Increased Cerebrospinal Fluid Levels of Soluble Triggering Receptor Expressed on Myeloid Cells 2 and Chitinase-3-Like Protein 1 in Idiopathic Normal-Pressure Hydrocephalus
Abstract
Background:
Neurodegenerative disease pathology is associated with neuroinflammation, but evidence on idiopathic normal pressure hydrocephalus (iNPH) remains limited and cerebrospinal fluid (CSF) biomarker profiles need to be elucidated.
Objective:
To investigate whether iNPH pathological mechanisms are associated with greater CSF markers of core Alzheimer’s disease pathology (amyloid-β42 (Aβ42), phosphorylated tau (P-tau)), neurodegeneration (total tau (T-tau)), and neuroinflammation (soluble triggering receptor expressed on myeloid cells 2 (sTREM2), chitinase-3-like protein 1 (YKL-40)).
Methods:
The study analyzed lumbar CSF samples from 63 patients with iNPH and 20 age-matched orthopedic surgery patients who had no preoperative gait or cognitive impairment (control group). Aβ42, T-tau, P-tau, sTREM2, and YKL-40 in different subgroups were investigated.
Results:
CSF sTREM2 levels were significantly higher in the iNPH group than in the control group, but no significant between-group difference was noted in YKL-40. Moreover, YKL-40 levels were significantly higher in the tap test non-responders than in the tap test responders (p = 0.021). At the 1-year follow-up after shunt surgery, the CSF P-tau levels were significantly lower (p = 0.020) in those with gait improvement and the CSF sTREM2 levels were significantly lower (p = 0.041) in those with cognitive improvement. In subgroup analysis, CSF sTREM2 levels were strongly correlated with CSF YKL-40 in the iNPH group (r = 0.443, p < 0.001), especially in the tap test non-responders (r = 0.653, p = 0.002).
Conclusion:
YKL-40 and sTREM2 are disease-specific markers of neuroinflammation, showing higher CSF levels in iNPH. In addition, sTREM2 is positively associated with YKL-40, indicating that interactions of glial cells play an important role in iNPH pathogenesis.
INTRODUCTION
Idiopathic normal pressure hydrocephalus (iNPH) is a treatable dementia in adults that is characterized as a surgically reversible neurological disorder with symptoms including but not limited to dementia, gait disturbance, and urinary incontinence [1]. Normal pressure hydrocephalus includes both idiopathic and secondary forms and iNPH is considered in the absence of secondary factors such as head trauma, intracranial infection, or subarachnoid hemorrhage. Recent research suggests that iNPH is a complication of neurodegenerative diseases that might constitute a vicious, deregulating loop. Potential etiologic factors and pathophysiological changes eventually result in the clinical symptoms of iNPH [2]. iNPH has long been conceptualized as a form of “treatable dementia.” The surgical insertion of a shunt to bypass the normal cerebrospinal fluid (CSF) circulation improves the symptoms in most cases, but improvements in these symptoms have been variable and unsatisfactory [3]. Shunts used mainly include ventriculoperitoneal and lumboperitoneal shunts, and these can improve symptoms in approximately 60– 93.3% of patients [4, 5]. Pathophysiological factors cause white matter and gray matter lesions, such as hypoperfusion, glymphatic system injury, metabolic disorders, neuroinflammation, astrogliosis, and blood-brain barrier disruption, ultimately leading to various iNPH symptoms [2].
Neuroinflammatory responses characterized by the release of inflammatory mediators such as cytokines and chemokines (e.g., tumor necrosis factor (TNF)-α, tumor growth factor-β1, monocyte chemoattractant protein-1, interleukin (IL)-8, and IL-6) are significantly increased in iNPH [6–8]. Neuroinflammation has been suggested to be involved in the pathogenesis of iNPH, and it may occur independently or in conjunction with other pathological processes. Neuroinflammation, a defense mechanism, initially protects the brain by clearing or inhibiting various pathogens. However, persistent inflammation is harmful and can induce the onset of neurodegenerative diseases that involves the activation of microglia and astrocytes [9]. As key regulators of the inflammatory response in the central nervous system (CNS), microglia and astrocytes were traditionally classified as neurotoxic (M1 microglia and A1 astrocytes) or neuroprotective (M2 microglia and A2 astrocytes) and their activation was heterogeneous [10]. Triggering receptor expressed on myeloid cells 2 (TREM2) is a natural immune receptor selectively expressed by microglia in the CNS involved in cell survival promotion, phagocytosis regulation, and inflammatory signaling inhibition [11]. The extracellular structure of TREM2 is cleaved on the cell surface, releasing soluble triggering receptor expressed on myeloid cells 2 (sTREM2) that can be measured in the CSF [12, 13].
Chitinase-3-like protein 1 (YKL-40) is abundantly expressed in activated astrocytes and plays key roles in inflammation, proliferation, angiogenesis, and tissue remodeling in neuroinflammatory conditions. Cytokines released from macrophages can induce YKL-40 transcription, resulting in morphological changes and altered mobility of astrocytic cells [14, 15]. sTREM2 [16–19] and YKL-40 [20–22] in CSF are significantly increased with various degenerative diseases. It has also been shown that increased microglia-derived sTREM2 is associated with glia-mediated immune responses, with a positive correlation with the astrocyte CSF marker YKL-40 [23]. Previous studies have found that neuroinflammation with microglial activation and astrogliosis also plays a role in neurodegenerative disease and dementia [24]. The glymphatic system has recently provided a new direction for the study of the mechanisms underlying iNPH. Subarachnoid CSF enters the cerebral stroma through arterial pulsation along the periarterial space and through the aquaporin 4 transporter in the terminal of astrocytes. The CSF mixes the interstitial fluid and moves through the interstitial space to the space around the veins and nerves, through which it is removed from the brain [25]. A biopsy study found glial cell proliferation in the iNPH cortex [26], especially astrocytes [27, 28].
The purpose of this study was to explore the role of microglia and astrocyte in the pathogenesis of iNPH. To achieve this goal, we investigated the CSF levels of sTREM2, YKL-40, and Alzheimer’s disease (AD) biomarkers (amyloid-β42 (Aβ42), phosphorylated tau (P-tau), and total tau (T-tau)) in iNPH and examined the correlation between different CSF biomarkers.
MATERIALS AND METHODS
Study design and patients
This study was approved by our institutional review board and was conducted according to the tenets of the Declaration of Helsinki. Written informed consent was obtained from all participants and their caregivers.
This study recruited 63 iNPH patients at the Department of Neurology of Huadong Hospital Affiliated to Fudan University between January 2020 and October 2021. iNPH was diagnosed according to the “Guidelines for Management of Idiopathic Normal Pressure Hydrocephalus (Third Edition)” criteria [29]. The inclusion criteria were: age ≥60 years, presence of typical clinical symptoms of the triad for more than 6 mouths, Evans’ index of >0.3, and normal CSF opening pressure (≤20 cm H2O). Exclusion criteria included: factors associated with secondary hydrocephalus, such as head trauma, intracerebral hemorrhage, meningitis, or any other known cause of secondary hydrocephalus.
Concurrently, 20 age-matched orthopedic surgery patients due to hip or knee arthroplasty were selected as control group. They were defined as subjects with Mini-Mental State Examination (MMSE) score ≥26 and subjected to brain MRI and neurological examination. Patients with clinical and radiological signs of NPH or other neurodegenerative diseases were not included. CSF samples were obtained during lumbar anesthesia before orthopedic surgery. The patient selection flowchart is shown in Fig. 1.
Fig. 1
Patient selection flowchart. Sixty-three patients with suspected idiopathic normal pressure hydrocephalus (iNPH) and twenty control patients who underwent orthopedic surgery were included in the study.
