Neurovascular Correlates of Cobalamin, Folate, and Homocysteine in Dementia
Abstract
Background:
Cobalamin (Cbl) and folate are common supplements clinicians prescribe as an adjuvant therapy for dementia patients, on the presumption of their neurotrophic and/or homocysteine (Hcy) lowering effect. However, the treatment efficacy has been found mixed and the effects of Cbl/folate/Hcy on the human brain remain to be elucidated.
Objective:
To explore the neurovascular correlates of Cbl/folate/Hcy in Alzheimer’s disease (AD) and subcortical ischemic vascular dementia (SIVD).
Methods:
Sixty-seven AD patients and 57 SIVD patients were prospectively and consecutively recruited from an outpatient clinic. Multimodal 3-Tesla magnetic resonance imaging was performed to quantitatively evaluate cerebral blood flow (CBF) and white matter integrity. The relationship between neuroimaging metrics and the serum levels of Cbl/folate/Hcy was examined by using the Kruskal-Wallis test, partial correlation analysis, and moderation analysis, at a significance level of 0.05.
Results:
As a whole, CBF mainly associated with Cbl/folate while white matter hyperintensities exclusively associated with Hcy. As compared with AD, SIVD exhibited more noticeable CBF correlates (spatially widespread with Cbl and focal with folate). In SIVD, a bilateral Cbl-moderated CBF coupling was found between medial prefrontal cortex and ipsilateral basal ganglia, while in the fronto-subcortical white matter tracts, elevated Hcy was associated with imaging metrics indicative of increased injury in both axon and myelin sheath.
Conclusions:
We identified the neurovascular correlates of previously reported neurotrophic effect of Cbl/folate and neurotoxic effect of Hcy in dementia. The correlates exhibited distinct patterns in AD and SIVD. The findings may help improving the formulation of supplemental Cbl/folate treatment for dementia.
INTRODUCTION
Cobalamin (Cbl, also known as vitamin B12) and folate (also known as vitamin B9) are two micronutrients with critical roles in the human central nervous system [1]. Previous studies suggest that these micronutrients have neurotrophic effects [2–4] and their deficiencies may cause detrimental alterations to cerebral function [2, 3, 5] and structures [5–7]. Specifically, both Cbl and folate facilitate the remethylation of homocysteine (Hcy), a non-proteinogenic amino acid byproduct formed in the metabolism process of methionine. An elevated level of Hcy has been noted as a modifiable risk factor of dementia [8] and other neurological diseases such as stroke and cerebral small vessel disease [9], presumably due to the build-up of free radicals [1] alongside endothelial dysfunction [9] and accumulation of extracellular matrix [3].
Cbl and folate are commonly prescribed as supplements to prevent the progression of dementia and cognitive decline, although mixed results have been reported in regard to their efficacy [10, 11]. The inconsistencies/controversies could stem from the heterogeneous pathophysiological substrates of dementia. Take vascular dementia (VaD) and Alzheimer’s disease (AD) for example, two most prevalent types of dementia that account for 20–30% and 60–70% of dementia cases, respectively [12, 13]. While VaD, as the name suggests, is caused by impaired cerebral blood flow (CBF), AD is generally associated with proteinopathy such as amyloid and tau in the brain. Hcy may thus affect VaD and AD through different pathways which in turn are differentially modulated by Cbl and folate.
Previous studies evaluated the effects of Cbl, folate, and Hcy on the brain separately [2, 3, 5, 14, 15]. The effects had been primarily assumed on CBF [2, 3, 5], blood-brain barrier [3], and white matter [5, 14, 15]. However, it remains unclear whether their effects are interrelated and/or concentration dependent, and the neural correlates are not fully understood. Therefore, this study had two aims. First, we explored the association between the serum levels of Cbl/folate/Hcy and regional CBF and white matter integrity. Second, we tested the hypothesis that the effect of Cbl/folate/Hcy is differential in subcortical ischemic vascular dementia (SIVD, the most common subtype of VaD [16]) and AD.
MATERIALS AND METHODS
The framework of this study is summarized in Fig. 1.
Clinical registry
This is a cross-sectional observational study approved by the institutional review board. From an outpatient clinic, a total of 124 patients with clinically-diagnosed dementia according to published guidelines were prospectively recruited: 57 with SIVD [17], 67 with AD [18]. All patients received a serology test and a brain magnetic resonance (MR) imaging exam. Demographic data and cognitive status evaluated by the Mini-Mental State Examination (MMSE) and the clinical dementia rating were recorded. All patients provided written informed consent. None of the patients had significant artery stenosis or occlusion according to MR images (see below).
Fig. 1
Study framework. A) The first part of study was to explore neurovascular correlates of cobalamin (Cbl), folate, and homocysteine (Hcy) in dementia. B) The second part of study was to investigate the differential neurovascular effects of Cbl/folate/Hcy in Alzheimer’s disease (AD) and subcortical ischemic vascular disease (SIVD). ASL, arterial spin labeling; DTI, diffusion tensor imaging; FLAIR, fluid-attenuated inversion recovery; ROI, region of interest.
