Isolation of bacterial endophytes from Actinidia chinensis and preliminary studies on their possible use as antagonists against Pseudomonas syringae pv. actinidiae
Abstract
BACKGROUND: The bacterial canker, caused by Pseudomonas syringae pv. actinidiae, is the most severe disease of cultivated Actinidia spp. The pathogen is systemic and not easily controlled by agrochemical means.
OBJECTIVE: Our aim was to search, select and identify, among kiwifruit bacterial endophytes, possible antagonists able to control Pseudomonas syringae pv. actinidiae.
METHODS: Several kiwifruit production areas were inspected, in order to find host plants without any disease symptom inside severely affected orchards. From those plants, endophytes were isolated, selected, tested for their ability to inhibit the growth of the pathogen and identified.
RESULTS: A set of 65 different bacterial endophytes was isolated and tested: several of them were able to inhibit the growth of Pseudomonas syringae pv. actinidiae in vitro. None of the antagonists proved to possess either ice nucleation activity or transferable copper resistance. Taxonomically, antagonists belonged to the families of Pseudomonadaceae and Enterobacteriaceae.
CONCLUSIONS: Effective bacterial antagonists were found as endophytes in kiwifruit plants and bearing features of safety of use and negligible risk for the crop. Such finding makes it possible to select the most prospective of them, in order to develop efficient biopesticides able control the bacterial canker in commercial orchards.
Abbreviations
Psa | Pseudomonas syringae pv. actinidiae |
INA | Ice nucleation activity |
AIA | Average Inhibition Area |
1Introduction
The bacterial canker is the most dangerous disease of cultivated Actinidia spp. [1, 2]. The causal agent is the Gram negative rod Pseudomonas syringae pv. actinidiae (Psa). Initially, the pathogen has a significant epiphytic phase on all aerial parts of the host plants, especially on leaves and flowers [3, 4]: after penetration into its host, the pathogen may start an infection process as a systemic endophyte, thus leading to the development of typical disease symptoms and, in several cases, death of the host plant [5]. Overwintering cankers, present on vines and trunks, are the source of primary inoculum. In early springtime, cankers start bleeding, thus causing the evasion and dissemination of Psa and the expression of symptoms. Later, when leaves are developing, necrotic spots surrounded by a chlorotic halo may appear on them, as the result of secondary infections. Additionally, infected flowers may necrotize and fruits might be affected as well, resulting misshapen and later necrotizing [6]. Control of the bacterial canker is quite laborious for at least three reasons: 1) the high virulence of the Psa strains responsible of the latest epidemics in Italy and New Zealand [5, 7]; 2) the high susceptibility of Actinidia chinensis (the species with yellow fleshed fruits) to Psa [5]; and 3) the lack of effective systemic bactericides, since copper compounds may be used to reduce secondary inocula present in the orchards, but they are not able to kill the pathogen systemically [5]. During the recent epidemic events (2008– 2014), hundreds of hectares of affected kiwifruit orchards have been uprooted throughout Italy, in order to eradicate the disease and its pathogen. This was done because of the lack of alternatives for controlling the disease: additionally, orchard destruction followed mandatory official inspections, according the European Council Directive 2000/29/EC [8] and the European Commission Decision 2012/756/EU [9].
In agriculture, control of bacterial diseases has been frequently achieved by using microbial antagonists, in the framework of integrated pest management strategies: these beneficial organisms are present inside microbial communities living on aerial part surfaces [10, 11], in the rhizosphere [12, 13] and inside plants as resident endophytes [14]. Beneficial endophytes may promote plant growth by protecting their host plants from phytopathogenic agents using several and different mechanisms, including production of antibiotics and siderophores [15, 16].
The endophytic life of Psa in kiwifruit plants is essential for the bacterial canker development. Psa, as an endophyte, cannot be efficiently controlled by any known agrochemical, although preliminary experiments suggest that acybenzolar-S-methyl (Bion) may induce a protective state in Actinidia spp. against the pathogen [17]. Therefore, the search of one or more bacterial antagonists able to penetrate into actinidia plants, colonise them and act as efficient inhibitors of Psa endophytic growth appears a good strategy to complement copper sprays, commonly done to limit Psa epiphytic colonisation. Isolation, screening and identification of antagonistic endophytes from plants and trees are usually performed to identify microorganisms able to efficiently colonise the target host plants and act as inhibitors of one or more pathogens in their endophytic phase. Before their possible use, such antagonists must prove to be of no clinical relevance and have no chances to cause possible damages to the host plant. Therefore, the objective of our study was to make an initial investigation on the still unknown endophytic bacterial community of Actinidia chinensis, with particular emphasis on rhizosphere competent microorganisms able to penetrate the roots into their hosts. Our aim was to search for possible resident endophytes, starting their initial characterisation and checking their efficacy in inhibiting Psa in vitro, in order to propose them in a possible strategy for an effective bacterial canker management.
2Material and methods
2.1Plant material
Field inspections were extensively made in 2010– 2013 in the Regions Emilia Romagna and Lazio (North-eastern and Central Italy, respectively). During inspections in affected orchards (over 70 to 90% of severely diseased trees), a few kiwifruit trees were noted with no visible symptoms, side by side with decaying and dying trees. The age of trees in different orchards varied from 5 to 10 years old and the species considered wasA. chinensis, the highly susceptible yellow fleshed kiwifruit. Sixteen trees were collected in different orchards, cutting them at the soil level, below the grafting point, and at the cordons (Fig. 1). From those trees endophytes isolation was attempted.
2.2Isolation of endophytes
From the trunk of sampled kiwifruit plants, 1-2 cm thick disks were cut with a sterile saw every 20 cm, starting from the cordons, then downwards to the grafting point (Fig. 1). For each plant, eight disks were taken, one below the grafting point, two at the base of the permanent cordons and five along the trunk, above the grafting point: in total 128 disks were used for isolation. Those disks were then debarked, thoroughly washed in 70% ethanol and flamed; then, each of them was cut in a few pieces and put into Erlenmeyer flasks containing 100 ml of sterile phosphate buffered saline (per litre: NaCl 137 mmol, KCl 2.7 mmol, Na2HPO4 · 2 H2O 8.1 mmol, KH2PO4 1.76 mmol; pH = 7.4). Flasks were then put on a rotary shaker at 90 rpm for 30 minutes at room temperature. After shaking, the washing buffer was filtered through sterile gauze and centrifuged at 10,000× g for 20 minutes at 4°C. The resulting pellet was diluted with 1– 1.5 ml of the same buffer to obtain the final concentrate (FC). The FC and its 10-fold dilutions were plated onto Nutrient Sucrose Agar (NSA). For each of the 112 samples, 4-5 colonies with different morphology were chosen and purified.
2.3Selection of endophytes
Endophytes were routinely grown on NSA. In order to avoid collection of duplicates, the selected bacterial colonies were subject to genomic fingerprinting with rep-PCR, using the BOX primer [18]. The whole collection was kept in vials at – 80°C in LB broth, added with glycerol (20%, v/v, final concentration). In order to avoid selection of putative antagonists with the ability to act as ice nucleating bacteria, ice nucleation activity (INA) was measured and assessed according Lindow et al. [19]. The nucleation activity was assessed at six different temperatures (from –2, to –7°C).
