Enlarged Perivascular Spaces and Dementia: A Systematic Review
Abstract
Perivascular compartments surrounding the penetrating arteries in the brain are part of a physiologic system, which facilitates fluids exchange and clearance of solutes from the brain. The perivascular compartments become visible on MRI when enlarged and are commonly referred to as perivascular spaces (ePVS). Previous studies on the association between ePVS and dementia have been inconsistent due to varying methods of measuring ePVS. As a frame of reference for future MRI studies on ePVS, we systematically review the literature on ePVS as a marker of vascular brain injury related to dementia from population-based as well as hospital-based settings. We identified three longitudinal and ten cross-sectional studies involving 7,581 persons. Potential outcomes were all-cause dementia, Alzheimer’s disease, and vascular dementia. There was considerable heterogeneity in ePVS assessment: with studies using either visual inspection or segmentation, examining different brain locations and implementing different grading scales. Moreover, out of the total of 13 studies, all five studies on vascular dementia reported an association with presence of basal ganglia ePVS after adjustment for age, gender, and white matter hyperintensities. For seven studies on Alzheimer’s disease and all-cause dementia, the results were ambiguous. This review did not identify an independent association of ePVS with prevalent or incident dementia. Harmonized methods for ePVS assessment, tested across different populations, may benefit future MRI studies on ePVS and dementia.
INTRODUCTION
Dementia is a common age-related brain disease with an estimated prevalence of 47 million cases worldwide in 2015 [1]. Vascular pathology is commonly implicated in both vascular dementia (VaD) and Alzheimer’s disease (AD), the two main subtypes of dementia [2]. This vascular pathology affects small vessels in the brain such as arteries, arterioles, venules, and capillaries and hence referred to as cerebral small vessel disease (CSVD) [3, 4]. CSVD results in ischemic, hemorrhagic, and inflammatory damage through diminished blood flow and disruption of the blood-brain barrier [5, 6]. Magnetic resonance imaging (MRI) markers of CSVD include white matter hyperintensities (WMH), lacunes, and microbleeds (CMB) [3]. The presence of these CSVD markers have been associated with a higher incidence of dementia and cognitive decline in the elderly [7, 8].
Accumulating evidence suggest that perivascular compartments may be another feature of CSVD [9]. Perivascular compartments surround perforating cerebral arteries and are involved in the in- and outflow of fluid and solutes between the arteries and the brain interstitial fluid. Vascular stiffening, inflammation, protein deposits, and disruption of the blood-brain barrier, partly due to CSVD decreases the clearance of solutes from the brain interstitial fluid [9, 10]. As a consequence, perivascular compartment may enlarge, which increases their visibility on MRI as spaces with a signal intensity similar to that of cerebrospinal fluid. Recently, enlarged perivascular spaces (ePVS) have been associated with a range of diseases like traumatic brain injury, stroke, and idiopathic normal pressure hydrocephalus. Moreover, the presence of ePVS has been linked with increased amyloid-β (Aβ) deposition in the leptomeningeal arteries [11]. In animal models, it was demonstrated that amyloid drains out of the brain along the basement membranes between smooth muscle cells in the tunica media of cerebral arteries, referred to as the intramural peri-arterial drainage pathway [10]. The ePVS are reported to develop secondary to obstruction by cellular waste and impaired interstitial fluid elimination [11, 12]. Because of the association between ePVS and Aβ clearance, several studies have focused on ePVS and dementia, especially AD.
However, previous studies on the determinants of ePVS and its consequences have been inconsistent with some data reporting associations with dementia whereas others did not. These differences may be attributed to varying methods of assessing ePVS, different study designs and study populations among others. It is important to compile data from published studies on ePVS and dementia, which will provide a frame of reference for future studies on ePVS.
The aim of the present study was to systematically review the literature on MRI studies on ePVS as a marker of brain injury and its relationship to dementia from population-based as well as hospital-based settings.
MATERIALS AND METHODS
Study selection
The Preferred Reporting Items for Systematic Reviews and Meta-Analysis (PRISMA) guidelines were employed in this study [13]. We searched PubMed for peer-reviewed articles published between 1966 and 1 December 2018. The following search terms were included: (PERIVASCULAR or VIRCHOW-ROBIN) and (NEUROIMAGING or MAGNETIC-RESONANCE-IMAGING or MRI or MR) and (ALZHEIMER or VASCULAR-DEMENTIA or MIXED-DEMENTIA or DEMENTIA).
The bibliographies of relevant articles were screened as well. Relevant studies were then selected by screening of titles and abstracts. Retrospective and prospective studies were considered eligible if they assessed ePVS on MRI at baseline with follow-up for the development of dementia. Cross-sectional studies were eligible if they assessed ePVS on MRI for patients with dementia and controls. Moreover, NOCTH 3 gene hereditary disorder, i.e., cerebral autosomal dominant arteriopathy with subcortical infarcts and leukoencephalopathy (CADASIL), which are likely to affect cognition due to vascular damage were also included [14]. Other conditions such as traumatic brain injury, sleep disturbance, or hydrocephalus which may affect the cerebral drainage system through non-vascular causes were excluded.
All included studies should define ePVS as follows: fluid-filled spaces that follow the typical course of an artery as it goes through the grey or white matter [4]. The STRIVE consortium recommend 3 mm as a size criterion to discriminate perivascular spaces from lacunes of presumed vascular origin, as lesions less than 3 mm in diameter are more likely to be perivascular spaces. On MRI, PVS are mostly linear with signal intensity similar to that of cerebrospinal fluid on all sequences. Perivascular spaces can be found in the basal ganglia, white matter, and brainstem [4]. Lacunes are mostly round or ovoid with a hyperintensive rim on MRI.
