Effects of 6-Month Combined Physical Exercise and Cognitive Training on Neuropsychological and Neurophysiological Function in Older Adults with Subjective Cognitive Decline: A Randomized Controlled Trial
Abstract
Background:
Multidomain intervention may delay or ameliorate cognitive decline in older adults at risk of Alzheimer’s disease, particularly in the memory and inhibitory functions. However, no study systematically investigates the changes of brain function in cognitively-normal elderly with subjective cognitive decline (SCD) when they receive multidomain intervention.
Objective:
We aimed to examine whether a multidomain intervention could improve neuropsychological function and neurophysiological activities related to memory and inhibitory function in SCD subjects.
Methods:
Eight clusters with a total of 50 community-dwelling SCD older adults were single-blind, randomized into intervention group, which received physical and cognitive training, or control group, which received treatment as usual. For the neuropsychological function, a composite Z score from six cognitive tests was calculated and compared between two groups. For the neurophysiological activities, event-related potentials (ERPs) of memory function, including mismatch negativity (MMN) and memory-P3, as well as ERPs of inhibitory function, including sensory gating (SG) and inhibition-P3, were measured. Assessments were performed at baseline (T1), end of the intervention (T2), and 6 months after T2 (T3).
Results:
For the neuropsychological function, the effect was not observed after the intervention. For the neurophysiological activities, improved MMN responses of ΔT2–T1 were observed in the intervention group versus the control group. The multidomain intervention produced a sustained effect on memory-P3 latencies of ΔT3–T1. However, there were no significant differences in changes of SG and inhibition-P3 between intervention and control groups.
Conclusions:
While not impactful on neuropsychological function, multidomain intervention enhances specific neurophysiological activities associated with memory function.
INTRODUCTION
Although mild cognitive impairment (MCI) has been considered as an intermediate stage between normal aging and dementia, there is increasing attention to the pre-MCI stage, called subjective cognitive decline (SCD). The operational definition of SCD refers to self-experienced persistent decline in cognitive abilities as compared with the previously normal status, along with normal age- and education-adjusted performance in standardized neuropsychological tests [1, 2]. The identification of SCD is clinically important since the existing literature has revealed that older adults with SCD have 1.64 to 5.1 times the risk of developing MCI or dementia than those without SCD [3–5]. However, due to the absence of an effective cure for dementia at present, early non-pharmacological intervention at SCD stage is definitely an promising opportunity to improve cognitive function or prevent cognitive deline.
Since cognitive decline has multiple causes, multidomain interventions which target several modifiable factors simultaneously are considered appropriate for addressing concurrent age-related deterioration in mental and physical functions. Up to the present, there were several randomized controlled trials (RCT) devoted to determining the effectiveness of multidomain intervention on improving cognition or reducing dementia incidence in older adults with SCD [6–8] or those at risk of cognitive decline [9, 10]. Although some of the trials failed to find significant effects on cognitive decline [7, 8], there were still many studies showing that the combination of different components could significantly enhance cognitive performance in older adults with SCD. For example, the FINGER trial demonstrated that a 2-year multidomain intervention of diet, physical exercise, and cognitive training improved cognitive performance in at-risk elderly people [10]. A recent RCT study also showed that immediately after a 9-month multidomain intervention of meditation, physical exercise, cognitive training, and nutrition counseling, cognitive performance was significantly enhanced in community-dwelling older adults who were at risk of cognitive decline [11]. Several studies employing comparable multidomain intervention approaches but over a shorter training duration (e.g., 2 to 6 months) have also yielded promising outcomes in older adults with SCD [9, 12]. Upon reviewing these studies, neuropsychological assessments served as the primary outcomes. However, the neurophysiological plasticity underlying the benefit of multimodal intervention has been unclear. Hence, non-invasive electroencephalographic (EEG) recordings, in conjunction with suitable experimental tasks, are considered as a feasible method to examine the effects of multimodal intervention on brain’s plastic changes in the community-dwelling older adults with SCD [13, 14].
In the present study, we focused on the electrophysiological responses related to memory and inhibitory control with the reasons that accumulative evidence has indicated these two aspects were substantially manifested in older adults with cognitive decline [15, 16]. In terms of memory function, the electrophysiological signals of sensory memory and working memory were examined. Mismatch negativity (MMN), considered as a bottom-up memory function, is the brain’s ability to automatically detect the changes of the environment and can reflect the integrity of sensory memory function [17, 18]. On the other hand, working memory is a relatively top-down process of memory function which requires attention allocation and context updating [19, 20]. The P3 component, a positive deflection around 300 to 600 ms after the stimulus onset, has been considered as an electrophysiological indicator of working memory [21, 22]. This component related to working memory, called memory-P3 in the present study, usually shows maximal amplitudes over the centro-parietal region of the scalp. In terms of inhibitory control, the electrophysiological signals of sensory gating and response inhibition were examined. Sensory gating (SG), considered as a bottom-up inhibitory function, is the brain’s ability to automatically filter out repetitive sensory inputs, and thus serves a protective function to avoid sensory inundation [23, 24]. On the other hand, response inhibition requires top-down modulation of cognitive control to withhold motor responses to non-target stimuli. The N2 and P3 components were two obvious electrophysiological markers induced by successful inhibition to Nogo stimuli in a Go-Nogo task [25–27]. The N2 component peaks around 200 to 300 ms over the frontocentral region, and its amplitude is found to be larger in the responses to Nogo than Go stimuli; the P3 component, occurring around 300 to 600 ms after the stimulus onset, is observed in the parietal region in responses to Go stimuli and in the frontocentral region in responses to Nogo stimuli [28, 29]. Unlike N2 that is involved in many cognitive functions such as response inhibition, conflict detection and error monitoring, the P3 component is more related to motor inhibition and stimulus evaluation [30–32]. Therefore, the P3 response to successful inhibition, called inhibition-P3, is applied in the present study.
Up to the date, there has been no RCT study examining the effects of the combination of physical exercise and cognitive training on both neuropsychological and neurophysiological functions in older adults with SCD. There has been ample evidence showing that the combination of physical exercise and cognitive training could enhance cognitive function among older adults with cognitive decline [33, 34]. Engaging in physical exercise is linked to various protective mechanisms, such as increased cerebral blood flow and neural connectivity [35, 36], as well as elevated levels of brain-derived neurotrophic factor [37, 38]; cognitive training can amplify the aforementioned plastic changes by offering the brain new and challenging experiences [39]. From the perspective of feasibility, the combination of these two methods has been shown to be accepted by community-dwelling older adults in Taiwan [40, 41]. Thus, the first goal of the present study was to determine the effects of multifaceted intervention of physical exercise and cognitive training on neuropsychological function related to memory and inhibitory function. The existing studies showed that a composite Z score of cognitive tests is now considered to be a better endpoint [42]. Hence, the primary outcome was the change in cognitive performance measured by composite Z score from 6 cognitive tests related to memory and inhibitory function. The second goal of the present study was to determine the effects of this multifaceted intervention on neural activities related to memory and inhibitory function. Hence, the secondary outcomes were the changes in ERPs of memory function (i.e., MMN and memory-P3) and inhibitory control (i.e., SG and inhibition-P3). Moreover, we also aimed to examine the associations between neuropsychological performance and neurophysiological function.
In summary, this 6-month cluster-randomized controlled trial was designed to examine whether the combined physical exercise and cognitive training could improve neuropsychological and neurophysiological functions in community-dwelling older adults with SCD. For cognitively-normal older adults with SCD, although the single domain of physical exercise did not yield a significantly beneficial effect on the cognitive performance [43, 44], a meta-analysis study showed that cognitive training led to significant improvement in cognitive tests despite a small effect size [45]. With the combination of these two intervention components, we hypothesized that the intervention group that received physical and cognitive training would show improved neuropsychological function (i.e., primary outcome) as compared to the control group that received treatment as usual. We also hypothesized that the SCD subjects receiving multidomain intervention would showed improved neurophysiological functions (i.e., secondary outcomes) as compared to those receiving treatment as usual. This hypothesis was based on the synergistic effect of the physical exercise and cognitive training on the cerebral blood flow and neural connectivity as mentioned in the previous section [35, 36, 39]. Finally, we assumed that there existed an association wherein enhanced cognitive performance would be correlated with improved neural responses after the intervention.
METHODS
Study design
This was a cluster-randomized, parallel-group, single-blind trial involving a 6-month intervention of physical exercise and cognitive training on older adults with SCD, followed by an additional 6-month follow-up to determine any potential sustained effect on cognitive function. Eight local community facilities and day care centers in Taipei City were enrolled and randomized into either intervention (4 clusters, n = 33) or control (4 clusters, n = 17) group between November 2019 and February 2022. Due to the COVID-19 pandemic, one cluster of the intervention groups (n = 9) stopped for 6 weeks from May 10 to June 18 in 2021. These last suspended 12 sessions were then additionally provided 5 months later.
The randomization of intervention or control group was carried out using a web-based randomization table with a ratio of 1 : 1 at the cluster level. Since each intervention cluster had more subjects than control clusters, the total number of subjects in the intervention group was therefore more than that in the control group. All the outcome measures were performed and analyzed by a trained research assistant, who was blinded to the group allocation. Given the absence of prior research on the effects of a 6-month combined physical exercise and cognitive training regimen for older adults with SCD, the sample size was not determined through a rigorous power analysis. Instead, we adhered to the approach used in previous studies examining the effectiveness of non-pharmacological interventions for community-dwelling older adults, with a target of at least 10–20 individuals per group [46, 47].
This study was approved by the ethics committee of the Taipei Veterans General Hospital (No. 2019-06-002A), and registered with ClinicalTrial.gov (ID: NCT04162990). All experiments were carried out in accordance with relevant guidelines and regulations (Declaration of Helsinki), and all participants provided written informed consent before participating in this study.
