Early Benefits with Potential Long-Term Risks of a Comprehensive Intervention on Serum Cortisol Levels and Cognitive Performance in Patients with Alzheimer’s Disease
Abstract
Background:
Elevated cortisol levels represent a risk factor for Alzheimer’s disease (AD), prompting treatments to lower hormone concentrations for preventive or therapeutic purposes.
Objective:
To assess the efficacy of a comprehensive intervention (CI) in modulating serum cortisol levels in patients with AD.
Methods:
CI consisted in a 2-month protocol involving cognitive stimulation, psychological support, lifestyle guidance, leisure activities, and socialization. AD subjects were randomly assigned to experimental (EG, n = 45) and control (CG, n = 45) groups. A wide range of sociodemographic, cognitive, psychosocial, and functional conditions were evaluated before, at the conclusion, and 24 months after CI. Data about lifestyle and drug prescription were also recorded.
Results:
Baseline evaluations revealed that higher cortisol levels correlated with worse cognitive status (higher CDR and ADAS-Cog values and lower MMSE scores), increased depressive symptoms, and reduced physical and social engagement. Following CI, EG exhibited reduced cortisol levels, improved overall cognitive status, and enhanced verbal working memory and executive functions compared to CG. However, at the 24-month follow-up, EG displayed a rebound effect, characterized by elevated cortisol levels and cognitive decline compared to CG.
Conclusions:
These findings strengthen the adverse relationship between excessive cortisol and deficits in cognition/behavior in AD, demonstrate the short-term benefits of CI, and emphasize the potential long-term risks, which may be attributed to the fragile nature of the AD brain. Comprehensive interventions can yield positive results, but careful calibration of type and duration is necessary, considering disease progression and the potential need for re-administration.
INTRODUCTION
The hypothalamus-pituitary-adrenal (HPA) axis is the key neuroendocrine system involved in the physiological response to stress. During aging, the HPA axis undergoes multiple alterations, giving rise to increased circulating cortisol levels, dysregulation of the negative feedback loop controlling cortisol secretion, and a decline in the diurnal cortisol rhythm [1]. The progressive disturbance of stress homeostasis can increase the vulnerability to Alzheimer’s disease (AD) [2]. Indeed, prolonged exposure to cortisol has detrimental effects on the brain, particularly in regions rich in glucocorticoid receptors such as the hippocampus and frontal cortex [3, 4]. This exposure can lead to the deposition of amyloid-β plaques, accumulation of tau tangles, impairment of mitochondrial function, activation of neuroinflammation, and, ultimately, neurodegeneration [5].
Since high levels of cortisol are recognized as a risk factor for AD onset and progression, particularly towards its severe stages [6], several strategies to decrease circulating hormone concentration have been suggested as potential preventive or therapeutic interventions. These approaches range from simple changes in daily habits [7, 8] to more complex procedure such as extracorporeal apheresis [9].
Given that studies conducted in animal models of AD have yielded findings that mirror the stress-induced initiation and exacerbation of AD-related pathology in humans [10], and considering the well-established impact of environmental enrichment on cognition and behavior through HPA axis regulation in animals [11], we applied a similar approach to a patient cohort. In this study, we implemented a comprehensive 2-month protocol, including cognitive stimulation, engagement in leisure activities, and social interaction. This approach challenges the achievement of environmental enrichment in a more complex experimental setting, characterized by differences in genetic backgrounds, cognitive reserve, daily habits, as well as the influence of comorbidities and medications. Our objective was to evaluate the effectiveness of the protocol in reducing cortisol production and to investigate potential correlations between hormone level modulation, cognitive performance, and psychological well-being. We also conducted a 24-month follow-up to assess the long-term effects of the intervention. We enrolled AD subjects to explore the potential for alleviating symptoms, enhancing quality of life, and improving overall patient management.
MATERIALS AND METHODS
Subjects
Ninety community-dwelling AD patients residing in the Marche Region were enrolled from the Evaluation of Alzheimer’s Unit at the IRCCS INRCA Hospital in Fermo (Italy). The research was approved by the Institutional Ethical Committee (code SC/12/301) and was conducted according to the principles delineated in the Declaration of Helsinki. Each participant provided informed consent to participate in the study after a thorough evaluation by healthcare experts, ensuring the participant’s ability to comprehend the content of the document, in the presence of a caregiver. The detailed protocol was previously reported by Giuli and colleagues [12]. Briefly, participants were diagnosed with possible or probable AD based on the diagnostic criteria outlined in the DSM-IV [13] or the NINCDS-ADRDA criteria [14]. In addition, we conducted diagnostic imaging scans and assessed thyroid hormone, vitamin B12, and folic acid levels to rule out factors that might cause severe cognitive deficits other than those associated with AD. In agreement with McKhann and colleagues (2011) [14], the evaluation of cerebrospinal fluid (CSF) biomarkers would enhance the certainty of the possible/probable AD diagnosis. However, the sampling of CSF via lumbar puncture is an invasive procedure that can lead to side effects. Therefore, we opted for a conservative approach, basing the diagnosis on the mandatory criteria. While acknowledging that this decision might introduce some bias, we prioritized ethical considerations, as we deemed the benefits did not outweigh the risks. Inclusion criteria encompassed individuals aged 65 years or older who were available throughout both the training and testing phases, possessed Clinical Dementia Rating (CDR) scores of 1 (indicating mild dementia) or 2 (indicating moderate dementia), and had the active involvement of a caregiver. Exclusion criteria consisted of the presence of significant medical and psychiatric conditions, sensorimotor deficits that would impede participation in CI, prior engagement in any cognitive training program, severe AD, and the presence of neurodegenerative disorders other than AD. Cognitive tests (i.e., Mini-Mental State Examination (MMSE), Alzheimer’s Disease Assessment Scale-Cognitive (ADAS-Cog), Forward digit span, Reverse digit span, Corsi supraspan, Semantic verbal fluency, Immediate prose recall, Delayed prose recall, Total prose recall) were adjusted for age and schooling. Data regarding the sociodemographic, psychosocial, and functional conditions as well as data about lifestyle and drug prescription were also recorded. The study did not include subjects with chronic diseases that could potentially affect cortisol production, however, a patient was under treatment with corticosteroids. Since he/she exhibited no outlying parameters, we decided not to exclude him/her.