This review was limited to studies that examined ePVS in the basal ganglia (BG-PVS) and/or white matter (WM-PVS), as these brain areas are especially of interest in CSVD and are most commonly examined in previous research due to higher abundance of PVS in these brain regions. For the outcome, the analysis was restricted to results on all-cause dementia, AD, or VaD. Both clinical diagnoses by neurologists, as well as use of common diagnostic criteria for dementia such as the Diagnostic and Statistical Manual of Mental Disorders-Fourth Edition (DSM-IV), National Institute of Neurological and Communicative Disorders and Stroke and the Alzheimer’s Disease and Related Disorders Association (NINCDS-ADRDA) for AD or National Institute of Neurological Disorders and Stroke and Association Internationale pour la Recherché et l’ Enseignement en Neurosciences (NINDS-AIREN) for VaD were accepted [3, 15]. Postmortem studies, narrative reviews, editorials, and studies in languages other than English were excluded.
Data extraction
From all studies the following information were extracted: number of participants, mean age, study population, characteristics of the MRI protocol (field strength, sequences used), methodology for identification of ePVS, adjustment variables, and outcome definition. We also recorded the availability of effect estimates, i.e., hazard ratios and odds ratios.
RESULTS
The search terms resulted in 176 articles and review of references returned 1 more article. Thirteen articles met the aforementioned criteria (Fig. 1) [16–28]. Three studies analyzed longitudinal data [16, 17, 19] and ten were cross-sectional studies [18, 20–28]. Table 1 shows an overview of the included studies.
Fig.1
Flow-chart of article retrieval procedure.
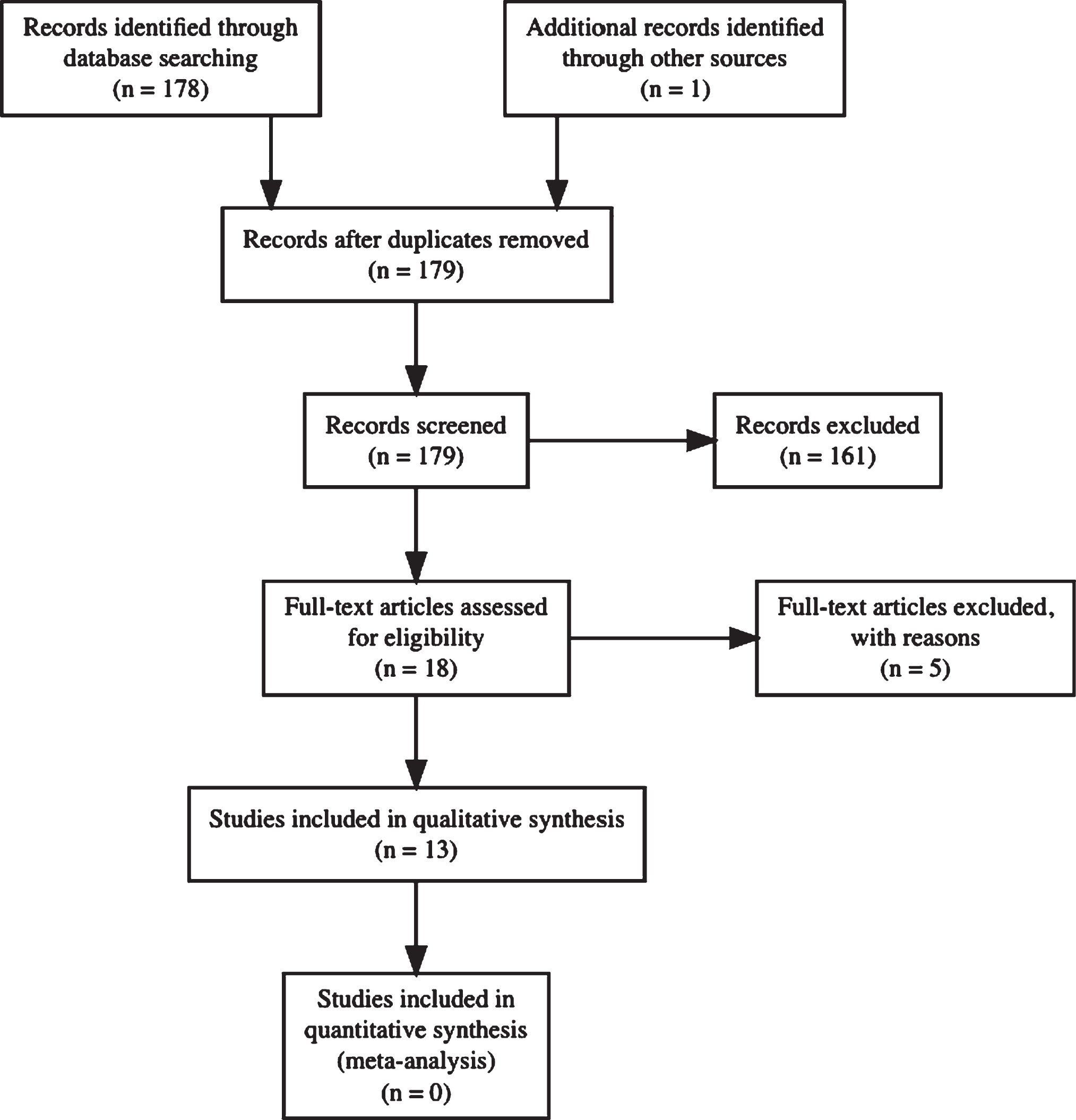
Table 1
Overview of included studies
Study | Year | Participants | Design | No. of subjects | MRI | Sequences | Mean Age (y) | ePVS locations | ePVS assessment |
Shams et al. [23] | 2017 | Memory clinic | Cross-sectional | 1504 | 1.5,3T | T2 | 63 | BG and WM | Count |
Banerjee et al. [28] | 2017 | Memory clinic | Cross-sectional | 226 | 3T | T1, T2, FLAIR | 72 | BG and WM | Count |
Cai et al. [24] | 2015 | General population | Cross-sectional | 8 | 7T | T2 | 78 | WM only | Volume |
Ramirez et al. [26] | 2015 | General population | Cross-sectional | 297 | 1.5T | T1, T2 | 72 | BG, WM and Total | Volume |
Hansen et al. [22] | 2015 | General population | Cross-sectional | 151 | 1.5T | T1, FLAIR | 75 | BG and WM | Count |
Burnett et al. [27] | 2014 | Hospital patients | Retrospective case-control | 79 | 1.5,3T | FLAIR | 83 | BG only | Count |
Yao et al. [18] | 2013 | CADASIL patients | Cross-sectional | 344 | 1.5T | T1, T2, FLAIR | 51 | BG and WM | Count |
Chen et al. [25] | 2011 | Memory clinic | Cross-sectional | 158 | 3T | T1 | 75 | BG, WM and Total | Count |
Patankar et al. [21] | 2004 | General population | Cross-sectional | 110 | 1.5T | T1, T2 | 73 | BG and WM | Count |
Heier et al. [20] | 1989 | Hospital patients | Case-control | 176 | 1.5T | ‘Echo’ | ** | BG and WM | Count |
Xiong et al. [19] | 2017 | CAA | Retrospective | 158 | 1.5T | T2 | 74 | BG and WM | Count |
Ding et al. [16] | 2017 | General population | Prospective | 2592 | 1.5T | T2, FLAIR | 75 | BG, WM and Total | Count |
Zhu et al. [17] | 2010 | General population | Prospective | 1778 | 1.5T | T1, T2 | 73 | BG and WM | Count |
ACD, all-cause dementia; AD, Alzheimer’s disease; VaD, vascular dementia; C, controls; WM, white matter; BG, basal ganglia; CADASIL, cerebral autosomal dominant arteriopathy with subcortical infarcts and leukoencephalopathy; CAA, cerebral amyloid angiopathy. **Not described.
