Resistance Training Maintains White Matter and Physical Function in Older Women with Cerebral Small Vessel Disease: An Exploratory Analysis of a Randomized Controlled Trial
Abstract
Background:
As the aging population grows, there is an increasing need to develop accessible interventions against risk factors for cognitive impairment and dementia, such as cerebral small vessel disease (CSVD). The progression of white matter hyperintensities (WMHs), a key hallmark of CSVD, can be slowed by resistance training (RT). We hypothesize RT preserves white matter integrity and that this preservation is associated with improved cognitive and physical function.
Objective:
To determine if RT preserves regional white matter integrity and if any changes are associated with cognitive and physical outcomes.
Methods:
Using magnetic resonance imaging data from a 12-month randomized controlled trial, we compared the effects of a twice-weekly 60-minute RT intervention versus active control on T1-weighted over T2-weighted ratio (T1w/T2w; a non-invasive proxy measure of white matter integrity) in a subset of study participants (N = 21 females, mean age = 69.7 years). We also examined the association between changes in T1w/T2w with two key outcomes of the parent study: (1) selective attention and conflict resolution, and (2) peak muscle power.
Results:
Compared with an active control group, RT increased T1w/T2w in the external capsule (p = 0.024) and posterior thalamic radiations (p = 0.013) to a greater degree. Increased T1w/T2w in the external capsule was associated with an increase in peak muscle power (p = 0.043) in the RT group.
Conclusion:
By maintaining white matter integrity, RT may be a promising intervention to counteract the pathological changes that accompany CSVD, while improving functional outcomes such as muscle power.
INTRODUCTION
White matter hyperintensities (WMHs) are a hallmark of cerebral small vessel disease (CSVD) and are highly prevalent in older adults (exceeding 90% of adults over the age of 60 in some population-based studies) [1]. Greater WMH volume is associated with increased dementia risk [2] and negatively impacts both cognitive (e.g., impaired executive functions) and physical (e.g., impaired balance, slower gait, reduced muscle strength) function [3–6]. Thus, interventions that slow the progression of WMHs are needed to reduce dementia risk and promote cognitive and physical outcomes in older adults. In particular, multiple longitudinal and randomized controlled trial (RCT) studies have linked physical inactivity to WMH progression; identifying exercise as a promising intervention [7–10].
Exercise can be broadly divided into aerobic training (AT) and resistance training (RT). A recent review found both affect brain structure and neurochemical markers of neuroplasticity to a similar degree, which was associated with improved motor (but not cognitive) outcomes in healthy older adults [11]. AT includes moderate-intensity activities sustained for longer periods of time, promoting cardiovascular fitness (e.g., running, swimming). Evidence for the beneficial effects of AT on maintaining white matter integrity and reducing WMH progression is robust [12–16]. However, one study found no white matter changes with a 12-week AT intervention, suggesting the exercise must be maintained for a certain duration to be effective [17]. Additionally, we previously demonstrated that AT’s effects on WMH progression may be sex-specific and only beneficial for males [18]. Thus, while AT shows promise, the parameters and recipient characteristics may need to be specified to produce beneficialeffects.
RT, a form of exercise that promotes muscular strength and endurance by contracting muscles against external force, can positively impact cognitive, physical, and brain outcomes in older adults [19–21]. Specifically, we previously demonstrated that older females who underwent 52 weeks of twice-weekly RT exhibited functional plasticity, improved executive functions, and increased peak muscle power compared with an active control group [22]. Notably, we found twice-weekly RT significantly reduced WMH progression [23]. This finding was corroborated by the Study of Mental and Resistance Training (SMART) trial, which showed RT reduced WMH progression while also improving global cognition in older adults with mild cognitive impairment [24]. However, the mechanisms by which RT protects against WMH progression and other white matter damage characteristic of CSVD is currently unknown.
A non-invasive and indirect measure of white matter integrity can be derived from dividing a T1-weighted (T1w) magnetic resonance imaging (MRI) scan by a T2-weighted (T2w) MRI scan (i.e., T1w/T2w) [25]. The structural composition of a tissue affects its longitudinal relaxation time (T1) and transverse relaxation time (T2). White matter’s short T1 leads to it appearing bright on T1-weighted images, whereas grey matter’s long T2 leads to it appearing bright on T2-weighted images [26]. Originally, because myelin increases the T1w signal while decreasing the T2w signal, dividing the former by the latter was proposed to enhance the signal primarily attributed to myelin [27]. However, recent literature suggests stronger correlations between the ratio and other neurological features such as neurite density [28]. Thus, the T1w/T2w ratio is increasingly being used as a measure of overall white matter integrity. Notably, a recent study found participants of a 6-month AT intervention showed increased regional T1w/T2w compared with a decrease in the active control. This increase in white matter integrity was correlated with an improvement in episodic memory performance (but not with an improvement in cardiorespiratory fitness, or changes in executive function or perceptual speed) [16]. Similar studies have yet to be done for RT, a gap this study attempted to fill.
We conducted an exploratory analysis of a 52-week randomized controlled trial of RT in community-dwelling females aged 65–75 years old to examine the effect of twice-weekly RT on T1w/T2w ratio. We hypothesized that 12 months of twice-weekly RT would increase or maintain T1w/T2w, suggesting RT benefits white matter integrity. We also hypothesized that an increase in T1w/T2w would be associated with improvements in: 1) executive functions, as measured by the Stroop Color-Word Test; and 2) peak muscle power, as measured by a leg press.
METHODS
Study design
We conducted a 52-week RCT of RT (clinicaltrials.gov Identifier: NCT00426881) with behavioral measurements at baseline, 26 weeks, and trial completion [22]. Assessors were trained by the research team and were blinded to group allocation of the participants. Magnetic resonance imaging (MRI) data was acquired at baseline and trial completion in a subset of eligible participants.
Participants
Details of the inclusion/exclusion criteria can be found in the parent study [22]. Briefly, we included community-dwelling females who: 1) were aged 65 to 75 years old and living in Metropolitan Vancouver; 2) were living independently in their own home; 3) scored ≥24/30 on the Mini-Mental State Examination (MMSE); and 4) had a visual acuity of ≥20/40, with or without corrective lenses. Individuals were excluded if they: 1) had a medical condition for which exercise is contraindicated; 2) regularly participated in RT in the previous 6 months; 3) had a neurodegenerative disease and/or stroke; 4) had depression; 5) were not fluent in English, 6) were taking cholinesterase inhibitors; and/or 7) were on estrogen replacement or testosterone therapy. Only females were included as evidence suggests there are sex differences in the cognitive response to exercise [29].
Figure 1, the CONSORT (Consolidated Standards of Reporting Trial) flowchart, shows the number and distribution of participants included in this exploratory analysis. For this exploratory analysis, we included only participants from the twice-weekly RT group and the active control group who completed MRI at baseline and trial completion. This is because our prior work showed once-weekly RT had no benefit for WMH progression compared with active control [23].
Fig. 1
Consolidated Standards of Reporting Trial Flowchart of Participants. MRI, magnetic resonance imaging; 1x RT, once-weekly resistance training; 2x RT, twice-weekly resistance training; BAT, balance and tone training.