2.4Preliminary assays
After isolation, the selected endophytes were subject to a few assays to check: assessment of Gram group, identity as possible Psa, possible pathogenicity, and production of pectolytic enzymes. Discrimination of Gram grouping was done using the KOH-sodiumdodecyl sulphate method, as described by Wada et al. [20]. Identity as Psa was checked with PCR, using the protocol of Rees-George et al. [21]. Pathogenicity of all isolates was assessed by the ability to induce a hypersensitivity reaction on tobacco mesophyll [22] and the production of pectolytic enzymes was assessed for endophytes with the potato rot assay [23].
2.5Copper resistance
In order to avoid the selection of putative antagonists showing resistance to copper compounds, a total of 64 bacterial isolates were assayed for their ability to grow on a medium supplemented with copper. Seven μl of a bacterial suspension (108 cfu ml–1, spectrophotometrically assessed) were pipetted onto Mannitol-Glutamate-Yeast agar (MGY) in series of Petri dishes, supplemented with 50, 100, and 200 ppm of Cu++ (as CuSO4 · 5 H2O) and then kept up to five days at 27°C. The growth of the macrocolonies was observed daily. As a control, the same isolate inoculations were done onto MGY agar, but without supplement of copper. As a copper resistant isolate, Xanthomonas arboricola pv. juglandis, strain DLS Xj79, was used as a reference.
2.6Biocontrol activity
The biocontrol activity of endophytes against Psa was assessed in vitro and was done using the Psa strain CRA-FRU 8.43, representative of the causal agent of the bacterial canker epidemic in Italy during the years 2008– 2014. In order to check if putative antagonists may show a general inhibitory effect against other phytopathogenic bacteria, together with Psa, the following Gram negative and Gram positive bacteria were considered: Erwinia amylovora, Xanthomonas arboricola pv. pruni, X. a. pv. juglandis, Xanthomonas oryzae pv. oryzae, Clavibacter michiganensis subsp. michiganensis, C. m. subsp. insidiosus, C. m. subsp. sepedonicus. For each putative bacterial antagonist, a 10 μl droplet of bacterial suspension at a concentration of 108 cfu ml–1, spectrophotometrically measured, was pipetted onto NSA in a Petri dish and then incubated for 48 hours at 27°C, to allow the growth of a macrocolony of the putative antagonist in the centre of the inoculated dish. Once the macrocolonies were grown, the Petri dishes were taken out from the incubator, opened inside a sterile hood and sprayed with a suspension of Psa, at a concentration of 106 cfu ml–1. The Petri dishes were then closed and placed again into the incubator at the same temperature as above, for additional 2 days, to allow growth of the pathogen. The inhibition activity of the putative bacterial antagonists against Psa was measured calculating the average inhibition area (AIA) around the macrocolony as AIA = (R2*3.14) – (r2*3.14) (Fig. 4). Inhibition experiments in vitro were done in triplicate and data were subject to one-way ANOVA.
2.7Iron competence
Iron competence of selected antagonists was assayed on King’s B medium agar plates, supplemented with 10 and 100 ppm of Fe+++. To obtain the final iron concentration, King’s medium B was supplemented with a calculated amount of a 0.1 M FeCl3 x 6H20 solution in 0.1 M of HCl. Iron competence was measured for four endophytes, with proven and strong antagonistic activity, later identified as: Pseudomonas synxantha, Enterobacter sp., Pseudomonas putida and Pseudomonas mendocina. Inoculation of antagonists onto KB medium was done by pipetting a 10 μl droplet of the prospective antagonist, at a concentration of 108 cfu ml–1 at the centre of the plate. Control plates, containing King’s medium B without addition of iron, were used in parallel to evaluate iron competence. Plates were then incubated at 25°C for 48 hours. Inoculation of Psa and evaluation of its inhibition was done as described above.
2.8Identification of antagonists
The identification of endophytes, showing a prospective biocontrol activity against Psa, was done by analysing their fatty acid methyl ester profiles (FAME) [24] and all profiles were compared with TSBA Sherlock® Microbial ID System (MIS) and NCPPB libraries (MIDI Inc., Newark, USA). Eventually, sequencing of the 16 S ribosomal RNA (rRNA) gene was done to confirm identity of possible antagonists [25].
3Results
3.1Isolation and purification of endophytes
Isolation and purification of bacterial endophytes from kiwifruit trunks led to a collection of 64 taxonomically different bacterial isolates. Additionally, Psa was also isolated and identified in some of the samples analysed during the search of endophytes. The endophytic presence of Psa was most frequent in the upper part of the trees, near the cordons, and decreased in number in the lower trunk disks (Fig. 2). Psa was never detected endophytically below the grafting point. The high heterogeneity of endophytes associated to actinidia plants was confirmed by the genomic fingerprinting using the BOX primer (Fig. 3). All 64 different endophytic isolates were subject to the HR assay: none of them elicited any necrosis, once injected into tobacco mesophyll. On the contrary, those additional endophytes identified as Psa gave a hypersensitive necrosis, thus confirming their pathogenicity. The presence of a set of pectolytic enzymes was shown in only four endophytic isolates out of 64: such four endophytes were able to rapidly macerate potato discs within 2-4 days, thus confirming the production and secretion of a set of plant cell degrading enzymes. Pectolytic endophytes were excluded from further studies.
3.2Ice nucleation activity
INA was found in some isolates from Actinidia spp. Eleven isolates showed an INA at – 7°C and at – 6°C; nine of which were still active in nucleating ice at – 5°C. Among them, six of the isolates were remarkably active in showing an INA: they caused freezing of pure water already at – 4°C. None of the other bacteria ever showed any INA.
3.3Tolerance to copper
The assays to confirm the possible presence among the endophytic bacteria of copper resistant/tolerant isolates showed that 4 of the endophytes were able to grow quite well on agar medium supplemented with 50 ppm of copper, forming macrocolonies comparable to the control ones. When copper concentration was increased to 100 ppm, none of the 64 endophytes of our collection was able to grow.
