Unlocking Modifiable Risk Factors for Alzheimer’s Disease: Does the Oral Microbiome Hold Some of the Keys?
Abstract
Advancing age is recognized as the primary risk factor for Alzheimer’s disease (AD); however approximately one third of dementia cases are attributable to modifiable risk factors such as hypertension, diabetes, smoking, and obesity. Recent research also implicates oral health and the oral microbiome in AD risk and pathophysiology. The oral microbiome contributes to the cerebrovascular and neurodegenerative pathology of AD via the inflammatory, vascular, neurotoxic, and oxidative stress pathways of known modifiable risk factors. This review proposes a conceptual framework that integrates the emerging evidence regarding the oral microbiome with established modifiable risk factors. There are numerous mechanisms by which the oral microbiome may interact with AD pathophysiology. Microbiota have immunomodulatory functions, including the activation of systemic pro-inflammatory cytokines. This inflammation can affect the integrity of the blood-brain barrier, which in turn modulates translocation of bacteria and their metabolites to brain parenchyma. Amyloid-β is an antimicrobial peptide, a feature which may in part explain its accumulation. There are microbial interactions with cardiovascular health, glucose tolerance, physical activity, and sleep, suggesting that these modifiable lifestyle risk factors of dementia may have microbial contributors. There is mounting evidence to suggest the relevance of oral health practices and the microbiome to AD. The conceptual framework presented here additionally demonstrates the potential for the oral microbiome to comprise a mechanistic intermediary between some lifestyle risk factors and AD pathophysiology. Future clinical studies may identify specific oral microbial targets and the optimum oral health practices to reduce dementia risk.
INTRODUCTION
Dementia represents the leading cause of disability in older people. Alzheimer’s disease (AD) is the most common cause of dementia, accounting for up to 70% of cases [1]. Following disappointing failed drug trials based on the amyloid hypothesis, and a recently approved treatment with uncertain clinical efficacy [2], there are ever louder calls to seek other lines of enquiry such as inflammatory, immune, and microbial mechanisms [3, 4]. Research leading to novel preventative and early intervention opportunities is critical since the disease process begins several decades prior to symptom onset [5]. As identified through the 2020 Lancet Commission into dementia, modifiable risk factors are considered to contribute to up to 40% of dementia risk, with obesity, hypertension, and hearing loss representing important midlife dementia risk factors and education playing a role earlier in life [6]. Later life risk factors include smoking, physical inactivity, diabetes, depression, and social isolation [6]. Whilst the Lancet Commission considers the role of head injury, excessive alcohol intake, and air pollution, observational studies also suggest that diet and sleep are probable modifiers of dementia risk [7]. At the same time, the role of oral disease and perturbation of the oral microbiome in neurodegenerative disease is being uncovered [8]. It is unlikely that these factors occur in isolation, and indeed lifestyle factors are deeply intertwined with the role of the gut microbiome in health and disease [9]. However, exactly how and to what degree lifestyle risk and protective factors relate to the oral microbiome in the context of AD has not been investigated.
In this article, we propose a conceptual framework in which the oral microbiome is a plausible causal intermediary between several of the lifestyle factors known to modify AD risk. Some interactions between lifestyle factors and the oral microbiome area likely to be direct and causal. Cardiometabolic factors (e.g., diabetes mellitus, obesity, hypertension) in particular have established causal effects and bidirectional interactions with the oral microbiome and correspondingly, to oral health and disease [10, 11]. The interactions between other lifestyle factors and the oral microbiome are better characterized indirect or due to shared ‘upstream’ risk factors (e.g., psychosocial factors, education level, and depression). Here we intend to synthesize the evidence for both direct and indirect interactions between lifestyle and oral microbiome-related factors to provide new insights into the modifiable drivers of AD. In doing so, we highlight gaps in research and future opportunities that may lead to novel avenues for prevention and early intervention.
AN OVERVIEW OF AD PATHOPHYSIOLOGY
AD and AD related dementias (AD/ADRD) represent the most common forms of dementia and include frontotemporal dementia, Lewy body dementia, vascular contributions to cognitive impairment and dementia, and mixed etiology dementias. The disease process contributing to AD is multifactorial and pathology overlaps with the ADRD subtypes. The neurotoxic pathologies key to AD are amyloid-β peptide (Aβ) plaques and neurofibrillary tangles (NFTs) of tau protein. Aβ pathology is caused by improper cleavage of the amyloid-β protein precursor resulting in the aggregation of Aβ fibrils and plaques, particularly in the medial temporal lobe [12]. Failure to clear Aβ is an important cause of AD and consequences of Aβ build up include neuronal degeneration, neuroinflammation, oxidative stress, microglia activation and dysfunction of the blood-brain barrier [13, 14]. Hyperphosphorylation of tau results in the formation of NFTs. The accumulation of NFTs in turn leads to loss of neuronal function and apoptosis [12]. Systemic inflammation, caused by an imbalance of anti-inflammatory and pro-inflammatory signaling is attributed to activated microglia cells and the release of cytokines [12]. These processes are thought to participate in a positive feedback loop of Aβ deposition, formation of NFTs, and synaptic and neuronal damage [13].
In AD, dysfunctional glucose metabolism in the brain results from Aβ-induced oxidative stress and leads to synaptic dysfunction and apoptosis [15]. Indeed, AD has been dubbed ‘type 3 diabetes’ by some [16, 17] due to the effects of impaired glucose tolerance on pathophysiological features of AD, including insulin growth factor signaling, oxidative stress, acetylcholine esterase activity regulation, Aβ formation, and NFT formation [18].
Lipid peroxidation, protein oxidation, and DNA oxidation are also involved in AD pathogenesis [19]. Oxidative stress disrupts membrane integrity, and damages Aβ, while Aβ deposition results in the further generation of reactive oxygen species [20]. Oxidative stress contributes to neurovascular dysfunction in AD. Free radicals induce inflammation and endothelial dysfunction, promoting vascular leakage and cytokine production [21].
Small vessel disease, microvascular injury, and microscopic lesions are common in AD [22]. Aβ is a powerful vasoconstrictor and can suppress endothelium-dependent responses, blood flow, and cerebrovascular autoregulation [21]. As compared to AD, vascular dementia is caused by impaired cerebral blood flow, resulting in hypoxia and dysfunctional blood-brain barrier permeability [14]. However, primary vascular pathologies also contribute to the development of AD dementia and the two conditions can be difficult to differentiate. Mixed pathology including cerebrovascular infarcts have been identified in close to half of cases with clinically probable AD dementia [23]. The neuropathological underpinnings of (clinically diagnosed) dementia due to presumed AD exists on a spectrum of vascular causes to AD causes, with most showing signs of mixed pathology [22].
In terms of other ADRD categories, the key defining characteristic of Lewy body dementia is neurodegeneration due to the abnormal accumulation of α-synuclein [24], and frontotemporal dementia is classified by atrophy to frontal and/or temporal lobes with clinical changes to personality and/or language function [25]. A more recently defined ADRD category is primary age-related tauopathy (PART), which shares the tau deposits and temporal lobe neurodegeneration seen in AD, but without the Aβ accumulation. PART is associated with a slower rate of cognitive decline than AD [26]. Limbic predominant age-related TDP-43 encephalopathy (LATE) shows stereotypical TDP-43 proteinopathy, with or without coexisting hippocampal sclerosis pathology and is most common in people over the age of 80 [27]. Due to similarities with the clinical symptoms of AD, 15–20% of clinically diagnosed AD cases could be attributable to LATE [28]. Therefore, an important consideration for studies which have not defined dementia subtype or defined AD based on clinical presentation alone is that some level of heterogeneity will exist with regard to underlying pathology within the category of ADRD. In order to consider differences in the underlying pathology, we use the term ‘AD dementia’ to refer to cases of clinically probable AD in the reviewed literature where possible, and ‘dementia’ where the studies have not specified the underlying pathology.
THE ORAL MICROBIOME AND AD
A number of pathways contribute to neurological disease, including the gut microbiome, which has seen significant recent interest with respect to relevance to AD and cognition [29, 30]. However, there is a growing rationale from epidemiological and experimental research which implicates the microbiome from sites external to the gut, in particular the oral cavity, in the pathogenesis of neurodegenerative disorders [31].
The oral cavity contains distinct microbial communities including in the oral mucosa, tongue, plaque, cheek, and gingiva, which collectively house over 700 prevalent bacteria, some 57% of which have been formally identified [32]. In healthy individuals, these microbes comprise mostly bacteria, with a smaller abundance of resident fungi, archea, and viruses [32]. While saliva has no indigenous microbes, bacteria from other oral surfaces are transferred to saliva and its composition is dominated by Streptococcus, Veilonella, and Prevotella [31].
As in human microbiomes of other niches, there is relative stability in the oral microbiome of an adult with good oral health [31]. The development of common oral diseases, such as caries and gum (periodontal) disease, is caused by members of the oral microbiome and typified by dysbiosis across the whole oral microial community [36]. Independent of oral health state, there are changes in the oral microbiome makeup across the life-course due to key milestones, such as birth [32], early feeding practices [33], the emergence of teeth, later dietary changes, lifestyle factors including smoking [34] and hormonal changes, such as during pregnancy [35]. These events see the gradual enrichment in diversity of the oral microbiome, from a narrow diversity ecosystem, dominated by Streptococcus, Veillonella, and Lactobacillus species in infancy [33], to one which has wider diversity in early adulthood, typified by an increased abundance of periodontal disease associated taxa, such as Porphymonas, Actinomyces, and Neisseria, in addition to Fusobacterium nucleatum [34]. Advancing age in adulthood, independent of AD, is generally associated with an increase in dysbiosis [35] in the oral microbiome, with an increased abundance of bacteria associated with periodontal disease [36], in addition to fungal species, Candida Albicans [37], which causes oral thrush. This increased disease potential oral environment in older age is driven by reduced production and quality of saliva, which hampers the buffering capacity of the oral environment and lessens oral microbial clearance [36], in addition to declining nutritional quality [37], which reduces diversity of available macronutrients and is driven by reduced masticatory function, tooth loss, and potentially impaired taste perception [38].
As we will describe in the following section, the oral microbiome has important immune interactions which are distinct from those of the gut microbiome [39]. However, the two sites are not entirely independent; while oral microbes are considered poor colonizers of the intestine, there is some evidence of microbial transfer from mouth to gut. An overlap of approximately half of bacterial species has been shown in oral and gut microbiota in healthy participants contributing to the Human Microbiome Project and in a study of patients with liver cirrhosis [40, 41]. Indeed, the ‘ectopic’ translocation of bacteria from oral cavity to large intestine via swallowed saliva represents a potential mechanism for observed links between periodontal and systemic diseases, in addition to the more direct route of translocation via bacteremia and systemic inflammation [42]. This hypothesis has been supported by evidence that the principal bacteria causing chronic periodontitis, Porphyromonas gingivalis (P. gingivalis) is associated with orodigestive cancers [43]. This same bacteria has been detected in circulating leukocytes and in aortic tissues, where it is thought to have pro-atherogenic effects [44].
Relevance of oral microbiome to AD
Converging evidence for the relevance of the oral microbiome to AD comes from epidemiological, postmortem, and pre-clinical studies. Large, well-designed epidemiological studies have demonstrated prospective associations between gum (periodontal) disease and tooth loss during early adulthood and subsequent diagnosis of AD dementia. For example, in studies of differential AD incidence in identical twins [45] and cohabiting nuns [46], measures of dental health and tooth loss were some of the few modifiable factors predicting AD onset in later life. Clinically apparent oral disease (caries and periodontitis) as well as serum antibodies are more prevalent in AD than in the healthy population [47, 48].
Postmortem studies show seven times greater load of the oral spirochetes bacteria [49] as well as lipopolysaccharides specific to oral bacteria P. gingivalis in the neural tissue of people with AD than in healthy control tissue [50], demonstrating bacterial translocation into the brain. Data from the large NHANES-III study showed that increased abundance of oral P. gingivalis IgG antibody was negatively associated with short-memory and verbal recall function in older adults [51]. Data from the same cohort found clear associations between oral health and cognitive function across adulthood, as well as P. gingivalis antibodies and incident AD and mortality [52, 53]. Serum antibodies for these periodontal disease bacteria are detectable at an elevated level in AD patients [54], while their abundance has also been shown to predict conversion to mild cognitive impairment and AD after a follow-up period of more than a decade [55].