Recruitment and participants
The principal investigator (CHC) reached out to community centers in Taipei City to explore the feasibility to conducting this study. A total of 8 community centers agreed to participate in this study. Fliers and informational sessions were distributed to community-dwelling older adults at these 8 sites. Those expressing interests in the study were then recruited and screened.
This study targeted community-dwelling older adults aged 50 years old or above. The eligible volunteers were screened by a 12-item SCD questionnaire [48, 49], in which they compared their current cognitive function with that of two years ago. The subjects with SCD must give a “yes” to at least one item, together with the confirmation by family or close friends. In addition, their objective performance on neuropsychological tests must be within normal range (scores of Cognitive Abilities Screening Instrument [CASI] > 79 out of 100) [50]. Exclusion criteria were that (1) participants or first-degree relatives are diagnosed with mental diseases; (2) participants have a history of severe neurological diseases or brain injury led to loss of consciousness; (3) participants have diagnosis of cardiovascular diseases; (4) participants have a history of alcohol, nicotine, or substance dependence; and (5) participants suffer from visual and hearing degeneration, including hearing aid users.
Intervention
The intervention group received one-hour physical exercise followed by one-hour cognitive training twice-weekly for 6 months (i.e., 48 sessions). The training sessions were conducted in a small group of 4–12 participants. Two certificated occupational therapists underwent training from the PI (CHC) prior to the beginning of this study in order to ensure consistency in intervention delivery. Each session of physical exercise included a 5–10 min of warm-up, followed by 30–40 min of physical exercise, and ended with 5–10 min of cool-down. The physical exercise program consisted of resistance training, balance training and aerobic exercise. The intensity of physical training was at a moderate level (about 3 to 6 METs), wherein the participants can comfortably talk but not sing. We applied the discontinuous manner wherein a short break among the training modes of resistance training, balance training, and aerobic exercise was provided. Thera-Band and water bottles were the primary equipment for resistance training, targeting the larger muscles of upper and lower extremities. Balance training in sitting and standing positions was performed through goal-directed activities. Aerobic exercise included aerobic dance and steeping, with a progressive intensity at a Borg Rating of Perceived Exertion (Borg RPE) of 13–16 (somewhat hard to hard). After physical exercise, the participants took part in 40–50 min of cognitive training, including visual and auditory attention, processing speed, visual and auditory memory, prospective memory, and executive function (e.g., abstract thinking, categorization, and reasoning). For each session, the occupational therapist focused on one of cognitive domains and delivered the training through the form of group activities. For the training of visual attention, the participants were, for example, instructed to point out the differences between two similar pictures or to search a target from a complex background. For the training of processing speed, the activities of bamboo dance or musical chairs were delivered. For the training of auditory memory, the news was broadcast to the participants and they had to carefully listen to the content for a subsequent recall. For the training of prospective memory, the participants were instructed to make a story based on given cues or to plan the sequence of stops on a shopping trip. For the training of executive function, the activities of word chain or riddles were delivered. Each cognitive domain was repeated for 5 to 6 sessions through different kinds of activities. Each session of cognitive training included a 5–10 min of instruction, followed by 30–40 min cognitive activities, and ended with 5–10 min of feedback to the activities.
Participants in the control group received 30-min face-to face health education at the beginning of the study (one session). After that, they were treated as usual without receiving any intervention component we provided. In other word, the control group kept their regular lifestyle.
Outcomes
For each participant, all the outcome measures were administered at baseline (T1), end of the intervention (T2), and 6 months after the intervention (T3).
Primary outcome: Neuropsychological function
Neuropsychological tests related to memory and inhibitory functions were assessed by a research assistant, including Chinese Version Verbal Learning Test (CVVLT), Logic Memory Test Part A of the Wechsler Memory Scale (LM), Taylor Complex Figure Test (CFT), Digit Span Backward (DSB), and Trail-Making Test–B (TMT–B). The general cognitive performance, CASI, was also assessed. A composite Z score of these 6 cognitive tests was calculated from each individual to serve as the primary outcome, with higher score suggesting better performance.
(1) CVVLT: This test was used to evaluate performance of immediate recall and delayed recall. Nine two-character nouns were spoken to the subjects for 4 learning trials. After each trial, they were asked to recall as many words as possible in any order. The total counts of accurate items were recorded for immediate recall (0–9 points) and after a 10-min delay for delayed recall (0–9 points). Higher scores indicating better performance of episodic memory [51, 52].
(2) LM: This test was also used to evaluate performance of immediate recall and delayed recall [53]. The subjects were presented with a brief spoken story, and then instructed to make an immediate recall (0–25 points) and another recall after a 30-min delay (i.e., delayed recall, 0–25 points). Higher scores indicated better performance of episodic memory.
(3) CFT: This test was used to assess visuospatial memory function [54]. The subjects were presented with a Taylor figure, and then performed a copy task. After that, they were asked to make an immediate recall (0–36 points) and another recall after a 10-min delay (i.e., delayed recall, 0–36 points). Higher scores indicating better performance of visuospatialmemory.
(4) DSB: This study was used to assess working memory [55]. The subjects were presented with a series of spoken digits, and then instructed to repeat the numbers in a reverse order (0–7 points). Higher scores indicated better performance of working memory.
(5) TMT–B: This test was used to evaluate central executive function of task-set inhibition and cognitive flexibility [56, 57]. The subjects were instructed to connect number and sequential numbers in Chinese characters (rather than English alphabets) in an alternating progressive sequence as quickly as possible [52]. Completion time in seconds was recorded, with higher scores representing poorer performance in TMT–B.
(6) CASI: This test was used to assess general cognition, which consisted of attention, orientation, verbal fluency, short-term memory, long-term memory, visual construction, abstract thinking, and judgement [50]. Higher scores indicated better performance of general cognition.
Secondary outcomes: Neurophysiological responses
Four event-related potential (ERP) responses were obtained based on 4 experimental blocks (i.e., oddball, 1-back, paired-stimulus, and Go-Nogo tasks), which were performed in a counterbalanced sequence among the subjects. Oddball and 1-back tasks were used to collect memory-related electrophysiological responses; paired-stimulus and Go-Nogo tasks were used to collect inhibition-related electrophysiological responses.
(1) Oddball paradigm: This task was designed to assess pre-attentive ability of change detection based on the sensory memory traces. During the ERP recordings, the subjects were presented with an auditory oddball paradigm, which consisted of repetitive standard stimuli (1000-Hz pure tones, 85%) and infrequent deviant stimuli (900-Hz pure tones, 15%) with an inter-stimulus of interval (ISI) was 1000 ms. In this experiment, the subjects were instructed to watch an emotionally-neural silent video with subtitles and ignore the auditory stimuli we delivered [48, 58]. MMN, reflecting the integrity of auditory sensory memory, was calculated from the subtraction of ERP responses to standards from those to deviants. Larger MMN amplitudes and/or shortened MMN latencies indicate better function. It has been shown that compared to individuals without SCD, those with SCD demonstrated reduced MMN amplitudes [48].
(2) 1-back task: This task was designed to evaluate working memory function. During the ERP recordings, the subjects were prompted to press a response button if the current geometrical figure (i.e., circle, triangle, square, Inverted triangle, or diamond) matched the one presented in the preceding trial. The task consisted of about 30% targets and about 70% non-targets with an ISIs between 1600-2000 ms. The instruction emphasized responding accurately and quickly. Successful responses to targets would induce P3 (i.e., memory-P3), which has been thought to engage attentional and memory mechanism [59]. Larger P3 amplitudes and/or shortened P3 latencies indicate better function.
(3) Paired-stimulus paradigm: This task was designed to assess pre-attentive ability to filter out redundant sensory information. During the ERP recordings, the subjects were presented with an auditory paired-stimulus paradigm, which consisted of a series of pairs of identical pure tones with an ISI of 500 ms and an inter-pair interval of 6000–8000 ms. In this experiment, the subjects were instructed to watch an emotionally-neural silent video with subtitles and ignore the auditory stimuli we delivered [53, 60]. SG, an electrophysiological marker of automatic inhibitory function, was calculated from the subtraction of ERP responses to the second stimuli (S2) from those to the first stimuli (S1) (i.e., “S1 – S2”). In the present study, N1 amplitude was utilized to calculate SG since this ERP component could be identified in every subject. Larger S1 – S2 differences indicate better SG function. It has been shown that compared to individuals without SCD, those with SCD demonstrated reduced SG function [24].
(4) Go-Nogo task: This task was designed to assess the ability of response inhibition. During the ERP recordings, the subjects were instructed to press a response button when they saw Go trials (i.e., 1, 2, 4, 5, 6, 8, 9), and withhold their responses to Nogo trials (i.e., 3, 7). The task consisted of about 85% Go trials and about 15% Nogo trials with ISIs between 1400–2200 ms. The instruction emphasized responding accurately and quickly. Successful responses to Nogo trials would induce P3 (i.e., inhibition-P3), which has been related to motor inhibition [61]. Larger inhibition-P3 amplitudes and/or shortened inhibition-P3 latencies indicate better response inhibition. This signal has been employed to investigate older adults with SCD through either observational or intervention designs [13, 62].
EEG recordings and analyses
Electrophysiological responses were recorded using a dry-electrode, battery-powered, portable DSI-24 EEG headset (WEARABLE Sensing Inc. San Diego, USA), which consists of 19 electrodes evenly distributed across the scalp corresponding to the 10-20 system, two electrodes at bilateral earlobes, and three built-in auxiliary inputs. Eye blinks and movements were recorded with electrooculograms, which were attached above the left orbit and below the right orbit. All the EEG data were online referenced to Pz, and the sampling rate was set at 300 Hz. The impedance of all the electrodes was kept below 1 Mohm.
All the ERP pre-processing was conducted using EEGLAB [63]. The offline data were re-referenced to the average of two-earlobe electrodes (i.e., A1 and A2), and the bandpass filter was set at [0.1, 30] Hz. Artifacts contaminated by eye movements were identified and removed using independent component analysis. Epochs with EEG activities exceeding±75 uV on the channels of interest were also removed from averaging.