3.4Biocontrol activity of endophytes
The biocontrol activity of endophytes against Psa was checked and measured for those isolates not causing a hypersensitive response on tobacco, or showing pectolytic or ice-nucleating activity, or growing on copper-enriched media. Among the endophytes, 14 isolates were able to significantly inhibit the growth on Psa in vitro: six of them were particularly active, giving an inhibition halo up to 1,000 mm2 (Table 1). In addition, some of the actinidia endophytes were able to inhibit several phytopathogenic bacteria besides Psa. In particular, four of the antagonists (isolates 63, 87A, 93A, 94A) were able to significantly inhibit the growth of Psa and other six important phytopathogenic bacteria (Table 1). In other cases, such as the isolate 80 C, antagonists were rather specific for Psa. A single isolate, strain 73B, had no significant inhibitory effects on Psa growth in vitro, but was active against Xanthomonas campestris pv. juglandis and Clavibacter michiganensis subsp. insidiosus. A quantitative comparison of the inhibitory effect of the eleven most active endophytic antagonists against Psa confirmed their activity (Fig. 4). In Table 2 our results show that different endophytic species may have different level of antagonistic activity and, also, among the same bacterial species, different isolates may show different ability to hinder growth of Psa, as in case of isolates 61A and 62 of Pantoea agglomerans, or isolates 65, 80C and 93A of Pseudomonas synxantha. The most active endophyte confirmed to be strain 65 (DLS65). The isolate DLS65 was identified as Pseudomonas synxantha using the fatty acid methyl ester profiles (FAME) and its identity was confirmed with the sequencing of the 16 S rRNA.
The other endophytes displaying an inhibitory activity against Psa were identified as: Pseudomonas putida biotype A, Pseudomonas fluorescens, Pseudomonas mendocina, Kluyvera intermedia, Pantoea agglomerans, and other two additional species belonging to the Enterobacteriaceae family (Table 2).
Strain DLS65, identified as Pseudomonas synxantha, induced the same level of inhibition on media with or without iron. For P. mendocina and P. putida no inhibition halo was observed. For Enterobacter sp. an inhibition area of approx. 600 mm2 was observed on control medium, whereas the inhibition halo was remarkably smaller (approx. 200 mm2) with 10 ppm of iron and was barely visible with 100 ppm of iron.
4Discussion
4.1Isolation and purification of endophytes
The isolation of endophytic bacteria from Actinidia spp. showed the great complexity of the microbial communities associated to commercial kiwifruit orchards: several dozens of different bacterial endophytes were found to colonize the trunk internally. Therefore, far from being poor in microbial diversity, the cultivated kiwifruit endophytic microbiota proved to be highly differentiated, as other authors showed for other plant species [26, 27]. During our numerous surveys and sampling activity aimed to collect endophytes, the bacterial pathogen Psa, causal agent of the bacterial canker of kiwifruit, was also consistently isolated during the study of the kiwifruit microbial communities, although only symptomless plants were chosen for isolating such endophytes. Interestingly, when searching Psa and antagonistic endophytes in the same symptomless trees, we frequently observed that Psa was easily detected, as an endophyte, in the upper part of the plants, starting from the main cordons. Samples taken from the lowest part of the plants frequently failed to lead to a positive Psa isolation. On the contrary, the most prospective antagonistic isolates, like Pseudomonas synxantha, were more frequently detected and isolated from the lower part of the plant, also below the grafting point. The main reason of that might be connected with the fact that Psa easily penetrates through leaf stomata and twig lenticels and wounds, colonizing its host plant basipetally, therefore with the chance to colonize the trunk starting from the canopy. Additionally, many kiwifruit endophytes identified in this study are known soil/rhizosphere bacteria [28, 29], able to enter the plant through the roots and colonizing the tree acropetally. Therefore, it might be possible that high number of such active endophytes in the lower part of the actinidia trees slows down or inhibits the movement of Psa from the canopy to the rootstock and the roots. This is in line with our results, where selected endophytes showed a high level of direct antagonism against Psa and other plant pathogenic bacteria. This antagonism might be, among other mechanisms, the result of the production of effective antibacterial metabolites, helping such endophytes during their plant tissue colonization, as preliminarily demonstrated by Tontou et al. [30].
4.2Selection of endophytes for biocontrol assays
The investigation inside the Actinidia spp. microbial community was focused on the search of prospective bacterial antagonists, with a relevant inhibitory or bactericidal activity against Psa, in order to develop a possible strategy for the biocontrol of the bacterial canker of kiwifruit, based on the use of such antagonists. Therefore, some biological/ecological aspects have been taken into consideration, when selecting endophytes with a possible application in the field. It is well known that many endophytes belong to the family Pseudomonadaceae and Enterobacteriaceae. Both families include bacterial species with a strong pectolytic activity: this activity is detrimental for a prospective antagonist, since it might cause damage to the plant tissue, if the organism is sprayed in high concentration on plant organs. Indeed, a marked pectolytic activity was associated to some of the endophytes: four out of 64 endophytes were positive and were excluded from further investigations. An additional ecological feature, which is not desirable for a prospective antagonist, is the possibility to act as an ice nucleating agent (INA+ bacterium). Several pseudomonads and some plant-associated enterobacteria have been shown to possess this feature [32]. These ice nucleating bacteria are particularly dangerous in areas where winter temperatures are significantly below 0°C for a longer period, especially in late wintertime or early spring, when the plants start to be active [33]. These INA+ microorganisms are able to catalyse ice formation at temperatures much higher than most organic and inorganic compounds do, thus inciting frost damages to plant tissues and organs already at – 2°C or somewhat lower. This activity is particularly evident in temperate climates, between – 5°C and – 2°C, which is the optimal climate for cultivation of commercial, high quality kiwifruits. Our results showed that INA+ bacteria were present, and were isolated from the microbial community of Actinidia spp.: six of which were particularly active in their ice nucleating activity. Therefore, all isolates showing any INA+ activity above – 7°C were excluded from further studies since, even if they would show some antagonistic activity against Psa, their use as biocontrol agents would pose an unacceptable risk to kiwifruit cultivation in temperate climates [33].
4.3Copper tolerance
Psa has been described as a phytopathogenic bacterium showing significant epiphytic and endophytic phases [3]. For this reason, any strategy for its control should necessarily consider the use of copper compounds to prevent a high population density of the pathogen and its penetration into the host through stomata, hydatodes, lenticels and wounds [34]. So far, copper resistant Psa strains have been described [35], but they were not reported during the recent, severe epidemics observed in the last 4-5 years [36]. Nonetheless, horizontal transfer of genetic sequences between Psa and other bacteria naturally present in kiwifruit orchards cannot be ruled out. Several bacterial isolates present as epiphytes in kiwifruit orchards were confirmed to be copper resistant or copper tolerant [30]: among them, at least two isolates, collected in kiwifruit orchards, have been demonstrated to be copper resistant and their ability to transfer such resistance to Psa has been studied [30]. The choice of a prospective antagonist to be used as biocontrol agent for the inhibition of the inoculum build up on plant organs, especially on leaves, should be done by carefully testing and confirming its susceptibility to low (<30 ppm) copper treatment rates, since the concentration of Cu++ sprayed in kiwifruit orchards during the chemical control of the bacterial canker implies the use of copper bactericides at a concentration starting from 30– 50 ppm [37, 38]. Altimira et al. [39] reported the presence of copper resistant bacteria and bacterial communities in agricultural soils with the presence of high copper pollutants, and kiwifruit orchards are notoriously sprayed with copper compounds several times a year, to control both phytopathogenic bacteria and some fungi [38, 40]. Therefore, the possibility of detecting copper resistance endophytes should not be excluded. In case, those copper resistant endophytes should not be taken into consideration, when selecting possible antagonists to be used against Psa during its endophytic phase.
