Association of Tooth Loss with Alzheimer’s Disease Tau Pathologies Assessed by Positron Emission Tomography
Abstract
Background:
Deterioration of the oral environment is one of the risk factors for dementia. A previous study of an Alzheimer’s disease (AD) model mouse suggests that tooth loss induces denervation of the mesencephalic trigeminal nucleus and neuroinflammation, possibly leading to accelerated tau dissemination from the nearby locus coeruleus (LC).
Objective:
To elucidate the relevance of oral conditions and amyloid-β (Aβ) and tau pathologies in human participants.
Methods:
We examined the number of remaining teeth and the biofilm–gingival interface index in 24 AD-spectrum patients and 19 age-matched healthy controls (HCs). They also underwent positron emission tomography (PET) imaging of Aβ and tau with specific radiotracers, 11C-PiB and 18F-PM-PBB3, respectively. All AD-spectrum patients were Aβ-positive, and all HCs were Aβ-negative. We analyzed the correlation between the oral parameters and radiotracer retention.
Results:
No differences were found in oral conditions between the AD and HC groups. 11C-PiB retentions did not correlate with the oral indices in either group. In AD-spectrum patients, brain-wide, voxel-based image analysis highlighted several regions, including the LC and associated brainstem substructures, as areas where 18F-PM-PBB3 retentions negatively correlated with the remaining teeth and revealed the correlation of tau deposits in the LC (r = –0.479, p = 0.018) primarily with the hippocampal and neighboring areas. The tau deposition in none of the brain regions was associated with the periodontal status.
Conclusions:
Our findings with previous preclinical evidence imply that tooth loss may enhance AD tau pathogenesis, promoting tau spreading from LC to the hippocampal formation.
INTRODUCTION
The number of people with dementia worldwide is estimated to increase from 57.4 million in 2019 to 152.8 million by 2050 [1]. Alzheimer’s disease (AD) is neuropathologically characterized by depositions of abnormal amyloid-β (Aβ) and phosphorylated tau aggregates in the brain. AD has recently been regarded as a continuum among three distinct clinically defined entities consisting of preclinical AD, prodromal AD (mild cognitive impairment [MCI]), and AD dementia [2]. To date, some advances in disease-modifying treatments of AD have been achieved, as represented by the review and approval of a few Aβ-targeting drugs by the US Food and Drug Administration [3–5]. However, there is still controversy regarding their effectiveness, adverse events, and costs in real-world clinical settings [6–8]. Hence, there is still an urgent requirement to identify modifiable risk factors for AD, such as education, vascular issues, and depression [9, 10] to prevent disease onset.
Deterioration of the oral environment, including tooth loss and periodontal disease, has been suggested as a factor predisposing to the development of dementia in several cohort studies [11–17]. Tooth loss in humans has been linked to the onset of dementia via various pathways [18], such as nutritional imbalances resulting from impaired masticatory function [19] and decreased cerebral blood flow in the prefrontal cortex [20]. In a nonclinical study using transgenic AD mice, tooth loss caused denervation of the trigeminal mesencephalic nucleus (Vmes), with its nerve endings at the root apex. This alteration was followed by degeneration of the locus coeruleus (LC) located near the Vmes, conceivably via activation of inflammatory microglia [21]. LC is also known as a region presenting the earliest tau pathology that occurs in youth [22, 23], and tau deposits in this location are likely to spread transsynaptically to the hippocampal formation at an initial tau pathology stage proposed by Braak and Braak [24]. However, a long-term gap exists between the occurrence of tau lesions and neuronal loss in the LC [25], and its pathophysiology is still unknown. In the aforementioned study using a mouse model, hippocampal degeneration and dementia-like behavior were also found following degeneration of Vmes and LC, suggesting the impact of tooth loss on the pathological progressions and neurofunctional deteriorations in AD [21].
Periodontal disease is an infectious inflammatory condition caused by periodontopathogenic bacteria, chronic exposure to which has been associated with an increased risk of the development of AD [26]. The potential link between periodontitis and AD can be direct, via the invasion of bacteria and its products into the brain, or indirect, via the systemic inflammation caused by the presence of periodontal bacteria [27]. Regarding the association with AD pathologies, studies have suggested that, by using model mice and postmortem brains, periodontal pathogens and their enzymes could promote the Aβ depositions and tau phosphorylation [28–30]. In humans, associations between the biofilm–gingival interface (BGI) index [31] and cognitive impairment have been reported in four studies [32–35]. Two of these studies indicated an association of the BGI index with the Mini-Mental State Examination (MMSE) score [34, 35], while the others reported no such links [32, 33].
A number of clinical studies using neuroimaging have examined the relationship between tooth loss, periodontal disease, and AD pathogenesis. In magnetic resonance imaging (MRI) studies, the number of remaining teeth was negatively correlated with gray matter volume [36, 37], particularly in the parahippocampal gyrus of AD patients [38, 39]. The magnitude of periodontal disease in cognitively normal participants was associated with the brain Aβ load in a positron emission tomography (PET) study [40], whereas a prospective cohort study did not show any significant association between periodontal disease and Aβ PET positivity [41]. Furthermore, no tau PET studies have explored the relationship between the number of remaining teeth and the severity of periodontitis and tau lesions in the brain. Thus, the association between the oral environment and AD pathogenesis in humans remains elusive.
The recent development of PET ligands has enabled us to assess tau deposits in humans [42]. Several tau PET ligands have been shown to quantify and visualize tau pathology with AD and aging, and their clinical application as diagnostic and possibly prognostic tools is anticipated [43]. 18F-PM-PBB3 (APN-1607/florzolotau) is one of the novel tau PET ligands, which captures tau lesions with high contrast [44], and its potential has already been demonstrated in several clinical studies of AD [45–48]. In the present study, we hypothesized that tooth loss could provoke neuronal damage in the brainstem and subsequent acceleration of AD neuropathologies, while periodontal disease has also been suggested to be linked with abnormal protein deposits in the brain. Consequently, we evaluated the number of remaining teeth and the severity of periodontitis in participants who underwent Aβ (11C-PiB) and tau (18F-PM-PBB3) PET scans in order to correlate these oral statuses with AD pathogenesis.
METHODS
Participants
We enrolled 51 participants aged over 65 in this study between January 2020 and June 2022. Participants were recruited from affiliated hospitals and the National Institutes for Quantum Science and Technology volunteer association. The subjects were comprised of 19 AD and 13 MCI cases diagnosed according to the National Institute of Neurological and Communicative Disorders and Stroke/Alzheimer’s Disease and Related Disorders Association criteria [49] and Petersen’s criteria [50], respectively. Nineteen participants who did not meet these criteria and had no history of neurologic and psychiatric disorders were included as healthy controls (HCs). All participants underwent screening to exclude those with any organic brain complications or disorders, such as altered states of consciousness, prior head injuries requiring hospitalization and treatment, or a history of apparent cerebral infarction or hemorrhage.
Participants subsequently underwent a battery of neuropsychological evaluations, MRI examinations, and Aβand tau PET scans as part of the clinical trials described below. The neuropsychological evaluations assessed cognitive, executive, and functional deficits using the MMSE, Frontal Assessment Battery (FAB), and Clinical Dementia Rating (CDR) scales. For participants experiencing difficulties understanding test instructions due to cognitive deficits, we accommodated the guidance by providing explanations in simpler language. Furthermore, we requested caregivers to accompany these subjects, which facilitated each participant’s successful completion of almost all testing procedures. The examination findings were analyzed to assess their consistency with the diagnostic criteria. Besides, three experienced PET experts (K.T., H.E., Y.T.) also assessed the presence or absence of Aβ deposition through visual inspection of 11C-PiB-PET images [51]. Seven MCI and one AD patient with no evidence of Aβ deposition were excluded from the study, and 24 subjects in the AD-spectrum patients (six MCI and 18 AD) with Aβ positivity and 19 HCs with Aβ negativity were included in the subsequent analyses. No participants had undergone tooth extraction, incision and drainage of a periodontal abscess, or tooth preparation between the PET scans and the oral examinations.
This study was conducted according to the Declaration of Helsinki on Biomedical Studies Involving Human Subjects and approved by the National Institutes for Quantum Science and Technology Certified Review Board (19-034) and the Institutional Review Board of the Tokyo Dental College (967). Written informed consent was obtained from all participants and spouses or other close family members when participants were cognitively impaired. This study was registered with UMIN Clinical Trial Registry (UMIN-CRT; number 000038858).
