaccharide-enriched fraction was evaluated for its anti-AGEs and free radical scavenging effects by the bovine serum albumin-fructose, and DPPH assays, respectively.
RESULTS: Fractionation of cranberry hull material yielded an oligosaccharide-enriched fraction named Cranf1b-CL. The 1H NMR and MALDI-TOF-MS revealed that Cranf1b-CL consists of oligosaccharides ranging primarily from 6-mers to 9-mers. The monosaccharide composition of Cranf1b-CL was arabinose (25%), galactose (5%), glucose (47%) and xylose (23%). In the bovine serum albumin-fructose assay, Cranf1b-CL inhibited AGEs formation in a concentration-dependent manner with comparable activity to the synthetic antiglycating agent, aminoguanidine, used as the positive control (57 vs. 75%; both at 500μg/mL). In the DPPH free radical scavenging assay, Cranf1b-CL showed superior activity to the synthetic commercial antioxidant, butylated hydroxytoluene, used as the positive control (IC50 = 680 vs. 2200μg/mL, respectively).
CONCLUSION: The in vitro anti-AGEs and free radical scavenging effects of cranberry oligosaccharides support previous data suggesting that these constituents may also contribute to biological effects of the whole fruit beyond its phenolic constituents alone. Also, this is the first study to evaluate a chemically characterized oligosaccharide fraction purified from the North American cranberry fruit for anti-AGEs and free radical scavenging properties.
1Introduction
The North American cranberry (Vaccinium macrocarpon Aiton) is widely consumed worldwide, both as a fruit and as processed products such as juice, sauces, and extracts. The cranberry fruit is a rich source of phytochemicals [1], in particular phenolic compounds belonging to the sub-classes flavonoids [2, 3], and proanthocyanidins (PACs) [4]. Moreover, phenolic-enriched cranberry extracts, and singly purified cranberry phenolic constituents have been extensively studied for a vast array of biological activities including antioxidant, antimicrobial, anti-inflammatory, anticancer, and anti-diabetic effects [5]. While the majority of these biological studies have focused on cranberry’s phenolic constituents, emerging data, including recent studies from our group and others [6, 7], suggest that the non-phenolic constituents, especially oligosaccharides, may also play a role in its health benefits.
Glycation occurs spontaneously both in vitro and in vivo wherein protein side chains non-enzymatically react with carbohydrates to form glycation adducts, referred to as advanced glycation end-products (AGEs). The gradual accumulation of AGEs in vivo has been implicated in the pathology of many chronic diseases including diabetic complications and neurodegenerative disorders [8, 9]. The build-up of AGEs is deleterious for two reasons. First, AGEs bind specifically to proteins including the receptor for AGEs (known as RAGE) which upregulates oxidative stress and inflammation [8] and is implicated in several diseases. Second, AGEs can enhance the crosslinking of extracellular proteins, resulting in a loss of functions [10]. Therefore, the reduction of AGEs formation using synthetic pharmaceutical agents, such as aminoguanidine (AG), has been investigated in clinical studies [11]. Given the undesirable side effects of several synthetic agents, including AG [11], the utilization of natural products as dietary intervention strategies for the prevention and therapy of AGEs-related diseases holds great promise [12].
While cranberry and its derived products have been extensively studied for a wide range of biological properties, to date, there is limited data on the effects of cranberry fruit constituents on the formation of AGEs [13, 14]. In these studies, a purified phenolic-enriched fraction, high in PACs content, was shown to inhibit protein glycation by scavenging reactive carbonyl species. However, the authors also reported that phenolic-free water fractions, purified from the cranberry fruit, also exerted antiglycative effects but the active constituents present therein were not identified [13, 14].
Our group has recently reported on the chemical characterization and antimicrobial effects of an oligosaccharide-enriched fraction purified from a water-methanol fraction of cranberry [6]. Therefore, we hypothesized that these oligosaccharide constituents could impart anti-AGEs effects to the fruit beyond its phenolic constituents alone. In light of the aforementioned points, and given our group’s ongoing research interest in identifying natural products with antiglycating activities [15–17], the current study was designed to evaluate the inhibitory effects of cranberry oligosaccharides against the formation of AGEs.
Herein, an oligosaccharide-enriched fraction (named Cranf1b-CL), purified from pectinase treated whole cranberry fruit material, was evaluated for its anti-AGEs and free radical scavenging effects in vitro. Bovine serum albumin (BSA) and D-fructose were used as the model protein and glycating agent, respectively, for the AGEs assay. Inhibition of AGEs formation by Cranf1b-CL was comparable to AG at equivalent concentrations. Cranf1b-CL was also found to have free radical scavenging activity superior to the synthetic antioxidant, butylated hydroxytoluene (BHT) when evaluated in the DPPH assay.
2Methods
2.1Chemicals
Bovine serum albumin (BSA), D-fructose, 2,5-dihydroxy benzoic acid (2,5-DHB), trifluoroacetic acid (TFA), methylglyoxal (MGO), 1,2-phenylenediamine (PD), 2,3-dimethylquinoxaline (DQ), 2,2-diphenyl-1-picrylhydrazyl (DPPH), and aminoguanidine hydrochloride (AG) were purchased from Sigma-Aldrich Chemical Co. (St. Louis, MO, USA). All solvents used were HPLC grade and purchased from Sigma-Aldrich Chemical Co. (St. Louis, MO, USA).
2.2Fractionation of cranberry material
Pectinase (Klerzyme 150, DSM Food Specialties, South Bend, IN, USA) treated cranberry hull powder was generously provided by Ocean Spray Cranberries, Inc. (Middleboro, MA, USA) and further fractionated as previously reported [6] with modification (shown in Scheme 1) using a CombiFlash Rf purification system (Teledyne ISCO, Inc., Lincoln, NE, USA). Briefly, 20 mL of an aqueous solution of the pectinase treated cranberry powder (100 mg/mL) was injected onto a RediSep GOLD C18 reverse-phase column (Teledyne ISCO, Inc., Lincoln, NE, USA) and eluted sequentially with 500 mL of de-ionized water, 500 mL of 15% methanol/water, and 500 mL of 100% methanol. Eluates from each gradient system were individually pooled and freeze-dried. Three major fractions, Cranf1 W (761 mg, 38.1%) eluted with 100% de-ionized water, Cranf1b (476 mg, 23.8%) eluted with 15% methanol/water, and Cranf1M (562 mg, 28.1%) eluted with 100% methanol were obtained.
