Effects of S-Adenosylmethionine on Cognition in Animals and Humans: A Systematic Review and Meta-Analysis of Randomized Controlled Trials
Abstract
Background:
There is increasing evidence that supplementation of S-adenosylmethionine (SAM) can improve cognitive function in animals and humans, although the outcomes are not always inconsistent.
Objective:
We conducted a systematic review and meta-analysis to evaluate the correlation between SAM supplementation and improved cognitive function.
Methods:
We searched studies in the PubMed, Cochrane Library, Embase, Web of Science, and Clinical Trials databases from January 1, 2002 to January 1, 2022. Risk of bias was assessed using the Cochrane risk of bias 2.0 (human studies) and the Systematic Review Center for Laboratory Animal Experimentation risk of bias (animal studies) tools; and evidence quality was evaluated using the Grading of Recommendations Assessment, Development, and Evaluation. STATA software was employed to perform meta-analysis, and the random-effects models was used to evaluate the standardized mean difference with 95% confidence intervals.
Results:
Out of the 2,375 studies screened, 30 studies met the inclusion criteria. Meta-analyses of animal (p = 0.213) and human (p = 0.047) studies showed that there were no significant differences between the SAM supplementation and control groups. The results of the subgroup analyses showed that the animals aged ≤8 weeks (p = 0.027) and the intervention duration >8 weeks (p = 0.009) were significantly different compared to the controls. Additionally, the Morris water maze test (p = 0.005) used to assess the cognitive level of the animals revealed that SAM could enhance spatial learning and memory in animals.
Conclusion:
SAM supplementation showed no significant improvement in cognition. Therefore, further studies are needed to assess the effectiveness of SAM supplementation.
INTRODUCTION
“Cognitive frailty”, which is a common clinical symptom, is defined by the presence of cognitive function decline and physical frailty simultaneously in older adults, without a clear diagnosis of dementia [1]. It is a precursor to numerous neurodegenerative diseases, including Parkinson’s disease, mild cognitive impairment (MCI), and Alzheimer’s disease (AD). As it is reversible, it is an available objective in terms of prevention [2]. AD is a chronic neurodegenerative disease that manifests in gradual cognitive function decline, such as memory and learning, progressing to dementia. With the rapid aging of the population, approximately one new case of AD every 3 seconds, and the number of cases is expected to increase to 150 million by 2050, imposing a significant economic burden on families and society [3]. However, early prevention can effectively control the progression of cognitive decline [4]. Therefore, there is an urgent need to identify effective interventions or supplements for delaying cognition decline.
Recently, S-adenosylmethionine (SAM), which was discovered by Italian scientists in 1952 [5], has emerged as a key factor influencing neurophysiological and psychophysiological functions in animals and humans, including neurodevelopment, emotion, and cognition [6]. SAM is a natural compound produced in the liver from adenosine triphosphate and methionine under enzymatic catalysis. As a methyl donor, SAM participates in deoxyribonucleic acid (DNA) and ribonucleic acid (RNA) methylation. Methylation refers to methyl group transfer from the active methyl compounds to other compounds through catalysis, including proteins and nucleic acids. These compounds can be chemically modified to generate important substances [7].
Several studies [5, 8] have shown that SAM can cross the blood-brain barrier in animals and humans, and plays an integral role in slowing cognitive decline by modulating important factors, such as the methionine metabolic cycle, methylation, and oxidation metabolism [9, 10]. It has been confirmed that low SAM levels in cerebrospinal fluid in some patients with dementia may lead to disorders of methylation metabolism in the nervous system, including hyperhomocysteine, folic acid, and vitamin B12 deficiency [8]. However, some studies have shown [9] that SAM does not affect the cognitive function of some patients. To date, animal and human studies have reported conflicting results, which present a challenge in drawing definitive conclusions regarding SAM’s improvement in cognitive performance.
Therefore, this systematic review and meta-analysis aimed to provide qualitative and quantitative results on the effects of SAM supplements on the cognition of animals and humans, to provide reliable references for subsequent studies.
MATERIALS AND METHODS
This meta-analysis was registered in PROSPERO (CRD42022316443) and was conducted following the Preferred Reporting Items for Systematic Reviews and Meta-Analyses guidelines [11].
Literature search strategy
A systematic literature search was conducted to identify studies on the effect of SAM supplementation on cognitive function in animals and humans. Five electronic databases were searched (PubMed, Cochrane Library, Embase, Web of Science, and Clinical Trials [https://clinicaltrials.gov/]) to identify potentially eligible articles from January 1, 2002 to January 1, 2022, using the following terms: (“S-adenosylmethionine” OR “S-adenosyl methionine” OR “S-adenosyl-L-methionine” OR “SAM” OR “SAM-e” OR “AdoMet”) AND (“Alzheimer Disease” OR “Alzheimer*” OR “dementia” OR “cognitive” OR “cognition”) AND (“RCT” OR “Random*” OR “control*” OR “randomised controlled trial”). The language of the study was limited to English. We screened the titles to exclude duplicate studies and reviews, and then screened the titles and abstracts to exclude inappropriate articles. Subsequently, the final inclusion was determined by reviewing the full text of the remaining studies. Gray literature and references of the selected articles were also examined to glean other possible publications (for a detailed description, see SupplementaryFile 1).
Inclusion and exclusion criteria
All studies included in the initial search strictly met the criteria of the Population, Intervention, Comparison, Outcome, and Study design framework, according to the Preferred Reporting Items for Systematic Reviews and Meta-Analyses recommendations [11].
Animals
a) Subjects were female or male rodents with or without cognitive impairment; b) The intervention was SAM supplements; c) Randomized controlled trials, including control and experimental groups; d) The outcome was a cognitive function test, including the Morris water maze (MWM) test, T- or Y-maze test, Novel Object Recognition (NOR) test, or passive avoidance test; and e) Studies without full text or primary data that could not be extracted electronically were excluded.
Humans
a) Participants were older than 18 years. There were no restrictions on baseline cognitive status due to the differences in the cognitive assessment criteria; b) Pregnant or lactating women, epilepsy, mania, bipolar affective disorder, and other potentially dangerous conditions were excluded; c) Experiments that including SAM intervention alone or in a nutritional supplement form (any dose or method of administration) to improve cognitive function; d) The same conditions were applied to the control group, except that there was no SAM or nutritional supplementation intervention; e) At least one cognitive function outcome was assessed; and f) Randomized controlled trials and open-label experiments were included.
Data extraction
The following information was extracted independently and cross-checked by two reviewers: Author(s), year, country, study design, sample size, age, diagnosis, intervention duration, and measurement of cognition. Furthermore, animal models were extracted for animal studies. Data were collected from the graph using the GetData software. The primary data were estimated based on the coordinate axis, and the mean and standard deviation were calculated statistically. If the data were unavailable, we contacted authorsfurther.
Risk of bias and quality assessment
Two authors independently assessed the risk of bias in human studies using the Cochrane RoB 2.0 tool [12] per protocol for parallel-group randomized trials, and in animal studies using the Systematic Review Centre for Laboratory Animal Experimentation tool [13]. The reviewers assessed each study item as “high risk”, “low risk”, or “unclear risk” of bias. A third reviewer discussed the results if any disagreements existed. The Grading of Recommendations Assessment, Development and Evaluation (GRADE) methodology was used to evaluate the quality of the body of retrieved evidence (GRADEpro; https://gdt.gradepro.org/app/#projects). The GRADE system assessed evidence quality in the following four classes: high, moderate, low, or very low. The initial grading would decrease if there were study limitations, inconsistencies, imprecision, indirectness, or publication bias [14].
Data synthesis and analysis
Statistical analysis was performed using the Stata software version 16.0 (StataCorp, College Station, TX, USA). Data were described as the standardized mean difference (SMD) and the effect size 95% confidence intervals (CI). A random effects model [15] was used to present the data. Statistical significance was set at p < 0.05. Furthermore, the degree of heterogeneity was assessed using the I2 statistic, with I2 values of 25, 50, and 75% being considered to indicate low, moderate, and high heterogeneity, respectively [16]. A sensitivity analysis was performed when high heterogeneity was present. In addition, subgroup analyses were performed by animal age, cognitive tests, intervention duration, cognitive domains, or cognitive scales. Funnel plots were used to examine the potential publication bias.
RESULTS
Study selection
The initial search identified 2,928 articles, with 403, 137, 2,000, 385, and 3 from PubMed, Cochrane, Embase, Web of Science, and Clinical Trials respectively (Fig. 1). After removing duplicates, 36 articles were screened based on their titles and abstracts, and 2,339 were excluded. The full texts of the remaining 36 articles were reviewed, and 19 were excluded because they did not meet the inclusion criteria. Overall, 17 studies were included in the systematic review and 13 in the meta-analysis.
Fig. 1
Flowchart for searching and selection of the included studies.
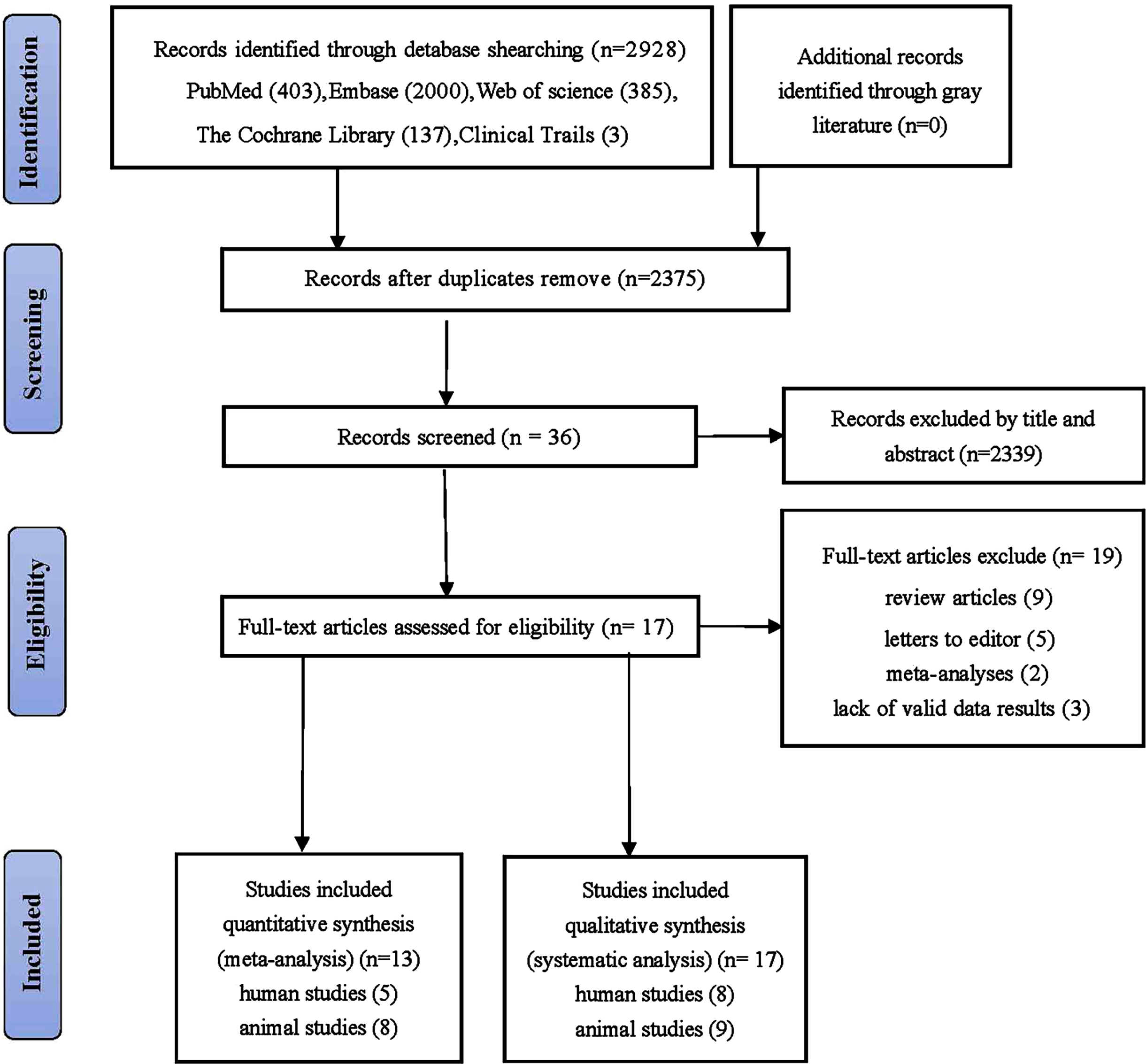
Study characteristics
Across the nine animal articles [17–25] included in the systematic review, eight studies [17–19, 21–25] contained meta-analysis that met the eligibility criteria (with some articles comprising of multiple eligible experiments or datasets). Three of the studies [19, 20, 21] were conducted in the United States, one in Denmark [22], two in China [18, 25], one in Italy [21], one in Australia [17], and one in Canada [21]. All studies were published between 2004 and 2020. Furthermore, the effect of SAM on spatial learning and memory was assessed using extra-maze cues to navigate in the MWM, spontaneous alternation in a T- or Y-maze, or NOR tasks. Moreover, six, three, and thirteen experiments used the MWM, NOR, and the T- or Y-maze, respectively (Table 1).
Table 1
Characteristics of included animal studies.
