Effectiveness of Simultaneous Combined Intervention for Enhancing Cognitive Function in Patients with Moderate Alzheimer’s Disease
Abstract
Background:
The evidence supporting the effectiveness of combined interventions in Alzheimer’s disease (AD) patients remains inconclusive.
Objective:
The aim of this study was to evaluate the mid- and long-term effectiveness of physical training, alone or combined with cognitive games, on cognitive performance in patients with moderate AD.
Methods:
Seventy-nine AD patients (≈73% females, age of ≈70±1 years) were randomly divided into three groups: aerobic-based training (AT-group, n = 27), aerobic-based training plus cognitive games (ACT-group, n = 25), and a control group engaged in reading (CG, n = 26), two sessions per week. Cognitive performance was evaluated at the start, 4th week (W4), end of the 8th week (W8), and after a 4-week detraining period (W12), using problem-solving (Tower-of-Hanoi), selective attention (Stroop-test), and working memory (Digit-Span-test) assessments. Stress levels and quality of life were also evaluated.
Results:
Aerobic and combined training induced a positive effect on all cognitive functions tested at W4 (except problem-solving) and W8 (all p < 0.001) with greater improvements in working-memory and problem-solving in ACT-group (p < 0.05). Depression levels also decreased significantly, and quality of life improved at W8 (p < 0.001) in both groups. After 4 weeks of detraining, the beneficial effect of AT and ACT was still observed. The CG did not show any significant improvements at all time points.
Conclusions:
Physical and cognitive interventions appear effective for improving cognitive-functions, quality-of-life, and reducing depression in AD patients. Combined training emerges as a more effective strategy to mitigate AD progression. Further research is necessary to validate these results and explore their potential for preventing early cognitive decline.
INTRODUCTION
Alzheimer’s disease (AD) is a chronic, progressive neurodegenerative disorder for which there is currently no known medical treatment [1]. As the disease progressively destroys brain structures, such as the hippocampus and internal olfactory cortex [2], it leads to loss of mental cognitive functions. Consequently, memory, attention, perception, and language are lost, leading to declines in activities of daily living, and diminution in quality of life [3].
The primary risk factor for AD is carefully related to the aging of population [4], and given increases in human life expectancy, the proportion of people with AD will increase dramatically independent of the development of efficient interventions [5]. According to the 2021 World Alzheimer’s Disease Report, the number of people diagnosed with cognitive deficit is currently 55 million people worldwide and expected to reach 78 million in 2030 [6]. The direct cost of dementia is estimated to double in 2030, to 2 trillion US dollars annually [7], with up to 85% of expenses being related to family and social care [8].
To reduce the cost of caring for patients with dementia, several studies have shown physical exercise is one of the least expensive, most promising, and effective approaches to potentially reducing cognitive deficits in patients with and at at-risk for AD [9, 10]. It should be acknowledged that the latest estimates suggest 9.6% of dementia cases are related to sedentary elderly aged over 65 years. Yet, after adjusting for the clustering of various risk factors, the proportion of cases directly attributable to physical inactivity is estimated at 1.6% [11].
Epidemiological studies suggest physical training can act as a non-pharmacological intervention to protect against AD [12, 13]. Regular aerobic training relates to beneficial effects on brain health by boosting brain plasticity [14], increasing brain neurogenesis [15], reducing hippocampal atrophy [16], and even increasing the hippocampal structure [17]. As a consequence, functional improvements, such as improved independence and cognitive functions of AD patients were related to these structural changes [13]. Nevertheless, studies on the effects of physical exercise in individuals with cognitive decline are less abundant and differ in efficacy [18–20]. The large variability in physical exercise protocols, study populations, and treatment further complicates the interpretation of the results [18–20].
Cognitive intervention was also shown as a non-pharmacological intervention to enhance cognitive performance for patients with AD. The results of a recent review by Bleibel et al. [21] demonstrated an enhancement of cognitive performance (including verbal fluency, language, processing global cognition, speed of information, attention, and memory) in adults engaged in music therapy, compared to control groups. Those authors highlighted the potential effectiveness of music therapy as a complementary treatment option for patients with AD and the importance of further research in this field. Recently, Chéour et al. [22] demonstrated that combined music therapy and physical rehabilitation had a greater effect on cognitive function [Alzheimer’s Disease Assessment Scale–Cognitive Subscale Total, the ADAS-Cog Memory subscale, and Mini-Mental State Examination (MMSE)].
A previous study indicates that combined physical exercise with cognitive games may enhance cognitive performance in individuals with preclinical and clinical dementia [23]. However, it remains uncertain whether combining these two approaches yields synergistic benefits or which specific intervention designs would maximize this positive impact [24–26]. Typically, these interventions employ sequential training, where physical exercises and cognitive training are performed separately. However, dual-task or simultaneous training, which involves conducting motor and/or cognitive training concurrently with physical exercise, may provide enhanced benefits [24]. The results from a systematic review and network meta-analysis by Bruderer-Hofstetter et al. [25] showed the efficacy, of separately and simultaneously performed multicomponent training in enhancing a number of cognitive functions in adults with normal cognition. However, inconsistent findings were drawn in favor of either separate or simultaneous delivery of MCTs. Tait et al. [26] provided an overview of the effects of combined sequential or simultaneous training on cognition in older adults. The authors concluded that, according to the available evidence, there are no clear conclusions regarding the effectiveness of sequential training compared to physical or cognitive training alone on cognitive performance [26]. Conversely, simultaneous training interventions, especially those that incorporate multimodal exercise programs with secondary tasks guided by sensory cues, have shown significant cognitive improvements in both healthy older adults and clinical populations [26]. However, more research is needed to identify the optimal features of such combined training programs which effectively enhance cognitive function in the elderly.
Overall, combined physical and cognitive intervention may be a beneficial strategy to prevent impairment in different cognitive domains. Limited research exists on the impact of combined training on the cognitive performance of AD patients. While some studies have found combined interventions positively affect cognitive function in older adults with mild cognitive impairment (MCI) or dementia [27, 28], others report no beneficial effects [25, 29]. Although previous research has established physical and cognitive interventions can enhance cognition in AD patients, the dose-effect relationship between physical activity and AD remains unclear.
Therefore, the purposes of the present study were (1) to determine the mid-term effect of 8-week physical training, alone or combined with cognitive games, on cognitive functions in patients with moderate AD, and (2) to explore the long-lasting effects of this bi-domain intervention following 4 weeks of detraining.
MATERIALS AND METHODS
Design of the study
A randomized controlled trial of 8 weeks of training intervention was carried out at the Laboratory of Exercise Physiology and Physiopathology (LR19ES09) in the Faculty of Medicine of Sousse, Tunisia. Cognitive assessments were conducted at baseline (W1), after 4 weeks (W4), at the end of the training (W8), and after a 1-month follow-up (4 weeks of detraining, W12). The cognitive evaluation was counterbalanced and randomized for all patients with AD. These evaluations were performed under the supervision of a trained PhD student. For the cognitive assessment of patients with AD in this study, a comprehensive neuropsychological evaluation was administered by a qualified neuropsychologist. Neuropsychological assessments were administered to all patients with moderate AD at baseline and at the end of the training (see Table 1).
Table 1
Experimental design
W0: Clinical | W1: Baseline | W2 | W3 | W4 : 2nd | W5 | W6 | W7 | W8 : 3thd | 4 | W12 : 4th | |
Assessment | cognitive | cognitive | evaluation | weeks | cognitive | ||||||
(CA) + 6MWT | evaluation (CE) | evaluation | CE + CA | of rest | evaluation | ||||||
Sessions / Week | 2 | 2 | 2 | 2 | 2 | 2 | 2 | 2 | |||
Group ‘AT’ | N = 27 | N = 27 | N = 27 | N = 27 | |||||||
Group ‘ACT’ | N = 25 | N = 25 | N = 25 | N = 25 | |||||||
Group ‘CG’ | N = 26 | N = 26 | N = 22 | N = 20 |
W, week, 6MWT; 6 Minutes’ Walk Test; AT, aerobic training group; ACT, simultaneous combined training group; CG, control group.
