Subcortical and Cortical Regions of Amyloid-β Pathology Measured by 11C-PiB PET Are Differentially Associated with Cognitive Functions and Stages of Disease in Memory Clinic Patients
Abstract
Background:
The effect of regional brain amyloid-β (Aβ) pathology on specific cognitive functions is incompletely known.
Objective:
The relationship between Aβ and cognitive functions was investigated in this cross-sectional multicenter study of memory clinic patients.
Methods:
The participants were patients diagnosed with Alzheimer’s disease (AD, n = 83), mild cognitive impairment (MCI, n = 60), and healthy controls (HC, n = 32), who had been scanned by 11C-PiB PET in 13 brain regions of both hemispheres and who had been assessed by cognitive tests covering seven domains.
Results:
Hierarchic multiple regression analyses were performed on each cognitive test as dependent variable, controlling for demographic characteristics and APOE status (block 1) and PiB measures in 13 brain regions (block 2) as independent variables. The model was highly significant for each cognitive test and most strongly for tests of episodic memory (learning and retention) versus PiB in putamen, visuospatially demanding tests (processing and retention) versus the occipital lobe, semantic fluency versus the parietal lobe, attention versus posterior gyrus cinguli, and executive function versus nucleus accumbens. In addition, education had a positively and APOE status a negatively significant effect on cognitive tests.
Conclusion:
Five subcortical and cortical regions with Aβ pathology are differentially associated with cognitive functions and stages of disease in memory clinic patients.
INTRODUCTION
A pathological hallmark of Alzheimer’s disease (AD) is the progressive accumulation of senile plaques in the brain containing amyloid-β (Aβ). The amount of pathological Aβ is possible to measure in vivo of Aβ by means of 11C-labeled Pittsburgh Compound B (11C-PiB) positron emission tomography (PET) retention [1–3]. This fibrillar Aβ marker can be observed in high amounts in the neocortex of patients diagnosed with AD [4–6] and patients with mild cognitive impairment (MCI), particularly those who later convert to AD [7, 8] and in some individuals evaluated as healthy [9]. In addition, high levels of 11C-PiB PET Aβ have been reported in subcortical brain regions such as the striatum (including caudate, putamen, and nucleus accumbens) in early stages of sporadic and familial AD [10–13]. Recently, it was suggested that it is possible to use regional PET Aβ for staging of AD from unimpaired aging to clinical diagnosis of AD [14]. In this study, the earliest PET Aβ accumulation was observed in precuneus, posterior cingulate, isthmus cingulate, insula, medial and lateral orbitofrontal cortices, and this pattern was common in cognitively unimpaired individuals and MCI patients.
A behavioral hallmark of AD is cognitive decline commonly evaluated by means of tests of memory and other cognitive domains. The first and most early cognitive change in typical AD is a decline in episodic memory during the preclinical stage of AD, when patients are not demented but symptomatic years ahead of the diagnosis of AD. Change of memory is followed by change in the executive and visuospatial functions in the prodromal stage closer to the diagnosis of AD, when multiple cognitive functions are affected without marked dysfunction in daily life, i.e., MCI. Finally, verbal functions become impaired in association with dysfunction in daily living in the early AD stage [15–17]. When a diagnosis is decided upon, the cognitive impairment encompasses several domains including memory and at least one other cognitive domain. In this way, it is possible to discern three stages of AD development: the single symptomatic stage many years ahead of a clinical diagnosis of AD, the prodromal stage years ahead of an AD diagnosis (impairment in multiple cognitive functions) and the early AD stage the diagnostic criteria are fulfilled, when there is clear dysfunction in daily living.
Most studies on the relationship between PiB PET and cognition have used global measures of neocortical PiB retention. In these studies, the association between PiB and cognition has been reported to be absent or weak [5, 18, 19], while it seems to be significant in MCI [5, 7, 20, 21] and shows mixed results in healthy aging possibly due to sample characteristics and variability of methods utilized [5, 20, 22]. Relatively few studies using PiB PET measures have examined the association between amyloid pathology in specific brain regions and tests in specific cognitive functions. When the relationship between specific regions and specific cognitive tests has been studied, marked associations have been reported for subcortical regions and episodic memory in heterogeneous samples of patients including AD, MCI, and healthy controls [8, 23].
In the present explorative study of brain-behavior associations in AD, the rationale was to focus on specific tests covering changes in AD in relation to regional brain PET Aβ pathology and possible modifying conditions. It is well known that there is an interaction between Aβ and the APOE gene [9, 24]. It is also well known that cognitive functions are influenced by cognitive reserve [25]. Cognition is determined by two major factors, the environment (e.g., education) and genetics (e.g., APOE), and the effect of these factors on the brain [26, 27].
The purpose of the study was to investigate the relationship between regional PET Aβ deposition in cortical and subcortical brain regions in each hemisphere, rather than global neocortical PiB, and the performance in AD-sensitive cognitive tests rather than global cognitive measures in patients with AD and MCI as well as healthy control (HC) individuals recruited from European memory clinics, i.e., three groups varying in level of cognitive performance. In addition, possible modifying conditions, education, and APOE status, were accounted for.
METHODS
Participants
The participants in the European PiB PET study [5] were recruited from five European PET centers (Leuven/Belgium, London/UK, Munich/Germany, Stockholm/Sweden, Turku/Finland. All patients had been referred from primary to a memory clinic at each European site because they had symptoms of suspected dementia. Patients diagnosed with AD or MCI were eligible for the study. All patients had participated in research studies on PiB PET at each center. Patients were excluded if they had psychiatric symptoms, signs of abuse, head trauma, or other neurologic conditions. This study included 215 individuals. The controls were recruited among carers and relatives. Only those controls, who did not report any cognitive problems were included into the study. Details of inclusion/exclusion criteria and technical scanning information has been presented previously [5].
In the present study on PiB and cognition, 27 of the 215 individuals were excluded as they did not participate in any assessment of cognitive functions and 10 individuals completed less than 5 cognitive tests. In addition, three participants (2 AD and 1 MCI) were excluded as they performed extremely poor, see Statistical analyses). Accordingly, 175 individuals completed both a PET scan and an assessment of cognitive functions. Among these subjects, 83 patients were diagnosed with AD, 60 with MCI, and 32 were HC subjects.
Diagnosis
The diagnosis was decided at each site by experienced experts based on all clinical information. The AD diagnosis fulfilled the NINCDS-ADRDA criteria for AD [28] and the DSM-IV criteria for dementia [29]. The MCI diagnosis was based on the modified Petersen criteria for MCI [30]. The healthy status of HC was checked by in-house examination at each site.
Clinical examination
All patients had been referred to and examined for suspected dementia development according to local routines at each center. A detailed description of examination routines at each center has been presented in a previous publication [5]. Neuropsychological testing was used to measure cognitive function following the standard procedures at each center, see below. The APOE genotype was analyzed at each center.
11C-PiB PET imaging
The synthesis of 11C-PiB was performed at each center according to good manufacturing practice. The PET examination was performed at each center following the routine procedure at each center. The imaging data were submitted to the Wolfson Molecular Imaging Centre, Manchester, UK, for central processing. The cerebellar region was used as reference. Twenty-three regions of interest (ROI) were anatomically defined, using the Montreal Neurological Institute atlas [31] and PiB values were normalized. All details of the whole PET procedure have been presented previously [5].
Neuropsychological assessment
Seven cognitive domains were covered by standard neuropsychological tests. The tests were administered and evaluated according to standard procedures [32] for each test. Global cognition was indicated by the Mini-Mental Status Examination (MMSE) [33]. For the domain of verbal learning, a list of words to-be-learned was presented several times to measure learning by total number correct responses. Directly after the learning test, a delayed free recall followed, i.e., verbal retention. The specific test used varied across centers (Rey Auditory Verbal Learning or ADAS-Cog or CERAD [32]. Accordingly, the test assessing episodic memory varied among centers although the format used for acquisition of words and delayed free recall was similar across centers. The visuospatial processing domain was assessed by copying a geometrical figure exemplified by the Rey-Osterrieth copy test [32]. This copy task was used to test unintentional learning and the retention of the figure after a delay indicating the visuospatial retention domain [32]. Animal naming in one minute was used by all centers to indicate the verbal fluency domain [32]. The domains of attention and executive function were assessed with the Trailmaking test A (TMTA) and B (TMTB) [32], respectively. As participants did not complete all neuropsychological tests, the sample size for each test is shown in Table 3. The total number of missing cognitive data was 198 (16.2%) out of maximum data points (175×7 = 1225). There was no significant difference between diagnostic groups regarding the number of missing data (χ2 = 11.41, df = 2, p > 0.1).