Study | Study design | Region | Animal | Sample size | Age (weeks) | Duration (weeks) | Measurement | Included in | |
models and Diseases | Control | Experimental | of cognition / cognitive tests | meta-analyses? | |||||
Tillmann et al., 2019 [24] | RCT | Denmark | FSL | 10 | 9 | 10.4±2.1 weeks | 4 weeks | NOR; Y-Maze | yes |
Chan et al., 2008A [19] | RCT | USA | C57B/6 | 7 | 7 | 36–48 weeks | 4 weeks | Y-maze | yes |
Chan et al., 2008B [19] | RCT | USA | ApoE – /– | 7 | 7 | 36–48 weeks | 4 weeks | Y-maze | yes |
Chan et al., 2008C [19] | RCT | USA | ApoE2 | 7 | 7 | 36–48 weeks | 4 weeks | Y-maze | yes |
Chan et al., 2008D [19] | RCT | USA | ApoE3 | 7 | 7 | 36–48 weeks | 4 weeks | Y-maze | yes |
Chan et al., 2008E [19] | RCT | USA | ApoE4 | 7 | 7 | 36–48 weeks | 4 weeks | Y-maze | yes |
Chan et al., 2008F [19] | RCT | USA | C57B/6 | 7 | 7 | 104–128 weeks | 4 weeks | Y-maze | yes |
Chan et al., 2008G [19] | RCT | USA | MTHFR+/– | 7 | 7 | 36–48 weeks | 4 weeks | Y-maze | yes |
Chan et al., 2008H [19] | RCT | USA | MTHFR+/+ | 7 | 7 | 36–48 weeks | 4 weeks | Y-maze | yes |
Tchantchou et al., 2004 [23] | RCT | USA | ApoE– /– | 3-4 | 3-4 | 36–48 weeks | 4 weeks | Y-maze; T-maze | yes |
Shea 2007A [20] | RCT | USA | normal C57B/6 | – | 8 | 36–48 weeks | 2 weeks-4 weeks | Y-maze | no |
Shea 2007B [20] | RCT | USA | ApoE4 | 6 | 6 | 36–48 weeks | 2 weeks-4 weeks | Y-maze | no |
Cao et al., 2008A [18] | RCT | China | Control | 10 | 9 | 3 weeks | 3 weeks | MWM | yes |
Cao et al., 2008B [18] | RCT | China | Pb exposure | 9 | 9 | 3 weeks | 3 weeks | MWM | yes |
Wan et al., 2020A [25] | RCT | China | C57 | 20 | 20 | 8 weeks | 28 weeks | MWM | yes |
Wan et al., 2020B [25] | RCT | China | APP/PS1 | 9–15 | 9–15 | 8 weeks | 28 weeks | MWM | yes |
Fuso et al., 2012A [21] | RCT | Italy | TgCRND8 | 10 | 9 | 12 weeks | 12 weeks | PAT; MWM | yes |
Fuso et al., 2012B [21] | RCT | Italy | 129Sv | 15 | 12 | 12 weeks | 12 weeks | PAT; MWM | yes |
Beauchamp et al., 2020A [17] | RCT | Australia | WT | 6 | 6 | 24 weeks | 3 weeks | Y-maze | no |
Beauchamp et al., 2020B [17] | RCT | Australia | rTg4510 | 7 | 7 | 24 weeks | 3 weeks | Y-maze | yes |
Gregoire et al., 2017A [22] | RCT | Canada | CD1 | 7 | 8 | 10–12 weeks | 12 weeks | PAT; NOR | yes |
Gregoire et al., 2017B [22] | RCT | Canada | CD1 | 6 | 6 | 10–12 weeks | 12 weeks | PAT; NOR | yes |
FSL, Flinders Sensitive Line; APOE, apolipoprotein; MTHFR, methylene tetrahydrofolate reductase; C57, wild-type C57BL/6J mice; APP/PS1, APPswe/PS1dE9 (APP/PS1) mice; WT, wild-type mice; NOR, novel object recognition; MWM, Morris water maze tests; PAT, passive avoidance test; –, data not available.
In this systematic review, eight human articles were included [10, 26–32]. Five articles were included in the meta-analysis. In addition, seven studies were conducted in the United States and one in Israel. All studies were published between 2008 and 2016. The included studies consisted of six randomized controlled trials [6, 10, 27–31] and two open-label trials [26, 31]. Four studies [26, 28, 29, 31] examined cognition in patients with AD, whereas four [6, 10, 27, 30] were conducted on MCI, depression, schizophrenia, or patients without dementia. Six studies [6, 26, 28–31] investigated the effect of a nutriceutical formulation diet containing SAM; only two studies [10, 27]evaluated the effect of SAM supplementation. Furthermore, the impact of diet intervention on cognition was assessed through the measurement tool. Four studies [26, 28–30] used the Clock Drawing tests and Dementia Rating Scale, one study [31] used the Neuropsychiatric Inventory (NPI), one study [6] employed the California Verbal Learning Test II and Trail-making test, one study [9] used the Global cognitive scores, and one study [27] used the Massachusetts General Hospital Cognitive and Physical Functioning Questionnaire (Table 2).
Table 2
Characteristics of included human studies
Study | Study design | Region | Diseases | Sample size | Age (mean±SD) | Duration | Measurement of cognition/ | Included in | ||
Control | Experimental | Control | Experimental | (weeks) | cognitive tests | meta-analyses? | ||||
Chanet et al., 2008 [26] | Open-Label Study | USA | AD | 21 | – | 48 weeks | DRS; NPI; Clox; ADCS-ADL | no | ||
Remington et al., 2009 [28] | RCT | USA | AD | 6 | 6 | – | 36 weeks | DRS; NPI; Clox; ADCS-ADL | yes | |
Remington et al., 2015A [29] | RCT | USA | AD | 44 | 62 | 79.7±8.6 | 78.7±7.9 | 24 weeks | DRS; NPI; Clox; ADL | yes |
Remington et al., 2015B [30] | RCT | USA | MCI | 12 | 22 | 79.7±8.6 | 78.7±7.9 | 24 weeks | DRS; Clox | yes |
Remington et al., 2016 [31] | Open-Label Study | USA | AD | 24 | 78.4±5.7 | 52 weeks | ADL; NPI | no | ||
Chan et al., 2010 [6] | RCT | USA | subjects without dementia | 56 | 59 | – | 12 weeks | CVLT II; Trail-making test | yes | |
Strous et al., 2009 [9] | RCT | Israel | schizophrenia | 8 | 7 | 43.7±14.8 | 39±8.9 | 9 weeks | Global cognitive scores | no |
Levkovitz et al., 2012 [27] | RCT | USA | MDD | 19 | 27 | 50.5±9.7 | 54.3±13.5 | 6 weeks | CPFQ | yes |
RCT, randomized controlled trial; SD, standard deviation; AD, Alzheimer’s disease; MCI, mild cognitive impairment; MDD, Major depressive disorder; DRS, Dementia Rating Scale; NPI, Neuropsychiatric Inventory; Clox, Clock Drawing Tests; ADCS-ADL, Alzheimer’s Disease Cooperative Study– Activities of Daily Living; CVLT II, California Verbal Learning Test II; CPFQ, Massachusetts General Hospital Cognitive and Physical Functioning Questionnaire; –, data not available.
Study quality
Animals
All nine animal studies included showed that the selection bias resulting from random sequences was unclear. Although randomization was reported in most articles, the method was not described in sufficient detail. Overall, 100% of the allocation concealment (selection bias) and blinding (performance bias) results showed an unclear risk. Approximately 40% of the studies showed a low risk of attrition bias in follow-up with missing or incomplete outcome data. In addition, 55% of the studies indicated a low risk of reporting bias. We considered that other bias tests were at high risk because none of the articles provided sufficient information regarding the results. (Supplementary File 2; Fig. 2A).
Fig. 2A
Risk-of-bias assessments of the included animal studies (domains from the Cochrane Handbook for Systematic Reviews of Interventions).
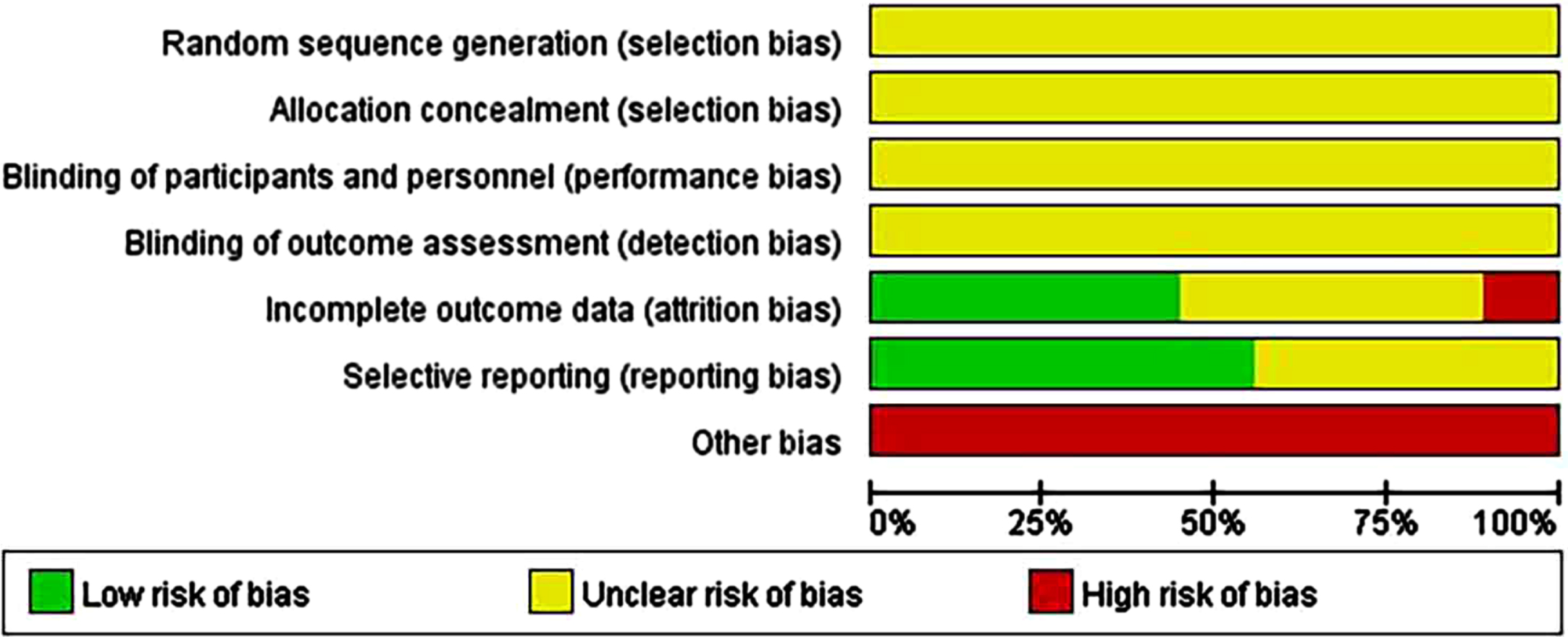
Humans
In six human studies, the minority described randomization methods. In addition, 50% of the studies did not describe the allocation concealment methods in sufficient detail; 70% of human studies were regarded as having an unclear risk of selection bias. Overall, 60% –90% of the included studies were considered to have a low risk of bias for blinding of outcome assessments (detection bias), incomplete outcome data (attrition bias), and selective reporting (reporting bias). All human studies considered as a high risk of other biases included those lacking sample size analysis and those with images rather than concrete data (Supplementary File 3; Fig. 2B).
Fig. 2B
Risk-of-bias assessments of the included human studies (domains from the Cochrane Handbook for Systematic Reviews of Interventions).
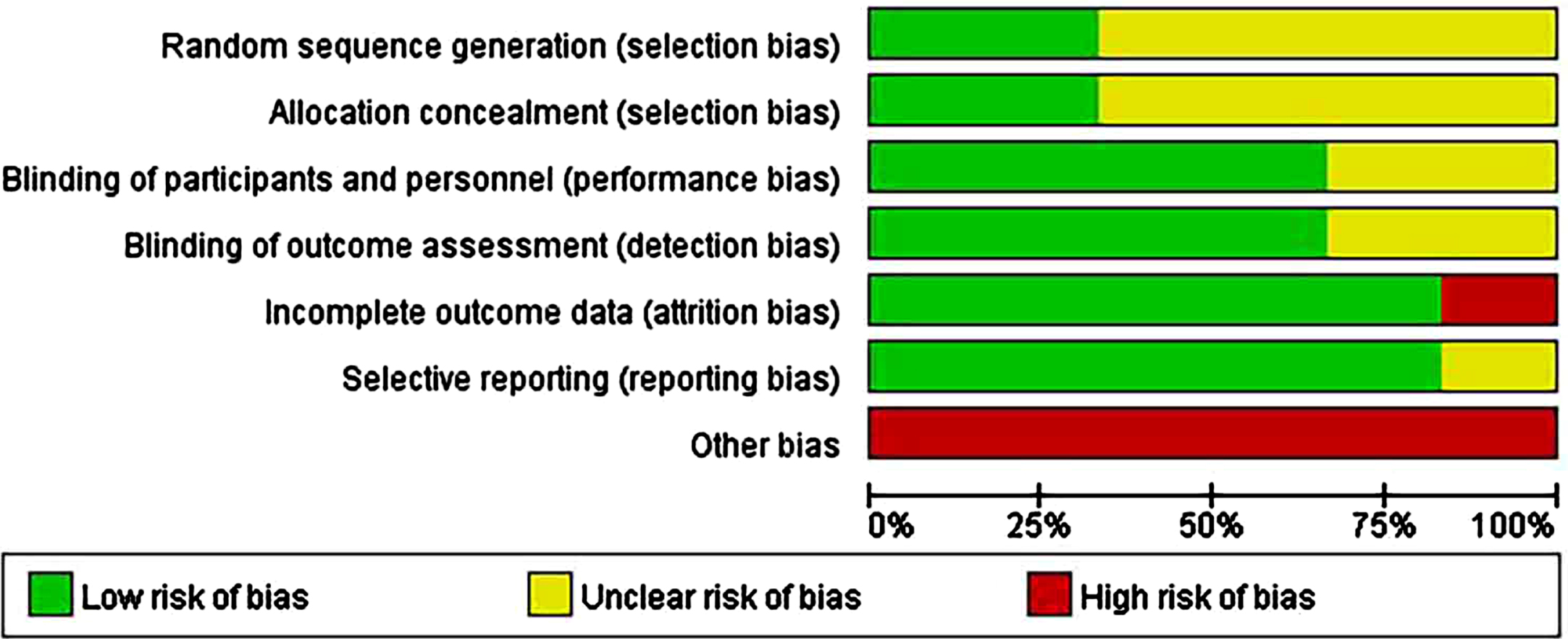
Qualitative analysis
Qualitative analysis of animal studies
Overall, nine studies reported an association between SAM supplements and enhanced cognitive function. Four studies [17, 18, 20, 25] revealed that SAM could improve the spatial memory deficit of animals; however, SAM might have neuroprotective effects only in some kinds of cognitive disorder and does not affect learning and memory under normal conditions [18, 21]. Fuso et al. [21] clearly demonstrated that SAM inhibits the progression of Alzheimer-like features. Furthermore, Gregoire et al. [22] reported that chronic systemic administration of SAM reduces cognitive impairment. A study showed that mice at 9 months of age do not exhibit signs of neuronal trauma when maintained on deficient SAM, whereas increases oxidative damage and impairs performance in the Y-maze and T-maze in normal mice aged 2–2.5 years [23]. Therefore, this indicates that SAM effects may depend on age. In contrast, Tillmann et al. [24] reported that monotherapy with SAM had no impact on cognition.
