Transcranial Photobiomodulation Treatment: Significant Improvements in Four Ex-Football Players with Possible Chronic Traumatic Encephalopathy
Abstract
Background:
Chronic traumatic encephalopathy, diagnosed postmortem (hyperphosphorylated tau), is preceded by traumatic encephalopathy syndrome with worsening cognition and behavior/mood disturbances, over years. Transcranial photobiomodulation (tPBM) may promote improvements by increasing ATP in compromised/stressed cells and increasing local blood, lymphatic vessel vasodilation.
Objective:
Aim 1: Examine cognition, behavior/mood changes Post-tPBM. Aim 2: MRI changes - resting-state functional-connectivity MRI: salience, central executive, default mode networks (SN, CEN, DMN); magnetic resonance spectroscopy, cingulate cortex.
Methods:
Four ex-players with traumatic encephalopathy syndrome/possible chronic traumatic encephalopathy, playing 11– 16 years, received In-office, red/near-infrared tPBM to scalp, 3x/week for 6 weeks. Two had cavum septum pellucidum.
Results:
The three younger cases (ages 55, 57, 65) improved 2 SD (p < 0.05) on three to six neuropsychological tests/subtests at 1 week or 1 month Post-tPBM, compared to Pre-Treatment, while the older case (age 74) improved by 1.5 SD on three tests. There was significant improvement at 1 month on post-traumatic stress disorder (PTSD), depression, pain, and sleep. One case discontinued narcotic pain medications and had reduced tinnitus. The possible placebo effect is unknown. At 2 months Post-tPBM, two cases regressed. Then, home tPBM was applied to only cortical nodes, DMN (12 weeks); again, significant improvements were seen. Significant correlations for increased SN functional connectivity (FC) over time, with executive function, attention, PTSD, pain, and sleep; and CEN FC, with verbal learning/memory, depression. Increased n-acetyl-aspartate (NAA) (oxygen consumption, mitochondria) was present in anterior cingulate cortex (ACC), parallel to less pain and PTSD.
Conclusion:
After tPBM, these ex-football players improved. Significant correlations of increased SN FC and CEN FC with specific cognitive tests and behavior/mood ratings, plus increased NAA in ACC support beneficial effects from tPBM.
INTRODUCTION
Chronic traumatic encephalopathy (CTE)
CTE is a progressive neurodegenerative disease diagnosed only at postmortem where hyperphosphorylated tau (p-tau) deposits are present in neurofibrillary tangles located in an irregular pattern around small blood vessels in deep cortical sulci [1, 2]. CTE is preceded by worsening cognition, behavior, and mood over time, e.g., traumatic encephalopathy syndrome (TES) [3–7]. CTE is primarily observed in athletes with repetitive head impacts (RHI) from contact/collision sports [1, 4, 6, 8–16]; and in veterans with blast-traumatic brain injury (TBI) due to injurious head acceleration [17, 18]. Different neurotrauma injuries trigger common pathogenic mechanisms inducing convergent CTE pathology [19].
The four stages of CTE ranging from mild to severe, have pathology based on density and regional deposition of p-tau across the brain, primarily in dorsolateral frontal cortex, superior temporal cortex, entorhinal cortex, amygdala, and brainstem locus coeruleus [1, 6, 15, 16]. The earliest stage with the youngest cases shows p-tau only in dorsolateral frontal cortex and locus coeruleus [20].
CTE has been observed in teenagers and up to age 79 in tackle football players [1, 6]. Among 202 ex-football players, CTE was diagnosed in 87% [6]. Severe CTE pathology was present in the majority of former college (56% ), semi-professional (56% ), and professional (86% ) football players, where 95% had cognitive disturbance, and 89% showed behavior/mood disturbance. Age of first exposure (AFE) to tackle football at less than 12 years predicted earlier onset of cognitive and behavior/mood disturbances; youth exposure may reduce resiliency to late-life neuropathology [21]. A greater proportion with severe CTE had played as linemen (offense or defense) [6]. National Football League (NFL) linemen had higher plasma t-tau from blood protein analysis, relative to non-linemen [22], and higher t-tau concentrations in lumbar puncture cerebrospinal fluid [23]. Among collegiate football players, offensive linemen reported returning to play while still experiencing post-impact symptoms, although not concussion [24]. Increased years of playing is associated with greater CTE severity [25]. Those with CTE were 10 times more likely to have played football for at least 14.5 years; sensitivity and specificity were maximized at 11 years.