The oligosaccharide constituents of Cran1b were purified using a Hypersep Hypercarbon solid phase extraction (SPE) cartridge (1 gram, Thermo Scientific, Waltham, MA, USA). The SPE cartridge was first conditioned by eluting with 6 mL of acetonitrile/H2O (50%, v/v) and then washed three times with 6 mL of deionized H2O. Two mL of an aqueous solution of Cran1b (5 mg/mL) was slowly eluted through the cartridge and washed three times with 6 mL of deionized water. The oligosaccharide-enriched fraction was then eluted with 6 mL of acetonitrile/ H2O (30%) acidified with 0.1% trifluoroacetic acid (TFA). The resulting fraction was then finally purified using a C18 reverse-phase SPE cartridge (50 mg, Fisher Scientific, Waltham, MA, USA), by eluting with deionized H2O, and dried in vacuo to yield Cranf1b-CL (7.5 mg).
2.3Nuclear magnetic resonance (NMR) spectroscopy
Five mg of Cranf1b-CL was dissolved in 0.5 mL D2O (99.99%, Sigma-Aldrich, St. Louis, MO, USA) and transferred to a 5 mm NMR tube. 1H NMR spectrum was recorded at 25 °C on a Bruker 300 MHz spectrometer (Billerica, MA, USA).
2.4Matrix-assisted laser desorption/ionization-time of flight (MALDI-TOF) mass spectrometry (MS)
The molecular sizes of oligosaccharides in Cranf1b-CL were recorded by MALDI-TOF-MS (Axima Performance, Shimadzu, Kyoto, Japan) as previously reported using 2,5-dihydroxy benzoic acid (2,5-DHB) as the matrix [18]. Briefly, 2,5-DHB matrix solution was prepared as 50% acetonitrile/H2O solution at 10 mg/mL. Two μL of Cranf1b-CL aqueous solution (5 mg/mL) was mixed well with 2μL of the matrix solution then 2μL of the mixture was spotted onto a MALDI plate, air dried, and analyzed in positive reflectron mode with the power set at 80 kV.
2.5Monosaccharide composition analyses
Cranf1b-CL (200μg) was hydrolyzed with 200μL of 2M TFA in an ampoule for 2 h at 120 °C. The hydrolyte was dried under a stream of nitrogen. The residual acid was removed by adding 200μL of isopropanol and dried in vacuo. The monosaccharide composition of Cranf1b-CL hydrolyte was analyzed by High Performance Anion Exchange Chromatography with Pulsed Amperometric Detection (HPAEC-PAD) [19] using a Hamilton RCX-30 250/4.6 column (Metrohm AG, Riverview, FL, USA) on a 940 Professional IC Vario system (Metrohm AG, Riverview, FL, USA) and eluted with isocratic 16 mM NaOH at 1 mL/min. Fucose, arabinose, rhamnose, galactose, glucose, xylose and fructose were used as monosaccharide standards. Unhydrolyzed aqueous solution of Cranf1b-CL was prepared (1 mg/mL) and its monosaccharide content was quantitatively analyzed by HPAEC-PAD eluting with 100 mM NaOH at 1 mL/min.
2.6DPPH free radical scavenging assay
The free radical scavenging capacity of Cranf1b-CL was evaluated by the 2,2-diphenyl-1-picrylhydrazyl (DPPH) assay based on our previously reported method [20]. Briefly, 100μL of test samples and 100μL of a 0.20 mM DPPH in 50% aqueous methanol solution were mixed for 30 min at room temperature. The absorbance was read at 517 nm using a micro-plate reader (SpectraMax M2, Molecular Devices Corp., Sunnyvale, CA, USA). The scavenging capacity of the sample was calculated as follows: [(ΔAcontrol – ΔAsample)/ΔAcontrol]×100%.
2.7Advanced glycation end-products (AGEs) assay
The inhibitory effect of Cranf1b-CL on the formation of advanced glycation end-products (AGEs) was evaluated by the BSA-fructose assay wherein bovine serum albumin (BSA; 10 mg/mL) and D-fructose (100 mM) were used as the model protein and glycating agent, respectively as previously reported [17]. Treatments included varying concentrations of Cranf1b-CL ranging from 20 to 500μg/mL and a synthetic anti-AGEs agent, aminoguanidine (AG), at equivalent concentrations, which served as the positive control. Each sample was then incubated in the dark at 37 °C for 60 h and their intrinsic fluorescence was measured using a Spectra Max M2 spectrometer (Molecular Devices, Sunnyvale, CA, USA) at an excitation wavelength of 340 nm and an emission wavelength of 435 nm. Statistical analysis was conducted using one-way factorial ANOVA with Tukey-Kramer post hoc comparisons. All results were expressed as means±SD (n = 3) with significance (*) defined as p≤0.05.
2.8Methylglyoxal (MGO) trapping assays
Cranf1b-CL was evaluated for methylglyoxal (MGO) trapping capacity based on our previously reported method [17]. A reaction solution consisting of MGO (5 mM), PD (derivatization reagent, 20 mM), and DQ (internal standard, 5 mM) were freshly prepared in 0.1 M phosphate buffer, pH 7.4. Cranf1b-CL (0.25 mL of 1000μg/mL) was added to MGO (0.25 mL of 5 mM). After incubating at 37 °C for 1 h, 0.125 mL of PD and DQ were each added to mixture. The reaction was kept at room temperature for 30 min. The amount of derivative of residual MGO (2-methylquinoxaline, 2-MQ) was quantified by HPLC-DAD. The percentage decrease of MGO was calculated using the following equation: % MGO decrease = [1 - (MGO amounts in solution with tested sample/MGO amounts in control solution)]×100%.
3Results
3.1Purification of cranberry oligosaccharides
Pectinase treatment is a common process in cranberry juice production and is used to help extract, clarify, and modify the juice [21]. Therefore, starting from an pectinase enzyme treated cranberry powder, an oligosaccharide-enriched fraction, Cranf1b (23.8%; the 15% methanol:H2O eluate) was obtained from C18 reverse phase flash chromatography (Scheme 1). The oligosaccharides of Cranf1b were further purified by porous graphitized carbon solid phase extraction (SPE) and C18 SPE to remove the residual monosaccharides, salts, and other trace contaminants (proteins, lipids, and pigments). The 1H NMR spectrum (Fig. 1) of Cranf1b-CL showed proton resonances between 3.0 ppm and 4.5 ppm, and a group of resonances between 4.5 ppm and 5.3 ppm that are consistent with characteristic signals for anomeric protons in carbohydrates. Notably, there are no visible aromatic protons between 6.5 ppm and 8.0 ppm in the 1H NMR spectrum of Cranf1b-CL indicating that no phenolic components are present in this fraction. This confirmed that Cranf1b-CL was a “phenolic-free” and “oligosaccharide-enriched” fraction purified from cranberry fruit.