Comprehensive intervention (CI)
Each AD patient was randomly assigned to either the experimental group (EG, n = 45) or the control group (CG, n = 45) using a computerized random number generator. The principal investigator received a list of subject IDs and their respective intervention groups. EG subjects underwent a comprehensive multi-modal stimulation training, comprising ten 45-min sessions conducted weekly for approximately 2 months. These sessions focused on individualized goal identification and the practice of tailored strategies. Cognitive enhancement was achieved through mnemonic techniques, including orientation, daily activity planning, and the improvement of episodic and prospective memory. The intervention also aimed to reduce functional disability and maintain cognitive reserve by promoting leisure activities, socialization, healthy lifestyle and nutrition, and offering support for psychological disorders to both patients and caregivers. Participants were additionally instructed to engage in daily cognitive homework, with caregiver assistance. This homework emphasized learning strategies related to categorization, clustering, attention, and visuospatial processes.
CG received no sham protocol but instead received guidance on maintaining a healthy lifestyle and specific psychological support.
A complete re-evaluation (i.e., cortisol levels, cognitive, psychosocial, and functional status) of all patients was performed after the 2-month protocol and at 24 months after the enrollment.
Serum cortisol assay
All blood withdrawals were done between 8 : 00 and 9 : 00 am in a fasting state. Serum cortisol level was evaluated by LIAISON® Cortisol kit (DiaSorin S.p.A., Italy), and data were expressed as ng/mL.
Statistical analyses
Variables’ normality was evaluated by Kolmogorov-Smirnov test. Normally distributed variables were expressed as mean±SEM, while non normally distributed variables as median [lower-upper 95% CI]. Dichotomic variables were expressed as percentage. Comparisons between two groups were performed by t-Student’s, Mann-Whitney or χ2 test, and those among three groups by one-way ANOVA or Kruskal-Wallis’s test. p values were adjusted to account for multiple comparisons by Tukey’s (one-way ANOVA) or Dunn’s (Kruskal-Wallis’s test) tests. Pearson’s or Spearman’s coefficient was applied to assess correlations between variables. Significance was set at p < 0.05. To determine the statistical power of our study, a post hoc calculation was conducted, specifically considering the effect of CI on serum cortisol levels (main outcome of this study). By utilizing the difference between two independent means (two-tailed), an effect size of 0.82 (representing the difference in percent change of cortisol levels between CG and EG), and an α-error of 0.05, we obtained a power value of 0.93. We used a post hoc analysis to assess data robustness due to the difference in sample sizes between the present study (n = 90) and the source cohort, “My Mind Project” (n = 100), from which we obtained subject information and blood samples [12]. We encountered 10 missing data points for cortisol assessment.
RESULTS
Table 1 presents a summary of the basal sociodemographic, psychosocial, functional, and cognitive characteristics of the entire AD group as well as the AD subjects subdivided into CG and EG. Additionally, lifestyle factors, medication prescriptions, and serum cortisol levels are reported. CG and EG did not exhibit any significant differences, except for the forward digit span test, where EG had a significantly lower score than CG. This indicates a poorer working memory and attention in EG, despite the random assignment. To ensure unbiased analysis, we excluded data from this test from further consideration. In terms of age, while no significant differences were observed between CG and EG, we conducted additional analyses using correlation tests for all variables that were not previously adjusted. These analyses revealed no statistically significant associations for GDS-30 (rho = –0.0060, p = 0.957), LSSN (r = 0.0014, p = 0.990), PASE (r = –0.1627, p = 0.130), and serum cortisol levels (r = 0.0279, p = 0.795). Furthermore, our investigation did not uncover any significant differences when comparing subjects with different smoking habits (p = 0.468) or medication usage (AChEI: p = 0.649; NMDA receptor antagonist, p = 0.786; benzodiazepines, p = 0.289; antidepressant, p = 0.792; lipid-lowering medications, p = 0.338; antihypertensives, p = 0.966).