Participants
The preliminary sample size determination was guided by Beck’s [30] methodology, utilizing G *Power software (version 3.1.9.2, Faul et al. [31], from Kiel University, Germany). The analysis framework chosen was an F test (ANOVA: repeated measures, within-between interaction) involving three different groups over four-time points. We set the Type I error probability (α) at 0.05 and aimed for a power (1-β) of 0.95, with an assumed correlation of 0.5 among repeated measures. Drawing from Ben Ayed et al. [32] and collaborative discussions, we anticipated a medium effect size, designated as partial eta-squared of 0.06 (equivalent to an f value of 0.25), necessitating a minimum of 45 participants. A total of 79 patients with moderate AD voluntarily participated in the study. Four patients in the control group dropped the intervention after 8-week period and two other patients in CG dropped after 4 weeks of detraining. All patients were clinically diagnosed with moderate AD at the Department of Neurology, Sahloul Hospital, Sousse, Tunisia according to the International Working Group-2 (IWG-2) diagnostic criteria [33].
The study’s eligibility criteria included individuals aged 65 to 75 years, living independently, outside of institutional settings, scoring between 10 and 19 on the MMSE [34], possessing normal or corrected-to-normal vision and color perception, and capable of daily movement without technical support.
Exclusion criteria were as follows: inability to provide written informed consent; cognitive or functional impairments hindering study participation; cardiovascular diseases; acute lower back or extremity pain; peripheral neuropathy; orthopedic and rheumatic conditions; and severe psychiatric disorders (depression score < 5). Additionally, potential participants were required to undergo a risk factor assessment, including cardiovascular, and other comorbidities, prior to enrollment.
The study’s protocol received approval from the Research Ethics Committee of the Faculty of Medicine, Sousse, Tunisia (IRB 00008931).
Seventy-nine patients with moderate AD provided informed consent. Patients with AD were randomly assigned to 3 groups: a simultaneous combined training group (ACT group, n = 25), an aerobic training group (AT group, n = 27), or simply reading for control group (CG group, n = 26).
Training protocol
All patients were randomly assigned to 8 weeks, twice-weekly of either AT group, ACT group, or a reading control group (total of intervention group was 16 sessions).
The Aerobic Training consisted of an aerobic physical activity program. Before the physical exercise, the seat on the ergocycle (Labyrinth, Paris, France) was regulated to the patient height. After a 5-min warm-up, patients with AD performed 20 min of exercise at a moderate intensity, i.e., 60% of the maximal heart rate (MHR) measured at the end of the 6-Minute Walk Test (6MWT) [35, 36], followed by a 5–10-min cooldown. One week preceding the experimentation period, MHRs were measured during a familiarization session at the end of the 6MWT. During the physical exercise sessions, patients were monitored with heart rate monitors (Polar V800, USA), and they were requested to report their rates of perceived exertion (RPE) at the conclusion of each aerobic training session.
In line with the findings of a meta-analysis by Zhu et al. [24], the aerobic-cognitive training conducted was a form of dual task training. Patients with AD in the combined group performed the same training regimen as those in the AT group, with the additional incorporation of three simple cognitive tasks displayed on a computer screen. These tasks, including visual scanning, working memory, information processing speed, selective attention, arithmetic, and perception exercises, were designed as straightforward and engaging cognitive activities engaging various cognitive processes within the same session. A team of medical experts, particularly neuropsychologists, developed these games to target cognitive functions in patients with AD, with each task lasting approximately 5 min. To avoid repeated boot effects, three different tasks were introduced at each session from those used previously. The scores for these cognitive games were not recorded, as they were intended to be a supplementary component of the AT.
Patients with moderate AD in the control group were asked to read a religious book for 20 min instead of engaging in physical training. All participants were advised to refrain from engaging in strenuous physical activities or any particularly fatiguing efforts prior to each session. Furthermore, to guarantee uniformity in testing conditions and minimize the influence of circadian rhythms on outcomes [37, 38], all sessions were conducted at the same time of day during the morning hours.
Measures
Neuropsychological assessments were used for subjective evaluations of daily activity performance, including the MMSE test [34, 39], the 16-item free and cued recall test (RL/RI-16) [40], the clock test [41], and the fluency test [42]. Additionally, the 15-item Geriatric Depression Scale (GDS) [43] was used for depression screening, and the World Health Organization Quality of Life-BREF (WHOQOL-BREF-100) [44] was utilized for quality of life; the full procedure is defined in detail in a previous publication by our team [32, 45].
Cognitive function (i.e., working memory, selective attention, and problem-solving) was assessed 5–10 min after finished interventions to permit heart rate to return to a resting value (≤10% above the individual baseline rate). To avoid an order effect, cognitive assessments were randomly counterbalanced for all AD patients. Following the instructions for the cognitive tasks, the experimenter sat alongside the patients, observing their activity without any visible reaction. Furthermore, these tests were maintained as investigational scenarios.
The French version of Victoria of Stroop color-word task was used to evaluate selective attention [46] and resistance to interference [47]. The Victoria Stroop Test (VST) presents a short version of the original Stroop test. It consists of three subtests, each presenting a stimulus card with six rows of four items. The first subtest (VST1) displays 24 dots in green, blue, red and yellow colors in a pseudorandom sequence. The second subtest (VST2) presents 24 common words, written in the four different colors. The third subtest (VST3) shows 24 color words (green, yellow, blue, and red) written in a color that does not match the word’s meaning (e.g., “green” is written in blue ink). Additionally, we incorporated an experimental condition requiring patients to read color names written in black ink. This condition was designed to investigate automatic reading behavior with a limited number of items and to create interference scenarios.
Working memory was explored using the forward and backward digits span test [48–50]. On the computer screen, digits were displayed at a pace of one digit per second. Subjects participated in two experimental conditions: in the forward condition, they were required to recall and list the digits in the same sequence as presented, while in the backward condition, they had to list the digits in reverse order. Performance in both conditions was assessed based on the number of correctly recalled items [49, 50].
The problem-solving function was assessed using the Tower of Hanoi test [51]. In this test, patients were required to rebuild a pyramid with three disks, using the middle disk for necessary moves, to shift it from the right-hand peg to the left-hand peg. The rules stipulated patients could only move one disk at a time and were not allowed to place a larger disk on top of a smaller one. Time taken and the number of moves made were recorded. Seven disk displacements were required, at minimum, to resolve this test.
The complete description of cognitive tests is provided in thorough detail in our prior publication [32, 45].
Statistical analysis
All Analyses were conducted using SPSS software (IBM® SPSS® Statistics version 26.0). Descriptive statistics were summarized as mean (M) and standard deviation (SD) for the MMSE, Stroop, tower of Hanoi, and digit span tests. Furthermore, we calculated baseline and post-intervention values (at W4, W8, and W12) to assess the magnitude of significant differences. The percentage of performance gain was calculated as follows: [(post value –pre value)/ (pre value)]×100. The Shapiro–Wilks W-test was used to check the normality of the distribution.
The main effect of groups was explored using the Kruskal–Wallis non-parametric test. When appropriate, post hoc pairwise comparisons were performed, and the results were interpreted using a Bonferroni correction. The Friedman non-parametric test was employed to explore the time assessment (baseline, W4 and W8) of each group. The paired Wilcoxon test was used to interpret within-group results of neuropsychological measures (Baseline and W8) and the persistent effect (W8 and W12). Statistical significance for all analyses was accepted at the level of p < 0.05.
RESULTS
Participant characteristics
Patients with moderate AD were randomly assigned to one of three groups. Across all participants, the mean age was 70.14±4.61 years, body mass averaged 75.38±8.57 kg, body mass index (BMI) was 28.60±3.84, and the distribution between female and male participants was 65.4% and 34.6%, respectively. No significant differences were found between the groups regarding age, body mass, and BMI (p > 0.05), indicating the sample was homogeneous with respect to these parameters.