Although the complete genetic basis of this feature is still unknown and is under investigation [30] and none of the endophytes collected in this work was copper resistant, a selective pressure might lead to the diffusion of copper resistance traits in the agricultural environment. Endophytes are not affected by antimicrobial sprays, including copper formulates; however, some of the strains isolated in this work belong to the soil saprophytic microflora, such as Pseudomonas synxantha or Kluyvera intermedia. These saprophytes may then colonise the rhizosphere of cultivated plants and enter into the plant tissues, starting their endophytic life and, in some cases, interact with the host plant physiology [26].
4.4Expression of antagonistic activity
The antagonistic activity of an endophyte against Psa showed differences among bacterial species and among isolates belonging to the same species. For instance, strain 65, strain 80 C and strain 93A, identified as Pseudomonas synxantha, or strain 61A and 62, both identified as Pantoea agglomerans, showed a difference in their inhibitory activity against Psa. Since all endophytes have been isolated from Actinidia chinensis, these differences in their inhibitory activity might be related to the geographical origin of the host plants. The two most important areas of kiwifruit cultivation in Italy are Lazio (Central Italy, South of Rome) and Romagna (North-eastern Italy), and our sampling activity was done in those regions. Those areas are characterized by a markedly different type of soil (early plyocene sedimentary and alluvial soils in Romagna, volcanic and alluvial in Central Italy). Indeed, the most active endophytes where obtained from kiwifruit plants grown in Lazio. To our knowledge, no study has been ever attempted to correlate the aggressiveness of possible bacterial antagonists (therefore their ability to compete with other microbes) with the type of agricultural soil, where such efficient bacteria are naturally present.
Our results showed that the inhibitory activity of each antagonist was not equally expressed at the same level for any pathogen considered: indeed, antagonism was unequal in intensity, sometimes with big differences. This might be explained in two ways: a single or a set of molecules produced by the antagonist may show a pronounced specificity against a target phytopathogen, or being of a more general efficacy [41]. Alternatively, single plant pathogenic bacterial strains might have different, less or more efficient mechanisms able to detoxify such antibacterial compounds [42]. Actually, mutual inhibition of competitors is widespread among bacteria [42], especially inside the family Enterobacteriaceae and Pseudomonadaceae [43]. A deeper insight into the functioning of such microbial communities in kiwifruit orchards will then greatly help in the choice of prospective endophytes, to be exploited in the development and implementation of novel, microbial pesticides able to control the bacterial canker of Actinidia spp.
5Conclusions
Our results on searching for prospective bacterial antagonists to control Psa were really satisfactory, when considering endophytes. Some additional experiments have been done to isolate and characterize epiphytes as possible antagonists, but no bacterial isolate belonging to the epiphytic microflora of kiwifruit orchards gave any satisfactory and conclusive result (data non shown). An initial study on the mechanism of antagonism for a few of those endophytes considered in this study indicates that they may produce a non-proteic metabolite, possibly a lipopeptide, which may act as a bactericide on a range of other bacteria, included Psa [30].
Our findings support the fact that selected endophytes may be regarded as very prospective biocontrol agents against Psa, particularly considering that such endophytes belong to the natural microbiota associated with Actinidia spp. So, they may be able to colonise and persist inside the tissues and the vascular system of kiwifruit plants. Finally, preliminary experiments done with GFP-tagged mutants of some of the above described antagonists confirm their ability to move inside the plant after their experimental inoculation, and efficiently colonise the leaves and the stem of actinidia seedlings (data not published).
Conflict of interest
The authors declare that they have no conflict of interest.
Acknowledgments
This study was funded in part by Centro Ricerche Produzioni Vegetali (CRPV), Cesena, Italy, under the project: “Ricerche sul PSA kiwi e PPV drupacee”. The authors kindly acknowledge the Regional Phytosanitary Service, Bologna, Regione Emilia Romagna. Research on endophytes was done in the framework of the EU COST FA1103 Action: “Endophytes in Biotechnology and Agriculture”.
References
[1] | Takikawa Y , Serizawa S , Ichikawa T . Pseudomonas syringae pv. actinidiae pv. nov.: The causal bacterium of canker of kiwifruit in Japan. Ann Phytopathol Soc Japan. (1989) ;55: :437–444. |
[2] | Ferrante P , Scortichini M . Identification of Pseudomonas syringae pv. actinidiae as causal agent of bacterial canker of yellow kiwifruit (Actinidia chinensis Planchon) in central Italy. J Phytopathol. (2009) ;157: :768–770. |
[3] | Stefani E , Giovanardi D . Dissemination of Pseudomonas syringae pv. actinidiae and its epiphytic life on leaves and fruits. Phytopath Medit. (2011) ;50: (3):501–505. |
[4] | Tontou R , Giovanardi D , Stefani E . Pollen as a possible pathway for the dissemination of Pseudomonas syringae pv. actinidiae and bacterial canker of kiwifruit. Phytopath Medit. (2014) ;53: (2):333–339. |
[5] | Balestra GM , Mazzaglia A , Quattrucci A , Renzi M , Rossetti A . Current status on bacterial canker spread on kiwifruit in Italy. Austral Plant Dis Notes. (2009) ;4: :34–36. |
[6] | Serizawa S , Ichikawa T , Takikawa Y , Tsuyumu S , Goto M . Occurrence of bacterial canker of kiwifruit in Japan: Description of symptoms, isolation of the pathogen and screening of bactericides. Ann Phytopathol Soc Japan. (1989) ;55: :427–436. |
[7] | Vanneste JL , Yu J , Cornish DA , et al. Identification, virulence, and distribution of two biovars of Pseudomonas syringae pv. actinidiae in New Zealand. Plant Dis. (2013) ;97: :708–719. |
[8] | European Union. Council Directive 2000/29/EC of 8 May 2000 on protective measures against the introduction into the Community of organisms harmful to plants and plant products and against their spread within the Community. OJ of the European Union. 2000; L 169 of 10 July 2000:1–112. |
[9] | European Union. Commission Implementing Decision of 5 December 2012 as regards measures to prevent the introduction into and the spread within the Union of Pseudomonas syringae pv. actinidiae Takikawa, Serizawa, Ichikawa, Tsuyumu & Goto. OJ of the European Union. 2012; L 335 of 7 December 2012:49–54. |
[10] | Andrews JH , Harris RF . The ecology and biogeography of microorganisms on plant surfaces. Ann Rev Phytopathol. (2000) ;38: :145–180. |