Study populations
The review included 13 populations, with a total of 7,581 unique subjects. The sample size of individual studies ranged from 8 to 2,592 subjects. Within the cross-sectional studies, comparisons were done between subjects with AD (n = 864), VaD (n = 254), and normal controls. Three studies compared subjects with all-cause dementia to normal controls [18, 20, 27]. The mean age ranged from 50 to 83 years. The cross-sectional studies examined subjects from the general population [21, 22, 24–26], subjects diagnosed with CADASIL [18], and subjects who visited hospital for a wide range of neurological disorders such as memory or stroke clinics [20, 23, 27, 28].
The three longitudinal studies followed 4,528 unique subjects, with a mean age of 73.7 years. The follow-up time ranged from 2.3 years (median) to 5.2 years (mean). Two studies reported the number of cases of all-cause dementia [17, 19], whereas one study specified the subtypes, i.e., AD and VaD [16]. Two studies examined the general population and one study examined subjects with probable CAA. Exclusion criteria in most of these studies included: history of traumatic brain injury, presence of Parkinson’s disease, prior stroke, mixed-dementia, and presence of other neurologic diseases.
Measurement of ePVS
Although all studies defined ePVS, which fulfill the recently developed criteria of STandards for ReportIng Vascular changes on nEuroimaging (STRIVE) [3], multiple strategies were used for actual measurement of ePVS. Most commonly, ten studies counted all visible ePVS [17–23, 25, 27, 28] and one study counted only ePVS with a diameter larger than 3 mm [16]. Two studies measured the volume of ePVS [24, 26]. For location, eight studies measured ePVS separately in the WM (primarily the centrum semiovale) and BG. One study restricted analysis to ePVS in BG. Furthermore, different definitions were used to determine ‘severe’ burden of ePVS which were either based on categories (derived from counts data), size, or weightage of PVS. The following definitions were encountered: presence of >20 ePVS [19, 23, 27], presence of >10 ePVS [17, 18, 28], presence of ePVS > 2 mm [20], presence of ePVS > 3 mm [16], and weighting in the location of ePVS [21, 22]. Three studies used continuous measures for ePVS [22, 24, 26].
The most widely used scale was adapted from Doubal et al. [29]. This scale codes the burden of ePVS as following: 0 = no ePVS, 1 = 1–10 ePVS, 2 = 11–20 ePVS, 3 = 21–40 ePVS, and 4 = >40 ePVS. The number of ePVS was taken from the MRI slide with the most ePVS on standard axial images, and in case of asymmetry the most affected hemisphere was taken.
Eight studies used MRIs with field strength of 1.5 Tesla (1.5T) only. Four studies used both 1.5T and 3T or only 3T, and the remaining study used 7T MRI. For MRI protocols, nine studies analyzed ePVS on T2 sequence, the remaining four used T1 and/or Fluid Attenuated Inversion Recovery (FLAIR) sequences. Table 1 shows an overview of the methodologies of individual studies.
Prevalence of ePVS in subjects with dementia
Four studies specifically reported the burden of ePVS in subjects with dementia [21–23, 25]. All studies reported the presence of at least one visible ePVS in the WM or BG. In one 3T MRI study, 10.8% of AD subjects (mean age 74.6 years) had ‘diffuse’ ePVS, defined as ePVS in the WM, BG, hippocampus, and brainstem. These AD subjects had a total of 17 ePVS on average [25]. Another 3T MRI study found 40.9% of the subjects with AD related pathology to have severe WM-PVS compared to 14.7% of the subjects with subcortical vascular cognitive impairment. In the same study, 0.91% of subjects with AD related pathology showed severe BG-PVS compared to 9.5% of the subcortical vascular cognitive impairment [28]. In a study combining both 1.5T and 3T MRI, 21.3% of the AD subjects had more than 20 WM-ePVS and 5.4% had more than 20 BG-ePVS. For subjects with VaD, 29.6% had >20 WM-ePVS and 7.4% had >20 BG-ePVS [23]. A 1.5T MRI study reported ePVS for both AD (mean age, 74.1 years) and VaD (mean age, 76.9 years) subjects according to the following scale: 0 = no ePVS, 1 = 1–10, 2 = 11–20, 3 = 21–30, 4 = 31–40, and 5 = >40. The mean WM-ePVS scores were 2.61 (SD 0.1) for AD and 2.53 (SD 0.15) for VaD. The mean BG-ePVS scores were 2.22 (SD 0.09) for AD and 2.47 (SD 0.87) for VaD [22]. Another 1.5T MRI study on AD (mean age, 61.6 years) and VaD (mean age, 64.5 years) subjects, reported a mean WM-ePVS score of 0.74 (SD 0.6) and 1.03 (SD 0.39), respectively, where a score of 2 (the maximum) indicated more than 5 ePVS on one or both hemispheres [21]. Two studies utilized automatic segmentation to measure ePVS volume [24, 26]. The ‘quantified white matter PVS density’ in volume percentage on 7T MRI was 8.0 v/v% among 5 subjects with AD (mean age, 78.2 years) [24]. The ‘median white matter volume’ of PVS was 31.7 mm3 and the ‘median basal ganglia volume’ of PVS was 10.6 mm3 for subjects with AD (mean age, 72.7 years) [26].