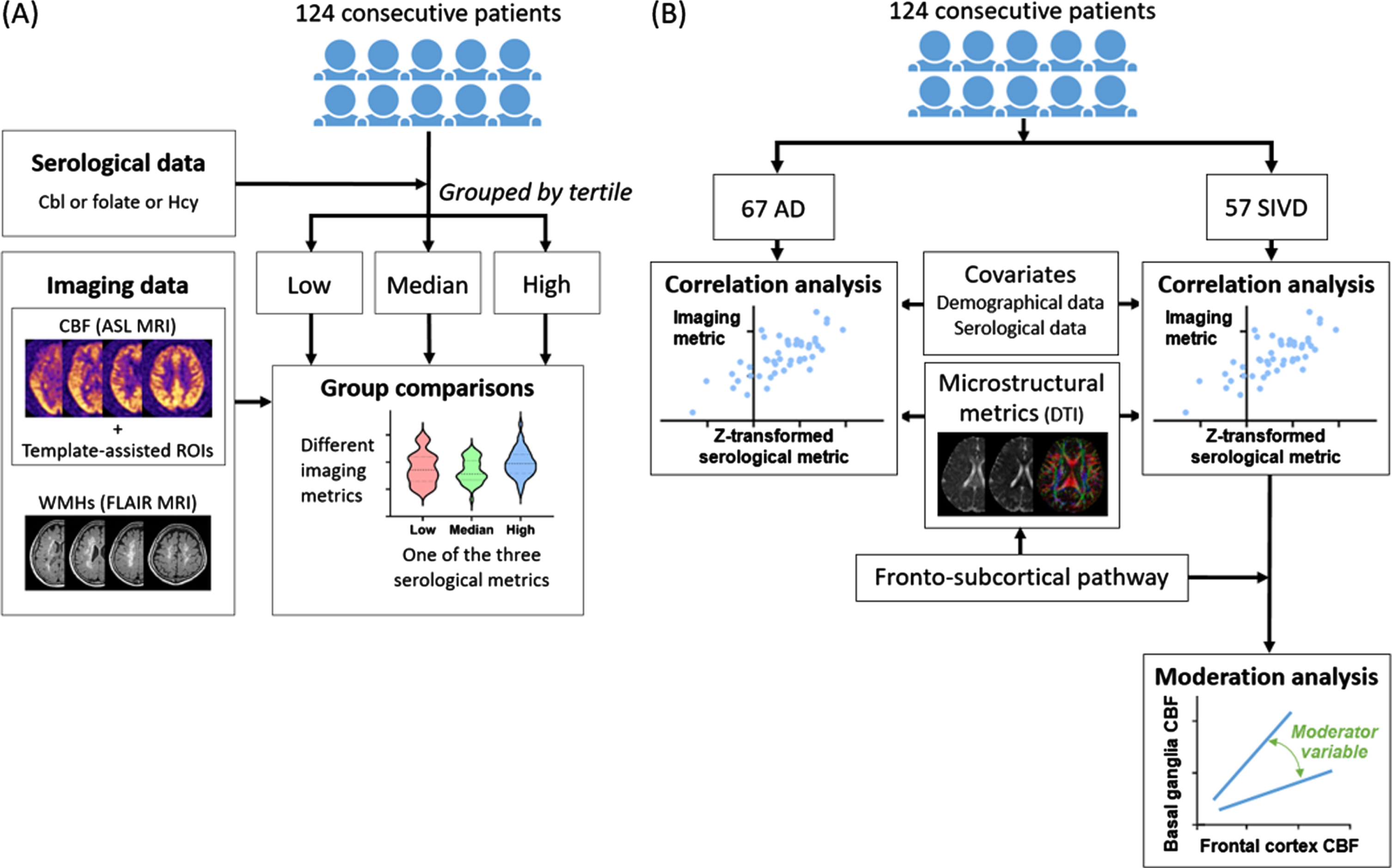
Serology test
The participants were asked to fast for 8 h before having the serology test. Blood samples were drawn from the antecubital vein, centrifuged within 10 min, and then stored below –20°C until analysis. Serum levels of Cbl and folate were measured by using competitive binding receptor assays (Beckman Coulter DxI 800). Serum levels of creatinine and Hcy were measured by using spectrophotometry (Beckman Coulter AU5800&DxC 700 AU).
MR imaging
MR imaging was performed on a 3-Tesla scanner (Discovery MR750, GE Medical Systems, Milwaukee, WI, USA) using an 8-channel phased-array head coil receiver. The imaging protocol included three-dimensional T1-weighted imaging, fluid-attenuated inversion recovery imaging, time-of-flight angiography, diffusion tensor imaging (DTI; diffusion encoding along 6 non-collinear directions, b-value = 0 and 1000 s/mm2, matrix size = 96×96, and slice thickness = 2.5 mm), and pseudo-continuous arterial spin labeling (PCASL; post-labeling delay = 1525 ms, matrix size = 128×128, and slice thickness = 5 mm). All participants were requested to refrain from caffeine ingestion [19] on the day of imaging exam.
Measurement of neurovascular image metrics
CBF maps were generated from PCASL images on the GE workstation (GE Healthcare). Regional CBF was then calculated within 14 regions of interest (ROIs) aggregated from the anatomical areas provided by the Automated Anatomical Labeling template [20]. We employed the hypothesis-driven selection of ROIs because including all the template ROIs (90 ROIs in the original version and 116 in the latest version) would lower the power to detect group difference after accounting for multiple comparisons. The hypothesis was that the areas where altered CBF was previously reported in AD and SIVD (e.g., frontotemporal area [21, 22] and basal ganglia [2, 5]) are more likely to be associated with neurovascular modulation. Specifically, seven ROIs were defined for both hemispheres. The ROIs and the contained areas (listed in parentheses) were: lateral prefrontal cortex (superior/middle/inferior gyrus), medial prefrontal cortex (medial prefrontal gyrus and anterior cingulate cortex), ventral prefrontal cortex (orbitofrontal gyrus and rectal gyrus), lateral temporal cortex (superior/middle/inferior temporal gyrus), medial temporal cortex (hippocampus, parahippocampus, and fusiform gyrus), parietal cortex (superior parietal lobule and precuneus), and basal ganglia (caudate nucleus, lentiform nucleus, and thalamus). By using the MRIcron software (https://www.nitrc.org/projects/mricron), a licensed neurologist with 11 years of clinical experience (MCT) visually inspected the registration between the CBF map and the template ROIs and, when necessary, manually modified the ROIs to reduce misregistration. The average CBF within each ROI was recorded.