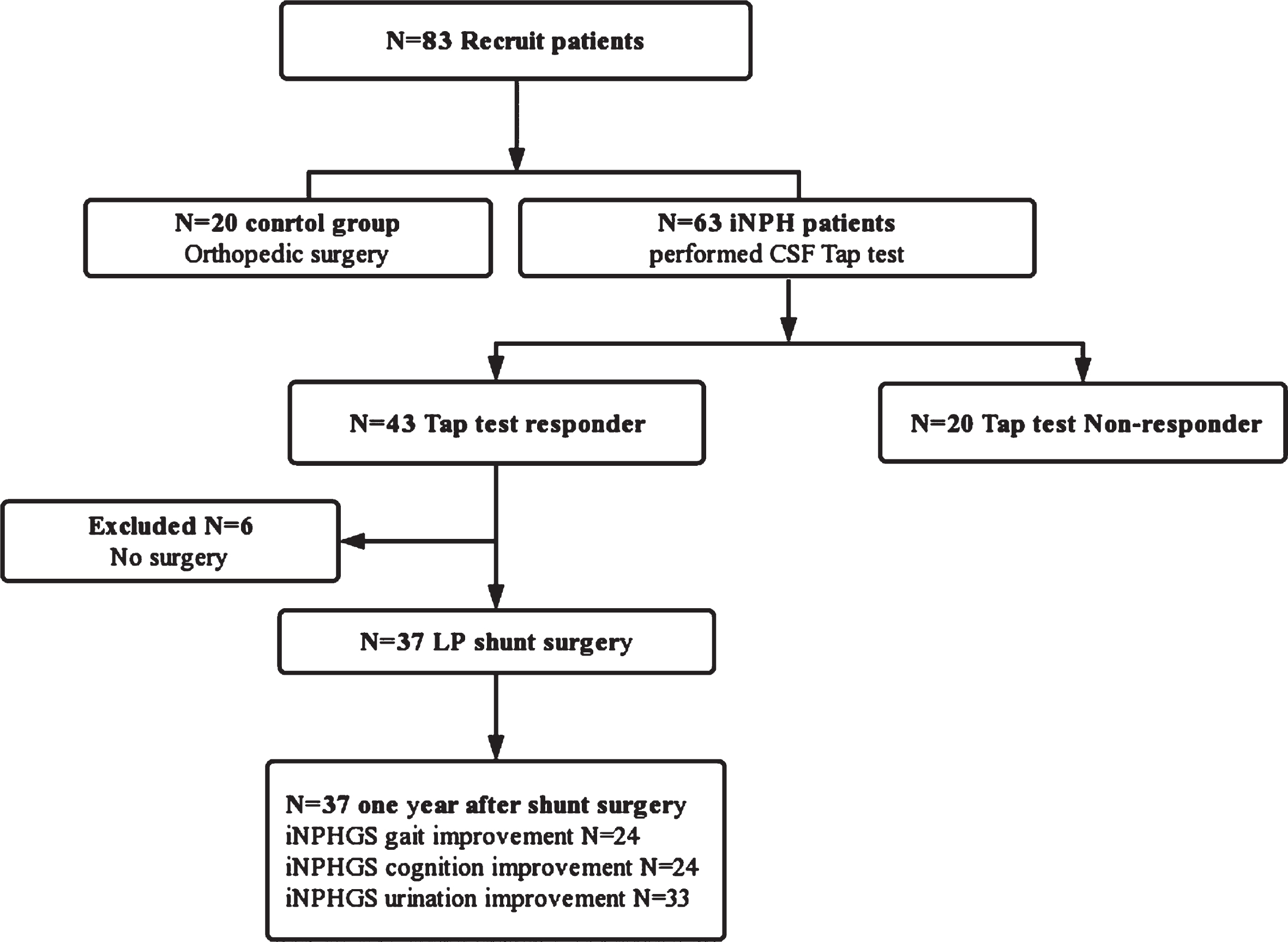
All iNPH patients underwent the tap test, and 43/63 patients were test responders. Among these test responders, 37 patients underwent shunt surgery, and 24 had gait improvement and 24 had cognition improvement at the 1-year follow-up. There were 33 surgical patients who had urine symptom improvement, but they could not be included in the analysis because the non-responder sample (n = 4) after shunting was small.
Clinical symptom assessment, tap test, and effect evaluation of shunt surgery
All patients clinically suspected of iNPH underwent rigorous clinical evaluation. Preoperatively, symptomatic status was evaluated by assessing the iNPH grading scale (iNPHGS) score; gait disorder was evaluated by using the 3 m Timed Up and Go test (3 m-TUG) and the timed 10 m walking test; and cognitive impairment was evaluated using the Mini-Mental State Examination (MMSE). The iNPHGS [30] is a clinical scale used to assess symptom severity (cognitive impairment, gait disturbance, and urination disturbance) in iNPH after unstructured interviews. Symptomatic status was measured again 1 year after shunt surgery using the iNPHGS.
A positive tap test was defined as the finding of any of the following within 1 week after CSF removal: A) symptomatic improvements: defined as an improvement of ≥1 grade on the iNPHGS in any domain of gait, cognition, urination, or its total; B) a decrease of >10% on the 3 m-TUG; or C) an increase of ≥3 points on the MMSE. Test responders underwent a lumboperitoneal shunt, and functional outcomes were followed up using the iNPHGS 1 year after shunting. Positive shunt surgery was defined as an improvement of ≥1 grade on the iNPHGS in any domain after one year following surgery. The patients were then divided into two groups according to the surgical outcomes: patients with improvement ≥1 in iNPHGS gait or cognition score were classified as the shunt responder group, while patients with no change or deterioration in iNPHGS gait or cognition score were classified as the no-response group.
Cerebrospinal fluid collection and examination
To identify patient response to CSF drainage, preoperative CSF was collected during diagnostic lumbar puncture. Lumbar puncture was performed in a lateral decubitus position between the intervertebral space L3–4/L4–5. CSF aliquots were stored as 1 ml samples in polypropylene tubes and frozen in liquid nitrogen at –80°C until analysis. In a validated laboratory, CSF levels of Aβ42, T-tau, P-tau, sTREM2, and YKL-40 were measured to minimize the variability in CSF assay results. According to the manufacturer’s protocol, the CSF samples were measured the concentrations of T-tau (Human T-TAU ELISA Kit, BIM Biosciences, Inc.), 181p-tau (Human p-Tau 181 ELISA Kit, BIM Biosciences, Inc.), and Aβ-42 (hA beta (aal-42) Oki (1 Kit), R&D Systems®, MN, USA), sTREM2 (Human TREM2 ELISA Kit, Abcam, Cambridge, England) and YKL-40 (Human YKL-40 ELISA Kit, Abcam, Cambridge, England) for enzyme-linked immunosorbent assays (ELISAs).
Statistical analysis
Measurement data with normal distribution were represented as mean±standard deviation. Independent sample t-tests were used to compare the measurement data with normal distributions, and χ2 tests were used to compare the conditional count data. Spearman or Pearson correlation analysis were used for correlation analysis. Statistical analyses were performed using SPSS software version 25.0. Statistical plots were drawn using GraphPad version 8.0. A p-value of 0.05 was considered statistically significant.
RESULTS
Patient characteristics
The iNPH group had a mean age of 74.8 (±8.7) years, and 22 and 41 of the patients were female and male, respectively. The mean time from symptom onset to hospital presentation was 25 months. The clinical data of the iNPH group are summarized in Table 1. All iNPH patients completed assessments before and after the tap test, including the MMSE, iNPHGS grading scale, 3 m-TUG, and the timed 10-m walking test. Overall, 43 and 20 patients were classified as tap test responders and non-responders, respectively. The patient characteristics according to the tap test response are summarized in Table 2. There were 37/43 responders who received lumboperitoneal shunts. At the 1-year postoperative follow-up, 24 had gait score improvement and 24 had cognition score improvement. Since 33/37 surgical patients had a urine improvement score of >1 point, they could not be included in this analysis because the non-response sample (n = 4) after shunting was small. The clinical data of these patients are summarized inTable 3.
Table 1
Preoperative clinicodemographic characteristics in the control and iNPH groups
Control group | iNPH group | p | |
Male patient | 9 (45.0%) | 41 (65.1%) | 0.110 |
Hypertension | 13 (65.0%) | 35 (55.6%) | 0.456 |
Diabetes mellitus | 7 (35.0%) | 22 (34.9%) | 0.139 |
Age, mean (SD), y | 71.4±6.3 | 74.8±8.7 | 0.107 |
CSF Aβ42 (pg/mL) | 461.29±122.82 | 331.00±194.36 | 0.001* |
CSF P-tau (pg/mL) | 57.52±10.34 | 48.41±12.52 | 0.002* |
CSF T-tau (pg/mL) | 193.35±17.13 | 150.37±29.81 | <0.001** |
CSF sTREM2 (pg/mL) | 13695.79±3867.07 | 21331.07±9489.94 | <0.001** |
CSF YKL-40 (pg/mL) | 127940.85±27162.45 | 126057.16±46813.79 | 0.825 |
*p < 0.05, **p < 0.001. iNPH, idiopathic normal pressure hydrocephalus; Aβ42, amyloid beta 42; P-tau, phosphorylated Tau; T-tau, total Tau; sTREM2, soluble triggering receptor expressed on myeloid cells 2; YKL-40, chitinase-3-like 1 protein.