Adjustment for covariates in ePVS studies
Besides one paper [24], all studies performed statistical analysis on the association between ePVS and dementia diagnosis within the context of multivariate models. Variables were included in statistical analysis on the basis of biology of the disease, as well as by significance testing, i.e., selection of only those variables with p-values less than 0.05 or 0.1 in preliminary analysis [19, 23]. Commonly included variables were age, gender, other MRI-markers, cardiovascular risk factors, education, and cognitive scores. Table 2 gives a full overview of variables included.
Table 2
Variables included in multivariate models
Study | Year | (Subsets of) variables included in models for statistical analysis |
Cai et al. [24] | 2015 | – |
Ramirez et al. [26] | 2015 | Age, Sex, Education, MMSE |
Hansen et al. [22] | 2015 | Age, Sex, WMH |
Patankar et al. [21] | 2004 | Age, Sex, WMH, Atrophy |
Chen et al. [25] | 2011 | Age, Sex, WMH, Atrophy, vascular risk factors, MMSE |
Shams et al. [23] | 2017 | Age, HT, WMH, CAA, MBs, CSS |
Yao et al. [18] | 2013 | Age, Sex, Education, ICH, WMH, CMB, Lacunes |
Heier et al. [20] | 1989 | Age, Sex, HT, WMH |
Burnett et al. [27] | 2014 | Age, Sex (matched) |
Xiong et al. [19] | 2017 | Age, MCI, GCA, MTA, SVD score |
Banerjee et al. [28] | 2017 | Age, HT, Diabetes, Cholesterol, Stroke, PiB positivity, APOE genotype, Lacunes, deep CMB |
Ding et al. [16] | 2017 | Age, Sex, BMI, Education, Depression, Smoking, HT, Cholesterol, WMH, Lacunes, MB, APOE genotype |
Zhu et al. [17] | 2010 | Age, Sex, Education, Depression, APOE ɛ4, vascular risk factors, WMH, Lacunes, BPF |
MMSE, Mini-Mental State Examination; WMH, white matter hyperintensities; HT, hypertension; CAA, cerebral amyloid angiopathy; MB, microbleeds; CSS, cortical superficial siderosis; GCA, global cortical atrophy; MCA, medial cortical atrophy; SVD, small vessel disease; BPF, brain parenchymal fraction; PD, Parkinson’s disease; TBI, traumatic brain injury; ICH, intracranial hemorrhage.
ePVS and prevalent dementia
Seven cross-sectional studies examined a link between ePVS and diagnosis of AD [21–26, 28]. Two out of six studies reported an association between BG-PVS and presence of AD [22, 25]. For WM-PVS, four out of seven studies found an association with presence of AD [22, 25, 26, 28]. The total volume of ePVS was not associated with diagnosis of AD, but for subjects within the top two quartiles, i.e., with a ‘severe’ volume of ePVS, AD diagnosis was more likely [26]. The single prospective study examining the incidence of AD did not find an association with presence of large PVS (>3 mm) at baseline [16]. Table 3 summarizes the studies on ePVS and AD.
Table 3
Cross-sectional analysis of ePVS and AD
Author | MRI | Exposure | Results (OR if reported with 95% CI) |
Shams et al. [23] | 1.5, 3T | >20 BG-PVS | AD, OR 3.2 (95% CI:0.4–25.6) |
>20 CSO-PVS | OR 1.0 (95% CI: 0.6–1.7) | ||
Hansen et al. [22] | 1.5T | BG-PVS score | Higher score in AD, p < 0.001 |
CSO-PVS score | No intergroup difference | ||
Total-PVS score | Total PVS score higher in AD compared to NC | ||
Patankar et al. [21] | 1.5T | BG-PVS score | No difference compared to NC |
CSO-PVS score | No difference compared to NC | ||
Banerjee et al. [28] | 3T | >10 BG-PVS | AD, OR 0.09 (95% CI: 0.04–0.21) |
>10 CSO-PVS | AD, OR 5.7 (95% CI: 3.0–10.8) | ||
Ramirez et al. [26] | 1.5T | BG-PVS volume | No significant difference |
WM-PVS volume | PVS volume in AD > C, p < 0.01 | ||
Chen et al. [25] | 3T | BG-PVS count | F = 3.52–13.76, p < 0.05 for PVS subscales |
WM-PVS count | F = 3.52–13.76, p < 0.05 for PVS subscales | ||
Cai et al. [24] | 7T | WM-PVS volume | Higher volume in AD compared NC, p < 0.05 |
AD, Alzheimer’s disease; VaD, vascular dementia; OR, odds ratio; CADASIL, cerebral autosomal dominant arteriopathy with subcortical infarcts and leukoencephalopathy.
Four cross-sectional studies examined the association between ePVS and diagnosis of VaD [21–23, 28]. All four studies found BG-ePVS to be associated with presence of VaD, except for one study on memory clinic patients, where the association on high burden of BG-ePVS (>20) and VaD attenuated in the presence of age, hypertension, probable CAA, presence of lacunes, presence of moderate to severe WMH, probable CAA, strictly deep CAA, strictly lobar CAA, CMB presence, presence of high CS-PVS grade (>20) and presence of cSS [23]. An association between increased burden of WM-ePVS and presence of VaD was reported for two of the four studies [21]. By contrast, another study comparing AD and VaD subjects reported that a high number of WM-ePVS was only associated with AD [28]. Only one prospective study reported an association between the presence of large BG-PVS (>3 mm) with incidence of VaD [16]. Table 4 summarizes the studies on ePVS and VaD.
