Preventing Loss of Independence through Exercise (PLIÉ): A Pilot Trial in Older Adults with Subjective Memory Decline and Mild Cognitive Impairment
Abstract
Background:
Preventing Loss of Independence through Exercise (PLIÉ) is a group movement program initially developed for people with mild-to-moderate dementia that integrates principles from several well-established traditions to specifically address the needs of people with cognitive impairment.
Objective:
To investigate whether PLIÉ would benefit cognitive and behavioral outcomes and functional brain connectivity in older adults with milder forms of cognitive impairment.
Methods:
Participants (≥55 y) with subjective memory decline (SMD) or mild cognitive impairment (MCI) were assessed with tests of cognitive and physical function, self-report questionnaires, and resting state functional magnetic resonance imaging (rs-fMRI) on a 3 Tesla scanner before and after participating in twice weekly PLIÉ classes for 12 weeks at the San Francisco Veterans Affairs Medical Center.
Results:
Eighteen participants completed the pre-post intervention pilot trial. We observed significant improvements on the Alzheimer’s Disease Assessment Scale cognitive subscale (ADAS-cog; effect size 0.34, p = 0.002) and enhanced functional connections between the medial prefrontal cortex (mPFC) and other nodes of the default mode network (DMN) after PLIÉ. Improvements (i.e., lower scores) on ADAS-cog were significantly correlated with enhanced functional connectivity between the mPFC and left lateral parietal cortex (Spearman’s ρ= –0.74, p = 0.001) and between the mPFC and right hippocampus (Spearman’s ρ= –0.83, p = 0.001). After completing PLIÉ, participants reported significant reductions in feelings of social isolation and improvements in well-being and interoceptive self-regulation.
Conclusion:
These preliminary findings of post-PLIÉ improvements in DMN functional connectivity, cognition, interoceptive self-regulation, well-being and reduced feelings of social isolation warrant larger randomized, controlled trials of PLIÉ in older adults with SMD and MCI.
INTRODUCTION
The prevalence of Alzheimer’s disease and related dementias (ADRD) has become a growing burden to individuals, families, and society. In the absence of efficacious pharmacological treatments that prevent, slow, or reverse cognitive decline associated with ADRD [1], it is important to investigate alternative forms of treatment. At the 2009 Summit on Integrative Medicine and the Health of the Public, the Institute of Medicine called for an integrative approach to health care, including a “seamless integration of resources to empower individuals to improve their health” [2]. We reflected this approach when we developed Preventing Loss of Independence through Exercise (PLIÉ), a group movement program that integrates principles from several well-established traditions including Feldenkrais Method®), Rosen Method, Tai Chi, and yoga, with elements from occupational therapy, physical therapy, and dance movement therapy.
PLIÉ is specifically designed to address the needs of people with cognitive impairment. The seven Guiding Principles of the program are: 1) repetition with variation (to promote procedural learning while maintaining engagement); 2) progressive, functional movement (to support basic functional movements such as standing safely from a seated position); 3) slow pace and step-by-step instruction (to enable participants to participate fully, experience feelings of success, and minimize cognitive demands); 4) participant-centered goal orientation (a goals assessment is performed at the beginning of the program, and instructors tailor content to address the personal interests and goals of participants); 5) body awareness, mindfulness and breathing (to bring non-judgmental awareness to the body in the present moment); 6) social interaction (participants sit in a circle, and many movements involve reaching across the circle to touch hands or elbows, or standing in a circle holding hands and moving together to facilitate social connection); and 7) positive emotions (participants are encouraged to move in ways that feel good, personally meaningful music is incorporated, and all classes end with sharing of appreciations and things that bring joy) [3, 4]. Consistent with the relationship-centered approach of integrative medicine [5], PLIÉ instructors are taught to incorporate these guiding principles in a flexible manner based on participants’ in-the-moment needs and experiences, rather than having a scripted “package of care.”
In a previous pilot study [4], we showed that PLIÉ produced potentially clinically meaningful (≥0.25 SDs) improvements on the Alzheimer’s Disease Assessment Scale, cognitive subscale (ADAS-cog), Short Physical Performance Battery (SPPB), and Quality of Life Scale in Alzheimer’s Disease (QOL-AD) in 12 patients with mild-to-moderate dementia. We also qualitatively analyzed the PLIÉ class videos and fieldnotes of the PLIÉ instructors [3]. Although not statistically significant, the combined quantitative and qualitative findings suggested that PLIÉ had potentially clinically meaningful changes. Because we did not have an opportunity to investigate the neural mechanisms underlying PLIÉ’s effect in our prior work [4], one goal of this study was to examine PLIÉ’s effect on brain function with resting-state functional magnetic resonance imaging (rs-fMRI).
PLIÉ was originally developed for people with dementia. However, research suggests that pharmacotherapy may be more effective if initiated earlier in the course of ADRD before neural damage has occurred [6]. While there has been no comparable research examining the impact of complementary and integrative therapies at different stages of ADRD, there is evidence that mind-body interventions can improve cognition and everyday functioning in older adults with mild cognitive impairment (MCI) [7–9]. Therefore, a second goal of this study was to investigate whether PLIÉ would similarly benefit people with milder forms of cognitive impairment. Because people with milder forms of cognitive impairment have less neurodegeneration than people with dementia, we posited that PLIÉ may have a greater likelihood of slowing cognitive decline in individuals with SMD and MCI than in people with dementia.
MCI is generally regarded as a transitory stage of cognitive decline between normal aging and dementia [10], although not every patient with MCI will progress to dementia [11]. There is also suggestive evidence that subjective reports of memory decline may be an important risk factor for future dementia or worsening of cognitive dysfunction [12], though psychological conditions such as anxiety, depression, and histrionic personality may also play a role in memory impairment [13]. We examined whether PLIÉ, which targets abilities that are relatively well-maintained in people with dementia (e.g., procedural memory, mindful body awareness, positive emotions, social connection) while minimizing cognitive demands, would also benefit people with MCI and subjective memory decline (SMD). Because MCI and SDM are different diagnoses, combining the two might increase the heterogeneity of the study sample. However, not everyone who experiences memory difficulties sees a neurologist for a formal diagnosis. Therefore, we felt that including individuals with SMD makes findings of the study more generalizable.
Extrapolating from our prior work on PLIÉ in people with dementia [4], we hypothesized that 12 weeks of PLIÉ would improve cognition in individuals with MCI and SMD. Based on neuroimaging findings from other mind-body [14, 15] and physical exercise [16] interventions in healthy older adults and individuals with MCI, we hypothesized that 12 weeks of PLIÉ would improve functional connectivity within the default mode network (DMN), a set of brain regions that are more active at rest in the “default” state [17–19]. DMN connectivity has been proposed to be a potentially useful noninvasive biomarker for assessing an intervention’s impact in adults with mild AD [20] because the DMN involves the same brain areas affected by cerebral atrophy, reduced metabolism, and amyloid in early AD [21].
METHODS
The study was approved by the institutional review boards at the University of California, San Francisco (UCSF), the San Francisco Veterans Affairs Medical Center (SFVAMC), and the Human-Subject Research Protection Organization at the Department of Defense. The study was designed as a single-arm, proof of concept, pre-post pilot trial and was registered with clinicaltrials.gov (NCT03526146). Data were collected at the SFVAMC from December 2018 to March 2020.
Participants
Study participants were recruited from August 2018 to December 2019 using online advertisements, flyers posted in the general community, and direct mail to MCI patients at the SFVAMC and UCSF. All participants provided informed consent and were paid for participation (up to $100 for completion of all study procedures).
Inclusion criteria were: 1) age ≥55 years; 2) diagnosis of MCI by a neurologist or primary care physician OR self-reporting of noticeable memory decline over the past year combined with objective cognitive impairment, as indicated by Montreal Cognitive Assessment (MoCA) [22] scores of 26 or lower; 3) English fluency; 4) ambulatory (e.g., able to take 2 steps without cane or walker); 5) willingness and ability to undergo 3 Tesla magnetic resonance imaging (MRI); 6) willingness and ability to participate in twice weekly hour-long PLIÉ classes at the San Francisco VA Medical Center for 12 weeks; and 7) living in the community in a private home or apartment.
Exclusion criteria were: 1) behavioral or physical issues that would be disruptive or dangerous to self or others (e.g., active psychosis, drug abuse, severe behavioral issues) in the group class setting; 2) physical or mental health condition that would make participation in group class difficult (e.g., severe physical limitations, limited life expectancy); 3) contraindications to safely undergoing MRI scan (e.g., claustrophobia severe enough to prevent MRI examination, presence of ferrometallic objects in the body that would interfere with MRI examination and/or cause a safety risk such as pace makers, implanted stimulators, pumps, etc.); 4) history of structural brain lesions or stroke temporally related to the onset or worsening of cognitive impairment; 5) plans to travel or move during the study period that would result in missing a substantial number of the PLIÉ classes; 6) plans to start/change any psychotropic medication, including start dementia medication (e.g., cholinesterase inhibitor or memantine) during study period; 7) current participation in another research study; 8) evidence of dementia (e.g., MoCA score < 18).
