TOMM40 ‘523 Genotype Distinguishes Patterns of Cognitive Improvement for Executive Function in APOEɛ3 Homozygotes
Abstract
Background:
TOMM40 ‘523 has been associated with cognitive performance and risk for developing Alzheimer’s disease independent of the effect of APOE genotype. Few studies have considered the longitudinal effect of this genotype on change in cognition over time.
Objective:
Our objective was to evaluate the relationship between TOMM40 genotype status and change in cognitive performance in the TOMMORROW study, which was designed to prospectively evaluate an algorithm that includes TOMM40 ‘523 for genetic risk for conversion to mild cognitive impairment.
Methods:
We used latent growth curve models to estimate the effect of TOMM40 allele carrier (short, very long) status on the intercept and slope of change in cognitive performance in four broad cognitive domains (attention, memory, executive function, and language) and a combined overall cognitive score over 30 months.
Results:
TOMM40 very long allele carriers had significantly lower baseline performance for the combined overall cognitive function score (B = –0.088, p = 0.034) and for the executive function domain score (B = –0.143, p = 0.013). Slopes for TOMM40 very long carriers had significantly greater increases over time for the executive function domain score only. In sensitivity analyses, the results for executive function were observed in participants who remained clinically stable, but not in those who progressed clinically over the study duration.
Conclusions:
Our results add to the growing body of evidence that TOMM40, in the absence of APOE ɛ4, may contribute to cognitive changes with aging and dementia and support the view that mitochondrial function is an important contributor to Alzheimer’s disease risk.
INTRODUCTION
A growing body of evidence suggests that TOMM40, a gene located next to APOE on chromosome 19, may influence the risk of developing Alzheimer’s disease (AD) [1]. Variants of this gene are associated with cognitive performance and rate of cognitive decline [2, 3], brain volume in dementia-associated regions [4–6], and amyloid-β-induced cellular damage [7]. The close proximity of TOMM40 and APOE genes makes it challenging to disassociate the independent effects of each, especially given the outsized effects of APOE ɛ4 compared to most single nucleotide polymorphisms. Despite this linkage disequilibrium, TOMM40 likely operates via a unique mechanism compared to the underlying processes of risk conferred by the APOE ɛ4 allele. TOMM40 encodes a protein critical to mitochondrial function and likely affects AD risk through effects on mitochondrial bioenergetic processes [8], whereas the mechanisms through which APOE confers risk may associate with lipid transport between cells, given the essential functions of lipids related to neuronal growth, maintenance, repair, and synaptic plasticity [9].
A variable length homopolymeric T variation has been identified in intron 6 of the TOMM40 gene, rs 10524523 (‘523) and is associated with earlier age of onset for late-onset AD. The alleles at this locus have been grouped into short (S) long (L) and very long (VL) depending on the T length [10]. However, understanding the molecular effect of this variant on AD etiology has been difficult given the high linkage disequilibrium in this region of chromosome 19. One way to disentangle these potentially confounded effects of TOMM40 ‘523 and APOE ɛ4 is to study the effects of TOMM40 ‘523 among APOE ɛ3 homozygotes, removing the well-established effect of ɛ4 alleles on cognitive function. Two previous studies focused solely on APOE ɛ3 homozygotes reported that carriers of two short alleles for TOMM40 ‘523 had lower baseline cognitive performance [11] and steeper rates of decline over longitudinal follow up [2]. Studies that did not restrict their samples to APOE ɛ3 homozygotes have nevertheless confirmed independent effects of TOMM40 from APOE ɛ4 in cognitive performance and decline [12–14], cognitive test-retest effects [15] and timing of dementia onset [10].
Few studies have conducted TOMM40 genotyping in large samples with well-characterized cognitive performance across multiple occasions [2, 11, 14, 15]. The goal of the current paper is to evaluate the relationship between TOMM40 genotype status and change in cognitive performance across 30 months of the TOMMORROW study, which was designed to prospectively evaluate an algorithm that includes TOMM40 ‘523 for genetic risk for conversion to mild cognitive impairment (MCI).
METHODS
Study design
The present study is a secondary analysis of data from the TOMMORROW study [16]. One of the primary objectives of the TOMMORROW study was to evaluate a genetic biomarker algorithm for timing of onset of MCI due to AD conferred by age, APOE, and TOMM40 ‘523. The second primary objective was to test the efficacy of low dose pioglitazone to delay the onset of MCI due to AD in cognitively normal older adult participants. The study randomized participants considered to be at “high risk” to receive pioglitazone treatment or placebo and assigned those at “low risk” to placebo only. To evaluate changes in cognition without influence by experimental treatment, we excluded participants randomized to receive pioglitazone (see Fig. 1). Receiving the drug could potentially confound the effects of genotype combinations on cognitive change over time. Although the study stratified participants into high and low risk categories for conversion to MCI based on age and genotype, we did not use this stratification for the present analysis, instead combining all participants assigned to placebo into one sample comprising a more continuous distribution of risk. To address our research question regarding the effects of TOMM40 ‘523 independent of the effects of APOE ɛ4, analyses included only APOE ɛ3/ɛ3 homozygotes. Finally, we excluded participants who carried a TOMM40 ‘523 Long allele, the TOMM40 allele typically linked to the APOE ɛ4 allele, to eliminate any confound of this association.
Fig. 1
Inclusion and exclusion of participants from analysis.