Table 4
Cross-sectional analysis of ePVS and VaD
Author | MRI | Exposure | Results (OR if reported with 95% CI) |
Shams et al. [23] | 1.5, 3T | >20 BG-PVS | OR 11.1 (95% CI: 1.1–112.2) |
>20 CSO-PVS | OR 1.3 (95% CI: 0.6–2.9) | ||
Hansen et al. [22] | 1.5T | BG-PVS score | Higher score in VaD, p < 0.001 |
CSO-PVS score | No intergroup difference | ||
Total-PVS score | Total PVS score higher in VaD compared to NC | ||
Patankar et al. [21] | 1.5T | BG-PVS score | Higher score in VaD, p < 0.001 |
CSO-PVS score | Higher score among VaD, p < 0.01 | ||
Banerjee et al. [28] | 3T | >10 BG-PVS | VaD OR 10.8 (95% CI: 4.6–25.2) |
>10 CSO-PVS | VaD OR 0.18 (95% CI: 0.09–0.34) |
AD, Alzheimer’s disease; VaD, vascular dementia; OR, odds ratio; CADASIL, cerebral autosomal dominant arteriopathy with subcortical infarcts and leukoencephalopathy; *Odds Ratios represent results from univariate analysis, multi-variate analysis was not reported.
Three cross-sectional studies examined ePVS in relation to presence of all-cause dementia [18, 20, 27]. Of these three, one study involving all-cause dementia from hospital records, reported that the association attenuated after adjustment for age [20]. Another hospital based case-control study did not find the diagnosis of dementia to be more frequent among cases with high burden of BG-ePVS compared to controls [27]. For subjects with CADASIL, presence of WM-ePVS was associated with diagnosis of all-cause dementia in a baseline model, but no significant difference was found after adjustment for WMH volume, lacunes, and CMBs [18]. In the same study an association between presence of BG-ePVS and all-cause dementia attenuated after adjustment for the ‘Brain Parenchymal Fraction’.
ePVS and incident dementia
Three longitudinal studies examined all-cause dementia [16, 17, 19]. An increased incidence of all-cause dementia for subjects with high burden of WM-ePVS (>10), was reported in a 1.5T MRI study using 6135 person-years of follow-up time (24 cases) [17]. In a retrospective cohort consisting of subjects with probable CAA without intracranial hemorrhage, a borderline association between high burden of WM-ePVS (>20 PVS) and incidence of dementia was observed which attenuated after adjusting for age, mild cognitive impairment at baseline, WMH grade (Fazekas score), global cortical atrophy, medial temporal atrophy, and the cerebral small vessel disease [19]. Baseline presence of large PVS (>3 mm) was associated with higher incidence of dementia (AD and VaD combined) in a model that adjusted for age, gender, and timing of the MRI-scan [16] (Table 5).
Table 5
ePVS and incidence of dementia
Study | Participants | Sample size | Exposure (presence of) | Outcome | Result | Follow-up |
Zhu et al. [17] | General population | 1778 | >10 WM-ePVS | All cause dementia or controls | HR 8.1 (95% CI:1.4–47.5) for all cause dementia | 3.5 years (median) |
>10 BG-ePVS | HR 3.4 (95% CI:0.5–22.1) for all cause dementia | |||||
Ding et al. [16] | General population | 2592 | 1 large BG-PVS (>3 mm) | All cause dementia, AD, VaD or controls | Increased HR for VaD (not reported) | 5.2 years (mean) |
1 large PVS total (>3 mm) | HR 3.34 (95% CI:1.41–7.93) for VaD | |||||
Xiong et al. [19] | CAA | 158 | >20 WM-ePVS | All cause dementia or controls | HR 1.28 (95% CI: 0.97–1.70) for all cause dementia* | 2.3 years (median) |
>20 BG-PVS | Difference not significant (not reported) |
AD, Alzheimer’s disease; VaD, vascular dementia; HR, hazard ratio; CAA, cerebral amyloid angiopathy. *Hazard ratio represent result from univariate analysis, multi-variate analysis result not reported.
DISCUSSION
This systematic review showed considerable heterogeneity in the assessment of ePVS and its link with dementia, which did not permit a valid meta-analysis. Multiple approaches for ePVS measurement (including size) and grading were identified within the span of 13 studies.
In both cross-sectional and longitudinal studies, multiple aspects of design and methodology might have influenced the results on the association between ePVS and dementia. Choices regarding the MRI protocol, cut-off values for ePVS quantification, brain region(s) for ePVS assessment, development of multivariate models and study populations, all potentially influenced the reported association between ePVS and dementia.
The results from previous studies offer minimal support for an association between BG-ePVS and VaD. All four cross-sectional studies and the single prospective study found BG-ePVS to be associated with presence of VaD. For AD, the results are less clear; four out of seven studies reported an association between WM-ePVS and presence of AD. An association of WM-ePVS and BG-ePVS with AD and VaD would suggest that the distribution of ePVS partly reflects the underlying disease process. Although there appears to be considerable overlap in BG-ePVS and WM-ePVS pathology [23], earlier studies did show BG-ePVS to be associated with the presence of hypertensive vasculopathy and WM-ePVS with presence of CAA [11, 30–32].
Impaired clearance of cerebral interstitial fluid and solutes is associated with different concurrent processes like inflammation, blood-brain barrier disruption, and impaired vascular pulsatility. The ePVS may be a biomarker for impaired waste clearance as well as CSVD, because deposition of Aβ plaques and hypertensive arteriosclerosis appear to be involved [9, 33]. Indeed, it has been shown before that perivascular spaces can only be detected around penetrating arteries in the basal ganglia and white matter. Perivascular spaces are not seen around cortical arteries as layers of smooth muscle, basement membrane, and one layer of leptomeninges are all compressed together [34]. The included populations showed considerable differences in the presence of CSVD risk factors. For example, the prevalence of hypertension ranged from 25% to about 80%. Two studies excluded subjects with prior (silent) stroke [19, 22]. Other studies specifically examined subjects with a high burden of CSVD [18, 19]. Enlarged perivascular spaces were associated with poor sleep quality among patients evaluated for cerebrovascular disease [35]. None of the studies considered for inclusion in this review excluded subjects due to presence of sleep disorders. One case-control study mentioned the number of included subjects with sleep disorders. Here, sleep disorders were not frequent among cases, i.e., those with an ‘état criblé’ on MRI [27].