Qualitative analysis of human studies
In human studies, SAM can delay cognitive decline (including baseline cognitive performance, behavioral and psychological symptoms of dementia, AD Cooperative Study– Activities of Daily Living, MCI, Chronic Schizophrenia and Depression) [10, 26, 27–29]. Simultaneously, Chan et al. [26] reported that cognitive improvement in SAM is closely related to supplementation time. Three studies [26, 28, 29] used the NPI to evaluate cognitive improvement in patients with AD. The results revealed that patients who received SAM for 9 months improved by approximately 30% in NPI, and those who received SAM for 1 year improved in many areas of NPI. However, a study [29] indicated that no significant improvement was observed in NPI after 3 months of SAM supplementation. An analysis of adults without dementia showed that participants supplemented with SAM had statistical significance and clinical improvement in the California Language Learning Test II and trail-making test [6]. Nevertheless, participants older than 74 years showed no significant improvements, which could be attributed to age-related cognitivedecline.
Results of the meta-analysis
Meta-analysis of animal studies
Experimental versus controls. The overall data from the eight animal studies involving 28 comparisons suggested that SAM supplements did not significantly improve cognition (SMD = –0.12, 95% Cl: [–0.30, 0.07], p = 0.213), and moderate heterogeneity was present (χ2 = 99.05, p < 0.001) (Fig. 3). The quality of evidence using the GRADE summary between SAM intervention and control for animals was considered to be moderate (Table 3).
Table 3
GRADE quality of evidence assessment of outcome
Outcome indicator | Number of | Heterogeneity | Model of | Group effect value | Estimated | 95% CI | Grade | ||
included cases | I2 | p | analysis | Z | p | value | |||
Between SAM intervention versus Control for animals | 537 | 72.7% | <0.0001 | Random effect | 0.02 | 0.984 | 0.00 (SMD) | –0.36, 0.36 | Moderate |
Between SAM intervention versus Control for human | 1296 | 41.4% | 0.025 | Random effect | 1.32 | 0.186 | 0.10 (SMD) | –0.05, 0.26 | High |
SMD, standardized mean difference; CI, confidence interval; GRADE, Grading of Recommendation Assessment, Development and Evaluation.
Fig. 3
Forest plot of 28 animal studies of overall effect of S-adenosylmethionine on cognition. SMD, Standardized mean difference; CI, confidence interval; p: heterogeneity p-value; SAM: S-adenosylmethionine; Control: placebo.
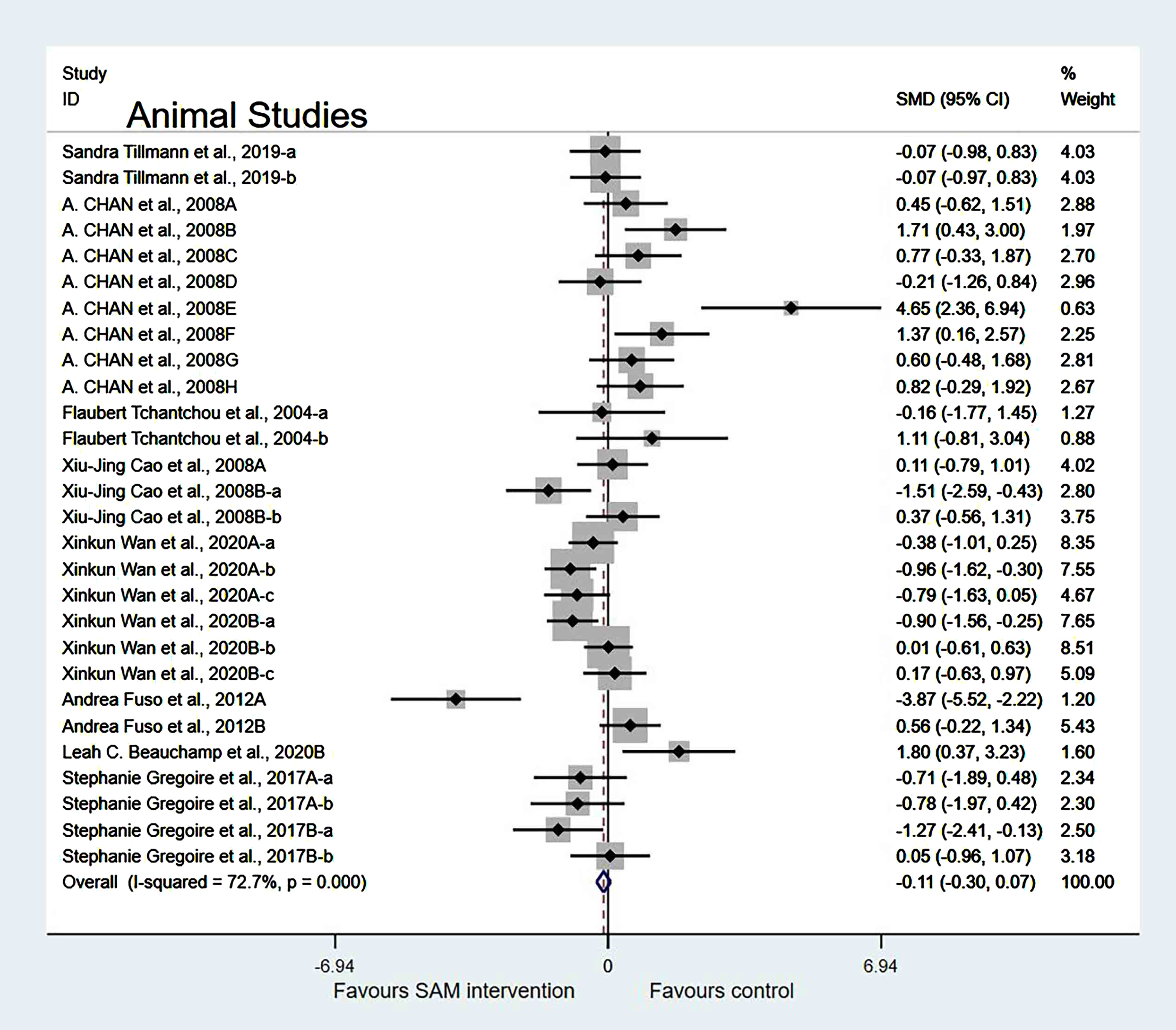
Subgroup analyses by animal age. The animal studies were divided into two subgroups based on animal age. The forest plot indicated that age of animals ≤8 weeks showed a significant difference (SMD = –0.42, 95% Cl: [–0.80, –0.05], p = 0.027). Meanwhile, age >8 weeks showed no significant difference in cognition (SMD = 0.28, 95% Cl: [–0.25, 0.80], p = 0.304) (Fig. 4).
Fig. 4
Forest plot of 28 animal studies of random-effects model subgroup analysis according to animal age. SMD, Standardized mean difference; CI, confidence interval; p: heterogeneity p-value; SAM: S-adenosylmethionine; Control: placebo.
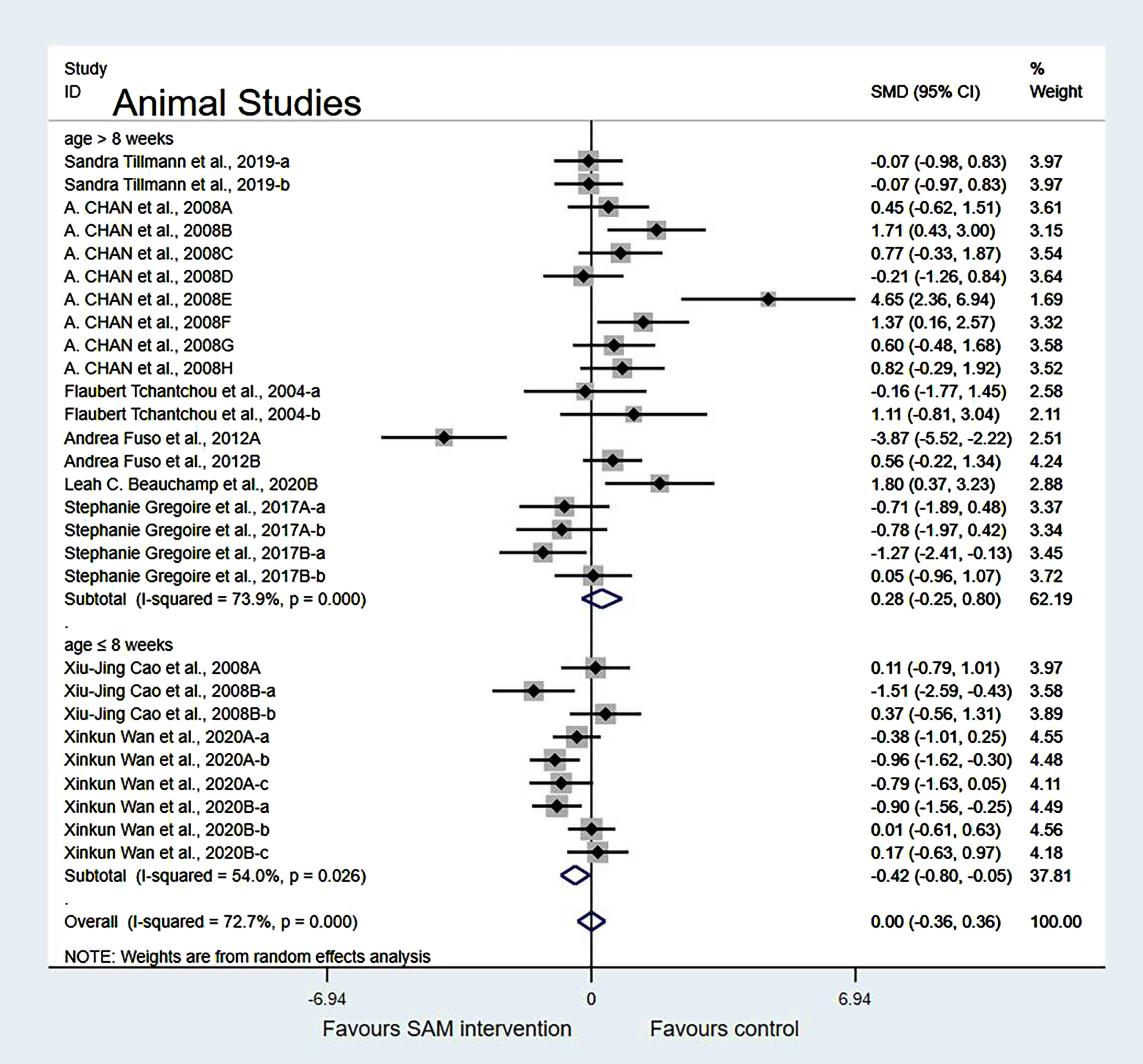
Subgroup analyses by cognition tests. Animal studies were categorized into the following three subgroups based on cognitive tests. Those using NOR (SMD = –0.20, 95% Cl: [–0.79, 0.38], p = 0.500) showed no significant difference in cognition; however, the Y-maze test (SMD = 0.65, 95% Cl: [0.21, 1.08], p = 0.003) and the MWM test (SMD = –0.82, 95% Cl: [–1.39, –0.25], p = 0.005) showed significant differences, which indicated that SAM significantly enhanced spatial cognitive function (Fig. 5).
Fig. 5
Forest plot of 16 animal studies of random-effects model subgroup analysis according to cognitive tests. SMD, Standardized mean difference; CI, confidence interval; p: heterogeneity p-value; SAM: S-adenosylmethionine; Control: placebo.
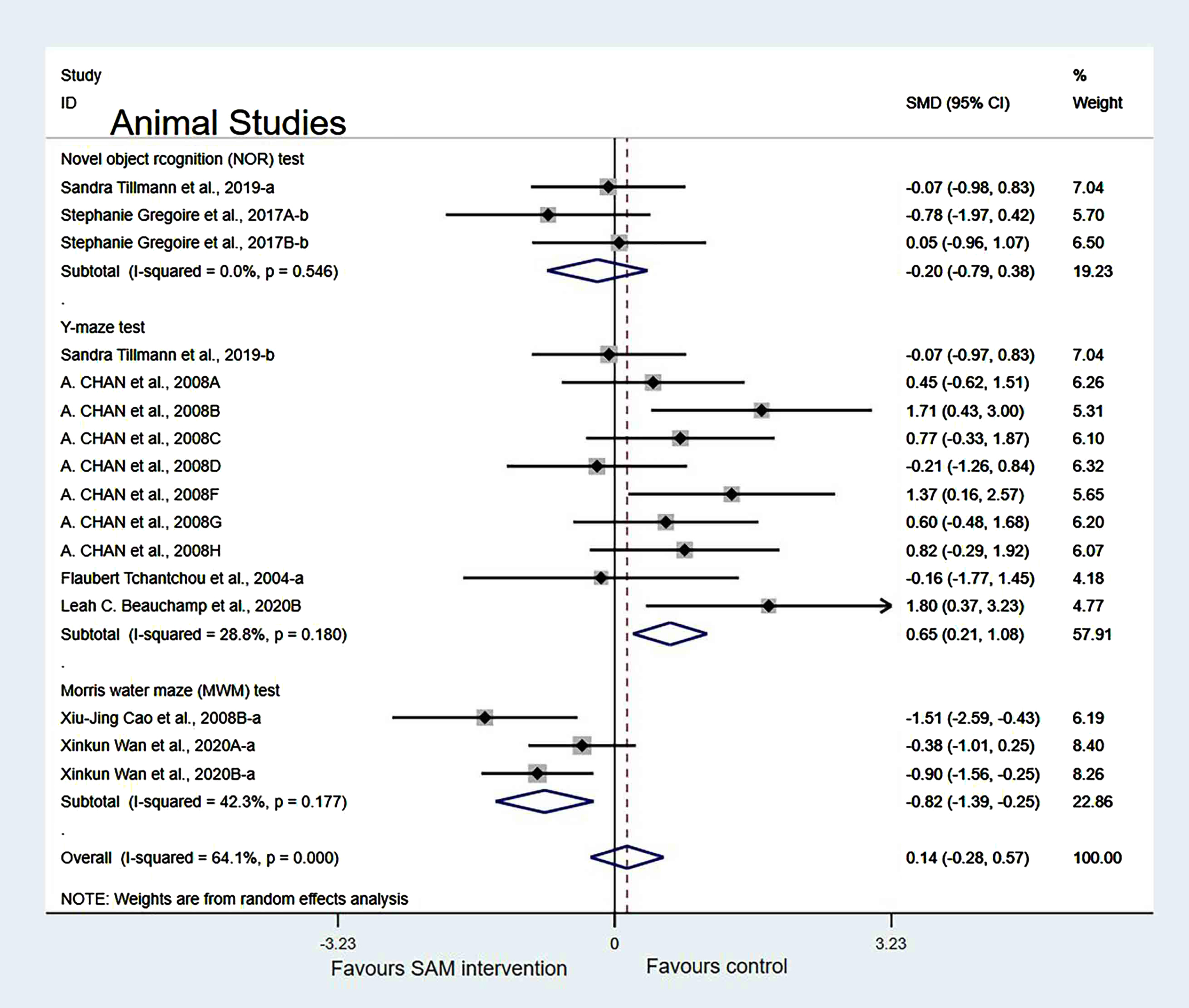
Subgroup analyses by intervention duration. When we analyzed the data according to SAM intervention duration (<8 weeks or >8 weeks), the forest plot showed that the duration of <8 weeks was not significantly different (SMD = 0.55, 95% Cl: [0.07, 1.03], p = 0.025). However, the duration of >8 weeks had a significant effect on cognition in animals (SMD = –0.59, 95% Cl: [–1.03, –0.15], p = 0.009) (Fig. 6).