Remaining teeth and periodontal condition of the participants
A dentist (H.M.) carried out comprehensive dental data acquisition. The dentist counted the number of remaining teeth, including the erupted third molars and residual roots, because the loss of these teeth providing no occlusion contact could also induce the neurodegeneration of Vmes [21]. The periodontal tissues were assessed using pocket probing depth (PPD, mm) and bleeding on probing (BOP, %). PPD is the distance from the base of the periodontal pocket to the gingival margin, which becomes deeper due to gingival swelling and irrevocable loss of connective tissue attachment due to tissue destruction associated with periodontal disease. BOP refers to the finding of bleeding from the periodontal pocket at the time of PPD measurement, and teeth without this finding are considered to be at low risk for periodontal disease progression. PPD was recorded at the worst site around each tooth, and teeth with BOP were recorded as positive. Additionally, the extent of BOP was calculated as a percentage of all teeth. With PPD and BOP, we determined each participant’s biofilm–gingival interface (BGI) index and classified it into the following five levels.
BGI-H: biofilm–gingival interface-healthy (PPD≤3 mm, BOP extent scores < 10%)
BGI-G: BGI-gingivitis (PPD≤3 mm, BOP extent scores > 10%)
P1: BGI-deep lesion/low bleeding (PPD≥4 mm, BOP extent scores < 10%)
P2: BGI-deep lesion/moderate bleeding (PPD≥4 mm, BOP extent scores 10% to 50%)
P3: BGI-deep lesion/severe bleeding (PPD≥4 mm, BOP extent scores≥50%)
The BGI index is a categorization of periodontal disease developed to reflect an individual’s inflammatory, microbial, and immune response statuses and is assessed using a combination of PPD and BOP [31].
PET and MRI data acquisition
Aβ and tau deposits in all participants were assessed using PET scans with 11C-PiB and 18F-PM-PBB3, respectively, as part of the clinical trials (UMIN-CRT; number 000026385, 000029608, 000030248, and 000043458). The scan protocols follow the procedures described elsewhere [44, 48]. Briefly, each PET scan was performed over 20 min. 11C-PiB PET images were acquired at 50–70 min after the radioligand injection (injected dose, 508.2±125.9 MBq; molar activity, 83.5±20.7 GBq/μmol), and 18F-PM-PBB3 PET images were obtained at 90–110 min after the injection (injected dose, 194.5±55.3 MBq; molar activity, 250.9±89.3 GBq/μmol). PET scans were mainly performed using a Biograph mCT flow system (Siemens Healthcare, Erlangen, Germany), and a Discovery MI (GE Healthcare, Chicago, IL, USA) was also used for some cases (five 11C-PiB scans and three 18F-PM-PBB3 scans in AD-spectrum patients and five 11C-PiB scans in HCs). The acquired PET images were reconstructed using the filtered back projection method with a Hanning filter (4.0 mm full-width at half-maximum). MRI examination was conducted along with PET scans by a 3-tesla scanner (MAGNETOM Verio; Siemens Healthcare, Erlangen, Germany). Structural three-dimensional T1-weighted images were acquired by magnetization prepared using a rapid acquisition gradient-echo sequence (echo time [TE], 1.95 ms; repetition time [TR], 2300 ms; inversion time [TI], 900 ms; field of view [FOV], 250 mm; flip angle, 9°; acquisition matrix, 512×512; and axial slice thickness, 1 mm).
Image analysis
All images were preprocessed using PMOD software (version 4.0, PMOD Technologies Ltd, Zürich, Switzerland), FreeSurfer 6.0 (https://surfer.nmr.mgh.harvard.edu/), and Statistical Parametric Mapping software (SPM12, Wellcome Department of Cognitive Neurology, London, UK). PET images were applied to motion correction and were coregistered with individual anatomical T1-weighted MR images. We generated parametric PET images showing the standardized uptake value ratio (SUVR), which is a ratio of the radioactivity concentration between each voxel and a reference region devoid of target elements such as Aβ and tau deposits. In this study, we defined the reference in the cerebellar cortex. PET images were then spatially normalized to the Montreal Neurologic Institute space using the Diffeomorphic Anatomical Registration Through Exponentiated Lie Algebra algorithm [52]. Normalized PET images were also smoothed with a Gaussian kernel at 8-mm full-width at half maximum in voxel-wise analyses.
We investigated the relationship between oral parameters and Aβ and tau deposits in the brain by conducting a voxel-wise, brain-wide analysis. Oral parameters were introduced as an explanatory variable in the SPM regression model. Then a T-map was generated to explore clusters of voxels showing significant correlations of local SUVR values for Aβ and tau PET tracers with oral parameters. Subsequently, post-hoc correlation analysis and group comparison of SUVRs were conducted by defining volumes of interest (VOI) on the anatomical structures containing the identified clusters by using preset template volumes derived from the automated anatomical labeling atlas (AAL atlas) [53]. We calculated an average SUVR in each defined VOIs and then conducted the correlation analysis and group comparison of the SUVR values. Furthermore, a voxel-wise correlational search of the whole brain was undertaken with tau PET tracer SUVR values in the above-mentioned VOIs as explanatory variables to pursue the possible propagation of tau pathologies. Considering the variability caused by different PET scanners, PET images used for voxel-wise analysis were restricted to those obtained with the Biograph mCT flow system (32 11C-PiB and 40 18F-PM-PBB3 PET images).
Statistical analyses
Demographic data, dental status, and VOI-based SUVR values were statistically analyzed using IBM SPSS (version 28, IBM Corp., Armonk, NY, USA) and GraphPad Prism (version 9, GraphPad Software, San Diego, CA, USA). Group comparisons were conducted using the Mann-Whitney U test and Pearson’s chi-squared test (sex and BGI index). Spearman’s rho test was used to evaluate correlations (p < 0.05). In voxel-wise analyses, the regression model was applied by SPM12. Given the limited sample size in this study, we included as covariates only the oral environment variables and demographic values showing the strongest correlation with the oral parameters. Likewise, in terms of threshold, we prioritized sensitivity by initially using a lenient threshold of p < 0.05 (uncorrected), which we subsequently adjusted to p < 0.001 (uncorrected), and finally to p < 0.05 (family-wise error; FWE -corrected). These thresholds are listed in each figure legend.
RESULTS
Demographic and dental data
The demographics of the participants are shown in Table 1. These values are listed as median [1st quartile, 3rd quartile]. No significant differences in sex, age, and years of schooling were found between the diagnostic groups. The AD-spectrum group showed significant cognitive deterioration as assessed by MMSE and FAB (p < 0.001), although the clinical dementia severity was relatively mild, with 22 out of 24 cases scored as CDR 0.5 or 1. Table 2 shows the dental data of both groups. We did not find any significant differences in oral parameters between the groups. Aging was negatively correlated with the number of remaining teeth in HCs (r = –0.476, p = 0.039), while no demographic data, including cognitive impairment, were correlated with oral parameters in AD-spectrum patients (Supplementary Table 1). Therefore, we treated age as a confounding factor in the neuroimaging analyses of HCs.
Table 1
Demographic data
HCs | AD | p | |
Number | 19 | 24 | – |
Sex (Female, Male) | 7, 12 | 14, 10 | 0.161 |
Age, y | 72.0 [71.0, 76.0] | 76.0 [73.5, 79.5] | 0.154 |
Years of schooling, y | 15.0 [14.0, 16.0] | 12.0 [12.0, 16.0] | 0.075 |
Onset, y | – | 2.00 [1.0, 4.0] | – |
MMSE | 29.0 [28.0, 30.0] | 22.0 [19.0, 24.3] | <0.001 |
FAB | 16.0 [15.5, 16.0] | 11.5 [9.8, 14.0] | <0.001 |
CDR | |||
0.5 | – | 8 | – |
1 | – | 14 | – |
2 | – | 2 | – |
3 | – | 0 | – |
Mann–Whitney U test, Pearson’s chi-squared test (sex). Values are listed as median [1st quartile, 3rd quartile]. AD, Alzheimer’s disease; CDR, Clinical Dementia Rating; FAB, frontal assessment battery; HCs, healthy controls; MMSE, Mini-Mental State Examination.
Table 2
Dental status
HCs (N = 19) | AD (N = 24) | p | |
Number of teeth | |||
Remaining teeth | 24.0 [20.5, 25.5] | 24.5 [17.3, 27.0] | 0.650 |
Periodontal disease | |||
BGI index | |||
BGI-H | 5 | 6 | 0.634 |
BGI-G | 0 | 2 | |
P1 | 10 | 12 | |
P2 | 4 | 4 | |
P3 | 0 | 0 |
Mann–Whitney U test (remaining teeth). Values listed as median [1st quartile, 3rd quartile], Pearson’s chi-squared test (BGI index). AD, Alzheimer’s disease; BGI, biofilm–gingival interface; HCs, healthy controls.