Eighteen participants completed the pre- and post-intervention assessments and the 12-week intervention. Of the 18 study completers, five had MCI diagnoses while 13 had SMD with no formal MCI diagnosis. We administered the MoCA to 12 of the 13 study completers with SMD at baseline to confirm memory impairment (i.e., MoCA < 26) and absence of dementia (MoCA > 17). One participant with SMD was enrolled prior to the modification of our study protocol to include the MoCA. However, her baseline ADAS-cog score, 18.7, suggested cognitive impairment without dementia. The MoCA score of another participant with SMD was only recorded as being within the inclusion range (≤26 but > 18) without noting the actual value. However, his baseline ADAS-cog score, 10.7, was also suggestive of cognitive impairment without dementia.
Intervention
Details of the PLIÉ intervention have been described elsewhere [3, 4]. Briefly, two PLIÉ classes were conducted each week for 12 weeks. Every class began with greetings and a brief check-in to ensure that everyone felt welcomed and acknowledged. During this portion of class, participants had an opportunity to interact with each other as a group. Next participants, seated in a circle facing each other, engaged in mindful body awareness exercises that involved massaging or tapping all major body parts and breathing exercises. The goal was to bring participants into awareness of their bodies and breathing in the present moment, and to create a calming ritual that is repeated at the beginning and end of each class. Participants then engaged in a series of seated and/or standing exercises that focused on increasing capacity to perform movements needed for daily function, such as increasing range of motion, transitioning smoothly from sitting to standing, and balancing while standing and moving. The movement sequences built slowly in complexity over the course of the program and progressed from more seated movements at the beginning of the program to more standing movements later in the program. The exact proportion of sitting to standing exercises varied depending on the functional ability of individuals in the class. Some of the movements were designed for self-to-self interaction while others were designed for self-to-other interactions. The instructors also facilitated social interaction between the participants and directed the participants to engage with each other through the movements. Mindful, seated rest periods were integrated throughout the program. Occasionally, personally meaningful music was included in parts of the classes. Each class ended with breathing exercises and an invitation for participants to share what made them feel grateful, appreciative, or happy. The goal of this was to facilitate positive feelings of social connection and to provide participants with another opportunity to interact as a group. The hour-long PLIÉ classes were held twice weekly over 12 weeks at the SFVAMC. All of the PLIÉ classes were led by a team of two instructors so that the participants could easily see one of the two instructors while sitting in a circle. The instructors compiled a log of the exercises performed at each class. The PLIÉ class logs were presented and discussed at weekly team meetings.
PLIÉ instructors
The same two PLIÉ instructors taught three groups of PLIÉ participants from March 2019 to February 2020. The maximum number of participants per class was ten, although class size varied from class to class depending on attendance. The senior PLIÉ instructor (JAL) has been leading PLIÉ classes and training PLIÉ instructors for 10 years. She is certified in the Feldenkrais Method® of Somatic Education in the US and Europe and has had a private somatic practice for 17 years. She also has experience in teaching movement in both one-on-one and in group formats to senior citizens and non-ambulatory adults with cerebral palsy and other complex cognitive, orthopedic and neurological issues. The assistant PLIÉ instructor (AL) had a 200-hour yoga teacher training certification and a Master of Science in behavioral health.
PLIÉ instructor training and fidelity assessment
All PLIÉ instructors are experienced in teaching mind-body movement (e.g., Feldenkrais, Yoga) to groups, and then receive in-depth training in PLIÉ that consists of a minimum of 6 months of observation, mentorship, and supervision with a lead PLIÉ Instructor. In addition to learning specific movements and body awareness exercises, PLIÉ instructors are taught to engage participants in the group experience and to foster relationships and social connections among group members. Interpersonal connections and group cohesion are developed from the beginning with participants introducing themselves, with the instructors modeling for members acknowledgment of individuals’ contributions, including interactive movements that involve others, and inviting sharing of experiences and appreciations, especially at the end of each PLIÉ session. The PLIÉ Instructors strive to create a safe, convivial, learning environment through their embodiment of PLIÉ principles such as communication in non-critical ways, encouraging change through discovery or guidance rather than correction, creating an exploratory, playful environment, and offering and modeling a welcoming, caring, interpersonal, shared learning experience. Fidelity to the program was assessed by 1) having PLIÉ instructors provide a descriptive summary and complete a detailed checklist of movements taught after each class; and 2) having one of the PLIÉ creators (DB, WM, MC) attend one class per month and complete a fidelity checklist to ensure adherence to the guiding principles.
Measures
Participants were assessed prior to (baseline) and after (post-treatment) completing 12-weeks of PLIÉ (24 classes). The primary neuroimaging outcome was functional connectivity in DMN. The secondary imaging outcomes were total and hippocampal subfield volumes.
To be consistent with our prior work, the primary behavioral outcome was cognition, assessed with the ADAS-cog [23]. Secondary behavioral outcome measures included the SPPB [24], Geriatric Depression Scale [25], QOL-AD [26], and self-reported measures of interoceptive self-regulation (i.e., ability to regulate distress by attention to body sensations) and interoceptive attention (i.e., ability to sustain and control attention to body sensations). Interoceptive self-regulation and attention were assessed with portions of the Multidimensional Assessment of Interoceptive Awareness, Version 2 (MAIA-2) [27]. To avoid overburdening study participants with the entire 37-item MAIA-2 scale, we focused on two interoceptive measures that had previously been shown to respond to mindfulness interventions [28]. Self-regulation in particular has been shown to be a key element of psychological health and a main aspect at the mind-body interface that improves with approaches aiming to foster mindful bodily awareness [29]. Other secondary outcome measures included the PROMIS Social Isolation measure [30] and the Neuro-QoL [31], which was used to assess feelings of well-being and lower extremity function. Because the PROMIS Social Isolation and Neuro-QoL were added later to the study, we only have these measures for 12 of the 18 completers.
Image acquisition
Imaging data was acquired on a Siemens Skyra 3-Tesla scanner at the SFVAMC. Participants’ heads were positioned comfortably within a 32-channel head coil, and head motion was minimized with firm cushions. During the resting-state functional MRI (rs-fMRI) scans, participants were instructed to close their eyes and to remain awake. The rs-fMRI images were acquired for 8 minutes and 12 seconds with an echo-planar imaging (EPI) sequence using the following parameters: 180 time points, repetition time: 3 s; echo time: 30 ms; flip angle: 80° acquisition matrix: 64×64; number of slices = 48; slice thickness = 3.3 mm, spatial resolution = 3.3×3.3×3.3 mm3. We also acquired anatomic images (3-dimensional MPRAGE) with the following parameters: repetition time: 2.5 s; echo time: 2.98 ms; inversion time: 1100 ms, 1.0×1.0×1.0 mm3 resolution; acquisition matrix 256×256 with 1 mm thick contiguous slices) for co-registration with the rs-fMRI data and quantification of total and hippocampal subfield volumes. Arterial spin labeled magnetic resonance imaging (MRI) was also acquired; however, this report will focus on PLIÉ’s effect on DMN functional connectivity and hippocampal subfield volumes.
Image preprocessing
The rs-fMRI data were preprocessed using the CONN toolbox [32] (version 19c) running on MATLAB version 2014a (MathWorks, Inc., Natick, MA, USA). Slice timing was corrected based on slice order. Using the SPM software platform, fMRI data were realigned and normalized in accordance with the standard Montreal Neurological Institute (MNI) template. The ART scrubbing procedure (http://www.nitrc.org/projects/artifact_detect/) was applied to handle image artifacts due to head movement. Next, fMRI data were bandpass filtered at 0.008–0.09 Hz, and discerned from the signal contributions from cerebrospinal fluid, brain white matter, and micro head movement (six parameters). Finally, a Gaussian filter kernel (full width at half maximum = 8 mm) was applied to spatially smooth all functional images.
Image analysis
We used the CONN toolbox [32] to analyze functional connectivity, utilizing the DMN regions of interest (ROIs) defined a priori within the CONN toolbox. These include the medial prefrontal cortex (mPFC), posterior cingulate cortex (PCC), and lateral parietal (LP) regions bilateral. Although the a priori CONN DMN ROIs does not include the hippocampus (HP), we also performed seed to voxel analyses to probe functional connectivity between the HP and other nodes of the DMN. For individual analyses, we calculated Pearson’s correlation coefficients between the time-course of activation within the DMN ROIs, the HP and the time-courses of all other voxels. This generated a seed-to-voxel connectivity matrix where positive correlation coefficients indicated positive functional connectivity and negative correlation coefficients showed negative functional connectivity. First-level correlations were then Fisher-transformed and subjected to second-level tests of ROI-to-ROI connectivity within the CONN DMN ROIs and HP to examine treatment effects (baseline > post-PLIÉ and post-PLIÉ > base). Clusters were defined by applying a high t-threshold of p < 0.001 (uncorrected) to individual voxels. For the extracted clusters, the threshold was p < 0.05. The false discovery rate (FDR) was used to correct the results. Independent tables of connectivity were obtained with the CONN DMN ROIs and hippocampus as targets. The following parameters were computed for each ROI pair: T-value, as well as uncorrected and corrected (FDR) p-values. Only pairs with p < 0.05 FDR corrected were accepted, which resulted in a minimum T-value of 2.90. Resting-state fMRI data from one participant was excluded from analysis due to excessive motion artifact at the baseline assessment. Additionally, we extracted first-level connectivity values from the DMN and hippocampal ROIs to examine the relationship between connectivity and performance on the ADAS-cog by using Spearman’s correlation analyses in SPSS.
