Effect of Multimodal Intervention in Individuals with Mild Cognitive Impairment: A Randomized Clinical Trial in Shanghai
Abstract
Background:
Previous trials have indicated that multimodal training could improve cognitive functions and moods in individuals with mild cognitive impairment (MCI). However, evidence was mainly obtained from studies in high-income countries.
Objective:
This trial aims to investigate the efficacy, safety, and potential mechanism of a multimodal intervention on cognitive function in individuals with MCI living in a community.
Methods:
In this single-blind, randomized controlled trial, 120 participants with MCI were randomly assigned to either the intervention group or the control group. The intervention group received the multimodal intervention, while the control group received regular health education. Neuropsychological tests and magnetic resonance imaging (MRI) were conducted at baseline and after the 12-week intervention.
Results:
Fifty-nine and fifty-seven participants respectively in the intervention and control groups completed the trial. The intervention group shown improvements in primary outcome, Mini-Mental State Exam (MMSE) total score (mean difference –0.96, 95% CI [–1.58, –0.34], p = 0.003), and secondary outcomes: MMSE recall (–0.39, 95% CI [–0.71, –0.07], p = 0.019), MMSE language (–0.26, 95% CI [–0.44, –0.07], p = 0.007), Auditory Verbal Learning Test instantaneous memory (–3.30, 95% CI [–5.70, –0.89], p = 0.008), Digit Symbol Substitution Test total score (–2.91, 95% CI [–5.67, –0.15], p = 0.039), digit span forwards (–1.25, 95% CI [–1.93, –0.56], p < 0.001), and Digit Span Test (–1.33, 95% CI [–2.33, –0.34], p = 0.009) compared to the control group. Improvements were observed in structural and functional connectivity related to language, concentration, executive function, memory, and recall functioning via MRI in the intervention group.
Conclusions:
The multimodal intervention improved cognitive function in individuals with MCI in cognitive performance and neuroimaging.
INTRODUCTION
The 2022 Revision of World Population Prospects estimated that the percentage of people aged 60 or older in the world population would be over 22% by 2050.1 As the population aging, the number of individuals with dementia is also increasing. Dementia is a neurological disorder encompassing a varies of symptoms and is the leading cause of disability among people over 65 years.2 This condition poses substantial burdens on families, society, and the economy. In fact, the financial burden of dementia is comparable to that of heart disease and cancer.3 Mild cognitive impairment (MCI) is an intermediate state between normal aging and dementia.4 The prevalence of MCI in adults older than 60 years is about 20%, and approximately 5–17% of MCI may progress to dementia annually.5
To date, pharmacologic therapeutics for dementia have shown limited efficacy,6 then non-pharmacological interventions in delaying or preventing the conversion from MCI to dementia have been emerged in several previous studies.7 Non-pharmacological interventions mainly included cognition-oriented intervention,8 exercise intervention,9 and mind and body practice.10 The multimodal non-pharmacological intervention program with two or more types of interventions has been recommended for treating people with dementia.11 Previous trials have indicated that multimodal training could improve cognitive functions and moods in individuals with MCI.12,13 However, these effective interventions are not yet widely deployed, and research evidence was mainly obtained from studies in high-income countries. There is a need to verify their study results in different populations with diverse culture and economic background.
Here we report a randomized controlled trial aiming to investigate the efficacy, safety, and potential mechanism of a multimodal intervention on cognitive function in individuals with MCI living in a community.
MATERIALS AND METHODS
Study design
This single-blind, randomized controlled trial was conducted in urban Shanghai, China, from July 2019 to December 2021. This study was approved by the Ethics Committee, School of Public Health, Fudan University (IRB # 2020-07-0840). The written informed consent was obtained from each participant. Trial registration: ClinicalTrials.govNCT05483790. Trial registration date: July 24, 2022.
Study participants
Study participants were recruited from the community residents living in Xinzhuang Town, Minhang District, Shanghai. The inclusion criteria included 1) aged ≥50 and ≤75 years; 2) with at least 6 years of education; 3) with a diagnosis of MCI according to the Peterson criteria:14 1) cognitive concern or complaint by the subject or the proxy, with Clinical Dementia Rating (CDR) = 0.5; 2) objective impairment in at least 1 cognitive domain based on performance 1.5 SD below the mean using the norms of Chinese population;15 3) essentially normal functional activities (determined from CDR and Activity of Daily Living (ADL); and 4) absence of dementia (by DSM-IV). The exclusion criteria included: 1) diagnosed neurological diseases causing cognitive decline, i.e., cerebrovascular disease, central nervous system infections, etc.; 2) diagnosed central nervous system diseases, i.e., brain tumor, epilepsy; 3) diagnosed psychiatric disorder; 4) severe disorders which may affect the safety, i.e., severe cardiac, renal, lung, liver or other diseases; 5) with problems of vision, hearing, or speaking, and were not able to cooperate the evaluations; 6) using pharmacologic therapeutics for cognitive impairment; 7) concurrent participation in other intervention trials.
The eligible participants completed questionnaires to collect demographic and lifestyle characteristics (e.g., age, sex, education level, ethnicity, marital status, weight, and height, drug history, daily activities, smoking habits, alcohol consumption, medical disorders, family history of dementia, etc.) at the baseline.
Randomization and blinding
Participants were randomly assigned by a 1:1 ratio to the intervention group and the control group. The randomization method was conducted by a biostatistician at Fudan University School of Public Health using an online program (http://www.graphpad.com/quickcalcs/index.cfm). A local health worker enrolled participants and assigned them to the two groups based on the randomization. Outcomes were assessed by investigators who were not involved in the intervention activities and were blinded to the group assignment.
Trial procedure
The trial is conducted by local health workers, who were trained by a team comprising neurologists and geriatrics experts from Huashan Hospital. The training manuals, demo videos, education resources (such as PowerPoint files and materials) and standardized health education study materials were also distributed to them.
As shown in Fig. 1, a multimodal intervention program (with schedule) was conducted in the intervention group for 12 weeks, including cognitive training, physical exercise, healthy lifestyle education, and computerized interaction training. Throughout the 12-week period, the control group only received 30-min health education through booklets, home visits, or phone calls once every week. The multimodal intervention program included the in-person training course (90-min, once per week for 6 weeks), computerized interaction training (30-min, 3 times per week for 6 weeks), and homework (30-min, every day for 12 weeks).
Fig. 1
Interventions in the intervention and the control group.