[11] | Lindow SE , Brandl MT . Microbiology of the phyllosphere. Appl Environ Microbiol. (2003) ;69: :1875–1883. |
[12] | Hartmann A , Schmid M , van Tuinen D , Berg G . Plant-driven selection of microbes. Plant Soil. (2009) ;321: :235–257. |
[13] | Berendsen RL , Pieterse CMJ , Bakker PAHM . The rhizosphere microbiome and plant health. Trends Plant Sci. (2012) ;17: :478–486. |
[14] | Gaiero RJ , McCall CA , Thompson KA , Day NJ , Best AS , Dunfield KE . Inside the root microbiome: Bacterial root endophytes and plant growth promotion. Am J Bot. (2013) ;100: (9):1738–1750. |
[15] | Duffy BK , Défago G . Environmental factors modulating antibiotic and siderophore biosynthesis by Pseudomonas fluorescens biocontrol strains. Appl Environ Microbiol. (1999) ;65: :2429–2438. |
[16] | Ramesh R , Joshi A , Hanekar MPG . Pseudomonads: Major antagonistic endophytic bacteria to suppress bacterial wilt pathogen, Ralstonia solanacearum in the eggplant (Solanum melongena L.). World J Microbiol Biotech. (2008) ;25: :47–55. |
[17] | Valente M , Ortugno C , Tosi L , Scannavini M , Pelliconi F , Fagioli L , Scortichini M , Vittone G , Fiorillo E , Pradolesi G , Donati G . BION® 50WG (Acybenziolar- S-methyl), systemic acquired resistance inducer: Efficacy in field against bacterial kiwifruit vine disease, caused by Pseudomonas syringae pv. actinidiae. Atti Giornate Fitopatologiche. (2014) ;2: :147–156 (In Italian). |
[18] | Versalovic J , Schneider M , DeBruijn FJ , Lupski JR . Genomic fingerprinting of bacteria using repetitive sequence based PCR (rep-PCR). Meth Mol Cell Biol. (1994) ;5: :25–40. |
[19] | Lindow SE , Arny DC , Upper CD . Bacterial ice nucleation. A factor in frost injury to plants. Plant Physiol. (1982) ;70: :1084–1089. |
[20] | Wada A , Kono M , Kawauchi S , Takagi Y , Morikawa T , Funakoshi K . Rapid discrimination of Gram-positive and Gram-negative bacteria in liquid samples by using NaOH-sodium dodecylsulphate solution and flow cytometry. PLOS one. (2012) ;7: (10):1–10. |
[21] | Rees-George J , Vanneste J , Cornish DA , Pushparajah IPS , Yu J , Templeton MD , Everett KR . Detection of Pseudomonas syringae pv. actinidiae using polymerase chain reaction (PCR) primers based on the 16S-23 S r DNA intertrascribed spacer region and comparison with PCR primers based on other gene regions. Plant Pathol. (2010) ;59: :453–464. |
[22] | Klement Z , Farkas GL , Lovrekovich L . Hypersensitive reaction induced by phytopathogenic bacteria in the tobacco leaf. Phytopathology. (1964) ;54: :474–477. |
[23] | Braun-Kiewnick A , Sands DC . Pseudomonas. In: Schaad NW, Jones JB and Chun W, editors. Laboratory Guide for Identification of Plant Pathogenic Bacteria, 3rd edition. St. Paul, APS Press; (2001) , p. 84–120. |
[24] | Weyent RS , Moss CW , Weaver RE , Hollis DG , Jordan JG , Cook EC , Daneshvar MI . Bacterial identification by cellular fatty acid analysis. In: Identification of unusual Gram-negative aerobic and facultatively anaerobic bacteria, 2nd ed. Baltimore, Williams & Wilkins. (1966) ; pp. 565–721. |
[25] | Kolbert CP , Persing DH . Ribosomal RNA sequencing as a tool for identification of bacterial pathogens. Curr Opin Microbiol. (1999) ;2: (3):299–305. |
[26] | Rosenblueth M , Martinez-Romero E. Bacterial endophytes and their interaction with hosts. MPMI. (2006) ;19: (8):827–837. |
[27] | Bacon CW , Hinton DM . Bacterial endophytes: The endophytic niche, its occupants, and its utility. In: Gnanamanickam SS, editor. Plant Associated Bacteria. Dordrecht, Springer; (2007) ; pp. 155–194. |
[28] | Lugtenberg BJJ , Dekkers LC . What makes Pseudomonas bacteria rhizosphere competent? Environ Microbiol. (1999) ;1: (1):9–13. |
[29] | Surhone LM , Tennoe MT , Henssonow SF . Pseudomonas synxantha. Saarbrücken, Germany; Betascript Publishing; (2011) . |
[30] | Tontou R , Gaggia F , Baffoni L , Devescovi G , Venturi V , Giovanardi D , Stefani E . Molecular identification of endophyte showing a strong antagonistic activity against Pseudomonas syringae pv. actinidiae. Plant Soil. (2015) . DOI: 10.1007/s11104-015-2624-0. |
[31] | Gnanamanickam SS , Immanuel JE, Epiphytic bacteria: Their ecology and functions. In: Gnanamanickam SS editor. Plant Associated Bacteria. Dordrecht, Springer; (2007) , pp. 131–53. |
[32] | Wolber PK . Bacterial ice nucleation. Adv Microbiol Physiol. (1992) ;34: :205–237. |
[33] | Kennelly MM , Cazorla FM , de Vicente A , Ramos C , Sundin GW . Pseudomonas syringae diseases of fruit trees. Progress towards understanding and control. Plant Dis. (2007) ;91: (1):4–17. |
[34] | Ferrante P , Fiorillo E , Marcelletti S , Marocchi F , Mastroleo M , Simeoni S , Scortichini M . The importance of the main colonisation and penetration sites of Pseudomonas syringae pv. actinidiae and prevailing weather conditions in the development of epidemics in yellow kiwifruit, recently observed in central Italy. J Plant Pathol. (2012) ;94: (2):455–461. |
[35] | Nakajima M , Goto M , Hibi T . Similarity between copper resistance genes from Pseudomonas syringae pv. actinidiae and P. syringae pv. tomato. J Gen Plant Pathol. (2002) ;68: :68–74. |
[36] | Marcelletti M , Ferrante P , Petriccione M , Firrao G , Scortichini M . Pseudomonas syringae pv. actinidiae draft genomes comparison reveal strain-specific features involved in adaptation and virulence to Actinidia species. PLoS One. (2011) . DOI: 10.1371/journal.pone.0027297 |
[37] | Gaskin RE , Horgan DB , Steele KD , van Leeuwen RM . The effect of rainfall on copper spray residues on kiwifruit foliage, fruit and canes. NZ Plant Protection. (2013) ;66: :199–203. |
[38] | Monchiero M , Gullino ML , Pugliese M , Spadaro D , Garibaldi A . Efficacy of different chemical and biological products in the control of Pseudomonas syringae pv. actinidiae on kiwifruit. Australasian Plant Pathol. (2014) . DOI: 10.1007/s13313-014-0328-1. |
[39] | Altimira F , Yanez C , Bravo G , Gonzalez M , Rojas LA , Seeger M . Characterisation of copper-resistance bacteria and bacterial communities from copper-polluted agricultural soils in Chile. BMC Microbiology. (2012) ;12: :193. doi: 10.1186/1471-2180-12-193. |
[40] | Fratarcangeli L , Rossetti A , Mazzagia A , Balestra GM . Il ruolo del rame nella lotta al cancro batterico del kiwi. L’Informatore Agrario. (2010) ;8: :52–56. |
[41] | Gross DC , Vidaver AK . Bacteriocins. In: Klement Z, Rudolph K, Sands DC, editors.Methods in Phytobacteriology, Budapest, Akademiai Kiado. (1990) ; pp. 245–249. |
[42] | Hibbing ME , Fuqua C , Parsek MR , Peterson SB . Bacterial competition: Surviving and thriving in the microbial jungle. Nat Rev Microbiol. (2010) ;8: (1):15–25. |
[43] | Tait K , Sutherland IW. Antagonistic interactions amongst bacteriocin-producing enteric bacteria in dual species biofilms. J Appl Microbiol. (2002) ;93: :345–352. |
Figures and Tables
Fig.1
Position of cut disks (approx. 1-2 cm thick) from kiwifruit plants for the isolation of endophytes. One disk was cut below the grafting point, two disks at the base of the main cordons, other disks were taken along the trunk.