Regarding MMN responses, the continuous EEG was segmented into epochs of 600 ms, with a 100-ms pre-stimulus baseline. Epochs were then averaged separately for the standards and deviants, and the MMN was calculated by subtracting the responses to standards from those to deviants (i.e., “Deviants” – “Standards”). The peak amplitude and peak latency of MMN responses were determined between 100 and 300 ms at the Fz electrode.
Regarding memory-P3 responses, the continuous EEG was segmented into epochs of 1100 ms, with a 200-ms pre-stimulus baseline. Epochs were then averaged for the successful targets. Although memory-P3 component could be identified at Fz, Cz, and Pz, we selected Pz as the channel of interest in the present study since this channel showed the maximal responses, which occurred around 300–650 ms.
Regarding SG, the continuous EEG was segmented into epochs of 500 ms, with a 100-ms pre-stimulus baseline. Epochs were then averaged separately for the S1 and S2. Peak amplitudes of N1 were determined between 70 and 150 ms for S1 and S2. The SG was then calculated as S1 – S2 differences at Cz electrode. Previous studies have shown that compared to the S2/S1 ratios, the S1 – S2 differences had better test-retest reliability [64, 65].
Regarding inhibition-P3 responses, the continuous EEG was segmented into epochs of 1100 ms, with a 200-ms pre-stimulus baseline. Epochs were then averaged for the successful Nogo trials. The inhibition-P3 was identified at Pz since this electrode showed the maximal responses, which occurred around 300–650 ms.
Statistical analysis
This study applied a per-protocol analysis, indicating that only those who completed the whole program were included in the analysis. This analysis could allow us to illustrate and report electrophysiological data more accurately. Considering the exploratory investigation on the neurophysiological responses, we opted for a simpler statistical procedure rather than a full-blown repeated measure ANOVA. Specifically, the changes scores from T1 to T2 (i.e., ΔT2–T1) and from T1 to T3 (i.e., ΔT3–T1) were calculated and then compared between control and intervention groups. Since most of the variables in the demographic information and some of the variables in the outcome measures did not follow normal distribution as evaluated by Kolmogorov-Smirnov one-sample test (p < 0.05) and a smaller sample size was found in the control group (n = 13), Mann-Whitney U tests or chi-square tests, as appropriate, were used to determine the group effects of on each dependent variable. The associations between neuropsychological performance and neurophysiological responses were further assessed using partial correlation coefficients (controlled variables = age, gender, education). Here, the significance of correlations was corrected for multiple comparisons using the Benjamini and Hochberg approach [66], and the false discovery rate (FDR) was set at 0.05. We reported original p values along with the results of the subsequent Benjamini– Hochberg procedure (i.e., significant or not).
All the data were analyzed using SPSS 19.0 (SPSS IBM, Armonk, NY, USA). The alpha level was set at 0.05 for each comparison.
RESULTS
Participant flow and program adherence
A total of 74 older adults were assessed for eligibility, and the final sample consisted of 50 subjects with SCD from 8 community centers (Fig. 1). The overall retention rate at the end of the intervention was 82% (41 out of 50), with the intervention group (72.73%, 24 out of 33) showing a lower retention rate (p = 0.020) compared to the control group (100%, 17 out of 17). The overall rate for completing 6-month follow-up assessment was 70% (35 out of 50), with the intervention group (66.67%, 22 out of 33) and control group (76.47%, 13 out of 17) showing similar retention rates (p = 0.533).
Fig. 1
Flowchart of participants.
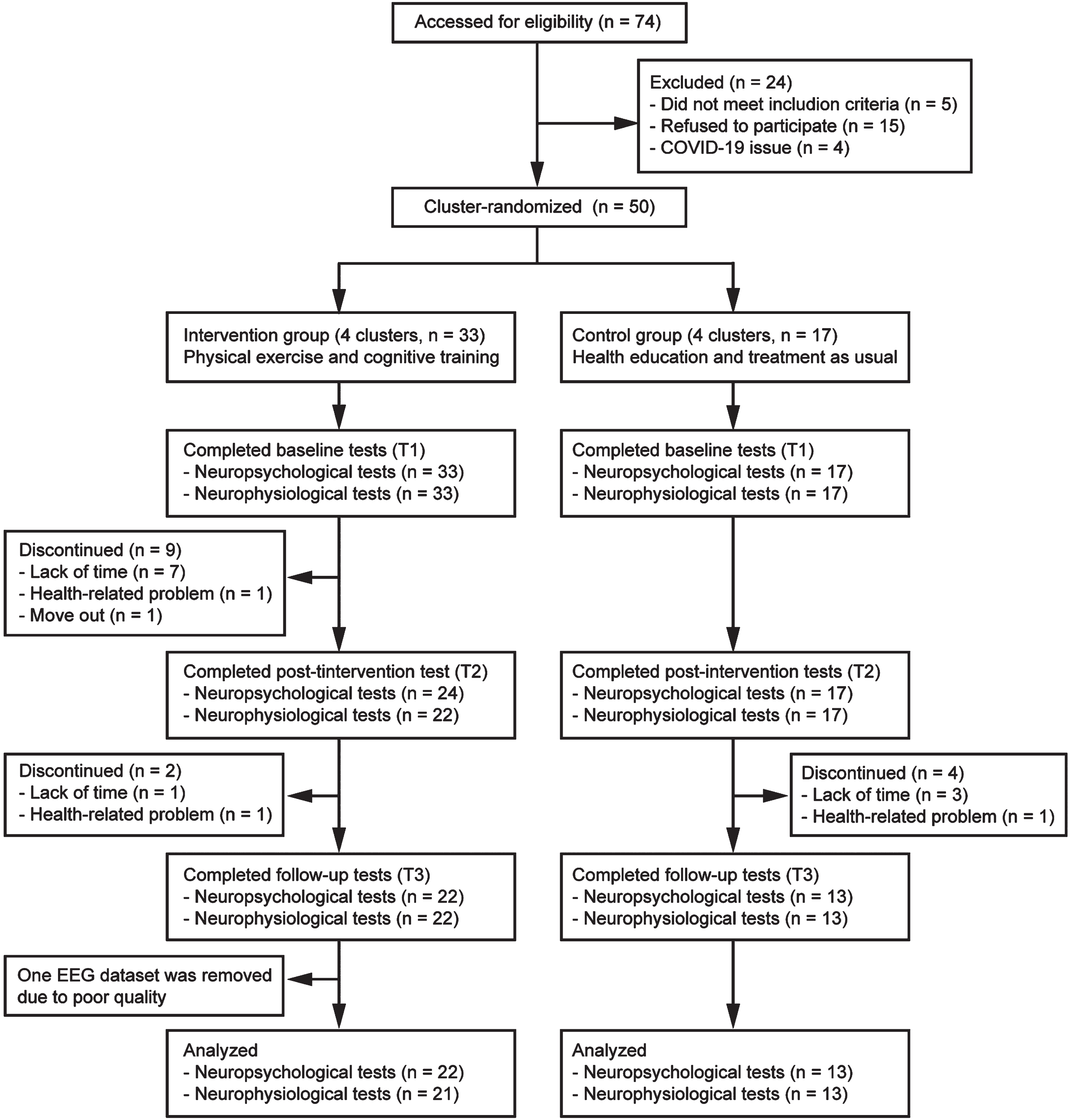
For those who completed the intervention program, the adherence rate was 88.54±2.17 % (42.74 visits out of 48 sessions on average). The majority of subjects in the intervention group self-reported that they were highly satisfied with the program, and no adverse events related to the present study were observed.
Baseline characteristics
The baseline characteristics of all the enrolled participants in each group are summarized in Table 1. There were no significant differences in demographic information (e.g., age, gender distribution, educational attainment, etc.) between intervention and control groups. The general cognitive function, depressive level, and scores of SCD questionnaire were also similar between these two groups. Furthermore, we also provided baseline characteristics of those who completed the whole intervention program (Supplementary Table 1).
Table 1
Baseline characteristics of control and intervention groups
Intervention (n = 33) | Control (n = 17) | ||
Age | 64.82±1.00 | 67.41±1.58 | 0.15 |
Sex (male/female) | 8/25 | 3/14 | 0.73 |
Education | 13.64±0.56 | 11.53±0.90 | 0.07 |
APOE4 (carrier/non-carrier) | 8/25 | 5/12 | 0.74 |
History of hypertension (yes/no)a | 4/29 | 5/12 | 0.24 |
History of diabetes (yes/no)a | 5/27b | 3/14 | 1.00 |
CASI | 93.94±0.72 | 92.88±0.82 | 0.25 |
MMSE | 28.79±0.22 | 28.41±0.32 | 0.30 |
SCD score | 4.39±0.47 | 5.82±0.93 | 0.25 |
GDS | 1.42±0.24 | 1.59±0.57 | 0.66 |
Data are presented as mean±standard error of the mean, unless otherwise specified. APOE4, Apolipoprotein E4; CASI, Cognitive Abilities Screening Instrument; MMSE, Mini-Mental State Examination; SCD, Subjective cognitive decline; GDS, Geriatric Depression Scale. aAll the participants who reported a history of hypertension or diabetes were controlled by medication. bOne subject’s information was missing.
Although we applied a cluster-randomized design, the baseline scores of CASI did not show significant differences among the 4 intervention clusters (Cluster 1 [n = 8] = 91.50±2.18, Cluster 2 [n = 4] = 94.75±1.93, Cluster 3 [n = 12] = 94.33 ±0.83, Cluster 4 [n = 9] = 95.22±0.10; F = 1.37, p = 0.272). These results suggest that the baseline cognitive function of the participants were similar among the intervention clusters.
Primary outcome: neuropsychological function
As shown in Table 2, there were no significant differences in the changes of composite Z scores between intervention and control groups, either for the immediate (i.e., ΔT2–T1, p = 0.234) and sustained (i.e., ΔT3–T1, p = 0.325) effects. The changes of Z scores in each neuropsychological assessment were also not significantly different between intervention and control groups (Supplementary Table 2).