PET assessments of Aβ and tau deposits in the brain
Figure 1 shows representative axial Aβ and tau PET images in HCs and AD-spectrum patients (two individuals in each group). Retentions of both PET tracers were enhanced in extensive cerebral cortical areas of AD-spectrum patients. Additionally, tau PET tracer retention in the brainstem was increased in a subset of AD-spectrum patients with poor oral condition, as exemplified by a case displayed in the rightmost column of Fig. 1, with 10 remaining teeth and BGI index of 4. Statistical parametric maps of the brain-wide group comparison illustrated Aβ and tau deposition in the AD-spectrum group. These data demonstrated significantly enhanced retentions of the two tracers in the frontal cortex, temporo-parietal junction and cingulate cortex of AD-spectrum patients. Furthermore, the tau PET tracer retention was increased in the medial temporal cortex, including the hippocampal formation and inferior and lateral temporal cortices of the AD-spectrum group compared with HCs (p < 0.05, family-wise error corrected at cluster level) (Supplementary Figure 1).
Fig. 1
Representative PET images of Aβ and tau in HCs and AD-spectrum patients. Axial 11C-PiB (Aβ) and 18F-PM-PBB3 (tau) PET images of individual participants. PET images were coregistered with the individual anatomical magnetic resonance images. Representative PET images were shown according to participant group, and good and poor oral condition, respectively. White arrowhead indicates tau PET tracer binding in the brainstem. Tau PET tracer retention was increased in the brainstem in a subset of AD-spectrum patients with poor oral parameters compared with HCs. AD, Alzheimer’s disease; BGI, biofilm–gingival interface; HCs, healthy controls; MMSE, Mini Mental State Examination; PET, positron emission tomography; SUVR, standard uptake value ratio.
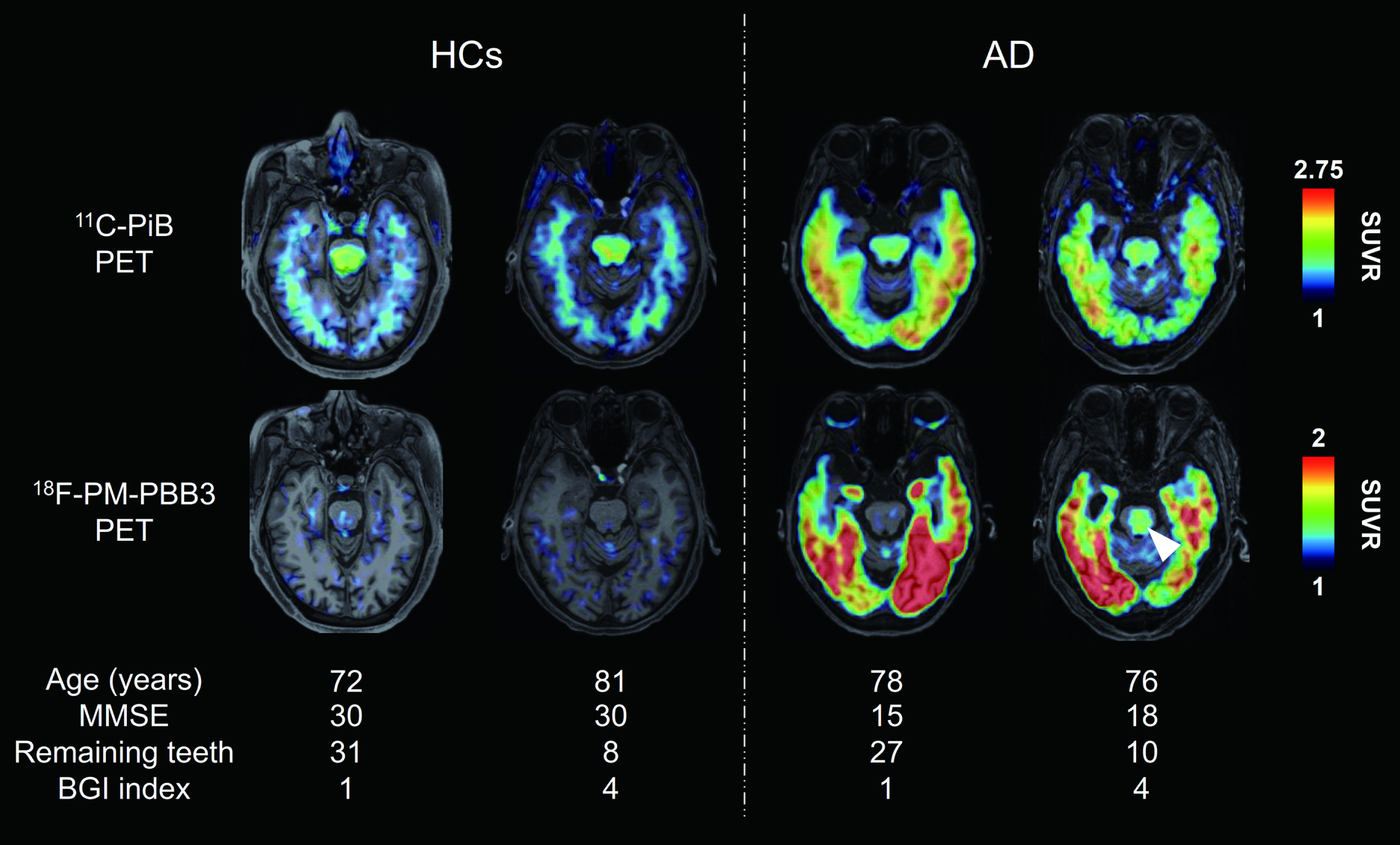
Correlations between oral parameters and PET tracer retention in the brain
We explored brain areas showing correlations of Aβ or tau depositions with the number of remaining teeth or the BGI index in each diagnostic group. A voxel-based analysis revealed that the number of remaining teeth in AD-spectrum patients showed a negative correlation with tau PET tracer retentions in the brainstem and frontal cortex. The brainstem cluster consisted mainly of the midbrain and pons, including the LC, while the frontal cortex cluster included the bilateral middle frontal, superior frontal, and inferior frontal gyri (see Supplementary Table 2). In contrast, no brain regions displayed associations between Aβ depositions and tooth number in these cases (Fig. 2). Neither Aβ nor tau lesions in AD-spectrum patients were correlated with the severity of periodontal status in the voxel-wise assay. In the brains of HCs, no areas exhibited associations of Aβ and tau PET signals with oral parameters.
Fig. 2
Correlations between oral parameters and PET tracer retention. A) T-score maps of correlation analyses between the number of remaining teeth and each PET tracer retention in HCs and AD-spectrum patients. B) T-score maps of correlation analyses between periodontal status (BGI index) and each PET tracer retention in HCs and AD-spectrum patients. Regions with significant correlations indicated an increase of tau PET tracer accumulation with a decrease in the number of remaining teeth (uncorrected, p < 0.05). The cluster size threshold was set to the expected voxel of each analysis. Results were expressed in the MNI coordinate space. AD, Alzheimer’s disease; BGI, biofilm–gingival interface; HCs, healthy controls; MNI, Montreal Neurological Institute; PET, positron emission tomography.