Table 2
Preoperative clinicodemographic characteristics in the tap test responders and non-responders
Tap test Responder | Tap test Non-Responder | p | |
Male patient | 28 (65.1%) | 13 (65.0%) | 0.993 |
Age, mean (SD), y | 75.01 (±9.21) | 74.21 (±7.80) | 0.725 |
Hypertension | 28 (65.1%) | 8 (40%) | 0.061 |
Diabetes mellitus | 10 (23.3%) | 4(20%) | 0.772 |
CSF pressure | 132.75 (±39.66) | 126.94 (±30.06) | 0.725 |
Duration of symptoms, mean (SD), mo | 25.11 (±20.36) | 26.80 (±19.97) | 0.764 |
Evan’s ratio, mean (SD) | 0.349 (±0.038) | 0.360 (±0.046) | 0.649 |
iNPHGS grading scale | 7.48 (±2.26) | 6.30 (±3.33) | 0.116 |
iNPHGS Gait | 2.49 (±0.65) | 2.36 (±1.16) | 0.627 |
iNPHGS Cognition | 2.57 (±0.76) | 2.36 (±1.01) | 0.413 |
iNPHGS Urination | 2.43 (±1.17) | 1.89 (±1.32) | 0.125 |
3-m Timed Up and Go test (3 m-TUG) (s) | 22.50 (±15.95) | 26.00 (±15.30) | 0.540 |
The timed 10 m walking test (s) | 19.38 (±15.54) | 25.40 (±17.66) | 0.301 |
MMSE | 17.76 (±8.24) | 12.43 (±9.13) | 0.113 |
CSF Aβ42 (pg/mL) | 332.17±200.32 | 328.95±185.89 | 0.952 |
CSF P-tau (pg/mL) | 44.97±12.34 | 55.80±9.46 | <0.001** |
CSF T-tau (pg/mL) | 179.86±49.39 | 223.21±37.87 | <0.001** |
CSF sTREM2 (pg/mL) | 21498.65±10413.14 | 20970.75±4785526 | 0.839 |
CSF YKL-40 (pg/mL) | 116840.23±47855.25 | 145873.55±38513.79 | 0.021* |
*p < 0.05, **p < 0.001. iNPH, idiopathic normal pressure hydrocephalus; iNPHGS, iNPH grading scale; CSF, cerebrospinal fluid; MMSE, Mini-Mental State Examination; Aβ42, amyloid-beta 42; P-tau, phosphorylated Tau; T-tau, total Tau; sTREM2, soluble triggering receptor expressed on myeloid cells 2; YKL-40, chitinase-3-like 1 protein.
Table 3
Correlation analyses of CSF biomarkers between different groups
Control group | iNPH group | Tap test Responder | Tap test Non-Responder | |
sTREM2 correlation | ||||
CSF Aβ42 | r = 0.634 | r = 0.283 | r = 0.261 | r = 0.373 |
p = 0.004* | p = 0.030 | p = 0.099 | p = 0.127 | |
CSF P-tau | r = 0.153 | r = –0.065 | r = –0.205 | r = 0.391 |
p = 0.530 | p = 0.621 | p = 0.200 | p = 0.098 | |
CSF T-tau | r = –0.009 | r = 0.018 | r = 0.053 | r = –0.080 |
p = 0.972 | p = 0.894 | p = 0.743 | p = 0.745 | |
CSF YKL-40 | r = 0.301 | r = 0.443 | r = 0.411 | r = 0.653 |
p = 0.210 | p < 0.001** | p = 0.008* | p = 0.002* | |
YKL-40 correlation | ||||
CSF Aβ42 | r = –0.332 | r = –0.062 | r = –0.088 | r = 0.062 |
p = 0.153 | p = 0.6443 | p = 0.588 | p = 0.807 | |
CSF P-tau | r = 0.082 | r = –0.082 | r = –0.177 | r = 0.276 |
p = 0.731 | p = 0.534 | p = 0.268 | p = 0.253 | |
CSF T-tau | r = –0.117 | r = 0.079 | r = 0.142 | r = –0.171 |
p = 0.623 | p = 0.547 | p = 0.376 | p = 0.485 | |
CSF sTREM2 | r = 0.301 | r = 0.443 | r = 0.411 | r = 0.653 |
p = 0.210 | p < 0.001*** | p = 0.008** | p = 0.002** |
*p < 0.05, **p < 0.001. iNPH, idiopathic normal pressure hydrocephalus; Aβ42, amyloid beta 42; P-tau, phosphorylated Tau; T-tau, total Tau; sTREM2, soluble triggering receptor expressed on myeloid cells 2; YKL-40, chitinase-3-like 1 protein.
Table 4
Preoperative clinicodemographic characteristics in the shunt responder and non-responder
iNPHGS Gait | p | iNPHGS cognition | p | |||
Shunt Responder (24) | Shunt Non-Responder (13) | Shunt Responder (24) | Shunt Non-Responder (13) | |||
Male patient | 19 (79.2%) | 6 (46.2%) | 0.054 | 17 (70.8%) | 8 (61.5%) | 0.564 |
Age, mean (SD), y | 75.04 (±8.03) | 74.62 (±11.68) | 0.468 | 71.79 (±9.37) | 80.15 (±6.85) | 0.008* |
Hypertension | 17 (70.8%) | 8 (61.6%) | 0.564 | 15 (62.5%) | 9 (69.2%) | 0.734 |
Diabetes mellitus | 3 (12.5%) | 5 (38.5%) | 0.067 | 3 (12.5%) | 3 (32.3%) | 0.643 |
CSF pressure | 131.14 (±36.61) | 133.08 (±50.52) | 0.896 | 144.35 (±42.68) | 107.92 (±27.51) | 0.004* |
Duration of symptoms, mean (SD), mo | 27.58 (±23.57) | 20.54 (±12.02) | 0.322 | 26.00 (±23.83) | 23.46 (±12.31) | 0.723 |
iNPHGS grading scale | 7.58 (±2.30) | 7.31 (±2.25) | 0.728 | 7.50 (±2.54) | 7.46 (±2.54) | 0.961 |
iNPHGS Gait | 2.63 (±0.57) | 2.23 (±0.73) | 0.078 | 2.42 (±0.72) | 2.62 (±0.51) | 0.383 |
iNPHGS Cognition | 2.58 (±0.83) | 2.54 (±0.66) | 0.868 | 2.67 (±0.87) | 2.38 (±0.51) | 0.291 |
iNPHGS Urination | 2.38 (±1.21) | 2.54 (±1.13) | 0.690 | 2.42 (±1.28) | 2.46 (±0.97) | 0.913 |
Evan’s ratio, mean (SD) | 0.349 (±0.036) | 0.344 (±0.033) | 0.663 | 0.357 (±0.038) | 0.329 (±0.018) | 0.004 |
iNPHGS grading scale | 3.79 (±1.82) | 6.23 (±3.06) | 0.018 | 3.75 (±1.92) | 6.31 (±2.87) | 0.003 |
iNPHGS Gait | 1.54 (±0.59) | 2.31 (±0.86) | 0.003 | 1.58 (±0.65) | 2.23 (±0.83) | 0.013 |
iNPHGS Cognition | 1.71 (±0.81) | 2.23 (±0.93) | 0.083 | 1.54 (±0.78) | 2.54 (±0.66) | <0.001 |
iNPHGS Urination | 0.54 (±0.72) | 1.69 (±1.49) | 0.019 | 0.63 (±0.77) | 1.54 (±1.56) | 0.066 |
CSF Aβ42 (pg/mL) | 270.99±113.11 | 304.67±188.29 | 0.612 | 288.16±140.183 | 194.64±89.15 | 0.041* |
CSF P-tau (pg/mL) | 45.37±12.79 | 55.71±11.28 | 0.020* | 47.39±13.37 | 51.96±12.58 | 0.318 |
CSF T-tau (pg/mL) | 152.36±32.42 | 145.55±24.96 | 0.515 | 156.63±30.28 | 137.67±25.69 | 0.064 |
CSF sTREM2 (pg/mL) | 17047.69±6262.70 | 19035.33±6437.16 | 0.409 | 17263.13±5649.31 | 27249.96±11852.13 | 0.021* |
CSF YKL-40 (pg/mL) | 108565.23±38369.20 | 106999.73±38799.22 | 0.912 | 109802.69±39478.85 | 96979.77±252998.62 | 0.281 |
*p < 0.05, **p < 0.001. iNPH, idiopathic normal pressure hydrocephalus; iNPHGS, iNPH grading scale; CSF, cerebrospinal fluid; MMSE, Mini-Mental State Examination. Aβ42, amyloid-beta 42; P-tau, phosphorylated Tau; T-tau, total Tau; sTREM2, soluble triggering receptor expressed on myeloid cells 2; YKL-40, chitinase-3-like 1 protein.
Difference in CSF biomarkers between the control and iNPH groups and between tap test responders and non-responders
Age, sex, hypertension, and diabetes mellitus were not significantly different between the control and iNPH groups (Table 1). However, there were significant between-group differences in the CSF levels of Aβ42 (p = 0.001), P-tau (p = 0.002), T-tau (p < 0.001), and sTREM2 (p < 0.001; Fig. 2Aa-d), but not in that of YKL-40 (p = 0.864; Fig. 2e). The demographic data, clinical characteristics, and CSF opening pressures during the tap test for the responders and non-responders are presented in Table 2. Figure 2B shows the difference in CSF protein levels between tap test responders and non-responders. There was a significant difference in CSF levels of P-tau (p < 0.001; Fig. 2Bb), T-tau (p < 0.001; Fig. 2Bc), and YKL-40 (p = 0.021; Fig. 2Be).