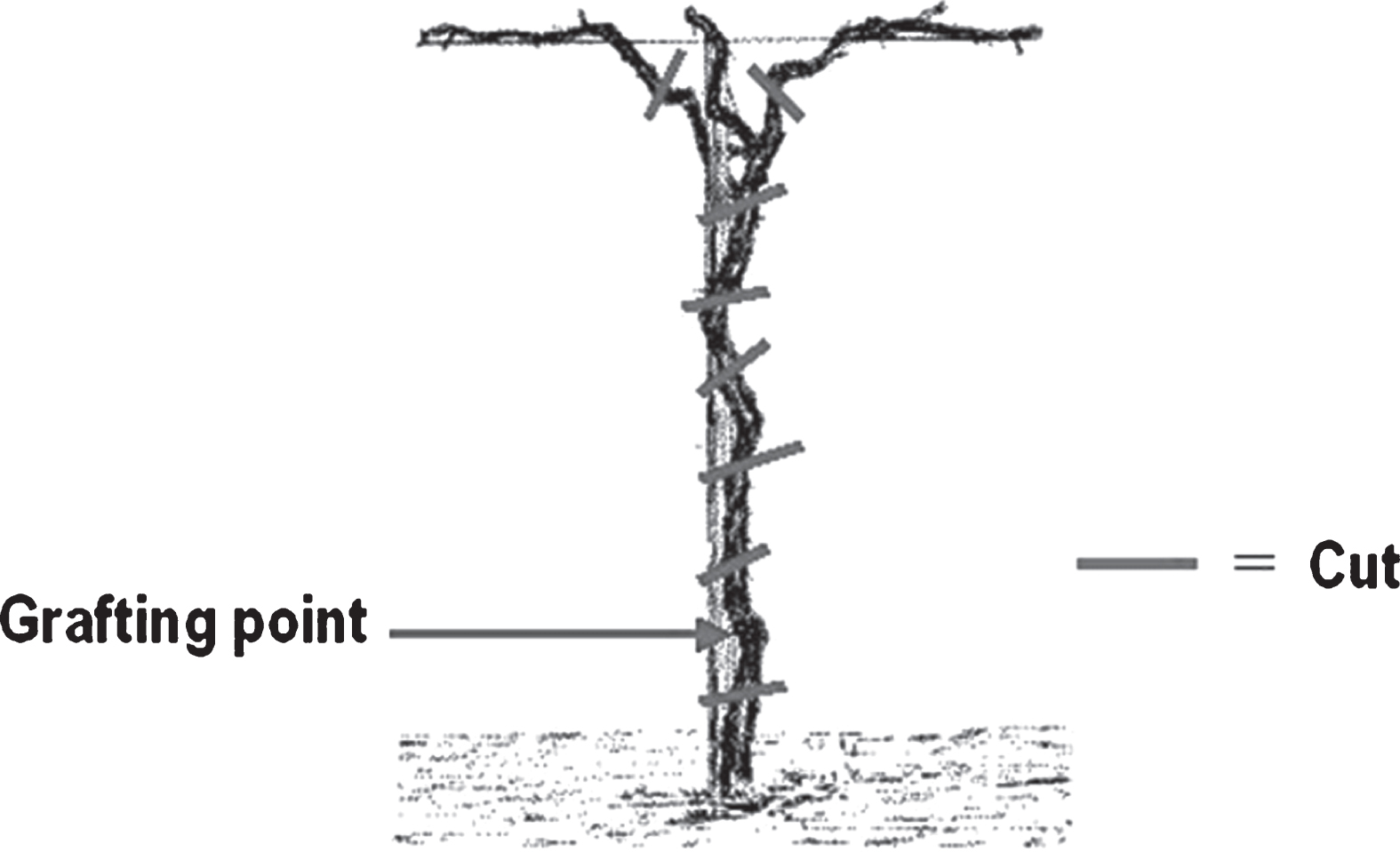
Fig.2
Detection of Pseudomonas syringae pv. actinidiae in Actinidia chinensis along the trunk, from the permanent cordon to the grafting point. The 12 trees were sampled in one orchard located in Lazio and presence of Psa was checked along the trunk every 20 cm. Incidence is referring to percentage of samples positive for Psa presence at different height on total plants analysed. No Psa was ever detected below the grafting point.