The volume of white matter hyperintensities (WMHs) was calculated from fluid-attenuated inversion recovery images by using the lesion prediction algorithm [23] as implemented in the lesion segmentation toolbox version 3.0.0 (http://www.statistical-modelling.de/lst.html) for Statistical Parametric Mapping (https://www.fil.ion.ucl.ac.uk/spm/). To assess the structural connectivity between the thalamus and medial prefrontal cortex, white matter tract-based analysis was performed by using the DSI Studio (http://dsi-studio.labsolver.org). Briefly, the diffusion tensor was voxel-wise estimated and then used to calculate diffusion metrics including fractional anisotropy (FA), mean diffusivity (MD), radial diffusivity (RD), and axial diffusivity (AxD). The International Consortium for Brain Mapping 152 template was then warped to the subject’s native coordinates. Two ROIs were assigned to track fibers that pass the thalamus (i.e., the seed ROI) and terminate at the ipsilateral medial prefrontal cortex (i.e., the end ROI). The location and boundary of ROIs were visually inspected and, when necessary, manually adjusted by the rater mentioned above. The deterministic tracking approach was used with the following parameters: FA threshold = 0.05, angular threshold = 45°, and minimum/maximum length = 10/200 mm. At last, the diffusion metrics were averaged within the obtained tract for each hemisphere separately.
Statistical analysis
The participants with outlier values for serology markers (absolute z-score>3) were excluded from subsequent statistical analysis (N = 3). Between-group effects were inspected by the Chi-squared test, the Mann-Whitney U test, or the Kruskal-Wallis test wherever appropriate. The relationship between serology markers and neurovascular image metrics was examined by partial correlation analysis. Moderation analysis was conducted after all variables underwent z transformation. All statistical tests were performed using the SPSS software version 28 (IBM, Armonk, New York). A p-value <0.05 was considered to be statistically significant.
RESULTS
Table 1 summarizes the demographic data of the participants included in the following analyses. None of the items was statistically different between SIVD and AD patients (p = 0.053 –0.854).
Dependence of CBF and WMHs on the status of Cbl/folate/Hcy
Based on Cbl tertiles, significant group difference was found in CBF within the left lateral prefrontal cortex (p = 0.039), but not in WMHs. The results of post-hoc comparisons are shown in Fig. 2A. Based on folate tertiles, significant group difference was found in CBF within the right parietal cortex (p = 0.034) and left medial temporal cortex (p = 0.018), but not in WMHs. The results of post-hoc comparisons are shown in Fig. 2B and 2C. Based on Hcy tertiles, significant group difference was found in WMHs (p = 0.003), but not in CBF. The results of post-hoc comparisons are shown in Fig. 2D.
Table 1
Demographics of the participants entered in statistical analysis (N = 124)
Mean | Range | ||
Age | (y) | 75.7 | 45–85 |
Gender | (N; male/female) | 42/82 | |
Dementia subtype | (N; SIVD/AD) | 57/67 | |
Educational level | (y) | 5.3 | 0–16 |
Creatinine | (mg/dL) | 1.3 | 0.5–11 |
Cbl | (pg/mL) | 755.2 | 85–7500 |
Folate | (ng/mL) | 14.1 | 4–85 |
Hcy | (μmol/L) | 13.1 | 6–36 |
Clinical Dementia Rating Scale Sum of Boxes | 4.9 | 0.5–14 | |
Mini-Mental State Examination | 18.9 | 6–26 |
SIVD, subcortical ischemic vascular disease; AD, Alzheimer’s disease; Cbl, cobalamin; Hcy, homocysteine.
Fig. 2
Association between serology metrics and neurovascular image metrics. A) Cerebral blood flow (CBF) comparisons among the groups defined by the tertiles of cobalamin (Cbl) level. Range of Cbl (pg/mL) [Case number] of Low = 85–342 [41]; Median = 348–685 [41]; High = 717–3094 [40]. B, C) CBF comparisons among the groups defined by the tertiles of folate level. Range of folate (ng/mL) [Case number] of Low = 4–9 [41]; Median = 9–15 [41]; High = 15–41 [41]. D) White matter hyperintensity (WMH) volume comparisons among groups defined by the tertiles of homocysteine (Hcy) level. Range of Hcy (μmol/L) [Case number] of Low = 5.58–10.20 [41]; Median = 10.21–14.08 [41]; High = 14.31–36.05 [39]. Two-way analysis of variance showed no significant interaction between dementia subtype and Cbl level (p = 0.319–0.407) or folate level (p = 0.514–0.669) on CBF, and no significant interaction between dementia subtype and homocysteine level on WMH volume (p = 0.545).
![Association between serology metrics and neurovascular image metrics. A) Cerebral blood flow (CBF) comparisons among the groups defined by the tertiles of cobalamin (Cbl) level. Range of Cbl (pg/mL) [Case number] of Low = 85–342 [41]; Median = 348–685 [41]; High = 717–3094 [40]. B, C) CBF comparisons among the groups defined by the tertiles of folate level. Range of folate (ng/mL) [Case number] of Low = 4–9 [41]; Median = 9–15 [41]; High = 15–41 [41]. D) White matter hyperintensity (WMH) volume comparisons among groups defined by the tertiles of homocysteine (Hcy) level. Range of Hcy (μmol/L) [Case number] of Low = 5.58–10.20 [41]; Median = 10.21–14.08 [41]; High = 14.31–36.05 [39]. Two-way analysis of variance showed no significant interaction between dementia subtype and Cbl level (p = 0.319–0.407) or folate level (p = 0.514–0.669) on CBF, and no significant interaction between dementia subtype and homocysteine level on WMH volume (p = 0.545).](https://content.iospress.com:443/media/jad/2023/96-3/jad-96-3-jad230763/jad-96-jad230763-g002.jpg)
3.2Correlates of Cbl/folate/Hcy with CBF and WMHs broken down by dementia type
After noting the different associations between Cbl/folate/Hcy and CBF, we examined their associations in SIVD and AD separately by using the partial correlation analysis controlling for demographic data and serology markers (Table 2). In SIVD patients, serum levels of Cbl were found to positively correlate with CBF in most of the areas investigated (r = 0.29–0.55, p = <0.001–0.046). By contrast, serum levels of folate appeared to negatively associate with CBF but the correlation was only significant in the right lateral prefrontal cortex (r = –0.31, p = 0.032). In AD patients, serum levels of folate appeared to positively associate with CBF but the correlation was only significant in the left medial prefrontal cortex (r = 0.28, p = 0.029); no consistent or statistical correlation was observed between Cbl and CBF. No correlation was found between Hcy and CBF regardless of dementia types.