Table 1
Sociodemographic data, functional assessment, cognitive and psychological profile, lifestyle, and pharmacological treatment of the entire Alzheimer’s disease (AD) cohort and the two subgroups
AD (n = 90) | CG (n = 45) | EG (n = 45) | p | |
Age (y) | 77.78±0.55 | 78.84±0.91 | 76.71±0.58 | 0.052 |
Gender (females/total) | 62/90 | 34/45 | 28/45 | 0.255 |
Marital status (%): | ||||
Married | 65 | 62 | 67 | 0.282 |
Unmarried | 3 | 2 | 4 | |
Widowed | 32 | 36 | 29 | |
Years of schooling | 5.00 [4.00–5.00] | 5.00 [3.00–5.00] | 5.00 [4.00–5.00] | 0.227 |
Age of pathology onset (y) | 75.80±0.57 | 76.39±0.96 | 75.21±0.62 | 0.306 |
Pathology duration (months) | 18 [12–24] | 20 [12–36] | 12 [6–24] | 0.119 |
GDS-30 (long version) | 8.5 [7.0–10.0] | 9.0 [6.0–11.0] | 8.0 [7.0–12.0] | 0.601 |
LSSN | 26.85±0.71 | 27.70±0.95 | 26.00±1.06 | 0.234 |
ADL | 6 [5–6] | 6 [5–6] | 6 [5–6] | 0.188 |
IADL | 3 [3–4] | 4 [2–4] | 3 [2–4] | 0.866 |
MMSE | 20.27±0.38 | 20.27±0.53 | 20.28±0.55 | 0.993 |
ADAS-Cog | 19 [17–22] | 17 [14–23] | 19 [17–23] | 0.390 |
CDR | 1 [1–2] | 1 [1–2] | 1 [1–2] | >0.999 |
Forward digit span | 4.00 [3.50–4.50] | 4.25 [3.75–4.75] | 3.50 [3.50–4.50] | 0.023 |
Reverse digit span | 2 [2–2] | 2 [2–2] | 2 [2–2] | 0.241 |
Corsi supraspan | 4.50 [3.75–4.75] | 4.50 [3.75–4.75] | 3.75 [3.50–4.75] | 0.590 |
Semantic verbal fluency | 1 [0–1] | 1 [10–1] | 0 [0–1] | 0.486 |
Immediate prose recall | 1.1 [0.0–3.0] | 0.0 [0.0–3.0] | 2.0 [1.0–4.0] | 0.065 |
Delayed prose recall | 0 [0–0] | 0 [0–1] | 0 [0–2] | 0.341 |
Total prose recall | 2.67 [1.50–5.0] | 1.60 [1.25–4.50] | 4.40 [2.00–6.25] | 0.104 |
PASE | 61.74±4.25 | 67.19±6.74 | 55.80±5.16 | 0.183 |
Smoking habits (%): | ||||
Never smoked | 60 | 69 | 51 | 0.139 |
Former smoker | 28 | 20 | 36 | |
Current smoker | 12 | 11 | 13 | |
AChEI (% of user) | 53 | 51 | 49 | >0.999 |
NMDA receptor antagonists (% of user) | 12 | 16 | 9 | 0.522 |
Benzodiazepines (% of user) | 11 | 9 | 13 | 0.739 |
Antidepressants (% of user) | 16 | 9 | 22 | 0.144 |
Lipid lowering medications (% of user) | 17 | 13 | 20 | 0.409 |
Antihypertensives (% of user) | 36 | 31 | 42 | 0.382 |
Corticosteroids (% of user) | 1% | 2% | 0% | >0.999 |
Serum cortisol level (ng/mL) | 13.0 [12.6–14.0] | 12.6 [12.9–14.2] | 13.5±2.9 | 0.399 |
GDS, Geriatric Depression Scale; LSNS, Lubben Social Network Scale; ADL, Activities of Daily Living; IADL, Instrumental Activities of Daily Living; MMSE, Mini-Mental State Examination; ADAS-Cog, Alzheimer’s Disease Assessment Scale-Cognitive; CDR, Clinical Dementia Rating; PASE, Physical Activity Scale for Elderly; AChEI, acetylcholinesterase inhibitors. Significant differences between patients successively subdivided into control (CG) and experimental (EG) groups are in bold.
At baseline, the higher the cortisol amount, the worse the cognitive status
Cortisol level was significantly higher in CDR2 (n = 39) than CDR1 (n = 51) patients (14.00 [13.30–15.16] versus 12.71 [11.78–13.65] ng/mL, p = 0.021) and significantly correlated with worse MMSE (p = 0.043, n = 88), ADAS-Cog (p = 0.008, n = 90) and Corsi supraspan (p = 0.018, n = 88) scores (Fig. 1A-C). No significance was found for the other tests (Supplementary Table 1).
Fig. 1
Serum cortisol level significantly correlates with cognitive performances and lifestyle in patients with Alzheimer’s disease. The higher the cortisol level, the worse the MMSE (A), the ADAS-Cog (B), and the Corsi supraspan (C) scores. The higher the serum cortisol level, the lower the physical activity (D), the lower the social network (E), and the higher the depressive mood (F). MMSE, Mini-Mental State Examination; ADAS-Cog, Alzheimer’s Disease Assessment Scale-Cognitive; PASE, Physical Activity Scale for Elderly; LSNS, Lubben Social Network Scale; GDS, Geriatric Depression Scale.