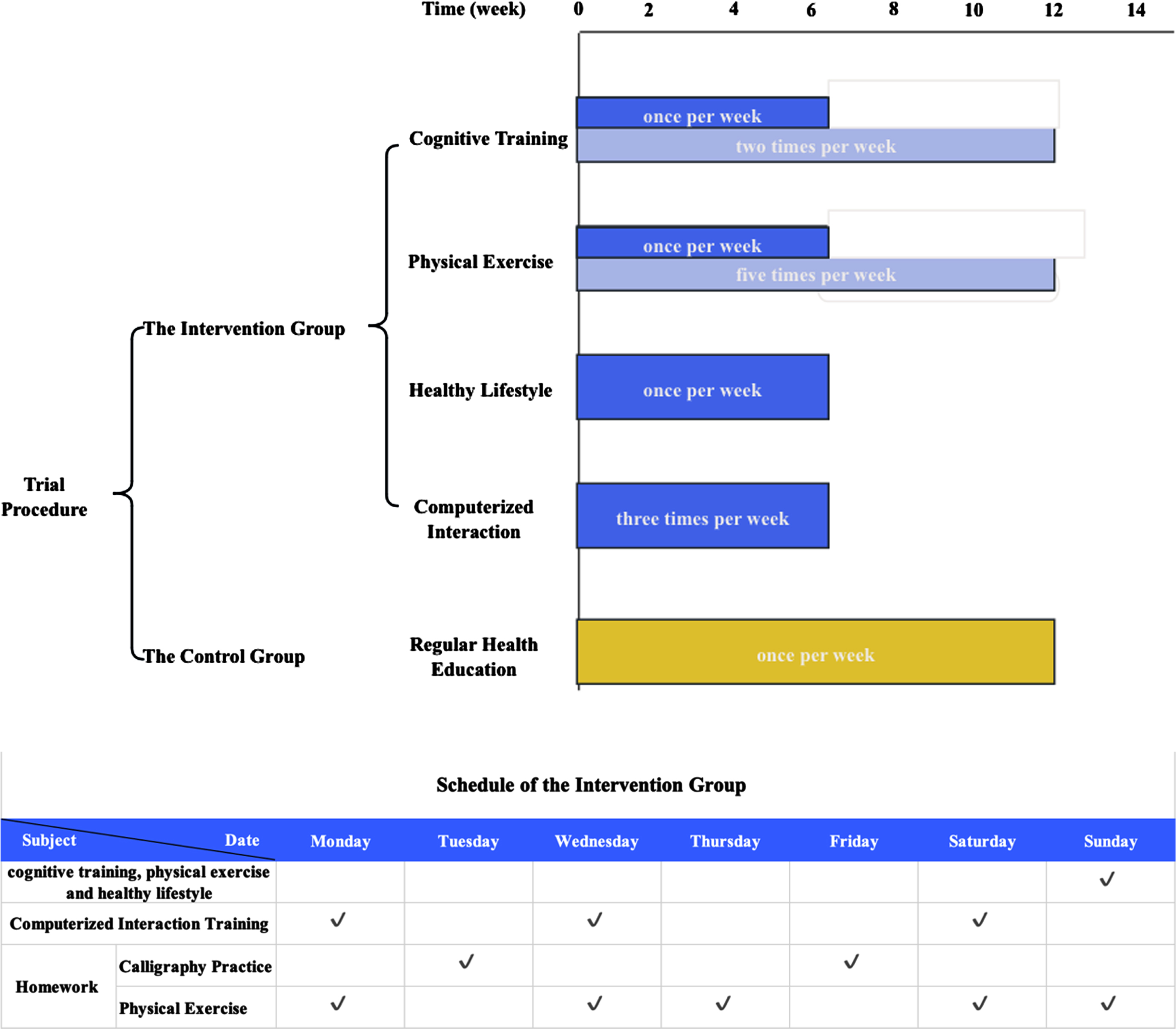
The in-person training course started with a 10-min period of reminiscence and discussion. Subsequently, cognitive training activities were conducted for 25 min, followed by a 15-min healthy lifestyle education. After a 10-min break, physical exercise training was administered for 15 min. Finally, participants summarized the all-training activities, either through writing or discussion, and planed their homework during a 15-min wrap-up session.
The cognitive training encompassed memory-related training (i.e., picture recall, categorization, cueing, repetition, association, sentence formation, story construction, utilization of the Roman room method, face-name association, and verbalization to aid retention) and attention-related training (i.e., character-picture association, idiom-picture association, and spot-the-difference tasks were incorporated). The physical exercise included finger exercises, elastic band exercises, Tai Chi/Baduanjin with guidance. The healthy lifestyle education included guidance on nutrition, sleep, stress management, and mood adjustment by teaching problem-solving skills, such as managing stressful life events and handling anger and frustration. Additionally, the intervention provided information and support to facilitate lifestyle changes and included discussions and practical exercises, such as tools for assessing dietary behavior.
The instructor-supervised, computer program-based computerized interaction training three times weekly included spatial cognition (i.e., participants manipulated identical cubes or two-dimensional figures in different orientations using a control handle), left and right brain balance tasks (i.e., replicating graphics from one computer screen to another using action tracking or screen touching technology), clock imitation exercises (i.e., participants mimicked the movements of hour and minute hands to match the time displayed on the computer screen), and automatic teller machine simulation (i.e., wherein participants performed transactions like cash withdrawals, money transfers, and balance checks using a computer interface similar to an ATM, while also recalling passwords and transaction amounts.
The every-day homework encompassed activities such as calligraphy practice (2 days per week) and physical exercise (the other 5 days per week). Participants were asked to complete their homework within approximately 30 min and share pictures or videos of their homework in a WeChat group each day, allowing for assessment of their adherence.
The quality control of the trial was conducted through regular supervision calls to confirm the adherence to the trial protocol. Participants who attended at least 80% of the activities were considered as good adherence.
Assessment of cognitive function
Cognitive functions were evaluated by neuropsychological tests at the baseline and right after the 12-week program finished. The Mini-Mental State Examination (MMSE) assesses various domains including orientation, instantaneous memory, attention, computation, recall, language and visuospatial.16 The Montreal Cognitive Assessment (MoCA) measured cognitive domains of visual perception, executive skills, language, attention, memory, and orientation.17 The Auditory Verbal Learning Test (AVLT) and the Logic Memory Test (LMT) reflected the memory function, the Trail Marking Test A (TMT-A)18 and the Digit Symbol Substitution Test (DSST) reflected the executive function, and the Digit Span Test (DST) reflected the concentration function.19
The primary outcome of this trial was the change in MMSE total score from baseline to the end of the 12-week program. Secondary outcomes included the changes of MMSE sub- scores, MoCA, AVLT, LMT, TMT-A, DSST, DST from baseline to the end of the 12-week program.
Assessment of safety
Adverse events were monitored throughout the study. At each session, trained workers asked participants and their caregivers if any adverse events had occurred since the last session. They also observed events during the sessions and inquired about adverse events during follow-up visits. Severe adverse events were defined as those resulting in death, persistent or significant disability or incapacity, immediate life-threatening situations, or requiring hospital admission or medical intervention to prevent any of these severe adverse events.
MRI acquisition
The magnetic resonance imaging (MRI) scan was administered at baseline and right after the 12-week program. The brain MRI protocols included the acquisition of 3D T1-weighted structural images, resting functional MRI (rfMRI) images, and diffusion-weighted imaging (DWI).
We used an MR-790 3T scanner (Unite Imaging Ltd, Shanghai, China) with a 32-channel head coil at the MR Lab of the Neurosurgery Department of Huashan Hospital, Fudan University. The parameters for the acquisition of T1 weighted images were as follows: a 3D fast spoil gradient echo sequence with TR/TE/TI = 8.1 ms/3.4 ms/1060 ms, flip angle = 8, and water excitation to suppress the fat of scalp and orbit, field of view (FOV) = 256×240 mm, matrix size = 320×300, 208 sagittal slices, voxel size = 0.8×0.8×0.8 mm, acceleration factor = 2 (parallel imaging), scan time = 6 min 39 s.
Both rfMRI and DWI were acquired using a single shot echo planner imaging sequence. The parameters of rfMRI: TR/TE = 700 ms/33 ms, FA = 52, echo spacing = 0.55 ms, BW = 2350 Hz/Pixel, FOV = 210×210 mm, matrix size = 84×84, 63 axial slices, voxel size = 2.5×2.5×2.5 mm, multiband factor = 7, phase encoding = PA (posterior - anterior), number of volumes = 700, scan time = 8 min 22 s. A double-echo gradient echo field map was acquired for correct the distortion from susceptibility difference: TR = 700 ms, TEs = 4.92, 7.38 ms, bandwidth = 800 Hz/Pixel, FA = 10, the geometric parameters were as same as the rfMRI’s, scan time = 58 s. The parameters of DWI were: TR/TE = 5640/82 ms, bandwidth = 2000 Hz/pixel, FOV = 210×210 mm, matrix size = 140*140, 99 axial slices, voxel size = 1.5×1.5×1.5 mm, phase encoding = PA, multi-shell, directions = 32, 64, b values = 1000, 2000 s/mm2, number of b0 = 2, scan time = 10 min 59 s. In addition, two other b0 images with an anterior-posterior phase encoding direction were acquired to correct susceptibility distortion.