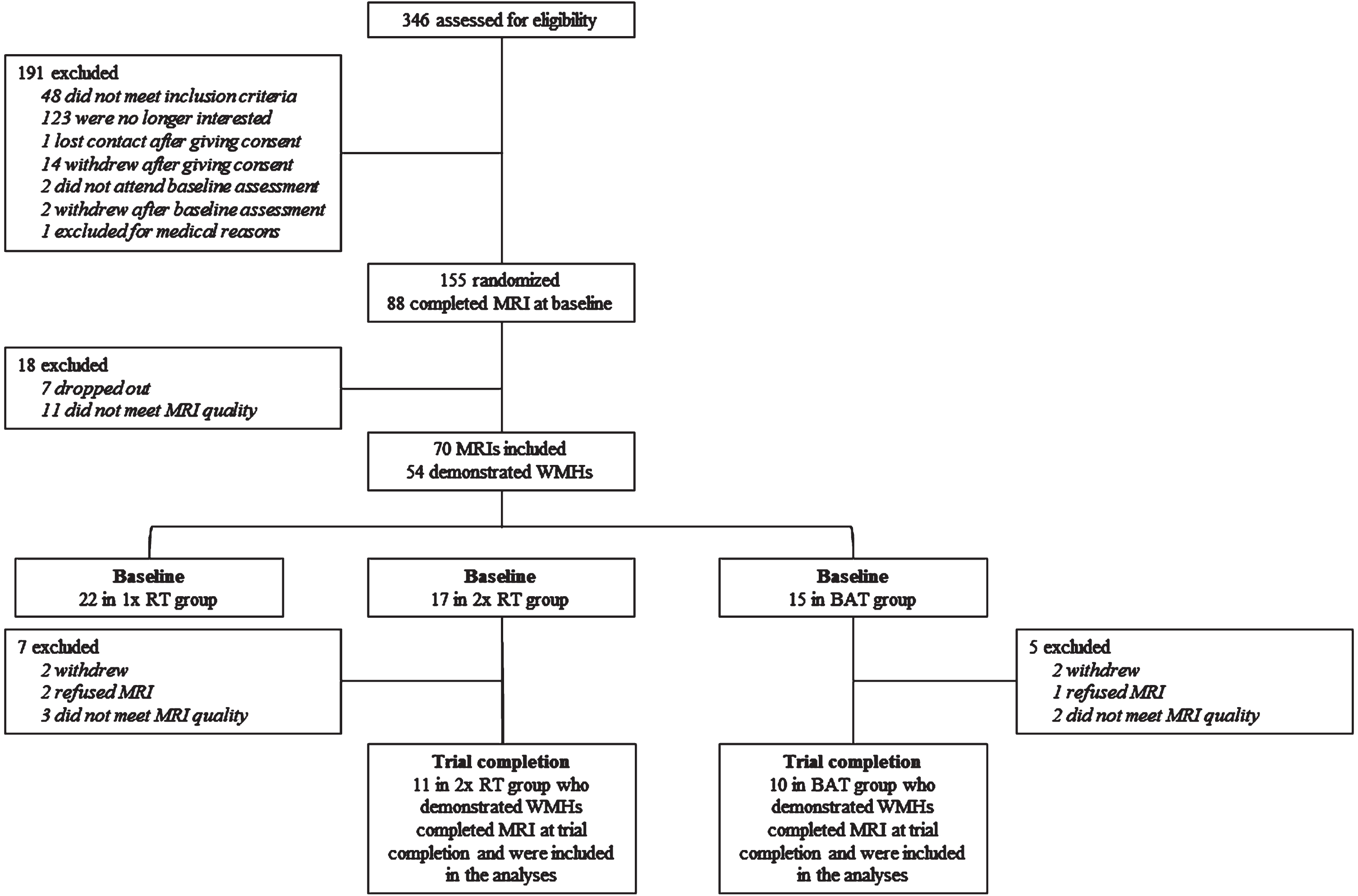
Ethical approval was obtained from the University of British Columbia Clinical Research Ethics Board (H06-03217) and the Vancouver Coastal Health Research Institute (V06-03216). All participants provided informed written consent.
Measurements
Descriptive measures included: age (in years), education level (high school graduate, trades or professional certificate, university certificate, or university degree), cognitive status (Montreal Cognitive Assessment [MoCA] – a measure of global cognition used to screen for mild cognitive impairment) [30], weight (in kilograms), and height (in centimeters).
Executive functions
The primary outcome of the parent study was the executive cognitive process of selective attention and conflict resolution as measured by the Stroop Color-Word Test, a measure with high test-retest reliability [22, 31, 32]. The test involved three conditions: the neutral, congruent, and incongruent condition. In the neutral condition (Stroop 1), participants read aloud different color names written in black ink (e.g., “blue”). In the congruent condition (Stroop 2), participants named the ink color of different colored x’s. Finally, in the incongruent condition (Stroop 3), participants named the ink color of incongruent color words (e.g., the word “blue” printed in red ink). Stroop interference, the measure of interest, was calculated by the time difference between the incongruent and congruent conditions (Stroop 3-2). A smaller time difference in Stroop 3-2 is indicative of better selective attention and conflict resolution. This executive function has previously been associated with mobility and shown to be responsive to exercise training [33–35].
Peak muscle power
Single-repetition maximum lift (1-RM) is the gold standard measure of muscle strength and has shown high test-retest reliability across studies [36]. A 15-min standard warm-up on a stationary bike was followed by an initial quadriceps 1-RM assessment using the Keiser air-pressured digital resistance leg press machine (Keiser Sports Health Equipment, Fresno, CA, USA) [22]. The maximum load (in newtons) participants were able to complete was recorded. This measure served as a basis for measuring peak muscle power as participants then completed 3 repetitions (each separated by a 30-s rest) at 6 different relative loads of their 1-RM (40%, 50%, 60%, 70%, 80%, and 90%) on an air-pressured digital resistance leg press machine. These relative loads were chosen to reduce the risk of injury. The concentric portion of the leg press repetition was performed as rapidly as possible, followed by a slow lowering of the load for a period of 3 s. The maximum quadriceps muscle power (in watts) was recorded with larger values indicating stronger muscle performance.
Magnetic resonance imaging (MRI)
Structural MRI data acquisition
T1w and T2w structural MRI scans were obtained using a Philips Achieva 3T scanner located at the UBC MRI Research Centre [22]. Scanning parameters for the T1w scans included a repetition time (TR) of 7.7 ms, an echo time (TE) of 3.6 ms, dimensions of 256×256×100 voxels, and a voxel size of 1.00×1.00×1.00 mm. For the T2 w scans, the TR was 5,431 ms and the TE was 90 ms [23]. T2 w scans had dimensions of 256×256×60 voxels and a voxel size of 0.937×0.937×3.000 mm.
T1w/T2w ratio analysis
The methods used to compute T1w/T2w ratios in this study were based on further refinements to the calibration pipeline as proposed by Nerland et al. [37]. Tools and toolboxes from the FMRIB Software Library (FSL) and Python were used. Steps of the workflow are laid out below.
For each timepoint, each participant’s T2w scan was co-registered to their respective T1w scan via affine transformation using FMRIB’s Linear Image Registration Tool (FLIRT) in FSL Version 6.0.5.1 [38, 39]. Subsequently, Optimized Brain Extraction for Pathological Brains (optiBET) was used to perform brain extraction on the T1w scan [40].
The images underwent a bias field correction to correct for low frequency intensity non-uniformity using the N4ITK algorithm in Python Version 3.10.1 [41]. Bias-corrected images then underwent intensity normalization to improve inter-subject comparability using fuzzy c-means (FCM)-based tissue-based mean normalization [42]. The final T1w/T2w ratio image was computed by dividing the bias-corrected and intensity-normalized T1w image by the bias-corrected and intensity-normalized T2w image.
Selection of regions of interest (ROIs)
Regional T1w/T2w ratios were extracted from a-priori selected regions of interest (ROIs) from each subject’s T1w/T2w images. This was done by first co-registering the T1w/T2w image to the standard John Hopkins University (JHU) space via affine transformation. Subsequently, ROIs were segmented based on the atlas labels from the JHU-MNI-ss (Eve) Atlas [43]. ROIs were selected from a list of brain areas previously identified to be relevant to our behavioral outcomes of interests. The final set of ROIs included the external capsule, internal capsule, corpus callosum, posterior thalamic radiations, superior corona radiata, superior longitudinal fasciculus, and cingulum. The corpus callosum, superior corona radiata and cingulum have all been shown to have roles in executive functions and memory [44–46]. In addition to links to cognition, the external capsule, internal capsule, posterior thalamic radiations, and superior longitudinal fasciculus have also been shown to have roles in motor control and function[45, 47].
White matter hyperintensity (WMH) segmentation
An experienced radiologist identified the WMHs on the MRI of each participant and marked them with digital seeds for subsequent automated processing. Briefly, the seeding procedure involved: 1) marking all distinct WMHs regardless of size; 2) using additional points if more than one point would help define the extent of the lesion; 3) placing at least one point near the center of each lesion [48]. The WMHs were then automatically segmented by computing the extent of each lesion. This method is accurate and robust compared with radiologist segmentations[48].
Intervention
Participants were assigned to one of three groups using a randomization sequence generated by http://www.randomization.com. This sequence was concealed until interventions were assigned and held independently and remotely by the Research Coordinator. Participants were enrolled and randomized by the Research Coordinator to one of: once-weekly resistance training (1x RT), twice-weekly resistance training (2x RT), or twice-weekly balance and tone training (BAT). This occurred after baseline assessments were completed. Both assessors and participants were blinded. For this exploratory analysis, we included only those in the 2x RT and BAT groups who completed MRI as 1x RT did not have an effect on WMH progression [23].
Sessions were scheduled on non-consecutive days. All sessions were 60 min, with each session composed of a 10-min warm-up, 40-min training (RT or BAT), and a 10-min cool-down. All classes were led by certified fitness instructors who received additional training and education from the study investigators.