Fig. 6
Forest plot of 28 animal studies of random-effects model subgroup analysis according to intervention duration. SMD, Standardized mean difference; CI, confidence interval; p: heterogeneity p-value; SAM: S-adenosylmethionine; Control: placebo.
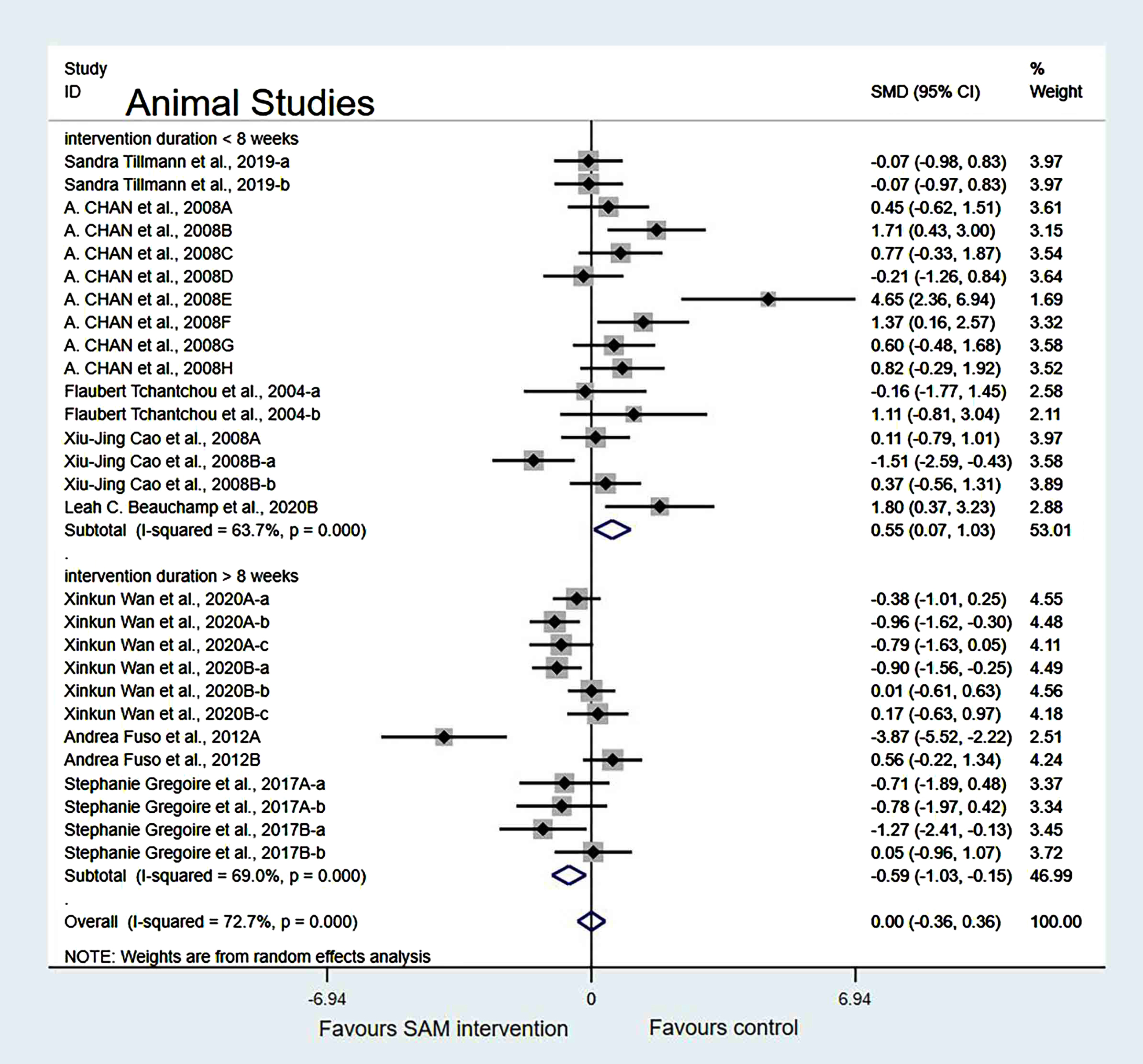
Sensitivity analysis. In the intervention duration of the subgroup, when we removed Chan et al. [18], Cao et al. [19], Wan et al. [21], and Fuso et al. [25] datasets, a slight decrease in the heterogeneity occurred when the test results were changed to 60.5% (χ2 = 55.73, p < 0.001). The combined effect sizes showed no significant differences (SMD = 0.11, 95% CI: [–0.23, 0.44], p = 0.251) (Fig. 7).
Fig. 7
Sensitivity meta-analysis of 23 animal studies between SAM intervention versus control according to intervention duration. SMD, Standardized mean difference; CI, confidence interval; p: heterogeneity p-value; SAM: S-adenosylmethionine; Control: placebo.
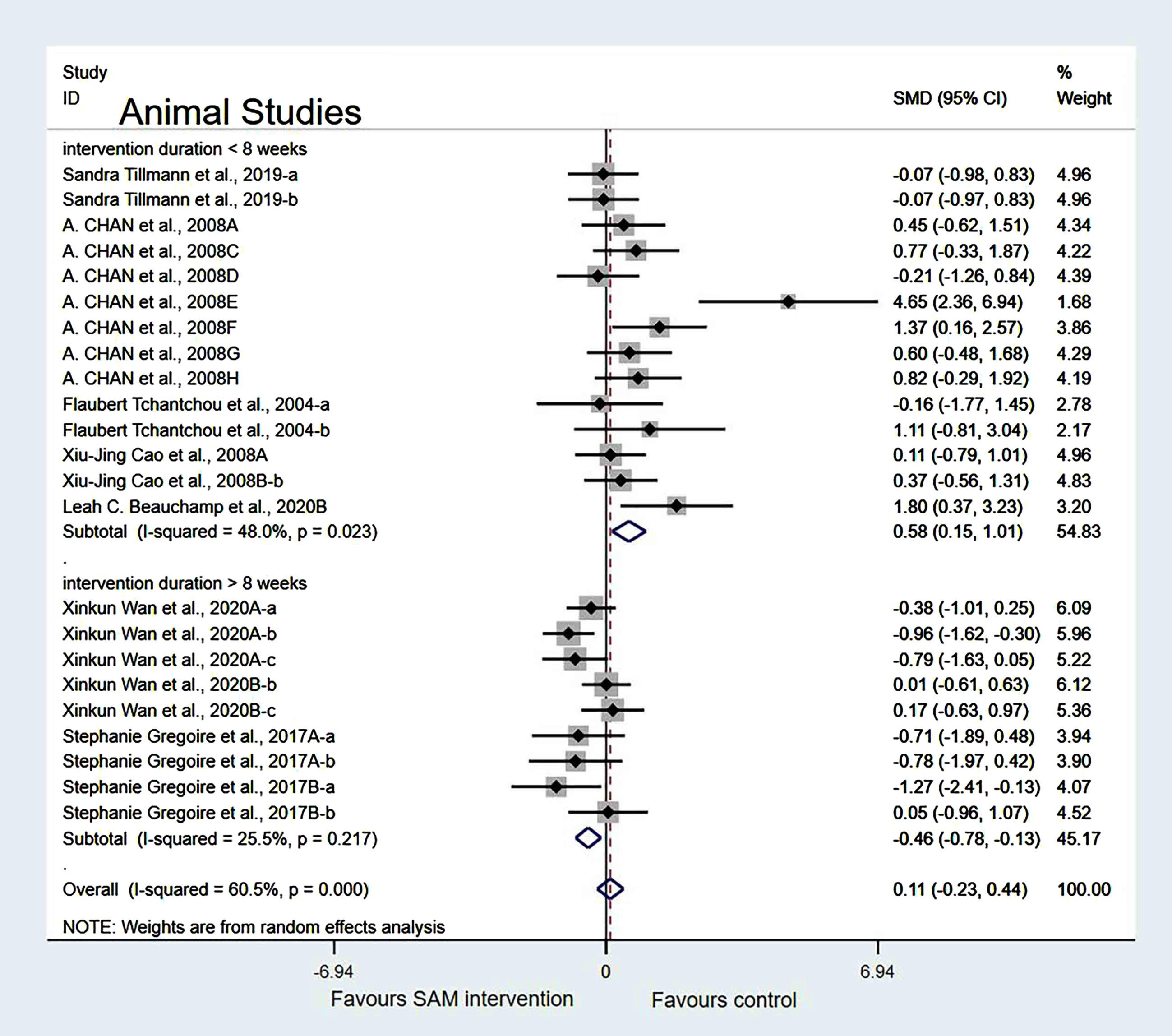
In the cognitive test subgroup, when Xinkun et al. [25] was removed[21, 22], the heterogeneity test results changed to 58.2% (χ2 = 33.48, p = 0.002); however, the difference was not statistically significant (SMD = 0.23, 95% CI: [–0.19, 0.66], p = 0.283) (Fig. 8).
Fig. 8
Sensitivity meta-analysis of 15 animal studies between SAM intervention versus control according to cognitive tests. SMD, Standardized mean difference; CI, confidence interval; p: heterogeneity p-value; SAM: S-adenosylmethionine; Control: placebo.
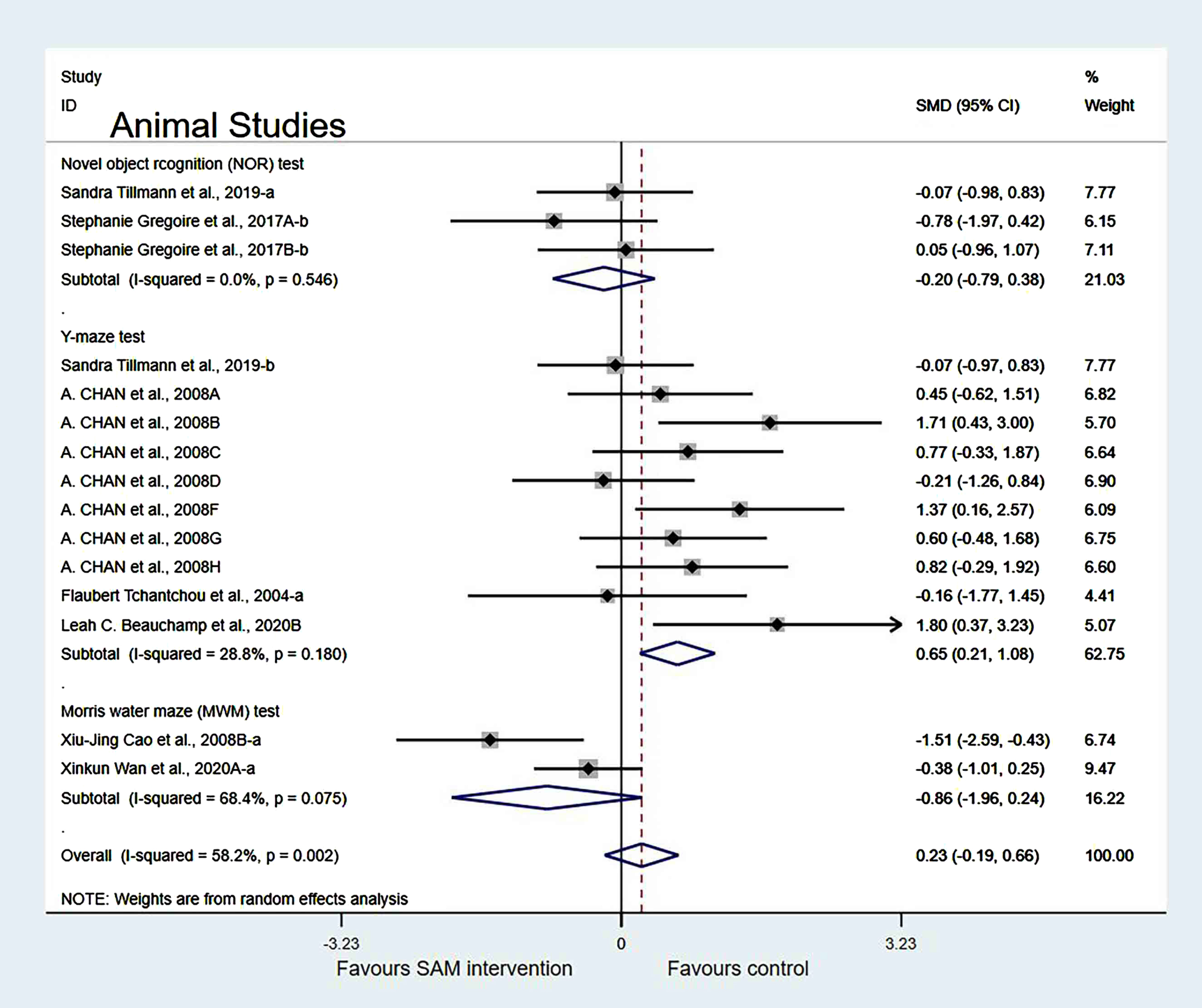
Finally, when Chan et al. [18], Cao et al. [19], Wan et al. [21], and Fuso et al. [25] were deleted simultaneously, the results of the meta-analysis did not change significantly (Fig. 9).