As shown in Table 2 and taking into account the standards for different neuropsychological scales as well as in accordance with the literature, the three groups of participants showed similar cognitive disorders. Regarding their emotional profile, AT and ACT participants had mild depressive symptoms, which were more marked compared to the CG. The results obtained for quality of life indicated that the majority of participants were not satisfied in the physical, psychological, and environmental domains but, on the opposite, rather satisfied in the field of social relations. However, a greater overall satisfaction was observed in the AT (p < 0.05).
Table 2
Participants’ demographic and clinical characteristics (CG, AT. and ACT groups)
Variable | CG (n = 26) | AT (n = 27) | ACT (n = 25) | Kruskal–Wallis | p |
M (SD) | M (SD) | M (SD) | |||
Age (y) | 71.04 (0.90) | 70.04 (0.86) | 69.32 (0.90) | χ2(2) = 3.063 | 0.21 |
Education (y) | 9.07 (0.49) | 9.44 (0.35) | 9.48 (0.37) | χ2(2) = 0.58 | 0.74 |
Disease duration | 5.38 (0.15) | 5.15 (0.15) | 5.36 (0.15) | χ2(2) = 0.52 | 0.76 |
Sex | χ2(2) = 10.11 | 0.006 | |||
Male | 15 (19.23) | 8(10.26) | 5 (5.13) | ||
Female | 11 (14.10) | 19 (24.36) | 21 (26.92) | ||
Marital status | χ2(2) = 6.41 | 0.04 | |||
Married | 25 (32.05) | 20 (25.64) | 23 (29.49) | ||
Widow | 1 (1.28) | 7 (8.97) | 2 (2.56) | ||
Neuropsychological measures | |||||
MMSE | 18.11 (0.07) | 18.03 (0.07) | 18.12 (0.08) | χ2(2) = 0.72 | 0.69 |
RL/RI 16 | 8.88 (0.16) | 8.81 (0.16) | 8.64 (0.17) | χ2(2) = 0.90 | 0.63 |
Letter fluency | 5.63 (0.17) | 5.52 (0.16) | 5.52 (0.17) | χ2(2) = 0.41 | 0.81 |
Category fluency | 7.00 (0.15) | 7.04 (0.15) | 7.24 (0.15) | χ2(2) = 1.23 | 0.53 |
Clock test | 1.50 (0.15) | 1.04 (0.14) | 1.20 (0.15) | χ2(2) = 5.03 | 0.08 |
Psychological measures | |||||
GDS 15 | 9.73 (0.22) | 8.85 (0.22) | 8.20 (0.22) | χ2(2) = 19.27 | 0.001 |
WHOQOL-BREF-100D1 | 13.23 (0.25) | 13.00 (0.24) | 13.16 (0.25) | χ2(2) = 0.41 | 0.81 |
WHOQOL-BREF-100D2 | 13.31 (0.23) | 13.26 (0.22) | 13.04 (0.23) | χ2(2) = 0.32 | 0.85 |
WHOQOL-BREF-100D3 | 13.38 (0.19) | 14.30 (0.19) | 13.72 (0.19) | χ2(2) = 10.58 | 0.005 |
WHOQOL-BREF-100D4 | 13.84 (0.25) | 13.85 (0.25) | 14.08 (0.26) | χ2(2) = 0.60 | 0.73 |
Physiological measures | |||||
6MWT (m) | 272.19 (44.27) | 316.88 (52.66) | 306.22 (45.05) | χ2(2) = 11.37 | 0.003 |
MHR (bpm) | 102.96 (7.18) | 106.96 (12.60) | 105.87 (12.60) | χ2(2) = 4.80 | 0.09 |
M, mean; SD, standard deviation; 1N (percentage per disease); MMSE, Mini-Mental Status Examination; GDS, Geriatric Depression Scale; WHOQOL-BREF, World Health Organization Quality of Life-BREF; D1, Physical health; D2, Psychiatry; D3, Social relationships; D4, Environment; 6MWT, the 6-Minute Walk Test; MHR, Maximum Heart Rate.
Neuropsychological results
After the 8 weeks of aerobic and combined training, a significant improvement of all neuropsychological assessments was observed (all p < 0.05; detailed results in Table 3). The patients in the control group showed no significant difference from pre- to post-reading session (detailed results in Table 3).
Table 3
Neuropsychological assessments of the control, aerobic and combined groups before and after the intervention period
Groups | CG (n = 22) | AT (n = 27) | ACT (n = 25) | |||||||||
Baseline | W8 | Wilcoxon | Baseline | W8 | Wilcoxon | Baseline | W8 | Wilcoxon | ||||
M (SD) | M (SD) | Z | p | M (SD) | M (SD) | Z | p | M (SD) | M (SD) | Z | p | |
MMSE | 18.11 (0.07) | 18.08 (0.41) | 0.00 | 1.00 | 18.03 (0.07) | 18.00 (0.50) | –2.88 | 0.00 | 18.12 (0.08) | 19.00 (0.57) | –3.90 | 0.00 |
RL/RI-16 | 8.88 (0.16) | 8.79 (0.86) | –1.41 | 0.15 | 8.81(0.16) | 9.00 (0.63) | –3.25 | 0.00 | 8.64 (0.17) | 10.00 (0.64) | –3.80 | 0.00 |
Letter fluency | 5.63 (0.17) | 5.43 (0.84) | –1.00 | 0.31 | 5.52 (0.16) | 6.00 (0.89) | –3.22 | 0.00 | 5.52 (0.17) | 7.00 (0.84) | –3.90 | 0.00 |
Category fluency | 7.00 (0.15) | 6.34 (0.71) | –2.50 | 0.01 | 7.04 (0.15) | 8.00 (0.69) | –3.33 | 0.00 | 7.24 (0.15) | 8.00 (0.53) | –3.14 | 0.00 |
Clock test | 1.50 (0.15) | 1.39 (0.58) | –0.44 | 0.65 | 1.04 (0.14) | 2.00 (0.55) | –3.90 | 0.00 | 1.20 (0.15) | 2.00 (0.55) | –3.55 | 0.00 |
GDS 15 items | 9.73 (0.22) | 8.84 (0.67) | –2.73 | 0.00 | 8.85 (0.22) | 6.00 (0.99) | –4.57 | 0.00 | 8.20 (0.22) | 5.00 (0.66) | –4.41 | 0.00 |
WHOQOL-BREF-100 D1 | 13.23 (0.25) | 12.65 (1.27) | –2.71 | 0.00 | 13.00 (0.24) | 14.00 (1.13) | –2.97 | 0.00 | 13.16 (0.25) | 14.00 (1.05) | –3.87 | 0.00 |
WHOQOL-BREF-100 D2 | 13.31 (0.23) | 12.91 (1.27) | –1.89 | 0.05 | 13.26 (0.22) | 14.00 (1.20) | –3.28 | 0.00 | 13.04 (0.23) | 14.00 (1.08) | –3.45 | 0.00 |
WHOQOL-BREF-100 D3 | 13.38 (0.19) | 12.65 (1.07) | –2.80 | 0.00 | 14.30 (0.19) | 15.00 (0.96) | –2.12 | 0.03 | 13.72 (0.19) | 14.00 (0.77) | –3.16 | 0.00 |
WHOQOL-BREF-100 D4 | 13.84 (0.25) | 13.65 (1.03) | –1.05 | 0.29 | 13.85 (0.25) | 14.00 (1.13) | –2.43 | 0.01 | 14.08 (0.26) | 15.00 (0.70) | –3.20 | 0.00 |
MMSE, Mini-Mental Status Examination; RL/RI-16, 16-item Free and Cued Recall; GDS, Geriatric Depression Scale; WHOQOL-BREF, World Health Organization Quality of Life-BREF; D1, physical health; D2, psychiatry; D3, social relationships; D4, environment; *p < 0.01; ***p < 0.001.