All the neuropsychological raw scores except MMSE and TMTA and TMTB were standardized by means of transformation into z-scores using age-matched normative values according to test manuals of each test at the University Clinic of Cologne, Germany, the responsible site for test data. For the MMSE test, normative data reported by Crum and associates [34] were used. The TMTA and TMTB test scores (latencies) were transformed to z-scores according to a meta-study on these tests [35] after excluding abnormal values (latencies≥200 s for TMTA and latencies≥300 s for TMTB) in order to normalize the distributions. In addition, participants with extremely poor performance were excluded if the mean z-score across the seven tests exceeded z = –5.
Statistical analyses
Multiple regression analyses (one for each cognitive domain) were conducted, with cognitive test as dependent variable using participants, who performed better than -5 in z-score on mean cognitive function across all seven tests. This criterion was chosen to exclude participants with extremely poor performance on specific tests. The independent variables years of education and APOE status (any versus no ɛ4 allele) were entered in the first block simultaneously. PiB measures in the 13 brain regions were entered in the second block stepwise (forward selection). Regression models were chosen only when multicollinearity diagnostics were acceptable (tolerance > 0.200 and variance inflation factor, VIF < 5). The selection of variables to include and exclude was default (p for inclusion = 0.01 and for exclusion = 0.05) to increase the demands on association between dependent and predictor variables. Pairwise exclusion of missing cases was used. A MANOVA was performed with brain regions as dependent variables (13 ROIs and 2 hemispheres), diagnostic group as independent variable and age, gender, education, and APOE as covariates. The impact of diagnostic group as independent variable and age, gender, education, and APOE was analyzed with one-way (group) ANOVA for each cognitive test. All analyses were performed with SPSS version 24.
RESULTS
Comparison of groups
The demographic and clinical characteristics of the three groups are presented in Table 1. There were no significant differences between the three groups in age (F < 1, df = 2/173, p > 0.1), gender distribution (χ2 = 1.89, df = 2/173, p > 0.1), or years of education (F < 1, df = 2/169, p > 0.1). The groups differed significantly regarding the APOE ɛ4 genotype (χ2 = 23.65, df = 2, p < 0.001), with a larger proportion of the ɛ4 allele in MCI versus HC (p < 0.01) and a larger proportion of ɛ4 in AD versus MCI (p < 0.05). Groups differed significantly on global cognition as measure by MMSE (F = 46.32, df = 2/168, p < 0.001); pairwise comparisons were significant (ps < 0.01, HC versus MCI and MCI versus AD, respectively).
Table 1
Demographic and clinical characteristics (MMSE and APOE) of three groups of subjects: Alzheimer’s disease (AD), mild cognitive impairment (MCI), and healthy controls (HC)
Group | ||||
Characteristic | AD | MCI | HC | p |
N (% females) | 83 (54%) | 60 (48%) | 32 (63%) | p > 0.1 |
Age (M±SD), y | 69.2±8.7 | 68.2±7.6 | 68.3±6.5 | p > 0.1 |
Education (M±SD), y | 11.6±3.5 | 12.1±4.3 | 11.5±3.4 | p > 0.1 |
MMSE (M±SD), raw score | 24.3±2.8 | 27.1±2.0 | 28.8±1.0 | p < 0.001 |
APOE, n (% ɛ4 carrier) | 55 (74%) | 33 (53%) | 7 (26%) | p < 0.001 |
PiB measures across 13 brain regions in AD, MCI, and HC
The PET PiB measures across 13 brain regions and two hemispheres in three groups of subjects (AD, MCI, and HC) are presented in Table 2 and visualized in Fig. 1. It should be noted that these PiB measures were not part of the diagnostic procedure. There was no difference in PiB between hemispheres in any region in the HC group (all ps > 0.1). However, in the MCI group, PiB was significantly larger in the right hemisphere (t = 2.57, df = 60, p < 0.01). In the AD group, PiB was significantly larger in three right hemisphere regions: the frontal, parietal, and in putamen (t = 6.20, df = 84, p < 0.001; t = 2.77, df = 84, p < 0.01; t = 2.72, df = 60, p < 0.01; respectively)
The PET PiB measures across 13 regions and two hemispheres (within subjects) with age, gender, education, and APOE as covariates showed that the main effect of ROIs was close to significant (λ = 0.79, F = 1.68, df = 12/76, p = 0.09, η2 = 0.22) and that the ROI by diagnosis interaction was significant (λ = 0.54, F = 2.29, df = 24/152, p < 0.001; η2 = 0.27) and that no other two-way interaction including ROI and other factors were significant (all ps > 0.1). Furthermore, the main effect of hemisphere was not significant as well as two-way interactions including hemisphere (all ps > 0.1). Finally, the main effect of diagnosis was markedly significant (F = 25.59, df = 2/151, p < 0.001, η2 = 0.25). The main effects of demographics and APOE ɛ4 (presence/absence) were not significant (all ps > 0.1).
Table 2
11C-PiB retention (M±SD) in relation to the cerebellum in 13 regions of interest in each hemisphere in three groups of individuals: Alzheimer’s disease (AD), mild cognitive impairment (MCI), and healthy controls (HC)
Group | |||
Region of interest | AD | MCI | HC |
Temporal, sin | 1.81±0.31 | 1.62±0.29 | 1.29±0.07 |
Temporal, dx | 1.83±0.31 | 1.60±0.29 | 1.29±0.09 |
Insula, sin | 1.77±0.32 | 1.57±0.28 | 1.30±0.09 |
Insula, dx | 1.78±0.33 | 1.58±0.28 | 1.30±0.09 |
Anterior Gyrus Cingulum, sin | 2.16±0.44 | 1.84±0.45 | 1.35±0.13 |
Anterior Gyrus Cingulum, dx | 2.14±0.44 | 1.82±0.42 | 1.31±0.11 |
Posterior Gyrus Cingulum, sin | 2.13±0.42 | 1.85±0.45 | 1.37±0.14 |
Posterior Gyrus Cingulum, dx | 2.14±0.43 | 1.86±0.46 | 1.37±0.14 |
Frontal, sin | 1.84±0.34 | 1.62±0.32 | 1.23±08 |
Frontal, dx | 1.89±0.35 | 1.64±0.33 | 1.25±0.08 |
Occipital, sin | 1.63±0.25 | 1.48±0.21 | 1.29±0.07 |
Occipital, dx | 1.63±0.25 | 1.48±0.23 | 1.28±0.08 |
Parietal, sin | 1.81±0.33 | 1.61±0.34 | 1.22±0.08 |
Parietal, dx | 1.84±0.34 | 1.62±0.35 | 1.22±0.09 |
Caudate, sin | 1.60±0.42 | 1.40±0.45 | 0.97±0.13 |
Caudate, dx | 1.60±0.42 | 1.40±0.46 | 0.96±0.15 |
Nucleus accumbens, sin | 1.93±0.40 | 1.63±0.47 | 1.07±0.12 |
Nucleus accumbens, dx | 1.90±0.43 | 1.58±0.51 | 1.05±0.15 |
Putamen, sin | 2.06±0.39 | 1.77±0.43 | 1.30±0.10 |
Putamen, dx | 2.11±0.40 | 1.80±0.43 | 1.29±0.09 |
Thalamus, sin | 1.29±0.24 | 1.27±0.24 | 1.12±0.12 |
Thalamus, dx | 1.30±0.22 | 1.25±0.22 | 1.10±0.10 |
Hippocampus, sin | 1.27±0.18 | 1.25±0.14 | 1.23±0.09 |
Hippocampus, dx | 1.28±0.15 | 1.26±0.13 | 1.24±0.08 |
Parahippocampus, sin | 1.42±0.22 | 1.32±0.15 | 1.22±0.10 |
Parahippocampus, dx | 1.44±0.21 | 1.32±0.15 | 1.21±0.08 |
Fig. 1
A bar graph of PIB value across 13 regions of interest for three diagnostic groups (AD, MCI, and Controls).