Table 2
Correlation between cobalamin/folate and regional cerebral blood flow
Cobalamin¶ | Folate§ | |||||||
SIVD | AD | SIVD | AD | |||||
L | R | L | R | L | R | L | R | |
Parietal cortex | 0.24 | 0.32* | 0.03 | 0.05 | –0.07 | 0.03 | 0.25 | 0.15 |
Lateral prefrontal cortex | 0.27 | 0.29* | 0.07 | –0.05 | –0.10 | –0.31* | 0.25 | 0.23 |
Medial prefrontal cortex | 0.55*** | 0.55*** | 0.04 | –0.11 | –0.15 | –0.06 | 0.28* | 0.13 |
Ventral prefrontal cortex | 0.37** | 0.35* | 0.03 | –0.02 | –0.17 | –0.14 | 0.23 | 0.08 |
Basal ganglia | 0.29* | 0.32* | 0.11 | –0.02 | –0.03 | –0.05 | 0.18 | 0.08 |
Lateral temporal lobe | 0.44** | 0.29* | –0.02 | –0.12 | –0.15 | –0.19 | 0.09 | 0.16 |
Medial temporal lobe. | 0.33* | 0.34* | –0.06 | –0.02 | 0.09 | –0.07 | 0.17 | 0.13 |
Partial correlation coefficients are reported. SIVD, subcortical ischemic vascular disease; AD, Alzheimer’s disease; L, left; R, right. ¶With age, education, creatinine, folate, and homocysteine as covariates. §With age, education, creatinine, cobalamin, and homocysteine as covariates. */**/***p < 0.05/0.01/0.001.
Simple correlation analysis showed a significant correlation between WMH volume and serum levels of Hcy in SIVD (r = 0.420; p = 0.001), but the correlation was marginal in AD (r = 0.241; p = 0.051). After controlling for demographic data and creatinine, only the correlation in SIVD remained significant (r = 0.294; p = 0.036)
CBF moderation by Cbl in SIVD
After noting the widespread correlation between serum levels of Cbl and CBF in SIVD patients, we explored the potential moderating role of Cbl in the fronto-subcortical circuits that were previously shown to interconnect cognitive hubs in SIVD [24, 25]. Among the frontal ROIs listed in Table 2, only the medial prefrontal cortex exhibited CBF correlation with the ipsilateral basal ganglia and was entered in moderation analysis. As summarized in Fig. 3, the analysis revealed a bilateral moderation effect of Cbl on the CBF coupling between the basal ganglia and ipsilateral medial prefrontal cortex (left/right: p = 0.039/0.014, 0.049/0.009 after controlling for Hcy level, and 0.015/0.051 after controlling for folate level).
Fig. 3
Breakdown of the moderation effect of cobalamin on the cerebral blood flow coupling between basal ganglia and medial prefrontal cortex in subcortical ischemic vascular disease. Moderation effects were found both before (subplots A and B for the left and right hemispheres, respectively) and after (subplots C and D for the left and right hemispheres, respectively) controlling for the effect of homocysteine. Cbl, cobalamin; BG, basal ganglia; MPFC, medial prefrontal cortex; L, left; R, right; CI, confidence interval.
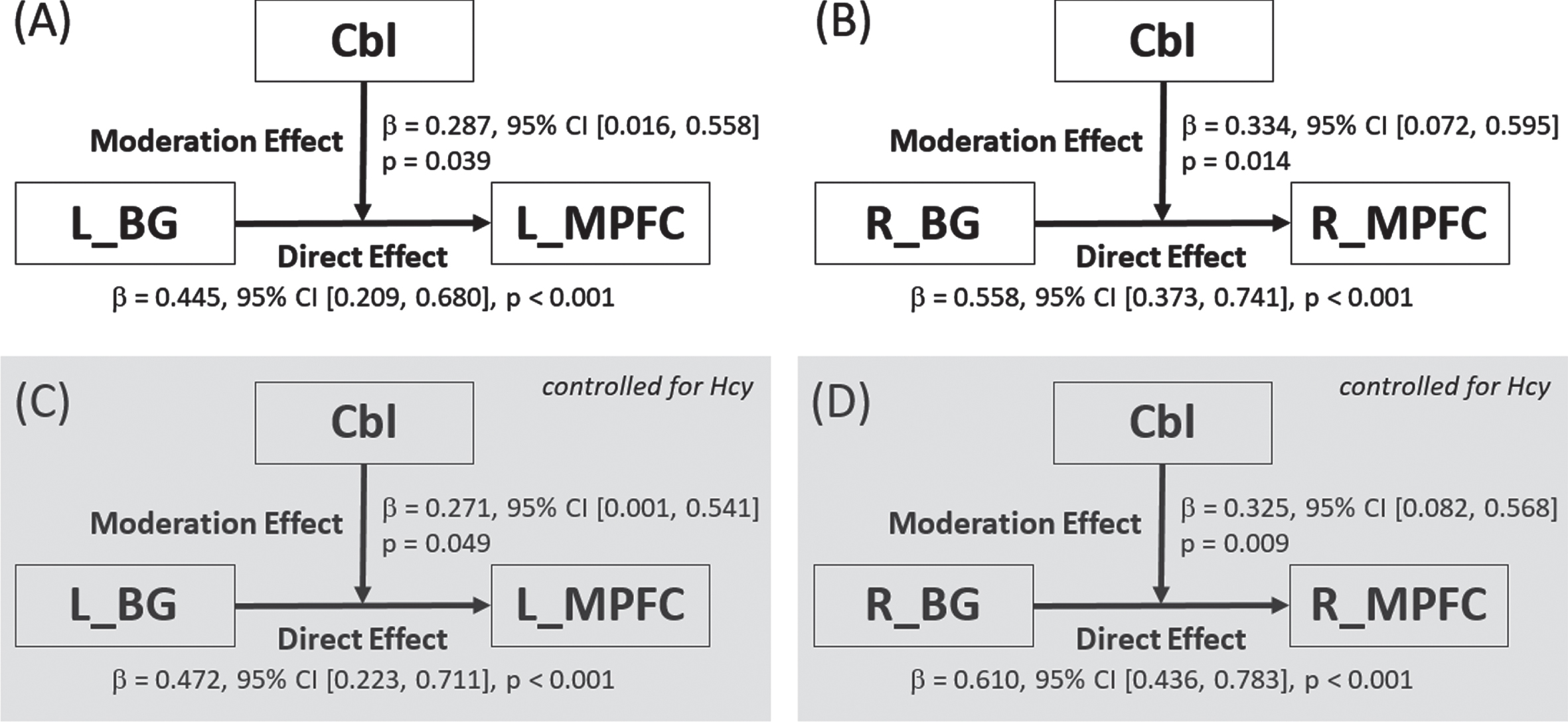
White matter microstructural correlates with the serum level of Hcy broken down by dementia type
Following the observed correlation between the serum level of Hcy and WMHs, we examined the microstructural connectivity of the fronto-subcortical circuits (between the medial prefrontal cortex and ipsilateral thalamus) by using the diffusion metrics derived from DTI (Table 3). In SIVD patients, Hcy level was found to negatively correlate with FA (r = –0.44, p = 0.001, for both left and right tracts) and positively correlate with all diffusivity indices (r = 0.28 –0.46, p = 0.001 –0.046, for both left and right tracts). In AD patients, Hcy level only correlated with the RD of the right tract (r = 0.26, p = 0.040). After controlling for demographic data and other serum markers, Hcy level still correlated with FA on the left side, and correlated with MD and RD on the right side in SIVD patients, whereas no correlation between Hcy level and diffusion indices remained in AD patients.
Table 3
Correlation between fronto-subcortical white matter connectivity and serum levels of cobalamin, folate, and homocysteine
Left | Right | ||||||||
FA | MD | AxD | RD | FA | MD | AxD | RD | ||
Cobalamin | SIVD | 0.06 | 0.03 | 0.02 | –0.08 | –0.04 | 0.13 | 0.18 | 0.11 |
AD | –0.14 | –0.05 | 0.04 | 0.10 | –0.07 | 0.07 | –0.01 | 0.10 | |