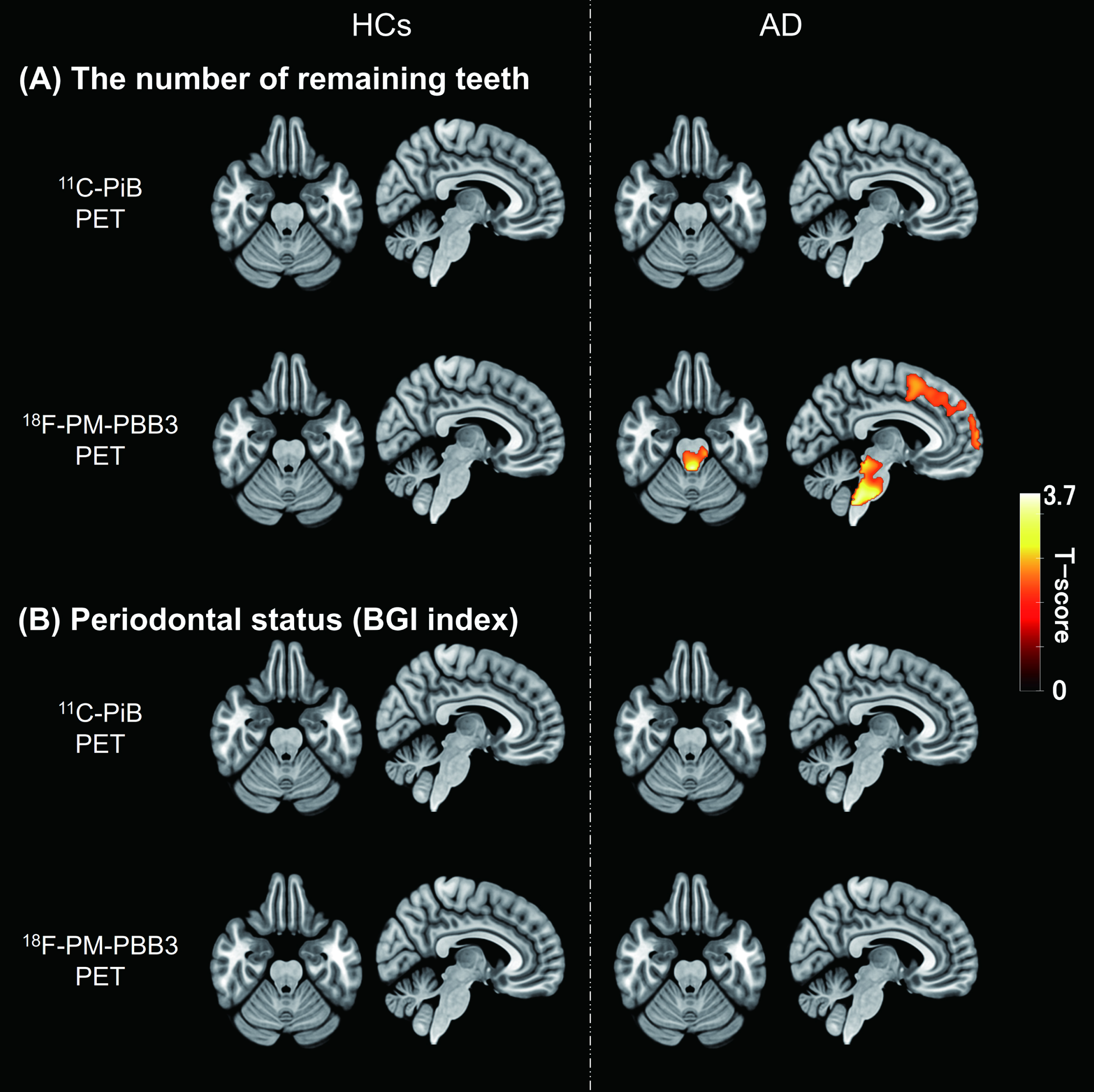
VOI-based examination of correlations between the number of remaining teeth and tau depositions
Since the brainstem, including the pons and the medial middle frontal cortex, was highlighted in the voxel-wise investigation, we further assessed the links between tau accumulations in the LC and frontal cortex, and tooth loss by defining VOIs in these anatomical structures, as depicted in Fig. 3A and Supplementary Figure 3, respectively. The group comparison of tau PET SUVR values in the LC did not show significant contrast between HC and AD-spectrum groups (p = 0.099, Fig. 3B), although SUVR in the LC was significantly correlated with the number of remaining teeth in AD-spectrum patients (r = –0.479, p = 0.018, confidence interval (CI) [–0.745, –0.081]) but not HCs (r = 0.375, p = 0.113, CI [–0.109, 0.716]), as illustrated in Fig. 3C. Conversely, SUVRs in the medial middle frontal cortex VOI demonstrated a significant difference between AD-spectrum patients and HCs (p < 0.0001, Supplementary Figure 2B) but was not significantly correlated with the number of remaining teeth in either of the two diagnostic groups (AD; r = –0.394, p = 0.057, CI [–0.695, 0.023], HCs; r = –0.169, p = 0.489, CI [–0.588, 0.322], Supplementary Figure 2C).
Fig. 3
Number of remaining teeth and correlation with tau lesions in the locus coeruleus and hippocampal region. A) Anatomical volume of interest of locus coeruleus (LC-VOI) on normalized PET and MRI images of HCs and AD. B) Group comparison of tau PET SUVR values in LC-VOI between HCs and AD groups. The p value was estimated by Mann-Whitney U-test. Open and filled circles indicate HCs and AD-spectrum patients, respectively. C) Correlations of tau PET SUVR values in LC-VOI and the number of remaining teeth in HC and AD groups; r and p values were assessed by Spearman’s rank correlation test. D) T-maps illustrate that tau PET tracer accumulation was increased in the hippocampal region in association with an increase to LC in AD (uncorrected, p < 0.001). The hippocampus VOI (red) is also overlaid in the right brain image. The cluster size threshold was set to zero according to the size of the LC-VOI. The statistical map was overlaid on the MNI brain. AD, Alzheimer’s disease; HCs, healthy controls; LC, locus coeruleus; MNI, Montreal Neurological Institute; PET, positron emission tomography; SUVR, standard uptake value ratio; VOI, volume of interest.
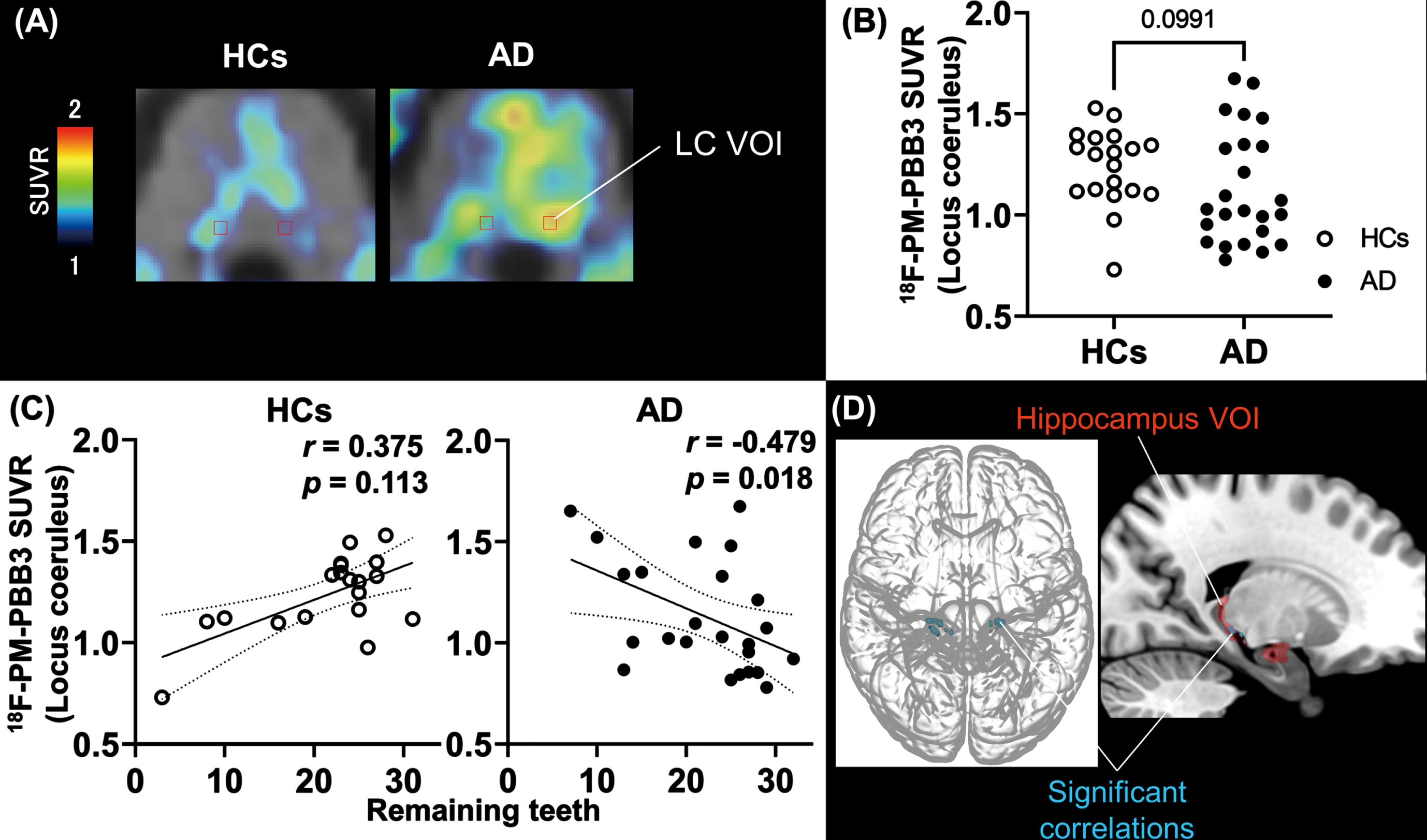
Correlations between tau loads in the LC and hippocampus
As tau tracer SUVRs in the LC but not frontal VOI presented a notable correlation with the tooth number in the AD-spectrum group, we sought brain areas with tau burdens closely associated with the LC tau load. SPM analysis with LC SUVRs as explanatory variables picked up hippocampal subregions (Fig. 3D), even after correction for multiple comparisons (Table 3). We also placed a VOI in the hippocampus, but correlations between local SUVR and tooth number were seen in neither AD nor HC group (AD, r = 0.009, p = 0.968; HCs, r = –0.116, p = 0.634). The results of all VOI-based correlation analyses are also included in Supplementary Table 1.