There is also a strong suggestion of interaction between the AD process and the host microbiome from studies of mice genetic variants bred to exhibit an AD phenotype, which have delayed disease onset if raised in a germ-free setting [56], though this finding is not specific to microbes in the oral cavity. For more details, see two recent, comprehensive reviews of this literature [31, 47].
Mechanistic interface between the oral microbiome and AD pathophysiology
The oral microbiome, in particular in association with periodontal disease, has demonstrated relevance to core features of AD neuropathology, including neuroinflammation, Aβ deposition, neurofibrillary tau protein, and the resulting neuronal dysfunction and cortical atrophy. Here we outline the evidence showing that mouth to brain interactions act on the key mechanisms underpinning lifestyle factors in AD across inflammatory, neurotoxic, oxidative stress, and vascular pathways (see Fig. 1). These pathways are not mutually exclusive, but deeply interactive, and reflect both direct and indirect interactions between microbes and central nervous system functioning.
Fig. 1
Conceptual framework in which the oral microbiome is a plausible causal intermediary between established lifestyle factors and AD risk, via vascular, inflammatory/immune, neurotoxic, and glucose metabolism pathways. The weight of the connecting lines reflect the strength of evidence linking the lifestyle factors to the oral microbiome, and we propose that oral health and hygiene warrants consideration as an additional risk modifier.
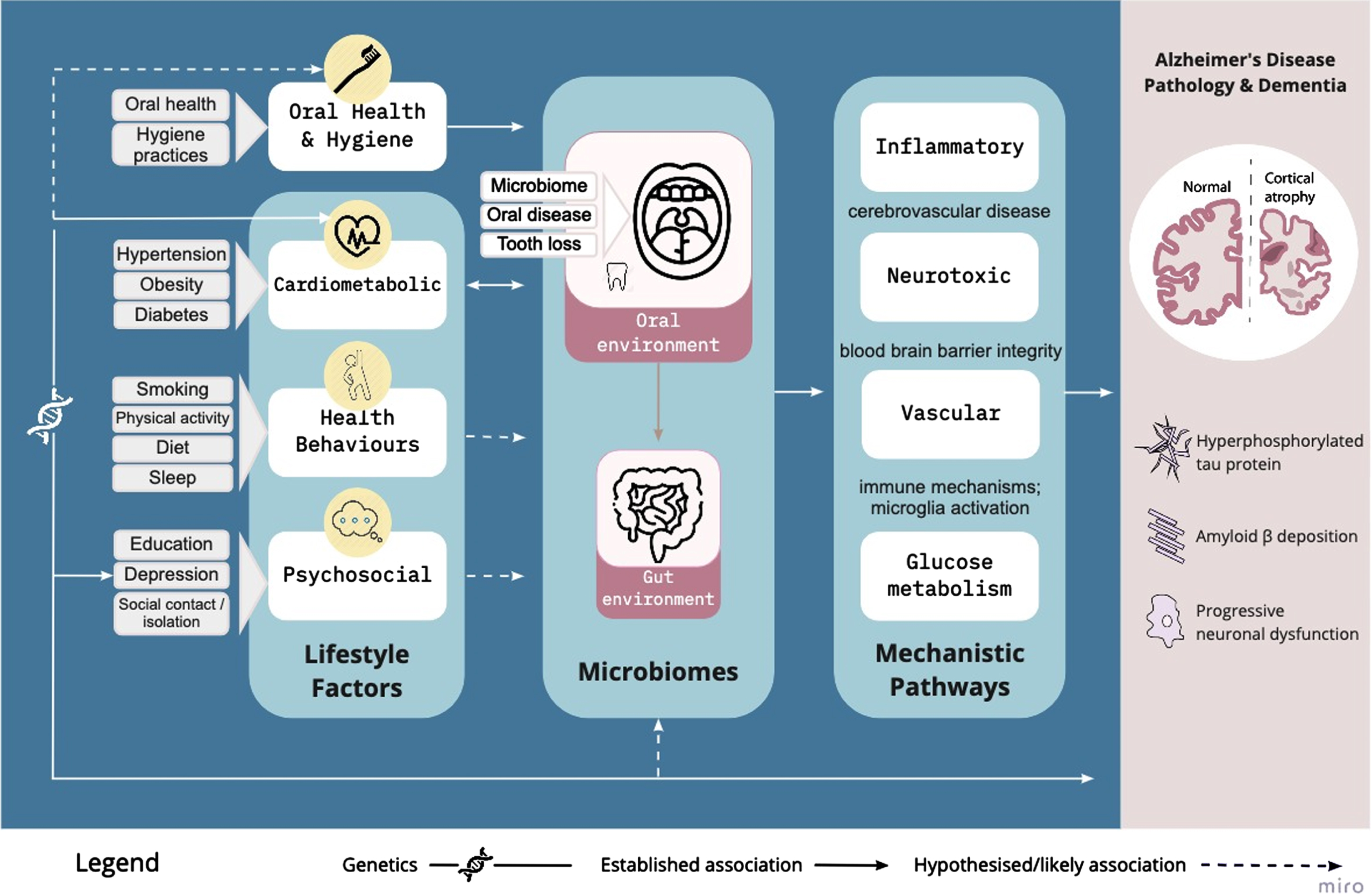
Physical routes of entry enable oral microbial transfer to the brain, including via the trigeminal nerve, and olfactory system, to link the oral and nasal cavities to the olfactory bulb in the brain [57]. Indeed, reduced olfaction is one of the early symptoms of AD [47]. The olfactory hypothesis is supported by several replicated findings of oral spirochetes bacteria in both human cerebrospinal fluid and cerebral cortical tissue; evidence which meets Koch’s and Hill’s postulates for causal relationships [49]. Oral to brain translocation of microbes is also demonstrated by high prevalence of DNA of the herpes simplex virus type 1 in the hippocampus and temporal and frontal brain regions in both aging and AD brain tissue, reported in numerous human studies [58]. Once in the brain, these bacteria have a neurotoxic effect, prompting a feedback cycle of neuroinflammation and neurodegeneration [58]. The presence of spirochetes prompts cultured neuronal cells to produce Aβ [59], while cultured neuronal cells exposed to lipopolysaccharide (endotoxins found in the outer layer of Gram-negative bacteria) produce hyperphosphorylated tau [60]. Indeed, Aβ has been posited to have an adaptive role as an antimicrobial peptide, binding to microorganisms and aggregating into oligomers and fibrils to protect cells from infection [61]. Collectively, the impacts of microbial translocation to neural tissue are likely to cause microglial activation, which further perpetuates proinflammatory cytokine production and is known to occur in AD. For a comprehensive review of these mechanisms, see [31].
As to the issue of how periodontitis and oral dysbiosis is causally involved, the translocation of over 150 species of oral bacteria to the systemic circulation, at least 32 species of which also cause infective endocarditis [62], may also contribute to the cycle of neuroinflammatory processes seen in AD. Bacteria can also enter the bloodstream through small tears to gingival crevices occurring during dental surgical procedures, and the regular oral hygiene practices of brushing and flossing, particularly in the context of periodontal disease [63]. The resulting immune response and systemic inflammatory cascade, as indicated by blood-based pro-inflammatory mediators (including IL1β, IL-6, TNFα, and CRP) can in turn weaken the cellular defenses of the blood-brain barrier to further neurotoxic bacterial translocation [47] and contribute to further neuroinflammatory processes, resulting in the increase of Aβ, tauopathy, and oxidative stress [64]. Evidence for increased systemic inflammation following periodontitis has been reported in multiple AD cohorts [65, 66], though one study found a reduced inflammatory response to severe periodontitis in AD patients compared to healthy controls [67], suggesting that further clarification may be required.
While associations between systemic immune response to periodontal pathogens and AD have been identified, a causal link in the absence of translocation of these bacteria to the brain has been difficult to resolve. Recent research into the periodontal disease pathogen and key oral biofilm builder, F. nucleatum, has demonstrated a possible mechanistic link between oral microbes and AD, in the absence of neuronal bacterial translocation [68]. In a mouse model of AD, the brains of mice infected with F. nucleatum compared to controls, displayed higher expression of TNFα and IL-1β genes, and greater amounts of tau proteins, even in the absence of F. nucleatum in the brain tissue. This neuronal inflammatory and neurodegenerative response in the presence of an oral F. nucleatum infection was thought to be mediated by lipopolysaccharides (LPS) on the bacteriums cell wall, with LPS extracted from F. nucleatum causing significant stimulation of microglia activation in vitro. Microglial activation is a central component of the neuroinflammatory cascade leading to cognitive decline in AD [69].
The gut microbiome, which has demonstrated impacts on brain function [70], may also mediate the oral-AD associations via swallowed saliva (as described at the start of this section). The role of the gut microbiome in AD is a separate active area of investigation, with recent reports showing differences in microbial composition in patients compared with age-matched controls [71, 72].
There may also be shared genetic contributions to the oral microbial environment and AD risk. While environmental factors have been shown to have a greater impact than genetics on the microbiome, genetics are also likely to play a role [48, 73]. Variants of the apolipoprotein E (APOE) and methylenetetrahydrofolate reductase (MTHFR) gene contribute to both AD and cardiovascular disease risk [74–76], and have as yet undefined associations with the oral microbiome.
MODIFIABLE LIFESTYLE FACTORS FOR AD AND DEMENTIA: ORAL MICROBIOME INTERACTIONS
In addition to demonstrating interfaces of the oral microbiome with AD, the conceptual framework shown in Fig. 1 proposes that the oral microbiome is strongly influenced by some of the established lifestyle factors for AD risk and is therefore a plausible causal intermediary between these (see Mechanistic Pathways, Fig. 1). As an understudied aspect of the mechanisms underpinning AD lifestyle risk factors to date, the oral microbiome may provide an alternative or additional health promotion target. The following section will outline cardiometabolic, health behavioral, educational, and psychosocial risk factors for and highlight potential interactions with the oral microbiome.
Cardio-metabolic dementia risk factors
A number of potentially modifiable health risk factors for dementia are linked to cerebrovascular and neurodegenerative pathologies which contribute to dementia via vascular, inflammatory, neurotoxic, and oxidative stress pathways [7]. Oral microbial interactions along the vascular pathway to AD risk are also compelling. Periodontal disease, which results from alterations to the oral microbiome, has associations with cardiovascular disorders, atherosclerosis, and diabetes [77]. These associations are thought to be underpinned by acute and chronic inflammation and insulin resistance which can contribute to cardiovascular disease pathogenesis [77, 78]. A recent study from the large Oral Infections, Glucose Intolerance, and Insulin Resistance Study (ORIGINS) dataset has identified an early microbial marker of poor cardiometabolic and periodontal health, further supporting their shared etiology [79].
Type 2 diabetes
Type 2 diabetes mellitus (T2DM) is characterized by hyperglycemia, hyperinsulinemia, and insulin resistance and may lead to a 1.5-fold increases in the risk of developing AD [80]. T2DM-induced cerebrovascular damage contributes to increased risk of vascular dementia as well as mixed pathology observed in AD [81]. Hyperglycemia can lead to advanced protein glycation, mitochondrial dysfunction, and oxidative stress [82]. Insulin receptors are found in the brain where insulin signaling contributes to neuromodulatory actions including synaptogenesis [83]. Insulin resistance in both T2DM and obesity is proposed to contribute to AD neuropathology by increasing inflammation, tau phosphorylation, Aβ deposition, and impeding Aβ clearance [82]. Additional factors including age, education, diabetes duration, glycemic control, and genetic predisposition may contribute to the association between T2DM and dementia [82]. Although considered to be a late life risk factor [6], brain functional changes linked to T2DM have been observed during midlife [84] suggesting T2DM could influence dementia risk decades prior to AD onset.
The oral microbiome is implicated in T2DM. Periodontal disease and the presence of periodontal pathogenic bacteria are independently associated with increased T2DM risk [85], while periodontal antibiotic treatment has the off-target effects of improving glycemic control and reduction of average blood glucose levels (HbA1C) [86, 87]. The relationship between diabetes and periodontal disease is likely to be bidirectional [88]. Systematic reviews and meta-analyses have established that periodontitis increases the risk of the development of diabetes and poor diabetic outcomes [85], and that treatment of periodontitis improves glycemic control, suggesting that pathogenic oral microbes may contribute to the pathogenesis or progression of T2DM [86, 87]. Conversely, a mouse study has shown that diabetes is causal of pathogenic changes to the oral microbiota [89]. In this study, the authors used transfer of oral microbiota to germ-free recipient mice to demonstrate that ‘diabetic’ dysbiotic changes in oral microbial composition are causal of increased inflammatory cytokine mRNA levels and periodontal bone resorption, mediated by the inflammatory marker IL-17. Furthermore, blocking of advanced glycosylated end products, produced in diabetes have been found to reduce TNFα, matrix metalloprotineases, and prediontal bone loss [90].