Fig. 2
Between-group comparisons of CSF biomarker levels: Aβ42 (a), P-tau (b), T-tau (c), sTREM2 (d), and YKL-40 (e). A) Control group versus iNPH group: Aβ42 (p = 0.001; Aa); P-tau (p = 0.002; Ab); T-tau (p < 0.001; Ac); sTREM2 (p < 0.001; Ad); and YKL-40 (p = 0.825; Ae). B) Tap test responders (+) versus non-responders (–): Aβ42 (p = 0.952; Ba); P-tau (p < 0.001; Bb); T-tau (p < 0.001; Bc); sTREM2 (p = 0.839; Bd); and YKL-40 (p = 0.040; Be). *p < 0.05, **p < 0.001.
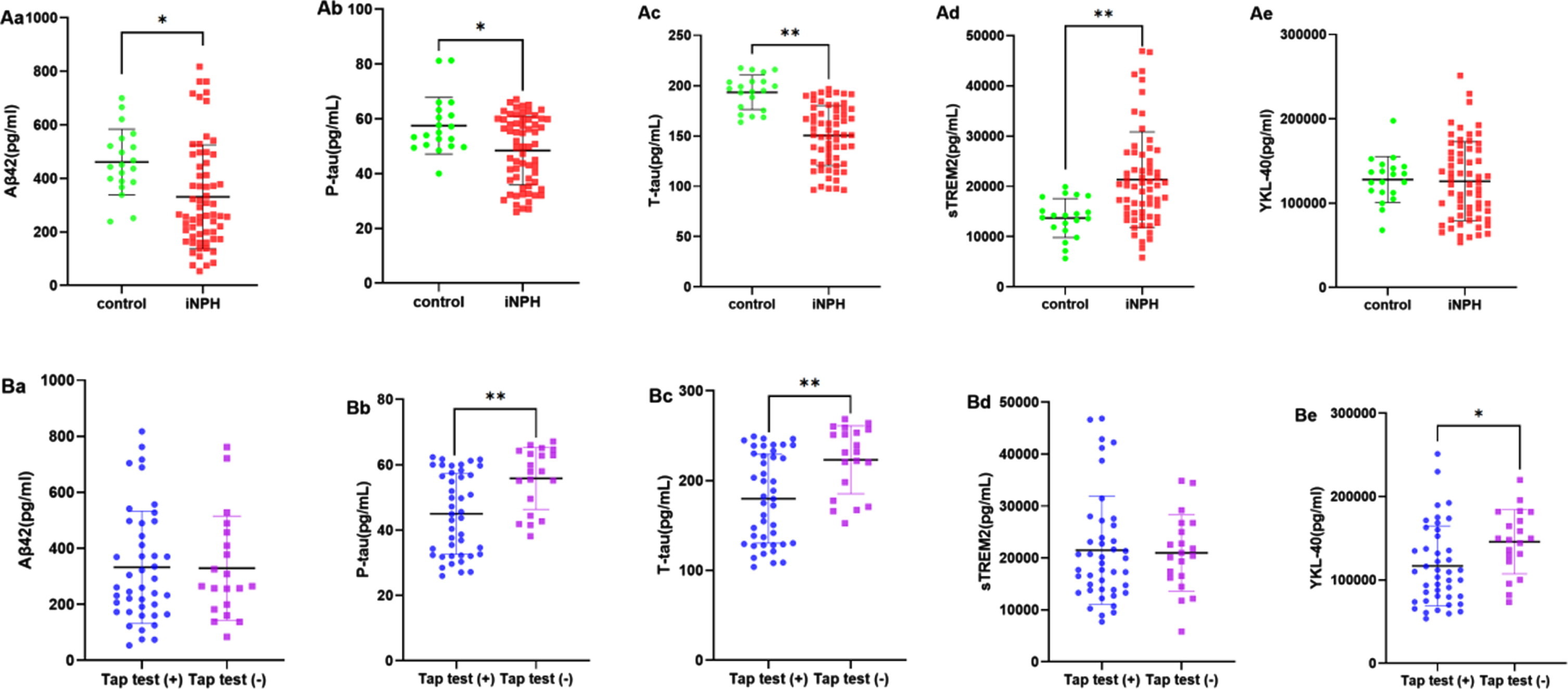
Correlation between different CSF biomarkers in iNPH
CSF sTREM2 levels were significantly correlated with CSF Aβ42 levels in the control group (r = 0.634, p = 0.004; Fig. 3A) but not in the iNPH group (r = 0.283, p = 0.030; Fig. 3B). To study the effect of astrocyte and microglia activation on the pathogenesis of iNPH and explore the possible mechanism, we explored the correlation between CSF levels of sTREM2 and YKL-40 in different groups. There was no correlation between the CSF YKL-40 and CSF sTREM2 levels in the control group (r = 0.301, p = 0.210; Fig. 3C), but a correlation was found in the iNPH group (r = 0.443, p < 0.001; Fig. 3D). Further, there was a stronger correlation between CSF sTREM2 levels and CSF YKL-40 levels in the tap test non-responder group (r = 0.653, p = 0.002; Fig. 3F) than in the tap test responder group (r = 0.411, p = 0.008; Fig. 3E).
Fig. 3
Correlation analysis among CSF biomarkers. A, B) sTREM2 and Aβ42. C-F sTREM2 and YKL-40. CSF sTREM2 levels (A) are correlated with CSF Aβ42 levels in the control group (r = 0.634, p = 0.004), (B) but not in the iNPH group (r = 0.283, p = 0.030). CSF YKL-40 levels are (C) not positively correlated with CSF sTREM2 levels in the control group but are (D) correlated in the iNPH group (r = 0.443, p < 0.001), (E) tap test responders (r = 0.411, p = 0.008), and (F) tap test non-responders (r = 0.653, p = 0.002).
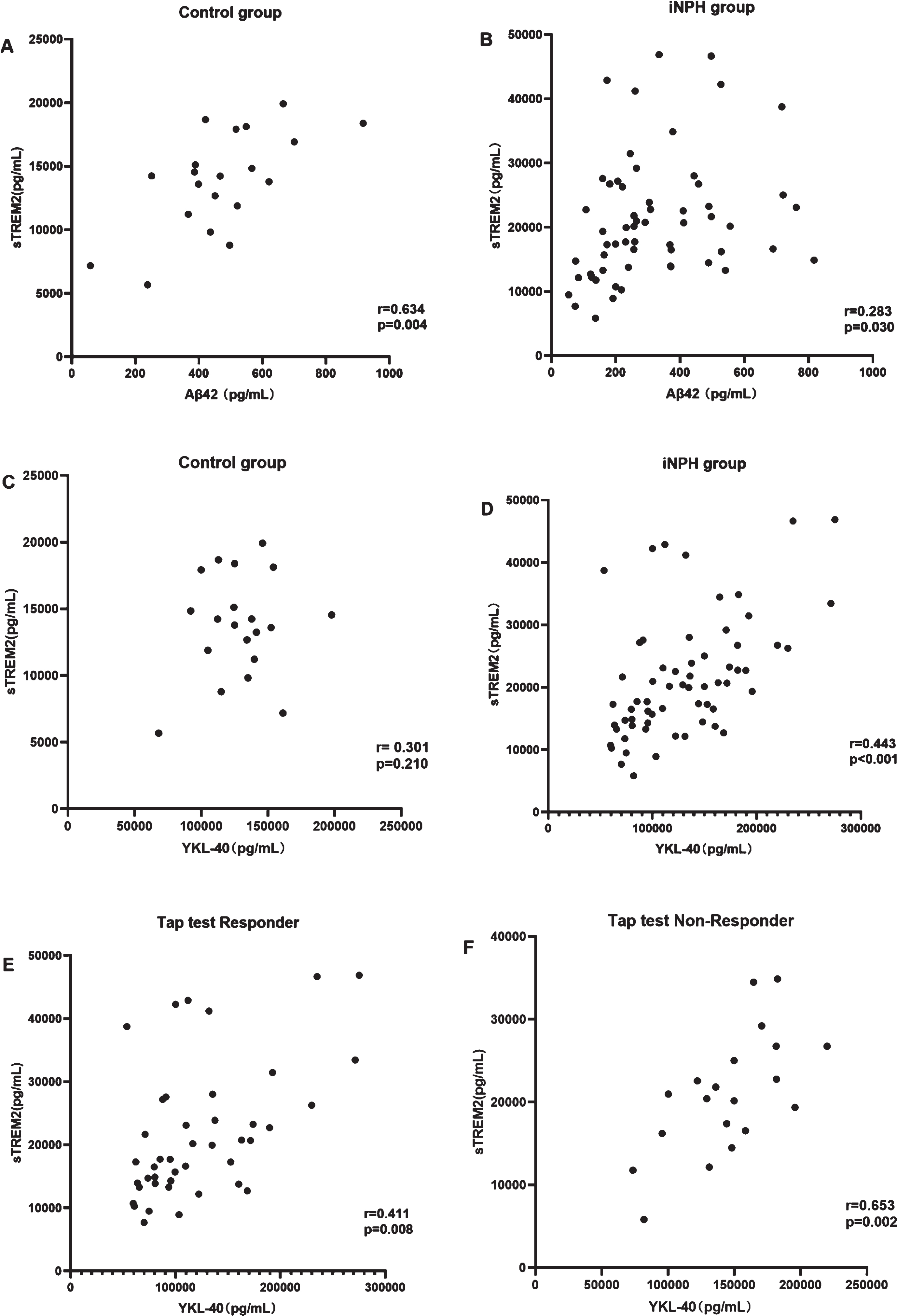
CSF biomarkers may play different roles in iNPH symptom improvement after shunt surgery
In the tap test responder group, 24 (64.9%) patients showed an improvement in the iNPHGS gait, 24 (64.9%) patients showed an improvement in iNPHGS cognition, 18 patients showed an improvement in the two domains, and 34 patients showed an improvement in iNPHGS urination. In the cognitive improvement subgroup, tap test non-responders were older than tap test responders (p = 0.008). There were no significant differences in other demographic characteristics (Table 3). In the iNPHGS gait improvement subgroup, there was a significant difference in CSF levels of P-tau (p = 0.020; Fig. 4Ab) but none in those of Aβ42 (p = 0.612), T-tau (p = 0.515), sTREM2 (p = 0.409), or YKL-40 (p = 0.912; Fig. 4Aa, c-e). Meanwhile, in the iNPHGS cognition improvement subgroup, there was a significant difference in CSF levels of Aβ42 (p = 0.041; Fig. 4Ba) and sTREM2 (p = 0.021; Fig. 4Bd) but none in those of P-tau (p = 0.318), T-tau (p = 0.064), or YKL-40 (p = 0.281; Fig. 4Bb-c, e).