Table 3
Cortical regions correlated with 18F-PM-PBB3 binding in locus coeruleus
Hemisphere | Anatomical region | peak-level | MNI coordinates | ||||
T | p (uncorrected) | p (FWE) | x | y | z | ||
R | Lingual gyrus* | 7.868 | 0.000 | 0.003 | 12 | –30 | –10.5 |
R | Hippocampus* | 7.306 | 0.000 | 0.006 | 15 | –27 | –9 |
L | Hippocampus* | 6.129 | 0.000 | 0.040 | –16.5 | –25.5 | –7.5 |
L | Parahippocampal gyrus | 5.113 | 0.000 | 0.200 | –13.5 | –27 | –15 |
L | Hippocampus | 4.598 | 0.000 | 0.412 | 15 | –33 | –3 |
Statistical significance threshold was set as p < 0.001 (uncorrected.) and *p < 0.05 (family-wise error corrected). Anatomical regions were detected by an automated anatomical labeling atlas. FWE, family-wise error; L, left; MNI, Montreal Neurological Institute; R, right.
DISCUSSION
To our knowledge, this is the first clinical study to investigate associations between oral parameters and AD pathology using both Aβ and tau PET. Our results demonstrated that the number of remaining teeth in AD-spectrum patients was negatively correlated with tau accumulation in the brainstem (Figs. 2A, 3C) based on both whole-brain and VOI-based analyses. Additional whole-brain analysis revealed a correlation between tau burdens in the LC and hippocampal formation was also found (Table 3, Fig. 3D). The conjunction between the current clinical findings and previous preclinical evidence [21] suggests that tooth loss may enhance tau pathogenesis in AD patients, promoting the spread of tau from the LC to the hippocampal formation. Meanwhile, there was no significant difference in tau PET tracer accumulation in the LC between AD-spectrum patients and HCs (Fig. 3B), nor was a periodontal disease associated with retentions of Aβ and tau tracers.
Besides the lack of associations between Aβ depositions and periodontal disease in HCs and AD-spectrum patients, which were in agreement with findings in a previous cohort study [41], the current work has provided new findings on the absence of links between Aβ pathologies and tooth number in the two diagnostic groups. Correspondingly, previous in vivo assays using AD mouse models indicated that tooth extraction reduced the number of pyramidal cells in the hippocampus but did not augment Aβ accumulations in the cerebral cortex [54–56]. Meanwhile, the absence of a significant correlation in HCs could be attributed to limitations of the statistical power due to the small sample size. Therefore, further investigations with a larger scale will be required to examine whether accumulations of Aβ aggregates at a low level judged as negative findings in HCs are associated with oral parameters. In addition, HCs with Aβ accumulations at moderate and high levels, defined as preclinical AD cases, will need to be incorporated in future works to assess the relationship between this early-stage neuropathology and oral conditions.
Unlike Aβ depositions, tau fibril formations in specific brain areas were tightly related to the tooth number in AD cases. While postmortem study reported no association between the progression of Braak tau stages and the number of remaining teeth [57], an AD model mouse study has shown Aβ and phosphorylated tau accumulation in Vmes before the appearance of AD pathology in the cerebral cortex [58]. Our data imply the contribution of tooth loss to the tau pathogenesis in the brainstem at prodromal and early clinical stages of AD, although there were no correlations of the tooth number with Aβ PET tracer retentions in this region (Supplementary Figure 3). This notion is in line with the enhanced tau accumulations in LC and hippocampal formation as initial events of tau pathogenesis.
Likewise, an AD mouse model exhibited a loss of neurons in the hippocampal region following tooth extraction, presumably as a consequence of neurodegenerative tau pathologies in the brainstem, including Vmes and LC [21]. Both structures are located in the dorsal brainstem, where Aβ and tau lesions could occur in humans [59]. Vmes, the only primary sensory neuron located within the central nervous system [60, 61], receives synaptic and diffusible transmissions of norepinephrine from the LC [62, 63], which may act as a suppressor of inflammatory changes [64]. Microglial activation might be provoked in Vmes by tooth extraction in the presence of Aβ [21, 65] and may expand to the neighboring LC, leading to hyperphosphorylation and aggregation of tau [66, 67] in these regions. Degenerative changes in LC neurons and consequent declines of the norepinephrine release could further accelerate inflammatory microgliosis in the Vmes and LC. Furthermore, the propagation of tau pathologies from the LC of AD patients may be reinforced in the presence of Aβ deposits [68] indirectly by tooth loss.
It should also be noted that norepinephrine neurons in the LC have long and widespread projections to the cerebral cortex, and the degeneration of LC neurons elicited by tooth loss may trigger disruptions of axonal cytoskeletons, accumulations of microtubule-unbound tau species, which are prone to aggregation around the axonal terminals. This process could be boosted in a brain area with a heavy Aβ burden, such as the frontal cortex, but may not necessarily be coordinated with the intensification of fibrillar tau assemblies in the somatodendritic compartment of LC neurons, as indicated by the lack of relationships between tau tracer retentions in the LC and frontal cortex VOIs (Supplementary Figure 4). Additionally, tau accumulation in the frontal cortex may involve tau hyperphosphorylation arising from cerebral hypoperfusion, as supported by numerous animal model studies and clinical investigations [69–71], where tooth loss leads to reduced masticatory function and cerebral blood flow in the frontal cortex [72, 73]. Nevertheless, we found only a tendency to the correlation between the middle frontal tau accumulations and missing teeth in the post-hoc analysis and no correlation between tau depositions in the frontal and LC areas, implying a close association of the tooth loss with tau pathologies in the LC rather than the frontal cortex.
By contrast, intimate correlations between tau depositions in the LC and hippocampal formation might stem from the dissemination of tau pathologies through the axonal pathway connecting these two areas. It should also be noted that the number of remaining teeth was correlated with atrophy of several brain areas enriched with tau deposits from an early stage of AD, including the parahippocampal gyrus and olfactory cortex (Supplementary Figure 5), consistent with previous studies [38, 39]. Despite this volumetric finding, there was a lack of direct correlation between tau tracer retentions in these regions and the remaining tooth number (Supplementary Figure 6). It is presumable that robust estimation of this association might be impeded by the radioactivity spillover from adjacent choroid plexus [44] and atrophy-induced partial volume effects on the apparent radio signals in the target area. While the correlation between SUVRs of these regions and tooth number was not significant even after the VOI-based partial volume correction (Supplementary Figure 6), the technical issues would be more precisely addressed by implementing deconvolution-based, voxel-wise partial volume correction as applied elsewhere [74].
In the present study, there were no differences in LC tau PET signals between HCs and AD-spectrum patients (Fig. 3B), which could be related to the fact that tau lesions in the LC could emerge in HCs as pretangles [22], neurofibrillary tangles, and neuropil threads [75]. Declines in the tooth number do not affect this aging-related tau accumulation but may promote the formation and dissemination of tau fibrils in the presence of Aβ deposits in AD. The Aβ species involved in this synergy at an early stage of AD pathologies might be oligomers rather than fibrils, as oligomeric Aβ assemblies could be located in LC neurons [76].
The present study had several limitations. First, the sample size was small, leading to the difficulty in conducting a stratified analysis of MCI and AD to reveal stage-specific insights and focus on different sub-types of cognitive impairments. Second, the timing of tooth loss was unknown. In patients with dementia, the oral environment could deteriorate due to poor hygiene associated with cognitive decline, and the temporality of the association with the onset of AD was unclear. However, because most AD-spectrum patients in this study had mild cognitive deterioration, it is conceivable that deterioration of the oral environment associated with cognitive decline was not substantial. Third, the number of remaining teeth and periodontal health were maintained in both groups compared with people of the same generation [77] and did not significantly deteriorate in the AD group. In particular, most participants had no or only mild periodontal disease (Table 2). Thus, further investigation is still needed to elucidate the impact of periodontal disease. Conversely, the present study allowed us to examine the effect of the number of remaining teeth on brain lesions in a group in which the influence of periodontal disease was controlled. Finally, as a technical limitation, the small size of the LC might make it challenging to assess tracer retention accurately within the resolution of the current PET scanner. VOI-based partial volume correction did not modify the outcomes (Supplementary Figure 7), and validation utilizing neuromelanin-sensitive MRI may be advantageous, analogous to other studies [78, 79] in the future.