In addition to the results presented in Table 3, changes in depression and quality of life score were evaluated immediately following the intervention and after a four-week detraining period. We found depression levels decreased significantly, and quality of life improved at week 8 (p < 0.001) in both experimental groups. The positive effects of aerobic training (AT) and aerobic-cognitive training (ACT) were maintained after four weeks of detraining, as evidenced by the absence of significant differences between W12 and W8 (p > 0.05).
Training-detraining effects on cognitive functions
As shown in Fig. 1, there were no significant differences between groups at baseline, regardless of the cognitive task (detailed results presented in Supplementary Table 1). After four weeks of training (W4), both the AT and ACT groups significantly improved the majority of cognitive performances (except Hanoi moves) compared to the CG group (all p < 0.001), with ACT achieving greater cognitive performance than AT (p = 0.000). The performance of the AT and ACT groups continued to improve at W8 compared to W4 (all p < 0.01; see detailed statistics in Supplementary Table 2), with ACT outperforming AT in all tested skills (all p < 0.001) except attention (p > 0.05). It is worth noting that the CG group’s performance remained unchanged throughout the 8-week intervention program.
Fig. 1
Cognitive performances in the different tests for control, aerobic and combined groups at baseline, after 4 weeks (W4), after 8 weeks (W8) of training, and after 4 weeks of detraining (W12). S: significant differences compared with CG *: significant difference between ACT and AT; a: significant compared to baseline (W4 versus baseline and W8 versus baseline); and b: significant differences between W4 and W8.
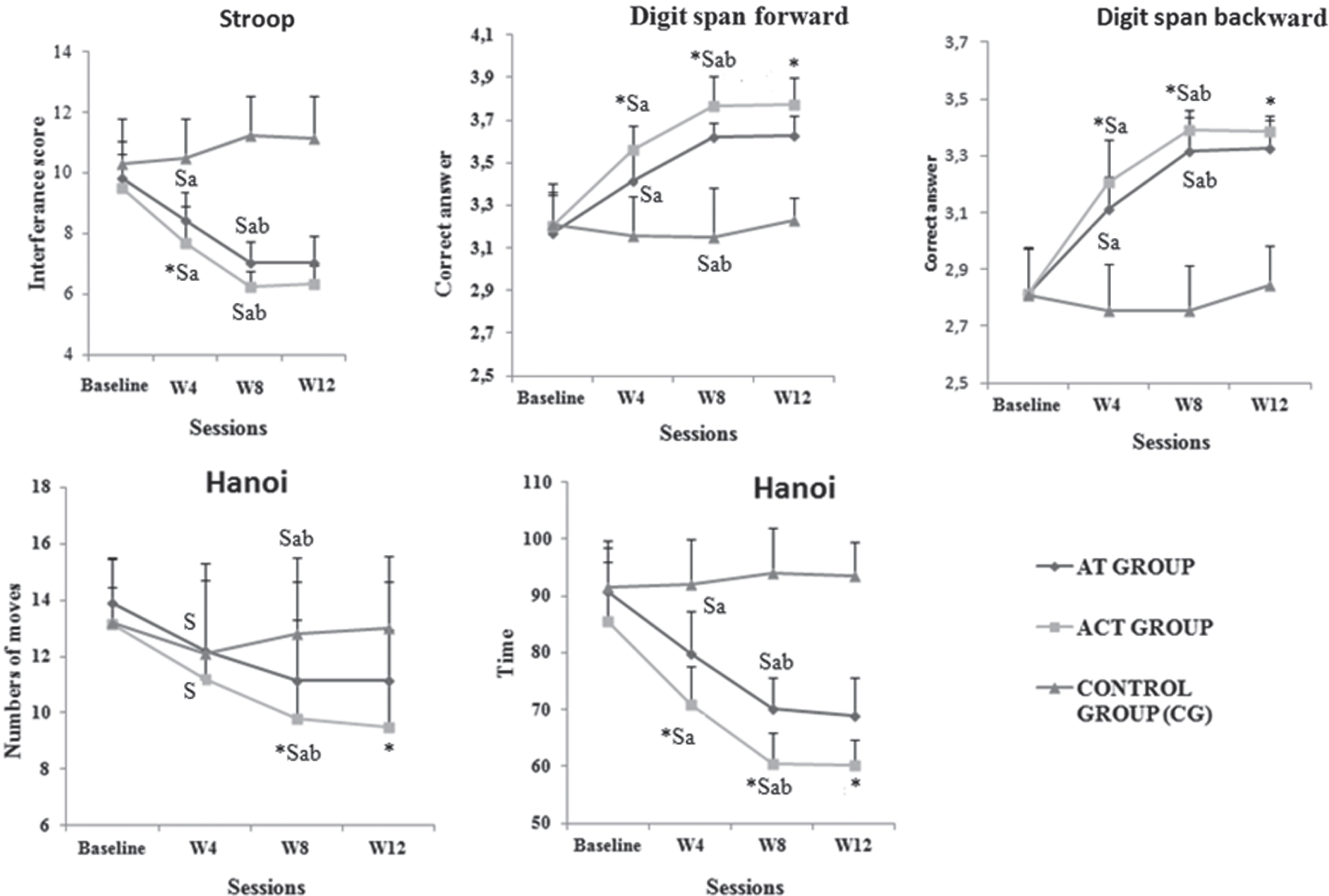
Following 4 weeks of detraining, a lasting effect was observed in the AT and ACT groups in all tested tasks (Fig. 2), as evidenced by the absence of a significant difference between W8 and W12 (detailed results presented in Supplementary Table 3). The improvements induced by training at W8 persisted at W12.
Fig. 2
Percent gain from baseline at W8 and W12 for the different tests among the aerobic and combined groups. *significant difference between experimental groups.
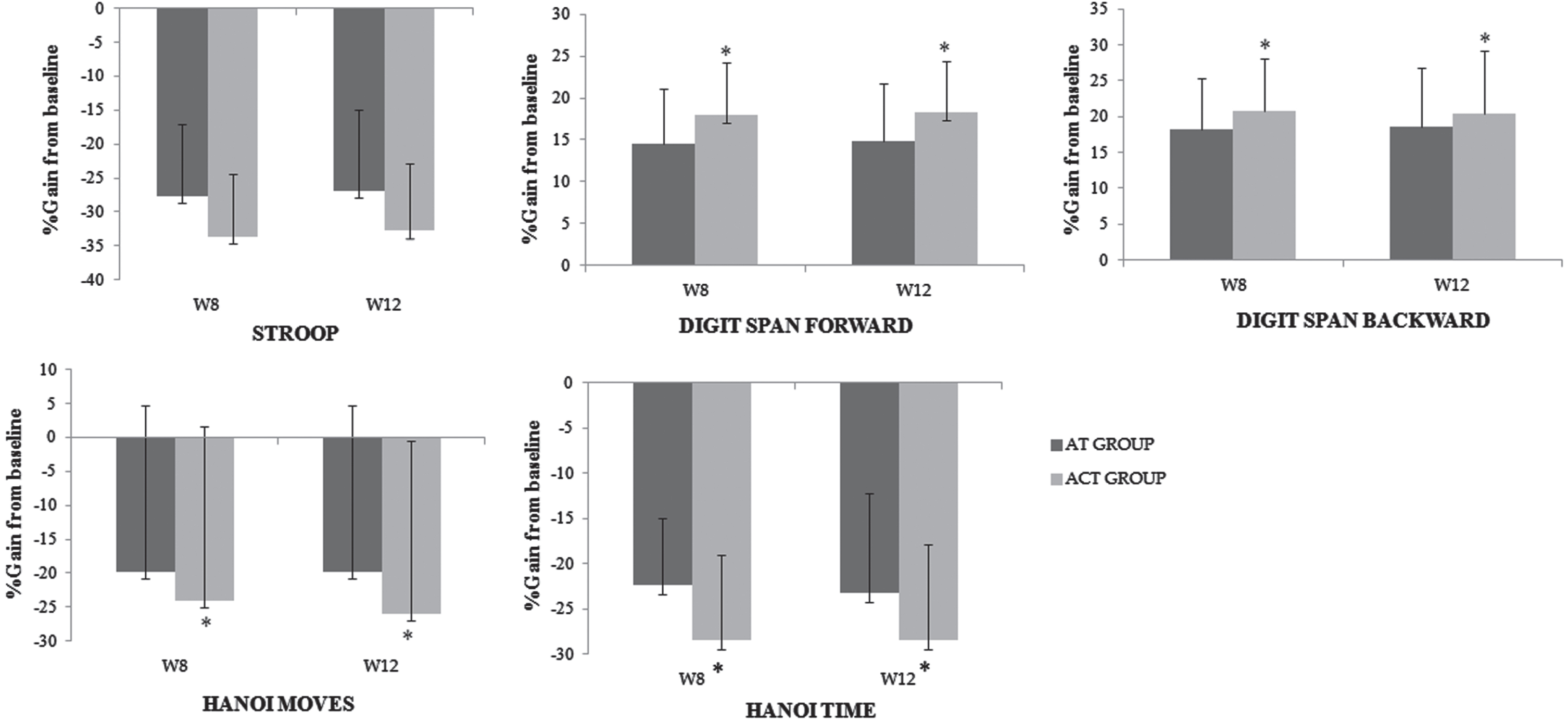
The improvements in cognitive function at W8 and W12, compared with the baseline values are presented in Fig. 2. For all tested functions after training and detaining periods, higher percentages of gain were recorded for the two experimental groups. For example, at both test sessions, our results showed significant improvements in Hanoi task performance with percent gains around 29% and 24% for ACT and AT, respectively (all p < 0.05). Additionally, significant enhancements were observed for the digit span forward condition at W12 (p = 0.04) with percentage of gain ≈19% versus ≈15% for ACT and AT, respectively.