Resistance training (RT)
The RT program consisted of free weights and Keiser® Pressurized Air system exercises including mini-squats, mini-lunges, biceps curls, calf raises, leg press, hamstring curls, triceps extension, seated row, and latissimus dorsi pull down. The program was high-intensity and progressive with participants starting at 50–60% of their 1-RM before progressing to 2 sets of 6 to 8 repetitions at 75–80% 1-RM by week 4. The 7-RM method was used to subsequently increase the training stimulus, when 2 sets of 6 to 8 repetitions were completed with proper form and without discomfort. The inter-set rest period was 1 min. The range of motion varied by the participant based on their individual comfort level.
Balance and Tone Training (BAT)
The BAT program involved stretching, range-of-motion exercises, basic core-strength exercises, balance exercises, and relaxation techniques. This training has not been shown to elicit improvements in cognition [33]. Thus, it was intended to control for potential confounding factors such as social interaction, secondary changes in lifestyle, and physical training provided by traveling to training centers[22].
Statistical analyses
All statistical analyses were conducted in IBM SPSS Statistics Version 28.0. Visual inspection of the data identified that the WMH volumes were not normally distributed; thus, we log-transformed them prior to our analyses. Analysis of covariance (ANCOVA) was used to compare the two behavioral outcomes, Stroop 3-2 and peak muscle power, between groups at trial completion. For Stroop 3-2, baseline age and education were included as covariates. For peak muscle power, baseline age was included as a covariate.
Between-group differences in regional T1w/T2w ratios at trial completion were compared by multivariate analysis of covariance (MANCOVA). In the model, baseline age, education, WMH volume (log-transformed), and T1w/T2w were included as covariates. Outliers (≥2 standard deviations from the mean) in the change in T1w/T2w for each region were identified. However, removal of these outliers (i.e., 1 for corpus callosum, 2 for external capsule, 2 for posterior thalamic radiations, 1 for superior corona radiata, 1 for superior longitudinal fasciculus, 1 for internal capsule, 1 for cingulum) did not alter the significance of the results and thus these data points were kept in our analyses.
Post-hoc partial correlations were performed for any ROIs identified as having a significant group difference in T1w/T2w at trial completion. These analyses investigated whether a change in T1w/T2w was associated with a change in: 1) Stroop Color-Word Test; and 2) peak muscle power. For Stroop Color-Word Test, we controlled for baseline age, education, and WMH volume (log-transformed). For peak muscle power, we controlled for baseline age and WMH volume (log-transformed). Change scores were calculated by subtracting baseline from trial completion values.
RESULTS
Participants
After removing 5 participants due to excessive head motion, data from 21 participants were included in the MANCOVA analyses (Fig. 1). The baseline mean age of this sample was 69.7 (3.0) years old, with a MoCA score of 25.7 (2.6), and WMH volume of 2187.6 (3767.2) mm3 (Table 1). No significant baseline differences were found between the two groups (using one-way ANOVA analyses). At trial completion, there was a significant between-group difference in peak muscle power (p = 0.036), but not in Stroop Color-Word Test (p = 0.884) (Table 2).
Table 1
Participant characteristics mean (SD) or n (%) (N = 21)
BAT (n = 10) | 2x RT (n = 11) | All (N = 21) | |||||
Baseline | Trial Completion | Baseline | Trial Completion | Baseline | Trial Completion | ||
Age (y) | 69.8 (3.0) | 69.6 (3.2) | 69.7 (3.0) | ||||
Height (cm) | 162.9 (5.7) | 162.2 (5.6) | 159.4 (6.9) | 159.7 (7.3) | 161.0 (6.5) | 160.9 (6.5) | |
Weight (kg) | 71.5 (10.1) | 70.3 (8.2) | 67.1 (13.3) | 67.5 (14.3) | 69.2 (11.8) | 68.8 (11.6) | |
Systolic Blood Pressure (mmHg) | 143.6 (18.2) | 131.1 (18.1)a | 130.5 (25.0) | 133.9 (18.2)a | 136.8 (22.5) | 132.5 (17.7)b | |
Diastolic Blood Pressure (mmHg) | 94.4 (26.2) | 79.0 (11.1)a | 79.5 (10.5) | 73.1 (10.9)a | 86.6 (20.6) | 76.1 (11.1)b | |
Education | High School Graduate | 1 (10.0) | 3 (27.3) | 4 (19.0) | |||
Trades or Professional Certificate | 2 (20.0) | 1 (9.1) | 3 (14.3) | ||||
University Certificate | 1 (10.0) | 1 (9.1) | 2 (9.5) | ||||
University Degree | 4 (40.0) | 6 (54.5) | 10 (47.6) | ||||
MoCA (/30) | 25.2 (2.5) | 25.5 (3.8) | 26.1 (2.8) | 24.8 (3.4) | 25.7 (2.6) | 25.1 (3.5) | |
WMH Volume (mm3) | 2434.3 (4864.9) | 1096.9 (1406.6)a | 1963.4 (2639.4) | 2077.8 (2884.9) | 2187.6 (3767.2) | 1636.4 (2337.6)c |
BAT, balance and tone training; 2x RT, twice-weekly resistance training; MoCA, Montreal Cognitive Assessment; WMH, white matter hyperintensity. an = 9 due to missing values at trial completion. bn = 18 due to missing values at trial completion. cn = 20 due to missing values at trial completion.
Table 2
Mean (SD) of behavioral outcome measures in the analyzed sample at baseline and trial completion (N = 15)
BAT (n = 6) | 2x RT (n = 9) | ||||
Baseline | Trial Completion | Baseline | Trial Completion | ||
Stroop 3-2 (s) | Part 1 | 37.4 (9.6) | 39.1 (11.2) | 36.9 (8.4) | 38.1 (7.5) |
Part 2 | 51.5 (8.3) | 55.1 (9.5) | 52.7 (12.0) | 51.7 (10.4) | |
Part 3 | 98.2 (11.8) | 97.6 (14.3) | 100.5 (20.8) | 97.6 (17.9) | |
Part 3-2 | 46.7 (12.0) | 42.5 (12.4) | 47.8 (17.4) | 45.9 (15.3) | |
Peak Muscle Power (W) | 684.0 (223.1) | 561.7 (155.9) | 615.0 (192.2) | 700.7 (186.3)* |
BAT, balance and tone training; 2x RT, twice-weekly resistance training. *Significantly different from the BAT group at trial completion (p < 0.05); controlled for baseline age and peak muscle power.
Effect of RT on regional T1w/T2w ratio
At trial completion, there were significant between-group differences in the external capsule T1w/T2w ratio (F = 6.173; p = 0.024) and the posterior thalamic radiations T1w/T2w ratio (F = 7.869; p = 0.013) (Figs. 2 and 3). Specifically, the external capsule T1w/T2w was increased in the 2x RT group (mean change in T1w/T2w = 0.02, standard deviation (SD) = 0.08) while it decreased in the BAT group (mean change = –0.06, SD = 0.11). In the posterior thalamic radiations, there was a significantly greater increase in T1w/T2w in the 2x RT group (mean change = 0.08, SD = 0.07) compared to the BAT group (mean change = 0.02, SD = 0.08) (Table 3). No other significant between-group differences were observed.
Fig. 2
Between-group difference in change in T1w/T2w in the external capsule. Circles correspond to each participant. BAT, balance and tone training; 2x RT, twice-weekly resistance training; T1w/T2w, T1-weighted over T2-weighted ratio.