Table 2
Change scores of the primary and secondary outcomes
Intervention group | Control group | p | |
Primary outcome | |||
Cognition (composite Z score) | |||
ΔT2–T1 | 0.05±0.07 | –0.09±0.16 | 0.234 |
ΔT3–T1 | 0.02±0.11 | –0.05±0.12 | 0.325 |
Secondary outcomes | |||
MMN amplitude (uV) | |||
ΔT2–T1 | –0.60±0.38 | 0.71±0.43 | 0.040* |
ΔT3–T1 | 0.10±0.35 | 0.21±0.50 | 0.316 |
MMN latency (ms) | |||
ΔT2–T1 | –35.08±17.76 | 7.95±19.19 | 0.023* |
ΔT3–T1 | –18.10±12.53 | –6.92±19.48 | 0.466 |
Memory-P3 amplitude (uV) | |||
ΔT2–T1 | 0.34±0.94 | 0.36±0.93 | 0.292 |
ΔT3–T1 | 0.41±0.88 | 0.83±0.77 | 0.256 |
Memory-P3 latency (ms) | |||
ΔT2–T1 | 13.97±35.66 | 26.41±18.23 | 0.239 |
ΔT3–T1 | –33.81±29.27 | 15.90±16.76 | 0.037* |
SG | |||
ΔT2–T1 | 0.73±0.56 | 0.25±1.22 | 0.342 |
ΔT3–T1 | –0.19±0.84 | –0.73±0.85 | 0.304 |
Inhibition-P3 amplitude (uV) | |||
ΔT2–T1 | 0.52±0.79 | –0.49±1.58 | 0.479 |
ΔT3–T1 | 1.36±0.64 | –1.46±2.03 | 0.062 |
Inhibition-P3 latency (ms) | |||
ΔT2–T1 | 13.33±22.59 | 20.77±20.12 | 0.111 |
ΔT3–T1 | 4.13±21.56 | 13.08±24.19 | 0.118 |
Data are presented as mean (M)±standard error of the mean (SEM). MMN, Mismatch negativity; SG, Sensory gating; T1, Baseline; T2, End of the intervention; T3, 6 months after the intervention. *p < 0.05.
We additionally assessed the primary outcome across the 4 intervention clusters to evaluate the impact of the cluster sites. The changes of Z scores for the immediate effects (i.e., ΔT2–T1) did not show significant differences (F = 2.23, p = 0.106). The findings suggest that the cluster sites did not have significant effects on the efficacy of the intervention.
Secondary outcomes: neurophysiological responses
Memory-related indicators
Figure 2 shows the grand-averaged MMN waveforms from T1 to T3 in the intervention and control groups. There were no significant differences in trial numbers of deviants between control and intervention groups at T1 (intervention = 116.05±1.71, control = 119.08±0.52, p = 0.182), T2 (intervention = 119.24±0.37, control = 115.08±2.71,p = 0.153), and T3 (intervention = 118.0±0.49, control = 117.08±1.56, p = 0.581). For the control group, the MMN responses were quite similar among 3 measurements; however, for the intervention group, the MMN amplitudes were larger and the MMN latencies were shortened in the T2 compared to those in the T1. The statistical results confirmed that the intervention group showed significant enhancement of MMN amplitudes compared to the control group (ΔT2–T1: intervention=–0.60±0.38 uV, control = 0.71±0.43 uV, p = 0.040). The intervention group also showed significant shortening of MMN latencies compared to the control group (ΔT2–T1: intervention=–35.08±17.76 ms, control = 7.95±19.19 ms, p = 0.023). However, such a beneficial effect did not sustain to T3 (Table 2).
Fig. 2
A) Grand-averaged waveforms of mismatch negativity (MMN) at Fz electrode in the intervention (n = 21) and control (n = 13) groups. The black, blue, and red traces indicate MMN activities at the baseline (T1), the end of the intervention (T2), and 6 months after the end of the intervention (T3), respectively. The topographic maps of peak MMN latencies at T1, T2, and T3 in each group are also illustrated. B) Larger MMN amplitudes (indicated by more negative values) and shortened MMN latencies (also indicated by more negative values) of ΔT2–T1 were observed in the intervention group as compared to the control group. The MMN amplitudes and latencies of ΔT3–T1 were not significantly different between these two groups.
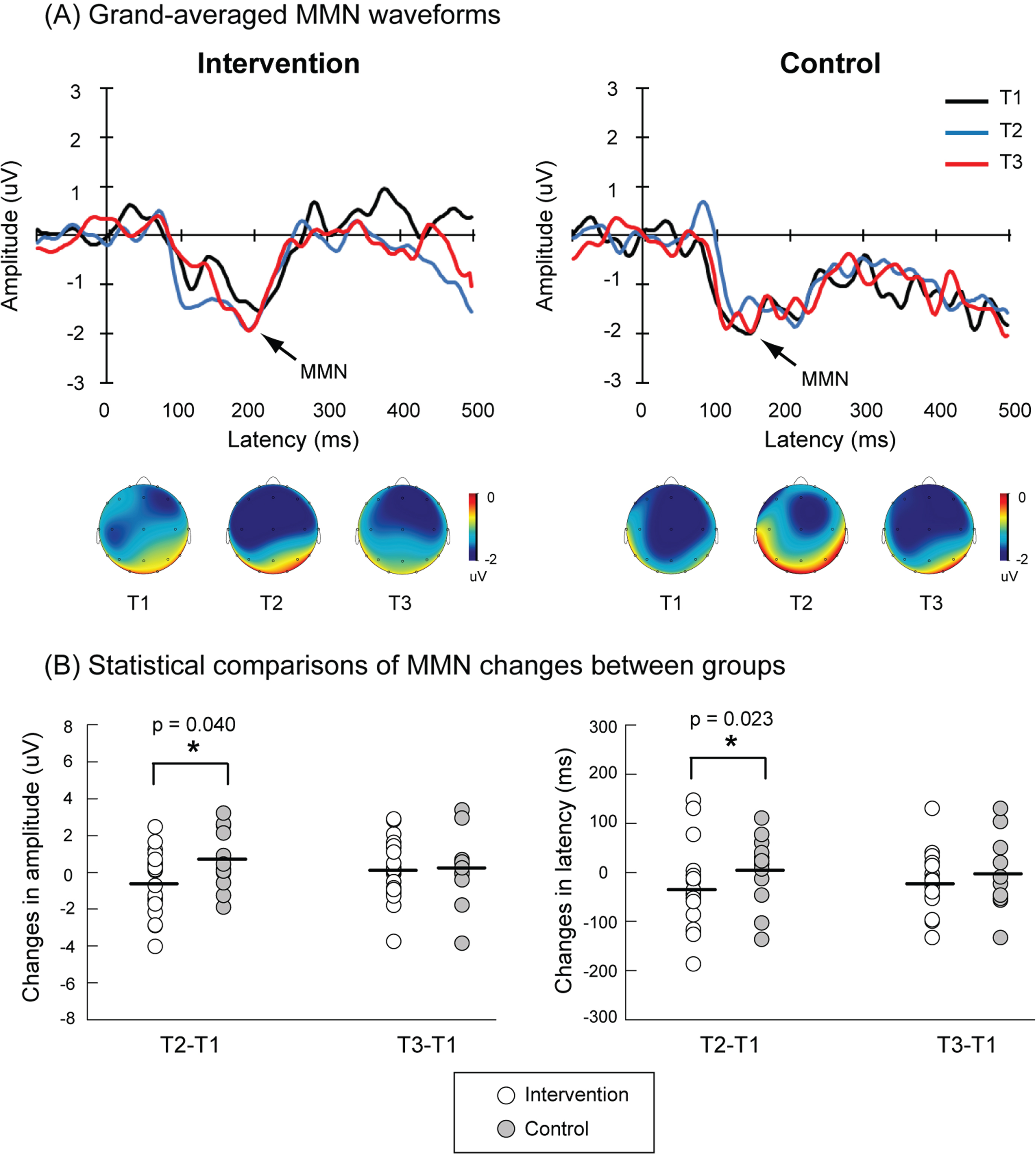
The accuracy rates did not significantly differ between intervention and control groups at T1, T2, and T3 (Supplementary Table 3). Figure 3 shows the grand-averaged ERPs to successful targets in the 1-back paradigm from T1 to T3. There were no significant differences in trial numbers of successful targets between control and intervention groups at T1 (intervention = 66.52±2.65, control = 65.0±2.62, p = 0.703), T2 (intervention = 70.86±1.21, control = 70.62±0.94, p = 0.888), and T3 (intervention = 66.48±0.94, control = 69.23±1.37, p = 0.327). Although the combined physical exercise and cognitive training did not produce immediate effects on the memory-P3 responses (Table 2), this multimodal intervention yielded a sustained improvement of memory-P3 latencies compared to the control group (ΔT3–T1: intervention=–33.81±29.27 ms, control = 15.90±16.76 ms, p = 0.037).
Fig. 3
A) Grand-averaged waveforms of P3 responses to the targets (i.e., memory-P3) during a 1-back task at Pz electrode in the intervention (n = 21) and control (n = 13) groups. The black, blue, and red traces indicate memory-P3 activities at the baseline (T1), the end of the intervention (T2), and 6 months after the end of the intervention (T3), respectively. The topographic maps of peak memory-P3 latencies at T1, T2, and T3 in each group are also illustrated. B) Significantly shortened memory-P3 latencies of ΔT3–T1 were observed in the intervention group as compared to the control group.