DISCUSSION
The aim of this study was to assess the efficacy of an 8-week simultaneous combined training intervention on cognitive function, depression levels, and quality of life in individuals with moderate AD in comparison to aerobic training. In addition, the study aimed to investigate beneficial effects persisted after one month of detraining. The results revealed after 8 weeks of training, patients in the combined group exhibited significant improvements in cognitive functions, particularly working memory and problem-solving compared to both the AT and CG groups. Notably, these beneficial effects of cognitive performances observed in the experimental groups persisted even after the detraining period, indicating a long-term effect. The present findings revealed that, compared to CG, simultaneous combined training was more effective in improving cognitive function, with greater gains in executive function at mid-term (at 4 and 8 weeks of training), persisting after 1 month of detraining. Regarding neuropsychological measures, the present findings also revealed significant improvements in depression scores and quality of life of the AT and ACT groups, compared to CG.
After physical and combined training, we observed an improvement in working memory in AD patients. Our findings are comparable to those demonstrated by Fonte et al. [52] who noted following 45 min of aerobic physical training at a moderate intensity (70% of MHR in AD subjects, a significant beneficial effect on the speed of the response and a small to moderate effect on accuracy during a memory test were observed.
We also found physical and combined training significantly improved attention after 8 weeks in AD patients. This seems partly consistent with previous studies [53–55]. For example, Castellano et al. [53] demonstrated aerobic physical training of 40 min of walking for 3 months, at a moderate intensity (60% of MHR) improved attention by Stroop test in patients suffering from AD. Okamura et al. [54] showed also that combined training of cognitive stimulation and an aerobic pedaling exercise of 5 mins/session (1 session or more/week) improved attention and concentration in AD patients. Kalbe et al. [55] found seven weeks of physical training, either alone or in combination, enhanced cognitive functioning in healthy adults. Improvements were observed in short-term verbal memory and attention following combined training. Additionally, they identified low baseline cognitive performance, lower education levels, lower baseline blood levels of brain-derived neurotrophic factor, and higher baseline blood levels of insulin-like growth factor 1 could predict cognitive function improvement one year after undergoing a combined physical and cognitive intervention. For the execution time of the Hanoi task, our results indicated the CG seemed to have lower performance than the patients in the combined and physical training groups. These findings are in line with those of Zhu et al. [24] who suggested combined interventions improved cognitive performance in healthy older adults, but with varied magnitude of changes across cognitive functions. These authors showed cognitive and physical intervention produced a moderate effect on global cognition, with only small effects on attention, memory, and executive function [24]. To date, a limited number of studies [28, 56, 57] have evaluated the impact of AT on cognitive performance in patients with mild to moderate AD. Wayne et al. [56] revealed in a meta-analysis Tai Chi had a beneficial effect on executive function in older adults. These findings underscore the potential of exercise as a beneficial component in the treatment regimen for patients with AD. Yu et al. [55] also noted 45 min of aerobic physical training at a moderate intensity (65% to 75% of reserve heart rate) for 6 months maintained executive function in AD patients. Recently, Parvin et al. [28] revealed an improvement in cognitive function, particularly in attention, working memory, and executive function for patients with AD following the 12 weeks of combined training. These authors demonstrated also significant improvements in the depression status of the dual-task group, compared to control group [28].
Depression is among the most prevalent risk factors for the development of AD, amplifying the adverse effects associated with this condition [58]. Data from non-pharmacological interventions revealed consistent short-term exercise training had positive effects on the mean score of depression in patients with mild AD [58]. The results of this study further illustrate the positive impact of combined training in alleviating depression. Several mechanisms can elucidate the effectiveness of physical interventions in addressing depression. One such mechanism involves the reduction of serum cortisol levels, as regular exercise has been shown to be achieved in patients with AD [59]. High cortisol levels induced by AD can have neurotoxic consequences on the hippocampus, leading to neurodegeneration, cognitive decline, depression and oxidative stress. Additionally, exercise contributes to the production of neurotransmitters, such as serotonin and dopamine [60], as well as brain-derived neurotrophic factor [61], which play pivotal roles in depression treatment. The benefits of exercise training in reducing depression involve improvements in independence, daily life activities, and mood [58].
Measuring quality of life outcomes is a potentially important factor in ensuring a patient with cognitive deficits can ‘live well’ with pathology [62]. In our study, the results presented an increase in quality-of-life scores after 2 months of physical and combined training. In contrast, no significant difference was observed for patients in CG. Our findings agree with the recent research by Cintoli et al. [62], who suggested 7 months of combined training can decrease neuropsychiatric symptoms and improve quality of life. Vidoni et al. [63] also showed an improvement in instrumental activities of daily living after 26 weeks of aerobic training for individuals diagnosed with mild-stage AD.
In our study, after both aerobic and combined interventions, improvements were observed in cognitive function. While our results may be related to the improvement of the neural and vascular mechanisms, we have not verified those mechanisms in the present study and recommend it as an area of further investigation. Our findings are in agreement with the study by Morris et al. [64], supporting the positive effects of physical training on cognitive functions in patients with AD. While numerous studies [28, 65] support the protective effect of physical activity on the aging brain, the precise mechanisms behind this benefit remain less understood. Several mechanisms have been proposed by which physical activity decreases the chances of AD, including the increase of blood flow to the brain, improvement of metabolic health, reducing the risk of diabetes and improving health conditions [65]. Recently, Wilckens et al. [66] suggested engaging in moderate exercise for more than 6 months has a beneficial impact on hippocampal volume, even in older populations vulnerable to hippocampal atrophy. Furthermore, a prior study highlighted the capacity of aerobic training to enhance neuroplasticity, support brain health, and promote synaptic plasticity in individuals with AD [28, 67]. The authors attributed the observed enhancements in brain function to the amplified physical activity levels of the participants, as evidenced by a reduction in the theta/alpha ratio [28].