Folate | SIVD | 0.14 | –0.14 | –0.22§ | –0.27*§ | –0.13 | 0.07 | 0.09 | 0.09 |
AD | –0.13 | –0.29* | –0.33**§ | –0.23 | 0.04 | –0.14 | –0.09 | –0.22 | |
Homocysteine | SIVD | –0.44**¶ | 0.28* | 0.33* | 0.35* | –0.44** | 0.44**¶ | 0.29* | 0.46**¶ |
AD | 0.14 | –0.02 | 0.09 | 0.02 | –0.19 | 0.24 | 0.23 | 0.26* |
SIVD, subcortical ischemic vascular disease; AD, Alzheimer’s disease; FA, fractional anisotropy; MD, mean diffusivity; AxD, axial diffusivity; RD, radial diffusivity. Case number in cobalamin analysis: SIVD (left/right) = 54/52; AD (left/right) = 64/65. Case number in folate analysis: SIVD (left/right) = 55/53; AD (left/right) = 64/65. Case number in homocysteine analysis: SIVD (left/right) = 53/51; AD (left/right) = 64/65. The case number varied due to incomplete/failed fiber tracking in some subjects. Pearson correlation coefficients by simple correlation analysis are reported, where */** indicate p < 0.05/0.01. Significant results by partial correlation analysis are marked by § for p < 0.05 after controlling for age, education, creatinine, cobalamin, and homocysteine, and ¶ for p < 0.05 after controlling for age, education, creatinine, cobalamin, and folate.
Interestingly, an inverse correlation between diffusivity indices and the serum level of folate was observed for the left tract. After controlling for demographic data and other serum markers, correlation remained for AxD (p = 0.03) and RD (p = 0.03) in SIVD and AxD (p = 0.02) in AD. Meanwhile, no correlation was found between the serum level of Cbl and diffusion indices.
DISCUSSION
In this study, we have explored and identified the neurovascular correlates of Cbl, folate, and Hcy from a cohort of dementia patients. Specifically, Cbl and folate were found to primarily associate with CBF, while Hcy exclusively associated with WMHs. When dementia types were considered, SIVD exhibited more noticeable associations between CBF/WMHs and Cbl/folate/Hcy than AD did. As far as the CBF association was concerned, Cbl had a widespread effect in SIVD but no significant effect in AD, while folate had a local effect in both SIVD and AD. Within the fronto-subcortical circuits, we identified Hcy-dependent white matter impairment and a Cbl-moderated CBF coupling in SIVD. These findings may advance current understanding of the neurotrophic roles of Cbl/folate and whereby improve the formulation of Cbl/folate treatment for dementia.
Few studies have addressed the neural or vascular correlates of Cbl, folate, and Hcy in humans[2, 5]. Some animal studies described Cbl/folate-related CBF alteration, albeit often along with an elevated Hcy level and comorbid conditions such as malnutrition, low body weight, and anemia [26–28]. Based on structural MR imaging, most previous studies reported Hcy-related brain volume reduction [15] at a macroscopic scale, while our study focused specifically on white matter lesion and microstructural changes of neuronal fibers. Accumulating evidence suggests that hyperhomocysteinemia could trigger and promote atherogenesis and thrombosis [29]. When small vessels are damaged, Hcy-related microangiopathy could cause WMH formation [30] and brain volume loss [15]. In line with published studies, our results confirmed the adverse effect of serum Hcy on white matter integrity, both macroscopically (i.e., in terms of WMH volume) and microscopically (i.e., in terms of DTI metrics). Furthermore, when compared with AD patients, the neurotoxic effect of Hcy on white matter was more noticeable in SIVD patients, impacting both axon and myelin sheath. Specifically, an elevated serum level of Hcy was found to associate with increased RD (positive correlation) and decreased FA (negative correlation), which together suggested myelin injury/degeneration (i.e., more pronounced diffusion perpendicular to fiber tracts along with diminished directional dependence of diffusion). On the other hand, an elevated level of plasma Hcy was found to associate with increased AxD (positive correlation), which had been shown to reflect axonal injury (e.g., greater extracellular water content as a consequence of white matter atrophy) [31].