3.2Analysis of cranberry oligosaccharides by MALDI-TOF mass spectrometry
MALDI-TOF mass spectrometry (Fig. 2) of Cranf1b-CL revealed several oligosaccharide sodium adduct ions [M+Na]+ that were composed of hexoses (Hex) and pentoses (Pen). Major adduct ions at m/z 923, 953, 1055, 1085, 1217, 1247 and 1379 were consistent with oligosaccharides with compositions of Hex3Pen3, Hex4Pen2, Hex3Pen4, Hex4Pen3, Hex4Pen4, Hex5Pen3 and Hex5Pen4, respectively.
3.3Monosaccharide composition analysis of cranberry oligosaccharides by HPAEC-PAD
The HPAEC-PAD chromatogram (Fig. 3) of hydrolyzed Cranf1b-CL indicated that Cranf1b-CL was composed of four major monosaccharides arabinose (25%), galactose (5%), glucose (47%) and xylose (23%). The monosaccharide content of unhydrolyzed Cranf1b-CL was also evaluated by HPAEC-PAD (Fig. 4) which showed only a trace amount (0.7%) of glucose present in Cranf1b-CL and no other measurable levels of monosaccharides (Fig. 4).
3.4Free radical scavenging activity of cranberry oligosaccharides
As shown in Fig. 5, Cranf1b-CL showed free radical scavenging capacity in a concentration-dependent manner. At concentrations ranging from 1250 –5000μg/mL, the oligosaccharides scavenged more than 50% of free radicals (59–84%, respectively). Also, Cranf1b-CL had an IC50 value of 680μg/mL, superior to the positive control, butylated hydroxytoulene (BHT), a synthetic commercial antioxidant, which had an IC50 value of 2200μg/mL. Notably, Cranf1b-CL (at 1000μg/mL) did not show any methylglyoxal (MGO) trapping capacity (data not shown).
3.5Anti-AGEs activity of cranberry oligosaccharides
For the anti-AGEs assay (Fig. 6), the glycation levels of a BSA-fructose solution (negative control), or BSA-fructose solutions co-treated with Cranf1b-CL (treatment) or with AG (positive control) were evaluated. After an incubation period of 60 h, all of the BSA-fructose solutions generated fluorescence detectable at 340 nm/435 nm confirming the formation of AGEs. However, solutions treated with Cranf1b-CL and AG yielded fluorescence significantly lower than that of negative control (p≤0.05). Among the Cranf1b-CL treated samples, the highest inhibitory effects were observed at 200 and 500μg/mL with reduction in AGEs formation by 53.3% and 56.8%, respectively. At equivalent concentrations, the inhibitory levels of AG were comparable to Cranf1b-CL with reduction in AGEs formation by 73.1% and 75.2%, respectively. However, at concentrations equal to or lower than 100μg/mL, Cranf1b-CL showed comparable or more potent anti-AGEs activities than AG. For example, at 50μg/mL, Cranf1b-CL reduced AGEs formation by 34.8% while AG reduced AGEs formation by 28.8% at an equivalent concentration of 50μg/mL.
4Discussion
Oligosaccharides show numerous biological activities including antioxidant [22, 23], anti-inflammatory [24], and immuno-stimulating effects [25] but those purified from cranberry have only been previously evaluated for antimicrobial properties [6, 7]. Therefore, in the current study, purified cranberry oiligosaccharides, namely, Cranf1b-CL, was tested for its in vitro antiglycative and free radical scavenging effects.
Overall, Cranf1b-CL inhibited the formation of AGEs in a concentration-dependent manner which is likely to be related to its oligosaccharide content. Notably, it has been reported that the antiglycating effects of the polyphenolic constituents, namely PACs, in cranberries are mediated by their reactive carbonyl species scavenging capacity [13]. In the current study, we evaluated the ability of Cranf1b-CL to trap the reactive carbonyl species, methylglyoxal (MGO), using methods previously reported by our group [17]. Interestingly, Cranf1b-CL did not show any MGO scavenging capacity suggesting that its mechanisms of antiglycative effects are different from the polyphenolic constituents of cranberries. Previous studies have shown that oligosaccharides purified from wheat bran show antiglycating effects mediated through metal chelating activities [26]. Therefore, further studies are warranted to evaluate the mechanisms of antiglycating activities of oligosaccharides purified from cranberry.
The limitations of our current study are common to in vitro studies which do not account for physiological relevant considerations such as bioavailability and metabolism. While there is limited data on the bioavailability and metabolism of oligosaccharides, recent studies have underscored the role of intestinal microbiota in their biotransformation [27–29]. Given the relatively large size of these molecules, it is unlikely that they would be absorbed directly as their intact forms. Thus, whether these compounds would be present in tissues in their bioactive (intact and/or metabolized) forms to exert potential anti-AGEs effects would require further in vivo studies. Nevertheless, the current study provides initial data to support the anti-AGEs and free radical scavenging potential of oligosaccharides purified from the North American cranberry fruit.
5Conclusion
In summary, a chemically characterized oligosaccharide-enriched fraction purified from cranberry fruit was shown to exert anti-AGEs and free radical scavenging effects. The current study adds to emerging data supporting the biological effects of cranberry oligosaccharides suggesting that these constituents may impart potential health benefits to the whole cranberry fruit beyond its phenolic constituents alone. Also, this is the first study to evaluate a chemically characterized oligosaccharide fraction purified from the North American cranberry fruit for anti-AGEs and free radical scavenging properties.
Acknowledgments
This project was partially supported by Ocean Spray Cranberries Inc. Instruments used for mass spectrometry and other analyses were supported through an Institutional Development Award (IDeA) from the National Institute of General Medical Sciences of the National Institute of Health (grant number 2 P20 GM103430). NMR data were acquired on instruments supported in part by the National Science Foundation EPSCoR Cooperative Agreement #EPS-1004057.