For the T1 MRI images, the CAT12 toolbox was initially used to perform quality control and exclude subjects with quality scores of “C+” or lower. Then, the recon-all command of FreeSurfer 6.0.0 was utilized for brain extraction, tissue segmentation, cortical reconstruction, and brain region labeling for each subject’s brain images. The image processing steps involved skull stripping, normalization, removal of the non-brain structure, brain tissue segmentation, and surface reconstruction. The brain images of each subject were nonlinearly aligned to the MNI152 template using the ANTs alignment toolkit for subsequent analysis of BOLD fMRI and DWI images. For rfMRI images, the AFNI toolkit was primarily used for processing. The first 5 time points of the rfMRI data were discarded. Head motion correction was performed, and assessment metrics measuring the degree of head rotation and displacement in all three directions were generated. Second step, the images of each subject were corrected for temporal layers, the images of the intermediate time points were aligned with the T1-weighted images, and head movement outlier time points in the temporal dimension were detected and interpolated using adjacent time points. The images were temporally corrected and aligned with the T1-weighted images. Head movement outlier time points were detected and interpolated. Regression analysis was conducted to remove the mean signal of white matter and ventricle, as well as head movement parameters as noise regressors. The data were band-pass filtered between 0.01 and 0.1 Hz. The whole-brain mean BOLD signal was adjusted, and the images were aligned to the MNI152 space using the alignment results of the T1-weighted images. Functional connectivity between all brain regions were calculated based on the DK+Aseg partitioning template. DWI images were processed using the FSL toolkit. The b0 images were aligned with the T1-weighted images, and corrections were applied for eddy currents and head motion. Outliers were detected and replaced. Probabilistic fiber tracking of DWI images was performed using the DK+Aseg parcellation template, resulting in a probabilistic white matter connectivity matrix for each brain region. Graph-theoretical metrics were extracted from the connectivity matrix to evaluate brain regions related to language, concentration, executive, memory, and recall functioning networks.
Statistical analysis
The sample size was determined to allow to detect a significant statistical difference based on the 90% statistical power from a calculation formula. This trial was designed to provide greater than to detect a 1-point increase in MMSE as significant at the two-sided α value of 0.05, and an estimate of the standard deviation of 1.6 for MMSE scores.20 Considering a 10% dropout rate, sixty participants each were required for the intervention group and the control group.
We conducted analyses using intention to treat principles. The student-t test was used to compare changes of neuropsychological test scores and MRI parameters. MRI parameters (additional corrections for multiple testing, false discovery rate) between the intervention and control groups. Changes were calculated as the scores minus the baseline scores. The spearman correlation test was utilized to analyze associations among changes in neuropsychological and MRI parameters in both groups by adjusting age and sex.
The statistical analyses were conducted using Stata software version 15.0, and a significance level of p < 0.05 was considered statistically significant.
RESULTS
The flowchart of participants enrollment is shown in Fig. 2. Among 120 randomized participants, 59 participants in the intervention group and 57 participants in the control group completed the trial and the assessment of the cognitive function and safety. Thirty-one participants (52.5%) and 30 participants (52.6%) in the intervention group and control group completed the MRI scan. Table 1 shows the demographic characteristics of the intervention group and the control group at baseline. Participants in the intervention and control groups were all Chinese. There’s no statistical significance of the baseline characteristics between the intervention group and control group.
Fig. 2
Flow of participants through the study.
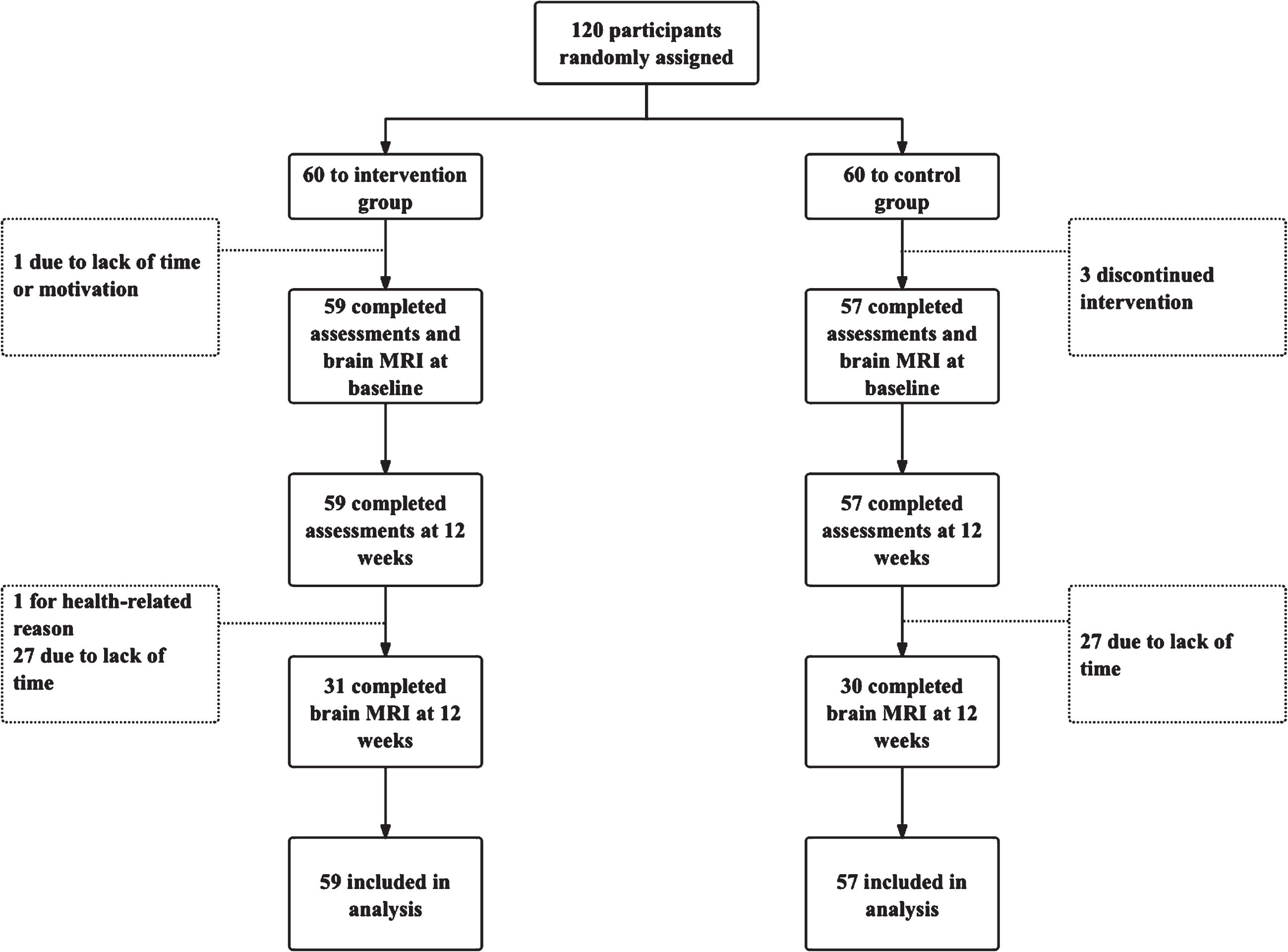
Table 1
Baseline characteristics of participants
Intervention group | Control group | |
(n = 59) | (n = 57) | |
Age, y, mean (SD) | 66.3 (4.4) | 67.8 (5.1) |
Sex | ||
Female, n (%) | 36 (61.0) | 35 (61.4) |
Male, n (%) | 23 (39.0) | 22 (38.6) |
Years of education | ||
<12 y, n (%) | 7 (11.9) | 7 (12.3) |
≥12 y, n (%) | 52 (88.1) | 50 (87.7) |
Marital status | ||
Married/cohabiting, n% | 55 (93.2) | 52 (91.2) |
Single, n% | 4 (6.8) | 5 (8.8) |
Lifestyle factors | ||
Body-mass index, Kg/m2, mean (SD) | 25.0 (3.3) | 24.1 (2.8) |
Current smokers, n (%) | 6 (10.2) | 10 (17.5) |
Current alcohol drinking, n (%) | 18 (30.5) | 9 (15.8) |
Medical disorders, n (%) | ||
Hypertension, n (%) | 25 (42.4) | 23 (40.4) |
Diabetes, n (%) | 13 (22.0) | 12 (21.1) |
Hypercholesterolemia, n (%) | 16 (27.1) | 14 (24.6) |
History of myocardial infarction, n (%) | 0 (0) | 1 (1.8) |
History of stroke, n (%) | 2 (3.4) | 0 (0) |
Family history of dementia, n (%) | 2 (3.4) | 6 (10.5) |
As shown in Table 2 and Fig. 3, significant improvements of cognitive functions were shown in the intervention group after the 12-week intervention. Compared to the control group, improvements were observed in MMSE total score (mean difference –0.96, 95% CI [–1.58, –0.34], p = 0.003), MMSE recall (–0.39, 95% CI [–0.71, –0.07], p = 0.019), MMSE language (–0.26, 95% CI [–0.44, –0.07], p = 0.007), AVLT instantaneous memory (–3.30, 95% CI [–5.70, –0.89], p = 0.008), DSST total score (–2.91, 95% CI [–5.67, –0.15], p = 0.039), digit span forwards (–1.25, 95% CI [–1.93, –0.56], p < 0.001) and DST (–1.33, 95% CI [–2.33, –0.34], p = 0.009) compared to the control group. No adverse events related to the intervention were reported from the participants.