With advancing age and high burden of CSVD, mixed pathology is also found in the brain which undermines the predictive abilities of individual brain regions for AD or VaD. Increasing burden of WMH with advancing age might obliterate ePVS grading [22]. Several studies which did adjust for variables like age, WMH, lacunes, and brain atrophy, reported an attenuation in the link between ePVS and dementia [17, 19, 20, 23]. Knowledge on potential confounders should be incorporated to elucidate to which extend the association between ePVS and dementia is influenced by other factors.
The studies that found an association between WM-ePVS and AD used stronger field strength: mostly 3T versus primarily 1.5T. The visibility of ePVS is influenced by the field strength and protocol of the MRI scanner. A comparison of 1.5T and 3T MRI, showed better definition of ePVS in the higher field strength, i.e., 3T MRI [26]. However, detection of more physiologic ePVS may result in over estimation of ePVS. A 7T MRI study on ePVS found on average 71 PVS (SD 28) in the WM among the general population. According to the methods encountered in the included studies, all subjects with 71 WM-ePVS would be placed in the highest category of ePVS burden (severe) [36]. The 7T MRI may have additional value through improved detection, morphological analysis, and 3D tracing of PVS. Further, the perforating arteries of PVS can be visualized noninvasively, hence vascular changes in relation to enlargement of PVS can be studied in vivo. However, few clinical 7T MRI studies on CSVD have been published and it will take time to develop new methodological standards. In this regard, (semi) automated methods become a necessity, as visual counting on 7T is not feasible due to increased number of visible perivascular spaces.
The visibility of PVS on MRI might not be sufficient to label them as pathologically enlarged. In a pathology study, size did appear to be the most important factor to discriminate PVS from lacunar infarcts [38]. Most ePVS are smaller than 3 mm, but the distinction from lacunes can be difficult and multiple studies acknowledged the potential misclassification of lacunes and ePVS. The location for ePVS measurement might have affected the results of studies as well. A significant association between WM-ePVS and dementia incidence, could not be replicated by another study on hippocampal ePVS within the same population, despite 74% more person-years of follow-up [17, 39].
The two studies that used automated segmentation for ePVS found an association between WM-ePVS and AD. These automated approaches take into account larger parts of the brain and therefore might be more representative. Visual counting of PVS is labor intensive and selection of the most severe slide might add subjectivity to the measurement. A recent study reported a good generalizability of single-slice counting to the total PVS within the whole brain [40]. On the contrary, another study found poor correlations between the single-slice visual rating scale from Doubal et al. (which selects the most severely affected slide) and whole-brain volumetrics of the WM-ePVS (ρ= 0.41) or BG-ePVS (ρ= 0.38) [26]. There is no international standard on ePVS assessment yet. However, the visual grading scale developed by Doubal et al. remains the most widely used in previous studies due to its easy administration. A recently published meta-analysis on cognitive impairment and dementia was able to harmonize ratings across five different studies [41]. Such initiatives might improve validation of results across countries. The cross-sectional studies in this review were unable to answer whether ePVS preceded other aspects of CSVD. CADASIL appeared to accelerate development of ePVS among subjects who are 50 years old as compared to general population where the higher burden of ePVS are only seen two decades later [18].
The strength of this review is that it gives a representative overview of current literature on ePVS and dementia, using a reproducible search strategy. This review identified strong heterogeneity in the assessment of ePVS. Because of the limited number of studies and use of multiple study-designs, the use of a validated questionnaire for quality assessment was not feasible. To present a comprehensive overview, both prospective and cross-sectional designs, small and large samples, and different study populations were accepted for inclusion. It was not possible to assess publication bias, because studies on ePVS are not necessarily registered in trial registries. We encountered considerable heterogeneity in methodologies and, possibly as a consequence, found inconsistent results on the association between ePVS and dementia.
Although guidelines exist on MR imaging of ePVS, a ‘gold standard’ for valid assessment of ePVS is lacking. Unfortunately, we were unable to overcome these aspects with a valid meta-analysis, due to insufficient effect measures and different study designs. Some aspects intrinsic to the studies might have influenced this review. Two studies of case-control design that retrospectively checked hospital records for cases of dementia questions the reliability of their case definition [20, 27]. The use of more consistent inclusion, and exclusion criteria, could help distinguish the role of CSVD from other pathogenic factors, like the presence of stroke, traumatic brain injury, or sleep disorder, that also contribute to impaired solute clearance and the development of dementia.
Conclusion
This review encountered considerable heterogeneity in the assessment of ePVS. More longitudinal data and analysis of ePVS within multivariate models that incorporate current understanding could help further elucidate the role of ePVS in dementia. Consistent and harmonized use of methods, tested across different populations, would benefit future MRI studies on the association between ePVS and dementia.
DISCLOSURE STATEMENT
Authors’ disclosures available online (https://www.j-alz.com/manuscript-disclosures/19-0527r2).