Fig. 9
Forest plot of 23 animal studies of overall effect of S-adenosylmethionine on cognition. SMD, Standardized mean difference; CI, confidence interval; p: heterogeneity p-value; SAM: S-adenosylmethionine; Control: placebo.
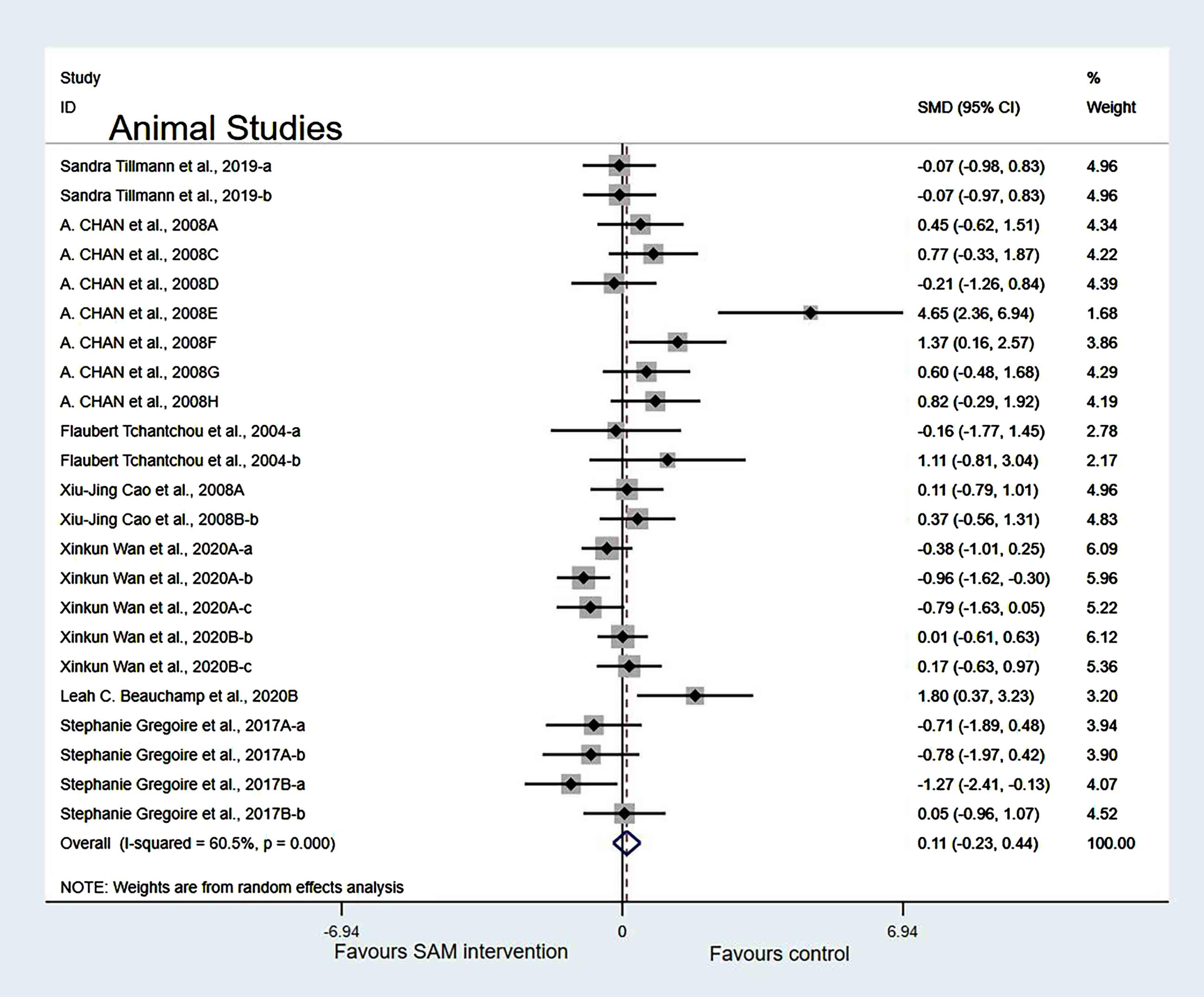
Publication bias. A funnel plot test on animal studies showed that eight datasets were located outside the dashed line, indicating a slight publication bias (Supplementary Figure 1).
Meta-analysis of human studies
Experimental versus controls. A meta-analysis of the five included human studies found no significant difference in cognitive function between the experimental and control groups (SMD = 0.10, 95% Cl: [–0.05, 0.26], p = 0.047), and there was a low heterogeneity (χ2 = 41.4%, p = 0.025) (Fig. 10). In addition, the quality of evidence using the GRADE summary between SAM intervention and control for humans was considered to be high (Table 3).
Fig. 10
Forest plot of 22 human studies of overall effect of S-adenosylmethionine on cognition. SMD, Standardized mean difference; CI, confidence interval; p: heterogeneity p-value; SAM: S-adenosylmethionine; Control: placebo.
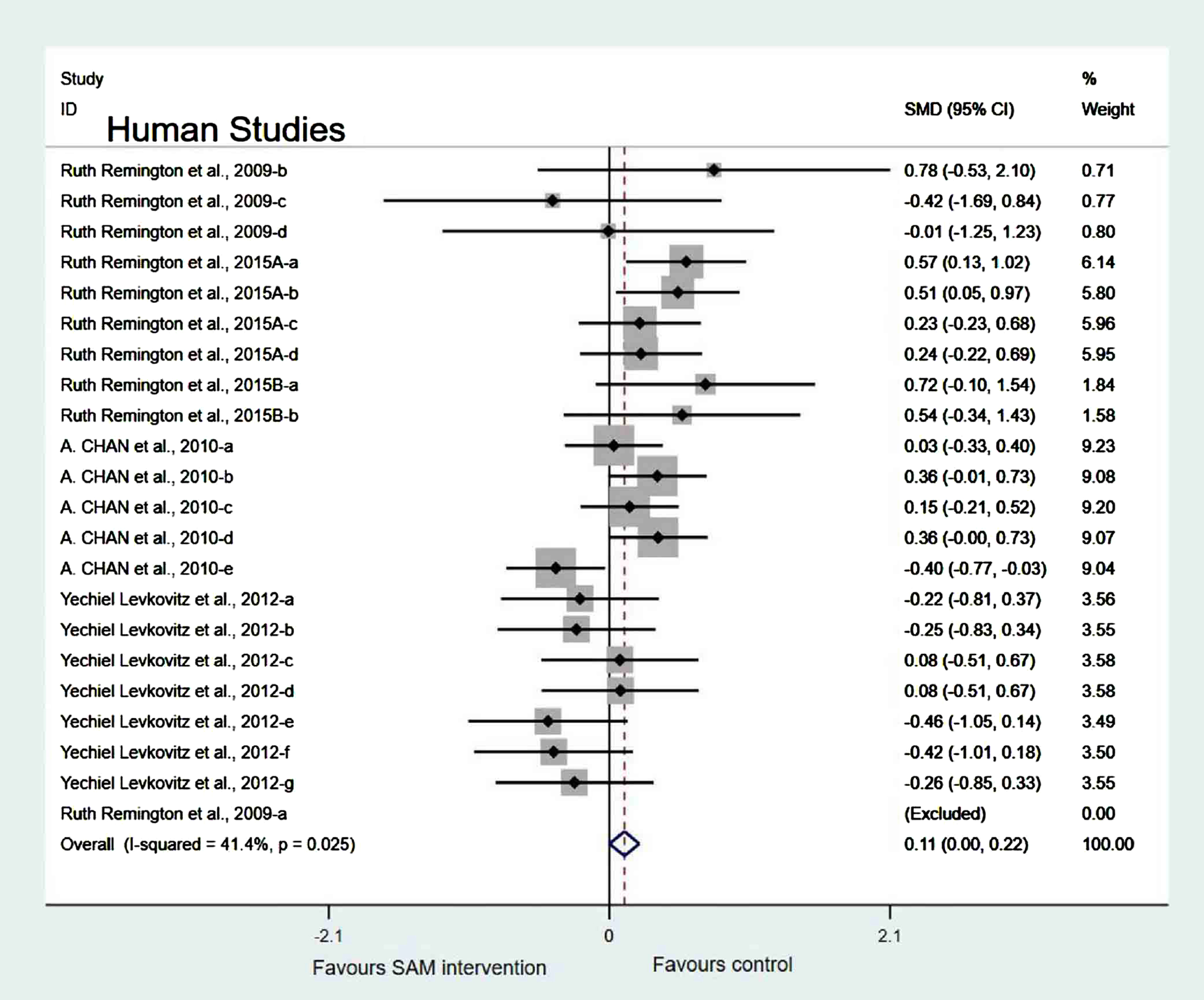
Subgroup analyses by cognitive domains. Human studies were categorized into three subgroups based on various cognitive domains. Global cognition was measured and the results showed a significant difference (SMD = 0.41, 95% Cl: [0.11, 0.70], p = 0.007). However, in the other two subgroups, different cognitive domains were measured using corresponding tests. Furthermore, executive function was measured and the results were insignificant (SMD = 0.25, 95% Cl: [–0.51, 1.02], p = 0.515), and the memory subgroup was evaluated to have no difference (SMD = 0.17, 95% Cl: [–0.03, 0.36], p = 0.098) (Fig. 11).
Fig. 11
Forest plot of 14 human studies of random-effects model subgroup analysis according to cognitive domains. SMD, Standardized mean difference; CI, confidence interval; p: heterogeneity p-value; SAM: S-adenosylmethionine; Control: placebo.
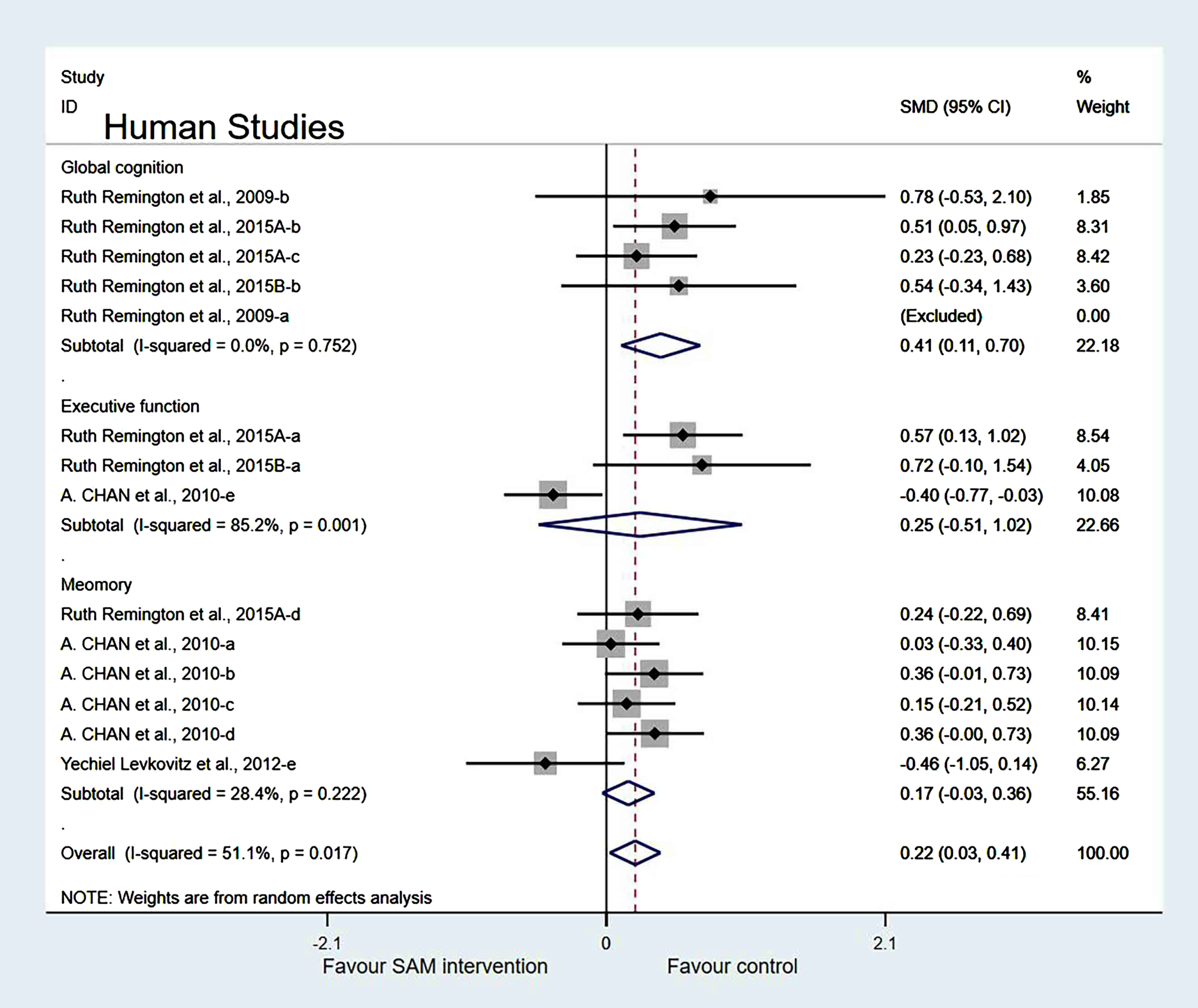
Subgroup analysis by measurement tool of cognition. Human studies were classified into two subgroups based on different cognitive scales for further investigation. Those using the Dementia Rating Scale (SMD = 0.36, 95% Cl: [0.11, 0.61], p = 0.005) and Clock Drawing test (SMD = 0.61, 95% Cl: [0.21, 1.00], p = 0.002) to assess cognitive level showed a significant difference (Fig. 12).
Fig. 12
Forest plot of 8 human studies of random-effects model subgroup analysis according to cognitive scales. SMD, Standardized mean difference; CI, confidence interval; p: heterogeneity p-value; SAM: S-adenosylmethionine; Control: placebo.