In humans, Long and colleagues (2017) showed reduced abundance of several bacteria within the Actinobacteria phylum, including genus Actinomyces, in the oral microbiota of an incident type 2 diabetic sample, and odds ratio of 0.27 of having the disease in people with high levels of oral Actinobacteria, suggesting it may have a protective effect [88]. The discrimination in the presence of genus Actinomyces was particularly clear, with 100% presence in diabetes cases and <3% of non-diabetic obese of healthy weight controls. Of course, there are other common risk factors to these non-communicable diseases including smoking and obesity and these may also implicate the oral microbiome [77, 88]. Findings from the ORIGINS indicated that amongst 300 adults free from T2DM, higher abundance of oral nitrate-reducing bacteria was associated with lower insulin resistance, plasma glucose, and systolic blood pressure in those without hypertension [91]. This work indicates that oral nitrate-reducing bacteria may contribute to regulation of insulin resistance, a mechanism relevant to T2DM and other cardiometabolic risk factors for dementia. To date, no studies have attempted to link impaired glucose regulation/T2DM, in combination with periodontitis, to increased risk of AD. More research is required to tease apart the causal directions of these relationships.
Hypertension
T2DM during midlife has a number of overlapping mechanisms with hypertension and obesity (e.g., see [82] for comprehensive review). Hypertension is not only a leading risk factor for atherosclerosis and stroke, hypertension during midlife also accounts for approximately 5% of dementia cases worldwide [92]. Leading to alterations of crucial regulatory components of the cerebral circulation including endothelial dysfunction, hypertension contributes to impairments to blood-brain barrier permeability, cerebrovascular reactivity, and blood flow autoregulation and to neural activation [93]. Hypertension causes damage to neural tissue in the form of microbleeds and macrobleeds leading to small vessel disease, which in turn is responsible for brain lesions and damage to the white matter [93]. Furthermore, hypertension increases Aβ deposition and potentially impairs the vascular clearance of Aβ [93].
Hypertension can be influenced by oral health. Results from the 2009–2014 National Health and Nutrition Examination Survey (NHANES) indicated that among adults using antihypertensive medication, periodontal disease is related to poorer systolic blood pressure control and higher risk of unsuccessful antihypertensive treatment [94]. While the underlying mechanisms are unclear, there is evidence indicating a role for endothelial dysfunction and nitric oxide deficiency in hypertension [95]. This is of relevance to the oral microbiome as nitrate is reduced to nitric oxide through an enterosalivary nitrate-nitrite nitric oxide pathway involving facultative anaerobic bacteria located in the oral cavity [96]. One hypothesis is that disruptions to this pathway, resulting in low nitric oxide availability, may occur due to diet, use of antibacterial mouthwash or presence of nitrate reducing bacteria such as Veillonella spp. [96]. Although a role of NO is established in the control of cerebrovascular reactivity, which may be a contributing factor to the development of AD and vascular dementia [93], no research has explicitly examined the links between the enterosalivary nitrate-nitrite nitric oxide pathway, hypertension, and subsequent risk of dementia.
Obesity
Obesity during midlife may further account for 2% of cases of dementia [92]; both overweight and obesity during midlife increase dementia risk [97]. A higher body mass index during midlife has been linked to an increased risk of dementia later in life, however lower weight tends to precede dementia in old age [98]. The impact of obesity may be due to both vascular diseases and metabolic alterations including insulin resistance and hyperinsulinemia [99]. Non-vascular pathways include chronic low-grade systemic inflammation, partially mediated by production of pro-inflammatory adipokines including interleukin 1 and interleukin 6 [100]. Leptin is secreted by adipose tissue and regulates food intake [100]. In late life higher circulating levels of leptin have been associated with reduced incidence of dementia [101, 102].
Obesity has a complex relationship with oral microbes and oral health. While there is no clear evidence for a positive association between caries and obesity [103], an association between worsening periodontal health and obesity has been identified, attributed to the chronic inflammatory state in periodontitis [104]. However, studies of the oral microbiome in obesity have to date not found an enrichment of periodontal disease associated species. Instead, obesity has been found to be associated with decreased levels of probiotic bacteria, such as Bifidobacterium longum in adults [11] and increased levels of Veillonella, Hameophillus, and Prevotella species in adolescents [105]. Aside from the link between periodontal disease and obesity, variation in the oral microbiome between individuals with obesity compared to those with normal weight has been linked to gut microbiome changes [106]. The possible causes of these associations include via inflammatory pathways, insulin resistance as a consequence of a chronic inflammatory state and (in the case of the gut microbiome), the extraction of energy from dietary intake [107]. Obesity, hypertension, and T2DM may have overlapping contributions to dementia risk via these routes, along with altered circulation due to endothelial dysfunction. Further research is needed to ascertain whether oral health, including presence of periodontitis and subsequent changes to the oral microbiome, are factors which contribute to increased dementia risk in these conditions.
Health behavioral risk factors
Smoking
Cigarette smoking is associated with an increased incidence of vascular risk factors for dementia including cardiovascular and cerebrovascular diseases [21]. Constituents of tobacco smoke are toxic to the brain, cardiovascular system, and lungs. Heavy smoking during midlife is linked to higher rates of AD and vascular dementia later in life [108]. Smoking causes damage to the endothelium, leading to atherosclerosis, white matter hyperintensities, and subcortical atrophy [19]. Cigarette smoke increases oxidative stress and reduces circulating concentrations of anti-oxidants [19]. Smoking induced oxidative stress contributes to glutamate toxicity, and heavy metals found in cigarette smoke contribute to Aβ aggregation [19, 109].
Smoking also changes the composition and predicted functions of the oral microbiome, with lower relative abundance of several taxa in the Proteobacteria phylum and genera Captnocytophaga Peptostreptococcus, and Leptotrichia observed in older adults who smoke [110]. These changes withstood adjustment for age and sex and were not observed in former smokers, suggesting that they may not be permanent. Inferred functional metagenomic analysis indicated that these depleted taxa corresponded to decreased aerobic respiration (Tricarboxylic Acid Cycle and oxidative phosphorylation) and increased anaerobic respiration (glycolysis, fructose, galactose, and sucrose metabolism) to enable oxygen independent carbohydrate metabolism [110]. A recent large study comparing smokers and nonsmokers in China also reported a number of compositional differences in smokers, with acid-production and amino-acid related pathways affected in functional analyses [111]. Smoking has been shown to increase risk of caries and periodontal disease [112, 113], both of which may relate to the observed changes to microbial composition. Exploring whether an altered oral microbiome, due to smoking, contributes to dementia risk is an important avenue for future research.
Physical activity
Low levels of physical activity have been associated with increased dementia risk [114]. It is estimated that approximately 13% of AD cases worldwide are attributed to physical inactivity [92]. Physical activity is a key determinant of the cardio-metabolic risk factors which are most relevant to AD risk during midlife [92]. Relevant mechanisms of adequate physical activity include benefits to vascular function by improving endothelial reactivity, insulin regulation, as well as reducing central arterial stiffness and chronic inflammation [115]. Direct effects on brain structure and function are considered to be mediated by pathways relevant to neurogenesis and neural plasticity [116]. In terms of potential attenuation of neurotoxicity, greater engagement in physical activity has been associated with lower brain and plasma levels of Aβ [117]. Level of engagement in physical activity may be most relevant to AD risk during midlife. For instance physical activity declines in the years preceding dementia diagnosis and over a longer term, neurodegeneration may contribute to behavioral alterations impacting physical activity engagement [118].
Nitrate-reducing activity of the oral microbiome is positively associated with cardiovascular fitness and reduction in blood pressure following exercise in healthy individuals [119, 120]. Decreased oral nitrate production may reflect reduced activity of some 200 oral microbes known to have nitrate reductase genes, rather than their abundance [121]. Exercise may impact the oral microbiome via a number of possible mechanisms [122, 123]. These include transient changes to salivary pH, lactate concentration in saliva, which change the environment and energy sources respectively for supporting the survival of oral microbes. There are also systemic changes to physiology which may result in oral microbial alterations: increased body temperature and changed blood flow distribution; anti-inflammatory myokines such as IL-6. Given that physical activity is proposed to influence dementia risk via multiple pathways [115], further work is required to uncover whether additional mechanisms, elicited by oral microbe changes, contribute to dementia risk.
Diet, alcohol, and sleep
Diet and sleep are additional important risk factors for dementia [1], although they are not yet considered in formal risk factor models or projections [92, 124]. Several dietary patterns have been associated with lower risk of AD including the Mediterranean diet, and the MIND diet which is a hybrid of the Mediterranean diet and the Dietary Approaches to Stop Hypertension diet [125]. The Mediterranean diet is primarily plant-based, and includes fermented dairy, fish, and small quantities of meat. Extra virgin olive oil is the main source of fat. Adherence to this dietary pattern has been consistently associated with better cognitive function and lower dementia risk, outside the Mediterranean region [96, 114, 126]. A high quality diet rich in B vitamins, omega 3 fatty acids, and flavonoids is considered to exert benefits to brain health via cerebrovascular and cardiovascular health, as well as direct effects on brain structural and functional integrity [127].
The impact of diet quality on the oral microbiome has not been comprehensively studied, though there is a well-established dental literature regarding the contribution of sugar-rich foods and beverages on the formation of biofilms, acidogenicity, caries, and gingivitis [128]. Excess sugar intake has been found to alter the predicted function of the oral microbiome to a more caries-like state, displaying a decreased potential for aerobic respiration, and increase in pathways for starch and sucrose metabolism, in addition to hydrolysis of glycosidic bonds [129]. Aside from sugar, bioactive food components such as polyphenols can also affect the oral microbiome via their antimicrobial and antibacterial properties. Clinical studies have demonstrated reduction in growth of Streptococci and P. gingivalis from pomegranate juice as mouth rinse and curcumin gel inserted into periodontal pockets oral products respectively, in people with periodontitis [130, 131]. Meanwhile in vitro studies using biofilm models of oral microbes present in supragingival plaque have demonstrated antimicrobial effects of red wine and dealcoholized wine on F. nucleatum and S. oralis [132], A. actinomycetemcomitans, and P. gingivalis [133]. The antiadhesive effects of wine extracts have also been demonstrated in vitro models [134]. While the antimicrobial effects of polyphenols on oral microbes are promising, clinical trials are needed to determine whether they have any corresponding effects on cognitive and other symptoms of AD.
In addition to the direct effects of single foods and their components, investigations of diet-types have revealed that a Western-style diet, high in refined carbohydrates predisposes the oral microbiome to a caries-promoting state in humans [135], and increases the likelihood of periodontal disease in companion animals [136]. In a small study of 49 people with overweight or obesity, an eight-week Mediterranean diet intervention, compared to usual diet, led to a changes in oral microbial composition [137]. Specifically, there was a decrease in relative abundance of operational taxonomic units assigned to genus Subdoligranulum and species Porphyromonas gingivalis, Prevotella intermedia, and Treponema denticola, which have been linked to periodontal disease. Interestingly, the influence of diet on the oral microbiome may be bidirectional, with the composition of the oral microbiome found to influence taste preference and eating habits [138].
Although the guidelines to limit alcohol vary across countries, it is typically recommended that alcohol intake does not exceed 8–14 units per week. Some studies have reported that low to moderate alcohol consumption may be a protective factor against dementia risk [139, 140], while the evidence suggests that drinking more than 21 units per week could increase dementia risk by 17%, compared to consuming less than 14 units [141]. Binge drinking can result in oral microbiome dysbiosis, increasing Porphyromonus spp and Neisseria spp, the two taxa proposed to overlap between binge drinkers’ oral microbiome and the microbiome in AD [142]. It has been hypothesized that high levels of ethanol in binge drinkers cause a shift in the microbiome, that leads to permeability changes in the blood-brain barrier, leading to alterations in multiple signaling pathways which could contribute to the pathogenesis of AD [142]. Alcohol may also impact pathways relevant to other aspects of oral health, with a study of over 35,000 adults from France indicating that greater alcohol intake increased the risk of self-reported severe periodontitis, with smoking found to further exacerbate this risk [143]. Interactions between alcohol and other health behavioral risk factors such as diet may be important to consider, especially given low alcohol intake is included in the Mediterraneandiet.