References
[1] | Pappas E , Schaich K . Phytochemicals of cranberries and cranberry products: Characterization, potential health effects, and processing stability. Crit Rev Food Sci Nutr. (2009) ;49: (9:741–81. |
[2] | Ohnishi R , Ito H , Kasajima N , Kaneda M , Kariyama R , Kumon H , et al. Urinary excretion of anthocyanins in humans after cranberry juice ingestion. Biosci, Biotechnol, Biochem. (2006) ;70: (7):1681–7. |
[3] | Chen H , Zuo Y , Deng Y . Separation and determination of flavonoids and other phenolic compounds in cranberry juice by high-performance liquid chromatography. J Chrom A. (2001) ;913: (1):387–95. |
[4] | Howell AB , Reed JD , Krueger CG , Winterbottom R , Cunningham DG , Leahy M . A-type cranberry proanthocyanidins and uropathogenic bacterial anti-adhesion activity. Phytochemistry. (2005) ;66: (18):2281–91. |
[5] | Crittenden R , Playne MJ . Production, properties and applications of food-grade oligosaccharides. Trends Food Sci Tech. (1996) ;7: (11):353–61. |
[6] | Sun J , Marais JP , Khoo C , LaPlante K , Vejborg RM , Givskov M , et al. Cranberry (Vaccinium macrocarpon) oligosaccharides decrease biofilm formation by uropathogenic Escherichia coli. J Funct Foods. (2015) ;17: , 235–42. |
[7] | Hotchkiss AT , Nunez A , Strahan GD , Chau H , White A , Marais J , et al. Cranberry xyloglucan structure and inhibition of Escherichia coli adhesion to epithelial cells. J Agric Food Chem. (2015) ;63: (23):5622–33. |
[8] | Yan SF , Ramasamy R , Schmidt AM . Mechanisms of disease: Advanced glycation end-products and their receptor in inflammation and diabetes complications. Nat Rev Endocrinol. (2008) ;4: (5):285–93. |
[9] | Li J , Liu D , Sun L , Lu Y , Zhang Z . Advanced glycation end products and neurodegenerative diseases: Mechanisms and perspective. J Neurol Sci. (2012) ;317: (1):1–5. |
[10] | Zieman SJ , Kass DA . Advanced glycation end product crosslinking in the cardiovascular system. Drugs. (2004) ;64: (5):459–70. |
[11] | Thornalley PJ . Use of aminoguanidine (Pimagedine) to prevent the formation of advanced glycation end products. Arch Biochem Biophys. (2003) ;419: (1):31–40. |
[12] | Wu C-H , Huang S-M , Lin J-A , Yen G-C . Inhibition of advanced glycation end product formation by foodstuffs. Food & Funct. (2011) ;2: (5):224–34. |
[13] | Liu H , Liu H , Wang W , Khoo C , Taylor J , Gu L . Cranberry phytochemicals inhibit glycation of human hemoglobin and serum albumin by scavenging reactive carbonyls. Food & Funct. (2011) ;2: (8):475–82. |
[14] | Wang W , Yagiz Y , Buran TJ , Nunes Cd N , Gu L . Phytochemicals from berries and grapes inhibited the formation of advanced glycation end-products by scavenging reactive carbonyls. Food Res Int. (2011) ;44: (9):2666–73. |
[15] | Zhang Y , Ma H , Liu W , Yuan T , Seeram NP . New antiglycative compounds from cumin (Cuminum cyminum) spice. J Agric Food Chem. (2015) ;63: (46):10097–102. |
[16] | Ma H , Liu W , Frost L , Wang L , Kong L , Dain JA , Seeram NP . The hydrolyzable gallotannin, penta-O-galloyl-β-D-glucopyranoside, inhibits the formation of advanced glycation end products by protecting protein structure. Mol Biosyst. (2015) ;11: (5):1338–47. |
[17] | Liu W , Ma H , Frost L , Yuan T , Dain JA , Seeram NP . Pomegranate phenolics inhibit formation of advanced glycation end products by scavenging reactive carbonyl species. Food Funct. (2014) ;5: (11):2996–3004. |
[18] | Hung W-T , Wang S-H , Chen Y-T , Yu H-M , Chen C-H , Yang W-B . MALDI-TOF MS analysis of native and permethylated or benzimidazole-derivatized polysaccharides. Molecules. (2012) ;17: (5):4950–61. |
[19] | Harazono A , Kobayashi T , Kawasaki N , Itoh S , Tada M , Hashii N , et al. A comparative study of monosaccharide composition analysis as a carbohydrate test for biopharmaceuticals. Biologicals. (2011) ;39: (3):171–80. |
[20] | Zhang L , Tu Z , Yuan T , Ma H , Niesen DB , Wang H , Seeram NP . New gallotannin and other phytochemicals from sycamore maple (Acer pseudoplatanus) leaves. Nat Prod Commun. (2015) ;10: (11):1977–80. |
[21] | Beveridge T , Wrolstad RE . Haze and cloud in apple juices. Crit Rev Food Sci Nutr. (1997) ;37: (1):75–91. |
[22] | Katapodis P , Vardakou M , Kalogeris E , Kekos D , Macris BJ , Christakopoulos P . Enzymic production of a feruloylated oligosaccharide with antioxidant activity from wheat flour arabinoxylan. Euro J Nutr. (2003) ;42: (1):55–60. |
[23] | Jiang G , Jiang Y , Yang B , Yu C , Tsao R , Zhang H , et al. Structural characteristics and antioxidant activities of oligosaccharides from longan fruit pericar. J Agric Food Chem. (2009) ;57: (19):9293–8. |
[24] | Ma P , Liu H-T , Wei P , Xu Q-S , Bai X-F , Du Y-G , et al. Chitosan oligosaccharides inhibit LPS-induced over-expression of IL-6 and TNF-α in RAW264. 7 macrophage cells through blockade of mitogen-activated protein kinase (MAPK) and PI3K/Akt signaling pathways. Carbohydr Polym. (2011) ;84: (4):1391–8. |
[25] | Nabarlatz D , Montané D , Kardošová A , Bekešová S , Hřríbalová V , Ebringerováa A . Almond shell xylo-oligosaccharides exhibiting immunostimulatory activity. Carbohydr Res. (2007) ;342: (8):1122–8. |
[26] | Wang J , Sun B , Cao Y , Yuan T . Protein glycation inhibitory activity of wheat bran feruloyl oligosaccharides. Food Chem. (2009) ;112: (2):350–3. |
[27] | Gänzle MG , Follador R . Metabolism of oligosaccharides and starch in lactobacilli: A review. Front Microbiol. (2012) ;3: , 340. |
[28] | Kaplan H , Hutkins RW . Metabolism of fructooligosaccharides by Lactobacillus paracasei 1195. Appl Environ Microbiol (2003) ;69: (4):2217–22. |
[29] | Kitaoka M . Bifidobacterial enzymes involved in the metabolism of human milk oligosaccharides. Adv Nutr. (2012) ;3: (3):422S–9S. |
Figures and Tables
Fig.1
1H NMR spectrum (300 MHz, D2O) of Cranf1b-CL.