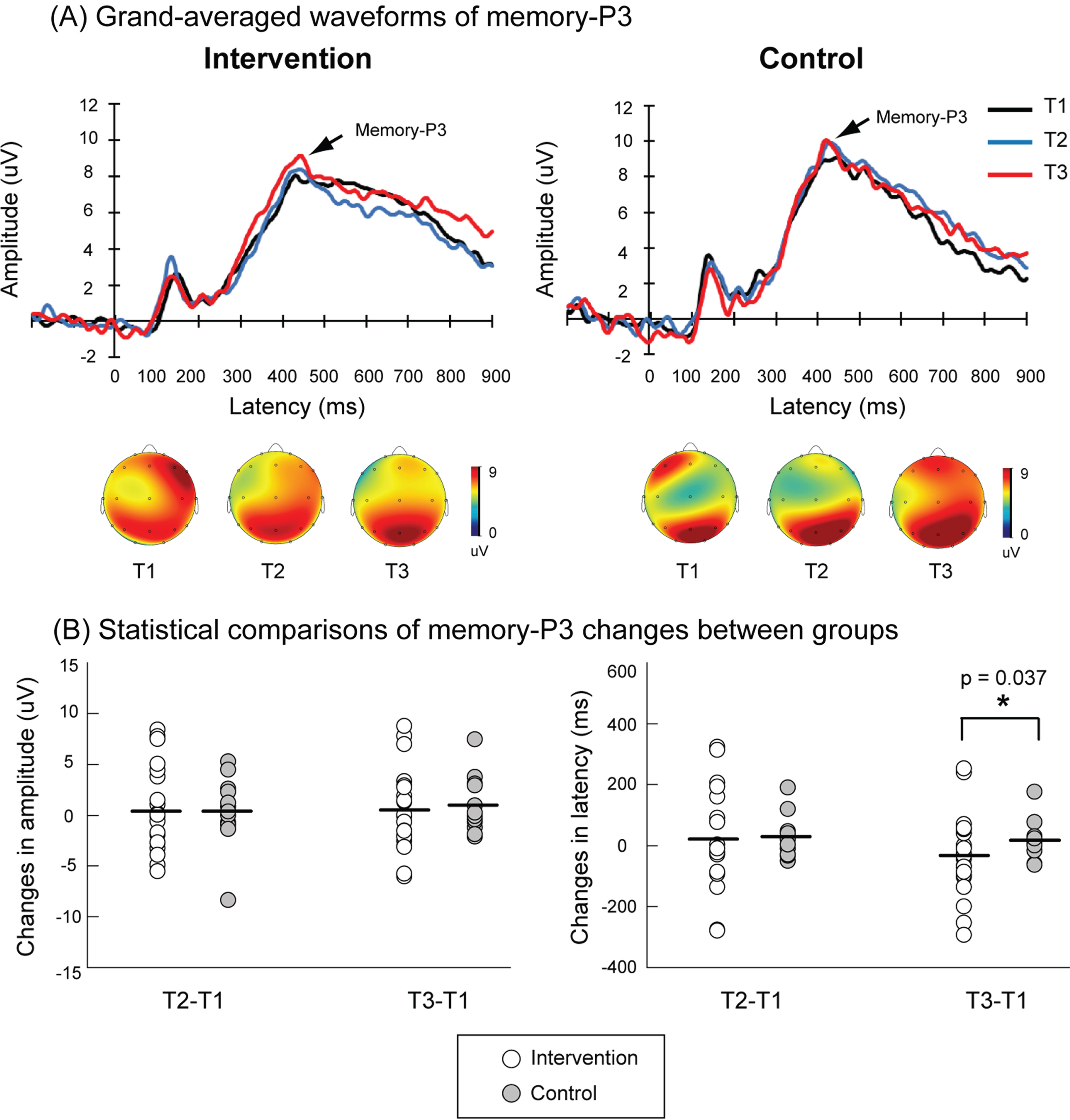
Inhibition-related indicators
There were no significant differences in SG function between intervention and control groups, either for the immediate or sustained effects (Table 2). For the trial number in the paired-stimulus paradigm, there were also no significant differences between control and intervention groups at T1 (intervention = 77.33±1.28, control = 79.08±0.43, p = 0.305), T2 (intervention = 78.95±0.76, control = 79.23±0.43, p = 0.788), and T3 (intervention = 79.48±0.31, control = 79.15±0.48, p = 0.555). The grand-averaged ERPs of SG were provided in the Supplementary Figure 1.
The accuracy rates for Go and Nogo trials did not significantly differ between intervention and control groups at T1, T2, and T3 (Supplementary Table 4). There were no significant differences in inhibition-P3 responses between intervention and control groups, either for the immediate or sustained effects (Table 2). For the trial number of Nogo stimuli in the Go-Nogo task, there were no significant differences between control and intervention groups at T1 (intervention = 73.90±4.03, control = 81.38±3.32, p = 0.203), T2 (intervention = 80.67±3.31, control = 82.38±2.94, p = 0.724), and T3 (intervention = 82.0±3.26, control = 76.54±4.39, p = 0.319). The grand-averaged ERPs of inhibition-P3 were provided in the Supplementary Figure 2.
Associations between neuropsychological and neurophysiological measures
Since the significant effects of intervention were found in MMN (i.e., amplitude and latency of ΔT2–T1) and memory-P3 (i.e., latency of ΔT3–T1) responses, we further determined whether the selective improvement in memory-related ERPs would be associated with beneficial changes in neuropsychological performance. The results showed neither ΔMMN amplitudes nor ΔMMN latencies were associated with change scores in neuropsychological tests after the correction for multiple comparisons. However, Δmemory-P3 latencies were associated with ΔLM_Immediate (partial r=–0.576, p = 0.006) and ΔLM_Delayed (partial r=–0.514, p = 0.015). After the corrections, Δmemory-P3 latencies were significantly and negatively correlated with only ΔLM_Immediate (partial r=–0.576, p = 0.006, significant after Benjamini– Hochberg procedure with FDR = 0.042), suggesting that more shortening of memory-P3 latencies after the intervention was concomitant with more gains in episodic memory function (Fig. 4).
Fig. 4
Left: Among the SCD subjects in the intervention group, more shortening of the memory-P3 latencies from T1 to T3 was significantly associated with more gains in raw scores of LM_Immediate from T1 to T3 (partial r = -0.576, p = 0.006, FDR = 0.042). Right: A similar pattern was also observed in the association between changes in memory-P3 latencies and changes in raw scores of LM_Delayed. However, the significance did not survive after the correction for multiple comparisons though a trend-level of significance (p = 0.015, FDR = 0.053) was detected. SCD, subjective cognitive decline; LM, Logic Memory Test Part A of the Wechsler Memory Scale; FDR, false discovery rate.
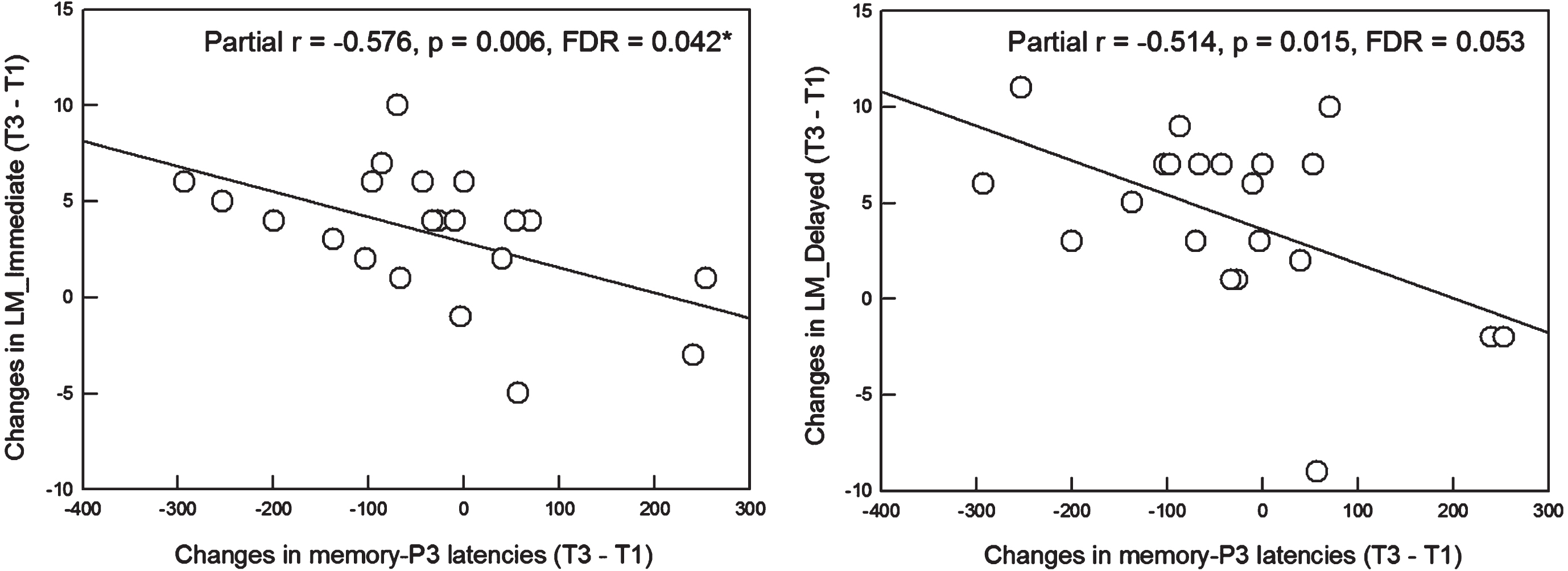
In addition to the per-protocol analysis, the results of primary and secondary outcomes were analyzed following the intention-to-treat analysis to address the higher drop-out rate in the intervention group (Supplementary Table 5).
DISCUSSION
This was the first study to determine the effects of 6-month combined physical exercise and cognitive training on neuropsychological and neurophysiological performance in community-dwelling older adults with SCD. Our findings showed that although there were no significant differences in neuropsychological performance between control and intervention groups, the multidomain intervention resulted in improved brain activities specifically related to memory function (i.e., MMN and memory-P3) as compared to the control group. Moreover, more shortening of memory-P3 latencies from T1 to T3 was associated with higher score changes of LM_Immediate from T1 to T3.