Despite these limitations, the first evaluation of oral parameters and AD pathologies using both Aβ and tau PET imaging in humans revealed a correlation between the number of remaining teeth and tau lesions in the brain. The current findings were in line with the hypotheses established by a preclinical study [21], which suggested that preventing tooth loss might be a practical approach to decelerating the progression of AD pathogenesis.
ACKNOWLEDGMENTS
The authors thank all patients and their caregivers for participation in this study, as well as researchers Keisuke Takahata, Kosei Hirata, and Masaki Oya, and radiochemists Kazunori Kawamura and Ming-Rong Zhang, and research ethics advisers at QST. We thank APRINOIA Therapeutics for kindly sharing a precursor of 18F-PM-PBB3. The authors acknowledge support with advice on statical analyses of the present study by Mutsumi Takagiwa at the Laboratory of Mathematics, Tokyo Dental College, and the recruitment of patients by Hitoshi Shinotoh at the Neurology Clinic Chiba, Shunichiro Shinagawa at the Department of Psychiatry, Jikei University School of Medicine, and by Shigeki Hirano at the Department of Neurology, Chiba University.
We also thank Helen Jeays, BDSc AE, from Edanz (https://jp.edanz.com/ac) for editing a draft of this manuscript.
FUNDING
This study was supported in part by MEXT/JSPS KAKENHI grant numbers 22K19636 to T.K.G., M.H., and T.G., JP16H05324 to M.H., JP18K07543 to H.S., and JP22K15776 to K.T., by AMED under grant numbers JP18dm0207018, JP19dm0207072, JP18dk0207026 to M.H., by J.S.T. grant numbers JPMJMS2024 to M.H., by the Private University Research Branding Project from MEXT of Japan to Tokyo Dental College to H.M., and by Biogen Idec Inc. and APRINOIA Therapeutics.
CONFLICT OF INTEREST
Hitoshi Shimada and Makoto Higuchi hold patents on compounds related to the present report (JP 5422782/EP 12 884 742.3/CA2894994/HK1208672).
DATA AVAILABILITY
The data that support the findings of this study are available from the corresponding author on reasonable request.
SUPPLEMENTARY MATERIAL
[1] The supplementary material is available in the electronic version of this article: https://dx.doi.org/10.3233/JAD-230581.
REFERENCES
[1] | GBD 2019 Dementia Forecasting Collaborators ((2022) ) Estimation of the global prevalence of dementia in 2019 and forecasted prevalence in 2050: An analysis for the Global Burden of Disease Study 2019.. Lancet Public Health 7: , e105–e125. |
[2] | Jack CR , Bennett DA , Blennow K , Carrillo MC , Dunn B , Haeberlein SB , Holtzman DM , Jagust W , Jessen F , Karlawish J , Liu E , Molinuevo JL , Montine T , Phelps C , Rankin KP , Rowe CC , Scheltens P , Siemers E , Snyder HM , Sperling R , Elliott C , Masliah E , Ryan L , Silverberg N ((2018) ) NIA-AA Research Framework: Toward a biological definition of Alzheimer’s disease. Alzheimers Dement 14: , 535–562. |
[3] | Rabinovici GD ((2021) ) Controversy and progress in Alzheimer’s disease - FDA approval of aducanumab. N Engl J Med 385: , 771–774. |
[4] | Mintun MA , Lo AC , Duggan Evans C , Wessels AM , Ardayfio PA , Andersen SW , Shcherbinin S , Sparks J , Sims JR , Brys M , Apostolova LG , Salloway SP , Skovronsky DM ((2021) ) Donanemab in early Alzheimer’s disease. N Engl J Med 384: , 1691–1704. |
[5] | Swanson CJ , Zhang Y , Dhadda S , Wang J , Kaplow J , Lai RYK , Lannfelt L , Bradley H , Rabe M , Koyama A , Reyderman L , Berry DA , Berry S , Gordon R , Kramer LD , Cummings JL ((2021) ) A randomized, double-blind, phase 2b proof-of-concept clinical trial in early Alzheimer’s disease with lecanemab, an anti-Aβ protofibril antibody. Alzheimers Res Ther 13: , 80. |
[6] | Whittington MD , Campbell JD , Rind D , Fluetsch N , Lin GA , Pearson SD ((2022) ) Cost-effectiveness and value-based pricing of aducanumab for patients with early Alzheimer disease.. Neurology 98: , e968–e977. |
[7] | Ross EL , Weinberg MS , Arnold SE ((2022) ) Cost-effectiveness of aducanumab and donanemab for early Alzheimer disease in the US. JAMA Neurol 79: , 478–487. |
[8] | Filippi M , Cecchetti G , Spinelli EG , Vezzulli P , Falini A , Agosta F ((2022) ) Amyloid-related imaging abnormalities and β-amyloid-targeting antibodies: A systematic review. JAMA Neurol 79: , 291–304. |
[9] | Xu W , Tan L , Wang HF , Jiang T , Tan MS , Tan L , Zhao QF , Li JQ , Wang J , Yu JT ((2015) ) Meta-analysis of modifiable risk factors for Alzheimer’s disease. J Neurol Neurosurg Psychiatry 86: , 1299–1306. |
[10] | Yu JT , Xu W , Tan CC , Andrieu S , Suckling J , Evangelou E , Pan A , Zhang C , Jia J , Feng L , Kua EH , Wang YJ , Wang HF , Tan MS , Li JQ , Hou XH , Wan Y , Tan L , Mok V , Tan L , Dong Q , Touchon J , Gauthier S , Aisen PS , Vellas B ((2020) ) Evidence-based prevention of Alzheimer’s disease: Systematic review and meta-analysis of 243 observational prospective studies and 153 randomised controlled trials. J Neurol Neurosurg Psychiatry 91: , 1201–1209. |
[11] | Takeuchi K , Ohara T , Furuta M , Takeshita T , Shibata Y , Hata J , Yoshida D , Yamashita Y , Ninomiya T ((2017) ) Tooth loss and risk of dementia in the community: The Hisayama Study.. J Am Geriatr Soc 65: , e95–e100. |
[12] | Yoo JJ , Yoon JH , Kang MJ , Kim M , Oh N ((2019) ) The effect of missing teeth on dementia in older people: A nationwide population-based cohort study in South Korea. BMC Oral Health 19: , 1–10. |
[13] | Sparks Stein P , Steffen MJ , Smith C , Jicha G , Ebersole JL , Abner E , Dawson D 3rd ((2012) ) Serum antibodies to periodontal pathogens are a risk factor for Alzheimer’s disease. Alzheimers Dement 8: , 196–203. |
[14] | Chen CK , Wu YT , Chang YC ((2017) ) Association between chronic periodontitis and the risk of Alzheimer’s disease: A retrospective, population-based, matched-cohort study. Alzheimers Res Ther 9: , 56. |
[15] | Lin CS ((2018) ) Revisiting the link between cognitive decline and masticatory dysfunction. BMC Geriatr 18: , 5. |
[16] | Thomson WM , Barak Y ((2021) ) Tooth loss and dementia: A critical examination. J Dent Res 100: , 226–231. |
[17] | Weijenberg RAF , Scherder EJA , Lobbezoo F ((2011) ) Mastication for the mind–the relationship between mastication and cognition in ageing and dementia. Neurosci Biobehav Rev 35: , 483–497. |
[18] | Jou YT ((2018) ) Dental deafferentation and brain damage: A review and a hypothesis. Kaohsiung J Med Sci 34: , 231–237. |
[19] | Yamamoto T , Kondo K , Hirai H , Nakade M , Aida J , Hirata Y ((2012) ) Association between self-reported dental health status and onset of dementia: A 4-year prospective cohort study of older Japanese adults from the Aichi Gerontological Evaluation Study (AGES) Project. Psychosom Med 74: , 241–248. |
[20] | Hasegawa Y , Ono T , Hori K , Nokubi T ((2007) ) Influence of human jaw movement on cerebral blood flow. J Dent Res 86: , 64–68. |
[21] | Goto T , Kuramoto E , Dhar A , Wang RPH , Seki H , Iwai H , Yamanaka A , Matsumoto SE , Hara H , Michikawa M , Ohyagi Y , Leung WK , Chang RCC ((2020) ) Neurodegeneration of trigeminal mesencephalic neurons by the tooth loss triggers the progression of Alzheimer’s disease in 3×Tg-AD model mice. J Alzheimers Dis 76: , 1443–1459. |