FreeSurfer [33] version 6.0 and FreeSurfer’s longitudinal stream [34] were used to process the structural MR images and to extract hippocampal subfield volumes. The FreeSurfer version 6.0 hippocampal segmentation algorithm predicts the location of hippocampal subregions by using a probabilistic atlas built from a combination of manual delineations of the hippocampal formation from 15 ultra-high-resolution (120μm3), ex vivo MRI scans showing definitive borders and manual annotations of surrounding subcortical structures from an independent dataset of 39 in vivo 1-mm T1 MRI scans. We visually checked for overestimation or underestimation of GM/WM matter boundaries and to identify brain areas erroneously excluded during skull stripping. We also checked that the hippocampal subregion masks were well positioned. For the current study, we focused on volumes of CA1, CA2/3, CA4, dentate gyrus, subiculum, and presubiculum. Total hippocampal volume was derived by summing the subregions and the tail of the hippocampus. Volumes of the left and right total hippocampus and hippocampal subregions were combined.
Statistical analysis
Statistical Analyses were conducted using IBM SPSS Statistics for Windows, Version 26.0 (Armonk, NY: IBM Corp). Descriptive statistics were summarized by presenting the number and percentage for categorical variables and mean and standard deviation (SD) for continuous variables. Shapiro-Wilks testing indicated that many of the behavioral outcome measures were non-normally distributed. Therefore, pre- to post-treatment effects on behavioral measures were evaluated with the Wilcoxon signed-rank test. Because Shapiro-Wilks tests indicated that total hippocampal and hippocampal subfield volumes were normally distributed, paired t-tests were used to evaluate pre- to post-treatment effects on hippocampal volumes. Within-group effect sizes were calculated by subtracting pre-PLIÉ from post-PLIÉ values and dividing by the baseline SD.
This pilot trial was designed as a “proof of concept” study to generate hypotheses to be tested in future, fully powered trials. According to the Oxford English Dictionary, “proof of concept” is a phrase attributable to “evidence, usually deriving from an experiment or pilot project, demonstrating that a design concept, business idea, etc., is feasible.” For this reason, we did not adjust for multiple comparisons. However, we included p-values to indicate the likelihood that the results for any single test are due to chance. To examine the relationship between the ADAS-cog scores and the strength of the connections between DMN ROIs, we extracted first-level connectivity values from the DMN ROIs and subjected these to Spearman’s correlation analyses in SPSS.
RESULTS
Figure 1 presents the Consolidated Standards of Reporting Trials (CONSORT) flow. Table 1 summarizes the demographic and clinical characteristics of the participants. Forty-one participants were consented for the study and 33 participants completed baseline (pre-PLIÉ) assessments. There were no significant demographic differences between participants who did and did not complete the baseline assessment.
Fig. 1
Flow diagram of participants through trial.
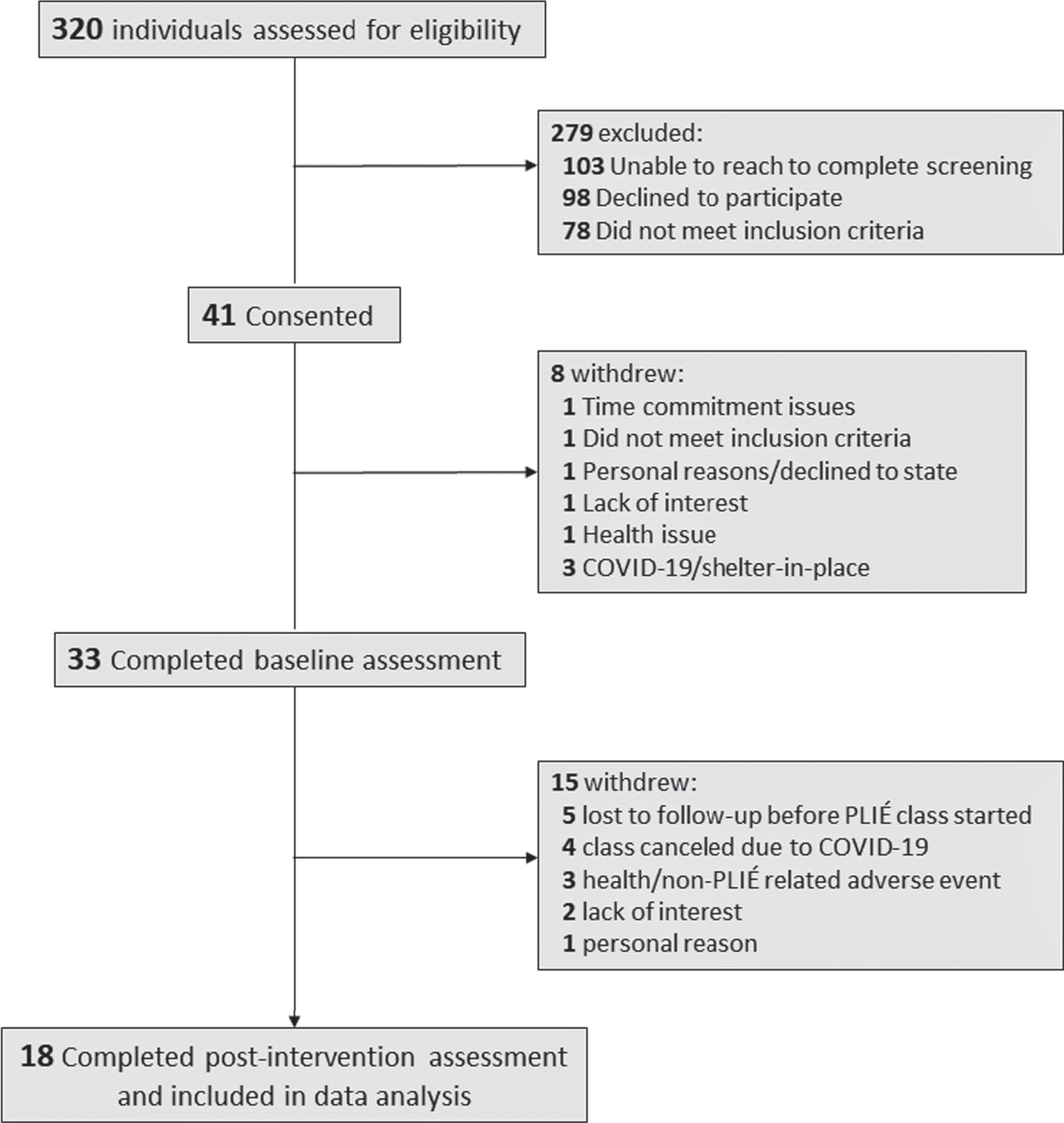
Table 1
Sociodemographic Characteristics of Study Completers
Screened for eligibility1 | Completed Screening | Enrolled in Study | Completed Study | |
N | 320 | 139 | 41 | 18 |
Sex, no. (%) | ||||
Male | 232 (73%) | 85 (61%) | 26 (63%) | 11 (61%) |
Female | 88 (28%) | 54 (39%) | 15 (37%) | 7 (39%) |
MCI diagnosis, no. (%) | – | 40 (29%) | 10 (24%) | 5 (28%) |
SMD, no. (%) | – | 87 (63%) | 31 (76%) | 13 (72%) |
Age, mean (SD) | – | 72.0 (11.0) | 73.1 (9.8) | 75.6 (9.1) |
Education, mean (SD), y | – | 15.7 (2.8) | 15.5 (2.1) | 14.8 (2.5) |
Race, no. (%) | ||||
Caucasian | – | 99 (71%) | 31 (76%) | 16 (89%) |
Asian/Pacific Islander | – | 10 (7%) | 4 (10%) | 2 (11%) |
Hispanic or Latino | – | 14 (10%) | 1 (2%) | 1 (6%) |
African American | – | 18 (13%) | 5 (12%) | 0 (0%) |
Other | – | 16 (12%) | 4 (10%) | 2 (11%) |
Marital status, no. (%) | ||||
Single | – | 25 (18%) | 7 (17%) | 4 (22%) |
Married/partnered | – | 62 (45%) | 20 (49%) | 9 (50%) |
Divorced | – | 29 (21%) | 6 (15%) | 1 (6%) |
Widowed | – | 23 (17%) | 8 (20%) | 4 (22%) |
Veterans, no. (%) | – | 68 (49%) | 22 (54%) | 9 (50%) |
MCI diagnosis, no. (%) | – | 51 (37%) | 16 (39%) | 5 (28%) |
TBI history2, no. (%) | – | 51 (37%) | 16 (39%) | 3 (17%) |
Baseline MoCA, mean (SD)2 | – | – | 24.0 (1.9)3 | 24.2 (2.0)4 |
SD, standard deviation; MCI, mild cognitive impairment; SMD, subjective memory decline; TBI, traumatic brain injury; MoCA, Montreal Cognitive Assessment 1181 individuals did not complete the telephone eligibility screening either because they were not interested in or were ineligible for the study. Thus, there is no demographic information about these individuals other than their sex. 2determined by Ohio State University Traumatic Brain Injury (OSU-TBI) Identification Method. 3data unavailable for 1 participant with SMD; the scores of the other 5 SMD participants were only recorded as being in the inclusion range (≤26 and > 18). 4data unavailable for 1 participant; the score for the other participant was recorded only as being in the inclusion range.