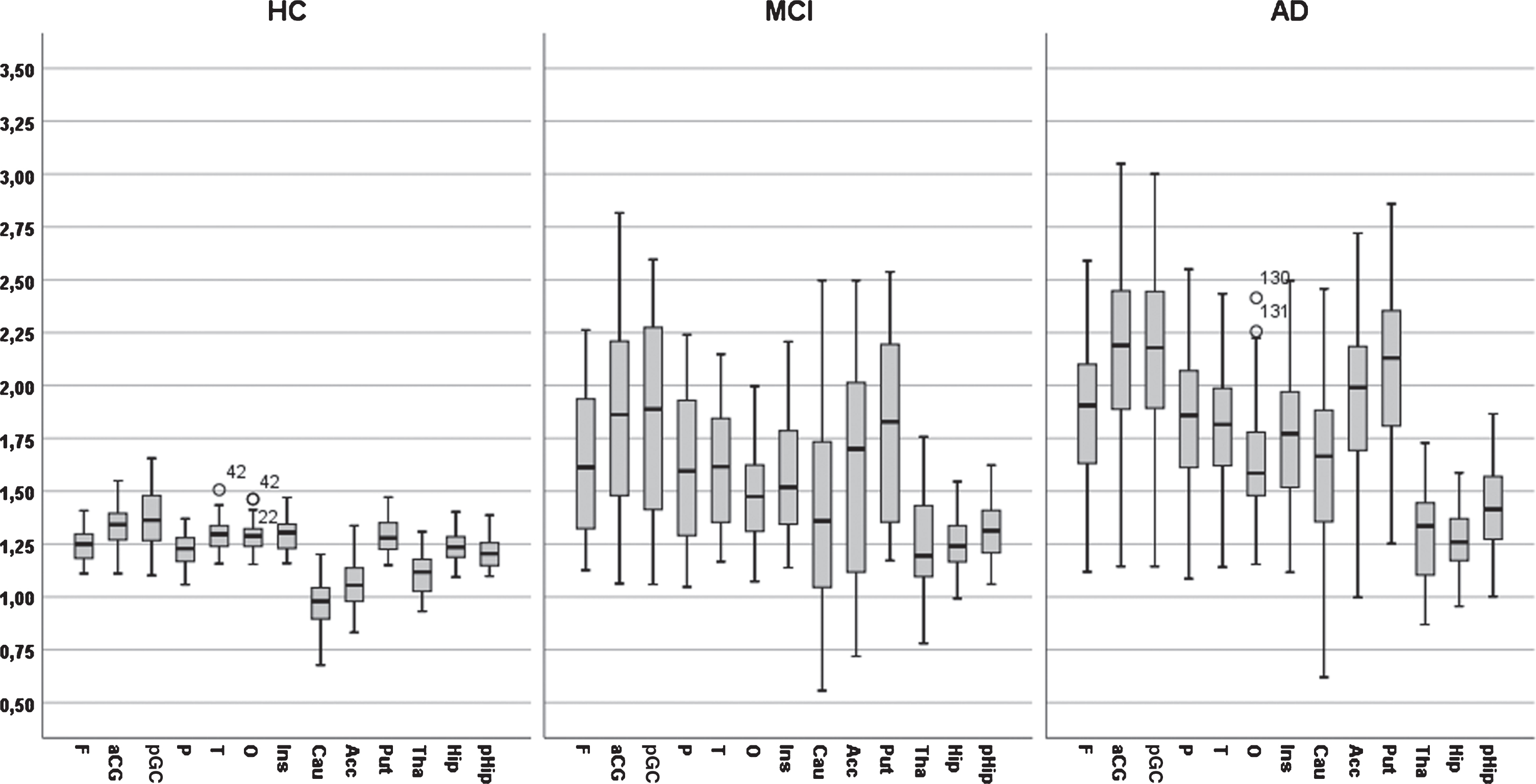
The interaction between diagnosis and ROI was due to a differential pattern of group differences across ROIs. Diagnostic groups differed markedly and significantly in 10 ROIs (frontal, parietal, occipital, temporal, anterior and posterior gyrus cinguli, caudate, putamen, and accumbens), significantly but less markedly in two ROIs (thalamus and parahippocampus) and not significantly in one ROI (hippocampus).
Test results across cognitive domains in AD, MCI, and HC
The neuropsychological results in z-scores (M±SD) for seven cognitive domains in the three diagnostic groups are displayed in Table 3. It should be noted that cognitive test results were part of the diagnostic procedure. The scores at each center have been reported previously [5]; these scores demonstrate that the controls performed well compared to normal values at each site. The effect of diagnosis was significant on all tests (all ps < 0.001). The effect of increasing age was significant in the figure copy, TMTA and TMTB tests (r = 0.17, p < 0.05; r=–0.29, p < 0.001; r = –0.28, p < 0.002; respectively), but not in the other tests (all ps > 0.1). Gender was not significant in any test (all ps > 0.1). Years of education was a significant positive factor in the verbal learning test (r = 0.22, p < 0.05). The presence of any APOE ɛ4 allele was a significant negative predictor on all seven tests (r varied from –0.21, p < 0.05 to –0.38, p < 0.001).
Table 3
Test results in z-score (Mean±SD) results on 7 neuropsychological tests in three groups of individuals: Alzheimer’s disease (AD), mild cognitive impairment (MCI), and healthy controls (HC)
Group | |||
Cognitive test | AD | MCI | HC |
Word list learning, n = 173 | –2.43±1.44 | –1.53±1.70 | +0.05±0.99 |
Word list retention, n = 175 | –3.82±1.42 | –2.54±1.84 | +0.03±1.00 |
Visuospatial processing (Design copy), n = 147 | –2.66±2.34 | –1.92±2.12 | ±0.00±1.00 |
Visuospatial retention (Design), n = 152 | –1.92±0.88 | –1.13±1.09 | ±0.00±1.00 |
Semantic fluency (Animal), n = 116 | –1.39±1.01 | –1.06±1.18 | +0.10±0.94 |
Attention (TMTA), n = 139 | –2.00±2.30 | –1.45±2.47 | –0.06±1.61 |
Executive function (TMTB), n = 123 | –1.90±1.45 | –0.56±1.36 | 0.30±0.62 |
Relationship between regional PiB measures and cognitive performance
For each cognitive domain, the relationship between the test and the predictors was computed using multiple regression analyses. In the first block of predictors, years of education, and number of APOE ɛ4 alleles were included simultaneously. Age and gender were not used as covariates because preliminary analyses showed that these variables did not correlate significantly with any cognitive test except one (age versus figure copy r = 0.20, df < 0.05). In the second block, all 13 ROIs in each hemisphere were included in stepwise order to find the most powerful ROIs.
In Table 4, the outcome of the seven multiple regression analyses is presented. All analyses were significant (all ps < 0.001) and the multiple correlation coefficients varied from 0.42 for semantic fluency to 0.64 for word list delayed recall. The first step revealed that years of education were a positive predictor in all cognitive tests. It was significant in s three tests (verbal learning, verbal retention, and executive function). The presence of APOE ɛ4 allele(s) was a negative factor in all tests and significant in two tests (verbal learning and retention). The second step revealed that PiB deposition in the left putamen was a significant negative predictor for performance in verbal learning and retention (see Fig. 3, that PiB deposition in the right occipital ROI was significant and negatively associated with visuospatial ability and retention, that PiB deposition in the left parietal ROI was significant an negatively associated with semantic fluency, that the right posterior gyrus cingulum was significant and negatively associated with attention (TMTA) and that PiB deposition in the left accumbens was significant and negatively associated with executive function (TMTB). In the third step, positive associations between PiB deposition and cognition were obtained showing a connection between PiB deposition in the right parietal region and attention (TMTA) as well as PiB deposition in left thalamus and executive function (TMTB). The latter findings seem to be unreasonable (see Discussion).