Table 2
Main estimates of intervention effect (cognitive function)
Intervention group (N = 59) | Control group (N = 57) | |||||||
Outcomes | Baseline | 12-week | Change from baseline | Baseline | 12-week | Change from baseline | Difference of changes between 2 groups [95% CI] | p |
MMSE | ||||||||
Total MMSE score | 28.4 (1.6) | 28.4 (1.5) | 0.7 (1.5) | 29.1 (1.0) | 28.2 (1.7) | –0.3 (1.9) | –0.96 [–1.58, –0.34] | 0.003 |
Orientation | 9.9 (0.2) | 9.8 (0.6) | 0.0 (0.3) | 9.9 (0.2) | 9.9 (0.4) | 0.1 (0.7) | 0.07 [–0.12, 0.26] | 0.461 |
Registration | 2.9 (0.4) | 2.9 (0.3) | 0.1 (0.3) | 3.0 (0.1) | 3.0 (0.0) | 0.1 (0.3) | –0.03 [–0.15, 0.08] | 0.586 |
Computation | 4.5 (0.9) | 4.5 (0.8) | –0.1 (1.0) | 4.5 (0.8) | 4.1 (1.3) | –0.4 (1.6) | –0.34 [–0.81, 0.14] | 0.165 |
Recall | 2.4 (0.8) | 2.4 (0.9) | 0.4 (0.9) | 2.7 (0.6) | 2.4 (0.9) | 0.0 (0.9) | –0.39 [–0.71, –0.07] | 0.019 |
Language | 7.8 (0.7) | 7.9 (0.3) | 0.2 (0.6) | 8.0 (0.3) | 7.9 (0.4) | –0.1 (0.3) | –0.26 [–0.44, –0.07] | 0.007 |
Structure imitation | 0.9 (0.3) | 0.8 (0.4) | 0.0 (0.3) | 0.9 (0.3) | 0.9 (0.3) | 0.0 (0.3) | 0.00 [–0.12, 0.12] | 0.984 |
Total MoCA score | 24.8 (3.9) | 23.5 (3.6) | 0.8 (2.7) | 25.6 (3.1) | 24.5 (3.4) | 1.1 (2.6) | 0.24 [–0.73, 1.21] | 0.626 |
AVLT instantaneous memory | 32.4 (6.7) | 32.0 (8.6) | 5.0 (7.3) | 37.4 (6.9) | 33.8 (8.6) | 1.7 (5.7) | –3.30 [–5.70, –0.89] | 0.008 |
AVLT delayed recall | 11.8 (3.1) | 11.3 (3.6) | 1.2 (3.5) | 13.0 (2.8) | 11.5 (3.9) | 0.2 (2.0) | –1.01 [–2.06, 0.04] | 0.059 |
LMT immediate recall | 10.7 (4.2) | 8.2 (4.7) | 0.5 (4.3) | 11.2 (4.4) | 9.0 (5.2) | 0.7 (3.5) | 0.23 [–1.22, 1.68] | 0.755 |
LMT delayed recall | 9.4 (4.1) | 7.5 (4.4) | 1.8 (4.7) | 11.2 (3.9) | 7.9 (5.2) | 0.4 (3.3) | –1.39 [–2.90, 0.11] | 0.069 |
TMT-A total(s) | 55.2 (22.1) | 58.4 (23.9) | –5.5 (21.1) | 49.6 (18.4) | 54.6 (25.9) | –3.8 (20.3) | 1.71 [–5.89, 9.32] | 0.657 |
DSST total score | 35.0 (10.6) | 34.7 (10.6) | 4.5 (7.8) | 39.5 (11.8) | 36.3 (11.4) | 1.6 (7.2) | –2.91 [–5.67, –0.15] | 0.039 |
DSST wrong number | 0.2 (0.9) | 0.6 (2.6) | –0.2 (0.9) | 0.1 (0.3) | 0.5 (2.5) | –0.2 (0.8) | –0.02 [–0.34, 0.29] | 0.883 |
DSF | 6.9 (2.3) | 6.8 (1.7) | 0.5 (2.1) | 7.4 (1.5) | 6.1 (1.4) | –0.7 (1.5) | –1.25 [–1.93, –0.56] | <0.001 |
DSB | 4.1 (1.7) | 3.8 (1.3) | 0.0 (1.7) | 4.1 (1.3) | 3.8 (1.6) | –0.1 (1.1) | –0.07 [–0.60, 0.46] | 0.792 |
DST | 11.0 (3.8) | 10.6 (2.6) | 0.5 (3.3) | 11.5 (2.4) | 9.8 (2.5) | –0.8 (2.0) | –1.33 [–2.33, –0.34] | 0.009 |
SD, standard deviation; MoCA, Montreal Cognitive Assessment; MMSE, Mini-Mental State Examination; AVLT, Auditory Verbal Learning Test; LMT, Logic memory test; TMT-A, Trail Making Test A; DSST, Digit Symbol Substitution Test; DSF, digit span forwards; DSB, digit span backwards; DST, digit span test. p value calculated by difference of changes (12-week scores minus the baseline scores) between two groups.
Fig. 3
Outcomes of MMSE total and sub-scores in the intervention and control group.