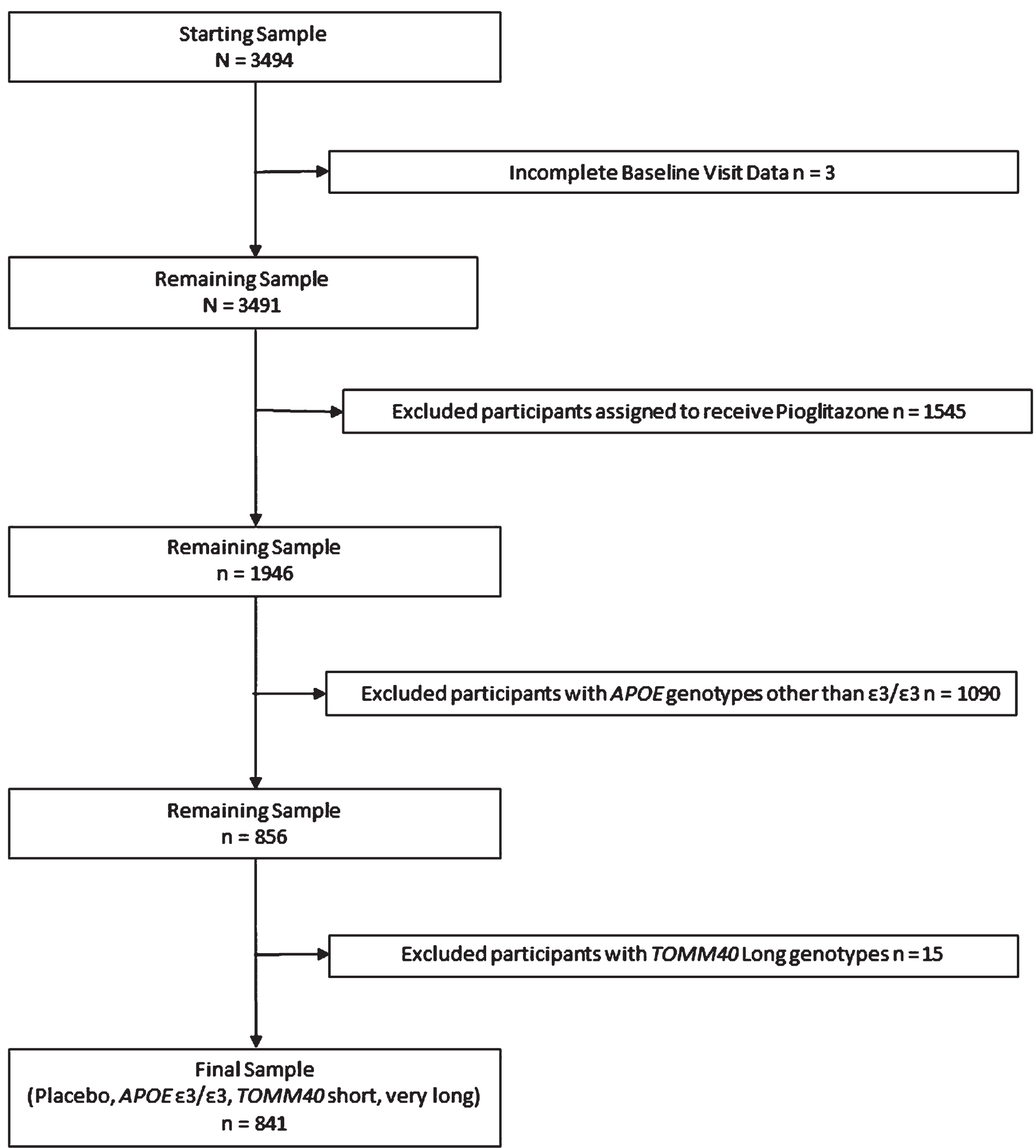
Participants
A complete description of participant selection and procedures has been published elsewhere [16]. Briefly, the original study included participants aged 65–83 at the initial screening visit. Study enrollment occurred between 2013 and 2015 and included 57 clinical sites from the United States, United Kingdom, Australia, Switzerland, and Germany. To be included, participants had to be able to perform cognitive tests within normal range and be fluent in the language in which tests were administered. The study excluded participants who were determined to have a Clinical Dementia Rating (CDR) scale global score greater than 0 or objectively measured memory scores falling lower than 1.5 standard deviations below the normative mean. Other exclusion criteria were any diagnosis of cognitive impairment or dementia, significant psychiatric illness, alcohol or drug abuse, or other diseases contraindicated for use with pioglitazone. Due to the study’s unequal randomization by high and low risk for conversion to MCI, the most frequent reason for screening failure was being at low genetic risk for development of MCI.
Procedures
The consent forms and process were approved by our institutional review board and was conducted in accord with the Helsinki Declaration of 1975. A three-visit enrollment process included a screening visit, a baseline visit, and a randomization visit. During the screening visit, participants were evaluated for cognitive status using the Mini-Mental State Examination (MMSE). If cognitively normal on this screen, blood samples were collected for genetic testing. At the baseline visit, inclusion and exclusion criteria for the study were reviewed and confirmed. The TOMMORROW neuropsychological test battery was administered to evaluate cognitive performance and confirm cognitive status as not impaired. If all entry criteria were met, participants were then randomized to active drug treatment or control based on a biomarker risk assessment algorithm. All low-risk participants were assigned to the placebo group and high-risk participants were randomized in a 1:1 ratio to drug or placebo.
Post-baseline neuropsychological assessments occurred every 6 months. The analysis described in this paper focused on the six-month post assessment follow up intervals, which we will refer to here as “waves.” The study duration was event-driven, where the primary endpoint event was conversion to MCI due to AD, and it anticipated a 5-year treatment phase to accumulate the predetermined event count. The study was terminated early following a pre-specified efficacy futility analysis, thus, available data for this analysis became sparse after 5 waves (30 months). To ensure accuracy and consistency across sites and time, the neuropsychological test results were centrally curated. To prevent practice effects common with repeated testing, the forms of the tests were counterbalanced across visits.
Measures
Neuropsychological Test Battery
A team of experts designed the neuropsychological assessment battery to identify individuals exhibiting early stages of cognitive impairment. The study team selected instruments for the test battery known to be appropriate for use in preclinical stages of disease and that have known psychometric and normative data in English-speaking populations. The study team conducted validation studies to ensure consistency across multiple languages of assessment in study participants [17]. Each measure represented one of five broad domains of cognitive function associated with age-related declines and development of AD (memory, language, attention, executive function, visuospatial/praxis), with no fewer than two measures assessed per domain. We used alternative versions of verbal learning and visual memory tests to minimize practice effects. Composite scores were calculated for the memory, language, attention, and executive function domains based on the mean-derived z-scores (Baseline Mean/Baseline Standard Deviation) of selected tests in the domain using non-missing baseline values and adjusting for age and education levels. Composite scores were based on the average z-scores for tests in these four domains. For the present study, we omitted tests that were not part of the previously estimated composite scores due to ceiling effects in cognitively-normal participants (visuospatial function and naming). The z-scores for the Trail Making test part A and part B were adjusted (multiplied by – 1.0) so that all measures in the battery were scaled in the same direction with high scores indicating high/good performance and low scores indicating low/poor performance. Table 1 summarizes the domains and measures selected for composite calculations. As observed with other AD prevention studies, the trial cohort was healthier overall, had higher education levels, and had a high representation of Caucasians than the corresponding general older adult population.