In contrast to our hypothesis, the current results showed that a 6-month multidomain intervention did not significantly improve composite Z scores of neuropsychological assessments (i.e., primary outcome) as compared to the control group. These findings were consistent with previous large-scale RCT studies on SCD subjects, such as MAPT [7], MEDEX [8], and PONDER [6], though the content of these multidomain interventions slightly differed from one another. However, the FINGER, which was the largest RCT study of multidomain intervention on at-risk elderly, showed that older adults receiving a 2-year multidomain intervention had improved cognitive performance, as indexed by a composite Z score based on the results of several cognitive tests, than those receiving general health advice [10]. One of the major reasons that could account for the inconsistent results was the intervention intensity and/or duration of the training protocol. For example, in the MAPT trial, the physical exercise and cognitive training was performed 2 hours/session, 12 sessions for the first 2 months, followed by 1 hour/session, 1 session/month for the remainder of 3 years. The intensity of FINGER trial was much higher: the physical exercise consisted of muscle strength training (1-3 sessions/week) and aerobic training (60-90 mins/session, 2-5 sessions/week) for 2 years; the cognitive training consisted of 10 group sessions and 144 individual sessions (10-15 mins/session, 3 sessions/week using web-based in-house developed computer program) for 1 year. Therefore, we reasoned that the lack of cognitive improvement in the present study was likely due to lower intensity and/or shorter duration of the training program. Nevertheless, it is worth noting that several multidomain intervention studies (although with slightly different training components compare to the present study) conducted with a shorter training period (e.g., equal to or less than 6 months) have demonstrated improvements in neuropsychological performance for older adults with SCD or at risk of dementia [9, 12, 47].
Despite no significant intervention effect on the neuropsychological performance in older adults with SCD, the secondary outcomes demonstrated selective improvements in neural activities after the 6-month multidomain intervention as compared to the control group. These improved ERPs indicators were particularly related to memory function (i.e., MMN and memory-P3 in the present study). Our previous observational study showed a reduction of MMN amplitude in older adults with SCD as compared to those without SCD [48], suggesting a deficit in auditory sensory memory and/or involuntary attention switch in SCD. The current work further demonstrated that individuals with SCD who received 6-month physical exercise and cognitive training showed enhanced MMN function compared to those who received treatment as usual. This enhancement was evident by larger MMN amplitudes and shortened MMN latencies. MMN is considered as an electrophysiological marker that reflects a pre-attentive ability of the brain in detecting the irregularities of the environment. The existing literature indicated that the neural generators of MMN located in the superior temporal gyrus (STG), inferior frontal gyrus, and/or inferior parietal lobule [17, 18]. Predictive coding hypothesis posits that the representation of acoustic regularities, formed by the standard stimuli and stored in the STG, builds the prediction of upcoming stimuli; the violation of sound regularities (i.e., the occurrence of deviant stimuli), would lead to prediction error and then elicit MMN responses [67, 68]. A series of our research works indicated that MMN was not only an objective indicator of SCD, but also a potential outcome measure that could reflect the treatment effects in SCD. However, such a beneficial effect was not retained at the follow-up period, suggesting the sustained effect was not easily achieved without continuous training.
Our results also showed that the memory-P3 latencies of ΔT3–T1 were shortened in the intervention group as compared to those in the control group. The shortening of memory-P3 latencies or the increase of memory-P3 amplitudes suggest improved working memory performance. Unlike MMN that reflects a pre-attentive response underlying sensory-memory mechanism, P3 responses to successful targets in the 1-back task has been associated with the top-down evaluation of ongoing context and working memory operations [59, 69]. The beneficial effect of multidomain intervention on memory-P3 latencies was observed at T3 rather than T2, suggesting that the relatively top-down memory function, compared to pre-attentive MMN, required longer time to accumulate training effects and then led to a reorganization of brain function. It should also be noted that Δmemory-P3 latencies had a significant and a trend-level associations with ΔLM_Immediate (adjusted p = 0.042) and ΔLM_Delayed (adjusted p = 0.053), respectively (Fig. 4). Our results provided a brain-and-behavior link showing that more shortening of memory-P3 latencies after the intervention was concomitant with more gains in episodic memory.
Although the neurophysiological indicators (i.e., secondary outcomes) could reflect the intervention effects in older adults with SCD, the neuropsychological assessments relevant for determination of cognitive impairments (i.e., primary outcome) failed to show improvement after a multimodal intervention in the present study. We reasoned that there were three interpretations to account for these findings. The first one was related to the intensity and/or duration of the intervention program, as discussed above. The second one was that we only recruited older adults with SCD whose cognitive abilities were within normal range. Thus, it might be challenging to detect significant enhancements in neuropsychological performance among these cognitively-normal older subjects. However, this does not imply that the effectiveness of this intervention program is limited to other populations. Previous studies have shown that the combination of physical exercise and cognitive training improved neuropsychological performance in older adults with MCI or at risk of dementia [40, 70–72]. The third one was that neuropsychological and neurophysiological outcomes might potentially represent different training targets for the evaluation of this intervention program, particularly in the cognitively-normal older adults with SCD. This was the first study that applied both neuropsychological and neurophysiological assessments as outcome measures in the evaluation of the effectiveness of multidomain intervention in SCD. Thus, this hypothesis needs further verification in the futurestudies.
Several limitations should be considered in the present study. First, the sample size was relatively small and thus the results might be underpowered. Second, the control group in the present study received treatment as usual. The lack of active control might overestimate the magnitude of changes in outcome measures. Third, the cluster-randomization design in the present study might lead to unsymmetrical distribution between subsamples. The main reason why we applied randomization at the clustered level, rather than at the individual level, was that this design overcame the traveling issue for the participants. All the intervention programs took place in the community centers, which are within walking-distance from the participants’ living place. Although we made this preparation in advance, the retention rate was still lower in the intervention group than in the control group from T1 to T2. Furthermore, the intervention was suspended for 6 weeks in one of the clusters due to the COVID-19 pandemic. Though the missing sessions were provided, the training effects might be compromised since the training effect was interrupted. Fourth, we did not know whether the participants, either in the control or intervention group, received other training activities. This might be a potential bias among the 8 studying clusters. Finally, the follow-up time of 6 months might be too short to observe the sustained effects of intervention on older adults with SCD. A previous meta-analysis study suggested that the follow-up of non-pharmacological intervention in SCD should be at least 1-year or longer [14]. One of the major reasons was that the training-induced brain plasticity might occur on a longer time scale in older adults as compared to younger counterparts [73, 74]. The lack of significant differences in the neuropsychological function might be attributed to the aforementioned reason.
In conclusion, this 6-month multidomain intervention on the community-dwelling older adults with SCD demonstrated selective improvement in neural activities particularly related to the memory domain. However, the effects of multidomain intervention on the neuropsychological function warrant further in-depth investigation (e.g., personalized training program, longer follow-up period, biochemical/neural mechanisms underlying cognitive changes).
AUTHOR CONTRIBUTIONS
Chia-Hsiung Cheng (Conceptualization; Formal analysis; Funding acquisition; Investigation; Methodology; Resources; Supervision; Writing – original draft; Writing – review & editing); Yu-Wei Hsieh (Formal analysis; Investigation; Methodology; Writing – review & editing); Chiung-Chih Chang (Methodology; Resources; Writing – review & editing); Fu-Jung Hsiao (Formal analysis; Methodology; Writing – review & editing); Li-Fen Chen (Resources; Writing – review & editing); Pei-Ning Wang (Resources; Writing – review & editing).
ACKNOWLEDGMENTS
During the preparation of this work, the author used ChatGPT in order to improve language and readability. After using this tool, the authors reviewed and edited the content as needed and take full responsibility for the content of the publication.
FUNDING
This work was supported by Chang Gung Memorial Hospital (CMRPD1K0581, CMRPD1M0741), Chang Gung University (BMRPE25), Healthy Aging Research Center, Chang Gung University (URRPD1P0131), Ministry of Science and Technology (MOST-108-2628-B-182-002, MOST-109-2628-B-182-012, MOST-110-2628-B-182-010), and National Science and Technology Council (NSTC-112-2410-H-182-030), Taiwan.
CONFLICT OF INTEREST
The authors have no conflict of interest to report.
DATA AVAILABILITY
Data available on request from the corresponding author with reasonable reasons.
SUPPLEMENTARY MATERIAL
[1] The supplementary material is available in the electronic version of this article: https://dx.doi.org/10.3233/JAD-231257.