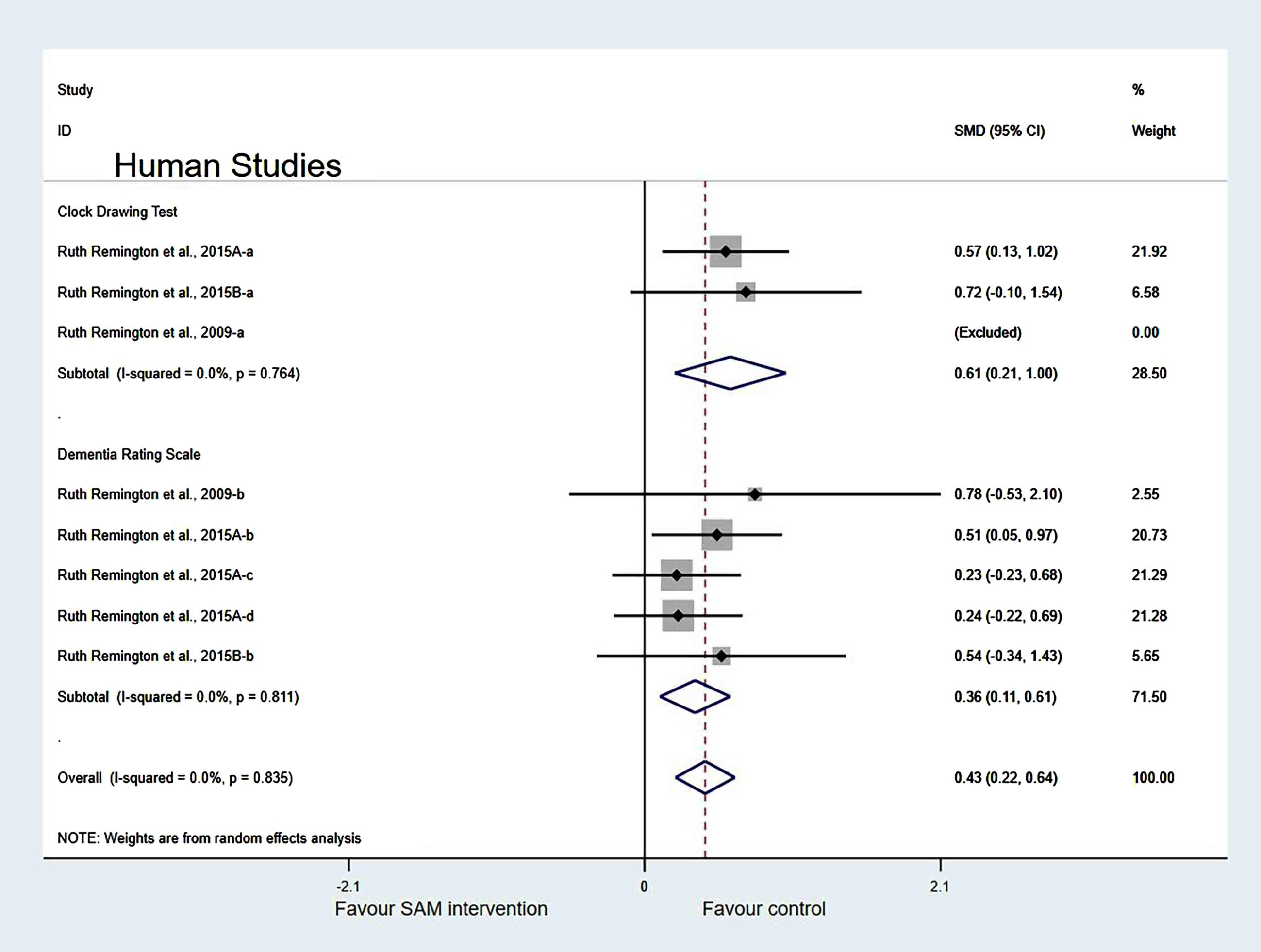
Sensitivity analyses. In the cognitive domains of the subgroup, when one of the Chan et al. [6] and Levkovitz et al. [27] datasets were eliminated, the heterogeneity significantly decreased (χ2 = 7.08, I2 = 0%, p = 0.718). The combined effect sizes showed higher cognitive function in the control group than in the experimental group, with significant differences (SMD = 0.31, 95% CI: [0.18, 0.45], p < 0.001) (Fig. 13).
Fig. 13
Sensitivity meta-analysis of 12 human studies between SAM intervention versus control according to cognitive domains. SMD, Standardized mean difference; CI, confidence interval; p: heterogeneity p-value; SAM: S-adenosylmethionine; Control: placebo.
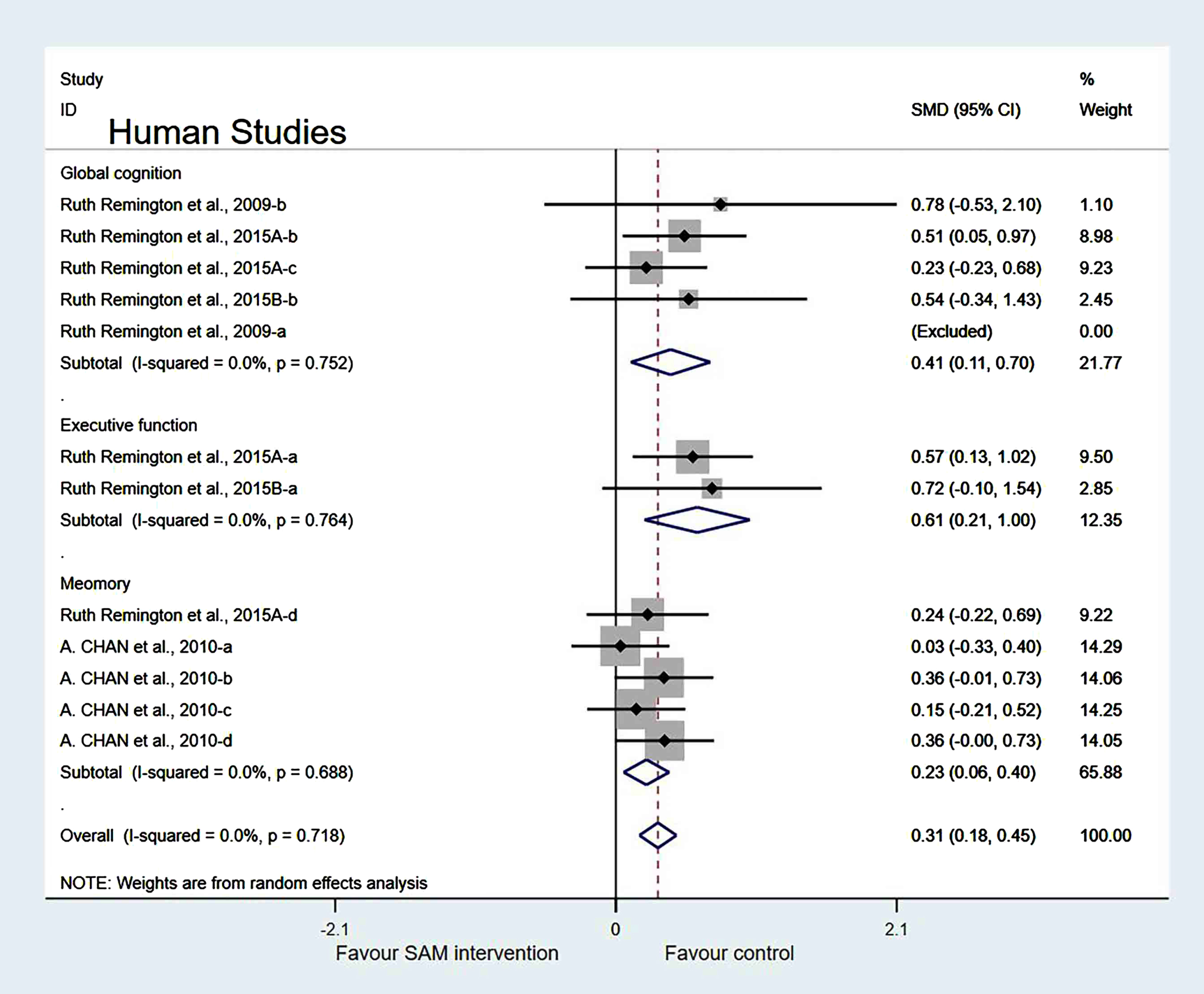
In the measurement tool of cognition, when two of the Remington et al. [29] datasets were removed, the heterogeneity test results were changed to 0% (χ2 = 0.29, I2 = 0%, p = 0.991). Additionally, the difference was statistically significant (SMD = 0.57, 95% CI: [0.30, 0.85], p < 0.001) (Fig. 14).
Fig. 14
Sensitivity meta-analysis of 6 human studies between SAM intervention versus control according to cognitive scales. SMD, Standardized mean difference; CI, confidence interval; p: heterogeneity p-value; SAM: S-adenosylmethionine; Control: placebo.
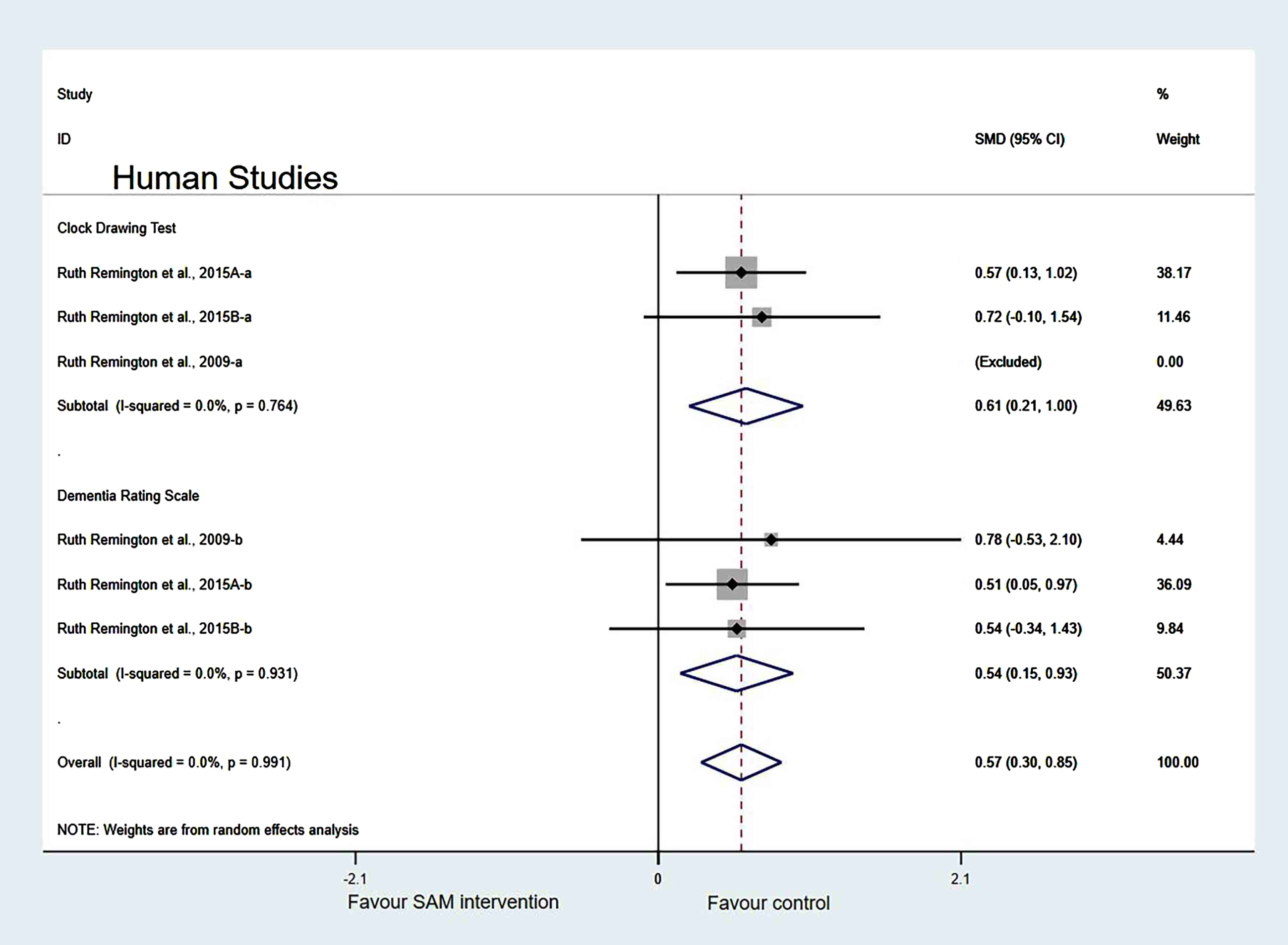
Finally, when we removed the Chan et al. [6], Levkovitz et al. [27], and Remington et al. [29] datasets simultaneously, the results of the meta-analysis were not significantly changed (Fig. 15).
Fig. 15
Forest plot of 18 human studies of overall effect of S-adenosylmethionine on cognition. SMD, Standardized mean difference; CI, confidence interval; p: heterogeneity p-value; SAM: S-adenosylmethionine; Control: placebo.