Table 1
Neuropsychological Test Composite Scores by Cognitive Domain
Cognitive Domain | Tests |
Attention | Wechsler Adult Intelligence Scale (WAIS-III) Digit Span Test – forward span |
Trail Making Test Part A | |
Episodic Memory | California Verbal Learning Test – 2nd Edition (CVLT-II)* |
Brief Visuospatial Memory Test – Revised (BVMT-R)* | |
Executive Function | Trail Making Test Part B |
Wechsler Adult Intelligence Scale (WAIS-III) | |
Digit Span Test – backward span | |
Language | Semantic fluency (animals) |
Lexical/phonemic fluency (F, A, and S) |
The scores for the tests in each domain were averaged to form a composite score, if there were no missing data. *Alternative versions of these tests were administered to minimize practice effects.
APOE and TOMM40 genotyping procedures
The TOMMORROW trial used Sanger sequencing methods followed by capillary electrophoresis to ascertain the TOMM40 ‘523 poly-T length and a pyrosequencing assay to determine the APOE genotype. Genetic testing was performed under an FDA Investigational Device Exemption in a College of American Pathologists and Clinical Laboratory Improvement Amendments accredited environment. Poly-T lengths were used to classify each TOMM40 allele according to the S, L, and VL convention from Roses et al. [10, 18].
Covariates
We included three covariates in the analyses age, sex, and years of education. Self-reported years of age and education are important predictors of cognitive performance. Self-reported sex was included as a potential predictor of cognitive performance, varying by cognitive domain. These covariates were collected by self-report at the screening visit. Educational attainment values were standardized using reported years of formal education and highest degree along with country-specific tables to assign years of education to report.
Statistical analysis
We used latent growth curve models to estimate the association of TOMM40 VL carrier status with the intercept and slope of cognitive change over five waves adjusting for covariates (age, years of education, and sex). To estimate group differences between participants who remained clinically stable (i.e., CDR = 0) and those who progressed clinically (i.e., CDR > 0), we conducted multiple group growth curve models. We estimated change in R2 between models with and without the predictor variable (TOMM40 allele status) as a measure of effect size, anticipating that effect sizes for single genes on cognition would be small to very small, on the order of 1% to 2%, as shown in prior similar research [11]. Missing data due to attrition was accounted for using a full information maximum likelihood algorithm with the understanding that data are likely not missing at random. To evaluate model fit, we used the ratio of χ2 to the model degrees of freedom and the Root Mean Squared Error of Approximation (RMSEA), a measure of the discrepancy between predicted and observed model values. Values closer to 0 indicate better fit (preferred values are < 0.09). Typically, these multiple fit indices are considered together as opposed to relying on any one indicator by itself. These latent models estimate all the pathways simultaneously which allows us to avoid multiple testing-induced inflation of type 1 error.
We estimated the statistical power for a latent growth curve model using Monte Carlo simulation following the guidelines of Muthén & Muthén [19]. The estimate included a normally distributed continuous dependent variable (i.e., cognitive scores), a categorical predictor (i.e., presence of TOMM40 very long alleles 0, 1), and adjustment for three covariates (i.e., age, sex, education). We estimated the power for five waves of longitudinal data based on the sample size of 841 observations available at baseline. The number of repetitions was set to 10,000 as recommended. We modeled missing data to match the pattern of longitudinal attrition observed in the data. The average was 7% attrition between waves [range 5% to 11%] with wave 5 representing 74% of the baseline observations. We estimated the effect size of the relationship between the independent and dependent variables to be small (i.e., β= 0.10 for the estimate of the slope of change over time reflecting an effect size of 0.32). Given a sample size of 841, we have 0.60 power to detect a small effect of TOMM40 VL (presence or absence) on the slope of change over time. All other estimates of independent variables on intercept and slope achieved an estimated power of 0.80 or greater. The small effect sizes of single genes on behavioral outcomes are well documented and require very large sample sizes to attain adequate statistical power. See Discussion for further detail.
RESULTS
We report descriptive statistics in Table 2. There were no statistically significant differences in mean age or years of education between carriers and non-carriers of TOMM40 VL alleles (p > 0.38). Using a χ2 test with Fisher’s exact estimation, there was no difference in the number of females and males in the groups with and without TOMM40 VL alleles (χ2(df) = 2.62 (1), p = 0.113).