Our results showed that both Cbl and folate correlated with CBF, in patterns distinguishable between SIVD and AD. The Cbl-CBF correlation was widespread (involving both cortical and subcortical areas and multiple lobes) in SIVD but absent in AD. By contrast, the folate-CBF correlation was focal in both SIVD and AD but involved different areas (left lateral prefrontal cortex and left medial prefrontal cortex, respectively). These findings raise the possibility that Cbl and folate may have differential effects on endothelial function and in turn vascular reactivity and/or neurovascular coupling. Specifically, the global Cbl-CBF correlation mirrors the clinical observation of CBF restoration after Cbl supplement [2, 5]. On the other hand, the focal folate-CBF correlation could be partly explained by a specialized folate transport system within the endothelial wall of cerebral capillaries. The dedicated endothelium-dependent response yields relative resilience to the stress/damage imposed by folate deficiency. Furthermore, SIVD and AD presenting different patterns of Cbl/folate-CBF correlation could be because vascular dysfunction/damage is the primary cause in the former but widely considered secondary in the latter [21]. The brain areas where CBF appears to be modulated by Cbl/folate may derive biomarkers useful for the planning and assessment of supplemental Cbl/folate treatment for dementia.
Our results showed that in SIVD patients, CBF was correlated between medial prefrontal cortex and basal ganglia in both hemispheres, and the coupling was positively moderated by Cbl beyond the effect of Hcy. The finding coincides with previous reports that fronto-subcortical circuits are accountable for the cognitive deficit in SIVD [24, 32] and vulnerable to Cbl deficiency [2]. Basal ganglia and medial prefrontal cortex are known for their critical roles in cognitive processes such as motivation and goal-directed behavior [25]. Since both Cbl deficiency [33] and SIVD [24] can lead to executive dysfunction, Cbl supplement may be of clinical interest to improve dysexecutive syndrome among those affected.
On the other hand, we found that the elevated serum level of Hcy was associated with increased microstructural injury along the white matter tracts connecting basal ganglia and medial prefrontal cortex, and the tract impairment was uncorrelated with Cbl. Taken together, these findings suggest that Cbl has a protective and moderating effect on the fronto-subcortical circuits via a neurovascular substrate dissociable from the nocuous effect of Hcy on white matter microstructure. This echoes the reported dissociation between functional/hemodynamic connectivity and structural connectivity [5].
Our data showed that the serum level of folate inversely correlated with white matter damage in both AD and SIVD. The folate-CBF correlation, however, was positive within the left medial prefrontal cortex in AD but negative within the left lateral prefrontal cortex in SIVD. This finding implies that the rescue effect of folate could reverse when microvasculature has been critically compromised. The paradoxical folate-CBF association could also stem from the obscure Cbl-folate interaction regarding which contradictory findings had been published. For example, high serum folate with a low Cbl status was reported to be cognitively nocuous among the elderly [34], neutral in young adult [35], or of positive effect on cognition [36]. An elevated level of folate was associated with both smaller brain volume [37] and a marginal effect on cerebral microstructural integrity [4]. Our results suggested that the folate status had a limited part in the moderation of Cbl on the fronto-subcortical CBF coupling. It is worth noting that anemia could give rise to CBF alteration according to animal studies that reported perturbed CBF in rats subjected to folate deprivation and with concomitant low red blood cell counts [26, 27]. However, a longitudinal observation among rats fed a folate-deficient diet later showed that anemia could not fully explain the observed CBF perturbation [27]. We acknowledge that the effect of anemia cannot be ruled out in this study, although the effect should be small considering that only approximately one-third of our subjects were classified to be anemic. Alternatively, this paradoxical folate-CBF association could be contributed by the U-shape relationship between folate and Hcy [38], or other biomarkers of the methionine cycle and transsulfuration pathway which are beyond the scope of the current research.
A few limitations of this study should be noted. First, our data did not include genetic variants regarding Cbl or folate metabolism (e.g., methylenetetrahydrofolate reductase) [39]. Second, we were unable to entirely exclude the effect of lifestyle behaviors. Dietary supplement use and malabsorption issues might vary among our elderly subjects. Given that recent diet and fortified supplement could change serum levels of Cbl and folate, the measured serology values might not be equivalent to biological availability (e.g., folate level in serum and red blood cells [40]; Cbl and holotranscobalamin [41]) or indicative of a long-term effect [40]. Third, this study lacked a healthy control group and thus the contribution of normal aging to the neurovascular correlates found in this study cannot be ruled out. Nonetheless, disease-related neurodegeneration appeared to play a major role given that statistical significance remained after age was controlled for in a large part of the partial correlation analyses and that the correlates exhibited different patterns in AD and SIVD (i.e., the patterns would be similar if normal aging was the dominant effect). Forth, causal inferences cannot be made based on the cross-sectional design of the present study, which warrants further research with a longitudinal and randomized design.
In summary, by using multimodal MR imaging, we identified the neurovascular correlates of previously reported neurotrophic effect of Cbl/folate and neurotoxic effect of Hcy in dementia. Mainly associated with CBF, Cbl had a widespread effect, involving both cortical and subcortical areas and multiple lobes, whereas the effect of folate was relatively focal. An elevated serum level of Hcy was associated with both macroscopic and microscopic white matter abnormalities. The correlates exhibited distinct patterns between AD and SIVD. Our results provided radiological/neurological insights to the roles of Cbl/folate/Hcy in dementia, which may help resolving the mixed findings with regard to Cbl/folate treatment efficacy and further improving the planning/assessment of supplemental Cbl/folate treatment for dementia.