Notably, even after one month of detraining, the greater beneficial effect observed in executive functions after the 8-week of combined training persisted in our patients with AD. Regardless of the enrollment group, these results highlight the long-lasting effect, offering a promising pathway in the rehabilitation domain. Considering the patients’ emotional well-being, beneficial effects have been demonstrated on depression and quality of life scores after both aerobic and combined interventions.
Limitations and perspectives
While the results presented above are positive, there are some limitations to the present study. First, we experienced some attrition, particularly among participants in the CG. Nevertheless, all participants, except for four patients in the CG, successfully completed the eight training sessions. Second, we did not have access to quantitative electroencephalogram, therefore future studies are encouraged to utilize functional and structural brain imaging to quantitively measure adaptations in the brain structure and function. Third, while beneficial effects persisted after four weeks, we did not monitor the duration of these changes. This highlights the need for follow-up assessments at 3, 6, or even 12 months to better understand the longevity of the intervention’s impact. Finally, future studies, incorporating larger sample sizes and accounting for potential confounding factors including sleep patterns, physical activity behaviors, and sex differences [68], are crucial to comprehensively understanding the impact of combined interventions. This research is necessary to identify the most effective training strategies and evaluate the sustained impact of such regimens on the cognitive functions of patients with AD.
Conclusion
The observed beneficial effects of combined training on cognitive functions, coupled with improvements in quality of life and even a reduction in depressive symptoms, underscores the potential utility, safety, and enduring efficacy of combined training as a strategy to mitigate the impairments associated with AD. To solidify these findings and explore potential implications for early cognitive decline prevention, further large-scale studies with extended follow-up periods are recommended.
AUTHOR CONTRIBUTIONS
Ines Ben Ayed (Conceptualization; Data curation; Investigation; Methodology; Validation; Writing – original draft); Achraf Ammar (Formal analysis; Funding acquisition; Project administration; Validation; Visualization; Writing – review & editing); Chirine Aouichaoui (Formal analysis; Validation; Writing – review & editing); Salma Naija (Investigation; Methodology; Validation; Writing – review & editing); Sana Ben Amor (Investigation; Methodology; Validation; Writing – review & editing); Jordan M. Glenn (Validation; Writing – review & editing); Hamdi Chtourou (Validation; Visualization; Writing – review & editing); Haitham Jahrami (Validation; Visualization; Writing – review & editing); Khaled Trabelsi (Validation; Visualization; Writing – review & editing); Yassine Trabelsi (Conceptualization; Investigation; Methodology; Supervision; Validation; Writing – review & editing); Farid El Massioui (Conceptualization; Investigation; Methodology; Project administration; Supervision; Validation; Visualization; Writing – review & editing).
ACKNOWLEDGMENTS
The authors have no acknowledgments to report.
FUNDING
The authors have no funding to report.
CONFLICT OF INTEREST
The authors have no conflict of interest to report.
DATA AVAILABILITY
The data are available from the first author upon reasonable request.
SUPPLEMENTARY MATERIAL
[1] The supplementary material is available in the electronic version of this article: https://dx.doi.org/10.3233/ADR-249003.
REFERENCES
[1] | Jia LF , Du YF , Chu L , Zhang Z , Li F , Lyu D , Li Y , Li Y , Zhu M , Jiao H , Song Y , Shi Y , Zhang H , Gong M , Wei C , Tang Y , Fang B , Guo D , Wang F , Zhou A , Chu C , Zuo X , Yu Y , Yuan Q , Wang W , Li F , Shi S , Yang H , Zhou C , Liao Z , Lv Y , Li Y , Kan M , Zhao H , Wang S , Yang S , Li H , Liu Z , Wang Q , Qin W , Jia J , COAST Group ((2020) ) Prevalence, risk factors, and management of dementia and mild cognitive impairment in adults aged 60 years or older in China: A cross-sectional study. Lancet Public Health 12: , E661–E71. |
[2] | Lane CA , Hardy J , Schott JM ((2018) ) Alzheimer’s disease. Eur J Neurol 1: , 59–70. |
[3] | McGough EL , Lin SY , Belza B , Becofsky KM , Jones DL , Liu M , Wilcox S , Logsdon RG ((2019) ) A Scoping review of physical performance outcome measures used in exercise interventions for older adults with Alzheimer disease and related dementias. J Geriatr Phys Ther 1: , 28–47. |
[4] | Serý O , Povová J , Balcar VJ ((2014) ) Perspectives in genetic prediction of Alzheimer’s disease. Neuro Endocrinol Lett 35: , 359–366. |
[5] | Winblad B , Amouyel P , Andrieu S , Ballard C , Brayne C , Brodaty H , Cedazo-Minguez A , Dubois B , Edvardsson D , Feldman H , Fratiglioni L , Frisoni GB , Gauthier S , Georges J , Graff C , Iqbal K , Jessen F , Johansson G , Jönsson L , Kivipelto M , Knapp M , Mangialasche F , Melis R , Nordberg A , Rikkert MO , Qiu C , Sakmar TP , Scheltens P , Schneider LS , Sperling R , Tjernberg LO , Waldemar G , Wimo A , Zetterberg H ((2016) ) Defeating Alzheimer’s disease and other dementias: A priority for European science and society. Lancet Neurol 15: , 455–532. |
[6] | Gauthier S , Rosa-Neto P , Morais JA , Webster C ((2021) ) World Alzheimer Report 2021. Journey through the diagnosis of dementia. Alzheimer’s Disease International, London. |
[7] | Patterson C ((2018) ) World Alzheimer Report 2018. The state of the art of dementia research: New frontiers. Alzheimer’s Disease International, London. |
[8] | Livingston G , Sommerlad A , Orgeta V , Costafreda SG , Huntley J , Ames D , Ballard C , Banerjee S , Burns A , Cohen-Mansfield J , Cooper C , Fox N , Gitlin LN , Howard R , Kales HC , Larson EB , Ritchie K , Rockwood K , Sampson EL , Samus Q , Schneider LS , Selbæk G , Teri L , Mukadam N ((2017) ) Dementia prevention, intervention, and care. Lancet 390: , 2673–2734. |
[9] | Gaitán JM , Moon HY , Stremlau M , Dubal DB , Cook DB , Okonkwo OC , van Praag H ((2021) ) Effects of Aerobic exercise training on systemic biomarkers and cognition in late middle-aged adults at risk for Alzheimer’s disease. Front Endocrinol (Lausanne) 12: , 660181. |
[10] | Rosenberg A , Ngandu T , Rusanen M , Antikainen R , Bäckman L , Havulinna S , Hänninen T , Laatikainen T , Lehtisalo J , Levälahti E , Lindström J , Paajanen T , Peltonen M , Soininen H , Stigsdotter-Neely A , Strandberg T , Tuomilehto J , Solomon A , Kivipelto M ((2018) ) Multidomain lifestyle intervention benefits a large elderly population at risk for cognitive decline and dementia regardless of base line characteristics. The FINGER trial. Alzheimers Dement 3: , 263–270. |