REFERENCES
[1] | Jessen F , Amariglio RE , Buckley RF , van der Flier WM , Han Y , Molinuevo JL , Rabin L , Rentz DM , Rodriguez-Gomez O , Saykin AJ , Sikkes SAM , Smart CM , Wolfsgruber S , Wagner M ((2020) ) The characterisation of subjective cognitive decline. Lancet Neurol 19: , 271–278. |
[2] | Jessen F , Amariglio RE , van Boxtel M , Breteler M , Ceccaldi M , Chetelat G , Dubois B , Dufouil C , Ellis KA , van der Flier WM , Glodzik L , van Harten AC , de Leon MJ , McHugh P , Mielke MM , Molinuevo JL , Mosconi L , Osorio RS , Perrotin A , Petersen RC , Rabin LA , Rami L , Reisberg B , Rentz DM , Sachdev PS , de la Sayette V , Saykin AJ , Scheltens P , Shulman MB , Slavin MJ , Sperling RA , Stewart R , Uspenskaya O , Vellas B , Visser PJ , Wagner M ((2014) ) A conceptual framework for research on subjective cognitive decline in preclinical Alzheimer’s disease. Alzheimers Dement 10: , 844–852. |
[3] | Buckley RF , Maruff P , Ames D , Bourgeat P , Martins RN , Masters CL , Rainey-Smith S , Lautenschlager N , Rowe CC , Savage G , Villemagne VL , Ellis KA ((2016) ) Subjective memory decline predicts greater rates of clinical progression in preclinical Alzheimer’s disease. Alzheimers Dement 12: , 796–804. |
[4] | Koppara A , Wagner M , Lange C , Ernst A , Wiese B , Konig HH , Brettschneider C , Riedel-Heller S , Luppa M , Weyerer S , Werle J , Bickel H , Mosch E , Pentzek M , Fuchs A , Wolfsgruber S , Beauducel A , Scherer M , Maier W , Jessen F ((2015) ) Cognitive performance before and after the onset of subjective cognitive decline in old age. Alzheimers Dement (Amst) 1: , 194–205. |
[5] | Reisberg B , Shulman MB , Torossian C , Leng L , Zhu W ((2010) ) Outcome over seven years of healthy adults with and without subjective cognitive impairment. Alzheimers Dement 6: , 11–24. |
[6] | Macpherson H , Brownell S , Harris E , Duckham RL , O’Connell S , Meyer BJ , Mirzaee S , Daly RM ((2022) ) Effects of a 6-month multifaceted diet and exercise intervention on cognition in older adults at risk of cognitive decline: The PONDER double-blind, placebo-controlled randomized trial. J Alzheimers Dis 89: , 247–263. |
[7] | Andrieu S , Guyonnet S , Coley N , Cantet C , Bonnefoy M , Bordes S , Bories L , Cufi MN , Dantoine T , Dartigues JF , Desclaux F , Gabelle A , Gasnier Y , Pesce A , Sudres K , Touchon J , Robert P , Rouaud O , Legrand P , Payoux P , Caubere JP , Weiner M , Carrie I , Ousset PJ , Vellas B ((2017) ) Effect of long-term omega 3 polyunsaturated fatty acid supplementation with or without multidomain intervention on cognitive function in elderly adults with memory complaints (MAPT): A randomised, placebo-controlled trial. Lancet Neurol 16: , 377–389. |
[8] | Lenze EJ , Voegtle M , Miller JP , Ances BM , Balota DA , Barch D , Depp CA , Diniz BS , Eyler LT , Foster ER , Gettinger TR , Head D , Hershey T , Klein S , Nichols JF , Nicol GE , Nishino T , Patterson BW , Rodebaugh TL , Schweiger J , Shimony JS , Sinacore DR , Snyder AZ , Tate S , Twamley EW , Wing D , Wu GF , Yang L , Yingling MD , Wetherell JL ((2022) ) Effects of mindfulness training and exercise on cognitive function in older adults: A randomized clinical trial. JAMA 328: , 2218–2229. |
[9] | McMaster M , Kim S , Clare L , Torres SJ , Cherbuin N , D’Este C , Anstey KJ ((2020) ) Lifestyle risk factors and cognitive outcomes from the multidomain dementia risk reduction randomized controlled trial, Body Brain Life for Cognitive Decline (BBL-CD). J Am Geriatr Soc 68: , 2629–2637. |
[10] | Ngandu T , Lehtisalo J , Solomon A , Levalahti E , Ahtiluoto S , Antikainen R , Backman L , Hanninen T , Jula A , Laatikainen T , Lindstrom J , Mangialasche F , Paajanen T , Pajala S , Peltonen M , Rauramaa R , Stigsdotter-Neely A , Strandberg T , Tuomilehto J , Soininen H , Kivipelto M ((2015) ) A 2 year multidomain intervention of diet, exercise, cognitive training, and vascular risk monitoring versus control to prevent cognitive decline in at-risk elderly people (FINGER): A randomised controlled trial. Lancet 385: , 2255–2263. |
[11] | Liu X , Ma Z , Zhu X , Zheng Z , Li J , Fu J , Shao Q , Han X , Wang X , Wang Z , Yin Z , Qiu C ((2023) ) Cognitive benefit of a multidomain intervention for older adults at risk of cognitive decline: A cluster-randomized controlled trial. Am J Geriatr Psychiatry 31: , 197–209. |
[12] | Chatterjee P , Kumar DA , Naqushbandi S , Chaudhary P , Khenduja P , Madan S , Fatma S , Khan MA , Singh V ((2022) ) Effect of Multimodal Intervention (computer based cognitive training, diet and exercise) in comparison to health awareness among older adults with Subjective Cognitive Impairment (MISCI-Trial)-A Pilot Randomized Control Trial. PLoS One 17: , e0276986. |
[13] | Smart CM , Segalowitz SJ , Mulligan BP , Koudys J , Gawryluk JR ((2016) ) Mindfulness training for older adults with subjective cognitive decline: Results from a pilot randomized controlled trial. J Alzheimers Dis 52: , 757–774. |
[14] | Smart CM , Karr JE , Areshenkoff CN , Rabin LA , Hudon C , Gates N , Ali JI , Arenaza-Urquijo EM , Buckley RF , Chetelat G , Hampel H , Jessen F , Marchant NL , Sikkes SAM , Tales A , van der Flier WM , Wesselman L ((2017) ) Non-pharmacologic interventions for older adults with subjective cognitive decline: Systematic review, meta-analysis, and preliminary recommendations. Neuropsychol Rev 27: , 245–257. |
[15] | Zanto TP , Gazzaley A ((2019) ) Aging of the frontal lobe. Handb Clin Neurol 163: , 369–389. |
[16] | Gazzaley A , Cooney JW , Rissman J , D’Esposito M ((2005) ) Top-down suppression deficit underlies working memory impairment in normal aging. Nat Neurosci 8: , 1298–1300. |
[17] | Naatanen R , Kujala T , Kreegipuu K , Carlson S , Escera C , Baldeweg T , Ponton C ((2011) ) The mismatch negativity: An index of cognitive decline in neuropsychiatric and neurological diseases and in ageing. Brain 134: , 3435–3453. |
[18] | Naatanen R , Kujala T , Escera C , Baldeweg T , Kreegipuu K , Carlson S , Ponton C ((2012) ) The mismatch negativity (MMN)–a unique window to disturbed central auditory processing in ageing and different clinical conditions. Clin Neurophysiol 123: , 424–458. |
[19] | Constantinidis C , Klingberg T ((2016) ) The neuroscience of working memory capacity and training. Nat Rev Neurosci 17: , 438–449. |
[20] | Baddeley A ((1992) ) Working memory. Science 255: , 556–559. |
[21] | Nikolin S , Tan YY , Martin D , Moffa A , Loo CK , Boonstra TW ((2021) ) Behavioural and neurophysiological differences in working memory function of depressed patients and healthy controls. J Affect Disord 295: , 559–568. |
[22] | Breitling C , Zaehle T , Dannhauer M , Tegelbeckers J , Flechtner HH , Krauel K ((2020) ) Comparison between conventional and HD-tDCS of the right inferior frontal gyrus in children and adolescents with ADHD. Clin Neurophysiol 131: , 1146–1154. |
[23] | Cheng CH , Chan PS , Liu CY , Hsu SC ((2016) ) Auditory sensory gating in patients with bipolar disorders: A meta-analysis. J Affect Disord 203: , 199–203. |
[24] | Sun HH , Lin MY , Nouchi R , Wang PN , Cheng CH ((2021) ) Neuromagnetic evidence of abnormal automatic inhibitory function in subjective memory complaint. Eur J Neurosci 53: , 3350–3361. |
[25] | Dierolf AM , Fechtner J , Bohnke R , Wolf OT , Naumann E ((2017) ) Influence of acute stress on response inhibition in healthy men: An ERP study. Psychophysiology 54: , 684–695. |
[26] | Cheng CH , Tsai HY , Cheng HN ((2019) ) The effect of age on N2 and P3 components: A meta-analysis of Go/Nogo tasks. Brain Cogn 135: , 103574. |
[27] | Lin YP , Liang HY , Chen YS , Lu CH , Wu YR , Chang YY , Lin WC ((2021) ) Objective assessment of impulse control disorder in patients with Parkinson’s disease using a low-cost LEGO-like EEG headset: A feasibility study. J Neuroeng Rehabil 18: , 109. |
[28] | Barry RJ , De Blasio FM , Borchard JP ((2014) ) Sequential processing in the equiprobable auditory Go/NoGo task: Children vs. adults. Clin Neurophysiol 125: , 1995–2006. |
[29] | Barry RJ , De Blasio FM , Cave AE ((2016) ) Sequential processing in young and older adults in the equiprobable auditory Go/NoGo task. Clin Neurophysiol 127: , 2273–2285. |
[30] | Smith JL , Johnstone SJ , Barry RJ ((2008) ) Movement-related potentials in the Go/NoGo task: The P3 reflects both cognitive and motor inhibition. Clin Neurophysiol 119: , 704–714. |
[31] | Bruin KJ , Wijers AA ((2002) ) Inhibition, response mode, and stimulus probability: A comparative event-related potential study. Clin Neurophysiol 113: , 1172–1182. |
[32] | Messerotti Benvenuti S , Sarlo M , Buodo G , Mento G , Palomba D ((2015) ) Influence of impulsiveness on emotional modulation of response inhibition: An ERP study. Clin Neurophysiol 126: , 1915–1925. |
[33] | Jia RX , Liang JH , Xu Y , Wang YQ ((2019) ) Effects of physical activity and exercise on the cognitive function of patients with Alzheimer disease: A meta-analysis. BMC Geriatr 19: , 181. |