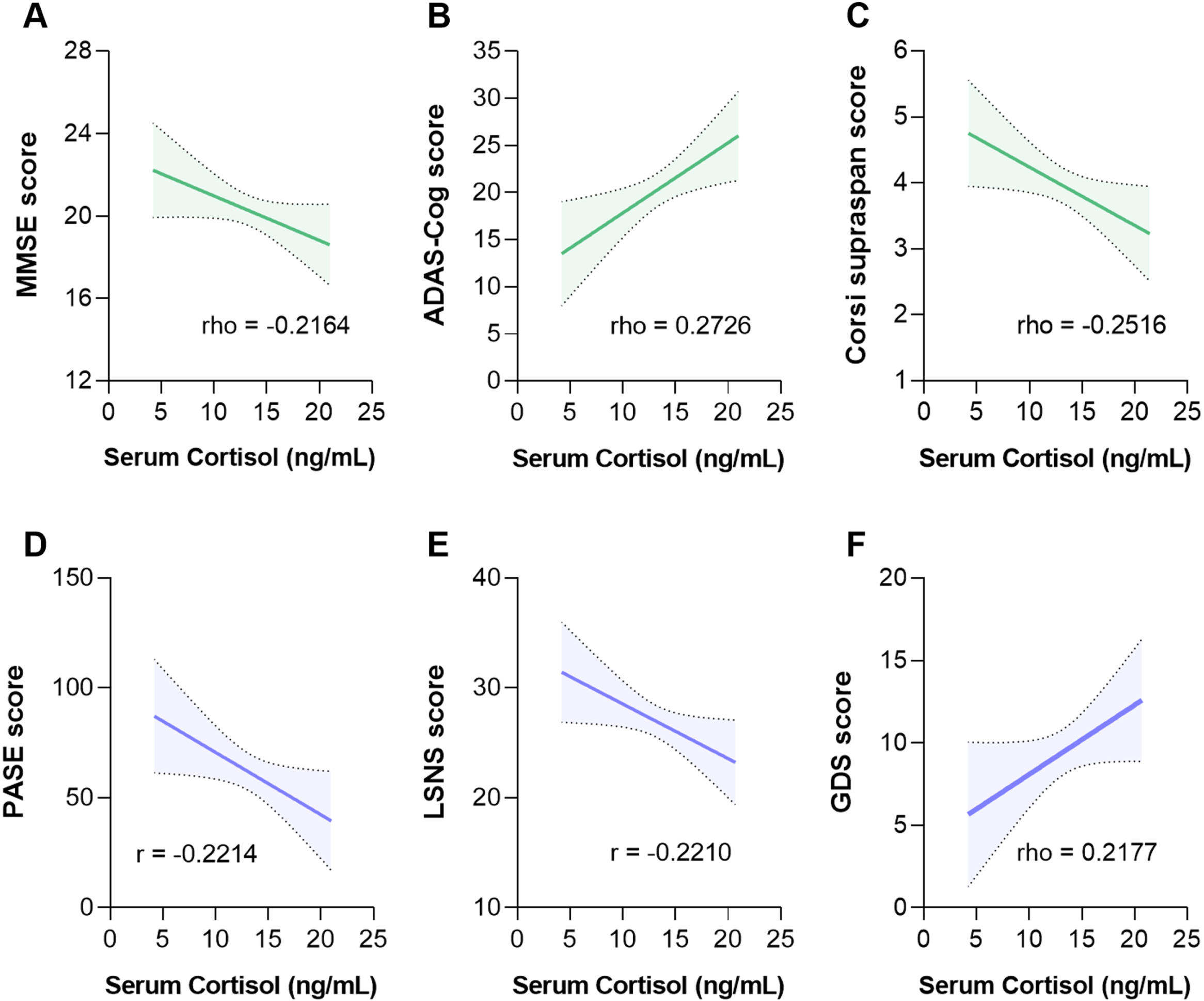
Basal healthy lifestyle was associated to lower cortisol level
The higher the physical activity (p = 0.039, n = 85 for PASE) and the social engagement (p = 0.042, n = 85 for LSNS), the lower the cortisol amount; the higher the depressive mood (p = 0.045, n = 85 for GDS), the higher the cortisol amount (Fig. 1D-F). No significance was found for smoking habits (p = 0.241, n = 86). Multiple linear regressions were applied to evaluate a possible cumulative effect of serum cortisol level, PASE, LSNS, and GDS on the cognitive domains. Although a collective significant effect was found for MMSE score (F1,83 = 7.460, p = 0.008) and ADAS-Cog score (F1,86 = 11.19, p = 0.010), the evaluation of the individual predictors showed that only the serum level of cortisol remained significant (MMSE: t = –2.3732, p = 0.008; ADAS-Cog: t = 3.345, p = 0.001), highlighting the key impact of the hormone on AD patients’ cognitive performance.
At baseline, medications did not influence serum cortisol level
No significant differences were observed in the serum cortisol levels between users and non-users of AChEI, NMDA receptor antagonists, benzodiazepines, antidepressants, lipid lowering medications, antihypertensives, and corticosteroids (Supplementary Table 2). This indicates that the pharmacological therapies employed for AD or other comorbidities in our cohort did not have a mitigating or exacerbating effect on the impact of cortisol on the cognitive abilities of patients.
The CI-related reduction of serum cortisol level mirrored the improvement of cognitive performances
Comparing the percent change (post-CI delta) after the 2-month protocol { × 100}, EG showed a significantly higher reduction of serum cortisol level (p = 0.001) and ADAS-Cog score (p = 0.001) and a significantly higher increase of MMSE (p = 0.007), reverse digit span (p = 0.004), and semantic fluency (p = 0.036) scores than CG (Fig. 2A-E). The preference for percent changes over absolute changes was made to ‘normalize’ the inter-individual differences, thus directing attention toward the impact of CI regardless of the initial parameter values for each subject. No significance was found for the other tests (Supplementary Table 3).
Fig. 2
The 2-month comprehensive intervention resulted in a significant reduction in serum cortisol levels and improvement in cognitive performance. EG exhibited a significantly greater reduction in serum cortisol level (A), a significantly greater improvement in general cognitive status (reflected by a reduction in ADAS-Cog (B) and an increase in MMSE (C) scores), and significantly better performance in the reverse digit span (D) and semantic fluency (E) tests compared to the CG. CG, control group; EG, experimental group; ADAS-Cog, Alzheimer’s Disease Assessment Scale-Cognitive; MMSE, Mini-Mental State Examination (MMSE). *p < 0.05, **p < 0.01, ***p < 0.001.