[11] | Livingston G , Huntley J , Sommerlad A , Ames D , Ballard C , Barnerjee S , Brayne C , Burns A , Cohen-Mansfield J , Cooper C , Costafreda SG , Dias A , Fox N , Gitlin LN , Howard R , Kales HC , Kivimäki M , Larson EB , Ogunniyi A , Orgeta V , Ritchie K , Rockwood K , Sampson EL , Samus Q , Schneider LS , Selbæk G , Teri L , Mukadam N ((2020) ) Dementia prevention, intervention, and care: 2020 report of the Lancet Commission. Lancet 396: , 413–446. |
[12] | De la Rosa A , Olaso-Gonzalez G , Arc-Chagnaud C , Millan F , Salvador-Pascual A , García-Lucerga C , Blasco-Lafarga C , Garcia-Dominguez E , Carretero A , Correas AG , Viña J , Gomez-Cabrera MC ((2020) ) Physical exercise in the prevention and treatment of Alzheimer’s disease. J Sport Health Sci 9: , 394–404. |
[13] | Jia RX , Liang JH , Xu Y , Wang YQ ((2019) ) Effects of physical activity and exercise on the cognitive function of patients with Alzheimer disease: A meta-analysis. BMC Geriatr 19: , 181. |
[14] | Zhang K , Jan YK , Liu Y , Zhao T , Zhang L , Liu R , Liu J , Cao C ((2022) ) Exercise intensity and brain plasticity: What’s the difference of brain structural and functional plasticity characteristics between elite aerobic and anaerobic athletes? Front Hum Neurosci 16: , 757522. |
[15] | Liu PZ , Nusslock R ((2018) ) Exercise-mediated neurogenesis in the hippocampus via BDNF. Front Neurosci 12: , 52. |
[16] | Tarumi T , Rossetti H , Thomas BP , Harris T , Tseng BY , Turner M , Wang C , German Z , Martin-Cook Z , Stowe AM , Womack KB , Mathews D , Kerwin DK , Hynan L , Diaz-Arrastia R , Lu H , Cullum CM , Zhang R ((2019) ) Exercise training in amnestic mild cognitive impairment: A one-year randomized controlled trial. J Alzheimers Dis 2: , 421–33. |
[17] | Hendrikse J , Chye Y , Thompson S , Rogasch NC , Suo C , Coxon JP , Yücel M ((2022) ) Regular aerobic exercise is positively associated with hippocampal structure and function in young and middle-aged adults. Hippocampus 3: , 137–52. |
[18] | Forbes D , Forbes SC , Blake CM , Thiessen EJ , Forbes S ((2015) ) Exercise programs for people with dementia. Cochrane Database Syst Rev 2015: , CD006489. |
[19] | Groot C , Hooghiemstra AM , Raijmakers PGHM , van Berckel BNM , Scheltens P , Scherder EJA , van der Flier VM , Ossenkoppele R ((2016) ) The effect of physical activity on cognitive function in patients with dementia: A meta-analysis of randomized control trials. Ageing Res Rev 25: , 13–23. |
[20] | Huuha AM , Norevik CS , Moreira JBN , Kobro-Flatmoen A , Scrimgeour N , Kivipelto M , Van Praag H , Ziaei M , Sando SB , Wisløff U , Tari AR ((2022) ) Can exercise training teach us how to treat Alzheimer’s disease? Ageing Res Rev 75: , 101559. |
[21] | Bleibel M , El Cheikh A , Said Sadier N , Abou-Abbas L ((2023) ) The effect of music therapy on cognitive functions in patients with Alzheimer’s disease: A systematic review of randomized controlled trials. Alzheimers Res Ther 15: , 65. |
[22] | Chéour S , Chéour C , Gendreau T , Bouazizi M , Singh KP , Saeidi A , Tao D , Supriya R , Bragazzi NL , Baker JS , Chéour F ((2023) ) Remediation of cognitive and motor functions in Tunisian elderly patients with mild Alzheimer’s disease: Implications of music therapy and/or physical rehabilitation. Front Aging Neurosci 15: , 1216052. |
[23] | Law LLF , Barnett F , Yau MK , Gray MA ((2014) ) Effects of combined cognitive and exercise interventions on cognition in older adults with and without cognitive impairment: A systematic review. Ageing Res Rev 15: , 61–75. |
[24] | Zhu X , Yin S , Lang M , He R , Li J ((2016) ) The more the better? a meta-analysis on effects of combined cognitive and physical intervention on cognition in healthy older adults. Ageing Res Rev 31: , 67–79. |
[25] | Bruderer-Hofstetter M , Rausch-Osthoff AK , Meichtry A , Münzer T , Niedermann K ((2018) ) Effective multicomponent interventions in comparison to active control and no interventions on physical capacity, cognitive function and instrumental activities of daily living in elderly people with and without mild impaired cognition –A systematic review and network meta-analysis. Ageing Res Rev 45: , 1–14. |
[26] | Tait JL , Duckham RL , Milte CM , Main LC , Daly RM ((2017) ) Influence of sequential vs. simultaneous dual-task exercise training on cognitive function in older adults. Front Aging Neurosci 9: , 368. |
[27] | Karssemeijer EGA , Aaronson JA , Bossers WJ , Smits T , Olde Rikkert MGM , Kessels RPC ((2017) ) Positive effects of combined cognitive and physical exercise training on cognitive function in older adults with mild cognitive impairment or dementia: A meta-analysis. Ageing Res Rev 40: , 75–83. |
[28] | Parvin E , Mohammadian F , Amani-Shalamzari S , Bayati M , Tazesh B ((2020) ) Dual-task training affect cognitive and physical performances and brain oscillation ratio of patients with Alzheimer’s disease: A randomized controlled trial. Front Aging Neurosci 12: , 605317. |
[29] | Yang C , Moore A , Mpofu E , Dorstyn D , Li Q , Yin C ((2020) ) Effectiveness of combined cognitive and physical interventions to enhance functioning in older adults with mild cognitive impairment: A systematic review of randomized controlled trials. Gerontologist 60: , 633–642. |
[30] | Beck TW ((2013) ) The importance of a priori sample size estimation in strength and conditioning research. J Strength Cond Res 27: , 2323–2337. |
[31] | Faul F , Erdfelder E , Lang AG , Buchner A ((2007) ) G *Power 3: A flexible statistical power analysis program for the social, behavioral, and biomedical sciences. Behav Res Methods 39: , 175–191. |
[32] | Ben Ayed I , Castor-Guyonvarch N , Amimour S , Naija S , Aouichaoui C , Ben Omor S , Tabka Z , El Massioui F ((2021) ) Acute exercise and cognitive function in Alzheimer’s disease. J Alzheimers Dis 82: , 749–760. |
[33] | Dubois B , Feldman HH , Jacova C , Hampel H , Molinuevo JL , Blennow K , DeKosky ST , Gauthier S , Selkoe D , Bateman R , Cappa S , Crutch S , Engelborghs S , Frisoni GB , Fox NC , Galasko D , Habert M , Jicha GA , Nordberg A , Pasquier F , Rabinovici G , Robert P , Rowe C , Salloway S , Sarazin M , Epelbaum S , de Souza LC , Vellas B , Visser PJ , Schneider L , Stern Y , Scheltens P , Cummings JL ((2014) ) Advancing research diagnostic criteria for Alzheimer’s disease: The IWG-2 criteria. Lancet Neurol 13: , 614–629. |
[34] | Folstein MF , Folstein SE , McHugh PR ((1975) ) “Mini-mental state”. A practical method for grading the cognitive state of patients for the clinician. J Psychiatr Res 12: , 189–198. |
[35] | Guyatt GH , Sullivan MJ , Thompson PJ , Fallen EL , Pugsley SO , Taylor DW , Berman LB ((1985) ) The 6-minute walk: A new measure of exercise capacity in patients with chronic heart failure. Can Med Assoc J 132: , 919–992. |
[36] | Brooks D , Solway S , Gibbons WJ ((2003) ) ATS statement on six-minute walk test. Am J Respir Crit Care Med 167: , 1287. |
[37] | Ammar A , Chtourou H , Souissi N ((2017) ) Effect of time-of-day on biochemical markers in response to physical exercise. J Strength Cond Res 31: , 272–282. |