Table 4
Multiple regression analyses of cognitive test results in relation to predictors: education and APOE status (first block) entered simultaneously and 13 measures of regional PiB deposition in the left and right hemisphere (second block) entered stepwise. The r2 change from step to step is presented for each analysis at each test
Model | Predictors | |||||||
1st step | 1st step | 2nd step | 3rd step | |||||
Cognitive domain | r | F | df | p t/p/β | edu t/p/β | APOE t/p/β | ROI t/p/β | ROI |
Verbal learning | 0.56 | 23.50 | 3/151 | 0.001 | edu | APOE | sPut | |
step 1: r2 = 0.16 | +1.96 | – 3.09 | – 5.99 | |||||
step 2: r2 = 0.16 | 0.05 | 0.01 | 0.001 | |||||
+0.13 | – 0.22 | – 0.43 | ||||||
Verbal retention | 0.64 | 35.06 | 3/152 | 0.001 | edu | APOE | sPut | |
step 1: r2 = 0.14 | +1.89 | – 2.51 | – 8.27 | |||||
step 2: r2 = 0.27 | 0.05 | 0.005 | 0.001 | |||||
+0.12 | – 0.17 | – 0.55 | ||||||
Visuospat. proc. | 0.42 | 10.22 | 3/142 | 0.001 | edu | APOE | dO | |
step 1: r2 = 0.05 | <1 | – 1.49 | – 4.65 | |||||
step 2: r2 = 0.13 | ns | ns | 0.001 | |||||
+0.01 | – 0.12 | – 0.37 | ||||||
Visuospat ret. | 0.59 | 26.04 | 3/145 | 0.001 | edu | APOE | dO | |
step 1: r2 = 0.07 | +1.52 | – 1.42 | – 7.84 | |||||
step 1: r2 = 0.28 | ns | ns | 0.001 | |||||
+0.10 | – 0.10 | – 0.55 | ||||||
Semantic fluency | 0.44 | 7.33 | 3/94 | 0.001 | edu | APOE | sP | |
step 1: r2 = 0.06 | +1.23 | – 1.021 | – 3.90 | |||||
step 2: r2 = 0.13 | ns | ns | 0.001 | |||||
+0.06 | – 0.10 | – 0.39 | ||||||
Attention | 0.48 | 9.19 | 4/122 | 0.001 | edu | APOE | dGCp | dP |
step 1: r2 = 0.07 | +1.68 | – 1.34 | – 4.32 | +3.47 | ||||
step 2: r2 = 0.09 | ns | ns | 0.001 | 0.001 | ||||
step 3: r2 = 0.08 | +0.13 | – 0.11 | – 1.39 | +1.12 | ||||
Exec. function | 0.61 | 17.70 | 4/108 | 0.001 | edu | APOE | sAcc | sThal |
step 1: r2 = 0.12 | +3.21 | – 1.21 | – 6.56 | +3.61 | ||||
step 2: r2 = 0.18 | 0.01 | ns | 0.001 | 0.001 | ||||
step 3: r2 = 0.08 | +0.25 | – 0.010 | – 0.69 | +0.70 |
r, multiple correlation coefficient; edu, years of education; APOE, number of ɛ4 allele(s); p, probability; β, beta weight; ROI, brain region of interest; F, frontal; P, parietal; O, occipital; T, temporal; GC, gyrus cingulum; Tha, thalamus; Put, putamen; a, anterior; p, posterior; s, sinister/left; d, dexter/right.
Fig. 2
A bar graph of test results across five cognitive domains in z-score for three diagnostic groups (AD, MCI, and Controls).
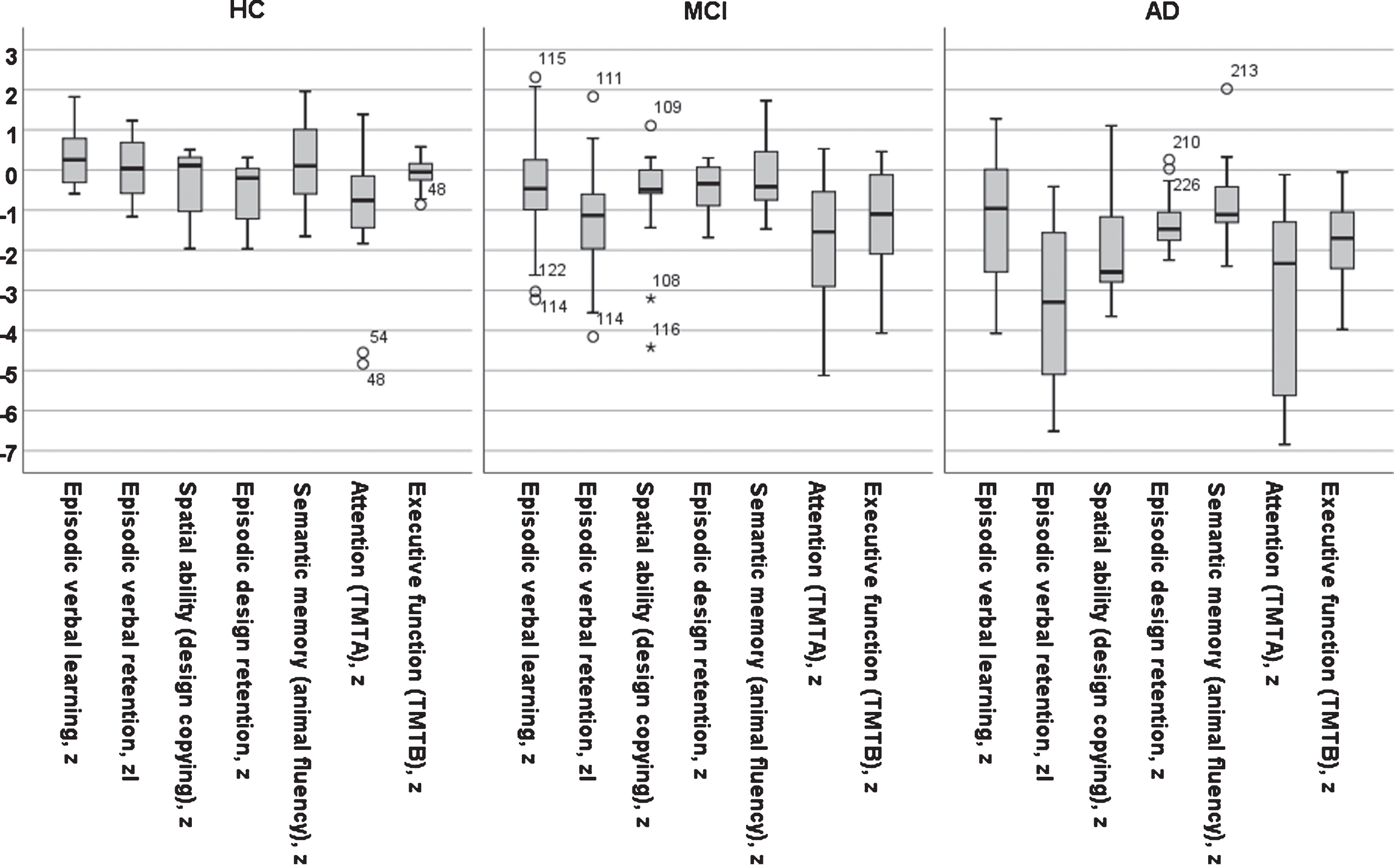
Fig. 3
Scatter plot of test results in verbal learning versus PIB value in putamen for three diagnostic groups (AD, MCI, and Controls) also showing the regression line using local weighted regression.