Fig. 4
Comparisons of preoperative biomarker findings between shunt responders and non-responders. Aa) CSF P-tau levels are significantly different (p = 0.020) in the iNPHGS gait improvement subgroup. Ba) CSF levels of Aβ42 (p = 0.041) and (Bd) sTREM2 (p = 0.021) are significantly different in the iNPHGS cognition improvement subgroup. iNPHGS gait (+): improvement in gait after shunt surgery as assessed by iNPHGS. iNPHGS gait (–): No improvement in gait after shunt surgery as assessed by iNPHGS. iNPHGS cognition (+): improvement in cognition after shunt surgery as assessed by iNPHGS. iNPHGS cognition (–): no improvement in cognition after shunt surgery as assessed by iNPHGS. *p < 0.05, **p < 0.001.
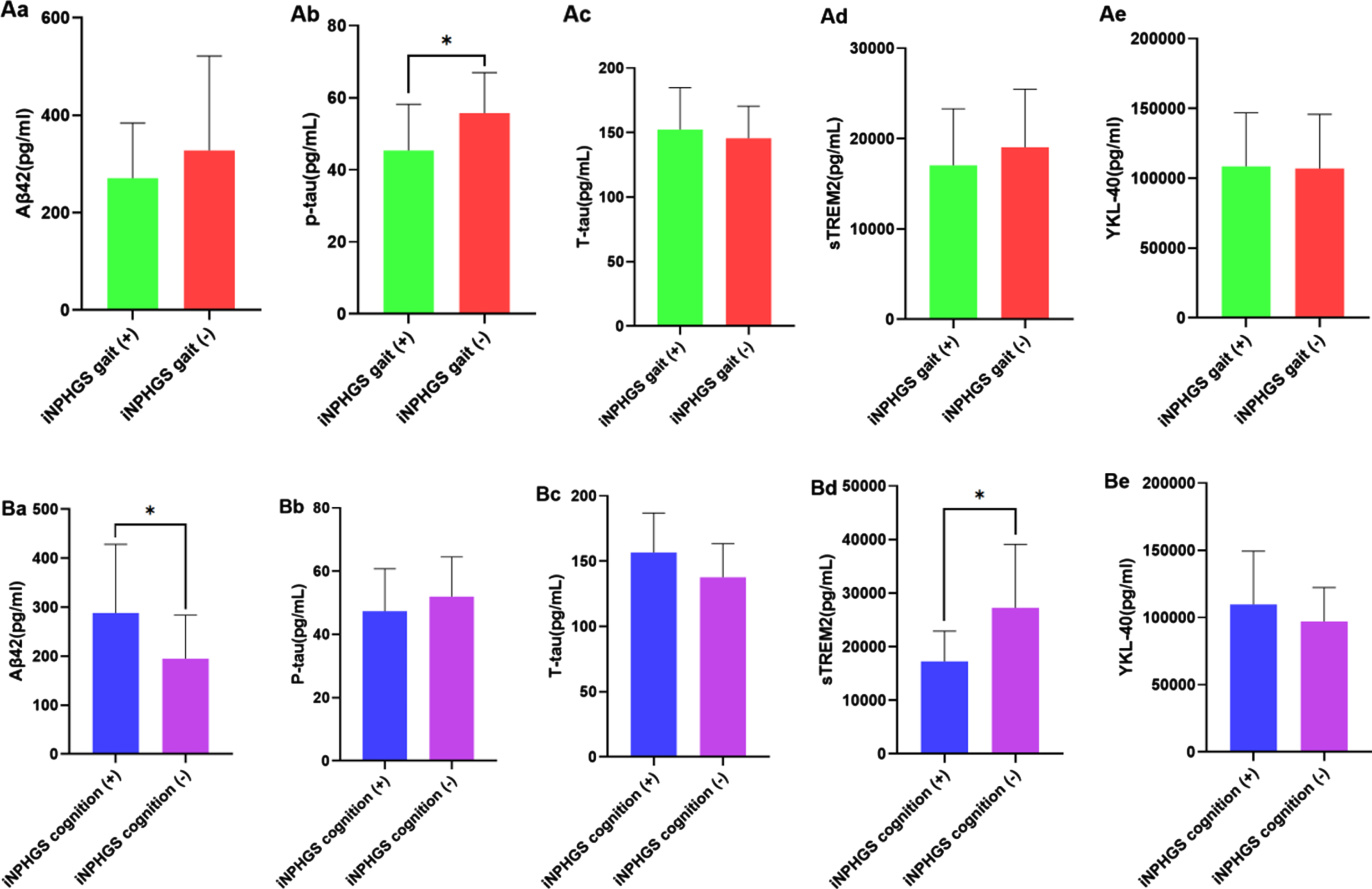
DISCUSSION
Evidence on CSF biomarker profiles in iNPH is sparse. This study found that CSF levels of Aβ42, P-tau, and T-tau were significantly lower while those of sTREM2 were higher in iNPH patients than in controls. In addition, CSF levels of P-tau, T-tau, and YKL-40 were significantly higher in tap test non-responders than in responders. Further, lower CSF P-tau levels were significantly correlated with improvement in gait dysfunction, whereas a higher CSF Aβ42 level and a lower CSF sTREM2 level were associated with cognitive improvement after shunt surgery. Finally, activation and interaction of microglia and astrocytes played an important role in the pathogenesis of iNPH.
The decrease in CSF Aβ42 level in iNPH may have a different mechanism from that in AD. Almost all APP-metabolites are suppressed in iNPH CSF, whereas Aβ42 is reduced but other Aβ-isoforms are not perturbed in AD [31]. Reduced iNPH CSF Aβ42 levels may be related to the smaller extracellular spaces during the sleep period [32], the association with perivascular lymphatic drainage pathways dysfunction [33], and hypometabolism in the periventricular zone and axonal degeneration [34]. There’s no denying that lower Aβ42 levels and high tau levels could also be the result of that coexistence with signs of AD pathophysiological changes occurred in not only iNPH but also normal cognitive individuals [35, 36].
Previous findings regarding CSF tau levels in iNPH have been inconsistent. CSF P-tau and T-tau levels are decreased in iNPH, which may be related to decreased extracellular fluid clearance and periventricular hypometabolism [37]. One study found higher ventricular CSF levels of T-tau and P-tau than lumbar CSF samples, implying an impairment in the circulation of CSF from the ventricle to the lumbar cistern in iNPH [31, 38].