[38] | Munnilari M , Bommasamudram T , Easow J , Tod D , Varamenti E , Edwards BJ , Ravindrakumar A , Gallagher C , Pullinger SA ((2023) ) Diurnal variation in variables related to cognitive performance: A systematic review. Sleep Breath 28: , 495–510. |
[39] | American Psychiatric Association (2013) Diagnostic and Statistical Manual of Mental Disorders: DSM-5. American Psychiatric Association, Washington, DC, USA. |
[40] | Dion M , Potvin O , Belleville S , Ferland G , Renaud M , Bherer L , Joubert S , Vallet GT , Simard M , Rouleau I , Lecomte S , Macoir J , Hudon C ((2015) ) Normative data for the Rappel libre/Rappel indicé à 16 items (16-item Free and Cued Recall) in the elderly Quebec-French population. , S1-S. Clin Neuropsychol 28: , S19–S19. |
[41] | Shulman KI ((2000) ) Clock-drawing: Is it the ideal cognitive screening test? Int J Geriatr Psychiatry 15: , 548–561. |
[42] | Gomez RG , White DA ((2006) ) Using verbal fluency to detect very mild dementia of the Alzheimer type. Arch Clin Neuropsychol 21: , 771–775. |
[43] | Brown PJ , Woods CM , Storandt M ((2007) ) Model stability of the 15-item Geriatric Depression Scale across cognitive impairment and severe depression. Psychol Aging 22: , 372–379. |
[44] | Lucas-Carrasco R , Skevington SM , Gómez-Benito J , Rejas J , March J ((2011) ) Using the WHOQOL-BREF in persons with dementia: A validation study. Alzheimer Dis Assoc Disord 25: , 345–351. |
[45] | Ben Ayed I , Aouichaoui C , Ammar A , Naija S , Tabka O , Jahrami H , Trabelsi K , Trabelsi Y , El Massioui N , El Massioui F ((2024) ) Mid-term and long-lasting psycho–cognitive benefits of bidomain training intervention in elderly individuals with mild cognitive impairment. Eur J Investig Health Psychol Educ 14: , 284–298. |
[46] | Troyer AK , Leach L , Strauss E ((2006) ) Aging and response inhibition: Normative data for the Victoria Stroop Test. Neuropsychol Dev Cogn B Aging Neuropsychol Cogn 13: , 20–35. |
[47] | Scarpina F , Tagini S ((2017) ) The Stroop Color and Word Test. Front Psychol 8: , 557. |
[48] | Gregoire J , Van der Linden M ((1997) ) Effect of age on forward and backward digit spans. Aging Neuropsychol Cogn 4: 140–149. |
[49] | Monaco M , Costa A , Caltagirone C , Carlesimo GA ((2013) ) Forward and backward span for verbal and visuo-spatial data: Standardization and normative data from an Italian adult population. Neurol Sci 34: , 749–754. |
[50] | Muangpaisan W , Intalapaporn S , Assantachai P ((2010) ) Digit span and verbal fluency tests in patients with mild cognitive impairment and normal subjects in Thai-community. J Med Assoc Thail 93: , 224–230. |
[51] | Anderson J , Douglass S ((2001) ) Tower of Hanoi: Evidence for the cost of goal retrieval. J Exp Psychol Learn Mem Cogn 27: , 1331–1346. |
[52] | Fonte C , Smania N , Pedrinolla A , Munari D , Gandolfi M , Picelli A , Varalta V , Benetti MV , Brugnera A , Federico A , Muti E , Tamburin S , Schena F , Venturelli M ((2019) ) Comparison between physical and cognitive treatment in patients with MIC and Alzheimer’s disease. Aging 11: , 3138–3155. |
[53] | Castellano CA , Paquet N , Dionne IJ , Imbeault H , Langlois F , Croteau E , Tremblay S , Fortier M , Matte JJ , Lacombe G , Fülöp T , Bocti C , Cunnane SC ((2017) ) A 3-month aerobic training program improves brain energy metabolism in mild Alzheimer’s disease: Preliminary results from a neuroimaging study. J Alzheimers Dis 56: , 1459–1468. |
[54] | Okamura H , Otani M , Shimoyama N , Fujii T ((2018) ) Combined exercise and cognitive training system for dementia patients: A randomized controlled trial. Dement Geriatr Cogn Disord 45: , 318–325. |
[55] | Kalbe E , Roheger M , Paluszak K , Meyer J , Becker J , Fink GR , Kukolja J , Rahn A , Szabados F , Wirth B , Kessler J ((2018) ) Effects of a cognitive training with and without additional physical activity in healthy older adults: A follow-up 1 year after a randomized controlled trial. Front Aging Neurosci 10: , 407. |
[56] | Wayne PM , Walsh JN , Taylor-Piliae RE , Wells RE , Papp KV , Donovan NJ , Yeh GY ((2014) ) Effect of tai chi on cognitive performance in older adults: Systematic review and meta-analysis . . J Am Geriatr Soc 62: , 25–39. |
[57] | Yu F , Vankee Lin F , Salisbury DL , Shah KN , Chow L , Vock D , Nelson NW , Porsteinsson AP , Jack C Jr ((2018) ) Efficacy and mechanisms of combined aerobic exercise and cognitive training in mild cognitive impairment: Study protocol of the ACT.. Trials 19: , 700. |
[58] | Azizan A , Anum A , Faisal A , Karnadipa T ((2023) ) The effects of exercise and reminiscence therapy on depression and quality of life among the older adults with mild Alzheimer’s disease. J Adv Res Appl Sci Eng Technol 29: , 185–197. |
[59] | Ouanes S , Popp J ((2019) ) High cortisol and the risk of dementia and Alzheimer’s disease: A review of the literature. Front Aging Neurosci 11: , 43. |
[60] | Paillard T , Rolland Y , de Souto Barreto P ((2015) ) Protective effects of physical exercise in Alzheimer’s disease and Parkinson’s disease: A narrative review. J Clin Neurol 11: , 212–219. |
[61] | Wang R , Holsinger RMD ((2018) ) Exercise-induced brain-derived neurotrophic factor expression: Therapeutic implications for Alzheimer’s dementia. Ageing Res Rev 48: , 109–121. |
[62] | Cintoli S , Radicchi C , Noale M , Maggi S , Meucci G , Tognoni G , Bonuccelli U , Sale A , Berardi N , Maffei L , Train the Brain Consortium ((2021) ) Effects of combined training on neuropsychiatric symptoms and quality of life in patients with cognitive decline. Aging Clin Exp Res 33: , 1249–1257. |
[63] | Vidoni ED , Perales J , Alshehri M , Giles A-M , Siengsukon CF , Burns JM ((2019) ) Aerobic exercise sustains performance of instrumental activities of daily living in early-stage Alzheimer disease. Geriatr Phys Ther 42: , E129–E134. |
[64] | Morris JK , Vidoni ED , Johnson DK , Sciver AV , Mahnken JD , Honea RA , Wilkins HM , Brooks WM , Billinger SA , Swerdlow RH , Burns JM ((2017) ) Aerobic exercise for Alzheimer’s disease: A randomized controlled pilot trial. PLoS One 12: , e0170547. |
[65] | Gallaway PJ , Miyake H , Buchowski MS , Shimada M , Yoshitake Y , Kim AS , Hongu N ((2017) ) Physical activity: A viable way to reduce the risks of mild cognitive impairment, Alzheimer’s disease and vascular dementia in older adults. Brain Sci 7: , 22. |
[66] | Wilckens KA , Stillman CM , Waiwood AM , Kang C , Leckie RL , Peven JC , Foust JE , Fraundorf SH , Erickson KI ((2021) ) Exercise interventions preserve hippocampal volume: A meta-analysis. Hippocampus 31: , 335–347. |
[67] | Chapman SB , Aslan S , Spence JS , Defina LF , Keebler MW , Didehbani N , Lu H ((2013) ) Shorter term aerobic exercise improves brain, cognition and cardiovascular fitness in aging. Front Aging Neurosci 5: , 75. |
[68] | Zhang Ya-Ru , Xu W , Zhang W , Wang Hui-Fa , Ou Ya-Nan , Qu Y , Shen X-N , Chen S-D , Wu K-M , Zhao Q-H , Zhang H-N , Sun L , Dong Q , Tan L , Feng L , Zhang C , Evangelou E , Smith AD , Yu J-T ((2022) ) Modifiable risk factors for incident dementia and cognitive impairment: An umbrella review of evidence. J Affect Disord 314: , 160–167. |