REFERENCES
[1] | Wu Y-T , Beiser AS , Breteler MMB , Fratiglioni L , Helmer C , Hendrie HC , Honda H , Ikram MA , Langa KM , Lobo A , Matthews FE , Ohara T , Pérès K , Qiu C , Seshadri S , Sjölund B-M , Skoog I , Brayne C ((2017) ) The changing prevalence and incidence of dementia over time - current evidence. Nat Rev Neurol 13: , 327–339. |
[2] | Toledo JB , Arnold SE , Raible K , Brettschneider J , Xie SX , Grossman M , Monsell SE , Kukull WA , Trojanowski JQ ((2013) ) Contribution of cerebrovascular disease in autopsy confirmed neurodegenerative disease cases in the National Alzheimer’s Coordinating Centre. Brain 136: , 2697–2706. |
[3] | Wardlaw JM , Smith EE , Biessels GJ , Cordonnier C , Fazekas F , Frayne R , Lindley RI , O’Brien JT , Barkhof F , Benavente OR , Black SE , Brayne C , Breteler M , Chabriat H , Decarli C , de Leeuw F-E , Doubal F , Duering M , Fox NC , Greenberg S , Hachinski V , Kilimann I , Mok V , Oostenbrugge R van , Pantoni L , Speck O , Stephan BCM , Teipel S , Viswanathan A , Werring D , Chen C , Smith C , van Buchem M , Norrving B , Gorelick PB , Dichgans M , STandards for ReportIng Vascular changes on nEuroimaging (STRIVE v1) ((2013) ) Neuroimaging standards for research into small vessel disease and its contribution to ageing and neurodegeneration. Lancet Neurol 12: , 822–838. |
[4] | Pantoni L ((2010) ) Cerebral small vessel disease: From pathogenesis and clinical characteristics to therapeutic challenges. Lancet Neurol 9: , 689–701. |
[5] | Kisler K , Nelson AR , Montagne A , Zlokovic BV ((2017) ) Cerebral blood flow regulation and neurovascular dysfunction in Alzheimer disease. Nat Rev Neurosci 18: , 419–434. |
[6] | Wardlaw JM ((2010) ) Blood-brain barrier and cerebral small vessel disease. J Neurol Sci 299: , 66–71. |
[7] | Debette S , Markus HS ((2010) ) The clinical importance of white matter hyperintensities on brain magnetic resonance imaging: Systematic review and meta-analysis. BMJ 341: , c3666. |
[8] | Charidimou A , Shams S , Romero JR , Ding J , Veltkamp R , Horstmann S , Eiriksdottir G , van Buchem MA , Gudnason V , Himali JJ , Gurol ME , Viswanathan A , Imaizumi T , Vernooij MW , Seshadri S , Greenberg SM , Benavente OR , Launer LJ , Shoamanesh A , International META-MICROBLEEDS Initiative ((2018) ) Clinical significance of cerebral microbleeds on MRI: A comprehensive meta-analysis of risk of intracerebral hemorrhage, ischemic stroke, mortality, and dementia in cohort studies (v1). Int J Stroke 13: , 454–468. |
[9] | Brown R , Benveniste H , Black SE , Charpak S , Dichgans M , Joutel A , Nedergaard M , Smith KJ , Zlokovic BV , Wardlaw JM ((2018) ) Understanding the role of the perivascular space in cerebral small vessel disease. Cardiovasc Res 114: , 1462–1473. |
[10] | Albargothy NJ , Johnston DA , MacGregor-Sharp M , Weller RO , Verma A , Hawkes CA , Carare RO ((2018) ) Convective influx/glymphatic system: Tracers injected into the CSF enter and leave the brain along separate periarterial basement membrane pathways. Acta Neuropathol 136: , 139–152. |
[11] | Weller RO , Boche D , Nicoll JAR ((2009) ) Microvasculature changes and cerebral amyloid angiopathy in Alzheimer’s disease and their potential impact on therapy. Acta Neuropathol 118: , 87–102. |
[12] | Rasmussen MK , Mestre H , Nedergaard M ((2018) ) The glymphatic pathway in neurological disorders. Lancet Neurol 17: , 1016–1024. |
[13] | Moher D , Liberati A , Tetzlaff J , Altman DG , PRISMA Group ((2009) ) Preferred reporting items for systematic reviews and meta-analyses: The PRISMA statement. PLoS Med 6: , e1000097. |
[14] | Ikram MA , Bersano A , Manso-Calderón R , Jia J-P , Schmidt H , Middleton L , Nacmias B , Siddiqi S , Adams HHH ((2017) ) Genetics of vascular dementia - review from the ICVD working group. BMC Med 15: , 48. |
[15] | McKhann G , Drachman D , Folstein M , Katzman R , Price D , Stadlan EM ((1984) ) Clinical diagnosis of Alzheimer’s disease: Report of the NINCDS-ADRDA Work Group under the auspices of Department of Health and Human Services Task Force on Alzheimer’s Disease. Neurology 34: , 939–944. |
[16] | Ding J , Sigurðsson S , Jónsson PV , Eiriksdottir G , Charidimou A , Lopez OL , van Buchem MA , Guðnason V , Launer LJ ((2017) ) Large perivascular spaces visible on magnetic resonance imaging, cerebral small vessel disease progression, and risk of dementia: The Age, Gene/Environment Susceptibility-Reykjavik Study. JAMA Neurol 74: , 1105–1112. |
[17] | Zhu Y-C , Dufouil C , Soumaré A , Mazoyer B , Chabriat H , Tzourio C ((2010) ) High degree of dilated Virchow-Robin spaces on MRI is associated with increased risk of dementia. J Alzheimers Dis 22: , 663–672. |
[18] | Yao M , Hervé D , Jouvent E , Duering M , Reyes S , Godin O , Guichard JP , Dichgans M , Chabriat H ((2014) ) Dilated perivascular spaces in small-vessel disease: A study in CADASIL. Cerebrovasc Dis 37: , 155–163. |
[19] | Xiong L , Boulouis G , Charidimou A , Roongpiboonsopit D , Jessel MJ , Pasi M , Reijmer YD , Fotiadis P , Ayres A , Merrill E , Schwab K , Blacker D , Gurol ME , Greenberg SM , Viswanathan A ((2018) ) Dementia incidence and predictors in cerebral amyloid angiopathy patients without intracerebral hemorrhage. J Cereb Blood Flow Metab 38: , 241–249. |