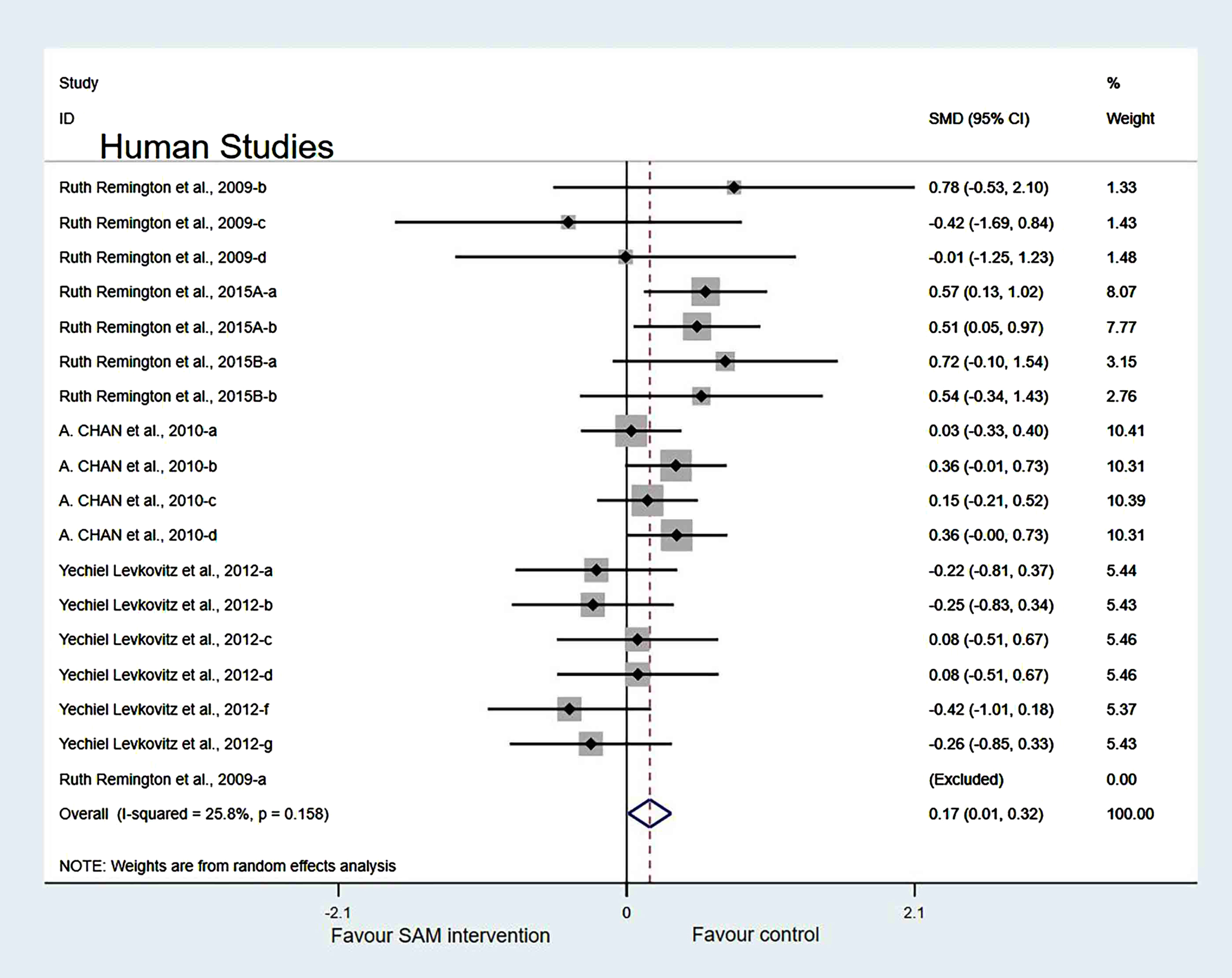
Publication bias. A funnel plot test on human studies showed that one study was located outside the dashed line and one study intersected with the dashed line; heterogeneity in others appeared approximately symmetric. This finding suggests that there was no significant publication bias (Supplementary Figure 2).
Results of evidence quality
Four outcomes were evaluated using the GRADE system. According to the evaluation results, high-quality evidence was found and one outcome provided moderate evidence. (Details in Supplementary File 4.)
DISCUSSION
We included all available animal and human data to provide the most comprehensive assessment to date of the effects of SAM supplementation on cognition in this systematic review and meta-analysis. Overall, according to our study, SAM supplementation improved the cognitive performance of animals, particularly regarding spatial learning and memory. In contrast, in human studies, the SAM intervention group showed no significant cognitive differences compared with the control group.
The following three points for qualitative analysis were concluded: a) According to the Y-maze and MWM tests, supplementation with SAM-containing feed or injection in mice and rats significantly improved spatial memory; b) Studies have shown that animal age influences the effects of SAM interventions; and c) Overall, SAM supplementation delayed cognitive decline in animals.
Our meta-analysis indicated the following five points: a) the age of animals ≤8 weeks and intervention duration >8 weeks could significantly improve the cognitive ability of animals; b) According to the MWM test subgroup analysis of animal studies, the escape latency in the experimental group was significantly better than that in the control group, implying that SAM supplementation improved spatial memory; the Y-maze showed a significant difference when SAM intervention was used to promote cognition; c) Subgroup analysis of human studies showed the same overall results, and SAM intervention had no significant effect on human cognition; d) Various cognitive tests have different effects on experimental results, whether in animal or human studies; and e) Sensitivity analyses suggested that the primary outcomes of the meta-analysis were affected by some studies; however, the results remained stable after excluding these studies.
SAM is a metabolite found in all living cells, and the adenosyl derivative of the amino acid methionine. It is a principal methyl-group donor in epigenetic regulation. Furthermore, SAM plays a central role in cellular biochemistry as a precursor to methylation, aminopropylation, and transsulfuration pathways [32–34]. It is widely used as a food supplement and marketed as a drug in some countries [33].
Neurodegenerative diseases, including MCI and AD, manifest as progressive cognitive decline and functional impairment. According to Talal et al. [35], DNA/RNA methylation plays a vital role in neurodegenerative processes. Furthermore, some studies have shown that decreased cognitive function pathogenesis is correlated with changes in DNA or RNA methylation [36–39]. DNA methyltransferase, which transfers the methyl group from SAM, the principal methyl donor, to the fifth position of cytosine (5-methylcytosine, 5-mC) on the DNA strand, performs DNA methylation, where SAM is the donor of methyl groups [35, 41, 42]. Hence, SAM will affect methylation as a methyl donor. But the research of Anier et al. [43] deemed that SAM only affected the expression of a limited number of genes and did not affect the vast majority of the genome, suggesting that SAM treatments, despite their global nature, do not result in a general silencing of gene expression. Thus, exogenous SAM treatment induces minor effects on whole-genome gene expression [43].
Some studies have demonstrated that the methylation levels of patients with AD continuously decrease with increasing age [44–46]. In addition, the methyl group is catalyzed by a donor substrate SAM to an adenosine residue of an RNA moiety along a specific sequence [47]. N6-methyladenosine (m6A), the addition of methyl groups to adenine residues, is the most abundant and is prevalent in brain tissue [47]. m6A modification is involved in various RNA mechanisms, most notably RNA stability and translational efficiency [48, 49]. Brain processes affected by m6A methylation may be involved in cognitive functions, including learning and memory, neurogenesis, neurodevelopment, stress response, myelination, and axon plasticity [8]. Moreover, it has been shown that enhanced memory retention of m6A plays a critical role in memory formation and consolidation [50, 51]. However, the results of the total meta-analysis showed that cognitive function was not significantly improved after SAM supplementation. We speculated that this was due to pathological changes of the participants developing into an irreversible stage or a small sample size. Although the specific mechanisms are yet to be fully elucidated, SAM levels can influence DNA/RNA methylation differently, which in turn affects cognitive levels.
In subgroup analyses, SAM intervention could significantly improve the cognitive function of animals when they were ≤8 weeks of age, which is probably because SAM can promote the development of cognition in mice or rats and prevent age-related cognitive decline [52]. Additionally, age is the greatest risk factor for AD [53]. The incidence of cognitive decline increases with increasing age. It has been confirmed that the pathological changes associated with ageing are similar to those of AD, including loss of brain size and weight, microglial degeneration, and breakdown of white matter fiber bundles [54].
Moreover, our subgroup analyses showed that when the SAM intervention time was >8 weeks, the beneficial effect on animals’ cognitive function was more obvious. This is consistent with previous studies [55, 56], which found that the longer the duration, the better the intervention. Thus, this finding may be related to the pathogenesis of AD. In the late stage of AD, synaptic receptors are reduced; protein degeneration and inflammation, intracellular nerve fiber tangles, and excessive phosphorylation are irreversible pathological changes that lead to gradual cognitive decline, making it difficult to reverse this process [57]. Therefore, early intervention is likely to be more effective. In animal studies, the reliability of the results was reduced because of the heterogeneity of the included studies. Hence, more rigorous experiments are required to determine the effects of SAM on cognition.
SAM did not improve cognition in animals, but when the cognitive test results were analyzed in subgroups, SAM supplementation significantly improved the animals’ cognition. Compared with the control group, MWM tests were more favorable in supporting SAM supplementation in improving cognition, but the results were inconsistent in the test of NOR. The MWM test measures spatial learning and memory, particularly long-term memory, through escape latency and the target quadrant residence time. The NOR detects whether animals have recognition defects [58]. This is consistent with a meta-analysis concluded that SAM supplementation enhanced spatial memory in patients with dementia [59]. Based on this, we inferred that SAM could promote spatial learning and memory abilities instead of animal object recognition. Regarding the subgroups in human studies based on cognitive domains and scales, the effects of SAM on global cognition were more significant than those on cognitive domains. Therefore, we speculate that the cognitive tests are more sensitive to cognitive changes.
However, the meta-analysis of the included human studies revealed that SAM did not significantly improve human cognition. This might be because of the small number of human studies included or that the animal phenotypes included differ from those of patients with AD, representing only a partial AD model and not revealing AD’s full pathology [60]. Meanwhile, various interference factors in human research and the unique social attributes of humans can also lead to different results in human and animal studies. Therefore, further studies are required to comprehensively analyze the effects of SAM on cognition in animal and human studies.
With a continuous increase in the degree of ageing, the incidence of chronic neurodegenerative disease is increasing, and cognitive decline is the primary functional disorder. This systematic review and meta-analysis aimed to explore the effects of SAM on cognitive decline. Therefore, this provides novel ideas for interventions to improve cognition. Moreover, conducting further clinical experiments and meta-analyses on the clinical control experiments is warranted.
Our study had certain strengths: a) We included only randomized controlled trials in the meta-analysis to improve the accuracy and reliability of the studies; b) Five databases were thoroughly searched to include a large sample and results from multiple measures of cognition and subgroups; and c) The selection and quality assessments of this meta-analysis were performed independently by two researchers, ensuring strict quality control during the study process, including data collection.
However, there were some limitations to the meta-analysis. First, participants were included with different types of diseases, and animal studies used various models of cognitive impairment, making the results less reliable. Second, in most studies, digital software was used to collect data, implying that errors may exist due to differences between the data obtained from the software and the original data. Third, most of the included human studies supplemented SAM in the form of a nutrient mixture, and we cannot be sure that the improvement was due to the total effect of SAM. In addition, human cognitive function evaluation lacks consistency and objectivity, necessitating more accurate methods. Furthermore, because of the included studies did not note the specific dose of SAM per kg body weight in animals, and we also did not describe this content. Finally, due to the limitations of our included literature, any culturing of healthy neurons in presence of various doses of SAM and their effect on overall epigenetics were not mentioned, which would be a limitation of the paper.
The results of our qualitative analyses support the idea that SAM supplementation can effectively improve cognition in animals and humans. However, our meta-analysis revealed that SAM supplementation does not significantly improve cognitive performance in animals or humans. Subgroup analyses of animal studies showed that age ≤8 weeks and intervention duration >8 weeks significantly improved spatial learning and memory. Therefore, the efficacy of SAM supplements should be interpreted with caution before definitive conclusions can be drawn, which will require further studies and broader clinical investigations.
ACKNOWLEDGMENTS
We are grateful to the National Natural Science Foundation of China for their financial support. Furthermore, we genuinely appreciate the hard work from the distinguished editor and reviewers.
FUNDING
This work was supported by the National Natural Science Foundation of China (82171582), the Science and Technology Research Project of Higher Education Institutions in Hebei Province (ZD2020105), and the Medical Science Research Project of Hebei Province (20220976).
PROSPERO registration number: CRD42022316443
CONFLICT OF INTEREST
The authors have no conflict of interest to report.
DATA AVAILABILITY
The data supporting the findings of this study are available within the article and/or its supplementary material.
SUPPLEMENTARY MATERIAL
[1] The supplementary material is available in the electronic version of this article: https://dx.doi.org/10.3233/JAD-221076.