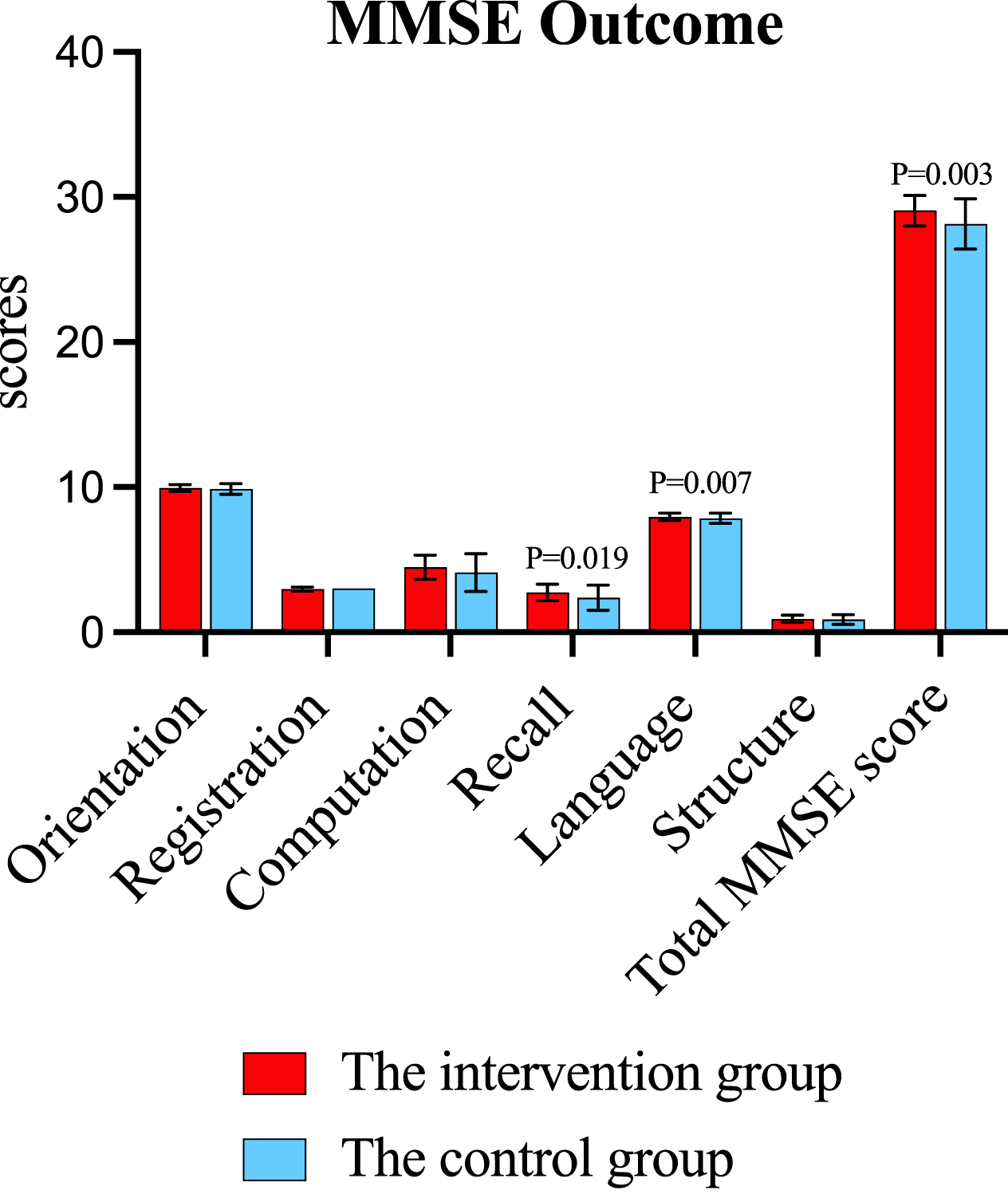
Figure 4 showed the changes of structural connectivity in DWI after the 12-week intervention. Compared to the control group, the intervention group exhibited heightened interactions in several regions, including the left thalamus proper-left isthmus cingulate cortex, left caudal middle frontal gyrus-left pars opercularis inferior frontal gyrus, left lateral orbitofrontal cortex-left precuneus cortex, and left pars orbitalis inferior frontal gyrus-left insula. These regions were associated with functions of language, executive, memory, and recall. Results remained significant after correction for multiple comparisons (false discovery rate).
Fig. 4
Changes in structural connectivity (diffusion-weighted imaging) after the intervention. a) language; b) executive; c) memory; d) recall; L, left; R, right. The intervention group showed improved in structural connectivity compared to the control group. The marked color of connecting line in the figure indicates the F value.
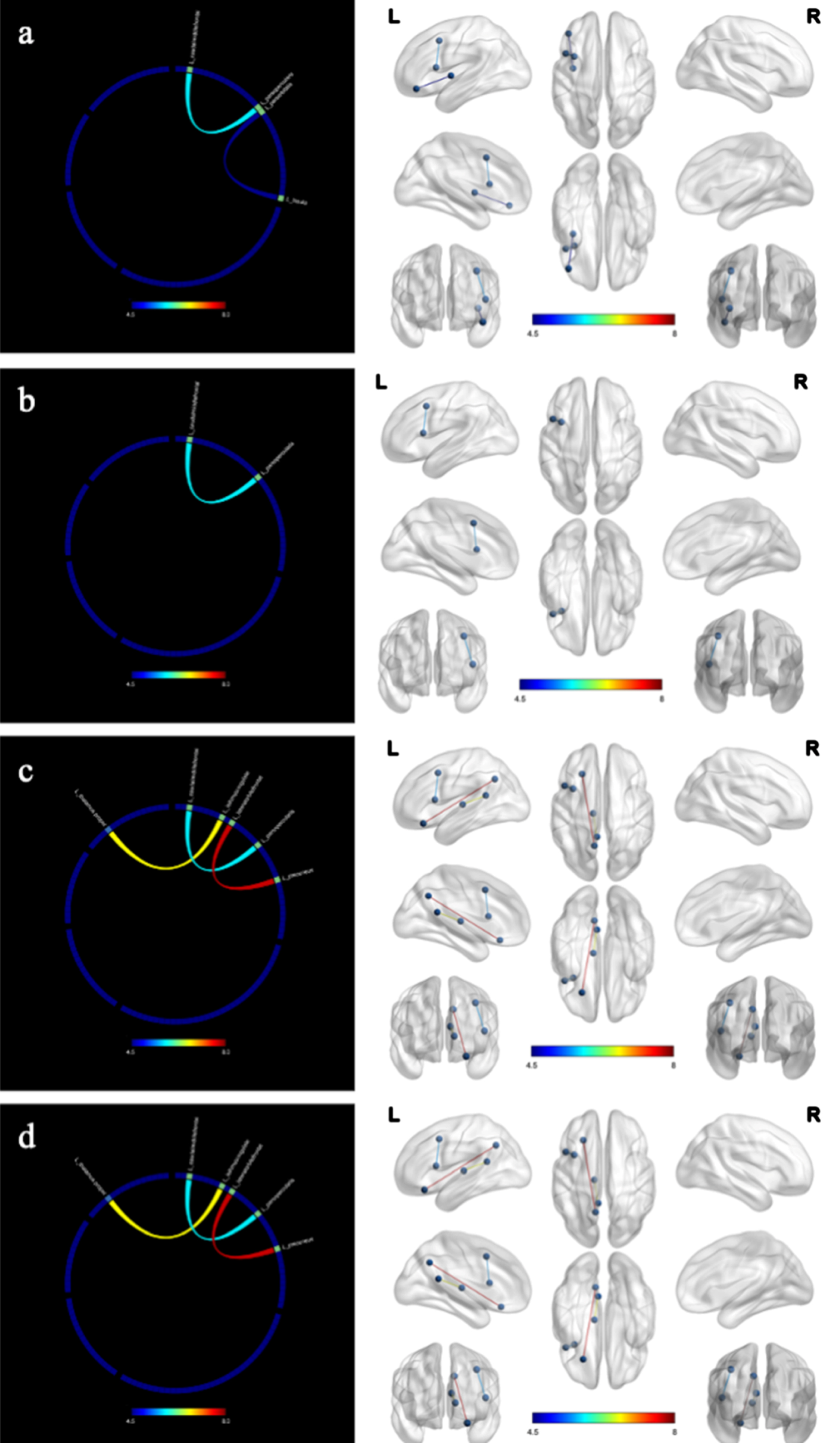
Figure 5 showed the changes of functional connectivity in resting state. The intervention group demonstrated improved functional connectivity associated with concentration, executive function, memory, and recall compared to the control group. The connectivity regions included brain stem-left frontal pole and right inferior temporal gyrus (ITG), left para hippocampal gyrus-bilateral caudal middle frontal gyrus and right temporal pole, left para hippocampal gyrus-right temporal pole, cerebellum cortex-left banks superior temporal sulcus.