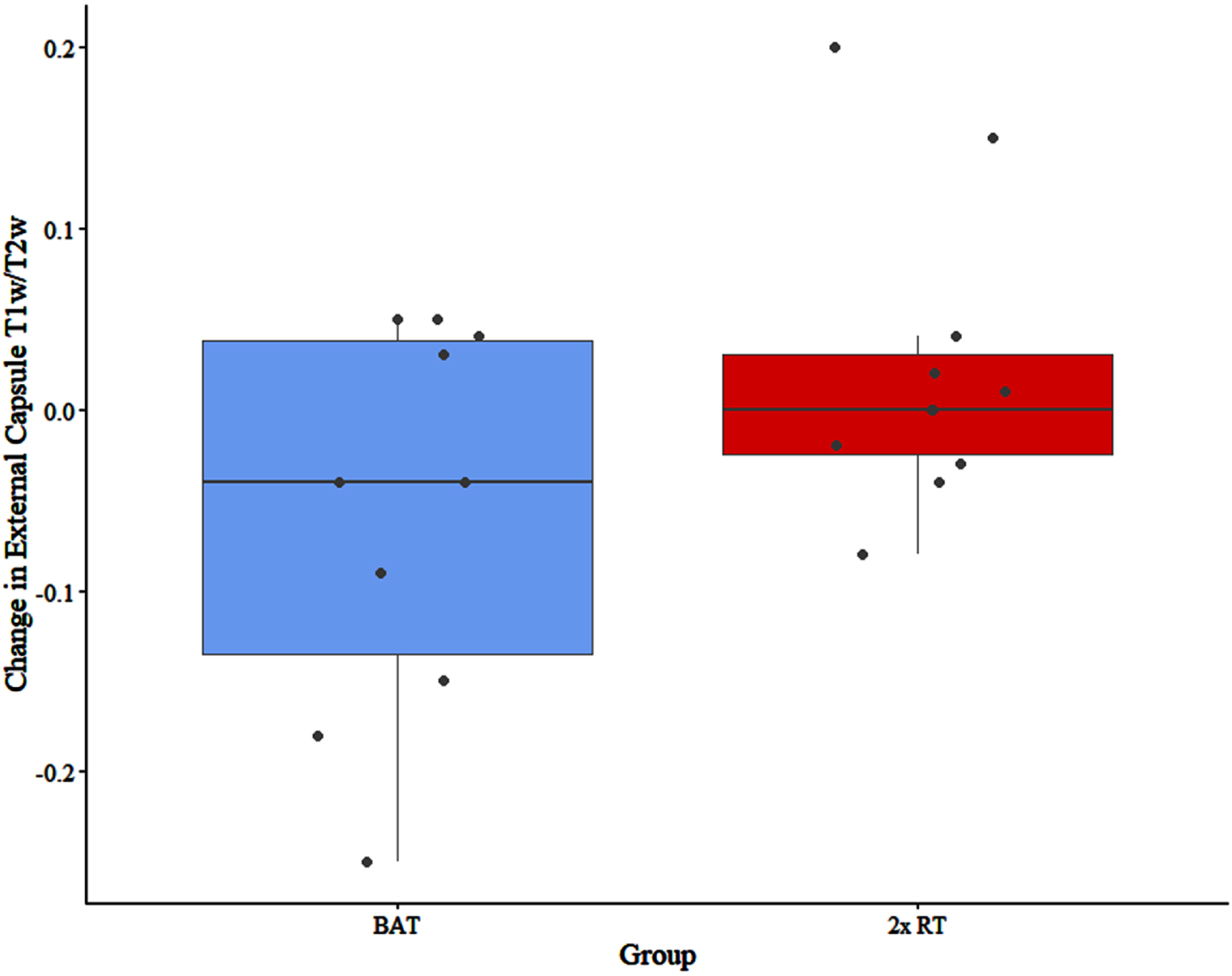
Fig. 3
Between-group difference in change in T1w/T2w in the posterior thalamic radiations. Circles correspond to each participant. BAT, balance and tone training; 2x RT, twice-weekly resistance training; T1w/T2w, T1-weighted over T2-weighted ratio.
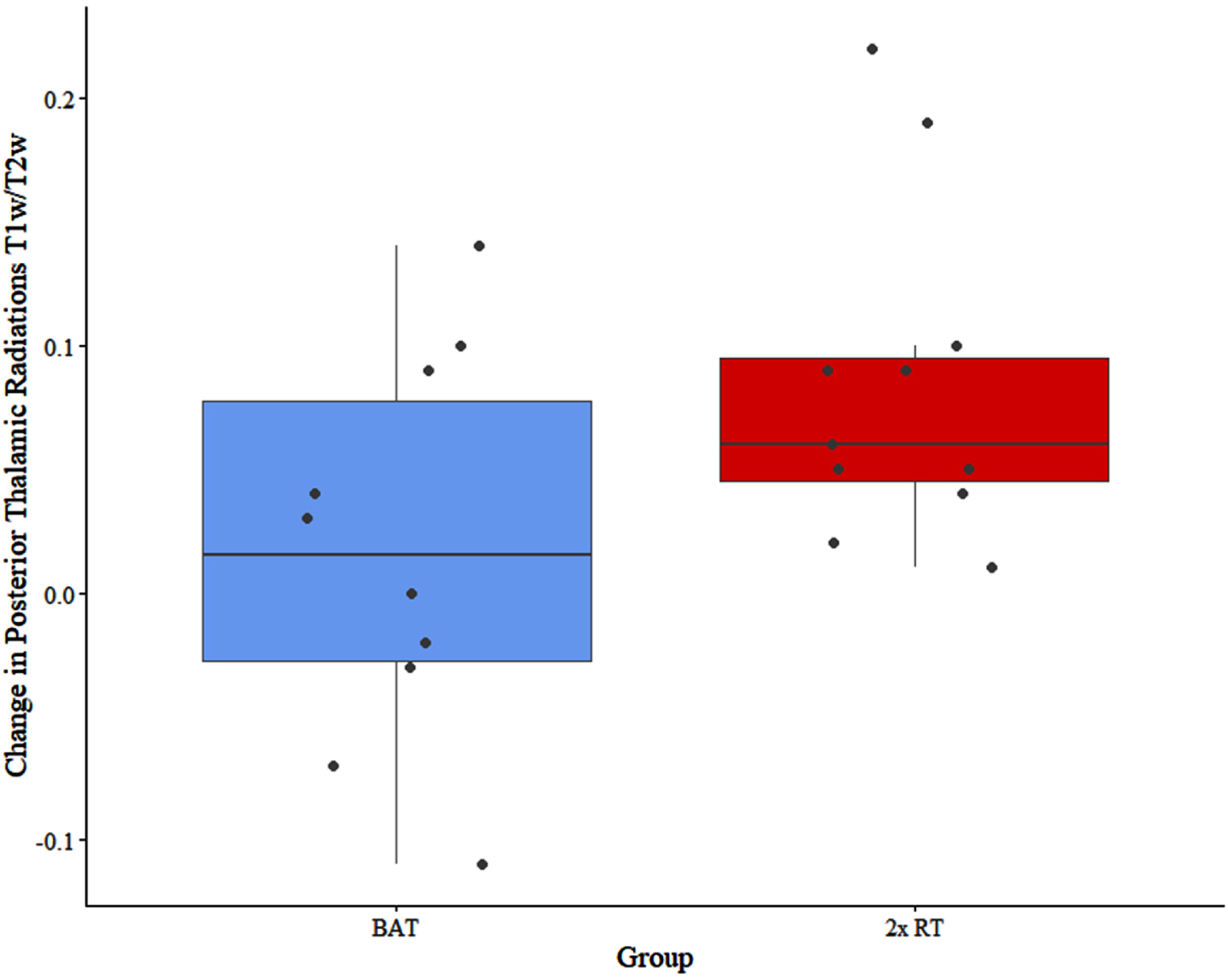
Table 3
Mean (SD) of regional T1w/T2w (N = 21)
BAT (n = 10) | 2x RT (n = 11) | |||
Baseline | Trial Completion | Baseline | Trial Completion | |
External Capsule | 2.42 (0.20) | 2.36 (0.26) | 2.26 (0.23) | 2.28 (0.26)* |
Internal Capsule | 2.74 (0.22) | 2.72 (0.20) | 2.58 (0.22) | 2.58 (0.22) |
Corpus Callosum | 2.43 (0.11) | 2.50 (0.18) | 2.46 (0.19) | 2.53 (0.18) |
Posterior Thalamic Radiations | 2.35 (0.19) | 2.36 (0.19) | 2.32 (0.20) | 2.40 (0.24)* |
Superior Corona Radiata | 2.48 (0.17) | 2.49 (0.20) | 2.40 (0.22) | 2.45 (0.23) |
Superior Longitudinal Fasciculus | 2.44 (0.16) | 2.43 (0.19) | 2.39 (0.24) | 2.43 (0.25) |
Cingulum | 2.29 (0.13) | 2.28 (0.17) | 2.28 (0.20) | 2.30 (0.21) |
T1w/T2w: T1-weighted over T2-weighted ratio; BAT: balance and tone training; 2x RT: twice-weekly resistance training. *Significantly different from the BAT group at trial completion (p < 0.05); controlled for baseline age, education, WMH volume (log-transformed), and T1w/T2w.
Partial correlations between change in T1w/T2w ratio and behavioral performance
Peak muscle power was only measured in a subset of participants who did not have any pre-existing knee, hip, or back conditions [22]. Thus, there was an analyzed sample of 15 participants (BAT: n = 6; 2x RT: n = 9) for the partial correlation analyses. In the 2x RT group, change in external capsule T1w/T2w was positively and significantly associated with an increase in peak muscle power (r = 0.771; p = 0.042). This association was not present for the BAT group (r = –0.014; p = 0.986) (Fig. 4). No other significant associations were found (Table 4).
Fig. 4
Correlation between changes in T1w/T2w in the external capsule and changes in peak muscle power. BAT, balance and tone training; 2x RT, twice-weekly resistance training; T1w/T2w, T1-weighted over T2-weighted ratio.