Table 2
Demographics
Starting Sample | Remaining Sample | Final Sample | |||||
All | Low-risk placebo | High-risk placebo | All | Low-risk placebo | High-risk placebo | All | |
n = 3,494 | (n = 432) | (n = 1,514) | (n = 1,946) | (n = 276) | (n = 565) | (n = 841) | |
Age (years)* | |||||||
Mean (SD) | 74.0 (5.3) | 70.3 (4.0) | 74.6 (5.3) | 73.7 (5.3) | 70.1 (3.7) | 78.8 (2.3) | 76.0 (5.0) |
Range | (65–83) | (65–83) | (65–83) | (65–83) | (65–81) | (68–83) | (65–83) |
Age | |||||||
<75 y | 1650 (47.2%) | 359 83.1%) | 631 (41.7%) | 990 (50.9%) | 230 (83.3%) | 4 (0.7%) | 234 (27.8%) |
≥75 y | 1844 (52.8%) | 73 (16.9%) | 883 (58.3%) | 956 (49.1%) | 46 (16.7%) | 561 (99.3%) | 607 (72.2%) |
Gender | |||||||
Male | 1573 (45.0%) | 174 (40.3%) | 666 (43.9%) | 840 (43.1%) | 115 (41.7%) | 267 (47.35) | 382 (45.4%) |
Female | 1921 (55.0%) | 258 (59.8%) | 848 (56.1%) | 1106 (56.9%) | 161 (58.3%) | 298 (52.7%) | 459 (54.6%) |
Race | |||||||
White | 3368 (96.4%) | 420 (97.2%) | 1461 (96.5%) | 1881 (96.7%) | 271 (98.2%) | 548 (97.0%) | 819 (97.4%) |
Black or African American | 87 (2.5%) | 10 (2.3%) | 38 (2.5%) | 48 (2.5%) | 4 (1.4%) | 9 (1.6%) | 13 (1.5%) |
Other | 39 (1.1%) | 2 (0.5%) | 15 (1.0%) | 17 (0.9%) | 1 (0.4%) | 8 (1.4%) | 9 (1.1%) |
Ethnicity | |||||||
Non-Hispanic/ Latino Caucasian | 3455 (98.9%) | 424 (98.1%) | 1495 (98.7%) | 1919 (98.6%) | 270 (97.8%) | 554 (98.1%) | 824 (98.0%) |
Hispanic or Latino | 39 (1.1%) | 8 (1.9%) | 19 (1.3%) | 27 (1.4%) | 6 (2.2%) | 11 (1.9%) | 17 (2.0%) |
Years of formal education | |||||||
Mean (SD) | 14.7 (3.0) | 14.7 (2.9) | 14.7 (2.9) | 14.7 (2.9) | 14.6 (2.9) | 14.7 (3.1) | 14.7 (3.0) |
Range | (2–20) | (2–20) | (3–20) | (2–20) | (7–20) | (6–20) | (6–20) |
Tomm40 | |||||||
S/S | 441 (12.6%) | 101 (23.4%) | 173 (11.55) | 274 (14.1%) | 87 (31.5%) | 161 (28.5%) | 248 (29.5%) |
S/VL | 1005 (28.8%) | 183 (42.4%) | 428 (28.4%) | 611 (31.4%) | 136 (49.3%) | 404 (71.5%) | 540 (64.2%) |
VL/VL | 182 (5.2%) | 98 (22.7%) | 37 (2.6%) | 135 (6.9%) | 53 (19.2%) | 53 (6.3%) | |
S/L | 539 (15.4%) | 50 (11.6%) | 235 (15.5%) | 285 (14.6%) | |||
L/VL | 1160 (33.2%) | 562 (37.1%) | 562 (28.9%) | ||||
L/L | 167 (4.8%) | 79 (5.2%) | 79 (4.1%) | ||||
Baseline MMSE | |||||||
Mean (SD) | 28.5 (1.4) | 28.7 (1.3) | 28.5 (1.4) | 28.6 (1.4) | 28.8 (1.3) | 28.4 (1.3) | 28.5 (1.4) |
Range | (23–30) | (25–30) | (20–30) | (20–30) | (25–30) | (20–30) | (20–30) |
We used latent growth curve models to evaluate the role of TOMM40 VL carrier status (carriers (1 to 2 alleles) versus non-carriers (0 alleles)) on the intercept (baseline cognitive score) and slope of change in cognitive scores over five waves of data collection (in 6 month increments for a total of 2 years), adjusting for covariates age, sex, and years of education. Table 3 shows the results of the models. TOMM40 VL carriers had significantly lower baseline performance for the overall cognitive function score (B = –0.088, p = 0.034; ΔR2 = 0.01) and for the executive function domain score (B = –0.143, p = 0.013; ΔR2 = 0.01). TOMM40 VL carriers improved significantly more rapidly for only for the executive function domain score (B = 0.027, p = 0.030; ΔR2 = 0.03). See Fig. 2 for a visual illustration of the differences between TOMM40 VL carriers and non-carriers in the executive function domain. Note that changes in performance overall were in the positive direction, so a positive slope indicates more rapidimprovement.
Table 3
Results of Latent Growth Curve Models, unstandardized estimates
Overall Cognition B, p | Memory B, p | Attention B, p | Executive Function B, p | Language B, p | |
Intercept | |||||
Age | –0.028, < 0.001 | –0.028, < 0.001 | –0.028, < 0.001 | –0.033, < 0.001 | –0.025, < 0.001 |
Sex | 0.107, 0.005 | 0.421, < 0.001 | –0.092, 0.063 | –0.001, 0.988 | 0.109, 0.049 |
Education | 0.045, < 0.001 | 0.042, < 0.001 | 0.033, < 0.001 | 0.052, < 0.001 | 0.056, < 0.001 |
TOMM40 VL (Carrier/Non-Carrier) | –0.088, 0.034 | –0.061, 0.287 | –0.086, 0.104 | –0.143, 0.013 | –0.065, 0.273 |
Slope | |||||
Age | –0.002, 0.001 | –0.005, < 0.001 | –0.001, 0.433 | –0.002, 0.092 | –0.002, 0.161 |
Sex | –0.008, 0.211 | –0.023, 0.030 | 0.006, 0.619 | –0.009, 0.447 | –0.007, 0.522 |
Education | 0.001, 0.569 | –0.002, 0.328 | 0.000, 0.829 | 0.003, 0.102 | 0.000, 0.869 |
TOMM40 VL (Carrier/Non-Carrier) | 0.008, 0.233 | –0.003, 0.787 | 0.016, 0.177 | 0.027, 0.030 | –0.007, 0.572 |
Model Fit | |||||
χ2 (df) | 27.72 (22) | 83.03 (22) | 12.41 (22) | 28.66 (22) | 12.28 (22) |
RMSEA | 0.018 | 0.057 | 0.000 | 0.019 | 0.000 |
CFI | 0.99 | 0.98 | 1.00 | 0.99 | 1.00 |
Fig. 2
Differences in executive function performance between TOMM40 VL allele carriers (red dashed line) and non-carriers (blue solid line).