An emerging literature also points to a role of sleep, with disordered REM sleep [144] and sleep apnea [145] associated with dementia risk. However, randomized controlled trials are still required to ascertain the impact of sleep interventions on cognitive and dementia incidence outcomes. The human microbiome seems to be affected by host sleep-wake cycles, with no clear evidence of the inverse. The composition of the gut microbiota fluctuate on the basis of circadian rhythms in the host, as demonstrated by microbial transfer studies from jetlagged individuals into wild-type and circadian rhythm disabled rodents [146]. IL-6, a cytokine with known effects on sleep, may underpin these associations [147]. The oral microbiome is altered in sleep disorders such as insomnia and obstructive sleep apnea [148, 149]; however, whether sleep is affected by the oral microbiome remains an open question. More research is required to understand how oral health interacts with diet and sleep and how these relationships may in turn influence risk of dementia.
Education and psychosocial factors
A low level of early life education has been associated with increased risk of dementia [1], and this effect has been shown to be independent of socio-economic status [150]. Engagement in cognitively stimulating activities from a range of sources may also modify risk across the lifespan [6]. For instance, lower all cause and AD dementia risk is associated with greater complexity of the primary lifetime occupation [151]. The cognitive reserve model attributes these findings to the flexibility and adaptability of both brain and cognitive networks, enabling resistance of an individual to the clinical manifestation of observable brain damage [152]. With respect to oral health relevance, systematic reviews have supported the intuition that socioeconomic factors such as education and income level are relevant to oral health, likely via access to dentistry and propensity for oral hygiene practices [153, 154].
Other relevant psychosocial factors include depression and social isolation, two chronic psychological stressors that increase the risk of dementia and AD [1, 7]. Recent meta-analysis shows a small but statistically significant contribution of social engagement and social network size to preventing cognitive decline in later life [155]. Common neurobiological pathways between psychological stress and dementia are numerous, including bidirectional risks with vascular health and disease, impaired hypothalamic-pituitary-axis feedback mechanisms, increased susceptibility to inflammation, glucocorticoid steroid levels, Aβ deposition, brain atrophy, and nerve growth factor and neurotrophin alterations [156, 157]. Late life depression can also reflect a potential prodromal symptom of dementia [158], therefore life stage may be an important consideration regarding the influence of depression on AD risk. Data from the two waves of the large NHANES study (n = 10,214) shows oral disease and mental illness co-occur in a dose-dependent manner, even when controlling for covariates such as body mass index and C-reactive protein [159]. Emerging findings from a smaller study in a sample of at-risk young people show oral microbiota composition differences on the basis of anxiety and depressive symptom severity, suggesting that the microbiome may contribute to the observed associations between oral and mental health [160]. One of the most compelling findings reported in this study was the association between Treponema species, which are associated with periodontal disease, and both anxiety and depressive symptoms; with taxa from this same genus demonstrating a causal role in AD pathology [49].
Oral health and hygiene
The accumulating evidence regarding the relevance of oral health to neurological dys/function, and the eminently preventable nature of the most common oral diseases together provide a strong rationale for its inclusion as a modifiable lifestyle factor in dementia prevention. While direct evidence of mechanisms from oral microbes to the pathophysiology of AD is still emerging, there is sufficient indication of oral disease as a risk factor for cardiometabolic health, a dementia risk factor in its own right.
There remain non-microbial and bidirectional explanations for associations between oral and cognitive health in older age. For example, saliva production decreases in normal aging and as a side-effect of common medications [161], with flow-on effects to oral microbiome composition and propensity for caries [162]. The onset of cognitive decline may also correspond to changes in dietary intake via altered food choice and reduced capacity for food preparation, oral function (e.g., mastication), and oral hygiene practices [163–165]. There is pre-clinical and human evidence to suggest that the reduction of mastication behaviors and jaw mobility may predispose to hippocampal neuronal loss and cognitive deficits [166]. Despite these considerations, it is noted that the relevance of oral health in healthy middle age does support the temporal causality of oral-brain associations, even if reverse causality considerations emerge in older age. Longitudinal studies, ideally beginning early in life to capture potentially relevant developmental exposures, will be needed in order to determine whether oral health and microbiome features drive progression of pathology in dementia.
UNANSWERED QUESTIONS AND FUTURE RESEARCH DIRECTIONS
A number of questions remain in order to understand the roles of the oral microbiome in AD, particularly with respect to establishing causality and mechanisms of action. Treponema, spirochaetes, and P. gingivalis are emerging as relevant oral microbial players to AD pathophysiology; however, identification of these to species and strain level is required for translation to useful interventions. Further, understanding the functions by which these and other bacteria may affect neurodegenerative processes will be essential to mapping the mechanistic pathways to target.
The role of environmental influences such as air pollution on the oral microbiome and dementia risk is emerging as an important area of enquiry [6]. Air pollution may impact dementia risk through the development of cardiovascular disease [167]. Exposure to air pollution has been implicated in the development of mouth cancer [168] and has been associated with heightened inflammation in the presence of periodontitis [169]. It may therefore be the case that environmental pollutants also affect dementia risk via their impacts on the oral microbiome.
Understanding mechanistic pathways between the oral microbiome and AD/ADRD pathology is an essential next step. As a part of this, the timing of any microbial contributions to the disease process must be delineated. Given the presence of AD pathophysiology decades prior to symptom onset, it is likely that any contribution from oral microbes would also occur pre-symptomatically. Could microbial exposures in childhood affect risk trajectory, as per the developmental origins of health and disease hypothesis, demonstrated for a vast array of non-communicable diseases [170]? Could the progression of disease be prevented or slowed even in later life via the management of lifestyle risk factors including oral hygiene?
It is currently unclear whether alterations to the oral microbiome may contribute to AD pathology by exacerbating modifiable dementia risk factors, or whether there is an additive role where the presence of both dementia risk factors and altered oral microbes intensify or accelerate pathology. More research is needed to understand the interaction between dementia risk factors and the oral microbiome. This is especially true for risk factors such as head injury and hearing loss, for which there is very limited research into a potential role of the oral microbiome in AD pathogenesis. The next logical questions therefore include: how might knowledge about the oral microbiome be used to reduce AD risk? Might this be possible directly, such as via mouth-directed psychobiotic treatments, or indirectly via modifiable determinants of problematic or dysfunctional oral microbes? Lifestyle risk factors are magnified for APOE4 carriers [171]; might the efficacy of oral interventions also differ by baseline genetic risk? Alternatively, data regarding the oral microbiome may be combined with demographic and lifestyle factors to improve predictive accuracy regarding future AD risk. To date, only four dementia prediction tools have been developed for adults in middle-age, with the remainder tested in late life, amnestic mild cognitive impairment, and diabetes, with area under the curve ranging from 0.7–0.8 [172]. Prediction accuracy and utility is likely to be enhanced when incorporating genetics, microbiome, and multiple established risk factors—not any one of these domains alone. A computational approach to demonstrate pathways between gut microbial metabolites (TMAO) and AD biomarkers [173] provides a useful proof of concept for an integrated data approach.
Answering these questions will require evidence from several study designs: longitudinal cohort studies with serial measurement of the oral microbiome, deep phenotyping of AD biomarkers such as sensitive measures of cognitive function, and all relevant potentially confounding factors; in vitro and in vivo mechanistic studies and pre-clinical and clinical trials. Clearly, the research methods and perspectives from collaboration from interdisciplinary groups will be required.
Translational potential
The translational potential is significant. There is already sufficient evidence to recommend universal oral healthcare, a sensible and feasible public health initiative endorsed by the World Health Assembly Resolution in 2021 [174]. A recent review of eight high-income jurisdictions worldwide revealed a wide range of dental health coverage, with most providing universal coverage for only basic services on the basis of strict eligibility criteria [175]. Such limitations in the provision of care are often associated with a lack of preventative intervention which is likely to be essential for lifelong maintenance of good oral health and the prevention of cardiometabolic and neurological sequelae of oral infection [175]. A 24-week oral health care intervention in people with mild AD showed small but significant positive effects on cognition and daily functioning measures, and changed the composition of the oral microbiome, though we note that the intervention included a number of components relating to broader aspects of lifestyle such as diet, exercise, and cognitively stimulating activity [176]. A quasi-experimental study of patients treated for periodontitis showed favorable effects on AD-related brain imaging after a mean follow-up period of 7 years [177]. Despite compelling observational evidence to suggest the beneficial role of non-steroidal anti-inflammatory drugs, interventions targeting systemic inflammation in this way have been less successful. The follow-up study of the Alzheimer’s Disease Anti-inflammatory Prevention Trial (ADAPT), which was halted after 3 years of enrolment due to cardiovascular safety concerns, showed no AD preventative effect of non-steroidal anti-inflammatory drugs celecoxib or naproxen in adults with a family history of dementia [178]. A large Australian randomized controlled trial of aspirin of almost 20,000 participants found no evidence of reduced risk of cognitive decline in the intervention group [179]. Inflammation on its own may therefore not be a straightforwardly effective target, and there may be other mechanisms underpinning the associations between oral health and AD risk.
The additional benefits of dental care and lifestyle-based public health interventions in their potential to reduce the risk of dementia and other non-communicable diseases may provide further motivation to various stakeholders for their inclusion in the broader healthcare landscape. For example, economic analysis of the benefits of oral health interventions to the socioeconomic burden of dementia may be requisite for the business case to broaden public dental health services. Oral health promotion messaging may be more effective when the salient topi of brain health and aging is included. And individuals may be more motivated to make lasting lifestyle changes when an additional benefit of supporting the oral-brain connection is clear, in a similar way that the gut microbiome has provided an alternative motive for diet improvement [180], quite apart from the stigmatizing (and difficult) goal of weight-loss or control of metabolic healthmarkers [181].
Future research may also inform more specific microbial interventions to enhance the efficacy of broad preventative and public health efforts, and possible individualization of targeted therapeutics beyond improvements in dental health outcomes. With respect to oral interventions targeting those at increased risk of AD, or with an existing diagnosis, different approaches may be required for different stages of the disease process. Further examples of potential novel interventions directed at the oral-brain axis may include probiotic supplements with the goal of decreasing oral inflammation and antibiotics for specific pathogens found to be relevant, in line with a growing literature regarding the effectiveness of gut-directed probiotic interventions for AD [182]. While novel therapies targeting the oral microbiome such as gingipain inhibitors, phototherapy, and targeted probiotic and prebiotic treatments have theoretical promise, they are yet to be tested [183]. Oral microbiome transplant has also been proposed [184] but no outcomes have been demonstrated to date. Risks of these novel approaches, in particular ‘whole of microbiome’ strategies such as microbial transplant, are that it is largely a black box. As for fecal microbiome transplants, increasingly trialed for a range of physical and mental conditions [185], the mechanisms of action remain unclear, and potential side-effects are prompting increased governance around donor screening protocols [186, 187].
CONCLUSION
There is a growing burden of AD/ARD on society, especially in low to middle income countries where population ageing is taking place. While mechanisms remain unknown, periodontitis and changes to the oral microbiome have been associated with a range of modifiable cardiometabolic, health and psychosocial dementia risk factors. Oral microbes including Treponema, spirochaetes, and P. gingivalis require further investigation as potential targets for intervention. Although the interactions between oral health, dementia risk factors and neuropathology are likely to be complex, uncovering these pathways may lead to new understanding of disease and preventative treatments.
ACKNOWLEDGMENTS
The authors would like to acknowledge the contribution of Jessica Batti in the design of Fig. 1.
FUNDING
The authors have no funding to report.
CONFLICT OF INTEREST
The authors have no conflict of interest to report.