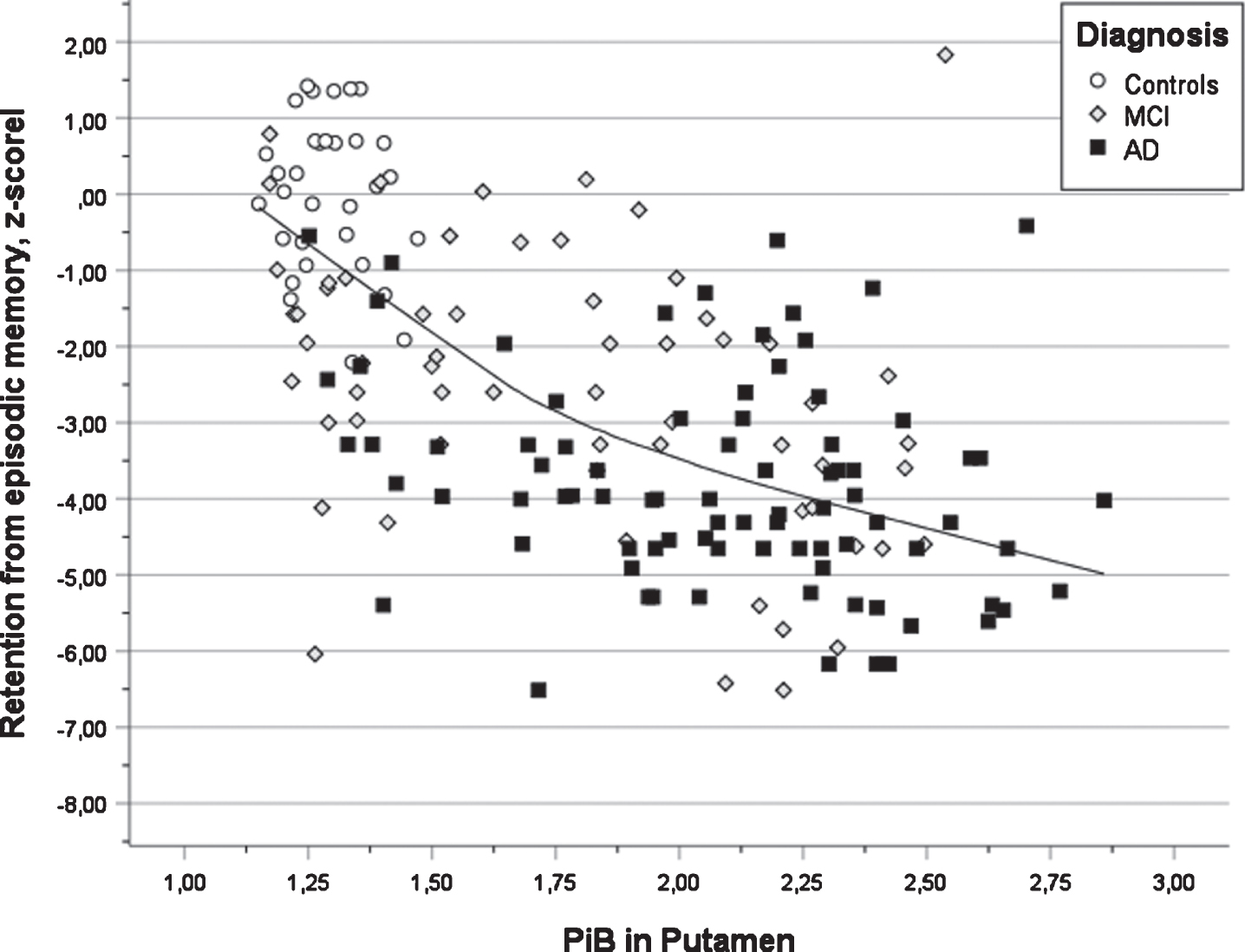
Fig. 4
Scatter plot of test results in executive function (TMTB) versus PIB value in nucleus accumbens for three diagnostic groups (AD, MCI, and Controls) also showing the regression line using local weighted regression.
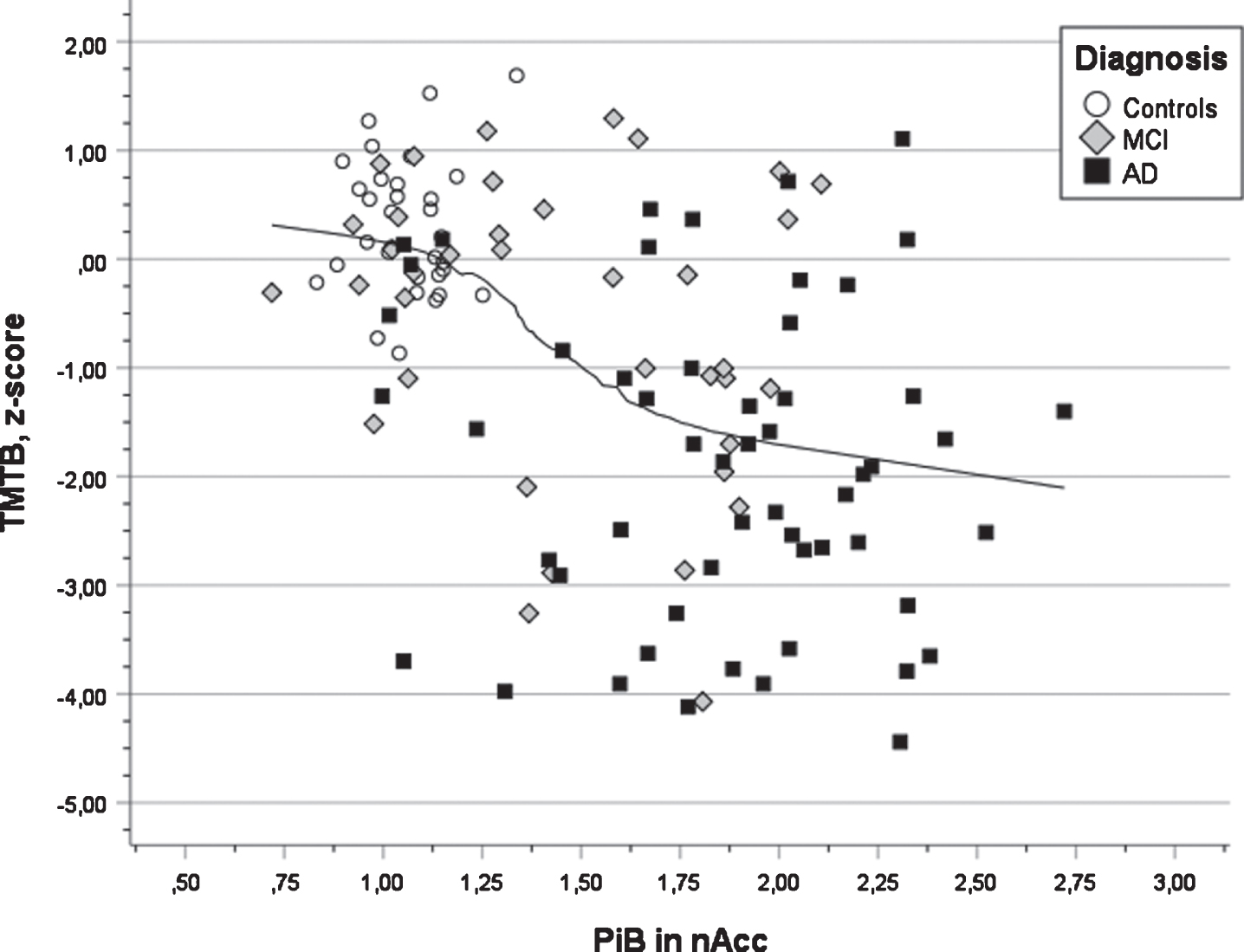
DISCUSSION
The present study investigated the in vivo regional Aβ brain pathology measured by PiB PET, indicating the presence of neuritic plaques [1–3], and cognitive functions in AD and MCI patients and healthy control subjects. Marked changes in subcortical and cortical brain regions and specific cognitive domains were found in the preclinical part of the disease course. Similar findings have been found in previous research [13]. However, a number of specific associations between PiB PET and cognitive function found in the present study have not been found previously.
Five distinct brain-behavior relationship were obtained between performance in specific cognitive tests and Aβ pathology. Aβ pathology in the left putamen was associated with verbal learning and retention, Aβ pathology in the right gyrus cinguli was associated with visual search in the TMTA test (attention), and Aβ pathology in the left nucleus accumbens was associated with the TMTB test (executive function). Furthermore, significant brain-behavior relationships were obtained between Aβ brain pathology in the right occipital region and visuospatial tasks (copying and delayed reproduction of target design) as well as the left parietal region and verbal fluency).