Fig. 5
Changes in functional connectivity (resting state) after the intervention. a) concentration; b) executive; c) memory; d) recall; L, left; R, right. The intervention group showed improved in functional connectivity compared to the control group. The marked color of connecting line in the figure indicates the F value.
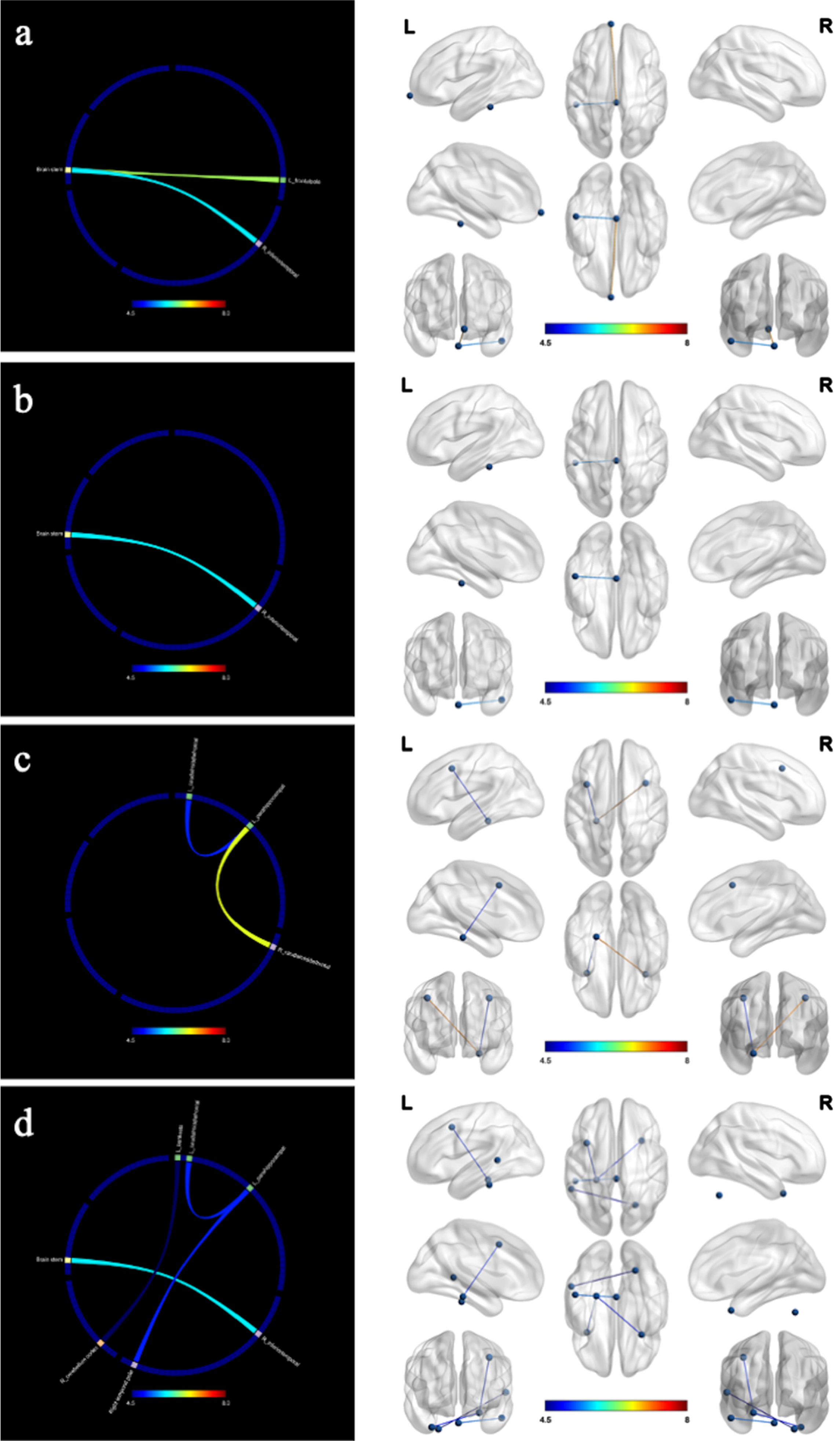
As showed in Fig. 6, after adjusting for age and gender, the spearman correlation analysis results revealed significant positive correlations between changes in neuropsychological and neuroimaging variables in the intervention group. Specifically, the change in the left caudal middle frontal gyrus-left pars opercularis inferior frontal gyrus was correlated with the change in MMSE language (r = 0.473, p = 0.020), while the change in the left caudal middle frontal gyrus-left para-hippocampal was correlated with the change in MMSE recall (r = 0.495, p = 0.014). Additionally, a positive correlation was observed between the change in the left caudal middle frontal gyrus-left pars opercularis inferior frontal gyrus and LMT Delayed recall (r = 0.468, p = 0.021), as well as between brain stem-right ITG and DST (r = 0.438, p = 0.032). No significant correlation was found between neuropsychological and neuroimaging variables in the control group.
Fig. 6
Correlations between neuropsychological tests and neuroimaging in the intervention group. L, left; R, right; MMSE, the Mini-Mental State Examination; LMT, Logic memory test; DST, digit span test. After adjusting for sex and age, the results remained significant.
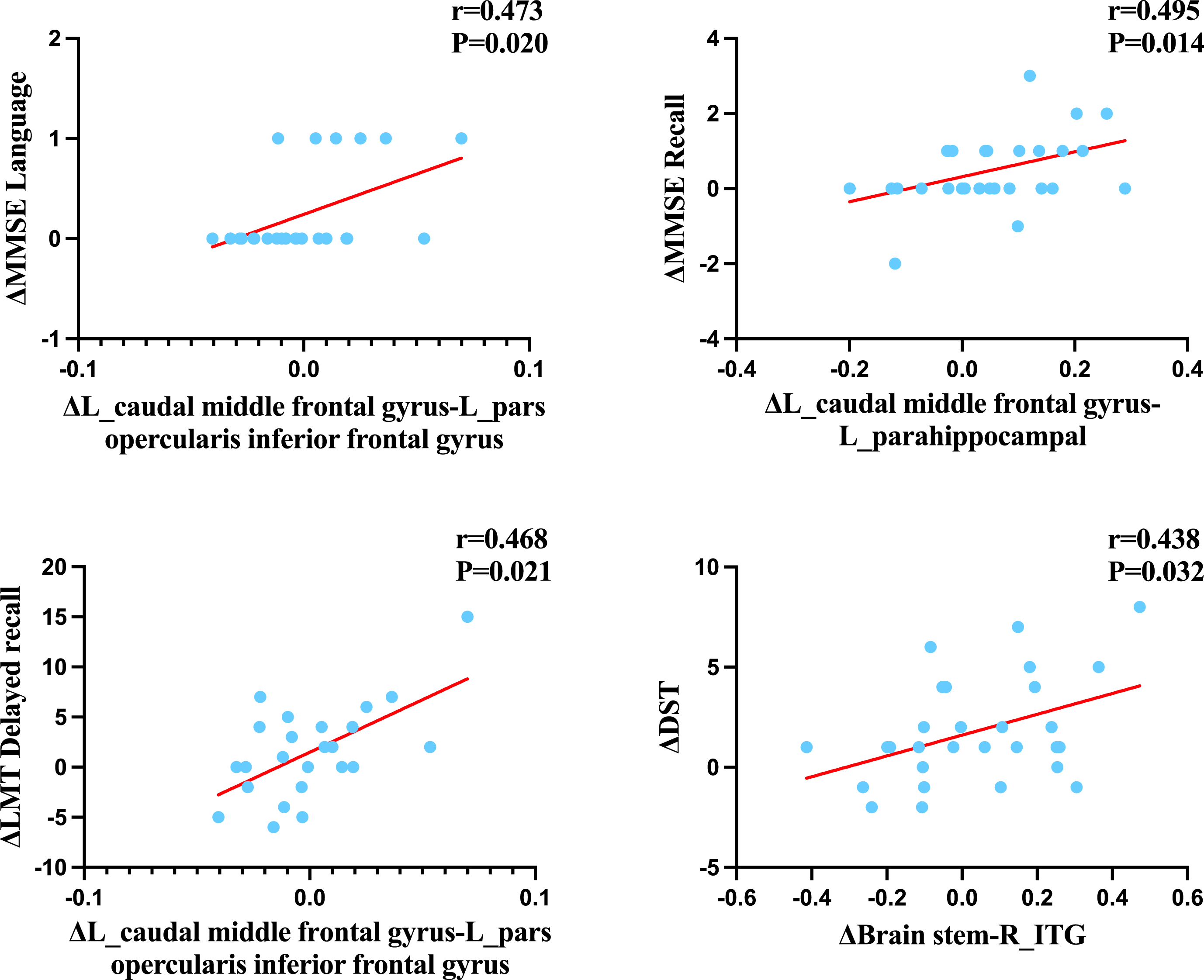
DISCUSSION
The findings of this 12-week randomized controlled trial indicated that the multimodal intervention led to enhancements in both cognitive function and neuroimaging. Participants in the intervention group exhibited improvements across diverse cognitive domains, including overall cognition, language, concentration, executive, memory, and recall function.