[22] | Braak H , Del Tredici K ((2011) ) The pathological process underlying Alzheimer’s disease in individuals under thirty. Acta Neuropathol 121: , 171–181. |
[23] | Giorgi FS , Ryskalin L , Ruffoli R , Biagioni F , Limanaqi F , Ferrucci M , Busceti CL , Bonuccelli U , Fornai F ((2017) ) The neuroanatomy of the reticular nucleus locus coeruleus in Alzheimer’s disease. Front Neuroanat 11: , 80. |
[24] | Braak H , Braak E ((1991) ) Neuropathological stageing of Alzheimer-related changes. Acta Neuropathol 82: , 239–259. |
[25] | Satoh A , Iijima KM ((2019) ) Roles of tau pathology in the locus coeruleus (LC) in age-associated pathophysiology and Alzheimer’s disease pathogenesis: Potential strategies to protect the LC against aging. Brain Res 1702: , 17–28. |
[26] | Hajishengallis G , Chavakis T ((2021) ) Local and systemic mechanisms linking periodontal disease and inflammatory comorbidities. Nat Rev Immunol 21: , 426–440. |
[27] | Wang RP-H , Ho YS , Leung WK , Goto T , Chang RC-C ((2019) ) Systemic inflammation linking chronic periodontitis to cognitive decline. Brain Behav Immun 81: , 63–73. |
[28] | Ishida N , Ishihara Y , Ishida K , Tada H , Funaki-Kato Y , Hagiwara M , Ferdous T , Abdullah M , Mitani A , Michikawa M , Matsushita K ((2017) ) Periodontitis induced by bacterial infection exacerbates features of Alzheimer’s disease in transgenic mice. NPJ Aging Mech Dis 3: , 15. |
[29] | Ilievski V , Zuchowska PK , Green SJ , Toth PT , Ragozzino ME , Le K , Aljewari HW , O’Brien-Simpson NM , Reynolds EC , Watanabe K ((2018) ) Chronic oral application of a periodontal pathogen results in brain inflammation, neurodegeneration and amyloid beta production in wild type mice.. PLoS One 13: , e0204941. |
[30] | Dominy SS , Lynch C , Ermini F , Benedyk M , Marczyk A , Konradi A , Nguyen M , Haditsch U , Raha D , Griffin C , Holsinger LJ , Arastu-Kapur S , Kaba S , Lee A , Ryder MI , Potempa B , Mydel P , Hellvard A , Adamowicz K , Hasturk H , Walker GD , Reynolds EC , Faull RLM , Curtis MA , Dragunow M , Potempa J ((2019) ) Porphyromonas gingivalis in Alzheimer’s disease brains: Evidence for disease causation and treatment with small-molecule inhibitors.. Sci Adv 5: , eaau3333. |
[31] | Offenbacher S , Barros SP , Beck JD ((2008) ) Rethinking periodontal inflammation. J Periodontol 79: , 1577–1584. |
[32] | Naorungroj S , Schoenbach VJ , Beck J , Mosley TH , Gottesman RF , Alonso A , Heiss G , Slade GD ((2013) ) Cross-sectional associations of oral health measures with cognitive function in late middle-aged adults: A community-based study. J Am Dent Assoc 144: , 1362–1371. |
[33] | Naorungroj S , Schoenbach VJ , Wruck L , Mosley TH , Gottesman RF , Alonso A , Heiss G , Beck J , Slade GD ((2015) ) Tooth loss, periodontal disease, and cognitive decline in the Atherosclerosis Risk in Communities (ARIC) study. Community Dent Oral Epidemiol 43: , 47–57. |
[34] | Kim D-H , Han G-S ((2022) ) The relationship between periodontal disease and cognitive impairment in older adults of Korea. Spec Care Dentist 42: , 170–176. |
[35] | Yang Y , Liang L , Cai J , You J , Liao X ((2023) ) Improving oral hygiene for better cognitive health: Interrelationships of oral hygiene habits, oral health status, and cognitive function in older adults. J Adv Nurs 00: , 1–12. |
[36] | Kobayashi T , Kubota M , Takahashi T , Nakasato A , Nomura T , Furuya J , Kondo H ((2018) ) Effects of tooth loss on brain structure: A voxel-based morphometry study. J Prosthodont Res 62: , 337–341. |
[37] | Dintica CS , Rizzuto D , Marseglia A , Kalpouzos G , Welmer AK , Wårdh I , Bäckman L , Xu W ((2018) ) Tooth loss is associated with accelerated cognitive decline and volumetric brain differences: A population-based study. Neurobiol Aging 67: , 23–30. |
[38] | Lin CS , Lin HH , Fann SW , Lee WJ , Hsu ML , Wang SJ , Fuh JL ((2020) ) Association between tooth loss and gray matter volume in cognitive impairment. Brain Imaging Behav 14: , 396–407. |
[39] | Egashira R , Umezaki Y , Mizutani S , Obata T , Yamaguchi M , Tamai K , Yoshida M , Makino M , Naito T ((2021) ) Relationship between cerebral atrophy and number of present teeth in elderly individuals with cognitive decline. Exp Gerontol 144: , 111189. |
[40] | Kamer AR , Pirraglia E , Tsui W , Rusinek H , Vallabhajosula S , Mosconi L , Yi L , McHugh P , Craig RG , Svetcov S , Linker R , Shi C , Glodzik L , Williams S , Corby P , Saxena D , de Leon MJ ((2015) ) Periodontal disease associates with higher brain amyloid load in normal elderly. Neurobiol Aging 36: , 627–633. |
[41] | Adam HS , Lakshminarayan K , Wang W , Norby FL , Mosley T , Walker KA , Gottesman RF , Meyer K , Hughes TM , Pankow JS , Wong DF , Jack CR Jr , Sen S , Lutsey PL , Beck J , Demmer RT ((2022) ) The prospective association between periodontal disease and brain imaging outcomes: The Atherosclerosis Risk in Communities study. J Clin Periodontol 49: , 322–334. |
[42] | Maschio C , Ni R ((2022) ) Amyloid and tau positron emission tomography imaging in Alzheimer’s disease and other tauopathies. Front Aging Neurosci 14: , 838034. |
[43] | Groot C , Villeneuve S , Smith R , Hansson O , Ossenkoppele R ((2022) ) Tau PET imaging in neurodegenerative disorders.. J Nucl Med 63: , 20S–26S. |
[44] | Tagai K , Ono M , Kubota M , Kitamura S , Takahata K , Seki C , Takado Y , Shinotoh H , Sano Y , Yamamoto Y , Matsuoka K , Takuwa H , Shimojo M , Takahashi M , Kawamura K , Kikuchi T , Okada M , Akiyama H , Suzuki H , Onaya M , Takeda T , Arai K , Arai N , Araki N , Saito Y , Trojanowski JQ , Lee VMY , Mishra SK , Yamaguchi Y , Kimura Y , Ichise M , Tomita Y , Zhang M-R , Suhara T , Shigeta M , Sahara N , Higuchi M , Shimada H ((2021) ) High-contrast in vivo imaging of tau pathologies in Alzheimer’s and non-Alzheimer’s disease tauopathies.. Neuron 109: , 42–58.e8. |
[45] | Lu J , Bao W , Li M , Li L , Zhang Z , Alberts I , Brendel M , Cumming P , Lu H , Xiao Z , Zuo C , Guan Y , Zhao Q , Rominger A ((2020) ) Associations of [18F]-APN-1607 tau PET binding in the brain of Alzheimer’s disease patients with cognition and glucose metabolism. Front Neurosci 14: , 604. |
[46] | Xu X , Ruan W , Liu F , Gai Y , Liu Q , Su Y , Liang Z , Sun X , Lan X ((2021) ) 18F-APN-1607 tau positron emission tomography imaging for evaluating disease progression in Alzheimer’s disease. Front Aging Neurosci 13: , 789054. |
[47] | Zhang H , Wang M , Lu J , Bao W , Li L , Jiang J , Zuo C , and Alzheimer’s Disease Neuroimaging Initiative ((2021) ) Parametric estimation of reference signal intensity for semi-quantification of tau deposition: A flortaucipir and [18F]-APN-1607 study. Front Neurosci 15: , 598234. |
[48] | Endo H , Tagai K , Ono M , Ikoma Y , Oyama A , Matsuoka K , Kokubo N , Hirata K , Sano Y , Oya M , Matsumoto H , Kurose S , Seki C , Shimizu H , Kakita A , Takahata K , Shinotoh H , Shimada H , Tokuda T , Kawamura K , Zhang M-R , Oishi K , Mori S , Takado Y , Higuchi M ((2022) ) A machine learning-based approach to discrimination of tauopathies using [18 F]PM-PBB3 PET images. Mov Disord 37: , 2236–2246. |
[49] | McKhann G , Drachman D , Folstein M , Katzman R , Price D , Stadlan EM ((1984) ) Clinical diagnosis of Alzheimer’s disease: Report of the NINCDS-ADRDA Work Group under the auspices of Department of Health and Human Services Task Force on Alzheimer’s Disease. Neurology 34: , 939–944. |