ACKNOWLEDGMENTS
The authors thank all patients for study participation.
FUNDING
This work was supported by the Taichung Tzu Chi Hospital (grant number TTCRD 110-10 and TTCRD 111-03) (to MCT).
CONFLICT OF INTEREST
The authors have no conflict of interest to report.
DATA AVAILABILITY
The data supporting the findings of this study are available from the corresponding author upon reasonable request.
REFERENCES
[1] | Burgess K , Bennett C , Mosnier H , Kwatra N , Bethel F , Jadavji NM ((2020) ) The antioxidant role of one-carbon metabolism on stroke. Antioxidants 9: , 1141. |
[2] | Tu M-C , Lo C-P , Chen C-Y , Huang C-F ((2015) ) Correlation of Tc-99m ethyl cysteinate dimer single-photon emission computed tomography and clinical presentations in patients with low cobalamin status. BMC Neurol 15: , 251. |
[3] | Braun DJ , Abner E , Bakshi V , Goulding DS , Grau EM , Lin A-L , Norris CM , Sudduth TL , Webster SJ , Wilcock DM ((2019) ) Blood flow deficits and cerebrovascular changes in a dietary model of hyperhomocysteinemia. ASN Neuro 11: , 1759091419865788. |
[4] | Beydoun MA , Shaked D , Hossain S , Beydoun HA , Katzel LI , Davatzikos C , Gullapalli RP , Seliger SL , Erus G , Evans MK ((2020) ) Vitamin D, folate, and cobalamin serum concentrations are related to brain volume and white matter integrity in urban adults. Front Aging Neurosci 12: , 140. |
[5] | Roy B , Trivedi R , Garg RK , Gupta PK , Tyagi R , Gupta RK ((2015) ) Assessment of functional and structural damage in brain parenchyma in patients with vitamin B12 deficiency: A longitudinal perfusion and diffusion tensor imaging study. Magn Reson Imaging 33: , 537–543. |
[6] | Hsu Y-H , Huang C-F , Lo C-P , Wang T-L , Tu M-C ((2016) ) Vitamin B12 deficiency: Characterization of psychometrics and MRI morphometrics. Nutr Neurosci 19: , 47–54. |
[7] | Köbe T , Witte AV , Schnelle A , Grittner U , Tesky VA , Pantel J , Schuchardt JP , Hahn A , Bohlken J , Rujescu D ((2016) ) Vitamin B-12 concentration, memory performance, and hippocampal structure in patients with mild cognitive impairment. Am J Clin Nutr 103: , 1045–1054. |
[8] | Smith AD , Refsum H , Bottiglieri T , Fenech M , Hooshmand B , McCaddon A , Miller JW , Rosenberg IH , Obeid R ((2018) ) Homocysteine and dementia: An international consensus statement. J Alzheimers Dis 62: , 561–570. |
[9] | Hassan A , Hunt BJ , O’Sullivan M , Bell R , D’Souza R , Jeffery S , Bamford JM , Markus HS ((2004) ) Homocysteine is a risk factor for cerebral small vessel disease, acting via endothelial dysfunction. Brain 127: , 212–219. |
[10] | Markun S , Gravestock I , Jäger L , Rosemann T , Pichierri G , Burgstaller JM ((2021) ) Effects of vitamin B12 supplementation on cognitive function, depressive symptoms, and fatigue: A systematic review, meta-analysis, and meta-regression. Nutrients 13: , 923. |
[11] | Li S , Guo Y , Men J , Fu H , Xu T ((2021) ) The preventive efficacy of vitamin B supplements on the cognitive decline of elderly adults: A systematic review and meta-analysis. BMC Geriatrics 21: , 1–14. |
[12] | Wolters FJ , Ikram MA ((2019) ) Epidemiology of vascular dementia. Arterioscler Thromb Vasc Biol 39: , 1542–1549. |
[13] | World Health Organization (2020) Dementia. https://www.who.int/news-room/factsheets/detail/dementia, Last updated September 21, 2020, Accessed on October 6, 2020. |
[14] | Scott TM , Tucker KL , Bhadelia A , Benjamin B , Patz S , Bhadelia R , Liebson E , Price LL , Griffith J , Rosenberg I ((2004) ) Homocysteine and B vitamins relate to brain volume and white-matter changes in geriatric patients with psychiatric disorders. Am J Geriatr Psychiatry 12: , 631–638. |
[15] | Tangney C , Aggarwal N , Li H , Wilson R , Decarli C , Evans D , Morris M ((2011) ) Vitamin B12, cognition, and brain MRI measures: A cross-sectional examination. Neurology 77: , 1276–1282. |
[16] | Bowler J ((2007) ) Modern concept of vascular cognitive impairment. Br Med Bull 83: , 291–305. |
[17] | Erkinjuntti T , Inzitari D , Pantoni L , Wallin A , Scheltens P , Rockwood K , Roman GC , Chui H , Desmond DW ((2000) ) Research criteria for subcortical vascular dementia in clinical trials. J Neural Transm Suppl 59: , 23–30. |
[18] | McKhann GM , Knopman DS , Chertkow H , Hyman BT , Jack CR Jr. , Kawas CH , Klunk WE , Koroshetz WJ , Manly JJ , Mayeux R , Mohs RC , Morris JC , Rossor MN , Scheltens P , Carrillo MC , Thies B , Weintraub S , Phelps CH ((2011) ) The diagnosis of dementia due to Alzheimer’s disease: Recommendations from the National Institute on Aging-Alzheimer’s Association workgroups on diagnostic guidelines for Alzheimer’s disease. Alzheimers Dement 7: , 263–269. |
[19] | Wu WC , Lien SH , Chang JH , Yang SC ((2014) ) Caffeine alters resting-state functional connectivity measured by blood oxygenation level-dependent MRI. NMR Biomed 27: , 444–452. |