The association between increased pathology in putamen and decreased performance in verbal learning and verbal retention (delayed recall) is in line with previous research [7, 11] and a review of functional magnetic resonance imaging (fMRI) studies regarding brain networks involved in episodic memory [36]. In vitro studies of Aβ deposits have reported that neuropathological changes occur within subcortical regions (e.g., striatum, thalamus, gyrus cinguli, amygdala) in phase three of the Thal scheme of AD development [37]. A recent study of in vivo PET Aβ suggested that changes in putamen was an early stage finding in AD patients [14]. Similar findings were obtained in a small study of PSEN1 mutation carriers, but these findings were not associated with cognition [38]. In vivo animal studies support the notion that the hippocampal and dorsolateral memory systems are complementary in learning [39]. Furthermore, the earliest cognitive changes in AD occur many years ahead of the clinical diagnosis in the preclinical stage [15–17]. The parallel changes in PiB PET and episodic memory were more pronounced in HC and MCI groups compared to MCI and AD groups in which suggests that this finding represents an early event in the AD disease course.
A second finding was the coupling between increased PiB PET in the right posterior cingulate gyrus and impaired performance in the TMTA task here interpreted as construct of attention. In a review of the role of posterior cingulate, it was reported that changes in posterior cingulate cortex are found not only in AD but many other diseases (e.g., traumatic brain injury, psychiatric syndromes, multiple sclerosis, etc.) in relation to regulating the arousal state, focused attention, and the breadth of attentional focus [40]. These changes have been shown using various methods like PET studies using glucose metabolism [41] and fMRI [36] and they have been shown to occur in the early stage of AD disease course [14]. Posterior cingulate has been pointed out as one part of a frontostriatal networks subserving orientation as well as goal-directed executive control and arousal/sustained attention [42].
A third finding was that performance in the TMTB test used to indicate executive function (demanding attention, mental control, and organization) was negatively associated with PET Aβ in nucleus accumbens, a part of the striatal region. This region has been suggested to be involved in three main phenomena: learning of associations between stimuli, actions and rewards; selection between competing response alternatives; and motivational modulation of motor behavior [39, 43], among which TMT performance is related to modulation of motor behavior. Similar observations have been reported by other researchers in various patient groups and based on clinical and experimental studies [44–48]. The TMTB test is regarded as a sensitive test for AD development and decline in the preclinical stage prior to the clinical diagnosis of AD [14–17].
Further, elevated PET Aβ abnormality in the right occipital lobe was negatively associated with copying of complex figural stimuli, for instance the copy task of the Rey-Osterrieth Complex Figure test and delayed recall of the same test (retrieval from visuospatial episodic memory). This finding may illustrate the brain activity in tasks requiring processing of visual stimuli [49, 50] occurring relatively late in the disease course [14].
Finally, there was a significant association between increased Aβ in the left parietal lobe and poor semantic fluency performance (Animal fluency) in agreement with previous research in memory clinic patient, when brain abnormality was measured by cortical thickness [51], brain atrophy [52, 53], and PET glucose metabolism [53]. The association between impaired parietal lobe and affected semantic processing seem to in the clinical stage of AD [14, 53].
In majority of relationships, PiB deposition was a negative factor for cognition. However, in two relationships, PiB Aβ was positively associated with cognition (attention versus right parietal lobe and executive versus left thalamus). In these two instances, there were both negative and positive ROI factors. The positive predictors are a paradoxical outcome, since PiB retention in all regions, except hippocampus, are negatively and significantly correlated with impaired performance in all seven cognitive tests. These positive effects are regarded as artefacts which is supported by statistical knowledge regarding the common reciprocal suppression phenomena in multiple regression analyses in which beta weights can become positive although they are expected to be negative under specific circumstances [54] and not a veridical neurocognitive phenomenon [55].
The effect of APOE ɛ4 on performance was significant and negative in two of the seven cognitive tests. Presence of the APOE ɛ4 allele(s) is known to be related to elevated PiB in MCI and AD [5]. In addition, the brain-behavior relationships were positively influenced by years of education in several cognitive tests, which is generally found in similar research and termed as cognitive reserve [25]. The effect of education is commonly interpreted as resistance to cognitive decline by shaping a repertoire of knowledge and strategies. Both the APOE and educational effects are examples of well-known biological and psychosocial factors influencing brain-behavior relationships [26].
The in vivo measurement of regional brain pathology and the comprehensive cognitive assessment in many memory clinic patients characterize the present study. These features made it possible to analyze brain-behavior relationships in some detail. Another feature was that the participants were recruited from five European memory clinics that supports the external validity and generalizability of the present results. A general drawback of this study relates to the cross-sectional design showing a single snapshot of brain-behavior events that are time-related and vary across disease course. It has to be noted that the results may be generalized to typical memory clinic patients including individuals with abnormal as well as normal neocortical PiB values. Another drawback relates to the statistical analyses, in which the chosen significance level was increased to avoid less powerful brain-behavior associations. If a standard Bonferroni correction had been applied, the reported p-values of the regression analyses and the significant ROIs would have survived this action. In this way some true findings may not have been identified due to type II error. The reliability of regional PiB measures has been questioned due to off-target PiB binding. However, in a subsample of participants selected from the original cohort [5], it was found that regions were identified with good precision [56] supporting the reliability of PiB regional values. Furthermore, the phenotype of AD has been considered as a relatively uniform diagnostic entity and not described as subtypes varying in important characteristic (e.g., age of onset, onset symptoms, brain atrophy, etc.) [57]. Finally, it should be kept in mind that there are many environmental and genetic factors that influence inter-individual variation in cognition, where not included in the present study.
In conclusion, five brain-behavior relationships were identified showing an association between amyloid pathology in specific subcortical and neocortical regions and impaired performance in specific cognitive tests. Aβ in subcortical compared to cortical regions seemed to occur early in the disease course. As already known, visuospatial processing is related to occipital pathology and retrieval from semantic memory is related to parietal pathology, which occur relatively late in close connection to the clinical diagnosis of AD. The abnormality of episodic memory versus Aβ in putamen, abnormality in attention versus Aβ in posterior gyrus cinguli as well as executive dysfunction versus nucleus accumbens are new findings.
ACKNOWLEDGMENTS
The original project and the multicentre analysis were funded by EC-FP6 Network of Excellence on Diagnostic Molecular Imaging (DiMI, LSHB-CT-2005-512146). The contribution of all the collaborators in the original European multicenter PET study, headed by professor Karl Herholz, is gratefully acknowledged. This specific study on PiB and cognition was funded by the Swedish Research Council (project 05817) and the Regional Agreement on Medical Training and Clinical Research by Stockholm County Council (ALF) as well as Swedish Research Council (VR/2017-02965 and VR/2017-06086).
Authors’ disclosures available online (https://www.j-alz.com/manuscript-disclosures/20-1612r2).