Treatment attrition
Eighteen of the 33 participants who completed baseline assessments also completed the intervention and post-PLIÉ assessments. These participants attended an average of 20±3 PLIÉ classes (range 14–24). Five participants dropped out after the baseline assessment and did not attend any PLIÉ classes. Six participants dropped out during the intervention, three for non-PLIÉ related health emergencies, three for other/personal reasons (e.g., lack of interest in the intervention or inability to commit to study schedule). Four participants were enrolled in a PLIÉ group that was subsequently canceled due to COVID-19. There were no significant demographic differences between the 18 study completers and the participants who did not complete the intervention. Five of the 18 study completers had MCI diagnoses, 13 had SMD.
Primary outcome measures
12-week change in ADAS-cog
Figure 2 summarizes the overall ADAS-cog score and scores of the objective (i.e., subject completed) ADAS-cog subcomponents at baseline and post-PLIÉ. On average, study completers showed a 2.8±3.0 point improvement on the overall ADAS-cog score after the intervention (pre-post PLIÉ effect size [ES]: 0.34, p = 0.002). Post-hoc examination of the objective ADAS-cog subcomponents revealed significant improvements on verbal episodic memory (i.e., immediate and delayed word recall).
Fig. 2
Total ADAS-cog scores and ADAS-cog sub-components at pre- (blue) and post- (red) PLIÉ in the 18 study completers.
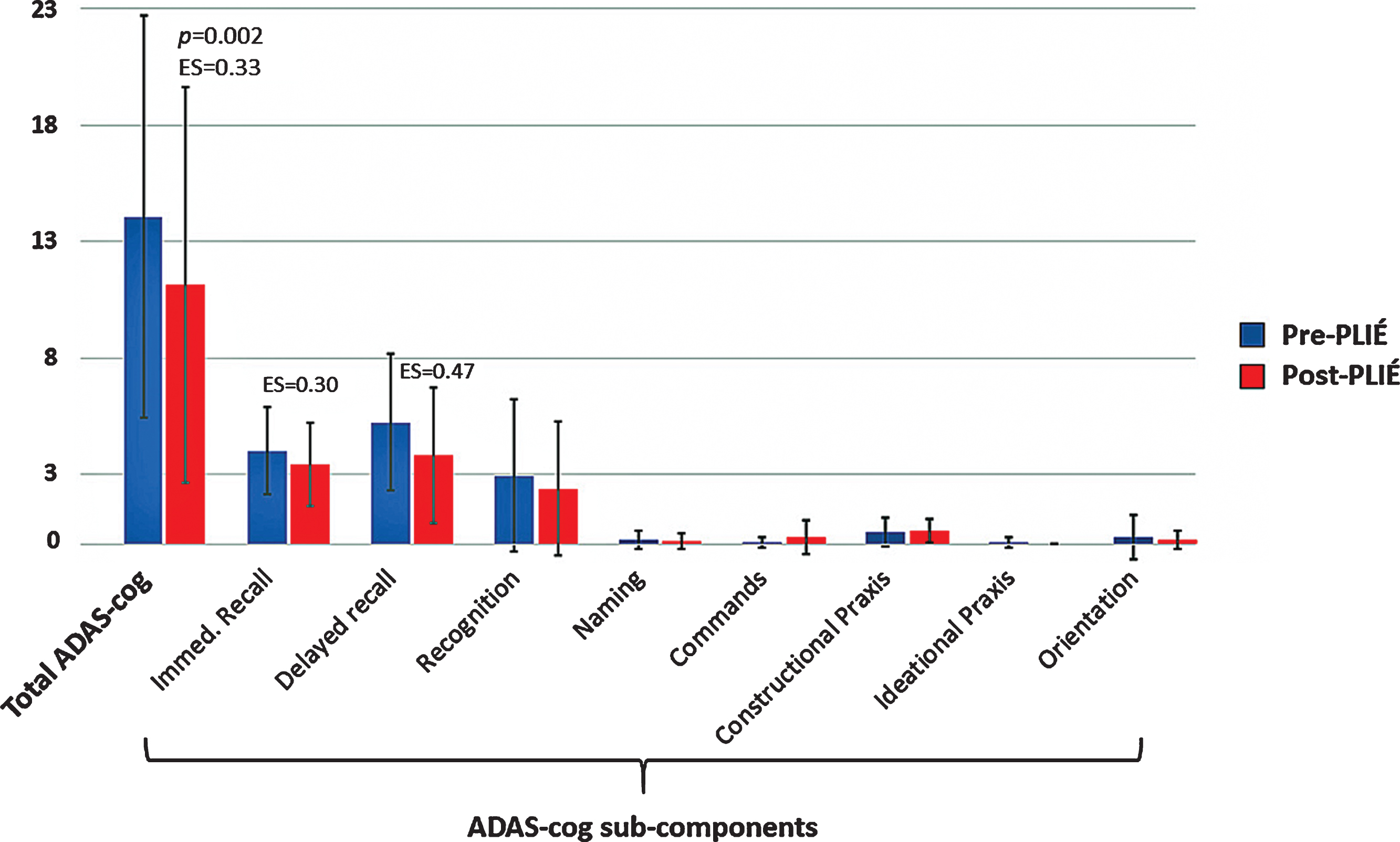
12-week change in DMN functional connectivity
After 12 weeks of PLIÉ, the connections between nodes of the DMN got stronger (pre-PLIÉ: F2,15 = 33.63, pFDR = 0.00002, post-PLIÉ: F2,15 = 55.73, pFDR = 0.000002). Table 2 summarizes the change in the strength of the connections between nodes of the DMN pre- and post-PLIÉ. At baseline, the mPFC was not functionally connected with other nodes of the DMN. However, after 12 weeks of PLIÉ, the mPFC was functionally connected with the PCC, left LP and right HP nodes of the DMN (see Fig. 3). Post-PLIÉ, the strength of the connection between the mPFC and right HP and between the mPFC and left LP were significantly correlated with better performance (i.e., lower scores) on the ADAS-cog (see Fig. 4) as well as verbal memory subscores of the ADAS-cog.
Table 2
Strength of functional connectivity within DMN nodes pre- and post-PLIÉ
Connection | Pre-PLIÉ | Post-PLIÉ | ||
t(16) | pFDR | t(16) | pFDR | |
1. RHP & LHP | 8.54 | 0.000001 | 8.22 | 0.000002 |
2. RLP & LLP | 7.44 | 0.000007 | 10.46 | < 0.000001 |
3. PCC & RLP | 6.03 | 0.000004 | 7.18 | 0.000001 |
4. PCC & LLP | 6.50 | 0.000002 | 8.15 | 0.000001 |
5. PCC & LHP | 3.56 | 0.004 | – | – |
6. mPFC & PCC | – | – | 2.26 | 0.06 |
7. mMPFC & LLP | – | – | 3.21 | 0.005 |
8. mPFC & RHP | – | – | 3.09 | 0.02 |
R, right; L, left; H, hippocampus; LP, lateral parietal; PCC, posterior cingulate cortex; mPFC, medial prefrontal cortex.
Fig. 3
Change in default mode network (DMN) connectivity before and after PLIÉ. The numbers on the connections correspond to numbers denoting the connections in Table 2. RHP, right hippocampus; LHP, left hippocampus; RLP, right lateral parietal; LLP, left lateral parietal; PCC, posterior cingulate cortex; mPFC, medial prefrontal cortex.