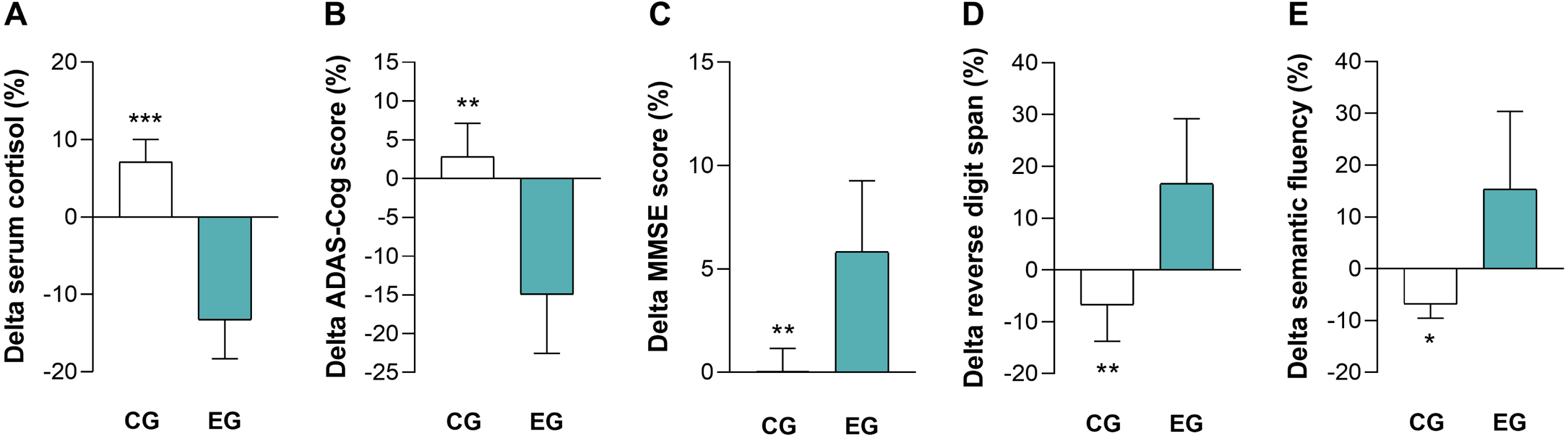
The basal concentration of serum cortisol significantly correlated with post-CI delta of MMSE (rho = 0.3411, p = 0.031) and ADAS-Cog (rho = –0.3329, p = 0.033) scores in EG but not in CG (MMSE: rho =-0.1010, p = 0.560; ADAS-Cog: rho =-0.0119, p = 0.938), suggesting a CI-related phenomenon. Thus, the baseline serum cortisol level appears to influence the response of AD patients to the intervention since patients with higher basal hormone concentration showed better improvement of cognitive status.
After the 24-month follow-up, EG exhibited a rebound effect in terms of serum cortisol level and cognitive performance
After 24 months, EG (n = 41) showed significantly higher percent increase of serum cortisol level (Fig. 3A), ADAS-Cog scores (Fig. 3B), and CDR values (Fig. 3D) as well as significantly higher percent reduction of MMSE scores (Fig. 3C) compared to CG (n = 36). No significant differences were found in the other cognitive tests (data not shown). Regarding the dropout rate (i.e., 4 subjects in EG and 9 subjects in CG), no statistical differences emerged (Fisher’s exact test, p = 0.756).
Fig. 3
After 24 months, EG showed an increase in serum cortisol level and a greatest cognitive decline compared to CG. Percent change versus baseline of serum cortisol level was significantly higher in EG to CG (A). Percent change versus baseline of ADAS-Cog scores was significantly higher in EG compared to CG (B). Percent change versus baseline of MMSE values was significantly lower in EG compared to CG (C). Percent change versus baseline of CDR values was significantly higher in EG compared to CG (D). CG, control group; EG, experimental group; ADAS-Cog, Alzheimer’s Disease Assessment Scale-Cognitive; MMSE, Mini-Mental State Examination; CDR, Clinical Dementia Rate. *p < 0.05, ***p < 0.001.
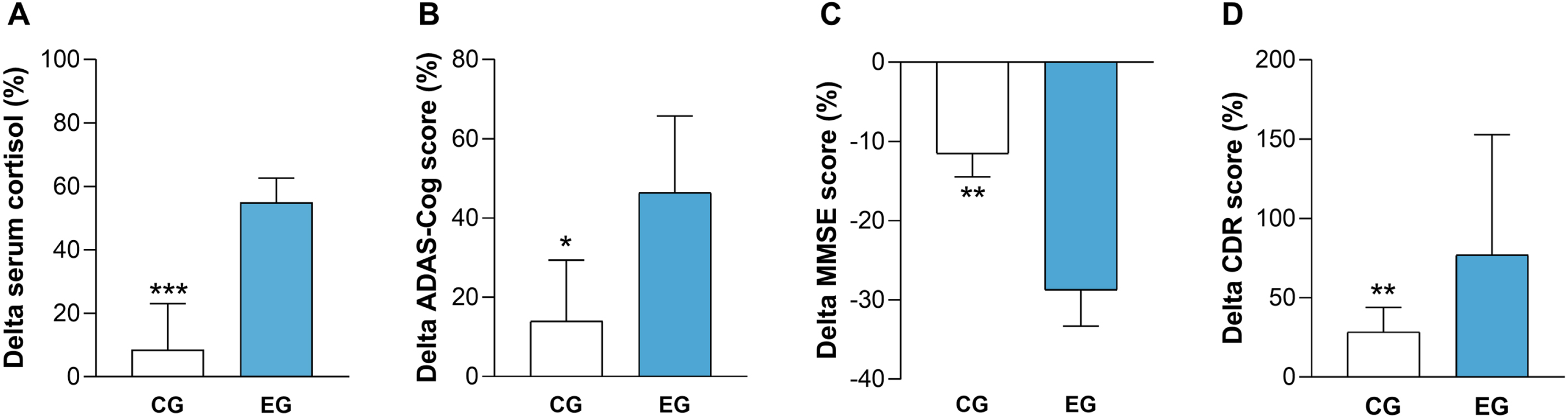
These findings suggest that following the positive outcomes observed immediately after the end of CI, AD patients who underwent the stimulation experienced a rebound effect characterized by a greater increase in serum cortisol level and a worsened cognitive impairment compared to the CG during the 24-month follow up.