The CSF sTREM2 level is an inflammatory marker of microglial activation, which is closely related to age. It remains unclear whether microglia activation has a protective or deleterious effect on the brain [39]. The aging-related increase in CSF sTREM2 levels may be in line with a protective response to rather mild neuroinjury [40]. A recent study showed that the levels of sTREM2 peaked at the initial stages of sporadic AD and was positively correlated with CSF T-tau and P-tau levels, suggesting that sTREM2 plays an important role in AD pathology and related to not only the clearance of Aβ plaques but also neurodegeneration [41]. The positive correlation between CSF Aβ42 levels and sTREM2 levels in dementia patients indicates that sTREM2 may not be associated with the extent of amyloid deposition [42], but instead participates in the Aβ precursor protein synthesis and metabolism [43]. In Aβ42-rich brain tissues, gliosis associated with vascular alterations is a common finding [26].
In the current study, CSF sTREM2 levels were significantly higher in iNPH than in age-matched aging controls, suggesting that microglial activation plays an important role in the pathophysiology of iNPH [44]. It is unclear whether microglia activation had a protective or deleterious effect on iNPH. However, we found a positive correlation between sTREM2 levels and Aβ42 in the age-matched control group, but no linear relationship between sTREM2 and Aβ42, P-tau, or T-tau in the iNPH group. This confirmed that iNPH may have a different mechanismfrom AD.
Silverberg suggests that the dementia in NPH is of multifactorial etiology and the lack of response may in part represent permanent tissue damage [45]. Astrocytes increase in reactivity after disease or brain damage and reactive astrocytes up-regulate many genes to form glial scars in the CNS [31, 46, 47]. AQP4 is the most comprehensive and specific astrocyte marker in the developing human brain, and YKL-40 positivity is usually found in reactive astroglia [48].
In the current study, the CSF YKL-40 levels in the tap test responders were lower than those in the non-responders and similar to those in the normal controls, possibly due to coincident reactive astrogliosis and associated depolarization of AQP4. Hua et al. found that CSF pressure was lower in tap test responders than in non-responders, suggesting better brain tissue compliance in patients who were tap test-positive [49]. Reactive proliferation of glial cells forms a glial scar in the brain tissue and causes a decrease in brain tissue compliance, and this may cause insensitivity to the tap test. Previous studies suggested that CSF YKL-40 levels in iNPH patients were similar to those in healthy controls, but this is possibly because tap test non-responders were not included in the analyses [50, 51].
The proinflammatory mediators IL-1β and TNF-α released from macrophages control YKL-40 expression in astrocytes by microglial stimuli from YKL-40 transcription, accompanied by morphological changes characteristic of reactive gliosis and increased migratory capacity [15]. Recent studies have shown that activated microglia induce neurotoxic reactive astrocyte activation by releasing cytokines, implying a cross-talk between astrocytes and microglia in neurodegenerative diseases and the complex connection of immune cells in the CNS [47]. In parallel, reactive changes of microglial cells and astrocyte morphology would possibly lead to an additional slowing of glymphatic flow [52]. The current study found that CSF sTREM2 and YKL-40 levels were closely correlated in iNPH, and the correlation was significantly higher in the tap test non-responders than in responders. This finding indicates that microglial activation may activate neurotoxic reactive astrocytes in the tap test non-responder group, causing decreased compliance in the brain tissue.
Recent studies found a significant correlation between clinical improvement and CSF removal. In fact, one study found that postoperative improvement was mainly manifested in motor symptoms, but there was no significant effect on cognitive impairment [53]. Another study showed that surgical treatment of iNPH was more successful in gait disturbance and urinary incontinence, but less effective in cognitive improvement [35]. We speculate that gait disorder and cognitive impairment in iNPH may have different pathogeneses. The higher the prevalence of AD pathology in cortical tissue, the higher the severity of dementia in NPH patients [54]. AD neuropathological findings are highly prevalent in patients receiving shunts for NPH, but the effect of shunts in iNPH patients with AD pathology is controversial. Studies have revealed poor outcomes in terms of cognitive improvement after shunt surgery in NPH patients with concomitant AD [55]. AD pathology on cortical brain biopsy predicts adverse outcomes after CSF shunting. Evidence of AD pathology was found on repeat biopsies in 13% of patients with initially normal cortical brain biopsy results, demonstrating that AD pathology could occur at different stages of NPH with disease progression [36]. In contrast, another study reported that patients with CSF constellations typical for AD showed significant improvement in cognitive and gait-related functions after CSF drainage [56].
Kazui et al. found that at 3 months after shunt, although almost all of the triad symptoms were decreased in iNPH patients with and without AD pathology, memory was only improved in iNPH patients without AD pathology [57]. In the current study, low CSF Aβ42 and high CSF sTREM2 levels had a strong correlation with poor cognitive improvement 1 year after shunting. We speculate that the activation of microglia may also play an important role in cognitive impairment of iNPH patients. However, several studies suggest a positive correlation between CSF sTREM2 levels and age [12, 58, 59]. In the current study, patients with poor cognitive improvement after shunt were older, suggesting that the higher CSF sTREM2 levels may be associated with advanced age. CSF tau levels reflect the function of nerve fibers and axons. Compared with T-tau, P-tau levels, especially 181P-tau levels, are less affected by changes in CSF dynamics and may reflect the pathological changes of the brain in iNPH more steadily [60]. CSF P-tau levels are significantly correlated with gait dysfunction in iNPH patients [35]. A survey showed low CSF P-tau levels was a useful prognostic biomarker for good clinical outcomes at least two years after shunting. CSF tau represents irreversible damage to nerve axons, and higher P-Tau and T-tau levels may represent pathological damage that has been occurring for a considerable time before clinical symptoms develop, with irreversible parenchymal damage already present in brain tissue, thus unresponsive to CSF removal and shunting [61].
Notably, this study focused on the role of neuroinflammation in the pathogenesis of iNPH. We included relatively normal control group and Tap test non-responders patients in the analysis cohort. It can provide a more comprehensive understanding of the biological mechanism of neuroinflammation in iNPH. Our study has some limitations. First, CSF protein levels were not measured after the intervention, and thus the effect of shunting on the concentration of these proteins was not determined. Second, other control groups, such as patients with AD or other neurodegenerative diseases, were not included. Third, concurrent AD as a comorbidity cannot be completely ruled out, and we cannot exclude the idea that some iNPH patients in our study could progress to develop classic AD.
Conclusion
CSF levels of YKL-40 and sTREM2 are disease-specific markers of neuroinflammation, showing higher levels in iNPH and being closely related to the response to the tap test and outcomes of shunt surgery. In addition, sTREM2 is positively associated with YKL-40, indicating that interactions of glial cells play an important role in the pathogenesis of iNPH and suggesting the role of astrocyte-microglia cross-talk during neurodegeneration.
ACKNOWLEDGMENTS
We appreciate all patients participating in this study. The authors would like to thank Editage (https://www.editage.cn) for English language editing.
FUNDING
This work was supported by Shanghai Sailing Program [Grant Number: 21YF1411600], Shanghai Municipal Key Clinical Specialty [Grant Number: shslczdzk02801], the projects of the National Natural Science Foundation of China (82201565) and Investigator Initiated Research Projects of Huadong Hospital [Grant Number: HDLC2022003].
CONFLICT OF INTEREST
The authors have no conflict of interest to report.
DATA AVAILABILITY STATEMENT
The data supporting the findings of this study are available on request from the corresponding author. The data are not publicly available due to privacy or ethical restrictions.