REFERENCES
[1] | Driscoll I , Troncoso JC , Rudow G , Sojkova J , Pletnikova O , Zhou Y , Kraut MA , Ferrucci L , Mathis CA , Klunk WE , O’Brien RJ , Davatzikos C , Wong DF , Resnick SM ((2012) ) Correspondence between in vivo (11)C-PiB-PET amyloid imaging and postmortem, region-matched assessment of plaques. Acta Neuropathol 124: , 823–831. |
[2] | Lowe VJ , Lundt ES , Albertson SM , Pszybelski SA , Senjem ML , Parisi JE , Kantarci K , Boeve B , Jones DT , Knopman D , Jack CR Jr , Dickson DW , Petersen RC , Murray ME ((2019) ) Neuroimaging correlates with neuropathologic schemes in neurodegenerative disease. Alzheimers Dement 15: , 927–939. |
[3] | Seo SW , Ayakta N , Grinberg LT , Villeneuve S , Lehmann M , Reed B , DeCarli C , Miller BL , Rosen HJ , Boxer AL , O’Neil JP , Jin LW , Seeley WW , Jagust WJ , Rabinovici GD ((2016) ) Regional correlations between [11C]PIB PET and post-mortem burden of amyloid-beta pathology in a diverse neuropathological cohort. Neuroimage Clin 11: , 130–137. |
[4] | Klunk WE , Engler H , Nordberg A , Wang Y , Blomqvist G , Holt DP , Bergström M , Savitcheva I , Huang GF , Estrada S , Ausén B , Debnath ML , Barletta J , Price JC , Sandell J , Lopresti BJ , Wall A , Koivisto P , Antoni G , Mathis CA , Långström B ((2004) ) Imaging brain amyloid in Alzheimer’s disease with Pittsburgh Compound-B. Ann Neurol 55: , 306–319. |
[5] | Nordberg A , Carter SF , Rinne J , Drzezga A , Brooks DJ , Vandenberghe R , Perani D , Forsberg A , Långström B , Scheinin N , Karrasch M , Någren K , Grimmer T , Miederer I , Edison P , Okello A , Van Laere K , Nelissen N , Vandenbulcke M , Garibotto V , Almkvist O , Kalbe E , Hinz R , Herholz K ((2013) ) A European multicentre PET study of fibrillar amyloid in Alzheimer’s disease. Eur J Nucl Med Mol Imaging 40: , 104–414. |
[6] | Villemagne VL , Pike KE , Chételat G , Ellis KA , Mulligan RS , Bourgeat P , Ackermann U , Jones G , Szoeke C , Salvado O , Martins R , O’Keefe G , Mathis CA , Klunk WE , Ames D , Masters CL , Rowe CC ((2011) ) Longitudinal assessment of Aβ and cognition in aging and Alzheimer disease. Ann Neurol 69: , 181–192. |
[7] | Forsberg A , Engler H , Almkvist O , Blomquist G , Hagman G , Wall A , Ringheim A , Långström B , Nordberg A ((2008) ) PET imaging of amyloid deposition in patients with mild cognitive impairment. Neurobiol Aging 29: , 1456–1465. |
[8] | Koivunen J , Karrasch M , Scheinin NM , Aalto S , Vahlberg T , Någren K , Helin S , Viitanen M , Rinne JO ((2012) ) Cognitive decline and amyloid accumulation in patients with mild cognitive impairment. Dement Geriatr Cogn Disord 34: , 31–37. |
[9] | Rodrigue KM , Kennedy KM , Devous MD Sr , Rieck JR , Hebrank AC , Diaz-Arrastia R , Mathews D , Park DC ((2012) ) β-Amyloid burden in healthy aging: Regional distribution and cognitive consequences. Neurology 78: , 387–395. |
[10] | Klunk WE , Price JC , Mathis CA , Tsopelas ND , Lopresti BJ , Ziolko SK , Bi W , Hoge JA , Cohen AD , Ikonomovic MD , Saxton JA , Snitz BE , Pollen DA , Moonis M , Lippa CF , Swearer JM , Johnson KA , Rentz DM , Fischman AJ , Aizenstein HJ , DeKosky ST ((2007) ) Amyloid deposition begins in the striatum of presenilin-1 mutation carriers from two unrelated pedigrees. J Neurosci 27: , 6174–6184. |
[11] | Koivunen J , Verkkoniemi A , Aalto S , Paetau A , Ahonen JP , Viitanen M , Någren K , Rokka J , Haaparanta M , Kalimo H , Rinne JO ((2008) ) PET amyloid ligand [11C]PIB uptake shows predominantly striatal increase in variant Alzheimer’s disease. Brain 131: , 1845–1853. |
[12] | Hanseeuw BJ , Lopera F , Sperling RA , Norton DJ , Guzman-Velez E , Baena A , Pardilla-Delgado E , Schultz AP , Gatchel J , Jin D , Chen K , Reiman EM , Johnson KA , Quiroz YT ((2019) ) Striatal amyloid is associated with tauopathy and memory decline in familial Alzheimer’s disease. Alzheimers Res Ther 11: , 17. |
[13] | Tentolouris-Piperas V , Ryan NS , Thomas DL , Kinnunen KM ((2017) ) Brain imaging evidence of early involvement of subcortical regions in familial and sporadic Alzheimer’s disease. Brain Res 1655: , 23–32. |
[14] | Mattsson N , Palmqvist S , Stomrud E , Vogel J , Hansson O ((2019) ) Staging β-amyloid pathology with amyloid positron emission tomography. JAMA Neurol 76: , 11. |
[15] | Almkvist O ((1996) ) Neuropsychological features of early Alzheimer’s disease: Preclinical and clinical stages. Acta Neurol Scand Suppl 165: , 63–71. |
[16] | Almkvist O , Rodriguez-Vieitez E , Thordardottir S , Amberla K , Axelman K , Basun H , Kinhult-Stålbom A , Lilius L , Remes A , Wahlund LO , Viitanen M , Lannfelt L , Graff C ((2017) ) Predicting cognitive decline across four decades in mutation carriers and non-carriers in autosomal-dominant Alzheimer’s disease. J Int Neuropsychol Soc 23: , 195–203. |
[17] | Mistridis P , Krumm S , Monsch AU , Berres M , Taylor KI ((2015) ) The 12 years preceding mild cognitive impairment due to Alzheimer’s disease: The temporal emergence of cognitive decline. J Alzheimers Dis 48: , 1095–1107. |
[18] | Ossenkoppele R , van der Flier W , Verfaillie SC , Vrenken H , Versteg A , van Schijndel RA , Sikkes SA , Twisk J , Adriaanse SM , Zwan MD , Boellard R , Windhorst AD , Barkhof F , Scheltens P , Lammertsma AA , van Berckel BN ((2014) ) Long-term effects of amyloid, hypometabolism, and atrophy on neuropsychological functions. Neurology 82: , 1768–1775. |
[19] | Pike KE , Ellis KA , Villemagne VL , Good N , Chételat G , Ames D , Szoeke C , Laws SM , Verdile G , Martins RN , Masters CL , Rowe CC ((2011) ) Cognition and beta-amyloid in preclinical Alzheimer’s disease: Data from the AIBL study. Neuropsychologia 49: , 2384–2390. |
[20] | Mormino EC , Kluth JT , Madison CM , Rabinovici GD , Baker SL , Miller BL , Koeppe RA , Mathis CA , Weiner MW , Jagust WJ ; Alzheimer’s Disease Neuroimaging Initiative ((2009) ) Episodic memory loss is related to hippocampal-mediated beta-amyloid deposition in elderly subjects. Brain 132: , 1310–1323. |
[21] | Pike KE , Savage G , Villemagne VL , Ng S , Moss SA , Maruff P , Mathis CA , Klunk WE , Masters CL , Rowe CC ((2007) ) Beta-amyloid imaging and memory in non-demented individuals: Evidence for preclinical Alzheimer’s disease. Brain 130: , 2837–2844. |
[22] | Lim YY , Ellis KA , Harrington K , Kamer A , Pietrzak RH , Bush AI , Darby D , Martins RN , Masters CL , Rowe CC , Savage G , Szoeke C , Villemagne VL , Ames D , Maruff P ; AIBL Research Group ((2013) ) Cognitive consequences of high Aβ amyloid in mild cognitive impairment and healthy older adults: Implications for early detection of Alzheimer’s disease. Neuropsychology 27: , 322–332. |
[23] | Engler H , Forsberg A , Almkvist O , Blomquist G , Larsson E , Savitcheva I , Wall A , Ringheim A , Långström B , Nordberg A ((2006) ) Two-year follow-up of amyloid deposition in patients with Alzheimer’s disease. Brain 129: , 2856–2866. |
[24] | Rowe CC , Ng S , Ackermann U , Gong SJ , Pike K , Savage G , Cowie TF , Dickinson KL , Maruff P , Darby D , Smith C , Woodward M , Merory J , Tochon-Danguy H , O’Keefe G , Klunk WE , Mathis CA , Price JC , Masters CL , Villemagne VL ((2009) ) Imaging beta-amyloid burden in aging and dementia. Neurology 68: , 1718–1725. |