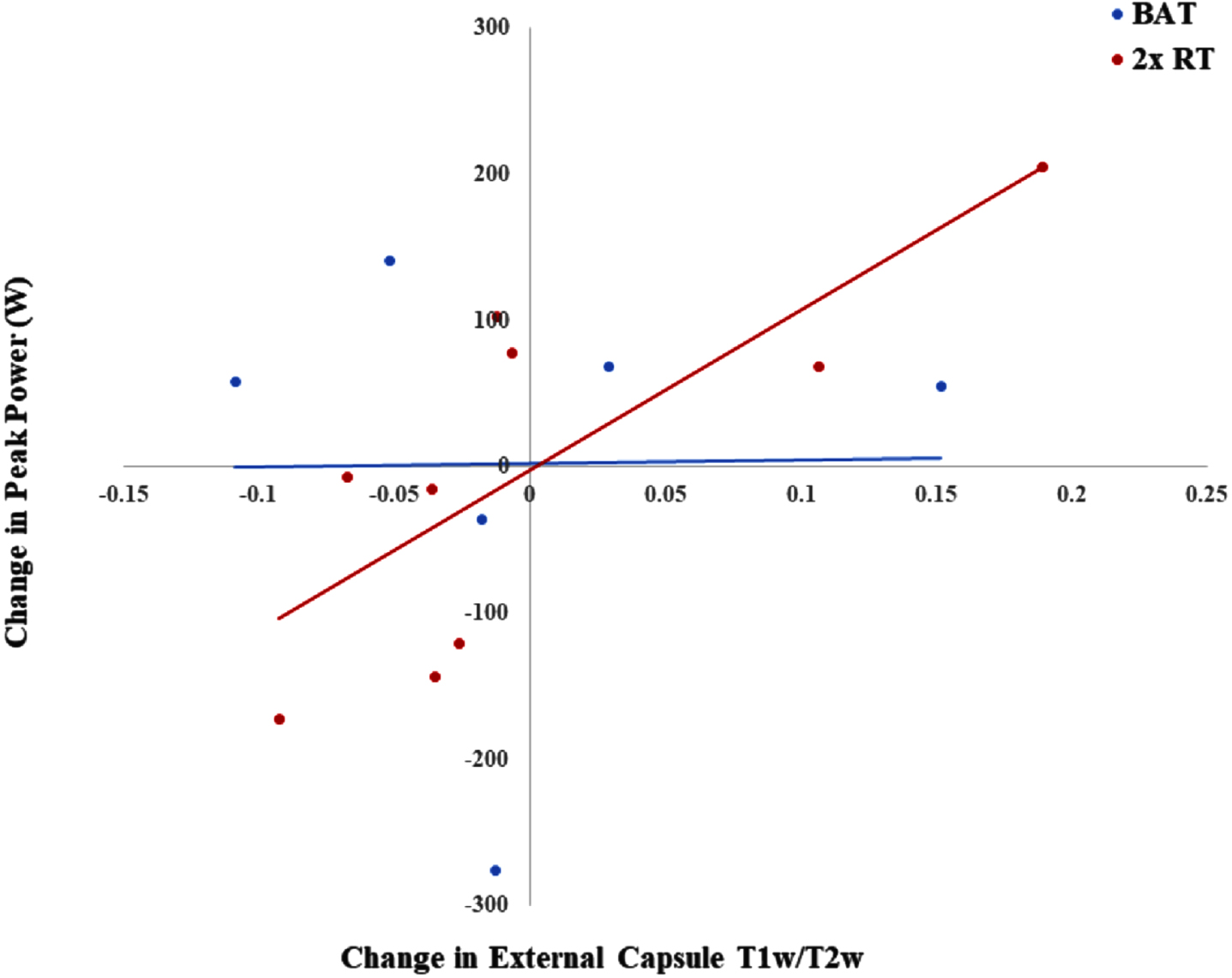
Table 4
Partial correlations between change in regional T1w/T2w and change in cognition and muscle strength in the 2x RT group (n = 9)
External | Posterior Thalamic | ||
Capsule | Radiations | ||
Stroop 3-2 | r | 0.654 | 0.681 |
p | 0.159 | 0.136 | |
Peak Muscle Power | r | 0.771 | 0.591 |
p | 0.042* | 0.162 |
T1w/T2w: T1-weighted over T2-weighted ratio; 2x RT: twice-weekly resistance training. Controlled for baseline age and WMH volume (log-transformed). *p < 0.05.
DISCUSSION
This study is the first to explore the effect of 52 weeks of twice-weekly RT on T1w/T2w ratios in older females. Compared with the active control group, we found that twice-weekly RT increased the T1w/T2w ratio in the external capsule and posterior thalamic radiations. Increased peak muscle power due to 2x RT was also significantly associated with the slight increase of T1w/T2w in the external capsule.
Our results concur and extend prior findings that show higher self-reported physical activity is positively associated with white matter integrity (as measured by diffusion tensor imaging) in the external capsule [49]. The external capsule is a passageway for a multitude of fibers from cortical regions (e.g., ventral and medial prefrontal cortex, ventral premotor cortex, inferotemporal region) to the striatum [45]. Thus, it facilitates the basal ganglia in various motor, cognitive, and affective functions. The posterior thalamic radiations are another important passageway that connect the cortex, basal ganglia, and thalamus [47, 50]. Both structures show reduced white matter integrity (as measured by myelin water fraction and measures derived from diffusion-weighted imaging) with age [51, 52]. Moreover, in one study characterizing WMH development in major white matter tracts, the posterior thalamic radiations was identified as one of the tracts most susceptible to WMH progression [53]. Given both the external capsule and posterior thalamic radiations are sensitive to aging effects, they are potential targets for interventions.
A previous systematic review has shown that links between physical and cognitive function may have a physiological correlate in positive associations between muscle size and both whole brain and white matter volume [54]. Evidence in support of muscle-brain crosstalk suggests exercise stimulates peripheral factors that cross the blood-brain barrier (BBB) and increases neuronal gene expression for protective factors [55]. These include brain-derived neurotrophic factor (BDNF), insulin like growth factor-1 (IGF-1), and vascular endothelial growth factor (VEGF); these factors may be involved in slowing or reversing pathological white matter changes associated with WMHs and consequently improve cognitive outcomes through this and other mechanisms of neuroplasticity [56, 57]. In particular, once IGF-1 crosses the BBB, it is involved in neuroplastic changes including synaptic processes such as long-term potentiation, angiogenesis, axonal growth, dendritic maturation, and synaptogenesis [19]. These roles may explain the protective role IGF-1 has been found to have in previous rodent studies. Transgenic mice with increased IGF-1 expression have more abundant and thicker myelin [58]. Additionally, rodents with hypoxic-ischemic brain injury showed an uptake of centrally administered IGF-1 by subcortical white matter tracts including the external capsule [59]. Lin and colleagues [60] also found IGF-1 can reduce the amount of white matter degeneration in the external capsule following hypoxic exposure in rodents. Collectively, this suggests the external capsule may be one region that is more receptive to the effects of IGF-1 on white matter integrity. Considering prior RCTs show RT increases IGF-1 levels and that this increase is associated with RT-induced improvements in cognitive and functional outcomes [61–63], IGF-1 promotion by RT may preferentially protect white matter within the external capsule. However, as our study did not collect IGF-1, future randomized controlled trials of RT with both neuroimaging and IGF-1 as outcomes are needed to confirm this hypothesis. Concurrently, a population-dependent investigation of other potential underlying molecular mechanisms, related cerebral changes, and influencing behavioral mechanisms (such as sleep) is needed to fully characterize the impact of RT on white matter integrity. For example, the upregulation of VEGF and endothelial nitric oxide synthase could decrease oxidative stress; promoting cerebrovascular function, reducing WMH progression, and improving downstream cognitive outcomes [20].
While the exact functions of the external capsule have yet to be elucidated, lesions in this region cause muscle spasticity [64–67]. Our finding that RT-induced improvements in external capsule white matter integrity was associated with increased peak muscle power further supports the notion that the external capsule has an important role in motor control. Peak muscle power, defined as the maximum force generated by a muscle over a specific period of time, is more predictive of functional status and functional dependency than aerobic capacity and muscle strength, defined as the maximum force generated by a muscle in a single contraction [68].
Contrary to our hypothesis, there was no significant association between changes in T1w/T2w ratios and change in executive functions, as measured by the Stroop Color-Word Test. The literature on the external capsule’s role in cognitive function is limited. White matter hyperintensities in the external capsule were previously associated with attention dysfunction [69], suggesting a potential role of this region in focused and sustained attention. Since the Stroop Color-Word Test measures selective attention, the external capsule may be more relevant for cognitive domains that were not assessed in this specific study.