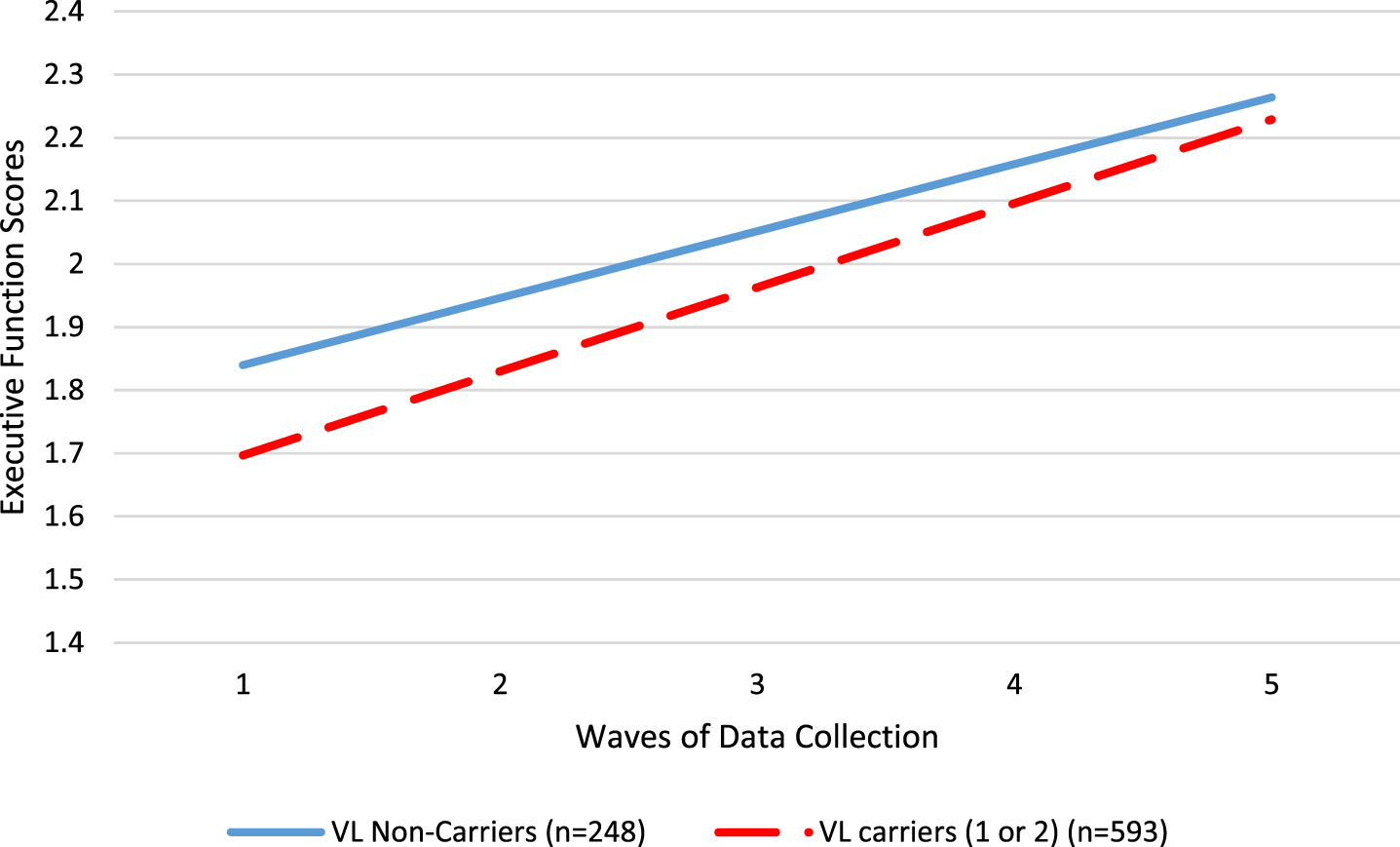
To evaluate whether these relationships differed between clinically stable participants (i.e., CDR = 0 at all timepoints, n = 679) and those who progressed clinically (CDR > 0 at any point, n = 161), we repeated the models in a multiple group analysis. Results indicated that the relationship between TOMM40 carrier status and executive function was present only in the clinically stable group (intercept B = –0.163, p = 0.006, slope B = 0.027, p = 0.004), but not in the group who progressed clinically (intercept B = –0.018, p = 0.904, slope B = 0.018, p = 0.551). The pattern of results in the other cognitive domains was consistent with the analysis of the total sample. For the full results of the sensitivity analysis, see Supplementary Table 1.
DISCUSSION
Our results suggest that TOMM40 VL allele carriers display a different pattern of cognitive performance and longitudinal change compared to those who do not carry a VL allele. In particular, TOMM40 VL allele carriers started at lower levels at baseline in overall cognitive scores and in executive function domain scores but showed greater rates of improvement over time in executive function, nearly catching up with those who carried two TOMM40 S alleles. It is worthwhile to note that, in sensitivity analysis, these results appeared to be driven by clinically stable participants, and not observed among the smaller subsample of participants who progressed clinically toward impairment. Previous studies report mixed results regarding which allele combination (short, long, very long) appears to be most advantageous in protecting cognitive performance and buffering against cognitive decline. Three prior longitudinal studies reported that VL carriers demonstrated superior performance at baseline in a variety of cognitive domains [3, 11, 14], though Yu et al. [2] reported no differences in baseline performance. Regarding change over time, three studies showed that VL carriers had a longitudinal advantage [2, 3, 20], while two showed that S carriers had slower declines [14] or stronger learning effects [15]. The findings of the current study suggested that the disadvantage seen for VL carriers at baseline, lessened over time by a faster rate of improvement, adding yet another pattern of results for consideration. Because our results showed this effect in those who remained unimpaired over the study duration, it is important to consider that TOMM40 may have different effects in individuals with cognitiveimpairment.
Signs of antagonistic pleiotropy are common in research on the effects of genotypes on behavioral outcomes over time. That is, a genotype that appears to have an advantage in earlier stages of life may confer a disadvantage later in life. Some research has shown that APOE ɛ4 carriers outperform non ɛ4 carriers earlier in life, but decline more rapidly or show less cognitive resilience over time [21]. One conclusion we draw from these mixed findings in existing literature is that timing of assessment is critical for understanding who will appear to perform better on any cross-sectional occasion. Cross-sectional findings cannot substitute for longitudinal evaluation of cognitive change. It is also important to place our findings in the context of cognitively normal participants who are unlikely to decline over the span of two to three years, especially on cognitive assessments designed to detect early signs of dementia. Previous research has been mixed in its level of detailed characterization of the cognitive status of participants included in analyses. Patterns of cognitive decline observed among individuals at risk for developing dementia are likely to differ from patterns of improvement over time (likely due to practice effects and rates of learning) that are seen in studies of cognitively normal older adults, such as the sample included in the present study.