[34] | Gavelin HM , Dong C , Minkov R , Bahar-Fuchs A , Ellis KA , Lautenschlager NT , Mellow ML , Wade AT , Smith AE , Finke C , Krohn S , Lampit A ((2021) ) Combined physical and cognitive training for older adults with and without cognitive impairment: A systematic review and network meta-analysis of randomized controlled trials. Ageing Res Rev 66: , 101232. |
[35] | Hillman CH , Erickson KI , Kramer AF ((2008) ) Be smart, exercise your heart: Exercise effects on brain and cognition. Nat Rev Neurosci 9: , 58–65. |
[36] | Burdette JH , Laurienti PJ , Espeland MA , Morgan A , Telesford Q , Vechlekar CD , Hayasaka S , Jennings JM , Katula JA , Kraft RA , Rejeski WJ ((2010) ) Using network science to evaluate exercise-associated brain changes in older adults. Front Aging Neurosci 2: , 23. |
[37] | Hakansson K , Ledreux A , Daffner K , Terjestam Y , Bergman P , Carlsson R , Kivipelto M , Winblad B , Granholm AC , Mohammed AK ((2017) ) BDNF responses in healthy older persons to 35 minutes of physical exercise, cognitive training, and mindfulness: Associations with working memory function. J Alzheimers Dis 55: , 645–657. |
[38] | Kennedy G , Hardman RJ , Macpherson H , Scholey AB , Pipingas A ((2017) ) How does exercise reduce the rate of age-associated cognitive decline? A review of potential mechanisms. J Alzheimers Dis 55: , 1–18. |
[39] | Herold F , Hamacher D , Schega L , Muller NG ((2018) ) Thinking while moving or moving while thinking - concepts of motor-cognitive training for cognitive performance enhancement. Front Aging Neurosci 10: , 228. |
[40] | Chuang IC , Chen IC , Su KH , Wu YR , Wu CY ((2023) ) The effects of high versus low frequency of combined physical and cognitive training on cognitive function in older adults with cognitive decline: A quasi-experimental study. BMC Geriatr 23: , 94. |
[41] | Yeh TT , Chang KC , Wu CY , Chen CJ , Chuang IC ((2022) ) Clinical efficacy of aerobic exercise combined with computer-based cognitive training in stroke: A multicenter randomized controlled trial. Top Stroke Rehabil 29: , 255–264. |
[42] | Donohue MC , Sperling RA , Salmon DP , Rentz DM , Raman R , Thomas RG , Weiner M , Aisen PS ((2014) ) The preclinical Alzheimer cognitive composite: Measuring amyloid-related decline. JAMA Neurol 71: , 961–970. |
[43] | Barnes DE , Santos-Modesitt W , Poelke G , Kramer AF , Castro C , Middleton LE , Yaffe K ((2013) ) The Mental Activity and eXercise (MAX) trial: A randomized controlled trial to enhance cognitive function in older adults. JAMA Intern Med 173: , 797–804. |
[44] | Lautenschlager NT , Cox KL , Flicker L , Foster JK , van Bockxmeer FM , Xiao J , Greenop KR , Almeida OP ((2008) ) Effect of physical activity on cognitive function in older adults at risk for Alzheimer disease: A randomized trial. JAMA 300: , 1027–1037. |
[45] | Bhome R , Berry AJ , Huntley JD , Howard RJ ((2018) ) Interventions for subjective cognitive decline: Systematic review and meta-analysis. BMJ Open 8: , e021610. |
[46] | Pang Y , Kim O ((2021) ) Effects of smartphone-based compensatory cognitive training and physical activity on cognition, depression, and self-esteem in women with subjective cognitive decline. Brain Sci 11: , 1029. |
[47] | Park JE , Jeon SY , Kim SA , Kim JH , Kim SH , Lee KW , Hwang YJ , Jung G , Suk HW , Park S , Lee DY ((2019) ) A multidomain intervention for modifying lifestyle habits reduces the dementia risk in community-dwelling older adults: A single-blinded randomized controlled pilot study. J Alzheimers Dis 70: , 51–60. |
[48] | Cheng CH , Chang CC , Chao YP , Lu H , Peng SW , Wang PN ((2021) ) Altered mismatch response precedes gray matter atrophy in subjective cognitive decline. Psychophysiology 58: , e13820. |
[49] | Cheng CH , Wang PN , Mao HF , Hsiao FJ ((2020) ) Subjective cognitive decline detected by the oscillatory connectivity in the default mode network: A magnetoencephalographic study. Aging (Albany NY) 12: , 3911–3925. |
[50] | Lin KN , Wang PN , Liu HC , Teng EL ((2012) ) [Cognitive Abilities Screening Instrument, Chinese Version 2.0 (CASI C-2.0): Administration and clinical application]. Acta Neurol Taiwan 21: , 180–189. |
[51] | Chang CC , Kramer JH , Lin KN , Chang WN , Wang YL , Huang CW , Lin YT , Chen C , Wang PN ((2010) ) Validating the Chinese version of the Verbal Learning Test for screening Alzheimer’s disease. J Int Neuropsychol Soc 16: , 244–251. |
[52] | Yang SH , Wang PN , Cheng CH ((2019) ) Altered auditory repetition suppression and MMNm in relation to cognitive tests in older adults. Biol Psychol 146: , 107725. |
[53] | Cheng CH , Hsiao FJ , Hsieh YW , Wang PN ((2020) ) Dysfunction of inferior parietal lobule during sensory gating in patients with amnestic mild cognitive impairment. Front Aging Neurosci 12: , 39. |
[54] | Takayama Y ((2010) ) A delayed recall battery as a sensitive screening for mild cognitive impairment: Follow-up study of memory clinic patients after 10 years. J Med Dent Sci 57: , 177–184. |
[55] | Muangpaisan W , Intalapaporn S , Assantachai P ((2010) ) Digit span and verbal fluency tests in patients with mild cognitive impairment and normal subjects in Thai-community. J Med Assoc Thai 93: , 224–230. |
[56] | Arbuthnott K , Frank J ((2000) ) Trail making test, part B as a measure of executive control: Validation using a set-switching paradigm. J Clin Exp Neuropsychol 22: , 518–528. |
[57] | Kortte KB , Horner MD , Windham WK ((2002) ) The trail making test, part B: Cognitive flexibility or ability to maintain set? Appl Neuropsychol 9: , 106–109. |
[58] | Chen PY , Hsu HY , Chao YP , Nouchi R , Wang PN , Cheng CH ((2021) ) Altered mismatch response of inferior parietal lobule in amnestic mild cognitive impairment: A magnetoencephalographic study. CNS Neurosci Ther 27: , 1136–1145. |
[59] | Polich J ((2007) ) Updating P300: An integrative theory of P3a and P3b. Clin Neurophysiol 118: , 2128–2148. |
[60] | Jian JR , Lin YY , Connor LT , Cheng CH ((2022) ) Revisiting the relationship between neural correlates of sensory gating and self-reported sensory gating inventory: An MEG investigation. Neurosci Lett 766: , 136336. |
[61] | Lin MY , Tseng YJ , Cheng CH ((2018) ) Age effects on spatiotemporal dynamics of response inhibition: An MEG study. Front Aging Neurosci 10: , 386. |
[62] | Susana CF , Monica L , Fernando D ((2021) ) Event-related brain potential indexes provide evidence for some decline in healthy people with subjective memory complaints during target evaluation and response inhibition processing. Neurobiol Learn Mem 182: , 107450. |
[63] | Delorme A , Makeig S ((2004) ) EEGLAB: An open source toolbox for analysis of single-trial EEG dynamics including independent component analysis. J Neurosci Methods 134: , 9–21. |
[64] | Fuerst DR , Gallinat J , Boutros NN ((2007) ) Range of sensory gating values and test-retest reliability in normal subjects. Psychophysiology 44: , 620–626. |
[65] | Rentzsch J , Jockers-Scherubl MC , Boutros NN , Gallinat J ((2008) ) Test-retest reliability of P50, N100 and P200 auditory sensory gating in healthy subjects. Int J Psychophysiol 67: , 81–90. |
[66] | Benjamini Y , Hochberg Y ((1995) ) Controlling the false discovery rate: A practical and powerful approach to multiple testing. J R Statist Soc 57: , 289–300. |
[67] | Garrido MI , Kilner JM , Stephan KE , Friston KJ ((2009) ) The mismatch negativity: A review of underlying mechanisms. Clin Neurophysiol 120: , 453–463. |
[68] | Winkler I , Denham SL , Nelken I ((2009) ) Modeling the auditory scene: Predictive regularity representations and perceptual objects. Trends Cogn Sci 13: , 532–540. |
[69] | Kok A ((1997) ) Event-related-potential (ERP) reflections of mental resources: A review and synthesis. Biol Psychol 45: , 19–56. |
[70] | Montero-Odasso M , Zou G , Speechley M , Almeida QJ , Liu-Ambrose T , Middleton LE , Camicioli R , Bray NW , Li KZH , Fraser S , Pieruccini-Faria F , Berryman N , Lussier M , Shoemaker JK , Son S , Bherer L ((2023) ) Effects of exercise alone or combined with cognitive training and vitamin D supplementation to improve cognition in adults with mild cognitive impairment: A randomized clinical trial. JAMA Netw Open 6: , e2324465. |
[71] | Gomez-Soria I , Marin-Puyalto J , Peralta-Marrupe P , Latorre E , Calatayud E ((2022) ) Effects of multi-component non-pharmacological interventions on cognition in participants with mild cognitive impairment: A systematic review and meta-analysis. Arch Gerontol Geriatr 103: , 104751. |
[72] | Griffiths J , Thaikruea L , Wongpakaran N , Munkhetvit P , Kittisares A , Varnado P ((2020) ) Effects of combined physical movement activity and multifaceted cognitive training in older people with mild neurocognitive disorder in a rural community: A randomized control trial. Dement Geriatr Cogn Disord 49: , 194–201. |
[73] | Jellinger KA , Attems J ((2013) ) Neuropathological approaches to cerebral aging and neuroplasticity. Dialogues Clin Neurosci 15: , 29–43. |
[74] | Rebok GW , Ball K , Guey LT , Jones RN , Kim HY , King JW , Marsiske M , Morris JN , Tennstedt SL , Unverzagt FW , Willis SL ((2014) ) Ten-year effects of the advanced cognitive training for independent and vital elderly cognitive training trial on cognition and everyday functioning in older adults. J Am Geriatr Soc 62: , 16–24. |