REFERENCES
[1] | Elena Gomez-Gomez M , Zapico SC ((2019) ) Frailty, cognitive decline, neurodegenerative diseases and nutrition interventions. Int J Mol Sci 20: , 2842. |
[2] | Dominguez LJ , Veronese N , Vernuccio L , Catanese G , Inzerillo F , Salemi G , Barbagallo M ((2021) ) Nutrition, physical activity, and other lifestyle factors in the prevention of cognitive decline and dementia. Nutrients 13: , 4080. |
[3] | Dumurgier J , Sabia S ((2020) ) Epidemiology of Alzheimer’s disease: Latest trends. Rev Prat 70: , 149–151. |
[4] | Matej R , Tesar A , Rusina R ((2019) ) Alzheimer’s disease and other neurodegenerative dementias in comorbidity: A clinical and neuropathological overview. Clin Biochem 73: , 26–31. |
[5] | Cantoni GL ((1953) ) S-Adenosylmethionine; a new intermediate formed enzymatically from L-methionine and adenosinetriphosphate. J Biol Chem 204: , 403–416. |
[6] | Chan A , Remington R , Kotyla E , Lepore A , Zemianek J , Shea TB ((2010) ) A vitamin/nutriceutical formulation improves memory and cognitive performance in community-dwelling adults without dementia. J Nutr Health Aging 14: , 224–230. |
[7] | Ulmer DD , Vallee BL ((1965) ) Extrinsic cotton effects and the mechanism of enzyme action. Adv Enzymol Relat Areas Mol Biol 27: , 37–104. |
[8] | de Leeuw FA , van der Flier WM , Tijms BM , Scheltens P , Mendes VM , Manadas B , Bierau J , van Wijk N , van den Heuvel EGHM , Mohajeri MH , Teunissen CE , Kester MI ((2020) ) Specific nutritional biomarker profiles in mild cognitive impairment and subjective cognitive decline are associated with clinical progression: The NUDAD Project. J Am Med Dir Assoc 21: , 1513.e1–1513.e17. |
[9] | Strous RD , Ritsner MS , Adler S , Ratner Y , Maayan R , Kotler M , Lachman H , Weizman A ((2009) ) Improvement of aggressive behavior and quality of life impairment following S-Adenosyl-Methionine (SAM-e) augmentation in schizophrenia. Eur Neuropsychopharmacol 19: , 14–22. |
[10] | Cavallaro RA , Fuso A , Nicolia V , Scarpa S ((2010) ) S-Adenosylmethionine prevents oxidative stress and modulates glutathione metabolism in TgCRND8 mice fed a B-vitamin deficient diet. J Alzheimers Dis 20: , 997–1002. |
[11] | Page MJ , McKenzie JE , Bossuyt PM , Boutron I , Hoffmann TC , Mulrow CD , Shamseer L , Tetzlaff JM , Akl EA , Brennan SE , Chou R , Glanville J , Grimshaw JM , Hrobjartsson A , Lalu MM , Li T , Loder EW , Mayo-Wilson E , McDonald S , McGuinness LA , Stewart LA , Thomas J , Tricco AC , Welch VA , Whiting P , Moher D ((2021) ) The PRISMA 2020 statement: An updated guideline for reporting systematic reviews. J Clin Epidemiol 134: , 178–189. |
[12] | Sterne JAC , Savovic J , Page MJ , Elbers RG , Blencowe NS , Boutron I , Cates CJ , Cheng H-Y , Corbett MS , Eldridge SM , Emberson JR , Hernan MA , Hopewell S , Hrobjartsson A , Junqueira DR , Juni P , Kirkham JJ , Lasserson T , Li T , McAleenan A , Reeves BC , Shepperd S , Shrier I , Stewart LA , Tilling K , White IR , Whiting PF , Higgins JPT ((2019) ) RoB 2: A revised tool for assessing risk of bias in randomised trials. BMJ 366: , l4898. |
[13] | Hooijmans CR , Rovers MM , de Vries RBM , Leenaars M , Ritskes-Hoitinga M , Langendam MW ((2014) ) SYRCLE’s risk of bias tool for animal studies. BMC Med Res Methodol 14: , 43. |
[14] | Atkins D , Best D , Briss PA , Eccles M , Falck-Ytter Y , Flottorp S , Guyatt GH , Harbour RT , Haugh MC , Henry D , Hill S , Jaeschke R , Leng G , Liberati A , Magrini N , Mason J , Middleton P , Mrukowicz J , O’Connell D , Oxman AD , Phillips B , Schünemann HJ , Edejer T , Varonen H , Vist GE , Williams JW Jr , Zaza S ; GRADE Working Group ((2004) ) Grading quality of evidence and strength of recommendations. BMJ 328: , 1490. |
[15] | Borenstein M , Hedges LV , Higgins JPT , Rothstein HR ((2010) ) A basic introduction to fixed-effect and random-effects models for meta-analysis. Res Synth Methods 1: , 97–111. |
[16] | Higgins JPT , Thompson SG , Deeks JJ , Altman DG ((2003) ) Measuring inconsistency in meta-analyses. BMJ 327: , 557–560. |
[17] | Beauchamp LC , Liu XM , Sedjahtera A , Bogeski M , Vella LJ , Bush AI , Adlard PA , Barnham KJ ((2020) ) S-Adenosylmethionine rescues cognitive deficits in the rTg4510 animal model by stabilizing protein phosphatase 2A and reducing phosphorylated tau. J Alzheimers Dis 77: , 1705–1715. |
[18] | Cao XJ , Huang SH , Wang M , Chen JT , Ruan DY ((2008) ) S-adenosyl-L-methionine improves impaired hippocampal long-term potentiation and water maze performance induced by developmental lead exposure in rats. Eur J Pharmacol 595: , 30–34. |
[19] | Chan A , Tchantchou F , Graves V , Rozen R , Shea TB ((2008) ) Dietary and genetic compromise in folate availability reduces acetylcholine, cognitive performance and increases aggression: Critical role of S-adenosyl methionine. J Nutr Health Aging 12: , 252–261. |
[20] | Shea TB ((2007) ) Effects of dietary supplementation with N-acetyl cysteine, acetyl-L-carnitine and S-adenosyl methionine on cognitive performance and aggression in normal mice and mice expressing human ApoE4. Neuromolecular Med 9: , 264–269. |
[21] | Fuso A , Nicolia V , Ricceri L , Cavallaro RA , Isopi E , Mangia F , Fiorenza MT , Scarpa S ((2012) ) S-adenosylmethionine reduces the progress of the Alzheimer-like features induced by B-vitamin deficiency in mice. Neurobiol Aging 33: , 1482.e1–16. |
[22] | Gregoire S , Millecamps M , Naso L , Do Carmo S , Cuello AC , Szyf M , Stone LS ((2017) ) Therapeutic benefits of the methyl donor S-adenosylmethionine on nerve injury-induced mechanical hypersensitivity and cognitive impairment in mice. Pain 158: , 802–810. |
[23] | Tchantchou F , Graves M , Ortiz D , Rogers E , Shea TB ((2004) ) Dietary supplementation with 3-deaza adenosine, N-acetyl cysteine, and S-adenosyl methionine provide neuroprotection against multiple consequences of vitamin deficiency and oxidative challenge: Relevance to age-related neurodegeneration. Neuromolecular Med 6: , 93–103. |
[24] | Tillmann S , Happ DF , Mikkelsen PF , Geisel J , Wegener G , Obeid R ((2019) ) Behavioral and metabolic effects of S-adenosylmethionine and imipramine in the Flinders Sensitive Line rat model of depression. Behav Brain Res 364: , 274–280. |
[25] | Wan X , Ma B , Wang X , Guo C , Sun J , Cui J , Li L ((2020) ) S-Adenosylmethionine alleviates amyloid-beta-induced neural injury by enhancing trans-sulfuration pathway activity in astrocytes. J Alzheimers Dis 76: , 981–995. |
[26] | Chan A , Paskavitz J , Remington R , Rasmussen S , Shea TB ((2008) ) Efficacy of a vitamin/nutriceutical formulation for early-stage Alzheimer’s disease: A 1-year, open-label pilot study with a 16-month caregiver extension. Am J Alzheimers Dis Other Demen 23: , 571–585. |
[27] | Levkovitz Y , Alpert JE , Brintz CE , Mischoulon D , Papakostas GI ((2012) ) Effects of S-adenosylmethionine augmentation of serotonin-reuptake inhibitor antidepressants on cognitive symptoms of major depressive disorder. J Affect Disord 136: , 1174–1178. |
[28] | Remington R , Chan A , Paskavitz J , Shea TB ((2009) ) Efficacy of a vitamin/nutriceutical formulation for moderate-stage to later-stage Alzheimer’s disease: A Placebo-controlled Pilot Study. Am J Alzheimers Dis Other Demen 24: , 27–33. |
[29] | Remington R , Bechtel C , Larsen D , Samar A , Doshanjh L , Fishman P , Luo Y , Smyers K , Page R , Morrell C , Shea TB ((2015) ) A Phase II randomized clinical trial of a nutritional formulation for cognition and mood in Alzheimer’s disease. J Alzheimers Dis 45: , 395–405. |
[30] | Remington R , Lortie JJ , Hoffmann H , Page R , Morrell C , Shea TB ((2015) ) A nutritional formulation for cognitive performance in mild cognitive impairment: A placebo-controlled trial with an open-label extension. J Alzheimers Dis 48: , 591–595. |
[31] | Remington R , Bechtel C , Larsen D , Samar A , Page R , Morrell C , Shea TB ((2016) ) Maintenance of cognitive performance and mood for individuals with Alzheimer’s disease following consumption of a nutraceutical formulation: A one-year, open-label study. J Alzheimers Dis 51: , 991–995. |
[32] | Bottiglieri T ((2002) ) S-Adenosyl-L-methionine (SAMe): From the benchto the bedside–molecular basis of a pleiotrophic molecule. AmJ Clin Nutr 76: , 1151S–1157S. |
[33] | Francioso A , Fanelli S , D’Erme M , Lendaro E , Miraglia N , Fontana M , Cavallaro RA , Mosca L ((2021) ) Pharmacokinetic properties of a novel formulation of S-adenosyl-l-methionine phytate. Amino Acids 53: , 1559–1568. |
[34] | Shima H , Matsumoto M , Ishigami Y , Ebina M , Muto A , Sato Y , Kumagai S , Ochiai K , Suzuki T , Igarashi K ((2017) ) S-Adenosylmethionine synthesis is regulated by selective N-6-adenosine methylation and mRNA degradation involving METTL16 and YTHDC1. Cell Rep 21: , 3354–3363. |
[35] | Qazi TJ , Quan Z , Mir A , Qing H ((2018) ) Epigenetics in Alzheimer’s disease: Perspective of DNA methylation. Mol Neurobiol 55: , 1026–1044. |
[36] | Blanch M , Luis Mosquera J , Ansoleaga B , Ferrer I , Barrachina M ((2016) ) Altered mitochondrial DNA methylation pattern in Alzheimer disease-related pathology and in Parkinson disease. Am J Pathol 186: , 385–397. |
[37] | Han M , Liu Z , Xu Y , Liu X , Wang D , Li F , Wang Y , Bi J ((2020) ) Abnormality of m6A mRNA methylation is involved in Alzheimer’s disease. Front Neurosci 14: , 98. |
[38] | Li T , Wei Y , Qu M , Mou L , Miao J , Xi M , Liu Y , He R ((2021) ) Formaldehyde and de/methylation in age-related cognitive impairment. Genes 12: , 913. |
[39] | Wong M , Gertz B , Chestnut BA , Martin LJ ((2013) ) Mitochondrial DNMT3A and DNA methylation in skeletal muscle and CNS of transgenic mouse models of ALS. Front Cell Neurosci 7: , 279. |
[40] | Xu Y , Xu L , Han M , Liu X , Li F , Zhou X , Wang Y , Bi J ((2019) ) Altered mitochondrial DNA methylation and mitochondrial DNA copy number in an APP/PS1 transgenic mouse model of Alzheimer disease. Biochem Biophys Res Commun 520: , 41–46. |
[41] | Lan J , Hua S , He X , Zhang Y ((2010) ) DNA methyltransferases and methyl-binding proteins of mammals. Acta Biochim Biophys Sin 42: , 243–252. |
[42] | MacDonald JL , Roskams AJ ((2009) ) Epigenetic regulation of nervous system development by DNA methylation and histone deacetylation. Prog Neurobiol 88: , 170–183. |
[43] | Anier K , Zharkovsky A , Kalda A ((2013) ) S-adenosylmethionine modifies cocaine-induced DNA methylation and increases locomotor sensitization in mice. Int J Neuropsychopharmacol 16: , 2053–2066. |
[44] | Bernstein AI , Lin Y , Street RC , Lin L , Dai Q , Yu L , Bao H , Gearing M , Lah JJ , Nelson PT , He C , Levey AI , Mulle JG , Duan R , Jin P ((2016) ) 5-Hydroxymethylation-associated epigenetic modifiers of Alzheimer’s disease modulate Tau-induced neurotoxicity. Hum Mol Genet 25: , 2437–2450. |
[45] | Shu L , Sun W , Li L , Xu Z , Lin L , Xie P , Shen H , Huang L , Xu Q , Jin P , Li X ((2016) ) Genome-wide alteration of 5-hydroxymenthylcytosine in a mouse model of Alzheimer’s disease. BMC Genomics 17: , 381. |
[46] | Wang SC , Oelze B , Schumacher A ((2008) ) Age-specific epigenetic drift in late-onset Alzheimer’s disease. PLoS One 3: , e2698. |
[47] | Bokar JA , Shambaugh ME , Polayes D , Matera AG , Rottman FM ((1997) ) Purification and cDNA cloning of the AdoMet-binding subunit of the human mRNA (N6-adenosine)-methyltransferase. RNA 3: , 1233–1247. |
[48] | Chen XY , Zhang J , Zhu JS ((2019) ) The role of m(6)A RNA methylation in human cancer. Mol Cancer 18: , 103. |
[49] | Meyer KD , Patil DP , Zhou J , Zinoviev A , Skabkin MA , Elemento O , Pestova TV , Qian SB , Jaffrey SR ((2015) ) 5 ’ UTR m(6)A promotes cap-independent translation. Cell 163: , 999–1010. |
[50] | Walters BJ , Mercaldo V , Gillon CJ , Yip M , Neve RL , Boyce FM , Frankland PW , Josselyn SA ((2017) ) The role of the RNA demethylase FTO (Fat Mass and Obesity-Associated) and mRNA methylation in hippocampal memory formation. Neuropsychopharmacology 42: , 1502–1510. |
[51] | Widagdo J , Zhao QY , Kempen MJ , Tan MC , Ratnu VS , Wei W , Leighton L , Spadaro PA , Edson J , Anggono V , Bredy TW ((2016) ) Experience-dependent accumulation of N-6-methyladenosine in the prefrontal cortex is associated with memory processes in mice. J Neurosci 36: , 6771–6777. |
[52] | Blusztajn JK , Slack BE , Mellott TJ ((2017) ) Neuroprotective actions of dietary choline. Nutrients 9: , 815. |
[53] | Armstrong RA ((2019) ) Risk factors for Alzheimer’s disease. Folia Neuropathol 57: , 87–105. |
[54] | Soria Lopez JA , Gonzalez HM , Leger GC ((2019) ) Alzheimer’s disease. Handb Clin Neurol 167: , 231–255. |
[55] | Doraiswamy PM , Krishnan KRR , Anand R , Sohn H , Danyluk J , Hartman RD , Veach J ((2002) ) Long-term effects of rivastigmine in moderately severe Alzheimer’s disease: Does early initiation of therapy offer sustained benefits? Prog Neuropsychopharmacol Biol Psychiatry 26: , 705–712. |
[56] | Farlow M , Anand R , Messina J Jr , Hartman R , Veach J ((2000) ) A 52-week study of the efficacy of rivastigmine in patients with mild to moderately severe Alzheimer’s disease. Eur Neurol 44: , 236–241. |
[57] | Brewer GJ ((2010) ) Why vitamin E therapy fails for treatment of Alzheimer’s disease. J Alzheimers Dis 19: , 27–30. |
[58] | Linh My Thi L , Mai Thanh Thi N , Hai Xuan N , Phu Hoang D , Nhan Trung N , Hung Manh T , Hoa Thi N , Nui Minh N , Min BS , Kim JA , Choi JS , Mao Van C ((2016) ) Anti-cholinesterases and memory improving effects of Vietnamese Xylia xylocarpa. Chem Cent J 10: , 48. |
[59] | Montgomery SE , Sepehry AA , Wangsgaard JD , Koenig JE ((2014) ) the effect of S-Adenosylmethionine on cognitive performance in mice: An animal model meta-analysis. PLoS One 9: , e107756. |
[60] | Nakai T , Yamada K , Mizoguchi H ((2021) ) Alzheimer’s disease animal models: Elucidation of biomarkers and therapeutic approaches for cognitive impairment. Int J Mol Sci 22: , 5549. |