Previous studies provide clues about potential confounds that lead to mixed results across studies including age at time of cognitive testing [15], family history of AD [3], and APOE ɛ4 carrier status [2, 11], all of which may moderate these observed associations between TOMM40 carrier status and cognitive performance and decline. Caselli et al. [15] reported a diminished test-retest effect for memory performance in TOMM40 VL carriers only among the younger portion of their sample (younger than age 60). Willette et al. [3] highlighted that TOMM40 VL carriers had better memory performance, but only when they were also negative for a family history of AD. Arpawong [12] demonstrated that the effects of APOE ɛ4 genotype are separable from the effects of TOMM40 genotype, reporting that APOE was associated with delayed memory recall, while TOMM40 was associated with immediate memory recall. It is reasonable that in the present study we observed genotype differences in executive function, as opposed to language, attention, or memory in high functioning, cognitively normal older adults, as executive function tasks are some of the earliest to decline as part of normal cognitive aging [22]. Of note, due to ceiling effects in our tests of visuospatial function, we were unable to draw conclusions about effects of TOMM40 genotype on this domain of cognitive performance that has been observed in previous studies [23]. Previous studies of the effects of TOMM40 genotype have reported influences on cognitive performance in a variety of domains including memory, visuospatial function, and language [2, 3, 11, 12, 14]. Our results suggest executive performance, which is typically associated with frontal lobe function, is particularly sensitive to TOMM40 genotype. We are unaware of a strongly established biological mechanism, such as regional variations in brain TOMM40 expression, that might account for this finding. TOMM40 has been found to associate with white matter integrity, though not restricted to the frontal lobe [24]. Advancing age is known to particularly affect executive function performance [25], as opposed to the amnestic state that presents as the most common AD clinical manifestation. Perhaps this indicates TOMM40’s effects on cognition pertain to the aging axis in cognitively normal older adults, rather than the more classical AD-associated bimesiotemporal axis. Since our findings contrast with previous literature, it is also possible that this may reflect limitations of measurement or study design rather than true underlying mechanisticrelationships.
Studies of single nucleotide polymorphisms as predictors of behavioral outcomes, such as cognitive performance, typically report very small effect sizes. This may be in part due to complex interactions of multiple genes that are not included in the analyses, and in part due to the difficulty in accurate measurement of behavioral traits compared to more objectively observed traits [26, 27]. The “fourth law of behavior genetics” suggests that complex behaviors are associated with many genes, each of which can only account for a tiny fraction of the total variability in the behavior [28]. Genes with easily detectable effect sizes such as APOE are rare[27].
Despite these disadvantages, there is firm justification for evaluating the role of TOMM40 on cognition. A body of research consistently suggests it plays a role in cognition and there are well-described mechanisms through which TOMM40 might influence AD risk and cognitive aging [8]. TOMM40 encodes a critical mitochondrial protein and different ‘523 poly-T lengths very likely affect neural mitochondrial function. The effects of the different TOMM40 ‘523 alleles on mitochondrial function itself are currently not well characterized; however, ‘523 poly-T length is known to influence TOMM40 transcription, and ultimately, protein levels [18, 29].
We chose to use growth-curve modeling because it allows us to estimate the trajectories of change over time and use predictors such as TOMM40 carrier status to describe group differences in the intercepts and slopes of the change in cognitive performance over time. We observed the effects of TOMM40 VL alleles on change over time only in the domain of executive function in this sample of high functioning, cognitively normal older adults. These results suggest that carriers of one or more VL TOMM40 allele may have advantages over time in the rates of cognitive improvement. Many studies of cognitive change over time in older adults display declines over years. By contrast, we did not detect declines, only greater or lesser degrees of improvement, likely due to the high functioning nature of our sample. These contrasting results suggest that participant age and timing of the arc of cognitive change during cognitive testing are important factors to account for when comparing findings across studies. Previous studies of the role of TOMM40 on longitudinal cognitive change vary in their inclusion of participants with cognitive impairment or clinically confirmed normal cognition. It is unclear from these studies whether participants were high functioning overall or included a wider range of cognitive status including normal cognition, MCI, and AD. Some studies did not report whether the cognitive status of their participants remained normal over time.
The present study was undertaken using the TOMMORROW dataset as it included a rigorously administered and curated neuropsychological testing program [30], included a large number of subjects that would enable analysis without the confounding influence of the APOE ɛ4 allele, and the data on the genetic and cognitive evaluation was collected prospectively in a blinded fashion thereby reducing the possibility of bias. However, our study has several limitations. It is possible that our observation period was too short (total of 30 months) to detect age-related declines in the cognitive performance of pre-symptomatic older adults. This is shorter than the follow-up period of previous reports [2, 11, 14, 15]. Thus, our ability to detect cognitive change is limited, particularly in our high-functioning sample selected to maximize the distinction between high and low risk for conversion to MCI. Although the test battery was designed to detect early evidence of change, it may not be sufficiently sensitive in this cohort. As a clinical trial cohort, our sample is highly educated, in better health than the general population, and is mostly Caucasian. Furthermore, the clinical trial population reflects the randomization criteria utilized in the TOMMORROW study and therefore is not representative of the general older adult population. We excluded participants who were determined by CDR scale to be not cognitively normal or memory scores lower than 1.5 standard deviations below the mean, thus ensuring a high performing sample and limiting our generalizability. Finally, though the present study was unable to conduct neuroimaging to identify the neural mechanisms by which TOMM40 may influence cognition, we refer readers to relevant studies of the effects of TOMM40 on areas of the brain that are commonly associated with memory and visuospatial function [4, 6]. To our knowledge, no associations with areas of the brain associated with executive function (i.e., prefrontal cortex) have been reported. Finally, our study did not use biomarkers to evaluate the mechanisms by which TOMM40 may influence brain or cognitive function. Many new AD biomarkers and imaging techniques have become widely available since to the initiation of this study and would not have been practical to implement in such a large, globally enrolledcohort.