REFERENCES
[1] | Adams RD , Fisher CM , Hakim S , Ojemann RG , Sweet WH ((1965) ) Symptomatic occult hydrocephalus with “normal” cerebrospinal-fluid pressure. A treatable syndrome. N Engl J Med 273: , 117–126. |
[2] | Wang Z , Zhang Y , Hu F , Ding J , Wang X ((2020) ) Pathogenesis and pathophysiology of idiopathic normal pressure hydrocephalus. CNS Neurosci Ther 26: , 1230–1240. |
[3] | Nunn AC , Jones HE , Morosanu CO , Singleton W , Williams MA , Nagel SJ , Luciano MG , Zwimpfer TJ , Holubkov R , Wisoff JH , McKhann GM 2nd , Hamilton MG , Edwards RJ ((2021) ) Extended lumbar drainage in idiopathic normal pressure hydrocephalus: A systematic review and meta-analysis of diagnostic test accuracy. Br J Neurosurg 35: , 285–291. |
[4] | Reddy GK , Bollam P , Caldito G ((2014) ) Long-term outcomes of ventriculoperitoneal shunt surgery in patients with hydrocephalus. World Neurosurg 81: , 404–410. |
[5] | Grasso G , Torregrossa F , Leone L , Frisella A , Landi A ((2019) ) Long-term efficacy of shunt therapy in idiopathic normal pressure hydrocephalus. World Neurosurg 129: , e458–e463. |
[6] | Castaneyra-Ruiz L , Gonzalez-Marrero I , Carmona-Calero EM , Abreu-Gonzalez P , Lecuona M , Brage L , Rodríguez EM , Castañeyra-Perdomo A ((2016) ) Cerebrospinal fluid levels of tumor necrosis factor alpha and aquaporin 1 in patients with mild cognitive impairment and idiopathic normal pressure hydrocephalus. Clin Neurol Neurosurg 146: , 76–81. |
[7] | Tarkowski E , Tullberg M , Fredman P , Wikkelso C ((2003) ) Normal pressure hydrocephalus triggers intrathecal production of TNF-alpha. Neurobiol Aging 24: , 707–714. |
[8] | Czubowicz K , Glowacki M , Fersten E , Kozlowska E , Strosznajder RP , Czernicki Z ((2017) ) Levels of selected pro- and anti-inflammatory cytokines in cerebrospinal fluid in patients with hydrocephalus. Folia Neuropathol 55: , 301–307. |
[9] | Wyss-Coray T , Mucke L ((2002) ) Inflammation in neurodegenerative disease— a double-edged sword. Neuron 35: , 419–432. |
[10] | Kwon HS , Koh S ((2020) ) Neuroinflammation in neurodegenerative disorders: The roles of microglia and astrocytes. Transl Neurodegener 9: , 42. |
[11] | Yeh FL , Hansen DV , Sheng M ((2017) ) TREM2, microglia, and neurodegenerative diseases. Trends Mol Med 23: , 512–533. |
[12] | Henjum K , Almdahl IS , Årskog V , Minthon L , Hansson O , Fladby T , Nilsson LN ((2016) ) Cerebrospinal fluid soluble TREM2 in aging and Alzheimer’s disease. Alzheimers Res Ther 8: , 17. |
[13] | Ohara T , Hata J , Tanaka M , Honda T , Yamakage H , Yoshida D , Inoue T , Hirakawa Y , Kusakabe T , Shibata M , Teraoka T , Kitazono T , Kanba S , Satoh-Asahara N , Ninomiya T ((2019) ) Serum soluble triggering receptor expressed on myeloid cells 2 as a biomarker for incident dementia: The Hisayama Study. Ann Neurol 85: , 47–58. |
[14] | Rehli M , Niller H , Ammon C , Langmann S , Schwarzfischer L , Andreesen R , Krause SW ((2003) ) Transcriptional regulation of CHI3L1, a marker gene for late stages of macrophage differentiation. J Biol Chem 278: , 44058–44067. |
[15] | Bonneh-Barkay D , Bissel SJ , Kofler J , Starkey A , Wang G , Wiley CA ((2012) ) Astrocyte and macrophage regulation of YKL-40 expression and cellular response in neuroinflammation. Brain Pathol 22: , 530–546. |
[16] | Ulrich JD , Ulland TK , Colonna M , Holtzman DM ((2017) ) Elucidating the role of TREM2 in Alzheimer’s disease. Neuron 94: , 237–248. |
[17] | Piccio L , Buonsanti C , Cella M , Tassi I , Schmidt RE , Fenoglio C , Rinker J 2nd , Naismith RT , Panina-Bordignon P , Passini N , Galimberti D , Scarpini E , Colonna M , Cross AH ((2008) ) Identification of soluble TREM-2 in the cerebrospinal fluid and its association with multiple sclerosis and CNS inflammation. Brain 131: , 3081–3091. |
[18] | Ohrfelt A , Axelsson M , Malmestrom C , Novakova L , Heslegrave A , Blennow K , Lycke J , Zetterberg H ((2016) ) Soluble TREM-2 in cerebrospinal fluid from patients with multiple sclerosis treated with natalizumab or mitoxantrone. Mult Scler 22: , 1587–1595. |
[19] | Peng G , Qiu J , Liu H , Zhou M , Huang S , Guo W , Lin Y , Chen X , Li Z , Li G , Zhang W , Zhang Y , Li X , Wu Z , Wei L , Yang X , Zhu X , Mo M , Xu P ((2020) ) Analysis of cerebrospinal fluid soluble TREM2 and polymorphisms in sporadic Parkinson’s disease in a Chinese population. J Mol Neurosci 70: , 294–301. |
[20] | Baldacci F , Lista S , Palermo G , Giorgi FS , Vergallo A , Hampel H ((2019) ) The neuroinflammatory biomarker YKL-40 for neurodegenerative diseases: Advances in development. Expert Rev Proteomics 16: , 593–600. |
[21] | Llorens F , Thune K , Tahir W , Kanata E , Diaz-Lucena D , Xanthopoulos K , Kovatsi E , Pleschka C , Garcia-Esparcia P , Schmitz M , Ozbay D , Correia S , Correia  , Milosevic I , Andréoletti O , Fernández-Borges N , Vorberg IM , Glatzel M , Sklaviadis T , Torres JM , Krasemann S , Sánchez-Valle R , Ferrer I , Zerr I ((2017) ) YKL-40 in the brain and cerebrospinal fluid of neurodegenerative dementias. Mol Neurodegener 12: , 83. |
[22] | Alcolea D , Vilaplana E , Suárez-Calvet M , Illán-Gala I , Blesa R , Clarimón J , Lladó A , Sánchez-Valle R , Molinuevo JL , García-Ribas G , Compta Y , Martí MJ , Piñol-Ripoll G , Amer-Ferrer G , Noguera A , García-Martín A , Fortea J , Lleó A ((2017) ) CSF sAPPbeta, YKL-40, and neurofilament light in frontotemporal lobar degeneration. Neurology 89: , 178–188. |
[23] | Heslegrave A , Heywood W , Paterson R , Magdalinou N , Svensson J , Johansson P , Öhrfelt A , Blennow K , Hardy J , Schott J , Mills K , Zetterberg H ((2016) ) Increased cerebrospinal fluid soluble TREM2 concentration in Alzheimer’s disease. Mol Neurodegener 11: , 3. |
[24] | Amor S , Peferoen LA , Vogel DY , Breur M , van der Valk P , Baker D , van Noort JM ((2014) ) Inflammation in neurodegenerative diseases–an update. Immunology 142: , 151–166. |
[25] | Tan C , Wang X , Wang Y , Wang C , Tang Z , Zhang Z , Liu J , Xiao G ((2021) ) The pathogenesis based on the glymphatic system, diagnosis, and treatment of idiopathic normal pressure hydrocephalus. Clin Interv Aging 16: , 139–153. |
[26] | Abu HS , Virhammar J , Sehlin D , Alafuzoff I , Cesarini KG , Marklund N ((2018) ) Brain tissue Abeta42 levels are linked to shunt response in idiopathic normal pressure hydrocephalus. J Neurosurg 130: , 121–129. |
[27] | Zhou B , Zuo YX , Jiang RT ((2019) ) Astrocyte morphology: Diversity, plasticity, and role in neurological diseases. CNS Neurosci Ther 25: , 665–673. |
[28] | Eide PK , Hansson HA ((2018) ) Astrogliosis and impaired aquaporin-4 and dystrophin systems in idiopathic normal pressure hydrocephalus. Neuropathol Appl Neurobiol 44: , 474–490. |
[29] | Nakajima M , Yamada S , Miyajima M , Ishii K , Kuriyama N , Kazui H , Kanemoto H , Suehiro T , Yoshiyama K , Kameda M , Kajimoto Y , Mase M , Murai H , Kita D , Kimura T , Samejima N , Tokuda T , Kaijima M , Akiba C , Kawamura K , Atsuchi M , Hirata Y , Matsumae M , Sasaki M , Yamashita F , Aoki S , Irie R , Miyake H , Kato T , Mori E , Ishikawa M , Date I , Arai H; research committee of idiopathic normal pressure hydrocephalus ((2021) ) Guidelines for management of idiopathic normal pressure hydrocephalus (Third Edition): Endorsed by the japanese society of normal pressure hydrocephalus. Neurol Med Chir (Tokyo) 61: , 63–97. |
[30] | Mori E , Ishikawa M , Kato T , Kazui H , Miyake H , Miyajima M , Nakajima M , Hashimoto M , Kuriyama N , Tokuda T , Ishii K , Kaijima M , Hirata Y , Saito M , Arai H; Japanese Society of Normal Pressure Hydrocephalus ((2012) ) Guidelines for management of idiopathic normal pressure hydrocephalus: Second edition. Neurol Med Chir (Tokyo) 52: , 775–809. |