DISCUSSION
The present study showed a correlation between elevated levels of circulating cortisol and impaired cognitive status in patients with AD: higher concentrations of the hormone were associated with higher CDR and ADAS-Cog scores, along with lower MMSE and Corsi supraspan values. Our research also revealed that elevated cortisol levels are part of a vicious cycle involving low physical activity, limited social engagement, and negative mood status.
Our findings are consistent with previous researchers that have analyzed the general cognitive status of AD patients [15–17] as well as specific domains as verbal fluency [18] and memory [19, 20]. The mechanisms underlying the detrimental effects of HPA axis dysfunction on the brain remain widely unknown. It is possible that several processes act together to contribute to these effects. Under overstimulation of glucocorticoid receptors, the activation of microglia can trigger neuroinflammation [21], excessive cytokine production can impair neurogenesis [22], specific posterior cortical regions may experience glucose hypometabolism [23], and interaction with Aβ oligomers may impair hippocampal synaptic long-term potentiation [24]. Besides, as AD progresses, the hypothalamus itself undergoes alterations, leading to a transition where it might shift from being regarded as a culprit to becoming a target for pathological mechanisms [25]. Regarding the topic of cortisol and lifestyle, the “stress hypothesis” proposes that the cognitive benefits associated with exercise in older adults may be partially mediated by changes in cortisol secretion patterns [7], and a recent study has shown a connection between low to moderate perceived social support, depressive symptoms, dysregulation of the HPA axis, and structural brain alterations in older adults [26]. This outcome holds significant importance as it strongly emphasizes the necessity of addressing modifiable risk factors to prevent the onset of AD or reduce the progression of functional decline.
Considering this perspective, in recent years, non-pharmacological interventions aimed at alleviating chronic stress parameters in AD have been assessed with promising achievements [27–29]. The approach employed in our study follows this trend. Subjects in the EG had significant reductions in serum cortisol levels, improvements in overall cognitive status, and enhancements in verbal working memory and executive functions compared to the control group. Importantly, individuals with higher baseline cortisol levels derived the greatest benefits from the cognitive intervention. It is plausible to hypothesize that individuals with more pronounced alterations in the HPA axis regained greater functional homeostasis, and the reduction of negative effects in brain areas particularly rich in hormone receptors (such as the hippocampus) may have supported greater improvements in cognitive performance. This finding highlights the significance of personalized medicine.
Despite the potential benefits, an overlooked aspect is the long-term consequences of implementing stimulating protocols in AD patients, as follow-up assessments are typically lacking. This presents a critical concern, as demonstrated in this study. After 24 months, the EG experienced a rebound effect characterized by elevated cortisol levels and worsening cognitive performance compared to CG. The “frail” nature of the AD brain may offer a plausible explanation for these findings. When stimulation was interrupted, the brain had to establish a new equilibrium. However, the previous homeostatic state might not be restored, potentially resulting in a deteriorated environment [30, 31]. This serves as a crucial warning, emphasizing the necessity to carefully calibrate the type and duration of the intervention and potentially readminister it in an adapted manner considering disease progression. Besides, recent findings from clinical trials indicate the need to develop longer multimodal interventions for older adults with impaired cognition [32].
This study has certain limitations. Firstly, the evaluation of serum cortisol levels was only conducted in the morning, neglecting diurnal variations. However, previous studies have indicated that the cortisol levels upon awakening are the ones that exhibit differences between AD patients and cognitively healthy controls [18, 33], and a recent meta-analysis has highlighted that hypersecretion, rather than circadian dysrhythmia, is characteristic of individuals with AD [34]. Secondly, the study lacks information regarding the genetic profile of the patients, specifically considering the influence of APOE ɛ4 on the detrimental consequences of HPA axis dysfunction [35]. However, it has been suggested that the APOE ɛ4 genotype is predictive of central nervous system cortisol levels but not serum cortisol amounts [36]. Thirdly, the use of a blind approach was not feasible, and a certain impact of the placebo effect cannot be ruled out. Additionally, the homogeneity of the cohort in terms of ethnicity and regionality that reduce the generalizability of the findings. The study also possesses strengths. The 24-month follow-up period revealed the potential risks associated with non-pharmacological interventions. Extensive data were collected to define the AD cohort, and the statistical power obtained through the sample size was exceptionally robust.
In conclusion, our study yielded the following findings: 1) we reinforced the possible association between excessively high serum levels of cortisol and worse cognitive performance in AD patients, and highlighted the importance of focusing on daily habits that can be easily modified through targeted interventions (baseline evaluations); 2) we provided evidence of the efficacy of CI and observed variations in subjects’ responses based on their basal hormone concentrations (immediately after CI); and 3) we reported the potential long-term risks associated with a limited period of stimulation, which can leave the brain incapable of readapting (after 24 months).