[50] | Petersen RC , Smith GE , Waring SC , Ivnik RJ , Tangalos EG , Kokmen E ((1999) ) Mild cognitive impairment: Clinical characterization and outcome. Arch Neurol 56: , 303–308. |
[51] | Yamane T , Ishii K , Sakata M , Ikari Y , Nishio T , Ishii K , Kato T , Ito K , Senda M ((2017) ) Inter-rater variability of visual interpretation and comparison with quantitative evaluation of 11C-PiB PET amyloid images of the Japanese Alzheimer’s Disease Neuroimaging Initiative (J-ADNI) multicenter study. Eur J Nucl Med Mol Imaging 44: , 850–857. |
[52] | Ashburner J ((2007) ) A fast diffeomorphic image registration algorithm. Neuroimage 38: , 95–113. |
[53] | Rolls ET , Huang CC , Lin CP , Feng J , Joliot M ((2020) ) Automated anatomical labelling atlas 3. Neuroimage 206: , 116189. |
[54] | Oue H , Miyamoto Y , Okada S , Koretake K , Jung CG , Michikawa M , Akagawa Y ((2013) ) Tooth loss induces memory impairment and neuronal cell loss in APP transgenic mice. Behav Brain Res 252: , 318–325. |
[55] | Oue H , Miyamoto Y , Koretake K , Okada S , Doi K , Jung C-G , Michikawa M , Akagawa Y ((2016) ) Tooth loss might not alter molecular pathogenesis in an aged transgenic Alzheimer’s disease model mouse. Gerodontology 33: , 308–314. |
[56] | Taslima F , Jung CG , Zhou C , Abdelhamid M , Abdullah M , Goto T , Saito T , Saido TC , Michikawa M ((2021) ) Tooth loss induces memory impairment and gliosis in App knock-in mouse models of Alzheimer’s disease. J Alzheimers Dis 80: , 1687–1704. |
[57] | Stein PS , Desrosiers M , Donegan SJ , Yepes JF , Kryscio RJ ((2007) ) Tooth loss, dementia and neuropathology in the Nun study. quiz. J Am Dent Assoc 138: , 1314–22; quiz 1381-2. |
[58] | Kuramoto E , Kitawaki A , Yagi T , Kono H , Matsumoto S-E , Hara H , Ohyagi Y , Iwai H , Yamanaka A , Goto T ((2022) ) Development of a system to analyze oral frailty associated with Alzheimer’s disease using a mouse model. Front Aging Neurosci 14: , 935033. |
[59] | Uematsu M , Nakamura A , Ebashi M , Hirokawa K , Takahashi R , Uchihara T ((2018) ) Brainstem tau pathology in Alzheimer’s disease is characterized by increase of three repeat tau and independent of amyloid β . Acta Neuropathol Commun 6: , 1. |
[60] | Capra NF , Wax TD ((1989) ) Distribution and central projections of primary afferent neurons that innervate the masseter muscle and mandibular periodontium: A double-label study. J Comp Neurol 279: , 341–352. |
[61] | Luo P , Li J ((1991) ) Monosynaptic connections between neurons of trigeminal mesencephalic nucleus and jaw-closing motoneurons in the rat: An intracellular horseradish peroxidase labelling study. Brain Res 559: , 267–275. |
[62] | Toyoda H , Won J , Kim W , Kim H , Davy O , Saito M , Kim D , Tanaka T , Kang Y , Oh SB ((2022) ) The nature of noradrenergic volume transmission from locus coeruleus to brainstem mesencephalic trigeminal sensory neurons. Front Cell Neurosci 16: , 841239. |
[63] | Takahashi T , Shirasu M , Shirasu M , Kubo KY , Onozuka M , Sato S , Itoh K , Nakamura H ((2010) ) The locus coeruleus projects to the mesencephalic trigeminal nucleus in rats. Neurosci Res 68: , 103–106. |
[64] | O’Neill E , Harkin A ((2018) ) Targeting the noradrenergic system for anti-inflammatory and neuroprotective effects: Implications for Parkinson’s disease. Neural Regeneration Res 13: , 1332–1337. |
[65] | Sonoda R , Kuramoto E , Minami S , Matsumoto SE , Ohyagi Y , Saito T , Saido T , Noguchi K , Goto T ((2023) ) Reduced Autophagy in Aged Trigeminal Neurons Causes Amyloid β Diffusion. J Dent Res 102: , 938–946. |
[66] | Hayes A , Thaker U , Iwatsubo T , Pickering-Brown SM , Mann DMA ((2002) ) Pathological relationships between microglial cell activity and tau and amyloid beta protein in patients with Alzheimer’s disease. Neurosci Lett 331: , 171–174. |
[67] | Kitazawa M , Yamasaki TR , LaFerla FM ((2004) ) Microglia as a potential bridge between the amyloid beta-peptide and tau. Ann N Y Acad Sci 1035: , 85–103. |
[68] | Busche MA , Hyman BT ((2020) ) Synergy between amyloid-β and tau in Alzheimer’s disease. Nat Neurosci 23: , 1183–1193. |
[69] | Park JH , Hong JH , Lee SW , Ji HD , Jung JA , Yoon KW , Lee JI , Won KS , Song BI , Kim HW ((2019) ) The effect of chronic cerebral hypoperfusion on the pathology of Alzheimer’s disease: A positron emission tomography study in rats. Sci Rep 9: , 14102. |
[70] | Shimada T , Shindo A , Matsuyama H , Yata K , Niwa A , Sasaki R , Ayaki T , Maki T , Wakita H , Tomimoto H ((2019) ) Chronic cerebral hypoperfusion upregulates leptin receptor expression in astrocytes and tau phosphorylation in tau transgenic mice. Neurosci Lett 704: , 133–140. |
[71] | Yamauchi H , Kagawa S , Kusano K , Ito M , Okuyama C ((2022) ) Misery perfusion and tau deposition in atherosclerotic major cerebral artery disease: A 18F-florzolotau positron emission tomography study.. Stroke 53: , e500–e503. |
[72] | Kamiya K , Narita N , Iwaki S ((2016) ) Improved prefrontal activity and chewing performance as function of wearing denture in partially edentulous elderly individuals: Functional near-infrared spectroscopy study. PLoS One 11: , e0158070. |
[73] | Onozuka M , Fujita M , Watanabe K , Hirano Y , Niwa M , Nishiyama K , Saito S ((2003) ) Age-related changes in brain regional activity during chewing: A functional magnetic resonance imaging study. J Dent Res 82: , 657–660. |
[74] | Tohka J , Reilhac A ((2008) ) Deconvolution-based partial volume correction in Raclopride-PET and Monte Carlo comparison to MR-based method. Neuroimage 39: , 1570–1584. |
[75] | Grudzien A , Shaw P , Weintraub S , Bigio E , Mash DC , Mesulam MM ((2007) ) Locus coeruleus neurofibrillary degeneration in aging, mild cognitive impairment and early Alzheimer’s disease. Neurobiol Aging 28: , 327–335. |
[76] | Kelly L , Seifi M , Ma R , Mitchell SJ , Rudolph U , Viola KL , Klein WL , Lambert JJ , Swinny JD ((2021) ) Identification of intraneuronal amyloid beta oligomers in locus coeruleus neurons of Alzheimer’s patients and their potential impact on inhibitory neurotransmitter receptors and neuronal excitability. Neuropathol Appl Neurobiol 47: , 488–505. |
[77] | Dental Health Division of Health Policy Bureau Ministry of Health, Labour andWelfare, Japan (2017) Survey of Dental Diseases 2016. https://www.mhlw.go.jp/toukei/list/dl/62-28-02.pdf, Last updated September 15, 2017, Accessed on January 20, 2023. |
[78] | Jacobs HIL , Becker JA , Kwong K , Engels-Domínguez N , Prokopiou PC , Papp KV , Properzi M , Hampton OL , d’Oleire Uquillas F , Sanchez JS , Rentz DM , El Fakhri G , Normandin MD , Price JC , Bennett DA , Sperling RA , Johnson KA ((2021) ) in vivo and neuropathology data support locus coeruleus integrity as indicator of Alzheimer’s disease pathology and cognitive decline.eabj. Sci Transl Med 13: , 2511. |
[79] | Dahl MJ , Mather M , Werkle-Bergner M , Kennedy BL , Guzman S , Hurth K , Miller CA , Qiao Y , Shi Y , Chui HC , Ringman JM ((2022) ) Locus coeruleus integrity is related to tau burden and memory loss in autosomal-dominant Alzheimer’s disease. Neurobiol Aging 112: , 39–54. |