[25] | Stern Y ((2009) ) Cognitive reserve. Neuropsychologia 47: , 2015–2028. |
[26] | Deary IJ ((2012) ) Intelligence. Annu Rev Psychol 63: , 453–482. |
[27] | Plassman BL , Williams JW Jr , Burke JR , Holsinger T , Benjamin S ((2010) ) Systematic review: Factors associated with risk for and possible prevention of cognitive decline in later life. Ann Intern Med 153: , 182–193. |
[28] | McKhann G , Drachman D , Folstein M , Katzman R , Price D , Stadlan EM ((1984) ) Clinical diagnosis of Alzheimer’s disease: Report of the NINCDS-ARDRA Work Group under the auspices of Department of Health and Human Services Task Force on Alzheimer’s Disease. Neurology 34: , 939–944. |
[29] | American Psychiatric Association (2000) Diagnostic and statistical manual of mental disorders (DSM-IV-TR), 4th ed, text revision. American Psychiatric Association, Washington DC. |
[30] | Petersen RC ((2004) ) Mild cognitive impairment as a diagnostic entity. J Intern Med 256: , 183–194. |
[31] | Collins DL , Neelin P , Peters TM , Evans AC ((1994) ) Automatic 3D intersubject registration of MR volumetric data in standardized Talairach space. J Comput Assist Tomogr 18: , 192–205. |
[32] | Lezak MD , Howieson DB Loring DW ((2004) ) Neuropsychological Assessment (4th ed.). Oxford University press, New York. |
[33] | Folstein MF , Folstein SE , McHugh PR ((1975) ) “Mini-mental state”. A practical method for grading the cognitive state of patients for the clinician. J Psychiatr Res 12: , 189–198. |
[34] | Crum RM , Anthony JC , Bassett SS , Folstein MF ((1993) ) Population-based norms for the Mini-Mental State Examination by age and educational level. JAMA 269: , 2386–2391. |
[35] | Mitrushina M , Boone KB , Razani J , D’Elia LF ((2005) ) Handbook of normative data for neuropsychological assessment. Oxford University press, New York. |
[36] | Li HJ , Hou XH , Liu HH , Yue CL , He Y , Zuo XN ((2015) ) Toward systems neuroscience in mild cognitive impairment and Alzheimer’s disease: A meta-analysis of 75 fMRI studies. Hum Brain Mapp 36: , 1217–1232. |
[37] | Thal DR , Rüb U , Orantes M , Braak H ((2002) ) Phases of A beta-deposition in the human brain and its relevance for the development of AD. Neurology 58: , 1791–1800. |
[38] | Villemagne VL , Ataka S , Mizuno T , Brooks WS , Wada Y , Kondo M , Jones G , Watanabe Y , Mulligan R , Nakagawa M , Miki T , Shimada H , O’Keefe GJ , Masters CL , Mori H , Rowe CC ((2009) ) High striatal amyloid beta-peptide deposition across different autosomal Alzheimer disease mutation types. Arch Neurol 66: , 1537–1544. |
[39] | Ferbinteanu J ((2020) ) The hippocampus and dorsolateral striatum integrate distinct types of memories through time and space, respectively. J Neurosci 40: , 9055–9065. |
[40] | Leech R , Sharp DJ ((2014) ) The role of the posterior cingulate cortex in cognition and disease. Brain 137: , 12–32. |
[41] | Scheltens NME , van der Weijden K , Adriaanse SM , van Assema D , Oomen PP , Krudop WA , Lammertsma AA , Barkhof F , Koene T , Teunissen CE , Scheltens P , van der Flier WM , Pijnenburg YAL , Yaqub M , Ossenkoppele R , van Berckel BNM ((2018) ) Hypometabolism of the posterior cingulate cortex is not restricted to Alzheimer’s disease. Neuroimage Clin 19: , 625–632. |
[42] | Bressler SL , Menon V ((2010) ) Large-scale brain networks in cognition: Emerging methods and principles. Trends Cogn Sci 14: , 277–290. |
[43] | Liljeholm M , O’Doherty JP ((2012) ) Contributions of the striatum to learning, motivation, and performance: An associative account. Trends Cogn Sci 16: , 467–475. |
[44] | de Jong LW , van der Hiele K , Veer IM , Houwing JJ , Westendorp RG , Bollen EL , de Bruin PW , Middelkoop HA , van Buchem MA , van der Grond J ((2008) ) Strongly reduced volumes of putamen and thalamus in Alzheimer’s disease: An MRI study. Brain 131: , 3277–3285. |
[45] | Postuma RB , Dagher A ((2006) ) Basal ganglia functional connectivity based on a meta-analysis of 126 positron emission tomography and functional magnetic resonance imaging publications. Cereb Cortex 16: , 1508–1521. |
[46] | Terada S , Sato S , Nagao S , Ikeda C , Shindo A , Hayashi S , Oshima E , Yokota O , Uchitomi Y ((2013) ) Trail making test B and brain perfusion imaging in mild cognitive impairment and mild Alzheimer’s disease. Psychiatry Res 213: , 249–255. |
[47] | Hellstrøm T , Westlye LT , Sigurdardottir S , Brunborg C , Soberg HL , Holthe Ø , Server A , Lund MJ , Andreassen OA , Andelic N ((2017) ) Longitudinal changes in brain morphology from 4 weeks to 12 months after mild traumatic brain injury: Associations with cognitive functions and clinical variables. Brain Inj 31: , 674–685. |
[48] | Eijsker N , van Wingen G , Smolders R , Smit DJA , Denys D ((2020) ) Exploring the role of the nucleus accumbens in adaptive behavior using concurrent intracranial and extracranial electrophysiological recordings in humans. eNeuro 7: , ENEURO.0105-20.2020. |
[49] | Cabeza R , Nyberg L ((2000) ) Imaging cognition II: An empirical review of 275 PET and fMRI studies. J Cogn Neurosci 12: , 1–47. |
[50] | Yeo BT , Krienen FM , Sepulcre J , Sabuncu MR , Lashkari D , Hollinshead M , Roffman JL , Smoller JW , Zöllei L , Polimeni JR , Fischl B , Liu H , Buckner RL ((2011) ) The organization of the human cerebral cortex estimated by intrinsic functional connectivity. J Neurophysiol 106: , 1125–1165. |
[51] | Jones SE , Idris A , Bullen JA , Miller JB , Sarah J. Banks SJ ((2019) ) Relationship between cortical thickness and fluency in the memory disorders clinic population. Neuropsychologia 129: , 294–301. |
[52] | Eastman JA , Hwang KS , Lazaris A , Chow N , Ramirez L , Babakchanian S , Woo E , Thompson PM , Apostolova LG ((2013) ) Cortical thickness and semantic fluency in Alzheimer’s disease and mild cognitive impairment. Am J Alzheimers Dis (Columbia) 1: , 81–92. |
[53] | Vonk JMJ , Bouteloup V , Mangin JF , Dubois B , Blanc F , Gabelle A , Ceccaldi M , Annweiler C , Krolak-Salmon P , Belin C , Rivasseau-Jonveaux T , Julian A , Sellal F , Magnin E , Chupin M , Habert MO , Chêne G , Dufouil C ; MEMENTO cohort Study Group ((2020) ) Semantic loss marks early Alzheimer’s disease-related neurodegeneration in older adults without dementia. Alzheimers Dement (Amst) 12: , e12066. |
[54] | Tzelgov J , Henik A ((1991) ) Suppression situations in psychological research: Definitions, implications, and applications. Psychol Bull 109: , 524–535. |
[55] | Jack AI , Dawson AJ , Begany KL , Leckie RL , Barry KP , Ciccia AH , Snyder AZ ((2013) ) fMRI reveals reciprocal inhibition between social and physical cognitive domains. Neuroimage 66: , 385–401. |
[56] | Edison P , Carter SF , Rinne JO , Gelosa G , Herholz K , Nordberg A , Brooks DJ , Hinz R ((2013) ) Comon of MRI based and PET template based approaches in the quantitative analysis of amyloid imaging with PIB-PET. Neuroimage 70: , 423–433 paris. |
[57] | Ferreira D , Nordberg A , Westman E ((2020) ) Biological subtypes of Alzheimer disease: A systematic review and meta-analysis. Neurology 94: , 436–448. |