Unique contributions of the present study include exclusion of APOE ɛ4 alleles to facilitate decoupling of the APOE ɛ4-TOMM40 ‘523 linkage disequilibrium, multiple cognitive tests summarized into distinct cognitive domains, and representation of participants from numerous countries around the world. Our results add to the growing body of evidence that TOMM40, in the absence of APOE ɛ4, may contribute to cognitive change with aging and dementia. Further, our findings support the increasingly accepted view that mitochondrial function is an important contributor to AD risk [31, 32]. These findings should be interpreted in the context of a growing body of converging evidence regarding the association of TOMM40 ‘523 with age at dementia onset [10], changes in brain volumes in dementia specific regions [4–6], and cognitive performance and decline [2, 3]. TOMM40 ‘523 merits investigation as a means of better understanding mechanisms, methods of early detection, and potential treatments for Alzheimer’s disease among those who do not carry an APOE ɛ4 allele.
ACKNOWLEDGMENTS
The authors gratefully acknowledge the study participants, their study partners, the clinical site Investigators, neuropsychologists, and staff for their commitment to executing the TOMMORROW study protocol. The authors acknowledge the contributions of Drs. Brenda Plassman, Heather Romero, and Kathleen Hayden of Duke University, who with KAW-B provided oversight of neuropsychology aspects of the TOMMORROW study. In addition, we recognize Dr. Richard Keefe, Alexandra Atkins, and Nicole Turcotte for their contributions to execution of the neuropsychological test battery. Thanks to Carl Chiang for reviewing and commenting on an early draft of the manuscript.
FUNDING
The TOMMORROW study was funded by Takeda Pharmaceuticals and Zinfandel Pharmaceuticals and the authors recognize the contributions of the Alliance team to study conduct.
CONFLICT OF INTEREST
AW has no conflicts of interest to report. RS is an Editorial Board Member of this journal but was not involved in the peer-review process nor had access to any information regarding its peer-review. DKB, SH, and AMS are full time employees of Zinfandel Pharmaceuticals. Additionally, AMS has a patent on the Biomarker Risk Assignment Algorithm. JW and RCA are full time employees of Takeda Pharmaceuticals. KAW-B received funding support from Takeda Pharmaceuticals during the study for work as the lead of the Neuropsychology Lead Office at Duke University. In addition, KAW-B reports personal fees from Biogen and a grant from VeraSci.
DATA AVAILABILITY
The datasets, including the redacted study protocol, redacted statistical analysis plan, and individual participants data supporting the results reported in this article, will be made available within 3 months from initial request, to researchers who provide a methodologically sound proposal. The data will be provided after its de-identification, in compliance with applicable privacy laws, data protection and requirements for consent and anonymization. Research proposal applications can be made to www.Vivli.org where they will be reviewed and approved by an independent review panel. After a signed data access agreement has been received, the study’s protocol, statistical analysis plan, blank case report forms, clinical study report, de-identified individual patient data, and a data dictionary defining each field in the dataset will be made available at www.Vivli.org.
SUPPLEMENTARY MATERIAL
[1] The supplementary material is available in the electronic version of this article: https://dx.doi.org/10.3233/JAD-230066.
REFERENCES
[1] | Bussies PL , Rajabli F , Griswold A , Dorfsman DA , Whitehead P , Adams LD , Mena PR , Cuccaro M , Haines JL , Byrd GS ((2020) ) Use of local genetic ancestry to assess TOMM40-523′ and risk for Alzheimer disease. Neurol Genet 6: , e404. |
[2] | Yu L , Lutz MW , Wilson RS , Burns DK , Roses AD , Saunders AM , Gaiteri C , De Jager PL , Barnes LL , Bennett DA ((2017) ) TOMM40′ 523 variant and cognitive decline in older persons with APOE ɛ3/3 genotype. Neurology 88: , 661–668. |
[3] | Willette AA , Webb JL , Lutz MW , Bendlin BB , Wennberg AM , Oh JM , Roses A , Koscik RL , Hermann BP , Dowling NM ((2017) ) Family history and TOMM40 ‘523 interactive associations with memory in middle-aged and Alzheimer’s disease cohorts. Alzheimers Dement 13: , 1217–1225. |
[4] | Burggren AC , Mahmood Z , Harrison TM , Siddarth P , Miller KJ , Small GW , Merrill DA , Bookheimer SY ((2017) ) Hippocampal thinning linked to longer TOMM40 poly-T variant lengths in the absence of the APOE ɛ4 variant. Alzheimers Dement 13: , 739–748. |
[5] | Laczó J , Andel R , Vyhnalek M , Matoska V , Kaplan V , Nedelska Z , Lerch O , Gazova I , Moffat SD , Hort J ((2015) ) The effect of TOMM40 on spatial navigation in amnestic mild cognitive impairment. Neurobiol Aging 36: , 2024–2033. |
[6] | Johnson SC , La Rue A , Hermann BP , Xu G , Koscik RL , Jonaitis EM , Bendlin BB , Hogan KJ , Roses AD , Saunders AM ((2011) ) The effect of TOMM40 poly-T length on gray matter volume and cognition in middle-aged persons with APOE ɛ3/ɛ3 genotype. Alzheimers Dement 7: , 456–465. |
[7] | Zeitlow K , Charlambous L , Ng I , Gagrani S , Mihovilovic M , Luo S , Rock DL , Saunders A , Roses AD , Gottschalk WK ((2017) ) The biological foundation of the genetic association of TOMM40 with late-onset Alzheimer’s disease. Biochim Biophys Acta Mol Basis Dis 1863: , 2973–2986. |
[8] | Chiba-Falek O , Gottschalk WK , Lutz MW ((2018) ) The effects of the TOMM40 poly-T alleles on Alzheimer’s disease phenotypes. Alzheimers Dement 14: , 692–698. |