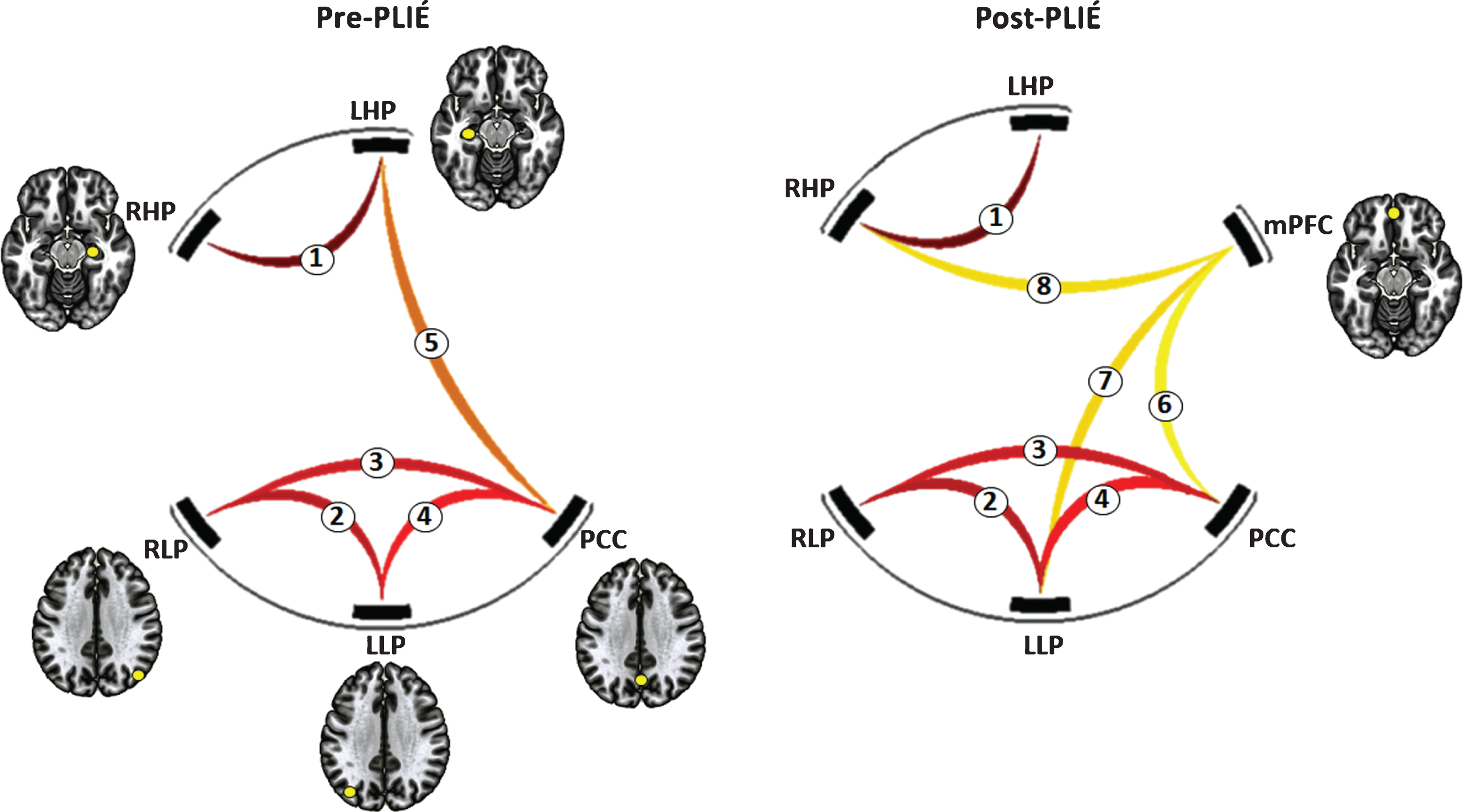
Fig. 4
Relationship between ADAS-cog scores and the strength of connection between the medial prefrontal cortex (mPFC) and (A) left lateral parietal cortex (LLP), connection #7 in Fig. 3, and (B) the right hippocampus (RHL), connection #8 in Fig. 3, after PLIÉ. Prior to PLIÉ, there were no significant correlations between ADAS-cog scores and the strength of these connections. Red circles represent participants with MCI.
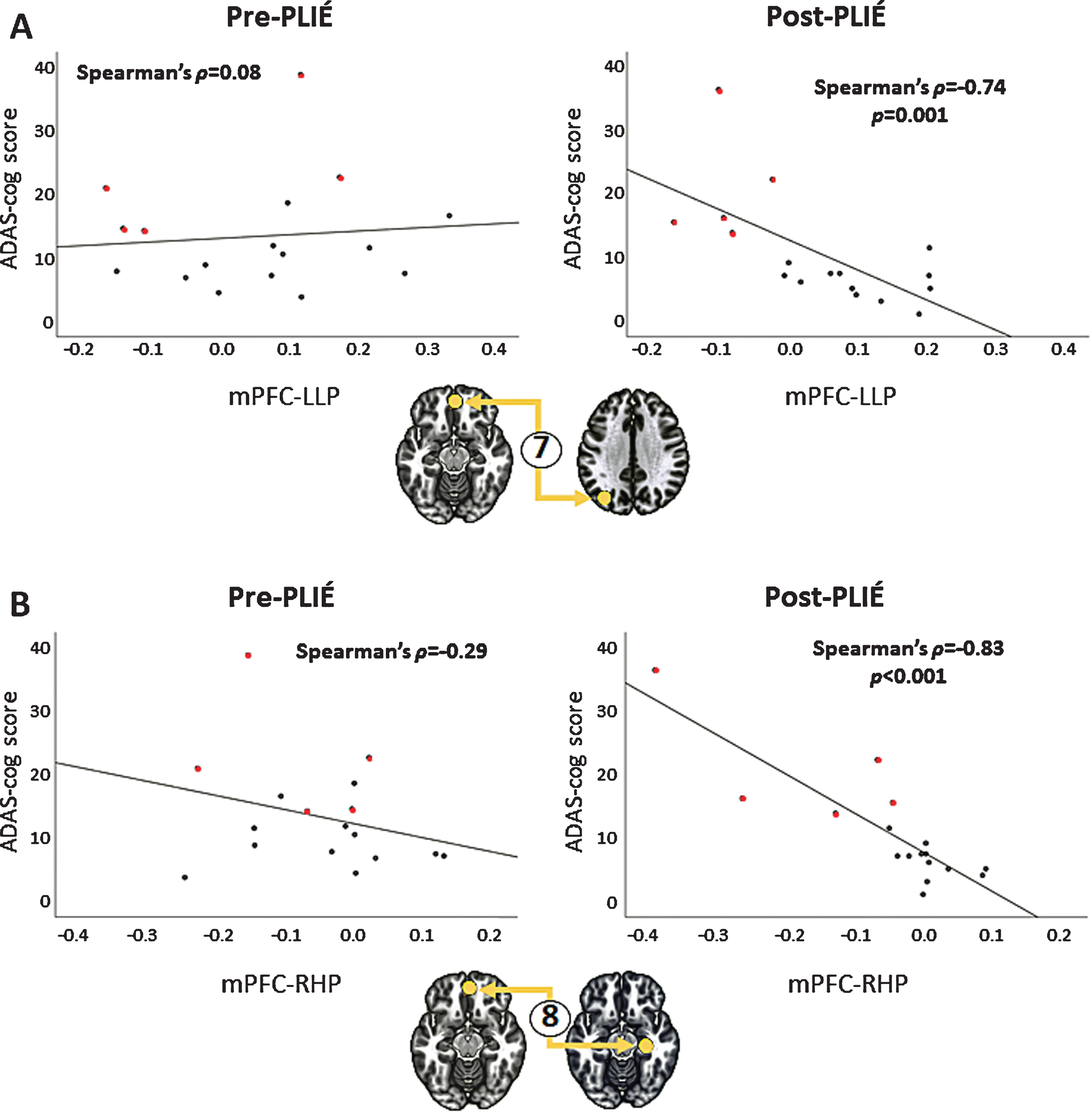
Secondary outcome measures
Table 3 summarizes secondary behavioral and imaging outcome measures pre- and post-PLIÉ. After 12 weeks of PLIÉ, there were significant improvements on self-reported measures of interoceptive self-regulation (ES = –0.72, p = 0.005), feelings of social isolation (ES = 0.70, p = 0.02), and well-being (ES = –0.56, p = 0.03). There were also trends of improvement in physical function, quality of life, interoceptive attention regulation and larger total hippocampal CA4 and dentate gyrus volumes after 12 weeks of PLIÉ.
Table 3
Correlation (Spearman’s ρ) between strength of DMN functional connectivity (β) and ADAS-cog total and memory subcomponent scores
ADAS-cog | Immediate Recall | Delayed Recall | ||||
Connection | Pre-PLIÉ | Post-PLIÉ | Pre-PLIÉ | Post-PLIÉ | Pre-PLIÉ | Post-PLIÉ |
1. RHP &LHP | –0.03 | –0.19 | 0.20 | –0.04 | –0.09 | –0.22 |
2. RLP &LLP | –0.02 | –0.24 | 0.13 | –0.04 | 0.06 | –0.11 |
3. PCC &RLP | 0.42 | 0.24 | 0.44 | 0.36 | 0.38 | 0.29 |
4. PCC &LLP | 0.22 | –0.36 | 0.17 | –0.18 | 0.34 | –0.30 |
5. PCC &LHP | 0.01 | –0.13 | –0.02 | –0.13 | –0.03 | 0.01 |
6. mPFC &PCC | –0.21 | –0.25 | –0.06 | –0.22 | –0.09 | –0.26 |
7. mPFC &LLP | 0.08 | –0.74a | –0.14 | –0.72a | –0.02 | –0.69b |
8. mPFC &RHP | –0.29 | –0.83c | –0.30 | –0.84d | –0.16 | –0.80e |
R, right; L, left; H, hippocampus; LP, lateral parietal; PCC, posterior cingulate cortex; mPFC, medial prefrontal cortex ap = 0.001, bp = 0.002, cp = 0.00004, dp = 0.00003, ep = 0.0001.