REFERENCES
[1] | Livingston G , Sommerlad A , Orgeta V , Costafreda SG , Huntley J , Ames D , Ballard C , Banerjee S , Burns A , Cohen-Mansfield J , Cooper C , Fox N , Gitlin LN , Howard R , Kales HC , Larson EB , Ritchie K , Rockwood K , Sampson EL , Samus Q , Schneider LS , Selbæk G , Teri L , Mukadam N ((2017) ) Dementia prevention, intervention, and care. Lancet 390: , 2673–2734. |
[2] | Steinbrook R ((2021) ) The accelerated approval of aducanumab for treatment of patients with Alzheimer disease. JAMA Intern Med 181: , 1281. |
[3] | Makin S ((2018) ) The amyloid hypothesis on trial. Nature 559: , S4–S7. |
[4] | Sochocka M , Donskow-Lysoniewska K , Diniz BS , Kurpas D , Brzozowska E , Leszek J ((2019) ) The gut microbiome alterations and inflammation-driven pathogenesis of Alzheimer’s disease-a critical review. Mol Neurobiol 56: , 1841–1851. |
[5] | Aisen PS , Cummings J , Jack CR Jr. , Morris JC , Sperling R , Frölich L , Jones RW , Dowsett SA , Matthews BR , Raskin J , Scheltens P , Dubois B ((2017) ) On the path to 2025: Understanding the Alzheimer’s disease continuum. Alzheimers Res Ther 9: , 60. |
[6] | Livingston G , Huntley J , Sommerlad A , Ames D , Ballard C , Banerjee S , Brayne C , Burns A , Cohen-Mansfield J , Cooper C , Costafreda SG , Dias A , Fox N , Gitlin LN , Howard R , Kales HC , Kivimäki M , Larson EB , Ogunniyi A , Orgeta V , Ritchie K , Rockwood K , Sampson EL , Samus Q , Schneider LS , Selbæk G , Teri L , Mukadam N ((2020) ) Dementia prevention, intervention, and care: 2020 report of the Lancet Commission. Lancet 396: , 413–446. |
[7] | Kivipelto M , Mangialasche F , Ngandu T ((2018) ) Lifestyle interventions to prevent cognitive impairment, dementia and Alzheimer disease. Nat Rev Neurol 14: , 653–666. |
[8] | Jungbauer G , Stahli A , Zhu X , Auber Alberi L , Sculean A , Eick S ((2022) ) Periodontal microorganisms and Alzheimer disease - A causative relationship? Periodontol 2000 89: , 59–82. |
[9] | Manor O , Dai CL , Kornilov SA , Smith B , Price ND , Lovejoy JC , Gibbons SM , Magis AT ((2020) ) Health and disease markers correlate with gut microbiome composition across thousands of people. Nat Commun 11: , 5206. |
[10] | Matsha TE , Prince Y , Davids S , Chikte U , Erasmus RT , Kengne AP , Davison GM ((2020) ) Oral microbiome signatures in diabetes mellitus and periodontal disease. J Dent Res 99: , 658–665. |
[11] | Yang Y , Cai Q , Zheng W , Steinwandel M , Blot WJ , Shu XO , Long J ((2019) ) Oral microbiome and obesity in a large study of low-income and African-American populations. J Oral Microbiol 11: , 1650597. |
[12] | Kinney JW , Bemiller SM , Murtishaw AS , Leisgang AM , Salazar AM , Lamb BT ((2018) ) Inflammation as a central mechanism in Alzheimer’s disease. Alzheimers Dement (N Y) 4: , 575–590. |
[13] | Wang J , Gu BJ , Masters CL , Wang Y-J ((2017) ) A systemic view of Alzheimer disease – insights from amyloid-[beta] metabolism beyond the brain. Nat Rev Neurol 13: , 612–623. |
[14] | Raz L , Knoefel J , Bhaskar K ((2016) ) The neuropathology and cerebrovascular mechanisms of dementia. J Cereb Blood Flow Metab 36: , 172–186. |
[15] | Butterfield DA , Halliwell B ((2019) ) Oxidative stress, dysfunctional glucose metabolism and Alzheimer disease. Nat Rev Neurosci 20: , 148–160. |
[16] | de la Monte SM , Wands JR ((2008) ) Alzheimer’s disease is type 3 diabetes - evidence reviewed. J Diabetes Sci Technol 2: , 1101–1113. |
[17] | Kroner Z ((2009) ) The relationship between Alzheimer’s disease and diabetes: Type 3 diabetes? Altern Med Rev 14: , 373–379. |
[18] | Kandimalla R , Thirumala V , Reddy PH ((2017) ) Is Alzheimer’s disease a type 3 diabetes? A critical appraisal. Biochim Biophys Acta 1863: , 1078–1089. |
[19] | Swan GE , Lessov-Schlaggar CN ((2007) ) The effects of tobacco smoke and nicotine on cognition and the brain. Neuropsychol Rev 17: , 259–273. |
[20] | Cheignon C , Tomas M , Bonnefont-Rousselot D , Faller P , Hureau C , Collin F ((2018) ) Oxidative stress and the amyloid beta peptide in Alzheimer’s disease. Redox Biol 14: , 450–464. |
[21] | Gorelick PB , Scuteri A , Black SE , Decarli C , Greenberg SM , Iadecola C , Launer LJ , Laurent S , Lopez OL , Nyenhuis D , Petersen RC , Schneider JA , Tzourio C , Arnett DK , Bennett DA , Chui HC , Higashida RT , Lindquist R , Nilsson PM , Roman GC , Sellke FW , Seshadri S ((2011) ) Vascular contributions to cognitive impairment and dementia: A statement for healthcare professionals from the American Heart Association/American Stroke Association. Stroke 42: , 2672–2713. |
[22] | Attems J , Jellinger KA ((2014) ) The overlap between vascular disease and Alzheimer’s disease - lessons from pathology. BMC Med 12: , 206. |
[23] | Schneider JA , Arvanitakis Z , Leurgans SE , Bennett DA ((2009) ) The neuropathology of probable Alzheimer disease and mild cognitive impairment. Ann Neurol 66: , 200–208. |
[24] | McKeith IG , Galasko D , Kosaka K , Perry EK , Dickson DW , Hansen LA , Salmon DP , Lowe J , Mirra SS , Byrne EJ , Lennox G , Quinn NP , Edwardson JA , Ince PG , Bergeron C , Burns A , Miller BL , Lovestone S , Collerton D , Jansen ENH , Ballard C , De Vos RAI , Wilcock GK , Jellinger KA , Perry RH ((1996) ) Consensus guidelines for the clinical and pathologic diagnosis of dementia with Lewy bodies (DLB): Report of the consortium on DLB international workshop. Neurology 47: , 1113–1124. |
[25] | McKhann GM , Albert MS , Grossman M , Miller B , Dickson D , Trojanowski JQ ((2001) ) Clinical and pathological diagnosis of frontotemporal dementia: Report of the Work Group on Frontotemporal Dementia and Pick’s Disease. Arch Neurol 58: , 1803–1809. |
[26] | Teylan M , Mock C , Gauthreaux K , Chen Y-C , Chan KCG , Hassenstab J , Besser LM , Kukull WA , Crary JF ((2020) ) Cognitive trajectory in mild cognitive impairment due to primary age-related tauopathy. Brain 143: , 611–621. |
[27] | Nelson PT , Dickson DW , Trojanowski JQ , Jack CR , Boyle PA , Arfanakis K , Rademakers R , Alafuzoff I , Attems J , Brayne C , Coyle-Gilchrist ITS , Chui HC , Fardo DW , Flanagan ME , Halliday G , Hokkanen SRK , Hunter S , Jicha GA , Katsumata Y , Kawas CH , Keene CD , Kovacs GG , Kukull WA , Levey AI , Makkinejad N , Montine TJ , Murayama S , Murray ME , Nag S , Rissman RA , Seeley WW , Sperling RA , White III CL , Yu L , Schneider JA ((2019) ) Limbic-predominant age-related TDP-43 encephalopathy (LATE): Consensus working group report. Brain 142: , 1503–1527. |
[28] | James BD , Wilson RS , Boyle PA , Trojanowski JQ , Bennett DA , Schneider JA ((2016) ) TDP-43 stage, mixed pathologies, and clinical Alzheimer’s-type dementia. Brain 139: , 2983–2993. |
[29] | Tremlett H , Bauer KC , Appel-Cresswell S , Finlay BB , Waubant E ((2017) ) The gut microbiome in human neurological disease: A review. Ann Neurol 81: , 369–382. |
[30] | Meyer K , Lulla A , Debroy K , Shikany JM , Yaffe K , Meirelles O , Launer LJ ((2022) ) Association of the gut microbiota with cognitive function in midlife. JAMA Network Open 5: , e2143941. |
[31] | Sureda A , Daglia M , Argüelles Castilla S , Sanadgol N , Fazel Nabavi S , Khan H , Belwal T , Jeandet P , Marchese A , Pistollato F , Forbes-Hernandez T , Battino M , Berindan-Neagoe I , D’Onofrio G , Nabavi SM ((2019) ) Oral microbiota and Alzheimer’s disease: Do all roads lead to Rome? Pharmacol Res 151: , 104582. |
[32] | Dewhirst FE , Chen T , Izard J , Paster BJ , Tanner ACR , Yu WH , Lakshmanan A , Wade WG ((2010) ) The human oral microbiome. J Bacteriol 192: , 5002–5017. |
[33] | Dzidic M , Collado MC , Abrahamsson T , Artacho A , Stensson M , Jenmalm MC , Mira A ((2018) ) Oral microbiome development during childhood: An ecological succession influenced by postnatal factors and associated with tooth decay. ISME J 12: , 2292–2306. |
[34] | Lif Holgerson P , Esberg A , Sjodin A , West CE , Johansson I ((2020) ) A longitudinal study of the development of the saliva microbiome in infants 2 days to 5 years compared to the microbiome in adolescents. Sci Rep 10: , 9629. |
[35] | Liu S , Wang Y , Zhao L , Sun X , Feng Q ((2020) ) Microbiome succession with increasing age in three oral sites. Aging (Albany NY) 12: , 7874–7907. |
[36] | Willis JR , Saus E , Iraola-Guzmán S , Ksiezopolska E , Cozzuto L , Bejarano LA , Andreu-Somavilla N , Alloza-Trabado M , Blanco A , Puig-Sola A , Broglio E , Carolis C , Ponomarenko J , Hecht J , Gabaldón T ((2022) ) Citizen-science reveals changes in the oral microbiome in Spain through age and lifestyle factors. NPJ Biofilms Microbiomes 8: , 38. |
[37] | Fluitman KS , van den Broek TJ , Nieuwdorp M , Visser M , RG IJ , Keijser BJF ((2021) ) Associations of the oral microbiota and Candida with taste, smell, appetite and undernutrition in older adults. Sci Rep 11: , 23254. |
[38] | Kim HE ((2021) ) Influential factors of masticatory performance in older adults: A cross-sectional study. Int J Environ Res Public Health 18: , 4286. |
[39] | Scholz F , Badgley BD , Sadowsky MJ , Kaplan DH ((2014) ) Immune mediated shaping of microflora community composition depends on barrier site, PLoS One 9: , e84019. |
[40] | Qin N , Yang F , Li A , Prifti E , Chen Y , Shao L , Guo J , Le Chatelier E , Yao J , Wu L , Zhou J , Ni S , Liu L , Pons N , Batto JM , Kennedy SP , Leonard P , Yuan C , Ding W , Chen Y , Hu X , Zheng B , Qian G , Xu W , Ehrlich SD , Zheng S , Li L ((2014) ) Alterations of the human gut microbiome in liver cirrhosis. Nature 513: , 59–64. |
[41] | Segata N , Haake SK , Mannon P , Lemon KP , Waldron L , Gevers D , Huttenhower C , Izard J ((2012) ) Composition of the adult digestivetract bacterial microbiome based on seven mouth surfaces, tonsils,throat and stool samples, Genome Biol 13: , R42. |
[42] | Olsen I , Yamazaki K ((2019) ) Can oral bacteria affect the microbiome of the gut? J Oral Microbiol 11: , 1586422. |
[43] | Olsen I , Yilmaz O ((2019) ) Possible role of Porphyromonas gingivalis in orodigestive cancers. J Oral Microbiol 11: , 1563410. |
[44] | Hajishengallis G ((2015) ) Periodontitis: From microbial immune subversion to systemic inflammation. Nat Rev Immunol 15: , 30–44. |
[45] | Gatz M , Mortimer JA , Fratiglioni L , Johansson B , Berg S , Reynolds CA , Pedersen NL ((2006) ) Potentially modifiable risk factors for dementia in identical twins. Alzheimers Dement 2: , 110–117. |
[46] | Stein PS , Desrosiers M , Donegan SJ , Yepes JF , Kryscio RJ ((2007) ) Tooth loss, dementia and neuropathology in the Nun Study. J Am Dent Assoc 138: , 1314–1322. |