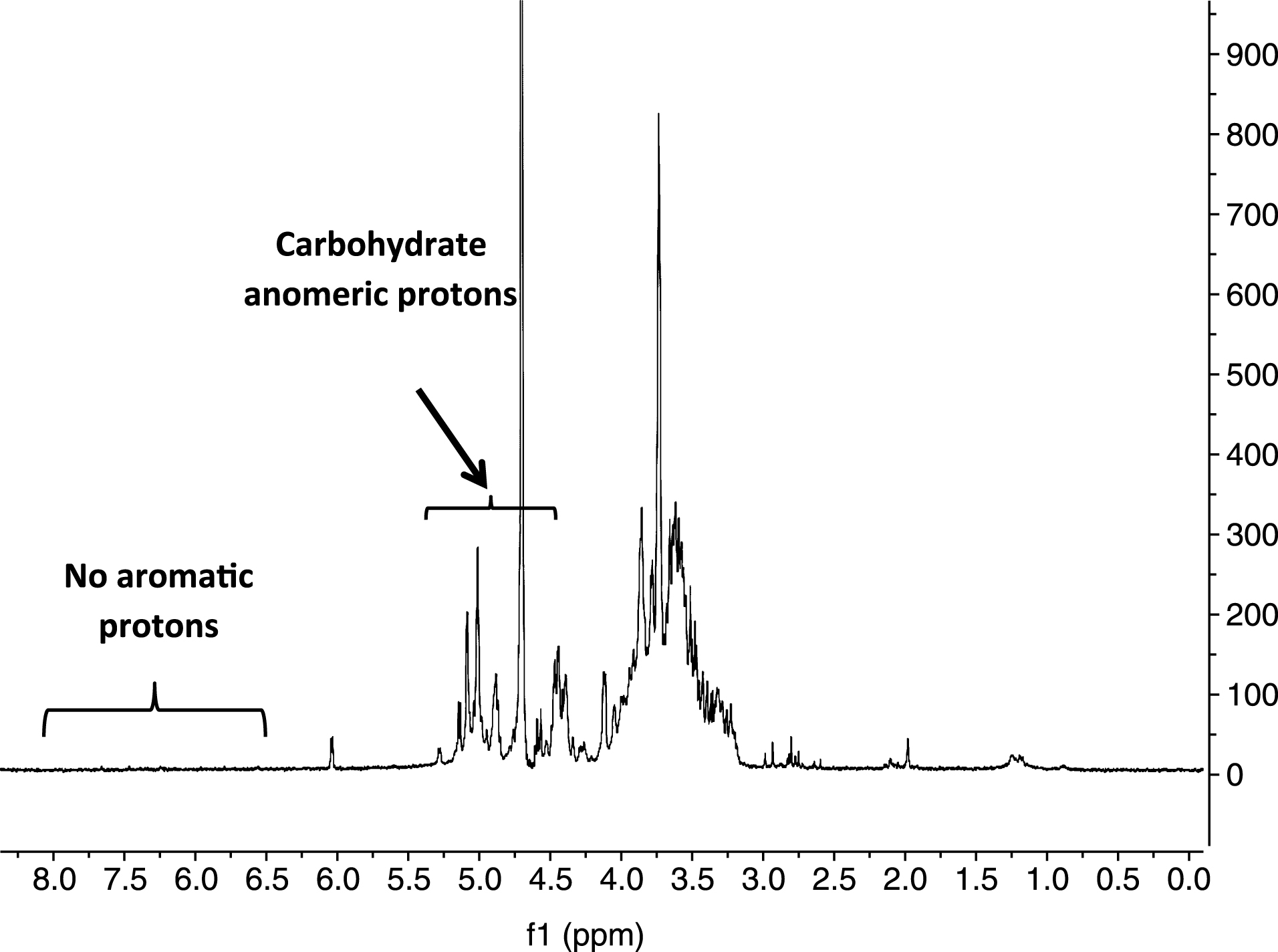
Fig.2
MALDI-TOF-MS of Cranf1b-CL. H3P3: [Hexose3Pentose3+Na]+, m/z 923; H4P2: [Hexose4Pentose2+Na]+, m/z 953; H3P4: [Hexose3Pentose4+Na]+, m/z 1055; H4P3: [Hexose4Pentose3+Na]+, m/z 1085; H4P4: [Hexose4Pentose4+Na]+, m/z 1217; H5P3: [Hexose5Pentose3+Na]+, m/z 1247; H5P4: [Hexose5Pentose4+Na]+, m/z 1379.
![MALDI-TOF-MS of Cranf1b-CL. H3P3: [Hexose3Pentose3+Na]+, m/z 923; H4P2: [Hexose4Pentose2+Na]+, m/z 953; H3P4: [Hexose3Pentose4+Na]+, m/z 1055; H4P3: [Hexose4Pentose3+Na]+, m/z 1085; H4P4: [Hexose4Pentose4+Na]+, m/z 1217; H5P3: [Hexose5Pentose3+Na]+, m/z 1247; H5P4: [Hexose5Pentose4+Na]+, m/z 1379.](https://content.iospress.com:443/media/jbr/2016/6-2/jbr-6-2-jbr126/jbr-6-jbr126-g002.jpg)
Fig.3
HPAEC-PAD chromatogram of monosaccharide components in hydrolyzed Cranf1b-CL.