Table 4
Pre- and Post-PLIÉ effects on secondary behavioral and imaging outcome measures
Pre-PLIÉ (SD) | Post-PLIÉ (SD) | Effect size (95%CI) | p | |
Behavioral Measures | ||||
MAIA-2 Self-regulation | 3.3 (1.4) | 4.1 (0.7) | –0.72 (–1.40, –1.07) | 0.005c |
MAIA-2 Attention Regulation | 3.1 (1.3) | 3.6 (0.8) | –0.46 (–0.20, 1.25) | 0.07c |
PROMIS Social Isolationa,b | 9.6 (2.7) | 7.9 (2.1) | 0.70 (–0.12, 1.80) | 0.02c |
NeuroQOL- Well Beinga | 34.7 (4.6) | 37.2 (4.3) | –0.56 (–1.38, –0.68) | 0.03c |
NeuroQOL- Lower Extremity Functiona | 38.0 (2.1) | 37.2 (3.2) | 0.30 (–0.51, 0.99) | 0.13c |
SPPB | 8.6 (2.1) | 9.4 (2.3) | –0.36 (–1.02, –0.38) | 0.06c |
QOL-AD | 35.6 (6.0) | 37.2 (6.2) | –0.26 (–0.92, –0.18) | 0.08c |
GDSb | 3.8 (2.5) | 3.1 (2.8) | 0.26 (–0.39, 0.85) | 0.11c |
Total and Hippocampal subfield volume (mm3) | ||||
Total Hippocampus | 6321.71 (1197.06) | 6369.19 (1234.85) | –0.04 (–0.69, 0.26) | 0.07d |
CA1 | 1177.54 (251.78) | 1187.58 (255.87) | –0.04 (–0.69, 0.26) | 0.14 |
CA3 | 379.06 (87.45) | 382.36 (91.43) | –0.04 (–0.69, 0.26) | 0.25 |
CA4 | 492.44 (116.66) | 497.41 (121.69) | –0.04 (–0.70, 0.25) | 0.08d |
Dentate Gyrus | 548.36 (125.28) | 554.73 (130.50) | –0.05 (–0.70, 0.24) | 0.05d |
Presubiculum | 547.82 (106.35) | 552.83 (111.61) | –0.05 (–0.70, 0.24) | 0.32 |
Subsiculum | 768.15 (161.03) | 774.69 (159.14) | –0.04 (–0.69, 0.25) | 0.23 |
MAIA-2, Multidimenstional Assessment of Interoceptive Awareness 2, SPPB, Short Physical Performance Battery; QOL-AD, Quality of Life Alzheimer’s Disease; GDS, Geriatric Depression Scale aData available for 12 participants because measure was not implemented in first PLIÉ group blower score = better outcome cp-value calculated using Wilcoxon signed-rank test dp-value calculated from paired t-test.
DISCUSSION
This proof-of-concept trial examined the effects of a novel group movement intervention (i.e., PLIÉ) that integrates principles from several well-established traditions on cognitive and brain function in a group of older adults who did not meet criteria for dementia but were experiencing symptoms of cognitive decline. Consistent with our hypothesis, cognition in individuals with SMD (72%of the study sample) and MCI (28%of the study sample) did show an improvement after 12 weeks of PLIÉ as measured by the ADAS-cog. Although it has been proposed that the ADAS-cog may have limitations in more mildly impaired individuals, who tend to have scores close to the ceiling [35], we observed improvements in this study. This suggests that the ADAS-cog may have value in older adults with SMD and MCI. This finding adds to our previous work [4] and suggest that older adults with milder forms of cognitive impairment may also benefit from PLIÉ. Consistent with our hypothesis that 12 weeks of PLIÉ would improve DMN functional connectivity, we found increased functional connectivity after 12-weeks of PLIÉ that was correlated with better ADAS-cog scores. This finding is in line with other studies that have reported associations between increased DMN functional connectivity and better cognitive function [36–39].
The DMN is a network of interconnected brain regions that shows synchronized activity when the brain is at-rest and decreased activity when the subject is engaged in external tasks [17, 40]. Nodes of the DMN include the mPFC, PCC, LP, and medial temporal lobe structures including the HP [41, 42]. Consistent with reports that aging disrupts connectivity between the anterior and posterior nodes of the DMN [37, 43–46], we observed that the mPFC was not functionally connected with other nodes of the DMN at baseline in our participants, who were, on average, 76 years old. However, after 12 weeks of PLIÉ, the mPFC showed higher functional connectivity with the PCC, right HP, and left LP nodes of the DMN. Moreover, the strength of the connection between the mPFC and the right HP and between the mPFC and left LP were significantly correlated with ADAS-cog total and memory subscores post-PLIÉ. This is reminiscent of other studies that have reported significant relationships between connectivity of anterior to posterior nodes of the DMN and memory performance [46].
Our findings are also consistent with previous reports of increased DMN functional connectivity [47] and hippocampal networks [48] after aerobic training in healthy older adults and of improved cognition in individuals with MCI [49–52]. In a study that examined the effects of 12 weeks of aerobic walking exercise on DMN functional connectivity in older adults with MCI, Chirles et al. [16] reported increased functional connectivity between the PCC/precuneus and other DMN regions, including the left inferior parietal lobe. We also observed increased connectivity between the PCC and bilateral LP after 12 weeks of PLIÉ. Our results suggest that movement exercises that are not aerobic per se, but which focus on training procedural memory for basic functional movements and are designed to increase mindful body awareness and facilitate social connection provide (core principles of PLIÉ), also benefit individuals with SMD and MCI.
Our findings are also similar to those of a pilot study that examined the effects of mindfulness-based stress reduction (MBSR) versus usual care in individuals with MCI [14]. Although Wells and colleagues did not find significant improvements in ADAS-cog scores after 8 weeks of MBSR, they did report enhanced DMN connectivity between the PCC, mPFC, and HP after 8 weeks of MBSR compared to usual care [14]. We also observed trends of increased total hippocampal volume and increased CA4/dentate gyrus volume post-PLIÉ. Notably, the subgranular zone of the dentate gyrus is one of the two areas in the adult brain where neurogenesis occurs [53]. Interestingly, Wells et al. also found trends of reduced hippocampal atrophy in the MSBR group. In addition to elements of physical exercise, PLIÉ includes mindful body movements and mindful breathing. Thus, our findings are in line with prior reports of increased hippocampal volume after physical exercise [54] and MBSR [55] interventions. PLIÉ and MSBR also share other common elements such as weekly time commitment and attention from and interactions with an instructor.
The mindful body movements and breathing that are part of PLIÉ draw on meditation practices such as a form of moving meditation called brain-wave vibration meditation, which was designed to help quiet the mind and to release negative emotions through performing rhythmic movements and focusing on bodily sensations [56]. In a study that examined DMN functional connectivity, Jang et al. [56] reported that practitioners of brain-wave vibration meditation had increased functional connectivity within the DMN, particularly in the mPFC, compared to meditation-naïve control subjects.
PLIÉ also includes elements from yoga, the ancient mind-body practice that incorporates breathing, exercise, and meditation techniques. In a study with older adults with MCI, Eyre et al. [15] reported that yoga and a memory enhancement training program both improved verbal memory performance in participants with MCI. Furthermore, the improvements in verbal memory scores were correlated with increased functional connectivity between the DMN and frontal medial cortex after yoga and memory training. Although both memory training and yoga enhanced memory performance, Eyre et al. [57] found that only the yoga intervention also improved executive function, depressive symptoms, and resilience in older adults with MCI. The measure of “resilience” assessed by Eyre et al. [57] may be viewed as a measure of the ability to cope with stress, which is somewhat similar to the self-regulation measure that we examined with the MAIA-2 questionnaire [27]. A sample item of the MAIA-2 self-regulation scale is “When I feel overwhelmed, I can find a calm place inside.” Notably, there were significant post-PLIÉ improvements on the MAIA-2 measure of interoceptive self-regulation, as well as significant post-PLIÉ reductions in feelings of social isolation (PROMIS), and enhanced feelings of well-being (NeuroQOL). All of these may be construed as improved mood/reduced depression post-PLIÉ.
Although we hypothesized that PLIÉ may have a greater likelihood of slowing cognitive decline in individuals with less neurodegeneration (e.g., people with SMD and MCI) than people with dementia, our prior studies suggest that PLIÉ has beneficial effects in people at all stages of cognitive decline/neurodegeneration. Specifically, we have found that PLIÉ improves quality of life in people with dementia [4, 58, 59], and we have successfully delivered PLIÉ to individuals with dementia in multiple settings (e.g., adult day centers, community settings, nursing homes). PLIÉ is highly flexible and may be adapted to suit the needs of participants with different levels of cognitive and functional impairment.