Future studies might consider multicenter recruitment to ensure the inclusion of diverse ethnicities and lifestyles. Blood analysis might be compared with more accessible matrices like saliva. Additionally, it would be desirable to investigate the influence of circadian hormone variations on the wide range of parameters analyzed in this project.
ACKNOWLEDGMENTS
The authors have no acknowledgments to report.
FUNDING
The study was supported by “Ricerca Finalizzata” grant 154/GR-2009-1584108 (Italian Ministry of Health and Regione Marche).
CONFLICT OF INTEREST
The authors have no conflict of interest to report.
DATA AVAILABILITY
The data supporting the findings of this study are available on request from the corresponding author. The data are not publicly available due to privacy or ethical restrictions.
SUPPLEMENTARY MATERIAL
[1] The supplementary material is available in the electronic version of this article: https://dx.doi.org/10.3233/ADR-230125.
REFERENCES
[1] | Stamou MI , Colling C , Dichtel LE ((2023) ) Adrenal aging and its effects on the stress response and immunosenescence. Maturitas 168: , 13–19. |
[2] | Ouanes S , Popp J ((2019) ) High cortisol and the risk of dementia and Alzheimer’s disease: A review of the literature. Front Aging Neurosci 11: , 43. |
[3] | Joëls M , Vreugdenhil E ((1998) ) Corticosteroids in the brain. Cellular and molecular actions. Mol Neurobiol 17: , 87–108. |
[4] | Furay AR , Bruestle AE , Herman JP ((2008) ) The role of the forebrain glucocorticoid receptor in acute and chronic stress. Endocrinology 149: , 5482–5490. |
[5] | Hafdi M , Hoevenaar-Blom MP , Richard E ((2021) ) Multi-domain interventions for the prevention of dementia and cognitive decline. Cochrane Database Syst Rev 11: , CD013572. |
[6] | Lyons CE , Bartolomucci A ((2020) ) Stress and Alzheimer’s disease: A senescence link? Neurosci Biobehav Rev 115: , 285–298. |
[7] | Tortosa-Martínez J , Manchado C , Cortell-Tormo JM , Chulvi-Medrano I ((2018) ) Exercise, the diurnal cycle of cortisol and cognitive impairment in older adults. Neurobiol Stress 9: , 40–47. |
[8] | Pistollato F , Sumalla Cano S , Elio I , Masias Vergara M , Giampieri F , Battino M ((2016) ) Associations between sleep, cortisol regulation, and diet: Possible implications for the risk of Alzheimer disease. Adv Nutr 7: , 679–689. |
[9] | Bornstein SR , Voit-Bak K , Rosenthal P , Tselmin S , Julius U , Schatz U , Boehm BO , Thuret S , Kempermann G , Reichmann H , Chrousos GP , Licinio J , Wong ML , Schally AV , Straube R ((2020) ) Extracorporeal apheresis therapy for Alzheimer disease-targeting lipids, stress, and inflammation. Mol Psychiatry 25: , 275–282. |
[10] | Carroll JC , Iba M , Bangasser DA , Valentino RJ , James MH , Brunden KR , Lee V M-Y , John , Trojanowski JQ ((2011) ) Chronic stress exacerbates tau pathology, neurodegeneration, and cognitive performance through a corticotropin-releasing factor receptor-dependent mechanism in a transgenic mouse model of tauopathy. J Neurosci 3: , 14436–14449. |
[11] | Crofton EJ , Zhang Y , Green TA ((2015) ) Inoculation stress hypothesis of environmental enrichment. Neurosci Biobehav Rev 49: , 19–31. |
[12] | Giuli C , Fattoretti P , Gagliardi C , Mocchegiani E , Venarucci D , Balietti M , Casoli T , Costarelli L , Giacconi R , Malavolta M , Papa R , Lattanzio F , Postacchini D ((2017) ) My Mind Project: The effects of cognitive training for elderly—the study protocol of a prospective randomized intervention study. Aging Clin Exp Res 29: , 353–360. |
[13] | American Psychiatric Association ((2000) ) Diagnostic and statistical manual of mental disorders (4th ed.). American Psychiatric Association, Washington DC. |
[14] | McKhann GM , Knopman DS , Chertkow H , Hyman BT , Jack CR Jr , Kawas CH , Klunk WE , Koroshetz WJ , Manly JJ , Mayeux R , Mohs RC , Morris JC , Rossor MN , Scheltens P , Carrillo MC , Thies B , Weintraub S , Phelps CH ((2011) ) The diagnosis of dementia due to Alzheimer’s disease: Recommendations from the National Institute on Aging-Alzheimer’s Association workgroups on diagnostic guidelines for Alzheimer’s disease. Alzheimers Dement 7: , 263–269. |
[15] | Ouanes S , Clark C , Richiardi J , Maréchal B , Lewczuk P , Kornhuber J , Kirschbaum C , Popp J ((2022) ) Cerebrospinal fluid cortisol and dehydroepiandrosterone sulfate, Alzheimer’s Disease pathology, and cognitive decline. Front Aging Neurosci 14: , 892754. |
[16] | Yeram N , Dalvi S , Mankeshwar R , Patil V , Kale V , Jagiasi K , Abichandani L ((2021) ) Relationship between cortisol, Interleukin-6 and homocysteine in Alzheimer’s disease. Qatar Med J 2021: , 1–10. |