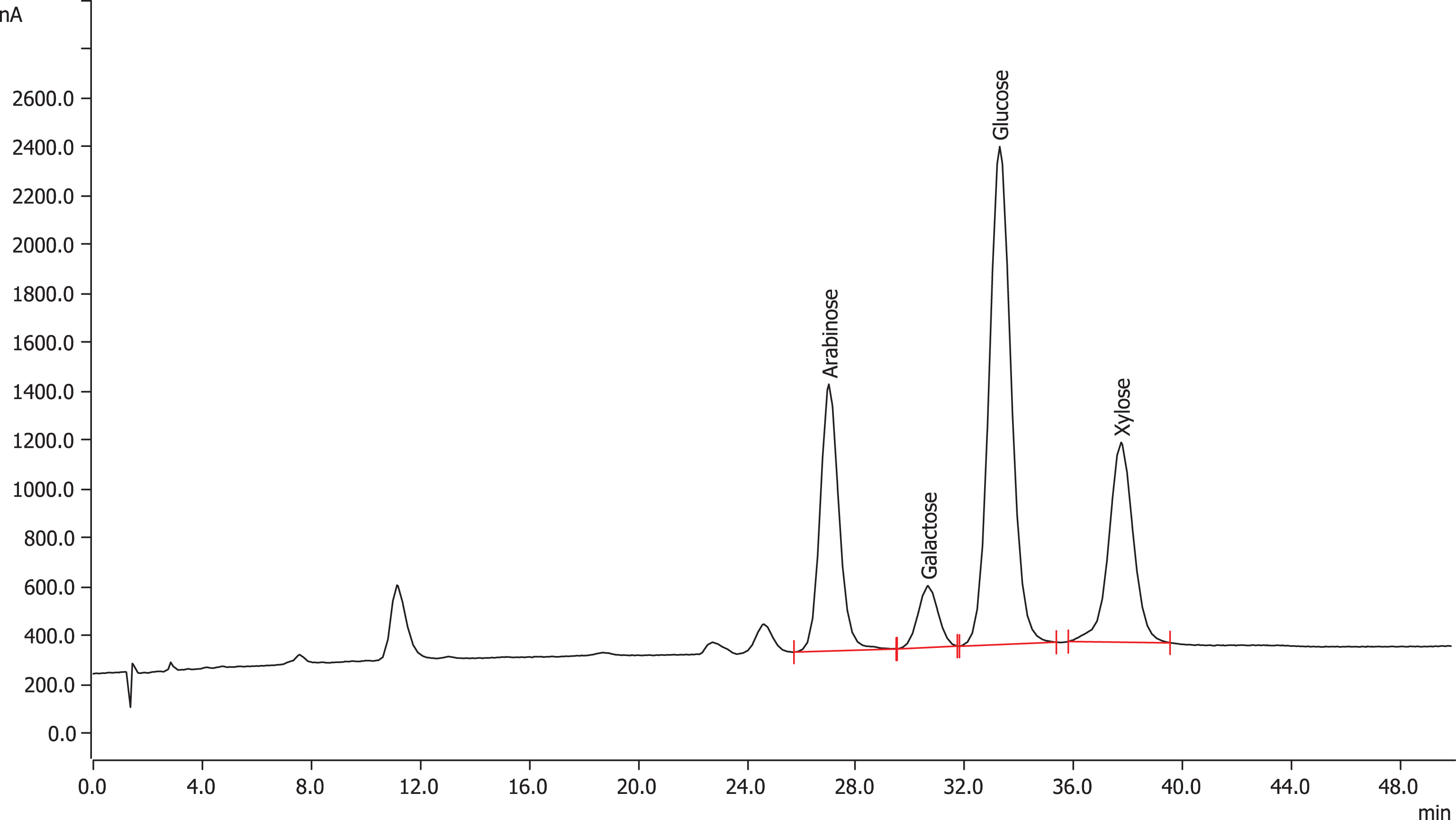
Fig.4
HPAEC-PAD chromatogram of monosaccharide components present in unhydrolyzed Cranf1b-CL.
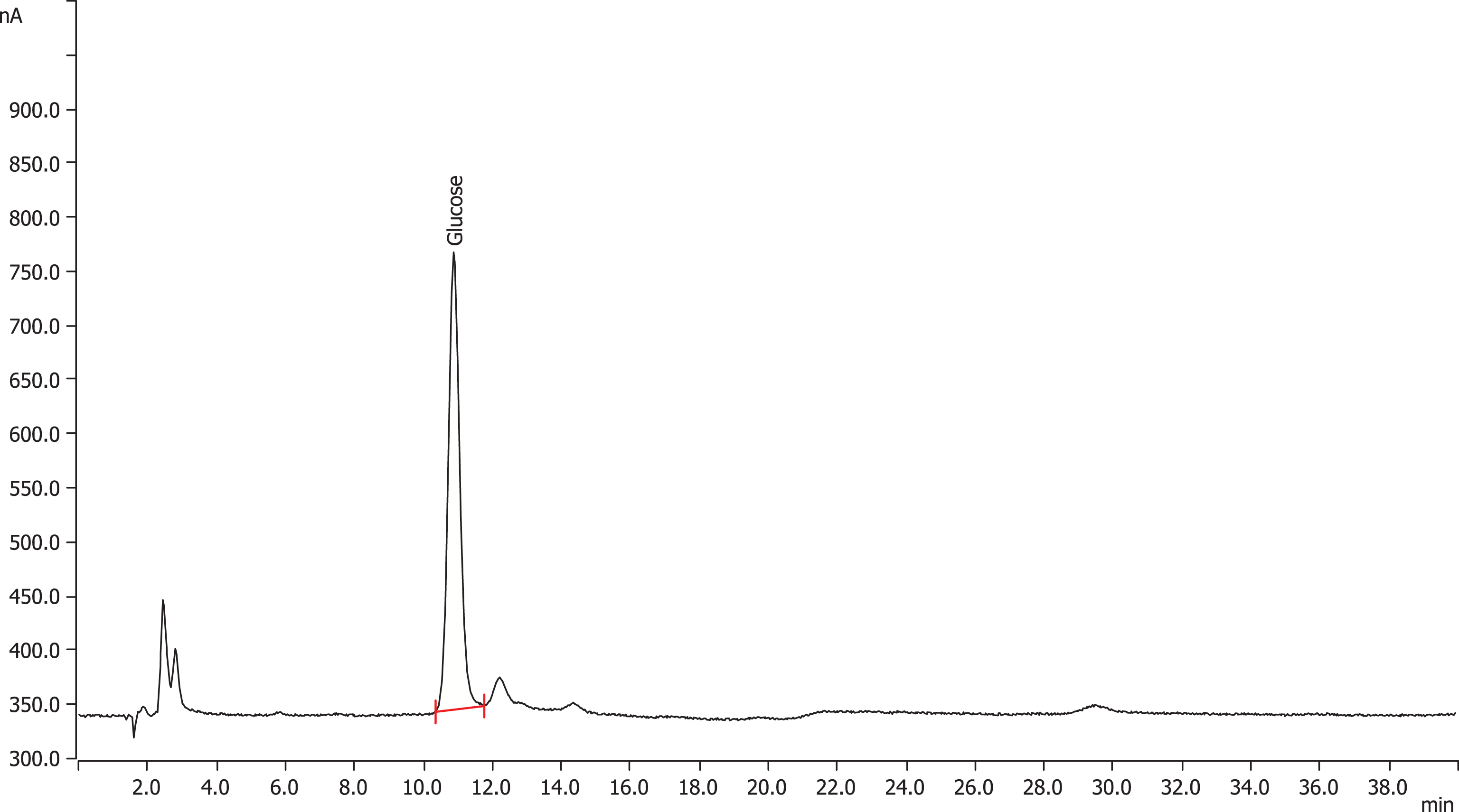
Fig.5
Cranf1b-CL showed free radical scavenging activity in the DPPH assay. Each experiment was conducted in triplicate and all data were expressed in mean±SD (n = 3). BHT (butylated hydroxytoluene) served as the positive control.