[47] | Shoemark DK , Allen SJ ((2015) ) The microbiome and disease: Reviewing the links between the oral microbiome, aging, and Alzheimer’s disease. J Alzheimers Dis 43: , 725–738. |
[48] | Stahringer SS , Clemente JC , Corley RP , Hewitt J , Knights D , Walters WA , Knight R , Krauter KS ((2012) ) Nurture trumps nature in a longitudinal survey of salivary bacterial communities in twins from early adolescence to early adulthood. Genome Res 22: , 2146–2152. |
[49] | Miklossy J ((2011) ) Alzheimer’s disease - a neurospirochetosis. Analysis of the evidence following Koch’s and Hill’s criteria. J Neuroinflammation 8: , 90–90. |
[50] | Poole S , Singhrao SK , Kesavalu L , Curtis MA , Crean S ((2013) ) Determining the presence of periodontopathic virulence factors inshort-term postmortem Alzheimer’s disease brain tissue. J Alzheimers Dis 36: , 665–677. |
[51] | Noble JM , Borrell LN , Papapanou PN , Elkind MSV , Scarmeas N , Wright CB ((2009) ) Periodontitis is associated with cognitive impairment among older adults: Analysis of NHANES-III. J Neurol Neurosurg Psychiatry 80: , 1206–1211. |
[52] | Stewart R , Sabbah W , Tsakos G , D’Aiuto F , Watt RG ((2008) ) Oral health and cognitive function in the Third National Health and Nutrition Examination Survey (NHANES III). Psychosom Med 70: , 936–941. |
[53] | Beydoun MA , Beydoun HA , Hossain S , El-Hajj ZW , Weiss J , Zonderman AB ((2020) ) Clinical and bacterial markers of periodontitis and their association with incident all-cause and Alzheimer’s disease dementia in a large national survey. J Alzheimers Dis 75: , 157–172. |
[54] | Kamer AR , Craig RG , Pirraglia E , Dasanayake AP , Norman G , Boylan RJ , Nehorayoff A , Glodzik L , Brys M , Leon MJD ((2010) ) TNF-α andantibodies to periodontal bacteria discriminate between Alzheimer sdisease patients and normal subjects. J Neuroimmunol 216: , 92–97. |
[55] | Sparks Stein P , Steffen MJ , Smith C , Jicha G , Ebersole JL , Abner E , Dawson D 3rd ((2012) ) Serum antibodies to periodontal pathogens are a risk factor for Alzheimer’s disease. Alzheimers Dement 8: , 196–203. |
[56] | Capsoni S , Maria Carucci N , Cattaneo A ((2012) ) Pathogen free conditions slow the onset of neurodegeneration in a mouse model of nerve growth factor deprivation. J Alzheimers Dis 30: , 1–6. |
[57] | Kristensson K ((2011) ) Microbes’ roadmap to neurons. Nat Rev Neurosci 12: , 345–357. |
[58] | Harris SA , Harris EA ((2015) ) Herpes simplex virus type 1 and other pathogens are key causative factors in sporadic Alzheimer’s disease. J Alzheimers Dis 48: , 319–353. |
[59] | Nessa BN , Tanaka T , Kamino K , Sadik G , Ansar AB , Kimura R , Tanii H , Okochi M , Morihara T , Tagami S , Kudo T , Takeda M ((2006) ) Toll-like receptor 3 mediated hyperphosphorylation of tau in human SH-SY5Y neuroblastoma cells. Psychiatry Clin Neurosci 60: , 1323–1316. |
[60] | Miklossy J , Kis A , Radenovic A , Miller L , Forro L , Martins R , Reiss K , Darbinian N , Darekar P , Mihaly L , Khalili K ((2006) ) Beta-amyloid deposition and Alzheimer’s type changes induced by Borrelia spirochetes. Neurobiol Aging 27: , 228–236. |
[61] | Fulop T , Witkowski JM , Bourgade K , Khalil A , Zerif E , Larbi A , Hirokawa K , Pawelec G , Bocti C , Lacombe G , Dupuis G , Frost EH ((2018) ) Can an infection hypothesis explain the beta amyloid hypothesis of Alzheimer’s disease? Front Aging Neurosci 10: , 224. |
[62] | Lockhart PB , Brennan MT , Sasser HC , Fox PC , Paster BJ , Bahrani-Mougeot FK ((2008) ) Bacteremia associated with toothbrushing and dental extraction. Circulation 117: , 3118–3125. |
[63] | Stentz R , Carvalho AL , Jones EJ , Carding SR ((2018) ) Fantastic voyage: The journey of intestinal microbiota-derived microvesicles through the body. Biochem Soc Trans 46: , 1021–1027. |
[64] | Bisht K , Sharma K , Tremblay M-È ((2018) ) Chronic stress as a risk factor for Alzheimer’s disease: Roles of microglia-mediated synaptic remodeling, inflammation, and oxidative stress. Neurobiol Stress 9: , 9–21. |
[65] | Farhad SZ , Amini S , Khalilian A , Barekatain M , Mafi M , Barekatain M , Rafei E ((2014) ) The effect of chronic periodontitis on serum levels of tumor necrosis factor-alpha in Alzheimer disease. Dent Res J (Isfahan) 11: , 549–552. |
[66] | Ide M , Harris M , Stevens A , Sussams R , Hopkins V , Culliford D , Fuller J , Ibbett P , Raybould R , Thomas R , Puenter U , Teeling J , Perry VH , Holmes C ((2016) ) Periodontitis and cognitive decline in Alzheimer’s disease. PLoS One 11: , e0151081. |
[67] | Gil Montoya JA , Barrios R , Sanchez-Lara I , Ramos P , Carnero C , Fornieles F , Montes J , Santana S , Luna JdD , Gonzalez-Moles MA ((2020) ) Systemic inflammatory impact of periodontitis on cognitive impairment. Gerodontology 37: , 11–18. |
[68] | Wu H , Qiu W , Zhu X , Li X , Xie Z , Carreras I , Dedeoglu A , Van Dyke T , Han YW , Karimbux N , Tu Q , Cheng L , Chen J ((2022) ) The periodontal pathogen Fusobacterium nucleatum exacerbates Alzheimer’s pathogenesis via specific pathways. Front Aging Neurosci 14: , 912709. |
[69] | Leszek J , E Barreto G , Gasiorowski K , Koutsouraki E , Aliev G ((2016) ) Inflammatory mechanisms and oxidative stress as key factors responsible for progression of neurodegeneration: Role of brain innate immune system. CNS Neurol Disord Drug Targets 15: , 329–336. |
[70] | Dinan TG , Cryan JF ((2015) ) The impact of gut microbiota on brain and behaviour. Curr Opin Clin Nutr Metab Care 18: , 552–558. |
[71] | Zhuang Z-Q , Shen L-L , Li W-W , Fu X , Zeng F , Gui L , Lü Y , Cai M , Zhu C , Tan Y-L , Zheng P , Li H-Y , Zhu J , Zhou H-D , Bu X-L , Wang Y-J ((2018) ) Gut microbiota is altered in patients with Alzheimer’s disease. J Alzheimers Dis 63: , 1337–1346. |
[72] | Vogt NM , Kerby RL , Dill-McFarland KA , Harding SJ , Merluzzi AP , Johnson SC , Carlsson CM , Asthana S , Zetterberg H , Blennow K , Bendlin BB , Rey FE ((2017) ) Gut microbiome alterations in Alzheimer’s disease. Sci Rep 7: , 13537. |
[73] | Blekhman R , Goodrich JK , Huang K , Sun Q , Bukowski R , Bell JT , Spector TD , Keinan A , Ley RE , Gevers D ((2015) ) Host genetic variation impacts microbiome composition across human body sites. Genome Biol 16: , 191. |
[74] | Martins IJ , Hone E , Foster JK , Sünram-Lea SI , Gnjec A , Fuller SJ , Nolan D , Gandy SE , Martins RN ((2006) ) Apolipoprotein E, cholesterol metabolism, diabetes, and the convergence of risk factors for Alzheimer’s disease and cardiovascular disease. Mol Psychiatry 11: , 721–736. |
[75] | Mansoori N , Tripathi M , Luthra K , Alam R , Lakshmy R , Sharma S , Arulselvi S , Parveen S , Mukhopadhyay AK ((2012) ) MTHFR (677 and 1298) and IL-6-174G/C genes in pathogenesis of Alzheimer’s and vascular dementia and their epistatic interaction, Neurobiol Aging 33: , 1003.e1–8. |
[76] | McIlroy SP , Dynan KB , Lawson JT , Patterson CC , Passmore AP ((2002) ) Moderately elevated plasma homocysteine, methylenetetrahydrofolate reductase genotype, and risk for stroke, vascular dementia, and Alzheimer disease in Northern Ireland. Stroke 33: , 2351–2356. |
[77] | Slocum C , Kramer C , Genco CA ((2016) ) Immune dysregulation mediated by the oral microbiome: Potential link to chronic inflammation and atherosclerosis. J Intern Med 280: , 114–128. |
[78] | Mathews MJ , Mathews EH , Mathews GE ((2016) ) Oral health and coronary heart disease. BMC Oral Health 16: , 122. |
[79] | Marotz C , Molinsky R , Martino C , Bohn B , Roy S , Rosenbaum M , Desvarieux M , Yuzefpolskaya M , Paster BJ , Jacobs DR ((2022) ) Early microbial markers of periodontal and cardiometabolic diseases in ORIGINS. NPJ Biofilms Microbiomes 8: , 30. |
[80] | Cheng G , Huang C , Deng H , Wang H ((2012) ) Diabetes as a risk factor for dementia and mild cognitive impairment: A meta-analysis of longitudinal studies. Intern Med J 42: , 484–491. |
[81] | Biessels GJ , Strachan MWJ , Visseren FLJ , Kappelle LJ , Whitmer RA ((2013) ) Dementia and cognitive decline in type 2 diabetes and prediabetic stages: Towards targeted interventions. Lancet Diabetes Endocrinol 2: , 246–255. |
[82] | Exalto LG , Whitmer RA , Kappele LJ , Biessels GJ ((2012) ) An update on type 2 diabetes, vascular dementia and Alzheimer’s disease. Exp Gerontol 47: , 858–864. |
[83] | Cholerton B , Baker LD , Craft S ((2013) ) Insulin, cognition, and dementia. Eur J Pharmacol 719: , 170–179. |
[84] | Macpherson H , Formica M , Harris E , Daly RM ((2017) ) Brain functional alterations in type 2 diabetes – A systematic review of fMRI studies. Front Neuroendocrinol 47: , 34–46. |
[85] | Borgnakke WS , Yl€ostalo PV , Taylor GW , Genco RJ ((2013) ) Effect of periodontal disease on diabetes: Systematic review of epidemiologic observational evidence, J Periodontol 84: , S135–S152. |
[86] | Corbella S , Francetti L , Taschieri S , De Siena F , Fabbro MD ((2013) ) Effect of periodontal treatment on glycemic control of patients with diabetes: A systematic review and meta-analysis. J Diabetes Investig 4: , 502–509. |
[87] | Baeza M , Morales A , Cisterna C , Cavalla F , Jara G , Isamitt Y , Pino P , Gamonal J ((2020) ) Effect of periodontal treatment in patients with periodontitis and diabetes: Systematic review and meta-analysis, J Appl Oral Sci 28: , e20190248. |
[88] | Long J , Cai Q , Steinwandel M , Hargreaves MK , Bordenstein SR , Blot WJ , Zheng W , Shu XO ((2017) ) Association of oral microbiome with type 2 diabetes risk. J Periodontal Res 52: , 636–643. |
[89] | Xiao E , Mattos M , Vieira GHA , Chen S , Corrêa JD , Wu Y , Albiero ML , Bittinger K , Graves DT ((2017) ) Diabetes enhances IL-17 expression and alters the oral microbiome to increase its pathogenicity, Cell Host Microbe 22: , 120–128.e124. |
[90] | Lalla E , Lamster IB , Feit M , Huang L , Spessot A , Qu W , Kislinger T , Lu Y , Stern DM , Schmidt AM ((2000) ) Blockade of RAGE suppresses periodontitis-associated bone loss in diabetic mice. J Clin Invest 105: , 1117–1124. |
[91] | Goh CE , Trinh P , Colombo PC , Genkinger JM , Mathema B , Uhlemann AC , LeDuc C , Leibel R , Rosenbaum M , Paster BJ ((2019) ) Association between nitrate-reducing oral bacteria and cardiometabolic outcomes: Results from ORIGINS, J Am Heart Assoc 8: , e013324. |
[92] | Barnes DE , Yaffe K ((2011) ) The projected effect of risk factor reduction on Alzheimer’s disease prevalence. Lancet Neurol 10: , 819–828. |
[93] | Faraco G , Iadecola C ((2013) ) Hypertension: A harbinger of stroke and dementia. Hypertension 62: , 810–817. |