There is a limited understanding of the function of the posterior thalamic radiations. Previous evidence has identified associations between the posterior thalamic radiations and visual short-term memory, processing speed, and higher motor functions such as understanding intention behind movements [53, 70, 71]. This suggests the posterior thalamic radiations may be more relevant for cognitive and physical functions that were not assessed in this study, thus explaining the lack of an association between change in the T1w/T2w ratio of the posterior thalamic radiations and changes in Stroop Color-Word Test or peak muscle power.
This study is not without its limitations. Our primary measure, the T1w/T2w ratio, is an indirect measure of white matter integrity. As a ratio of arbitrary values of intensities, it is prone to influence by extraneous factors such as tissue characteristics and artifacts. Our pipeline attempted to account for this by improving inter-subject comparability through methods such as bias-correction and intensity normalization. While originally proposed to be a measure of myelin, the T1w/T2w ratio has a low correlation with other measures of myelin (including myelin water fraction— the gold standard measure) [72, 73]. However, it has been correlated with other aspects of white matter including neurite density [28], axonal diameter [74], and iron content [75]. Other merits of the ratio include its accessibility due to its use of commonly acquired T1w and T2w scans, as well as its sensitivity and reliability in regions of more complex microarchitecture due to a lack of influence to fiber orientation [73]. Thus, the use of T1w/T2w is suitable and valuable for an exploratory study like this one.
Due to our small sample size and exploratory nature of the study, one methodological limitation is that we were unable to perform repeated measures or robust correlations. Future studies with larger samples are needed to confirm current results. In addition, the study may not have enough power to reveal potential significant differences in white matter integrity in other regions. Indeed, previous studies have shown associations between physical activity and white matter in the other five ROIs considered in this study [49, 76, 77]. However, it is important to consider that these associations may also be more applicable to changes induced by aerobic training, another form of exercise that is more extensively studied and proposed to act through different underlying neurobiological mechanisms [78]. Secondly, we used a supervised intervention in comparison to self-reported physical activity which could have led to different results. Another possible underlying reason for diverging results is varying covariate control. We did not control for blood pressure in our analyses as we found no significant between-group differences. However, it should be noted that blood pressure is a well-established contributing factor to WMH progression. Previous studies have shown associations between increases in either systolic blood pressure or diastolic blood pressure and increases in WMH volume; as well as proportional decreases in WMH volume following blood pressure control [79, 80]. Thus, even if the between-group difference in blood pressure was not significant, lower baseline systolic and diastolic blood pressure may contribute to differences in white matter integrity. Another limitation resulting from our sample is limited generalizability. Our sample only consisted of females without cognitive impairment who lived independently, thus our results cannot be generalized beyond this population. Despite these limitations, this is the first study (to our knowledge) investigating changes in T1w/T2w induced by RT. As such, it provides insights for future studies.
In conclusion, this study demonstrates the benefits of RT against CSVD and age-related functional decline by exploring its effects on white mattery integrity. Based on our findings, RT may promote white matter integrity in a key region associated with improvements in motor function. Future studies with larger samples should attempt to further elucidate the effects of RT on specific white matter substrates and the associated improvements in cognitive and motor outcomes. Building this body of knowledge will further validate the use of RT as an accessible lifestyle intervention for counteracting maladaptive changes that occur with aging. Our findings support the use of RT to promote muscle-brain crosstalk that contributes to beneficial neurological and functional outcomes.
ACKNOWLEDGMENTS
We thank the participants of the original Brain Power study.
FUNDING
Funding was provided by the Vancouver Foundation (BCM06– 0035), the Michael Smith Foundation for Health Research (MSFHR; CI-SCH-063 [05–0035]), and the Jack Brown and Family Alzheimer Research Foundation Society to TL-A. ED is a Michael Smith Foundation of Health Research Post-doctoral Fellow and TL-A is a Canada Research Chair (Tier I) in Healthy Aging.
CONFLICT OF INTEREST
The authors have no conflict of interest to report.
DATA AVAILABILITY
The data supporting the findings of this study are available on request from the corresponding author. The data are not publicly available due to privacy or ethical restrictions.
REFERENCES
[1] | de Leeuw FE , de Groot JC , Achten E , Oudkerk M , Ramos LM , Heijboer R , Hofman A , Jolles J , van Gijn J , Breteler MM ((2001) ) Prevalence of cerebral white matter lesions in elderly people: A population based magnetic resonance imaging study. The Rotterdam Scan Study. J Neurol Neurosurg Psychiatry 70: , 9–14. |
[2] | Prins ND , van Dijk EJ , den Heijer T , Vermeer SE , Koudstaal PJ , Oudkerk M , Hofman A , Breteler MMB ((2004) ) Cerebral white matter lesions and the risk of dementia. Arch Neurol 61: , 1531–1534. |
[3] | Wardlaw JM , Valdés Hernández MC , Muñoz-Maniega S ((2015) ) What are white matter hyperintensities madeof? J Am Heart Assoc 4: , e001140. |
[4] | Prins ND , Scheltens P ((2015) ) White matter hyperintensities, cognitive impairment and dementia: An update. Nat Rev Neurol 11: , 157–165. |
[5] | Smith EE , Saposnik G , Biessels GJ , Doubal FN , Fornage M , Gorelick PB , Greenberg SM , Higashida RT , Kasner SE , Seshadri S , American Heart Association Stroke Council; Council on Cardiovascular Radiology and Intervention; Council on Functional Genomics and Translational Biology; and Council on Hypertension ((2017) ) Prevention of stroke in patients with silent cerebrovascular disease: A scientific statement for healthcare professionals from the American Heart Association/American Stroke Association. Stroke 48: , e44–e71. |
[6] | Zheng JJJ , Delbaere K , Close JCT , Sachdev PS , Lord SR ((2011) ) Impact of white matter lesions on physical functioning and fall risk in older people. Stroke 42: , 2086–2090. |
[7] | Saczynski JS , Jonsdottir MK , Sigurdsson S , Eiriksdottir G , Jonsson PV , Garcia ME , Kjartansson O , van Buchem MA , Gudnason V , Launer LJ ((2008) ) White matter lesions and cognitive performance: The role of cognitively complex leisure activity. J Gerontol A Biol Sci Med Sci 63: , 848–854. |
[8] | Gow AJ , Bastin ME , Muñoz Maniega S , Valdés Hernández MC , Morris Z , Murray C , Royle NA , Starr JM , Deary IJ , Wardlaw JM ((2012) ) Neuroprotective lifestyles and the aging brain: Activity, atrohy, and white matter integrity. Neurology 79: , 1802–1808. |
[9] | Burzynska AZ , Chaddock-Heyman L , Voss MW , Wong CN , Gothe NP , Olson EA , Knecht A , Lewis A , Monti JM , Cooke GE , Wojcicki TR , Fanning J , Chung HD , Awick E , McAuley E , Kramer AF ((2014) ) Physical activity and cardiorespiratory fitness are beneficial for white matter in low-fit older adults. PLoS One 9: , e107413. |
[10] | Torres ER , Strack EF , Fernandez CE , Tumey TA , Hitchcock ME ((2015) ) Physical activity and white matter hyperintensities: A systematic review of quantitative studies. Prev Med Rep 2: , 319–325. |