[20] | Heier LA , Bauer CJ , Schwartz L , Zimmerman RD , Morgello S , Deck MD ((1989) ) Large Virchow-Robin spaces: MR-clinical correlation. AJNR Am J Neuroradiol 10: , 929–936. |
[21] | Patankar TF , Mitra D , Varma A , Snowden J , Neary D , Jackson A ((2005) ) Dilatation of the Virchow-Robin space is a sensitive indicator of cerebral microvascular disease: Study in elderly patients with dementia. AJNR Am J Neuroradiol 26: , 1512–1520. |
[22] | Hansen TP , Cain J , Thomas O , Jackson A ((2015) ) Dilated perivascular spaces in the Basal Ganglia are a biomarker of small-vessel disease in a very elderly population with dementia. AJNR Am J Neuroradiol 36: , 893–898. |
[23] | Shams S , Martola J , Charidimou A , Larvie M , Granberg T , Shams M , Kristoffersen-Wiberg M , Wahlund L-O ((2017) ) Topography and determinants of magnetic resonance imaging (MRI)-visible perivascular spaces in a large memory clinic cohort. J Am Heart Assoc 6: , e006279. |
[24] | Cai K , Tain R , Das S , Damen FC , Sui Y , Valyi-Nagy T , Elliott MA , Zhou XJ ((2015) ) The feasibility of quantitative MRI of perivascular spaces at 7T. J Neurosci Methods 256: , 151–156. |
[25] | Chen W , Song X , Zhang Y , Alzheimer’s Disease Neuroimaging Initiative ((2011) ) Assessment of the Virchow-Robin Spaces in Alzheimer disease, mild cognitive impairment, and normal aging, using high-field MR imaging. AJNR Am J Neuroradiol 32: , 1490–1495. |
[26] | Ramirez J , Berezuk C , McNeely AA , Scott CJM , Gao F , Black SE ((2015) ) Visible Virchow-Robin spaces on magnetic resonance imaging of Alzheimer’s disease patients and normal elderly from the Sunnybrook Dementia Study. J Alzheimers Dis 43: , 415–424. |
[27] | Burnett MS , Witte RJ , Ahlskog JE ((2014) ) Swiss cheese striatum: Clinical implications. JAMA Neurol 71: , 735–741. |
[28] | Banerjee G , Kim HJ , Fox Z , Jäger HR , Wilson D , Charidimou A , Na HK , Na DL , Seo SW , Werring DJ ((2017) ) MRI-visible perivascular space location is associated with Alzheimer’s disease independently of amyloid burden. Brain 140: , 1107–1116. |
[29] | Doubal FN , MacLullich AMJ , Ferguson KJ , Dennis MS , Wardlaw JM ((2010) ) Enlarged perivascular spaces on MRI are a feature of cerebral small vessel disease. Stroke 41: , 450–454. |
[30] | Klarenbeek P , van Oostenbrugge RJ , Lodder J , Rouhl RPW , Knottnerus ILH , Staals J ((2013) ) Higher ambulatory blood pressure relates to enlarged Virchow-Robin spaces in first-ever lacunar stroke patients. J Neurol 260: , 115–121. |
[31] | Esiri MM , Wilcock GK , Morris JH ((1997) ) Neuropathological assessment of the lesions of significance in vascular dementia. J Neurol Neurosurg Psychiatry 63: , 749–753. |
[32] | Charidimou A , Boulouis G , Pasi M , Auriel E , van Etten ES , Haley K , Ayres A , Schwab KM , Martinez-Ramirez S , Goldstein JN , Rosand J , Viswanathan A , Greenberg SM , Gurol ME ((2017) ) MRI-visible perivascular spaces in cerebral amyloid angiopathy and hypertensive arteriopathy. Neurology 88: , 1157–1164. |
[33] | Weller RO , Hawkes CA , Kalaria RN , Werring DJ , Carare RO ((2015) ) White matter changes in dementia: Role of impaired drainage of interstitial fluid. Brain Pathol 25: , 63–78. |
[34] | MacGregor Sharp M , Bulters D , Brandner S , Holton J , Verma A , Werring DJ , Carare RO ((2019) ) The fine anatomy of the perivascular compartment in the human brain: Relevance to dilatederivascular spaces in cerebral amyloid angiopathy. Neuropathol Appl Neurobiol 45: , 305–308. |
[35] | Berezuk C , Ramirez J , Gao F , Scott CJM , Huroy M , Swartz RH , Murray BJ , Black SE , Boulos MI ((2015) ) Virchow-Robin spaces: Correlations with polysomnography-derived sleep parameters. Sleep 38: , 853–858. |
[36] | Bouvy WH , Zwanenburg JJ , Reinink R , Wisse LE , Luijten PR , Kappelle LJ , Geerlings MI , Biessels GJ ((2016) ) Perivascular spaces on 7 Tesla brain MRI are related to markers of small vessel disease but not to age or cardiovascular risk factors. J Cereb Blood Flow Metab 36: , 1708–1717. |
[37] | Bouvy WH , Biessels GJ , Kuijf HJ , Kappelle LJ , Luijten PR , Zwanenburg JJM ((2014) ) Visualization of perivascular spaces and perforating arteries with 7 T magnetic resonance imaging. Invest Radiol 49: , 307–313. |
[38] | Bokura H , Kobayashi S , Yamaguchi S ((1998) ) Distinguishing silent lacunar infarction from enlarged Virchow-Robin spaces: A magnetic resonance imaging and pathological study. J Neurol 245: , 116–122. |
[39] | Yao M , Zhu Y-C , Soumaré A , Dufouil C , Mazoyer B , Tzourio C , Chabriat H ((2014) ) Hippocampal perivascular spaces are related to aging and blood pressure but not to cognition. Neurobiol Aging 35: , 2118–2125. |
[40] | Adams HHH , Hilal S , Schwingenschuh P , Wittfeld K , van der Lee SJ , DeCarli C , Vernooij MW , Katschnig-Winter P , Habes M , Chen C , Seshadri S , van Duijn CM , Ikram MK , Grabe HJ , Schmidt R , Ikram MA ((2015) ) A priori collaboration in population imaging: The Uniform Neuro-Imaging of Virchow-Robin Spaces Enlargement consortium. Alzheimers Dement (Amst) 1: , 513–520. |
[41] | Hilal S , Tan CS , Adams HHH , Habes M , Mok V , Venketasubramanian N , Hofer E , Ikram MK , Abrigo J , Vernooij MW , Chen C , Hosten N , Volzke H , Grabe HJ , Schmidt R , Ikram MA ((2018) ) Enlarged perivascular spaces and cognition: A meta-analysis of 5 population-based studies. Neurology 91: , e832–e842. |