The SMART study21 recruited individuals with MCI aged 55 or above who received 6 months physical exercise and cognitive training. Improvements were observed in global cognitive function, along with sustained benefits in executive function and overall cognition over 18 months. The FINGER study22 was a 2-year multidomain intervention included nutritional guidance, physical exercise, cognitive training, social activity, lifestyle intervention. It targeted individuals aged 60–77 years at risk of dementia and demonstrated certain and significant intervention effects on overall cognition, executive functioning, processing speed, and the prevention of cognitive decline. The COCOA23 trial, a 2-year prospective randomized controlled trial, also involved participants with early-stage AD aged over 50 years, who received telephonic personalized coaching for multiple lifestyle interventions. Multimodal lifestyle interventions have been proven effective for ameliorating cognitive decline and exhibited a larger effect size than pharmacological interventions. The multiple clinical studies mentioned above shared many similarities with our study. All participants in the studies have either been diagnosed with MCI or are at risk of developing dementia. They all incorporated exercise and cognitive training, resulting in either improvement or maintenance of cognitive function. Our study participants and those in the SMART trial were diagnosed as MCI, with a risk of developing dementia. Meanwhile, participants in the FINGER and COCOA studies were at risk of dementia or in the early stage of AD. Our study is comparable to SMART, FINGER, and COCOA, as all these studies suggested that multimodal interventions could improve cognitive function in individuals with pre-dementia conditions. Despite the relatively shorter duration of 12 weeks in our study, we still observed improvements in cognition.
It is worth noting the style and content of the intervention program in our study. The intervention comprised components targeting memory strategies, concentration training, and incorporated computerized cognitive training, which have been demonstrated to have moderate effects on overall cognition, attention, and memory function.24 The core of non-pharmacological intervention lies in their non-drug nature and their ability to tailor to individual capabilities. The multimodal non-pharmacological intervention approach, which encompassed various components and focused on cognitive training, underscores the importance of considering age and learning abilities when developing interventions to improve cognitive function. This intervention has demonstrated promise in improving cognitive function and served as a cost-effective alternative to pharmacological treatments. Furthermore, some cohort studies25,26 also supported the notion that individually tailored multimodal interventions or a precision medicine approach to treat cognitive decline in Alzheimer’s disease and MCI may be effective, especially with continued optimization over time.
To further investigate the potential mechanism of the intervention, structural and functional MRI were performed before and after the intervention. The results revealed significant intervention-induced improvements in both structural and functional connectivity associated with language, concentration, executive, memory, and recall. Within the connectivity regions, the left caudal middle frontal gyrus, ITG, and para-hippocampal were found to be associated with multiple cognitive domains. The frontal gyrus is implicated in various cognitive functions, such as orientation, memory, concentration, and execution.27 The middle frontal gyrus was the cortical focus for both the storage of semantic memory and the processing components of working memory. Moreover, a study28 supports the contention that frontal gyrus damage is a common pathology in Parkinson with cognitive dysfunction. The ITG is implicated in visual object recognition, decision-making, the ventral visual pathway, language processing, and impulsivity.29,30 The anterior-temporal system, including the temporal pole and the orbitofrontal cortex, is involved in object memory. The para-hippocampal cortex, which is part of the posterior-medial system, is differentially engaged in cognitive processes and memory encoding and retrieval.31,32 Neural function operates at multiple scales, ranging from individual cell connections to local anatomical areas and larger brain regions connected through neural pathways. Furthermore, dynamic changes in functional networks can lead to a reshaping of the physical structure of brain networks through plasticity.33 The intervention enhancing the connectivity between regions related to cognitive as mentioned above, may potentially contribute to reshaping the brain structure. The changes in brain structure and brain function resulted in significant improvements across a wide array of functions related to language, concentrate, executive, memory, and recall processing. Correlation analysis further confirmed the consistency between neuropsychological performance and brain neuroimaging. In general, the nature of changes in brain connectivity and their associations with various cognitive and psychiatric conditions is intricate. Further research is needed to better understand the underlying mechanisms of brain connectivity changes in cognitive impairment and their implications for cognitive function.
However, it is important to acknowledge the limitations of this study. First, due to the limited sample size and the fact that MRI data is not the primary outcome, we only adjusted age and sex as the background variables. After adjusting for age and sex, the spearman correlation analysis results still revealed significantly positive correlations between changes in neuropsychological and neuroimaging variables in the intervention group. Future large-sampled studies with MRI as the primary outcome would allow for the adjustment of background variables in the analysis of MRI data. Additionally, only half of the participants completed the MRI data collection due to health-related reasons and lack of time. The MRI completion rates were similar (52.5% in the intervention group and 52.6% in the control group). Overall, the relatively small sample size may cause insignificant correlations between changes in neuropsychological and neuroimaging variables, although we found some positive correlations in the intervention group adjusted for age and sex. Future studies with larger sample size are needed to explore the potential mechanism of the intervention effect on cognitive function based on the analysis of MRI data. Finally, the short follow-up time and the use of only one time point for outcome assessment during the intervention are additional limitations of our study. Future studies should include longer follow-up periods to observe the long-term effects of the multimodal non-pharmacological intervention and incorporate multiple assessment points to strengthen the conclusions.
In conclusion, the current study contributes to the growing body of research supporting the effectiveness and safety of the multimodal intervention in improving cognitive function and exploring the potential mechanism in older individuals with MCI. Continued research and exploration in this area can further refine and optimize these interventions for the well-being and cognitive health of older adults.
AUTHOR CONTRIBUTIONS
Meixiang Fan (Investigation; Writing – original draft; Writing – review & editing); Qingfeng Li (Data curation; Writing – original draft); Tingting Yang (Investigation; Methodology); Yinghua Yang (Investigation; Methodology; Supervision); Zhihua Chen (Investigation; Methodology; Supervision); Guo Xuan (Investigation); Ye Ruan (Investigation); Shuangyuan Sun (Investigation); Meng Wang (Investigation); Xiaoli Chen (Investigation); Yanyan Huang (Conceptualization; Investigation; Project administration; Supervision; Writing – review & editing); Zhi Yang (Data curation; Software); Ying Wang (Conceptualization; Methodology; Project administration; Supervision).
ACKNOWLEDGMENTS
We thank the study team members for their cooperation in data collection. We would like to thank the Xinzhuang Town Community Management Office for assistance with participants recruitment. We are grateful to staff members of the Shanghai Center for Disease Control and Prevention (SCDC), Xinzhuang Community Health Service Center of Minhang District, Medicine-Mental Health Center of Minhang District, and Yihua Jiang for their cooperation in the success of this study. We also sincerely thank Professor Ding Ding for valuable advice. The computerized cognitive training machines are provided by the VR lab at Fudan University as well as technological support without conflicts of interest. We also thank all trial participants for their cooperation.