[11] | Hortobágyi T , Vetrovsky T , Balbim GM , Sorte Silva NCB , Manca A , Deriu F , Kolmos M , Kruuse C , Liu-Ambrose T , Radák Z , Váczi M , Johansson H , dos Santos PCR , Franzén E , Granacher U ((2022) ) The impact of aerobicand resistance training intensity on markers of neuroplasticity in health and disease. Ageing Res Rev 80: , 101698. |
[12] | Tseng BY , Gundapuneedi T , Khan MA , Diaz-Arrastia R , Levine BD , Lu H , Huang H , Zhang R ((2013) ) White matter integrity in physically fit older adults. Neuroimage 82: , 510–516. |
[13] | Burzynska AZ , Jiao Y , Knecht AM , Fanning J , Awick EA , Chen T , Gothe N , Voss MW , McAuley E , Kramer AF ((2017) ) White matter integrity declined over 6-months, but dance intervention improved integrity of the fornix of older adults. Front Aging Neurosci 9: , 59. |
[14] | Voss MW , Heo S , Prakash RS , Erickson KI , Alves H , Chaddock L , Szabo AN , Mailey EL , Wójcicki TR , White SM , Gothe N , McAuley E , Sutton BP , Kramer AF ((2013) ) The influence of aerobic fitness on cerebral white matter integrity and cognitive function in older adults: Results of a one-year exercise intervention. Hum Brain Mapp 34: , 2972–2985. |
[15] | Svatkova A , Mandl RCW , Scheewe TW , Cahn W , Kahn RS , Hulshoff Pol HE ((2015) ) Physical exercise keeps the brain connected: Biking increases white matter integrity in patients with schizophrenia and healthy controls. Schizophr Bull 41: , 869–878. |
[16] | Mendez Colmenares A , Voss MW , Fanning J , Salerno EA , Gothe NP , Thomas ML , McAuley E , Kramer AF , Burzynska AZ ((2021) ) White matter plasticity in healthy older adults: The effects of aerobic exercise. Neuroimage 239: , 118305. |
[17] | Sexton CE , Betts JF , Dennis A , Doherty A , Leeson P , Holloway C , Dall’Armellina E , Winkler AM , Demnitz N , Wassenaar T , Dawes H , Johansen-Berg H ((2020) ) The effects of an aerobic training intervention on cognition, grey matter volumes and white matter microstructure. Physiol Behav 223: , 112923. |
[18] | Dao E , Barha CK , Best JR , Hsiung G-Y , Tam R , Liu-Ambrose T ((2019) ) The effect of aerobic exercise on white matter hyperintensity progression may vary by sex. Can J Aging 38: , 236–244. |
[19] | Herold F , Törpel A , Schega L , Müller NG ((2019) ) Functional and/or structural brain changes in response to resistance exercises and resistance training lead to cognitive improvements: A systematic review. Eur Rev Aging Phys Act 16: , 10. |
[20] | Bliss ES , Wong RH , Howe PR , Mills DE ((2021) ) Benefits of exercise training on cerebrovascular and cognitive function in ageing. J Cereb Blood Flow Metab 41: , 447–470. |
[21] | Landrigan J-F , Bell T , Crowe M , Clay OJ , Mirman D ((2020) ) Lifting cognition: A meta-analysis of effects of resistance exercise on cognition. Psychol Res 84: , 1167–1183. |
[22] | Liu-Ambrose T , Nagamatsu LS , Graf P , Beattie BL , Ashe MC , Handy TC ((2010) ) Resistance training and executive functions: A 12-month randomized controlled trial. Arch Intern Med 170: , 170–178. |
[23] | Bolandzadeh N , Tam R , Handy TC , Nagamatsu LS , Hsu CL , Davis JC , Dao E , Beattie BL , Liu-Ambrose T ((2015) ) Resistance training and white matter lesion progression in older women: Exploratory analysis of a 12-month randomized controlled trial. J Am Geriatr Soc 63: , 2052–2060. |
[24] | Suo C , Singh MF , Gates N , Wen W , Sachdev P , Brodaty H , Saigal N , Wilson GC , Meiklejohn J , Singh N , Baune BT , Baker M , Foroughi N , Wang Y , Mavros Y , Lampit A , Leung I , Valenzuela MJ ((2016) ) Therapeutically relevant structural and functional mechanisms triggered by physical and cognitive exercise. Mol Psychiatry 21: , 1633–1642. |
[25] | Ganzetti M , Wenderoth N , Mantini D ((2014) ) Whole brain myelin mapping using T1- and T2-weighted MR imaging data. Front Hum Neurosci 8: , 671. |
[26] | Paus T , Collins DL , Evans AC , Leonard G , Pike B , Zijdenbos A ((2001) ) Maturation of white matter in the human brain: A review of magnetic resonance studies. Brain Res Bull 54: , 255–266. |
[27] | Glasser MF , Van Essen DC ((2011) ) Mapping human cortical areas in vivo based on myelin content as revealed by T1- and T2-weighted MRI. J Neurosci 31: , 11597–11616. |
[28] | Preziosa P , Bouman PM , Kiljan S , Steenwijk MD , Meani A , Pouwels PJ , Rocca MA , Filippi M , Geurts JJG , Jonkman LE ((2021) ) Neurite density explains cortical T1-weighted/T2-weighted ratio in multiple sclerosis. J Neurol Neurosurg Psychiatry 92: , 790–792. |
[29] | Colcombe S , Kramer AF ((2003) ) Fitness effects on the cognitive function of older adults: A meta-analytic study. Psychol Sci 14: , 125–130. |
[30] | Nasreddine ZS , Phillips NA , Bédirian V , Charbonneau S , Whitehead V , Collin I , Cummings JL , Chertkow H ((2005) ) The Montreal Cognitive Assessment, MoCA: A brief screening tool for mild cognitive impairment. J Am GeriatrSoc 53: , 695–699. |
[31] | Graf P , Uttl B , Tuokko H ((1995) ) Color- and picture-word Stroop tests: Performance changes in old age. J Clin Exp Neuropsychol 17: , 390–415. |
[32] | Golden C , Freshwater S ((2002) ) The Stroop Color and Word Test: A Manual for Clinical and Experimental Uses, Stoelting Company, Chicago, IL. |
[33] | Colcombe SJ , Kramer AF , Erickson KI , Scalf P , McAuley E , Cohen NJ , Webb A , Jerome GJ , Marquez DX , Elavsky S ((2004) ) Cardiovascular fitness, cortical plasticity, and aging. Proc Natl Acad Sci U S A 101: , 3316–3321. |
[34] | Liu-Ambrose T , Donaldson MG , Ahamed Y , Graf P , Cook WL , Close J , Lord SR , Khan KM ((2008) ) Otago home-based strength and balance retraining improves executive functioning in older fallers: A randomized controlled trial. J Am Geriatr Soc 56: , 1821–1830. |
[35] | Liu-Ambrose T , Pang MYC , Eng JJ ((2007) ) Executive function is independently associated with performances of balance and mobility in community-dwelling older adults after mild stroke: Implications for falls prevention. Cerebrovasc Dis 23: , 203–210. |
[36] | Grgic J , Lazinica B , Schoenfeld BJ , Pedisic Z ((2020) ) Test-retest reliability of the one-repetition maximum (1RM) strength assessment: A systematic review. Sports Med Open 6: , 31. |
[37] | Nerland S , Jørgensen KN , Nordhøy W , Maximov II , Bugge RAB , Westlye LT , Andreassen OA , Geier OM , Agartz I ((2021) ) Multisite reproducibility and test-retest reliability of the T1w/T2w-ratio: A comparison of processing methods. Neuroimage 245: , 118709. |
[38] | Jenkinson M , Bannister P , Brady M , Smith S ((2002) ) Improved optimization for the robust and accurate linear registration and motion correction of brain images. Neuroimage 17: , 825–841. |
[39] | Jenkinson M , Smith S ((2001) ) A global optimisation method for robust affine registration of brain images. Med Image Anal 5: , 143–156. |
[40] | Lutkenhoff ES , Rosenberg M , Chiang J , Zhang K , Pickard JD , Owen AM , Monti MM ((2014) ) Optimized Brain Extraction for Pathological Brains (optiBET), PLoS One 9: , e115551. |
[41] | Tustison NJ , Avants BB , Cook PA , Zheng Y , Egan A , Yushkevich PA , Gee JC ((2010) ) N4ITK: Improved N3 biascorrection. IEEE Trans Med Imaging 29: , 1310–1320. |
[42] | Reinhold JC , Dewey BE , Carass A , Prince JL ((2018) ) Evaluating the impact of intensity normalization on MR image synthesis, Proc SPIE 10949: , 109493H. |
[43] | Oishi K, Faria AV, Mori S (2010) JHU-MNI-ss atlas, Johns Hopkins University School of Medicine, Department of Radiology, Center for Brain Imaging Science, https://neuroconductor.org/help/EveTemplate. |
[44] | Birdsill AC , Koscik RL , Jonaitis EM , Johnson SC , Okonkwo OC , Hermann BP , LaRue A , Sager MA , Bendlin BB ((2014) ) Regional white matter hyperintensities: Aging, Alzheimer’s disease risk, and cognitive function. Neurobiol Aging 35: , 769–776. |
[45] | Schmahmann JD , Smith EE , Eichler FS , Filley CM ((2008) ) Cerebral white matter. Ann N Y Acad Sci 1142: , 266–309. |