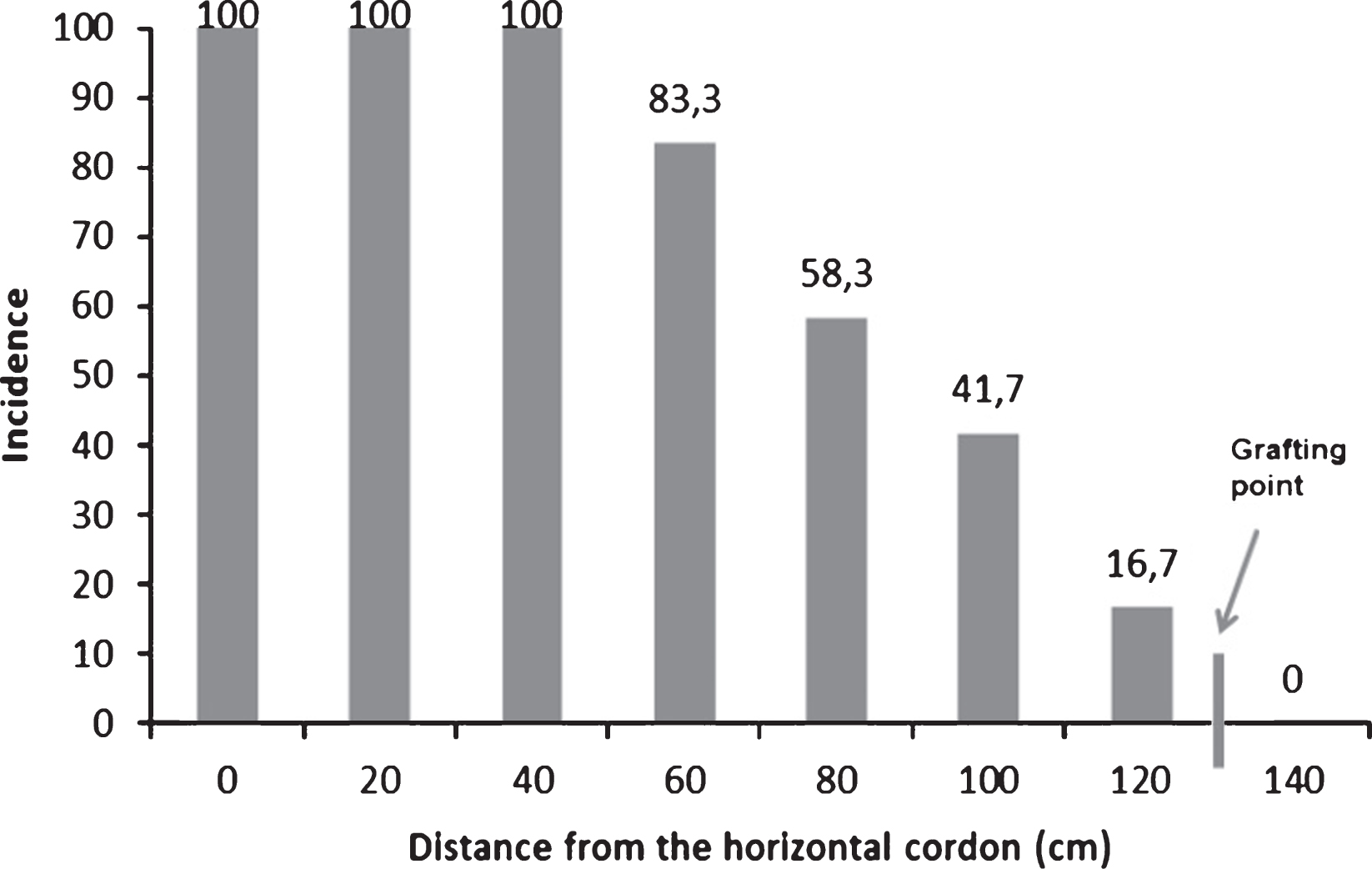
Fig.3
Comparison of electrophoretic patterns obtained after PCR amplification using the BOX primer (BOX-PCR) for different bacterial endophytes showing a strong antagonistic activity against Pseudomonas syringae pv. actinidiae. In the first and in the last lanes the 100 bp DNA ladder; lanes 1, 2 and 6: Pseudomonas fluorescens; lanes 3 and 4: Pantoea agglomerans; lane 5: Pseudomonas mendocina; lanes 7, 11 and 14 Pseudomonas synxantha; lane 8 and 13: Enterobacter spp.; lanes 9 and 15: Pseudomonas putida biotype A; lane 10 and 12: Kluyvera spp.
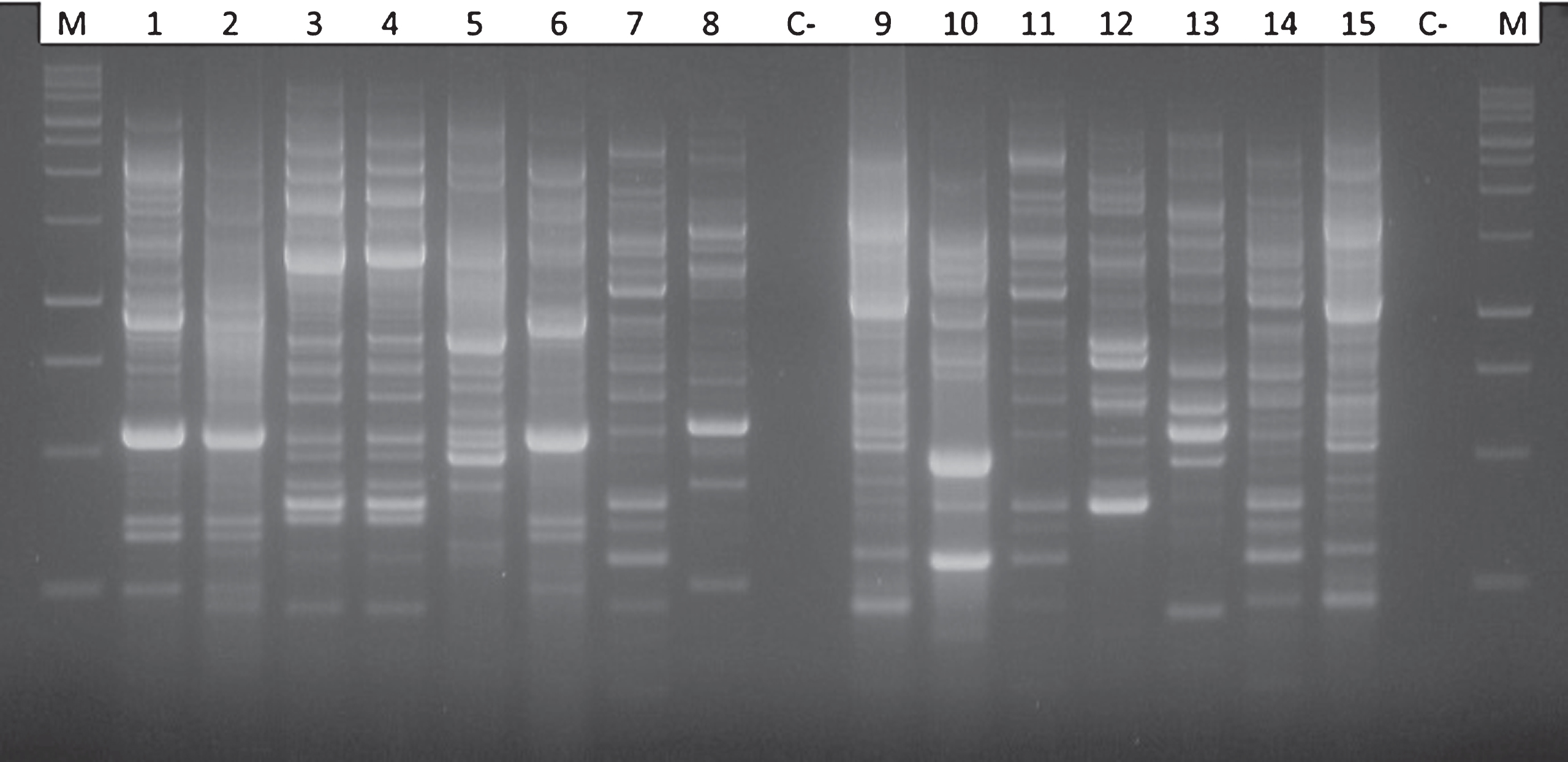
Fig.4
Inhibition halo developed around a macrocolony of Pseudomonas synxantha in a plate inoculated with the phytopathogenic bacterium Pseudomonas syringae pv. actinidiae. The inhibition halo was calculated as the result of following equation: AIA = (R2*3.14) – (r2*3.14).