This pilot trial had some limitations that should be noted. First, we did not have an active control group. Therefore, we cannot rule out the possibility that practice, context, or placebo effects may have contributed to the behavioral findings and self-report measures. However, it is more difficult to argue that the post-PLIÉ changes we observed on DMN functional connectivity and the trends of post-PLIÉ increases in hippocampal volume can be attributed to practice or placebo effects. Second, because the study only had one arm, the assessors were unblinded, which may have led to potential biases. A third limitation is that we performed numerous statistical tests without adjusting for multiple comparisons; therefore, there is a potential of false positives. The reason why we did not adjust for multiple comparison was because this was meant to be an initial, exploratory proof-of concept trial to generate hypotheses for future, fully powered trials. Therefore, we followed the general recommendations for exploratory pilot studies [60]. Another limitation is that with only 18 completers, these findings are preliminary and need confirmation through larger, controlled studies. Also, because only 18 of the 33 enrolled participants who completed baseline assessments, completed the intervention and the post-intervention assessments, it is conceivable that the study completers represent a self-selected group of individuals who noticed the benefits of PLIÉ and followed through with the program. Five participants who dropped out after completing the baseline assessment never attended any PLIÉ class. It is possible these five individuals were dissuaded by the commute to and from the SFVAMC, where the PLIÉ classes were held, unable/unwilling to commit to attending classes twice a week for 12 weeks, or only took part in baseline procedures for the $50 payment. Because these five participants never attended any PLIÉ classes, it is difficult to construe their dropping out as a reflection of the intervention. Three participants dropped out because of unanticipated medical emergencies. Only three participants dropped out after attending at least one PLIÉ class because they did not like the intervention or because of time commitment issues. When considered in this context, the dropout rate is comparable with many other intervention trials. A final limitation of the study is we did not follow-up with the study completers to investigate how long the benefits of PLIÉ can be maintained. These limitations notwithstanding, results from this proof-of-concept pilot trial provide preliminary evidence that PLIÉ may improve cognitive function, increase self-regulation, and reduce social isolation in people with MCI and SMD, and that the neurological mechanism of action may be increased DMN connectivity. Fully powered trials of PLIÉ are warranted to test this hypothesis.
ACKNOWLEDGMENTS
This work was supported by the Department of Defense U.S. Army Medical Research and Materiel Command through the Peer Reviewed Alzheimer’s Research Program Award No. W81XWH-17-1-0490. This material is the result of work supported with resources and the use of facilities at the San Francisco Veterans Affairs Health Care Center.
Authors’ disclosures available online (https://www.j-alz.com/manuscript-disclosures/21-0159r2).
REFERENCES
[1] | Moniz-Cook E , Vernooij-Dassen M , Woods B , Orrell M ((2011) ) Psychosocial interventions in dementia care research: The INTERDEM manifesto. Aging Ment Health 15: , 283–290. |
[2] | Institute of Medicine. ((2009) ) Integrative Medicine and the Health of the Public. A Summary of the February 2009 Summit. National Academies Press, Washington, D.C. |
[3] | Wu E , Barnes DE , Ackerman SL , Lee J , Chesney M , Mehling W ((2015) ) Preventing Loss of Independence through Exercise (PLIÉ): Qualitative analysis of a clinical trial in older adults with dementia. Aging Ment Health 19: , 353–362. |
[4] | Barnes DE , Mehling W , Wu E , Beristianos M , Yaffe K , Skultety K , Chesney MA ((2015) ) Preventing Loss of Independence through Exercise (PLIE): A pilot clinical trial in older adults with dementia. PLoS One 10: , e0113367. |
[5] | Rakle D ((2018) ) Integrative Medicine, Elsevier, Philadelphia, PA. |
[6] | Golde TE , Schneider LS , Koo EH ((2011) ) Anti-abeta therapeutics in Alzheimer’s disease: The need for a paradigm shift. Neuron 69: , 203–213. |
[7] | Farhang M , Miranda-Castillo C , Rubio M , Furtado G ((2019) ) Impact of mind-body interventions in older adults with mild cognitive impairment: A systematic review. Int Psychogeriatr 31: , 643–666. |
[8] | Brenes GA , Sohl S , Wells Re , Befus D , Campos CL , Danhuer SC ((2019) ) The effects of yoga on patients with mild cognitive impairment and dementia: A scoping review. Am J Geriatr Psychiatry 27: , 188–197. |
[9] | Lim KHL , Pysklywec A , Plante M , Demers L ((2019) ) The effectiveness of Tai Chi for short-term cognitive function improvement in the early stages of dementia in the elderly: A systematic literature review. Clin Interv Aging 14: , 827–839. |
[10] | Petersen RC , Caracciolo B , Brayne C , Gauthier S , Jelic V , Fratiglioni L ((2014) ) Mild cognitive impairment: A concept in evolution. J Intern Med 275: , 214–228. |
[11] | Pandya SY , Clem MA , Silva LM , Woon FL ((2016) ) Does mild cognitive impairment always lead to dementia? A review. J Neurol Sci 369: , 57–62. |
[12] | Reid LM , Maclullich AM ((2006) ) Subjective memory complaints and cognitive impairment in older people. Dement Geriatr Cogn Disord 22: , 471–485. |
[13] | Arbabi M , Zhand N , Eybpoosh S , Yazdi N , Ansari S , Ramezani M ((2015) ) Correlates of memory complaints and personality, depression, and anxiety in a memory clinic. Acta Med Iran 53: , 270–275. |
[14] | Wells RE , Yeh GY , Kerr CE , Wolkin J , Davis RB , Tan Y , Spaeth R , Wall RB , Walsh J , Kaptchuk T , Press D , Phillips RS , Kong J ((2013) ) Meditation’s impact on default mode network & hippocampus in mild cognitive impairment: A pilot study. Neurosci Lett 556: , 15–19. |
[15] | Eyre HA , Acevedo B , Yang H , Siddarth P , Van Dyk K , Ercoli L , Leaver AM , Cyr NS , Narr K , Baune BT , Khalsa DS , Lavretsky H ((2016) ) Changes in neural connectivity and memory following a yoga intervention for older adults: A pilot study. J Alzheimers Dis 52: , 673–684. |
[16] | Chirles TJ , Reiter K , Weiss LR , Alfini AJ , Nielson KA , Smith JC ((2017) ) Exercise training and functional connectivity changes in mild cognitive impairment and healthy elders. J Alzheimers Dis 57: , 845–856. |
[17] | Raichle ME , Snyder AZ ((2007) ) A default mode of brain function: A brief history of an evolving idea.1083-1090; discussion. Neuroimage 37: , 1097–1099. |
[18] | Greicius MD , Supekar K , Menon V , Dougherty RF ((2009) ) Resting-state functional connectivity reflects structural connectivity in the default mode network. Cereb Cortex 19: , 72–78. |
[19] | Mevel K , Chételat G , Eustache F , Desgranges B ((2011) ) The default mode network in healthy aging and Alzheimer’s disease. Int J Alzheimers Dis 2011: , 535816. |
[20] | Greicius MD , Srivastava G , Reiss AL , Menon V ((2004) ) Default-mode network activity distinguishes Alzheimer’s disease from healthy aging: Evidence from functional MRI. Proc Natl Acad Sci U S A 101: , 4637–4642. |
[21] | Buckner RL , Snyder AZ , Shannon BJ , LaRossa G , Sachs R , Fotenos AF , Sheline YI , Klunk WE , Mathis CA , Morris JC , Mintun MA ((2005) ) Molecular, structural, and functional characterization of Alzheimer’s disease: Evidence for a relationship between default activity, amyloid, and memory. J Neurosci 25: , 7709–7717. |
[22] | Nasreddine ZS , Phillips NA , Bédirian V , Charbonneau S , Whitehead V , Collin I , Cummings JL , Chertkow H ((2005) ) The Montreal Cognitive Assessment, MoCA: A brief screening tool for mild cognitive impairment. J Am Geriatr Soc 53: , 695–699. |
[23] | Rosen WG , Mohs RC , Davis KL ((1984) ) A new rating scale for Alzheimer’s disease. Am J Psychiatry 141: , 1356–1364. |
[24] | Guralnik JM , Simonsick EM , Ferrucci L , Glynn RJ , Berkman LF , Blazer DG , Scherr PA , Wallace RB ((1994) ) A short physical performance battery assessing lower extremity function: Association with self-reported disability and prediction of mortality and nursing home admission.M. J Gerontol 49: , 85–94. |