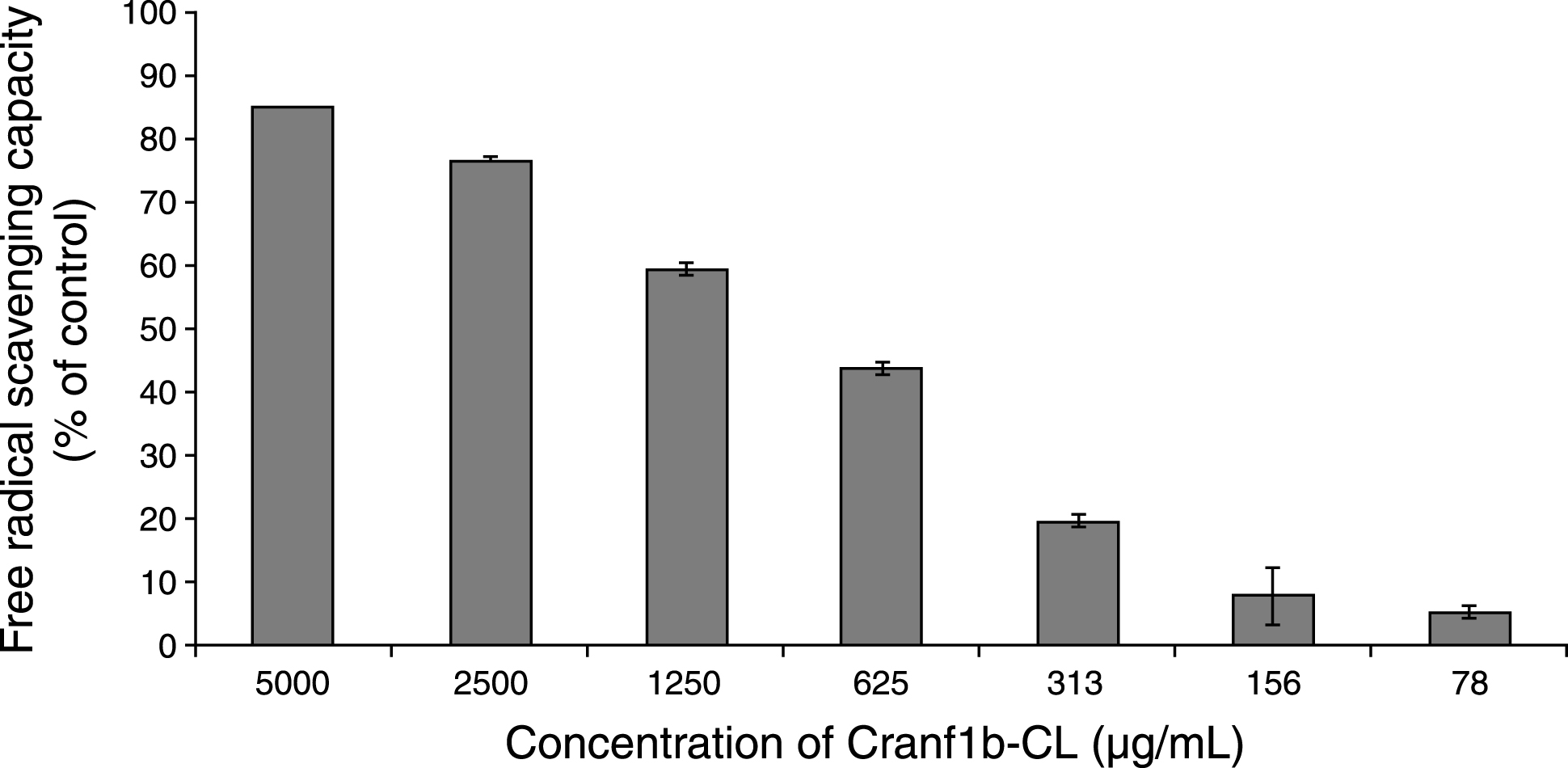
Fig.6
Advanced glycation end-product (AGEs) levels as characterized by intrinsic fluorescence (FU) in BSA-fructose solutions. Each experiment was conducted in triplicate and all data were expressed in mean±SD (n = 3) with significance (*) defined as p≤0.05. Aminoguanidine, a synthetic antiglycating agent, served as the positive control.