[17] | Huang CW , Lui CC , Chang WN , Lu CH , Wang YL , Chang CC ((2009) ) Elevated basal cortisol level predicts lower hippocampal volume and cognitive decline in Alzheimer’s disease. J Clin Neurosci 16: , 1283–1286. |
[18] | Dronse J , Ohndorf A , Richter N , Bischof GN , Fassbender R , Behfar Q , Gramespacher H , Dillen K , Jacobs HIL , Kukolja J , Fink GR , Onur OA ((2023) ) Serum cortisol is negatively related to hippocampal volume, brain structure, and memory performance in healthy aging and Alzheimer’s disease. Front Aging Neurosci 15: , 1154112. |
[19] | Holleman J , Adagunodo S , Kåreholt I , Hagman G , Aspö M , Udeh-Momoh CT , Solomon A , Kivipelto M , Sindi S ((2022) ) Cortisol, cognition and Alzheimer’s disease biomarkers among memory clinic patients. BMJ Neurol Open 4: , e000344. |
[20] | Peña-Bautista C , Baquero M , Ferrer I , Hervás D , Vento M , García-Blanco A , Cháfer-Pericás C ((2019) ) Neuropsychological assessment and cortisol levels in biofluids from early Alzheimer’s disease patients. Exp Gerontol 123: , 10–16. |
[21] | Armstrong AM , Porter T , Quek H , White A , Haynes J , Jackaman C , Villemagne V , Munyard K , Laws SM , Verdile G , Groth D ((2021) ) Chronic stress and Alzheimer’s disease: The interplay between the hypothalamic-pituitary-adrenal axis, genetics and microglia. Biol Rev Camb Philos Soc 96: , 2209–2228. |
[22] | Ricci S , Fuso A , Ippoliti F , Businaro R ((2012) ) Stress-induced cytokines and neuronal dysfunction in Alzheimer’s disease. J Alzheimers Dis 28: , 11–24. |
[23] | Wirth M , Lange C , Huijbers W ; Alzheimer’s Disease Neuroimaging Initiative ((2019) ) Plasma cortisol is associated with cerebral hypometabolism across the Alzheimer’s disease spectrum. Neurobiol Aging 84: , 80–89. |
[24] | Qi Y , Klyubin I , Ondrejcak T , Hu NW , Rowan MJ ((2021) ) Enduring glucocorticoid-evoked exacerbation of synaptic plasticity disruption in male rats modelling early Alzheimer’s disease amyloidosis. Neuropsychopharmacology 46: , 2170–2179. |
[25] | Ishii M , Iadecola C ((2015) ) Metabolic and non-cognitive manifestations of Alzheimer’s disease: The hypothalamus as both culprit and target of pathology. Cell Metab 22: , 761–776. |
[26] | van der Velpen IF , de Feijter M , Raina R , Özel F , Perry M , Ikram MA , Vernooij MW , Luik AI ((2023) ) Psychosocial health modifies associations between HPA-axis function and brain structure in older age. Psychoneuroendocrinology 153: , 106106. |
[27] | Chéour S , Chéour C , Kilani C , Guemri A , Zineddine D , Khélifa R , Supriya R , Bragazzi NL , Chéour F , Baker JS , Gaied-Chortane S ((2022) ) Salivary testosterone and cortisol levels in Tunisian elderly male patients with mild Alzheimer’s disease. Implications of musical therapy and/or physical rehabilitation. Front Physiol 13: , 839099. |
[28] | D’Cunha NM , McKune AJ , Isbel S , Kellett J , Georgousopoulou EN , Naumovski N ((2019) ) Psychophysiological responses in people living with dementia after an art gallery intervention: An exploratory study. J Alzheimers Dis 72: , 549–562. |
[29] | Venturelli M , Sollima A , Cè E , Limonta E , Bisconti AV , Brasioli A , Muti E , Esposito F ((2016) ) Effectiveness of exercise- and cognitive-based treatments on salivary cortisol levels and sundowning syndrome symptoms in patients with Alzheimer’s disease. J Alzheimers Dis 53: , 1631–1640. |
[30] | Balietti M , Giuli C , Fattoretti P , Fabbietti P , Papa R , Postacchini D , Conti F ((2017) ) Effect of a comprehensive intervention on plasma BDNF in patients with Alzheimer’s disease. J Alzheimers Dis 57: , 37–43. |
[31] | Clegg A , Young J , Iliffe S , Rikkert MO , Rockwood K ((2013) ) Frailty in elderly people. Lancet 381: , 752–762. |
[32] | Hafizi S , Rajji TK ((2023) ) Modifiable risk factors of dementia linked to excitation-inhibition imbalance. Ageing Res Rev 83: , 101804. |
[33] | Zheng B , Tal R , Yang Z , Middleton L , Udeh-Momoh C ((2020) ) Cortisol hypersecretion and the risk of Alzheimer’s disease: A systematic review and meta-analysis. Ageing Res Rev 64: , 101171. |
[34] | Saelzler UG , Verhaeghen P , Panizzon MS , Moffat SD ((2021) ) Intact circadian rhythm despite cortisol hypersecretion in Alzheimer’s disease: A meta-analysis. Psychoneuroendocrinology 132: , 105367. |
[35] | Gerritsen L , Comijs HC , Deeg DJH , Penninx B , Geerlings MI ((2011) ) Salivary cortisol, APOE-ɛ4 allele and cognitive decline in a prospective study of older persons. Neurobiol Aging 32: , 1615–1625. |
[36] | Fiocco AJ , Poirier J , Joober R , Nair NP , Lupien SJ ((2008) ) Acute and long-term associations between ApoE genetic polymorphism, cortisol levels, and declarative memory performance in older adults. Psychoneuroendocrinology 33: , 625–633. |