[31] | Anderson MA , Burda JE , Ren Y , Ao Y , O’Shea TM , Kawaguchi R , Coppola G , Khakh BS , Deming TJ , Sofroniew MV ((2016) ) Astrocyte scar formation aids central nervous system axon regeneration. Nature 532: , 195–200. |
[32] | Reeves BC , Karimy JK , Kundishora AJ , Mestre H , Cerci HM , Matouk C , Alper SL , Lundgaard I , Nedergaard M , Kahle KT ((2020) ) Glymphatic system impairment in Alzheimer’s disease and idiopathic normal pressure hydrocephalus. Trends Mol Med 26: , 285–295. |
[33] | Hladky SB , Barrand MA ((2014) ) Mechanisms of fluid movement into, through and out of the brain: Evaluation of the evidence. Fluids Barriers CNS 11: , 26 . |
[34] | Jeppsson A , Zetterberg H , Blennow K , Wikkelso C ((2013) ) Idiopathic normal-pressure hydrocephalus: Pathophysiology and diagnosis by CSF biomarkers. Neurology 80: , 1385–1392. |
[35] | Cabral D , Beach TG , Vedders L , Sue LI , Jacobson S , Myers K , Sabbagh MN ((2011) ) Frequency of Alzheimer’s disease pathology at autopsy in patients with clinical normal pressure hydrocephalus. Alzheimers Dement 7: , 509–513. |
[36] | Pomeraniec IJ , Bond AE , Lopes MB , Jane JS ((2016) ) Concurrent Alzheimer’s pathology in patients with clinical normal pressure hydrocephalus: Correlation of high-volume lumbar puncture results, cortical brain biopsies, and outcomes. J Neurosurg 124: , 382–388. |
[37] | Gordon PH ((2013) ) Amyotrophic lateral sclerosis: An update for 2013 clinical features, pathophysiology, management and therapeutic trials. Aging Dis 4: , 295–310. |
[38] | Seppälä TT , Nerg O , Koivisto AM , Rummukainen J , Puli L , Zetterberg H , Pyykkö OT , Helisalmi S , Alafuzoff I , Hiltunen M , Jääskeläinen JE , Rinne J , Soininen H , Leinonen V , Herukka SK ((2012) ) CSF biomarkers for Alzheimer disease correlate with cortical brain biopsy findings. Neurology 78: , 1568–1575. |
[39] | Konishi H , Kiyama H ((2018) ) Microglial TREM2/DAP12 signaling: A double-edged sword in neural diseases. Front Cell Neurosci 12: , 206. |
[40] | Suárez-Calvet M , Kleinberger G , Araque Caballero MÁ , Brendel M , Rominger A , Alcolea D , Fortea J , Lleó A , Blesa R , Gispert JD , Sánchez-Valle R , Antonell A , Rami L , Molinuevo JL , Brosseron F , Traschütz A , Heneka MT , Struyfs H , Engelborghs S , Sleegers K , Van Broeckhoven C , Zetterberg H , Nellgård B , Blennow K , Crispin A , Ewers M , Haass C ((2016) ) sTREM2 cerebrospinal fluid levels are a potential biomarker for microglia activity in early-stage Alzheimer’s disease and associate with neuronal injury markers. EMBO Mol Med 8: , 466–476. |
[41] | Singh AK , Mishra G , Maurya A , Awasthi R , Kumari K , Thakur A , Rai A , Rai GK , Sharma B , Kulkarni GT , Singh SK ((2019) ) Role of TREM2 in Alzheimer’s disease and its consequences on beta- amyloid, tau and neurofibrillary tangles. Curr Alzheimer Res 16: , 1216–1229. |
[42] | Henjum K , Quist-Paulsen E , Zetterberg H , Blennow K , Nilsson LNG , Watne LO ((2018) ) CSF sTREM2 in delirium— relation to Alzheimer’s disease CSF biomarkers Aβ42, t-tau and p-tau. J Neuroinflamm 15: , 304. |
[43] | Iwatsubo T , Mann DM , Odaka A , Suzuki N , Ihara Y ((1995) ) Amyloid beta protein (A beta) deposition: A beta 42(43) precedes A beta 40 in Down syndrome. Ann Neurol 37: , 294–249. |
[44] | Hickman S , Izzy S , Sen P , Morsett L , El KJ ((2018) ) Microglia in neurodegeneration. Nat Neurosci 21: , 1359–1469. |
[45] | Silverberg GD ((2004) ) Normal pressure hydrocephalus (NPH): Ischaemia, CSF stagnation or both. Brain 127: , 947–948. |
[46] | Zamanian JL , Xu L , Foo LC , Nouri N , Zhou L , Giffard RG , Barres BA ((2012) ) Genomic analysis of reactive astrogliosis. J Neurosci 32: , 6391–6410. |
[47] | Liddelow SA , Guttenplan KA , Clarke LE , Bennett FC , Bohlen CJ , Schirmer L , Bennett ML , Münch AE , Chung WS , Peterson TC , Wilton DK , Frouin A , Napier BA , Panicker N , Kumar M , Buckwalter MS , Rowitch DH , Dawson VL , Dawson TM , Stevens B , Barres BA ((2017) ) Neurotoxic reactive astrocytes are induced by activated microglia. Nature 541: , 481–487. |
[48] | Holst CB , Brochner CB , Vitting-Seerup K , Mollgard K ((2019) ) Astrogliogenesis in human fetal brain: Comlex spatiotemporal immunoreactivity patterns of GFAP, S100, AQP4 and YKL-40. J Anat 235: , 590–615. |
[49] | Hua R , Liu C , Liu X , Zhu J , Zhang J , Wang L , Shi Z , Li J , Kong S , Yang C , Liu N , Liu L , Sun J , Yang Q , Wu Y , Zhou Y , Li Y , Xing Y ((2021) ) Predictive value of cerebrospinal fluid biomarkers for tap test responsiveness in patients with suspected idiopathic normal pressure hydrocephalus. Front Aging Neurosci 13: , 665878. |
[50] | Jeppsson A , Höltta M , Zetterberg H , Blennow K , Wikkelsø C , Tullberg M ((2016) ) Amyloid mis-metabolism in idiopathic normal pressure hydrocephalus. Fluids Barriers CNS 13: , 13. |
[51] | Manniche C , Simonsen AH , Hasselbalch SG , Andreasson U , Zetterberg H , Blennow K , Høgh P , Juhler M , Hejl AM ((2020) ) Cerebrospinal fluid biomarkers to differentiate idiopathic normal pressure hydrocephalus from subcortical ischemic vascular disease. J Alzheimers Dis 75: , 937–947. |
[52] | Mogensen FL , Delle C , Nedergaard M ((2021) ) The glymphatic system (en)during inflammation. Int J Mol Sci 22: , 7491. |
[53] | Klassen BT , Ahlskog JE ((2011) ) Normal pressure hydrocephalus: How often does the diagnosis hold water? Neurology 77: , 1119–1125. |
[54] | Golomb J , Wisoff J , Miller DC , Boksay I , Kluger A , Weiner H , Salton J , Graves W ((2000) ) Alzheimer’s disease comorbidity in normal pressure hydrocephalus: Prevalence and shunt response. J Neurol Neurosurg Psychiatry 68: , 778–781. |
[55] | Silverberg GD , Mayo M , Saul T , Fellmann J , Carvalho J , McGuire D ((2008) ) Continuous CSF drainage in AD: Results of a double-blind, randomized, placebo-controlled study. Neurology 71: , 202–209. |
[56] | Muller-Schmitz K , Krasavina-Loka N , Yardimci T , Lipka T , Kolman A , Robbers S , Menge T , Kujovic M , Seitz RJ ((2020) ) Normal pressure hydrocephalus associated with Alzheimer’s disease. Ann Neurol 88: , 703–711. |
[57] | Kazui H , Kanemoto H , Yoshiyama K , Kishima H , Suzuki Y , Sato S , Suehiro T , Azuma S , Yoshimine T , Tanaka T ((2016) ) Association between high biomarker probability of Alzheimer’s disease and improvement of clinical outcomes after shunt surgery in patients with idiopathic normal pressure hydrocephalus. J Neurol Sci 369: , 236–241. |
[58] | Gispert JD , Suarez-Calvet M , Monte GC , Tucholka A , Falcon C , Rojas S , Rami L , Sánchez-Valle R , Lladó A , Kleinberger G , Haass C , Molinuevo JL ((2016) ) Cerebrospinal fluid sTREM2 levels are associated with gray matter volume increases and reduced diffusivity in early Alzheimer’s disease. Alzheimers Dement 12: , 1259–1272. |
[59] | Knapskog AB , Henjum K , Idland AV , Eldholm RS , Persson K , Saltvedt I , Watne LO , Engedal K , Nilsson LNG ((2020) ) Cerebrospinal fluid sTREM2 in Alzheimer’s disease: Comparisons between clinical presentation and AT classification. Sci Rep 10: , 15886. |
[60] | Su Z , Xu Z , Liu H , Liu S ((2015) ) Distance and similarity measures for dual hesitant fuzzy sets and their applications in pattern recognition. J Intel Fuzzy Syst 29: , 731–745. |
[61] | Migliorati K , Panciani PP , Pertichetti M , Borroni B , Archetti S , Rozzini L , Padovani A , Terzi L , Bruscella S , Fontanella MM ((2021) ) P-Tau as prognostic marker in long term follow up for patients with shunted iNPH. Neurol Res 43: , 78–85. |