[20] | Tzourio-Mazoyer N , Landeau B , Papathanassiou D , Crivello F , Etard O , Delcroix N , Mazoyer B , Joliot M ((2002) ) Automated anatomical labeling of activations in SPM using a macroscopic anatomical parcellation of the MNI MRI single-subject brain. Neuroimage 15: , 273–289. |
[21] | Tu MC , Chung HW , Hsu YH , Yang JJ , Wu WC ((2022) ) Stage-dependent cerebral blood flow and leukoaraiosis couplings in subcortical ischemic vascular disease and Alzheimer’s disease. J Alzheimers Dis 86: , 729–739. |
[22] | Swinford CG , Risacher SL , Wu YC , Apostolova LG , Gao S , Bice PJ , Saykin AJ ((2023) ) Altered cerebral blood flow in older adults with Alzheimer’s disease: A systematic review. Brain Imaging Behav 17: , 223–256. |
[23] | Schmidt P , Gaser C , Arsic M , Buck D , Förschler A , Berthele A , Hoshi M , Ilg R , Schmid VJ , Zimmer C ((2012) ) An automated tool for detection of FLAIR-hyperintense white-matter lesions in multiple sclerosis. Neuroimage 59: , 3774–3783. |
[24] | Hsu Y-H , Huang C-F , Lo C-P , Wang T-L , Yang C-C , Tu M-C ((2016) ) Frontal assessment battery as a useful tool to differentiate mild cognitive impairment due to subcortical ischemic vascular disease from Alzheimer disease. Dement Geriatr Cogn Disord 42: , 331–341. |
[25] | Orth L , Meeh J , Gur RC , Neuner I , Sarkheil P ((2022) ) Frontostriatal circuitry as a target for fMRI-based neurofeedback interventions: A systematic review. Front Hum Neurosci 16: , 933718. |
[26] | Hallacoglu B , Sassaroli A , Fantini S , Troen AM ((2011) ) Cerebral perfusion and oxygenation are impaired by folate deficiency in rat: Absolute measurements with noninvasive near-infrared spectroscopy. J Cereb Blood Flow Metab 31: , 1482–1492. |
[27] | Kim J-m , Lee H , Chang N ((2002) ) Hyperhomocysteinemia due to short-term folate deprivation is related to electron microscopic changes in the rat brain. J Nutr 132: , 3418–3421. |
[28] | Peng L , Dreumont N , Coelho D , Guéant J-L , Arnold C ((2016) ) Genetic animal models to decipher the pathogenic effects of vitamin B12 and folate deficiency. Biochimie 126: , 43–51. |
[29] | Sasaki T , Watanabe M , Nagai Y , Hoshi T , Takasawa M , Nukata M , Taguchi A , Kitagawa K , Kinoshita N , Matsumoto M ((2002) ) Association of plasma homocysteine concentration with atherosclerotic carotid plaques and lacunar infarction. Stroke 33: , 1493–1496. |
[30] | Bang OY , Chung J-W , Ryoo S , Moon GJ , Kim G-M , Chung C-S , Lee KH ((2016) ) Brain microangiopathy and macroangiopathy share common risk factors and biomarkers. Atherosclerosis 246: , 71–77. |
[31] | Thomason ME , Thompson PM ((2011) ) Diffusion imaging, white matter, and psychopathology. Ann Rev Clin Psychol 7: , 63–85. |
[32] | Roh JH , Lee J-H ((2014) ) Recent updates on subcortical ischemic vascular dementia. J Stroke 16: , 18. |
[33] | Soh Y , Lee DH , Won CW ((2020) ) Association between vitamin B12 levels and cognitive function in the elderly Korean population. Medicine 99: , e21371. |
[34] | Selhub J , Morris MS , Jacques PF ((2007) ) In vitamin B12 deficiency, higher serum folate is associated with increased total homocysteine and methylmalonic acid concentrations. Proc Natl Acad Sci U S A 104: , 19995–20000. |
[35] | Mills JL , Carter TC , Scott JM , Troendle JF , Gibney ER , Shane B , Kirke PN , Ueland PM , Brody LC , Molloy AM ((2011) ) Do high blood folate concentrations exacerbate metabolic abnormalities in people with low vitamin B-12 status? . Am J Clin Nutr 94: , 495–500. |
[36] | Doets EL , Ueland PM , Tell GS , Vollset SE , Nygård OK , Van’t Veer P , de Groot LC , Nurk E , Refsum H , Smith AD ((2014) ) Interactions between plasma concentrations of folate and markers of vitamin B12 status with cognitive performance in elderly people not exposed to folic acid fortification: The Hordaland Health Study. Br J Nutr 111: , 1085–1095. |
[37] | Van der Zwaluw NL , Brouwer-Brolsma EM , Van de Rest O , Van Wijngaarden JP , In’t Veld PH , Kourie DI , Swart KM , Enneman AW , Van Dijk SC , Van der Velde N ((2016) ) Folate and vitamin B12-related biomarkers in relation to brain volumes. Nutrients 9: , 8. |
[38] | Faux NG , Ellis KA , Porter L , Fowler CJ , Laws SM , Martins RN , Pertile KK , Rembach A , Rowe CC , Rumble RL ((2011) ) Homocysteine, vitamin B12, and folic acid levels in Alzheimer’s disease, mild cognitive impairment, and healthy elderly: Baseline characteristics in subjects of the Australian Imaging Biomarker Lifestyle study. J Alzheimers Dis 27: , 909–922. |
[39] | Zinck JW , de Groh M , MacFarlane AJ ((2015) ) Genetic modifiers of folate, vitamin B-12, and homocysteine status in a cross-sectional study of the Canadian population. Am J Clin Nutr 101: , 1295–1304. |
[40] | Amos R , Dawson D , Fish D , Leeming R , Linnell J ((1994) ) Guidelines on the investigation and diagnosis of cobalamin and folate deficiencies. Clin Lab Haematol 16: , 101–115. |
[41] | Vogiatzoglou A , Refsum H , Johnston C , Smith S , Bradley K , De Jager C , Budge M , Smith A ((2008) ) Vitamin B12 status and rate of brain volume loss in community-dwelling elderly. Neurology 71: , 826–832. |