[9] | O’Donoghue MC , Murphy SE , Zamboni G , Nobre AC , Mackay CE ((2018) ) APOE genotype and cognition in healthy individuals at risk of Alzheimer’s disease: A review. Cortex 104: , 103–123. |
[10] | Roses AD , Lutz MW , Amrine-Madsen H , Saunders AM , Crenshaw DG , Sundseth SS , Huentelman MJ , Welsh-Bohmer KA , Reiman EM ((2010) ) A TOMM40 variable-length polymorphism predicts the age of late-onset Alzheimer’s disease. Pharmacogenomics J 10: , 375–384. |
[11] | Watts A , Wilkins HM , Michaelis E , Swerdlow RH ((2019) ) TOMM40 ‘523 associations with baseline and longitudinal cognition in APOE ɛ3 homozygotes. J Alzheimers Dis 70: , 1059–1068. |
[12] | Arpawong TE , Pendleton N , Mekli K , McArdle JJ , Gatz M , Armoskus C , Knowles JA , Prescott CA ((2017) ) Genetic variants specific to aging-related verbal memory: Insights from GWASs in a population-based cohort. PloS One 12: , e0182448. |
[13] | Ferencz B , Laukka EJ , Lövdén M , Kalpouzos G , Keller L , Graff C , Wahlund L-O , Fratiglioni L , Bäckman L ((2013) ) The influence of APOE and TOMM40 polymorphisms on hippocampal volume and episodic memory in old age. Front Hum Neurosci 7: , 198. |
[14] | Payton A , Sindrewicz P , Pessoa V , Platt H , Horan M , Ollier W , Bubb VJ , Pendleton N , Quinn JP ((2016) ) A TOMM40 poly-T variant modulates gene expression and is associated with vocabulary ability and decline in nonpathologic aging. Neurobiol Aging 39: , 217-e1. |
[15] | Caselli RJ , Dueck AC , Huentelman MJ , Lutz MW , Saunders AM , Reiman EM , Roses AD ((2012) ) Longitudinal modeling of cognitive aging and the TOMM40 effect. Alzheimers Dement 8: , 490–495. |
[16] | Burns DK , Chiang C , Welsh-Bohmer KA , Brannan SK , Culp M , O’Neil J , Runyan G , Harrigan P , Plassman BL , Lutz M ((2019) ) The TOMMORROW study: Design of an Alzheimer’s disease delay-of-onset clinical trial. Alzheimers Dement (N Y) 5: , 661–670. |
[17] | Romero HR , Monsch AU , Hayden KM , Plassman BL , Atkins AS , Keefe RS , Brewster S , Chiang C , O’neil J , Runyan G ((2018) ) TOMMORROW neuropsychological battery: German language validation and normative study. Alzheimers Dement (N Y) 4: , 314–323. |
[18] | Linnertz C , Anderson L , Gottschalk W , Crenshaw D , Lutz MW , Allen J , Saith S , Mihovilovic M , Burke JR , Welsh-Bohmer KA ((2014) ) The cis-regulatory effect of an Alzheimer’s disease-associated poly-T locus on expression of TOMM40 and apolipoprotein E genes. Alzheimers Dement 10: , 541–551. |
[19] | Muthén LK , Muthén BO ((2002) ) How to use a Monte Carlo study to decide on sample size and determine power. Struct Equ Model 9: , 599–620. |
[20] | Zou H , Luo S , Lutz M , Bennett DA , Plassman BL , Welsh-Bohmer KA ((2022) ) Genotypic effect of the TOMM40’523 variant and APOE on longitudinal cognitive change over 4 years: The TOMMORROW Study. J Prev Alzheimers Dis 9: (Suppl 1), S60–S61. |
[21] | Mondadori CR , de Quervain DJ-F , Buchmann A , Mustovic H , Wollmer MA , Schmidt CF , Boesiger P , Hock C , Nitsch RM , Papassotiropoulos A ((2007) ) Better memory and neural efficiency in young apolipoprotein E ɛ4 carriers. Cereb Cortex 17: , 1934–1947. |
[22] | Lacreuse A , Raz N , Schmidtke D , Hopkins WD , Herndon JG ((2020) ) Age-related decline in executive function as a hallmark of cognitive ageing in primates: An overview of cognitive and neurobiological studies. Philos Trans R Soc B Biol Sci 375: , 20190618. |
[23] | Deters KD , Mormino EC , Yu L , Lutz MW , Bennett DA , Barnes LL ((2021) ) TOMM40-APOE haplotypes are associated with cognitive decline in non-demented Blacks. Alzheimers Dement 17: , 1287–1296. |
[24] | Lyall DM , Harris SE , Bastin ME , Maniega SM , Murray C , Lutz MW , Saunders AM , Roses AD , Hernández M del CV , Royle NA ((2014) ) Alzheimer’s disease susceptibility genes APOE and TOMM40, and brain white matter integrity in the Lothian Birth Cohort 1936. Neurobiol Aging 35: , 1513.e25–33. |
[25] | Buckner RL ((2004) ) Memory and executive function in aging and AD: Multiple factors that cause decline and reserve factors that compensate. Neuron 44: , 195–208. |
[26] | Chabris CF , Lee JJ , Benjamin DJ , Beauchamp JP , Glaeser EL , Borst G , Pinker S , Laibson DI ((2013) ) Why it is hard to find genes associated with social science traits: Theoretical and empirical considerations. Am J Public Health 103: , S152–S166. |
[27] | McGuffin P , Riley B , Plomin R ((2001) ) Toward behavioral genomics. Science 291: , 1232–1249. |
[28] | Chabris CF , Lee JJ , Cesarini D , Benjamin DJ , Laibson DI ((2015) ) The fourth law of behavior genetics. Curr Dir Psychol Sci 24: , 304–312. |
[29] | Bekris LM , Lutz F , Yu C-E ((2012) ) Functional analysis of APOE locus genetic variation implicates regional enhancers in the regulation of both TOMM40 and APOE. J Hum Genet 57: , 18–25. |
[30] | Schneider LS , Bennett DA , Farlow MR , Peskind ER , Raskind MA , Sano M , Stern Y , Haneline S , Welsh-Bohmer KA , O’Neil J , Walter R , Maresca S , Culp M , Alexander R , Saunders AM , Burns DK , Chiang C ((2022) ) Adjudicating mild cognitive impairment due to Alzheimer’s disease as a novel endpoint event in the TOMMORROW Prevention Clinical Trial. J Prev Alzheimers Dis 9: , 625–634. |
[31] | Swerdlow RH ((2012) ) Mitochondria and cell bioenergetics: Increasingly recognized components and a possible etiologic cause of Alzheimer’s disease. Antioxid Redox Signal 16: , 1434–1455. |
[32] | Swerdlow RH , Burns JM , Khan SM ((2014) ) The Alzheimer’s disease mitochondrial cascade hypothesis: Progress and perspectives. Biochim Biophys Acta 1842: , 1219–1231. |