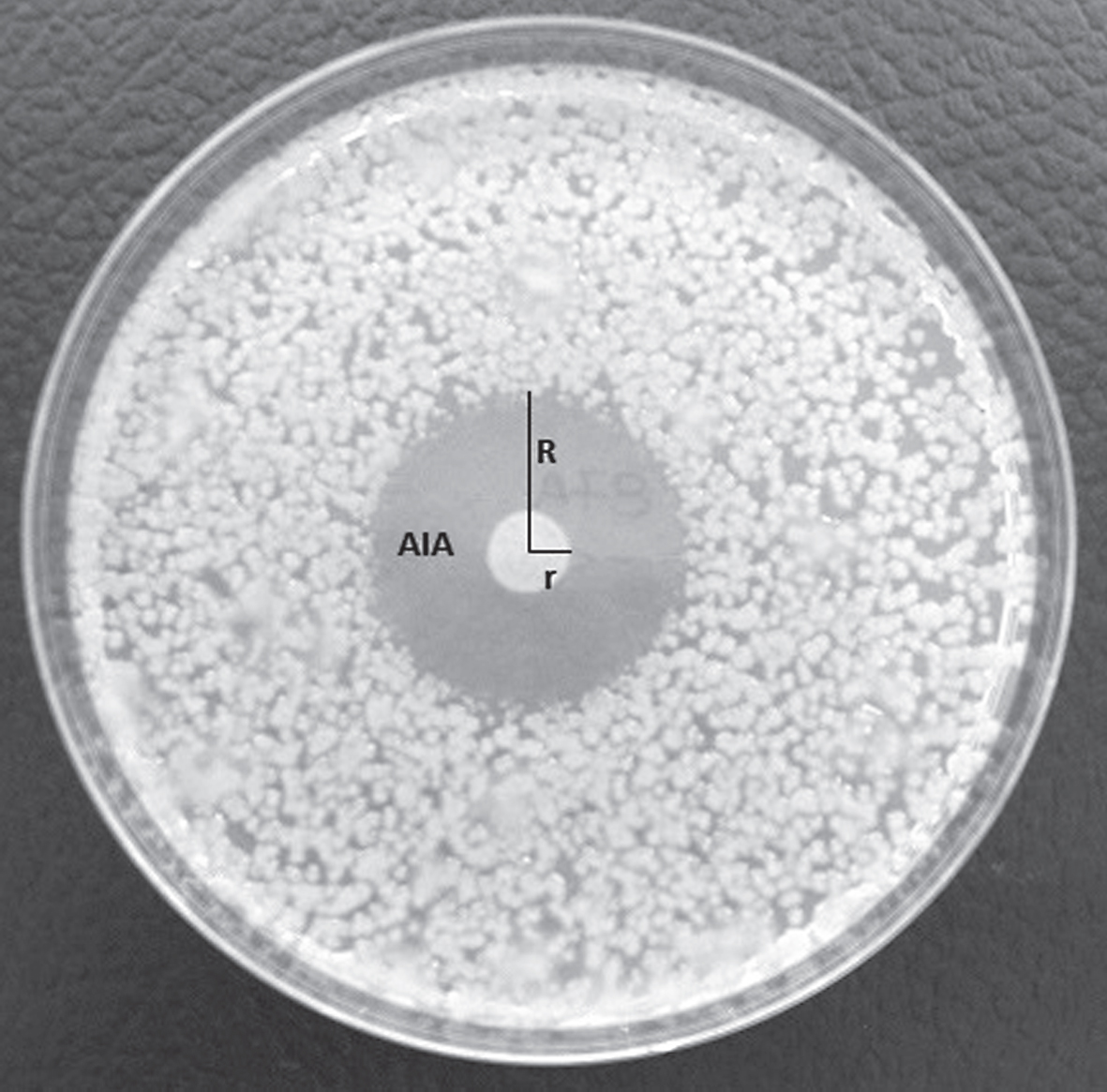
Table 1
Antagonistic activity in vitro of 14 selected endophytes from Actinidia spp. against Pseudomonas syringae pv. actinidiae and other six important phytopathogenic bacteria. The antagonistic activity is expressed as an index, related to Average Inhibition Area (AIA) around the colony calculated for three independent replicates: 0 = no inhibition; 1 = inhibition halo up to 100 mm2; 2 = inhibition halo up to 400 mm2; 3 = inhibition halo larger than 400 mm2
Antagonistic isolate Nr. | Phytopathogenic bacteria assayed | ||||||
Pseudomonas | Erwinia | Xanthomonas | Xanthomonas | Clavibacter | Clavibacter | Clavibacter | |
syringae pv. | amylovora | arboricola | arboricola | michiganensis | michiganensis | michiganensis | |
actinidiae | pv. pruni | pv. juglandis | subsp. | subsp. | subsp. | ||
michiganensis | insidiosus | sepedonicus | |||||
57A | 3 | 0 | 0 | 0 | 2 | 2 | 0 |
60 | 3 | 0 | 0 | 0 | 2 | 3 | 1 |
61A | 1 | 1 | 0 | 1 | 2 | 3 | 0 |
62 | 2 | 0 | 0 | 0 | 3 | 0 | 0 |
63 | 2 | 2 | 2 | 3 | 3 | 2 | 1 |
64 | 1 | 0 | 0 | 1 | 0 | 0 | 0 |
65 | 3 | 2 | 2 | 3 | 0 | 2 | 1 |
72C | 2 | 0 | 2 | 2 | 3 | 3 | 0 |
73B | 0 | 0 | 0 | 2 | 0 | 1 | 0 |
75B | 2 | 0 | 1 | 1 | 3 | 0 | 2 |
80C | 3 | 0 | 0 | 0 | 0 | 0 | 0 |
87A | 3 | 2 | 2 | 3 | 3 | 3 | 3 |
89C | 2 | 1 | 1 | 2 | 0 | 1 | 0 |
93A | 1 | 2 | 3 | 2 | 3 | 3 | 2 |
94A | 3 | 2 | 3 | 3 | 3 | 2 | 2 |
Table 2
Average inhibition area, calculated on agar plates, produced by a set of endophytic bacteria, isolated from Actinidia chinensis and showing an inhibitory effect on Pseudomonas syringae pv. actinidiae. The inhibition halo was calculated as the result of following equation: AIA = (R2*3.14) – (r2*3.14). Areas labeled with different letters are significantly different (P < 0.05, according to one-way ANOVA and T-test)
DLS collection number | Endophyte species | Average Inhibition Area (mm2±12) |
61A | Pantoea agglomerans | 53A |
62 | Pantoea agglomerans | 139B |
63 | Pseudomonas mendocina | 360BC |
64 | Pseudomonas fluorescens | 53A |
65 | Pseudomonas synxantha | 979D |
72C | Enterobacter sp. | 271B |
75B | Kluyvera intermedia | 263B |
80C | Pseudomonas synxantha | 527C |
87A | Kluyvera intermedia | 523C |
89C | Enterobacter sp. | 109B |
94A | Pseudomonas putida, biotype A | 688C |