[94] | Pietropaoli D , Pinto RD , Ferri C , Wright JT , Giannoni M , Ortu E , Monaco A ((2018) ) Poor oral health and blood pressure control among US hypertensive adults. Hypertension 72: , 1365–1373. |
[95] | Bryan NS , Tribble G , Angelov N ((2017) ) Oral microbiome and nitric oxide: The missing link in the management of blood pressure. Curr Hypertens Rep 19: , 33. |
[96] | Blekkenhorst LC , Bondonno NP , Liu AH , Ward NC , Prince RL , Lewis JR , Devine A , Croft KD , Hodgson JM , Bondonno CP ((2018) ) Nitrate, the oral microbiome, and cardiovascular health: A systematic literature review of human and animal studies. Am J Clin Nutr 107: , 504–522. |
[97] | Xu WL , Atti AR , Gatz M , Pedersen NL , Johansson B , Fratiglioni L ((2011) ) Midlife overweight and obesity increase late-life dementia risk: A population-based twin study. Neurology 76: , 1568–1574. |
[98] | Singh-Manoux A , Dugravot A , Shipley M , Brunner EJ , Elbaz A , Sabia S , Kivimaki M ((2018) ) Obesity trajectories and risk of dementia: 28 years of follow-up in the Whitehall II Study. Alzheimers Dement 14: , 178–186. |
[99] | Luchsinger JA , Gustafson DR ((2009) ) Adiposity and Alzheimer’s disease. Curr Opin Clin Nutr Metab Care 12: , 15–21. |
[100] | Kiliaan AJ , Arnoldussen IAC , Gustafson DR ((2014) ) Adipokines: A link between obesity and dementia? Lancet Neurol 13: , 913–923. |
[101] | Lieb W , Beiser AS , Vasan RS , Tan ZS , Au R , Harris TB , Roubenoff R , Auerbach S , DeCarli C , Wolf PA , Seshadri S ((2009) ) Association of plasma leptin levels with incident Alzheimer disease and MRI measures of brain aging. JAMA 302: , 2565–2572. |
[102] | Goodson JM , Groppo D , Halem S , Carpino E ((2009) ) Is obesity an oral bacterial disease? J Dent Res 88: , 519–523. |
[103] | Barrington G , Khan S , Kent K , Brennan DS , Crocombe LA , Bettiol S ((2019) ) Obesity, dietary sugar and dental caries in Australian adults. Int Dent J 69: , 383–391. |
[104] | Wood N , Johnson RB , Streckfus CF ((2003) ) Comparison of body composition and periodontal disease using nutritional assessment techniques: Third National Health and Nutrition Examination Survey (NHANES III). J Clin Periodontol 30: , 321–327. |
[105] | de Andrade PAM , Giovani PA , Araujo DS , de Souza AJ , Pedroni-Pereira A , Kantovitz KR , Andreote FD , Castelo PM , Nociti-Jr FH ((2020) ) Shifts in the bacterial community of saliva give insights on the relationship between obesity and oral microbiota in adolescents. Arch Microbiol 202: , 1085–1095. |
[106] | Benahmed AG , Gasmi A , Doşa A , Chirumbolo S , Mujawdiya PK , Aaseth J , Dadar M , Bjørklund G ((2020) ) Association between the gut and oral microbiome with obesity. Anaerobe 70: , 102248. |
[107] | Martinez-Herrera M , Silvestre-Rangil J , Silvestre FJ ((2017) ) Association between obesity and periodontal disease. A systematic review of epidemiological studies and controlled clinical trials, Med Oral Patol Oral Cir 22: , e708–e715. |
[108] | Rusanen M , Kivipelto M , Quesenberry CP Jr , Zhou J , Whitmer RA ((2011) ) Heavy smoking in midlife and long-term risk of Alzheimer disease and vascular dementia. Arch Intern Med 171: , 333–339. |
[109] | Wallin C , Sholts SB , Österlund N , Luo J , Jarvet J , Roos PM , Ilag L , Gräslund A , Wärmländer SKTS ((2017) ) Alzheimer’s disease and cigarette smoke components: Effects of nicotine, PAHs, and Cd(II), Cr(III), Pb(II), Pb(IV) ions on amyloid-β peptide aggregation. Sci Rep 7: , 14423. |
[110] | Wu J , Peters BA , Dominianni C , Zhang Y , Pei Z , Yang L , Ma Y , Purdue MP , Jacobs EJ , Gapstur SM , Li H , Alekseyenko AV , Hayes RB , Ahn J ((2016) ) Cigarette smoking and the oral microbiome in a large study of American adults. ISME J 10: , 2435–2446. |
[111] | Jia Y-J , Liao Y , He Y-Q , Zheng M-Q , Tong X-T , Xue W-Q , Zhang J-B , Yuan L-L , Zhang W-L , Jia W-H ((2021) ) Association between oral microbiota and cigarette smoking in the Chinese population. Front Cell Infect Microbiol 11: , 658203. |
[112] | Sheiham A , James WPT ((2014) ) A new understanding of the relationship between sugars, dental caries and fluoride use: Implications for limits on sugars consumption. Public Health Nutr 17: , 2176–2184. |
[113] | Bergstrom J ((2014) ) Smoking rate and periodontal disease prevalence: 40-year trends in Sweden 1970–2010. J Clin Periodontol 41: , 952–957. |
[114] | Hardman RJ , Kennedy G , Macpherson H , Scholey AB , Pipingas A ((2016) ) Adherence to a Mediterranean-style diet and effects on cognition in adults: A qualitative evaluation and systematic review of longitudinal and prospective trials. Front Nutr 3: , 22. |
[115] | Kennedy G , Hardman RJ , Macpherson H , Scholey AB , Pipingas A ((2017) ) How does exercise reduce the rate of age-associated cognitive decline? A review of potential mechanisms. J Alzheimers Dis 55: , 1–18. |
[116] | Portugal EMM , Cevada T , Sobral Monteiro-Junior R , Teixeira Guimarães T , Da Cruz Rubini E , Lattari E , Blois C , Camaz Deslandes A ((2013) ) Neuroscience of exercise: From neurobiology mechanisms to mental health. Neuropsychobiology 68: , 1–14. |
[117] | Brown BM , Peiffer JJ , Taddei K , Lui JK , Laws SM , Gupta VB , Taddei T , Ward VK , Rodrigues MA , Burnham S , Rainey-Smith SR , Villemagne VL , Bush A , Ellis KA , Masters CL , Ames D , Macaulay SL , Szoeke C , Rowe CC , Martins RN ((2013) ) Physical activity and amyloid-β plasma and brain levels: Results from the Australian Imaging, Biomarkers and Lifestyle Study of Ageing. Mol Psychiatry 18: , 875–881. |
[118] | Lefèvre-Arbogast S , Wagner M , Proust-Lima C , Samieri C ((2019) ) Nutrition and metabolic profiles in the natural history of dementia: Recent insights from systems biology and life course epidemiology. Curr Nutr Rep 8: , 256–269. |
[119] | Thomas B , Smallwood S , Cutler C , Bescos R ((2019) ) The oral nitrate-reducing capacity correlates with peak power output and peak oxygen uptake in healthy humans. Nitric Oxide 87: , 43–51. |
[120] | Cutler C , Kiernan M , Willis JR , Gallardo-Alfaro L , Casas-Agustench P , White D , Hickson M , Gabaldon T , Bescos R ((2019) ) Post-exercisehypotension and skeletal muscle oxygenation is regulated bynitrate-reducing activity of oral bacteria. Free Radic BiolMed 143: , 252–259. |
[121] | Kapil V , Rathod KS , Khambata RS , Bahra M , Velmurugan S , Purba A , S. Watson D , Barnes MR , Wade WG , Ahluwalia A ((2018) ) Sex differences in the nitrate-nitrite-NO⋅ pathway: Role of oral nitrate-reducing bacteria. Free Radic Biol Med 126: , 113–121. |
[122] | Mailing LJ , Allen JM , Buford TW , Fields CJ , Woods JA ((2019) ) Exercise and the gut microbiome: A review of the evidence, potential mechanisms, and implications for human health. Exerc Sport Sci Rev 47: , 75–85. |
[123] | Bescos R , Brookes ZLS , Belfield LA , Fernandez-Sanjurjo M , Casas-Agustench P ((2020) ) Modulation of oral microbiota: A new frontier in exercise supplementation. PharmaNutrition 14: , 100230. |
[124] | Kivipelto M , Ngandu T , Laatikainen T , Winblad B , Soininen H , Tuomilehto J ((2006) ) Risk score for the prediction of dementia risk in 20 years among middle aged people: A longitudinal, population-based study. Lancet Neurol 5: , 735–741. |
[125] | Morris MC , Tangney CC , Wang Y , Sacks FM , Bennett DA , Aggarwal NT ((2015) ) MIND diet associated with reduced incidence of Alzheimer’s disease. Alzheimers Dement 11: , 1007–1014. |
[126] | Grant MM , Jonsson D ((2019) ) Next generation sequencing discoveries of the nitrate-responsive oral microbiome and its effect on vascular responses. J Clin Med 8: , 1110. |
[127] | Reddan JM , Macpherson H , White DJ , Scholey A , Pipingas A ((2019) ) Examining the relationship between nutrition and cerebral structural integrity in older adults without dementia. Nutr Res Rev 32: , 79–98. |
[128] | Burt BA , Pai S ((2001) ) Sugar consumption and caries risk: A systematic review. J Dent Educ 65: , 1017–1023. |
[129] | Esberg A , Haworth S , Hasslof P , Lif Holgerson P , Johansson I ((2020) ) Oral microbiota profile associates with sugar intake and taste preference genes. Nutrients 12: , 681. |
[130] | Kote S , Kote S , Nagesh L ((2011) ) Effect of pomegranate juice on dental plaque microorganisms (streptococci and lactobacilli). Anc Sci Life 31: , 49. |
[131] | Bhatia M , Urolagin SS , Pentyala KB , Urolagin SB , Menaka K , Bhoi S ((2014) ) Novel therapeutic approach for the treatment of periodontitis by curcumin. J Clin Diagn Res 8: , ZC65. |
[132] | Muñoz-González I , Thurnheer T , Bartolome B , Moreno-Arribas MV ((2014) ) Red wine and oenological extracts display antimicrobial effects in an oral bacteria biofilm model. J Agric Food Chem 62: , 4731–4737. |
[133] | Sánchez MC , Ribeiro-Vidal H , Esteban-Fernández A , Bartolomé B , Figuero E , Moreno-Arribas MV , Sanz M , Herrera D ((2019) ) Antimicrobial activity of red wine and oenological extracts against periodontal pathogens in a validated oral biofilm model. BMC Complement Altern Med 19: , 145. |
[134] | Esteban-Fernández A , Zorraquín-Peña I , Ferrer MD , Mira A , Bartolomé Ba , Gonzalez de Llano D , Moreno-Arribas MV ((2018) ) Inhibition of oral pathogens adhesion to human gingival fibroblastsby wine polyphenols alone and in combination with an oral probiotic. J Agric Food Chem 66: , 2071–2082. |
[135] | Adler CJ , Dobney K , Weyrich LS , Kaidonis J , Walker AW , Haak W , Bradshaw CJ , Townsend G , Soltysiak A , Alt KW , Parkhill J , Cooper A ((2013) ) Sequencing ancient calcified dental plaque shows changes in oral microbiota with dietary shifts of the Neolithic and Industrial revolutions, Nat Genet 45: , 450–455, 455e451. |
[136] | Adler CJ , Malik R , Browne GV , Norris JM ((2016) ) Diet may influence the oral microbiome composition in cats. Microbiome 4: , 23. |
[137] | Laiola M , Filippis FD , Vitaglione P , Ercolini D , McBain AJ ((2020) ) A Mediterranean diet intervention reduces the levels of salivary periodontopathogenic bacteria in overweight and obese subjects, Appl Environ Microbiol 86: , e00777–00720. |
[138] | Cattaneo C , Gargari G , Koirala R , Laureati M , Riso P , Guglielmetti S , Pagliarini E ((2019) ) New insights into the relationship between taste perception and oral microbiota composition. Sci Rep 9: , 3549. |
[139] | Xu W , Wang H , Wan Y , Tan C , Li J , Tan L , Yu J-T ((2017) ) Alcohol consumption and dementia risk: A dose– response meta-analysis of prospective studies. Eur J Epidemiol 32: , 31–42. |