[46] | Stave EA , De Bellis MD , Hooper SR , Woolley DP , Chang SK , Chen SD ((2017) ) Dimensions of attention associated with the microstructure of corona radiata white matter. J Child Neurol 32: , 458–466. |
[47] | Chaddock-Heyman L , Erickson KI , Voss MW , Powers JP , Knecht AM , Pontifex MB , Drollette ES , Moore RD , Raine LB , Scudder MR , Hillman CH , Kramer AF ((2013) ) White matter microstructure is associated with cognitive control in children. Biol Psychol 94: , 109–115. |
[48] | McAusland J , Tam RC , Wong E , Riddehough A , Li DKB ((2010) ) Optimizing the use of radiologist seed points for improved multiple sclerosis lesion segmentation. IEEE Trans Biomed Eng 57: , 2689–2698. |
[49] | Strömmer JM , Davis SW , Henson RN , Tyler LK , Cam-CAN , Campbell KL ((2020) ) Physical activity predicts population-level age-related differences in frontal white matter. J Gerontol A Biol Sci Med Sci 75: , 236–243. |
[50] | George K , Das JM ((2022) ) Neuroanatomy, thalamocortical radiations, StatPearls. |
[51] | Dvorak AV , Swift-LaPointe T , Vavasour IM , Lee LE , Abel S , Russell-Schulz B , Graf C , Wurl A , Liu H , Laule C , Li DKB , Traboulsee A , Tam R , Boyd LA , MacKay AL , Kolind SH ((2021) ) An atlas for human brain myelin content throughout the adult life span. Sci Rep 11: , 269. |
[52] | Marstaller L , Williams M , Rich A , Savage G , Burianová H ((2015) ) Aging and large-scale functional networks: White matter integrity, gray matter volume, and functional connectivity in the resting state. Neuroscience 290: , 369–378. |
[53] | Seiler S , Fletcher E , Hassan-Ali K , Weinstein M , Beiser A , Himali JJ , Satizabal CL , Seshadri S , DeCarli C , Maillard P ((2018) ) Cerebral tract integrity relates to white matter hyperintensities, cortex volume, and cognition. Neurobiol Aging 72: , 14–22. |
[54] | Kilgour AHM , Todd OM , Starr JM ((2014) ) A systematic review of the evidence that brain structure is related to muscle structure and their relationship to brain and muscle function in humans over the lifecourse. BMC Geriatr 14: , 85. |
[55] | Pedersen BK ((2019) ) Physical activity and muscle– brain crosstalk. Nat Rev Endocrinol 15: , 383–392. |
[56] | Cotman CW , Berchtold NC , Christie L-A ((2007) ) Exercise builds brain health: Key roles of growth factor cascades and inflammation. Trends Neurosci 30: , 464–472. |
[57] | Marston KJ , Brown BM , Rainey-Smith SR , Peiffer JJ ((2019) ) Resistance exercise-induced responses in physiological factors linked with cognitive health. J Alzheimers Dis 68: , 39–64. |
[58] | Ye P , Carson J , D’Ercole AJ ((1995) ) in vivo actions ofinsulin-like growth factor-I (IGF-I) on brain myelination: Studiesof IGF-I and IGF binding protein-1 (IGFBP-1) transgenic mice. JNeurosci 15: , 7344–7356. |
[59] | Guan J , Beilharz EJ , Skinner SJM , Williams CE , Gluckman PD ((2000) ) Intracerebral transportation and cellular localisation of insulin-like growth factor-1 following central administration to rats with hypoxic– ischemic brain injury. Brain Res 853: , 163–173. |
[60] | Lin S , Fan L-W , Pang Y , Rhodes PG , Mitchell HJ , Cai Z ((2005) ) IGF-1 protects oligodendrocyte progenitor cells and improves neurological functions following cerebral hypoxia– ischemia in the neonatal rat. Brain Res 1063: , 15–26. |
[61] | Cassilhas RC , Viana VAR , Grassmann V , Santos RT , Santos RF , Tufik S , Mello MT ((2007) ) The impact of resistance exercise on the cognitive function of the elderly. Med Sci Sports Exerc 39: , 1401–1407. |
[62] | Jiang Q , Lou K , Hou L , Lu Y , Sun L , Tan SC , Low TY , Kord-Varkaneh H , Pang S ((2020) ) The effect of resistance training on serum insulin-like growth factor 1(IGF-1): A systematic review and meta-analysis. Complement Ther Med 50: , 102360. |
[63] | Tsai C-L , Wang C-H , Pan C-Y , Chen F-C ((2015) ) The effects of long-term resistance exercise on the relationshipbetween neurocognitive performance and GH, IGF-1, and homocysteine levels in the elderly. Front BehavNeurosci 9: , 23. |
[64] | Barlow SJ ((2016) ) Identifying the brain regions associated with acute spasticity in patients diagnosed with an ischemic stroke. Somatosens Mot Res 33: , 104–111. |
[65] | Cheung DK , Climans SA , Black SE , Gao F , Szilagyi GM , Mochizuki G ((2016) ) Lesion characteristics of individuals with upper limb spasticity after stroke. Neurorehabil Neural Repair 30: , 63–70. |
[66] | Lee KB , Hong BY , Kim JS , Sul B , Yoon SC , Ji E-K , Son DB , Hwang BY , Lim SH ((2019) ) Which brain lesions produce spasticity? An observational study on 45 stroke patients, PLoS One 14: , e0210038. |
[67] | Picelli A , Tamburin S , Gajofatto F , Zanette G , Praitano M , Saltuari L , Corradini C , Smania N ((2014) ) Association between severe upper limb spasticity and brain lesion location in stroke patients. Biomed Res Int 2014: , e162754. |
[68] | Foldvari M , Clark M , Laviolette LC , Bernstein MA , Kaliton D , Castaneda C , Pu CT , Hausdorff JM , Fielding RA , Singh MA ((2000) ) Association of muscle power with functional status in community-dwelling elderly women. J Gerontol A Biol Sci Med Sci 55: , M192–199. |
[69] | O’brien JT , Wiseman R , Burton EJ , Barber B , Wesnes K , Saxby B , Ford GA ((2002) ) Cognitive associations of subcortical white matter lesions in older people. Ann N Y Acad Sci 977: , 436–444. |
[70] | Menegaux A , Meng C , Neitzel J , Bäuml JG , Müller HJ , Bartmann P , Wolke D , Wohlschläger AM , Finke K , Sorg C ((2017) ) Impaired visual short-term memory capacity is distinctively associated with structural connectivity of the posterior thalamic radiation and the splenium of the corpus callosum in preterm-born adults. Neuroimage 150: , 68–76. |
[71] | Fogassi L , Luppino G ((2005) ) Motor functions of the parietal lobe. Curr Opin Neurobiol 15: , 626–631. |
[72] | Uddin MN , Figley TD , Marrie RA , Figley CR , CCOMS Study Group ((2018) ) Can T1w/T2w ratio be used as a myelin-specific measure in subcortical structures? Comparisons between FSE-based T1w/T2w ratios, GRASE-based T1w/T2w ratios and multi-echo GRASE-based myelin water fractions, NMR Biomed 31: , e3868. |
[73] | Uddin MN , Figley TD , Solar KG , Shatil AS , Figley CR ((2019) ) Comparisons between multi-component myelin water fraction, T1w/T2w ratio, and diffusion tensor imaging measures in healthy human brain structures. Sci Rep 9: , 2500. |
[74] | Arshad M , Stanley JA , Raz N ((2017) ) Test– retest reliability and concurrent validity of in vivo myelin content indices: Myelin water fraction and calibrated T1w/T2w image ratio. Hum Brain Mapp 38: , 1780–1790. |
[75] | Shams Z , Norris DG , Marques JP ((2019) ) A comparison of in vivo MRI based cortical myelin mapping using T1w/T2w and R1 mapping at 3T. PLoS One 14: , e0218089. |
[76] | Sexton CE , Betts JF , Demnitz N , Dawes H , Ebmeier KP , Johansen-Berg H ((2016) ) A systematic review of MRI studies examining the relationship between physical fitness and activity and the white matter of the ageing brain. Neuroimage 131: , 81–90. |
[77] | Won J , Callow DD , Pena GS , Gogniat MA , Kommula Y , Arnold-Nedimala NA , Jordan LS , Smith JC ((2021) ) Evidence for exercise-related plasticity in functional and structural neuralnetwork connectivity. Neurosci Biobehav Rev 131: , 923–940. |
[78] | Dao E , Hsiung G-YR , Liu-Ambrose T ((2018) ) The role of exercise in mitigating subcortical ischemic vascular cognitive impairment. J Neurochem 144: , 582–594. |
[79] | Wilkinson I , Webb AJS ((2022) ) Consistency of associations of systolic and diastolic blood pressure with white matter hyperintensities: A meta-analysis. Int J Stroke 17: , 291–298. |
[80] | Lai Y , Jiang C , Du X , Sang C , Guo X , Bai R , Tang R , Dong J , Ma C ((2020) ) Effect of intensive blood pressure control on the prevention of white matter hyperintensity: Systematic review and meta-analysis of randomized trials. J Clin Hypertens 22: , 1968–1973. |