[25] | Yesavage JA , Brink TL , Rose TL , Lum O , Huang V , Adey M , Leirer VO (1982-1983) Development and validation of Geriatric Depression Rating Scale: A preliminary report. J Psychiatr Res 17: , 37–49. |
[26] | Logsdon RG , Gibbons LE , McCurry SM , Teri L ((2002) ) Assessing quality of life in older adults with cognitive impairment. Psychosom Med 64: , 510–519. |
[27] | Mehling WE , Acree M , Stewart A , Silas J , Jones A ((2018) ) The Multidimensional Assessment of Interoceptive Awareness, Version 2 (MAIA-2). PLoS One 13: , e0208034. |
[28] | Bornemann B , Herbert BM , Mehling WE , Singer T ((2015) ) Differential changes in self-reported aspects of interoceptive awareness through 3 months of contemplative training. Front Psychol 5: , 1504. |
[29] | Gawande R , To MN , Pine E , Griswold T , Creedon TB , Brunel A , Lozada A , Loucks EB , Schuman-Olivier Z ((2019) ) Mindfulness training enhances self-regulation and facilitates health behavior change for primary care patients: A randomized controlled trial. J Gen Intern Med 34: , 293–302. |
[30] | PROMIS Short Form v2.0 - Social Isolation 4a (2018). https://www.healthmeasures.net/index.php?option=com_instruments&view=measure&id=209 Accessed on June 1, 2019. |
[31] | Cella D , Lai JS , Nowinski CJ , Victorson D , Peterman A , Miller D , Bethoux F , Heinemann A , Rubin S , Cavazos JE , Reder AT , Sufit R , Simuni T , Holmes GL , Siderowf A , Wojna V , Bode R , McKinney N , Podrabsky T , Wortman K , Choi S , Gershon R , Rothrock N , Moy C ((2012) ) Neuro-QOL: Brief measures of health-related quality of life for clinical research in neurology. Neurology 78: , 1860–1867. |
[32] | Whitfield-Gabrieli S , Nieto-Castanon A ((2012) ) Conn: A functional connectivity toolbox for correlated and anticorrelated brain networks. Brain Connect 2: , 125–141. |
[33] | Dale AM , Fischl B , Sereno MI ((1999) ) Cortical surface-based analysis. I. Segmentation and surface reconstruction. Neuroimage 9: , 179–194. |
[34] | Reuter M , Schmansky NJ , Rosas HD , Fischl B ((2012) ) Within-subject template estimation for unbiased longitudinal image analysis. Neuroimage 61: , 1402–1418. |
[35] | Kueper JK , Speechley M , Montero-Odasso M ((2018) ) The Alzheimer’s Disease Assessment Scale–Cognitive Subscale (ADAS-Cog): Modifications and responsiveness in pre-dementia populations. A narrative review. J Alzeimers Dis 63: , 423–444. |
[36] | Damoiseaux JS , Beckmann CF , Arigita EJ , Barkhof F , Scheltens P , Stam CJ , Smith SM , Rombouts SA ((2008) ) Reduced resting-state brain activity in the “default network” in normal aging. Cereb Cortex 18: , 1856–1864. |
[37] | Andrews-Hanna JR , Snyder AZ , Vincent JL , Lustig C , Head D , Raichle ME , Buckner RL ((2007) ) Disruption of large-scale brain systems in advanced aging. Neuron 56: , 924–935. |
[38] | Esposito F , Bertolino A , Scarabino T , Latorre V , Blasi G , Popolizio T , Tedeschi G , Cirillo S , Goebel R , Di Salle F ((2006) ) Independent component model of the default-mode brain function: Assessing the impact of active thinking. Brain Res Bull 70: , 263–269. |
[39] | Hampson M , Driesen NR , Skudlarski P , Gore JC , Constable RT ((2006) ) Brain connectivity related to working memory performance. J Neurosci 26: , 13338–13343. |
[40] | Fox MD , Raichle ME ((2007) ) Spontaneous fluctuations in brain activity observed with functional magnetic resonance imaging. Nat Rev Neurosci 8: , 700–711. |
[41] | Buckner RL , Andrews-Hanna JR , Schacter DL ((2008) ) The brain’s default network: Anatomy, function, and relevance to disease. Ann N Y Acad Sci 1124: , 1–38. |
[42] | Yeo BT , Krienen FM , Sepulcre J , Sabuncu MR , Lashkari D , Hollinshead M , Roffman JL , Smoller JW , Zöllei L , Polimeni JR , Fischl B , Liu H , Buckner RL ((2011) ) The organization of the human cerebral cortex estimated by intrinsic functional connectivity. J Neurophysiol 106: , 1125–1165. |
[43] | Tomasi D , Volkow ND ((2012) ) Aging and functional brain networks. Mol Psychiatry 17: , 549–58. |
[44] | Campbell KL , Grigg O , Saverino C , Churchill N , Grady CL ((2013) ) Age differences in the intrinsic functional connectivity of default network subsystems. Front Aging Neurosci 5: , 73. |
[45] | Mevel K , Landeau B , Fouquet M , La Joie R , Villain N , Mézenge F , Perrotin A , Eustache F , Desgranges B , Chételat G ((2013) ) Age effect on the default mode network, inner thoughts, and cognitive abilities. Neurobiol Aging 34: , 1292–1301. |
[46] | Vidal-Piñeiro D , Vallas-Pedret C , Fernandez-Cabello S , Arenaza-Urquijo EM , Sala-Llonch R , Solana E , Bargallo N , Junque C , Ros E , Bartres-Fez D ((2014) ) Decreased default mode network connectivity correlates with age-associated structural and cognitive changes. Front Aging Neurosci 6: , 256. |
[47] | Voss MW , Prakash RS , Erickson KI , Basak C , Chaddock L , Kim JS , Alves H , Heo S , Szabo AN , White SM , Wójcicki TR , Mailey EL , Gothe N , Olson EA , McAuley E , Kramer AF ((2010) ) Plasticity of brain networks in a randomized intervention trial of exercise training in older adults. Front Aging Neurosci 2: , 32. |
[48] | Burdette JH , Laurienti PJ , Espeland MA , Morgan A , Telesford Q , Vechlekar CD , Hayasaka S , Jennings JM , Katula JA , Kraft RA , Rejeski WJ ((2010) ) Using network science to evaluate exercise-associated brain changes in older adults. Front Aging Neurosci 2: , 23. |
[49] | Baker LD , Frank LL , Foster-Schubert K , Green PS , Wilkinson CW , McTiernan A , Plymate SR , Fishel MA , Watson GS , Cholerton BA , Duncan GE , Mehta PD , Craft S ((2010) ) Effects of aerobic exercise on mild cognitive impairment: A controlled trial. Arch Neurol 67: , 71–79. |
[50] | Lautenschlager NT , Cox KL , Flicker L , Foster JK , van Bockxmeer FM , Xiao J , Greenop KR , Almeida OP ((2008) ) Effect of physical activity on cognitive function in older adults at risk for Alzheimer disease: A randomized trial. JAMA 300: , 1027–1037. |
[51] | Lautenschlager NT , Cox KL , Ellis KA ((2019) ) Physical activity for cognitive health: What advice can we give to older adults with subjective cognitive decline and mild cognitive impairment? Dialogues Clin Neurosci 21: , 61–68. |
[52] | Smith JC , Nielson KA , Woodard JL , Seidenberg M , Rao SM ((2013) ) Physical activity and brain function in older adults at increased risk for Alzheimer’s disease. Brain Sci 3: , 54–58. |
[53] | Benarroch EE ((2013) ) Adult neurogenesis in the dentate gyrus. General concepts and potential implications. Neurology 81: , 1443–1452. |
[54] | Erickson KI , Voss MW , Prakash RS , Basak C , Szabo A , Chaddock L , Kim JS , HeoS , Alves H , White SM , Wojcicki TR , Mailey E , Vieira VJ , Martin SA , Pence BD , Woods J , McAuley E , Kramer AF ((2011) ) Exercise training increases size of hippocampus and improves memory. Proc Natl Acad Sci U S A 108: , 3017–3022. |
[55] | Holzel J , Carmody M , Vangel C , Congleton SM , Yerramsetti T , Gard SW , Lazar SW ((2011) ) Mindfulness practice leads to increases in regional brain gray matter density. Psychiatry Res 191: , 36–43. |
[56] | Jang JH , Jung WH , Kang DH , Byun MS , Kwon SJ , Choi CH , Kwon JS ((2011) ) Increased default mode network connectivity associated with meditation. Neurosci Lett 487: , 358–362. |
[57] | Eyre HA , Siddarth P , Acevedo B , Van Dyk K , Paholpak P , Ercoli L , St Cyr N , Yang H , Khalsa DS , Lavretsky H ((2017) ) A randomized controlled trial of Kundalini yoga in mild cognitive impairment. Int Psychogeriatr 29: , 557–567. |
[58] | Casey JJ , Harrison KL , Ventura MI , Mehling W , Barnes DE ((2020) ) An integrative group movement program for people with dementia and care partners together (Paired PLIÉ): Initial process evaluation. Aging Ment Health 24: , 971–977. |
[59] | Mehling WE , Scott TM , Duffy J , Whitmer RA , Chesney MA , Boscardin WJ , Barnes DE ((2020) ) Dyadic group exercises for persons with memory deficits and care partners: Mixed-method findings from the paired Preventing Loss of Independence through Exercise (PLIE) randomized trial. J Alzheimers Dis 78: , 1689–1706. |
[60] | Althouse AD ((2016) ) Adjust for multiple comparisons? It’s not that simple. Ann Thorac Surg 101: , 1644–1645. |