[140] | Letenneur L ((2004) ) Risk of dementia and alcohol and wine consumption: A review of recent results. Biol Res 37: , 189–193. |
[141] | Sabia S , Fayosse A , Dumurgier J , Dugravot A , Akbaraly T , Britton A , Kivimäki M , Singh-Manoux A ((2018) ) Alcohol consumption and risk of dementia: 23 year follow-up of Whitehall II cohort study, BMJ 362: , k2927. |
[142] | Yussof A , Yoon P , Krkljes C , Schweinberg S , Cottrell J , Chu T , Chang SL ((2020) ) A meta-analysis of the effect of binge drinking on the oral microbiome and its relation to Alzheimer’s disease. Sci Rep 10: , 19872. |
[143] | Hamdi Z , Detzen L , Fessi S , Julia C , Hercberg S , Czernichow S , Boillot A , Touvier M , Bouchard P , Andreeva VA , Carra MC ((2021) ) Alcoholic beverage consumption, smoking habits, and periodontitis: Across-sectional investigation of the NutriNet-Santé study. J Periodontol 92: , 727–737. |
[144] | Pase MP , Himali JJ , Grima NA , Beiser AS , Satizabal CL , Aparicio HJ , Thomas RJ , Gottlieb DJ , Auerbach SH , Seshadri S ((2017) ) Sleep architecture and the risk of incident dementia in the community. Neurology 89: , 1244–1250. |
[145] | Yaffe K , Laffan AM , Harrison SL , Redline S , Spira AP , Ensrud KE , Ancoli-Israel S , Stone KL ((2011) ) Sleep-disordered breathing, hypoxia, and risk of mild cognitive impairment and dementia in older women. JAMA 306: , 613–619. |
[146] | Thaiss CA , Zeevi D , Levy M , Zilberman-Schapira G , Suez J , Tengeler AC , Abramson L , Katz MN , Korem T , Zmora N ((2014) ) Transkingdom control of microbiota diurnal oscillations promotes metabolic homeostasis. Cell 159: , 514–529. |
[147] | Smith RP , Easson C , Lyle SM , Kapoor R , Donnelly CP , Davidson EJ , Parikh E , Lopez JV , Tartar JL ((2019) ) Gut microbiome diversity is associated with sleep physiology in humans, PLoS One 14: , e0222394. |
[148] | Liu M , Wang X , Wu F , Dai N , Chen M , Yu J , Guan J , Li F ((2020) ) Variations of oral microbiome in chronic insomnia patients with different tongue features. Am J Chin Med 48: , 923–944. |
[149] | Yang W , Shao L , Heizhati M , Wu T , Yao X , Wang Y , Wang L , Li N ((2019) ) Oropharyngeal microbiome in obstructive sleep apnea: Decreased diversity and abundance. J Clin Sleep Med 15: , 1777–1788. |
[150] | Karp A , Kåreholt I , Qiu C , Bellander T , Winblad B , Fratiglioni L ((2004) ) Relation of education and occupation-based socioeconomic status to incident Alzheimer’s disease. Am J Epidemiol 159: , 175–183. |
[151] | Andel R , Crowe M , Pedersen NL , Mortimer J , Crimmins E , Johansson B , Gatz M ((2005) ) Complexity of work and risk of Alzheimer’s disease: A population-based study of Swedish twins, J Gerontol B Psychol Sci Soc Sci 60: , P251–P258. |
[152] | Stern Y , Barnes CA , Grady C , Jones RN , Raz N ((2019) ) Brain reserve, cognitive reserve, compensation, and maintenance: Operationalization, validity, and mechanisms of cognitive resilience. Neurobiol Aging 83: , 124–129. |
[153] | Seerig LM , Nascimento GG , Peres MA , Horta BL , Demarco FF ((2015) ) Tooth loss in adults and income: Systematic review and meta-analysis. J Dent 43: , 1051–1059. |
[154] | Costa SM , Martins CC , Bonfim MdLC , Zina LG , Paiva SM , Pordeus IA , Abreu MHNG ((2012) ) A systematic review of socioeconomic indicators and dental caries in adults. Int J Environ Res Public Health 9: , 3540–3574. |
[155] | Evans IEM , Martyr A , Collins R , Brayne C , Clare L ((2019) ) Social isolation and cognitive function in later life: A systematic review and meta-analysis, J Alzheimers Dis 70: , S119–S144. |
[156] | Byers AL , Yaffe K ((2011) ) Depression and risk of developing dementia. Nat Rev Neurol 7: , 323–331. |
[157] | Friedler B , Crapser J , McCullough L ((2015) ) One is the deadliest number: The detrimental effects of social isolation on cerebrovascular diseases and cognition. Acta Neuropathol 129: , 493–509. |
[158] | Singh-Manoux A , Dugravot A , Fournier A , Abell J , Ebmeier K , Kivimäki M , Sabia S ((2017) ) Trajectories of depressive symptoms before diagnosis of dementia: A 28-year follow-up study. JAMA Psychiatry 74: , 712–718. |
[159] | O’Neil A , Berk M , Venugopal K , Kim S-W , Williams LJ , Jacka FN ((2014) ) The association between poor dental health and depression: Findings from a large-scale, population-based study (the NHANES study). Gen Hosp Psychiatry 36: , 266–270. |
[160] | Simpson CA , Adler C , du Plessis MR , Landau ER , Dashper SG , Reynolds EC , Schwartz OS , Simmons JG ((2020) ) Oral microbiome composition, but not diversity, is associated with adolescent anxiety and depression symptoms. Physiol Behav 226: , 113126. |
[161] | Turner MD , Ship JA ((2007) ) Dry mouth and its effects on the oral health of elderly people. J Am Dent Assoc 138: , S15–S20. |
[162] | Dodds MW , Johnson DA , Yeh C-K ((2005) ) Health benefits of saliva: A review. J Dent 33: , 223–233. |
[163] | Delwel S , Binnekade TT , Perez RS , Hertogh CM , Scherder EJ , Lobbezoo F ((2017) ) Oral health and orofacial pain in older people with dementia: A systematic review with focus on dental hard tissues. Clin Oral Investig 21: , 17–32. |
[164] | Eguchi Y , Matsuda Y , Matsuoka T , Kato Y , Ueno D , Mimura M , Narumoto J ((2020) ) Ability of elderly women with cognitive decline in food preparation and related factors, Alzheimers Dement 16: , e042332. |
[165] | Naorungroj S , Slade GD , Beck JD , Mosley TH , Gottesman RF , Alonso A , Heiss G ((2013) ) Cognitive decline and oral health in middle-aged adults in the ARIC study. J Dent Res 92: , 795–801. |
[166] | Weijenberg RAF , Delwel S , Ho BV , van der Maarel-Wierink CD , Lobbezoo F ((2019) ) Mind your teeth— The relationship between mastication and cognition. Gerodontology 36: , 2–7. |
[167] | Grande G , Ljungman PLS , Eneroth K , Bellander T , Rizzuto D ((2020) ) Association between cardiovascular disease and long-term exposure to air pollution with the risk of dementia. JAMA Neurol 77: , 801–809. |
[168] | Chu Y-H , Kao S-W , Tantoh DM , Ko P-C , Lan S-J , Liaw Y-P ((2019) ) Association between fine particulate matter and oral cancer among Taiwanese men. J Investig Med 67: , 34–38. |
[169] | Yang TH , Masumi S , Weng SP , Chen HW , Chuang HC , Chuang KJ ((2015) ) Personal exposure to particulate matter and inflammation among patients with periodontal disease. Sci Total Environ 502: , 585–589. |
[170] | Heindel JJ , Vandenberg LN ((2015) ) Developmental origins of health and disease: A paradigm for understanding disease cause and prevention. Curr Opin Pediatr 27: , 248–253. |
[171] | Kivipelto M , Rovio S , Ngandu T , Kåreholt I , Eskelinen M , Winblad B , Hachinski V , Cedazo-Minguez A , Soininen H , Tuomilehto J ((2008) ) Apolipoprotein E ɛ4 magnifies lifestyle risks for dementia: A population-based study. J Cell Mol Med 12: , 2762–2771. |
[172] | Hou X-H , Feng L , Zhang C , Cao X-P , Tan L , Yu J-T ((2019) ) Models for predicting risk of dementia: A systematic review. J Neurol Neurosurg Psychiatry 90: , 373. |
[173] | Xu R , Wang Q ((2016) ) Towards understanding brain-gut-microbiome connections in Alzheimer’s disease. BMC Syst Biol 10: , 63–63. |
[174] | World Health Assembly (2021) Oral health. Seventy-Fourth World Health Assembly, A74/VR/7. https://apps.who.int/gb/ebwha/pdf_files/WHA74/A74_R5-en.pdf. |
[175] | Allin S , Farmer J , Quiñonez C , Peckham A , Marchildon G , Panteli D , Henschke C , Fattore G , Lamloum D , Holden ACL , Rice T ((2020) ) Do health systems cover the mouth? Comparing dental care coverage for older adults in eight jurisdictions. Health Policy 124: , 998–1007. |
[176] | Chen L , Cao H , Wu X , Xu X , Ji X , Wang B , Zhang P , Li H ((2022) ) Effects of oral health intervention strategies on cognition and microbiota alterations in patients with mild Alzheimer’s disease: A randomized controlled trial. Geriatr Nurs 48: , 99–106. |
[177] | Schwahn C , Frenzel S , Holtfreter B , Van der Auwera S , Pink C , Bülow R , Friedrich N , Völzke H , Biffar R , Kocher T ((2022) ) Effect of periodontal treatment on preclinical Alzheimer’s disease— Results of a trial emulation approach. Alzheimers Dement 18: , 127–141. |
[178] | Alzheimer’s Disease Anti-inflammatory Prevention Trial Research Group ((2013) ) Results of a follow-up study to the randomized Alzheimer’s Disease Anti-inflammatory Prevention Trial (ADAPT). Alzheimers Dement 9: , 714–723. |
[179] | Ryan J , Storey E , Murray AM , Woods RL , Wolfe R , Reid CM , Nelson MR , Chong TT , Williamson JD , Ward SA ((2020) ) Randomized placebo-controlled trial of the effects of aspirin on dementia and cognitive decline, Neurology 95: , e320–e331. |
[180] | Dawson SL , Finlay-Jones A , Ball L , Rocks T , Jacka F ((2022) ) Supporting maternal and child mental health through dietary changes focused on the gut microbiota. Psychiatr Ann 52: , 51–55. |
[181] | Major B , Rathbone JA , Blodorn A , Hunger JM ((2020) ) The countervailing effects of weight stigma on weight-loss motivation and perceived capacity for weight control. Pers Soc Psychol Bull 46: , 1331–1343. |
[182] | Naomi R , Embong H , Othman F , Ghazi HF , Maruthey N , Bahari H ((2021) ) Probiotics for Alzheimer’s disease: A systematic review. Nutrients 14: , 20. |
[183] | Maitre Y , Mahalli R , Micheneau P , Delpierre A , Amador G , Denis F ((2021) ) Evidence and therapeutic perspectives in the relationship between the oral microbiome and Alzheimer’s disease: A systematic review. Int J Environ Res Public Health 18: , 11157. |
[184] | Nascimento MM ((2017) ) Oral microbiota transplant: A potential new therapy for oral diseases. J Calif Dent Assoc 45: , 565–568. |
[185] | Green JE , Davis JA , Berk M , Hair C , Loughman A , Castle D , Athan E , Nierenberg AA , Cryan JF , Jacka F , Marx W ((2020) ) Efficacy and safety of fecal microbiota transplantation for the treatment of diseases other than Clostridium difficile infection: A systematic review and meta-analysis. Gut Microbes 12: , 1–25. |
[186] | Cammarota G , Ianiro G , Tilg H , Rajilić-Stojanović M , Kump P , Satokari R , Sokol H , Arkkila P , Pintus C , Hart A ((2017) ) European consensus conference on faecal microbiota transplantation in clinical practice. Gut 66: , 569–580. |
[187] | Trubiano JA , Cheng A , Korman T , Roder C , Campbell A , May M , Blyth C , Ferguson J , Blackmore T , Riley T ((2016) ) Australasian Society of Infectious Diseases updated guidelines for the management of Clostridium difficile infection in adults and children in Australia and New Zealand. Intern Med J 46: , 479–493. |