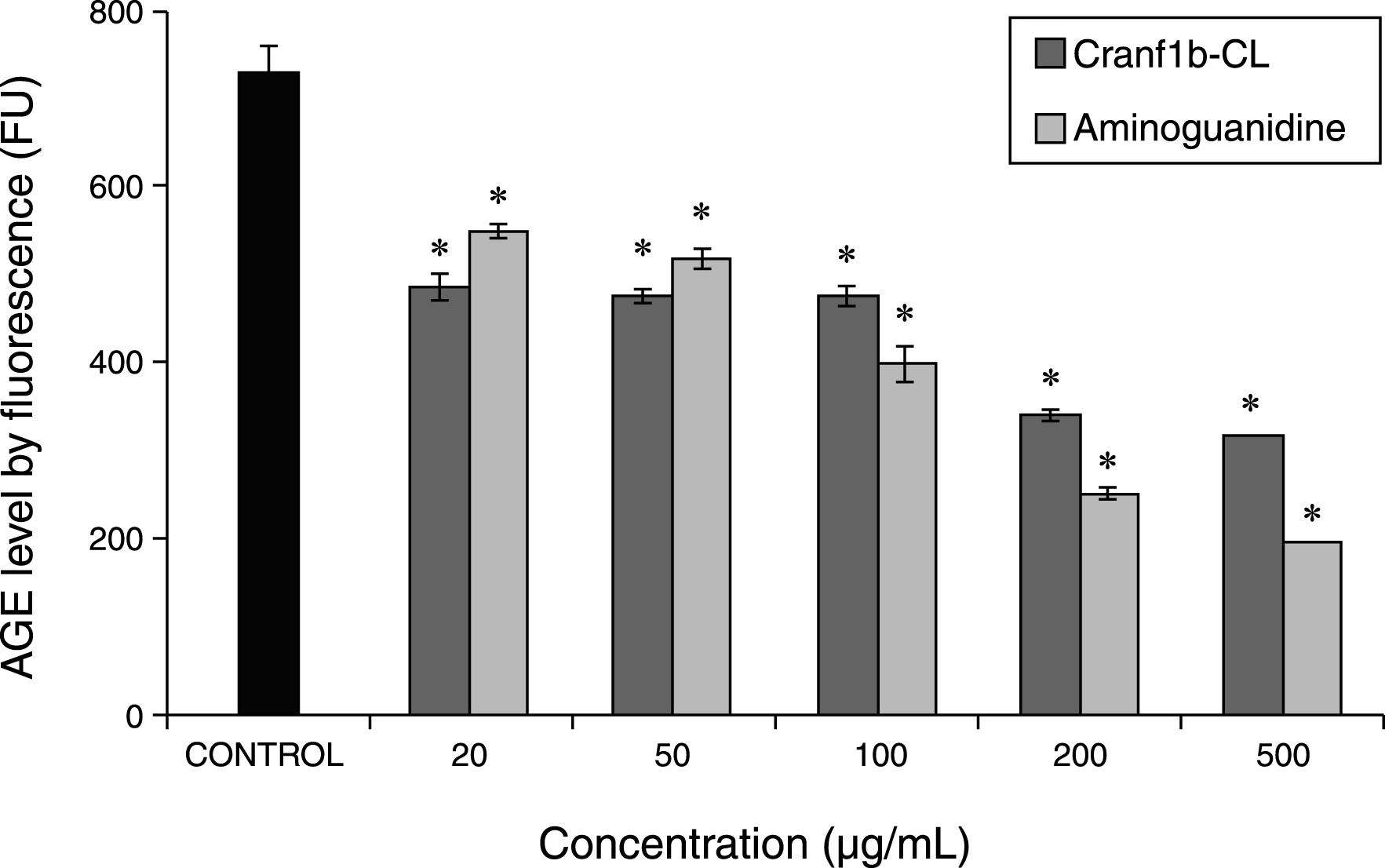
Scheme1
Fractionation and purification of cranberry materials.
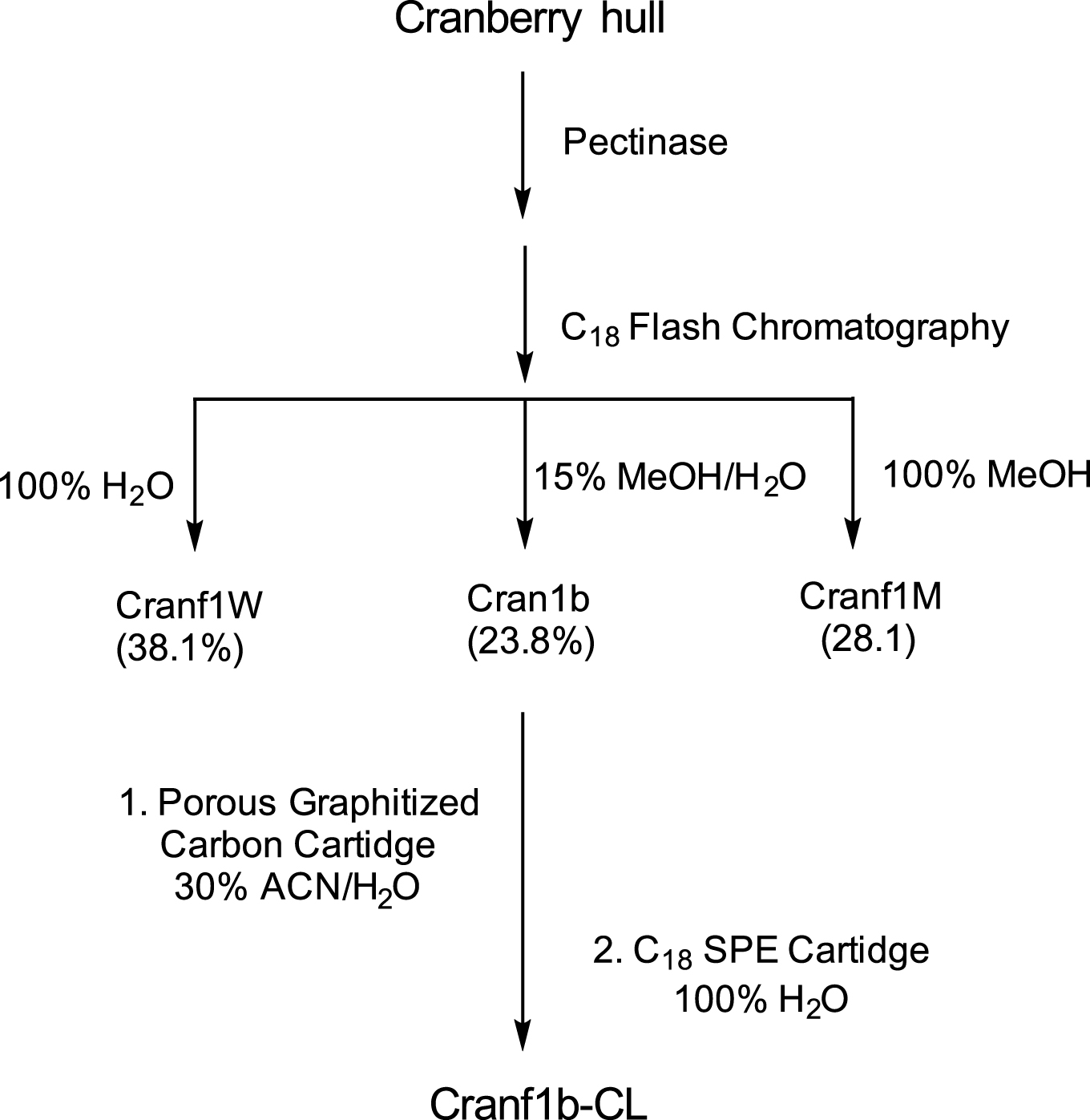