FUNDING
This work was supported by the National Key Research and Development Project (2017YFC1310504), Tianqiao and Chrissy Chen for Translational Research (No. 201801), Shanghai Municipal Science and Technology Major Project (No.2018SHZDZX01), Science and Technology Innovation 2030 Major Projects (2022ZD0211600). Funding sources was not involved in study design, collection, analysis, or interpretation of data, writing the report, or the decision to submit the manuscript for publication.
CONFLICT OF INTEREST
The authors have no conflict of interest to report.
DATA AVAILABILITY
The data supporting the findings of this study are available within the article.
REFERENCES
1. | United Nations Department of Economic and Social Affairs, https://population.un.org/wpp/ ((2019) ). |
2. | Charlson FJ , Baxter AJ and Cheng HG. The burden of mental, neurological, and substance use disorders in China and India: a systematic analysis of community representative epidemiological studies. Lancet (2016) ; 388: : 1984–1984. |
3. | Hurd MD , Martorell P and Langa KM. Monetary costs of dementia in the United States. N Engl J Med (2013) ; 369: : 489–490. |
4. | Petersen RC , Lopez O , Armstrong MJ , et al. Practice guideline update summary: Mild cognitive impairment: Report of the Guideline Development, Dissemination, and Implementation Subcommittee of the American Academy of Neurology. Neurology (2018) ; 90: : 126–135. |
5. | Jia L , Du Y , Chu L , et al. Prevalence, risk factors, and management of dementia and mild cognitive impairment in adults aged 60 years or older in China: a cross-sectional study. Lancet Public Health (2020) ; 5: : e661–e671. |
6. | Szeto JY and Lewis SJ. Current treatment options for Alzheimer’s disease and Parkinson’s disease dementia. Curr Neuropharmacol (2016) ; 14: : 326–338. |
7. | Horr T , Messinger-Rapport B and Pillai JA. Systematic review of strengths and limitations of randomized controlled trials for non-pharmacological interventions in mild cognitive impairment: focus on Alzheimer’s disease. J Nutr Health Aging (2015) ; 19: : 141–153. |
8. | Simon SS , Yokomizo JE and Bottino CM. Cognitive intervention in amnestic mild cognitive impairment: a systematic review. Neurosci Biobehav Rev (2012) ; 36: : 1163–1178. |
9. | Young KW , Ng P , Kwok T , et al. The effects of holistic health group interventions on improving the cognitive ability of persons with mild cognitive impairment: a randomized controlled trial. Clin Interv Aging (2017) ; 12: : 1543–1552. |
10. | Chan SCC , Chan CCH , Derbie AY , et al. Chinese calligraphy writing for augmenting attentional control and working memory of older adults at risk of mild cognitive impairment: a randomized controlled trial. J Alzheimers Dis (2017) ; 58: : 735–746. |
11. | Livingston G , Huntley J , Sommerlad A , et al. Dementia prevention, intervention, and care: 2020 report of the Lancet Commission. Lancet (2020) ; 396: : 413–446. |
12. | Karssemeijer EGA , Aaronson JA , Bossers WJ , et al. Positive effects of combined cognitive and physical exercise training on cognitive function in older adults with mild cognitive impairment or dementia: A meta-analysis. Ageing Res Rev (2017) ; 40: : 75–83. |
13. | Ham MJ , Kim S , Jo YJ , et al. The effect of a multimodal occupational therapy program with cognition-oriented approach on cognitive function and activities of daily living in patients with Alzheimer’s disease: a systematic review and meta-analysis of randomized controlled trials. Biomedicines (2021) ; 9: : 1951. |
14. | Petersen RC . Mild cognitive impairment as a diagnostic entity. J Intern Med (2004) ; 256: : 183–194. |
15. | Ding D , Zhao Q , Guo Q , et al. Prevalence of mild cognitive impairment in an urban community in China: a cross-sectional analysis of the Shanghai Aging Study. Alzheimers Dement (2015) ; 11: : 300–309 e302. |
16. | Wang ZYZ , M. Y. Application of the Chinese version of the Mini-Mental State Examination (MMSE). Shanghai Psychiatric Medicine (1989) ; 007: : 108–111. |
17. | Julayanont P , Tangwongchai S , Hemrungrojn S , et al. The Montreal Cognitive Assessment-Basic: a screening tool for mild cognitive impairment in illiterate and low-educated elderly adults. J Am Geriatr Soc (2015) ; 63: : 2550–2554. |
18. | Llinas-Regla J , Vilalta-Franch J , Lopez-Pousa S , et al. The Trail Making Test. Assessment (2017) ; 24: : 183–196. |
19. | Lezak MD . Neuropsychological Assessment. 2nd ed. New York: Oxford University Press, (1983) . |
20. | Lv X , Li W , Ma Y , et al. Cognitive decline and mortality among community-dwelling Chinese older people. BMC Med (2019) ; 17: : 63. |
21. | Fiatarone Singh MA , Gates N , Saigal N , et al. The Study of Mental and Resistance Training (SMART) study-resistance training and/or cognitive training in mild cognitive impairment: a randomized, double-blind, double-sham controlled trial. J Am Med Dir Assoc (2014) ; 15: : 873–880. |
22. | Ngandu T , Lehtisalo J , Solomon A , et al. A 2 year multidomain intervention of diet, exercise, cognitive training, and vascular risk monitoring versus control to prevent cognitive decline in at-risk elderly people (FINGER): a randomised controlled trial. Lancet (2015) ; 385: : 2255–2263. |
23. | Roach JC , Rapozo MK , Hara J , et al. A remotely coached multimodal lifestyle intervention for Alzheimer’s disease ameliorates functional and cognitive outcomes. J Alzheimers Dis (2023) ; 96: : 591–607. |
24. | Hill NT , Mowszowski L , Naismith SL , et al. Computerized cognitive training in older adults with mild cognitive impairment or dementia: a systematic review and meta-analysis. Am J Psychiatry (2017) ; 174: : 329–340. |
25. | Isaacson RS , Ganzer CA , Hristov H , et al. The clinical practice of risk reduction for Alzheimer’s disease: A precision medicine approach. Alzheimers Dement (2018) ; 14: : 1663–1673. |
26. | Toups K , Hathaway A , Gordon D , et al. Precision medicine approach to Alzheimer’s disease: successful pilot project. J Alzheimers Dis (2022) ; 88: : 1411–1421. |
27. | Hanganu A , Bedetti C , Jubault T , et al. Mild cognitive impairment in patients with Parkinson’s disease is associated with increased cortical degeneration. Mov Disord (2013) ; 28: : 1360–1369. |
28. | Thota N , Lenka A , George L , et al. Impaired frontal lobe functions in patients with Parkinson’s disease and psychosis. Asian J Psychiatr (2017) ; 30: : 192–195. |
29. | Giampiccolo D and Duffau H. Controversy over the temporal cortical terminations of the left arcuate fasciculus: a reappraisal. Brain (2022) ; 145: : 1242–1256. |
30. | Nobre AC , Allison T and McCarthy G. Word recognition in the human inferior temporal lobe. Nature (1994) ; 372: : 260–263. |
31. | Ranganath C and Ritchey M. Two cortical systems for memory-guided behaviour. Nat Rev Neurosci (2012) ; 13: : 713–726. |
32. | Berron D , Neumann K , Maass A , et al. Age-related functional changes in domain-specific medial temporal lobe pathways. Neurobiol Aging (2018) ; 65: : 86–97. |
33. | Candadai M , Setzler M , Izquierdo EJ , et al. Embodied dyadic interaction increases complexity of neural dynamics: a minimal agent-based simulation model. Front Psychol (2019) ; 10: : 540. |