Brain positron emission tomography (PET) scans with flortaucipir in former NFL players with cognitive and behavior/mood disturbances have shown significant increase in tau deposits in football players as a group, compared to age-matched healthy controls in three cortical areas: 1) bilateral superior frontal; 2) bilateral medial temporal, and 3) left parietal [26]. These overlap with cortical areas of p-tau deposits postmortem in CTE [1, 6, 20]. These PET scans are not refined enough to diagnose CTE in vivo, in individual cases.
Currently, medical history including participation in contact/collision sports with RHI, mild TBIs (mTBIs), or military exposure to blast-TBI, as well as presence of worsening cognitive and behavior/mood disturbances over time, are primary for TES to posit a future CTE diagnosis postmortem in a living person [3, 5, 6]. TES is not fully accounted for by any other neurologic, psychiatric, or medical condition [7].
Mechanisms of photobiomodulation (PBM)
PBM therapy is a safe, painless, noninvasive, nonthermal modality using primarily visible red wavelengths (600–700 nm), and/or non-visible, near-infrared (NIR) wavelengths (800–1100 nm) to stimulate, heal, and repair damaged or dying cells [27–31]. On the cellular level, photons in red/NIR wavelengths are absorbed in mitochondria by cytochrome C oxidase (CCO), terminal enzyme in the electron transport chain, particularly in hypoxic/stressed cells [32]. In these compromised cells, there is build-up of nitric oxide (NO) in CCO, resulting in low oxygen and low production of adenosine tri-phosphate (ATP) by mitochondria. Following application of red/NIR photons, there is release of NO from CCO to outside the cell wall, promoting local vasodilation, as well as increased production of ATP, thus improving mitochondrial and cellular function [27, 28, 33, 34].
Transcranial PBM (tPBM) promotes dilation of lymphatic vessels, as well as blood vessels [35]. In the human brain, lymphatic vessels within the dura mater run alongside blood vessels located in the superior sagittal and transverse sinuses [36–38], participating in waste clearance [36, 39, 40]. In mouse models of Alzheimer’s disease (AD), application of NIR laser to frontal scalp showed significant reduction of amyloid-β plaques, along with improved cognitive, memory, and neurological status, compared to untreated animals [41]. In TBI mice, impaired glymphatic function has promoted tau pathology [42]. Improving lymphatic drainage has been suggested as a novel target for treating neurological diseases [43].
Other PBM mechanisms include anti-inflammatory effects [44–46]; promotion of brain-derived neurotrophic factor (BDNF)—a neurotrophin associated with neural regeneration, dendritic sprouting, reconnection, and improved synaptic efficacy; promotion of Synapsin-1, associated with synaptogenesis [47, 48]; and less oxidative damage [46]. These effects have been reviewed [49–51].
Since 1981, the depth of photon penetration from scalp application using NIR laser on human cadavers has been examined [52]. Some photons from 808 nm, NIR laser have been detected at a depth of 4-5 cm [53]. There is deeper penetration from NIR, 830 nm light-emitting diodes (LED), than red, 633 nm [54]. Computer simulations have demonstrated that when an LED is placed on the scalp surface over a cortical target area, relatively more photons are delivered to that target area than others; photons in nearby regions follow an exponential decay [55, 56]. PBM research and clinical studies have been performed safely since the 1960 s [57]. PBM offers the possibility of endogenous, self-repair mechanisms without negative side effects.
Clinical tPBM studies in humans
When used with chronic mTBI, tPBM has improved cognition, mood, and sleep in open-protocol studies; the whole scalp was treated with red/NIR LEDs [58–61]. Significant improvements in executive function and verbal memory were present, lasting for 2 months after the final, 18th transcranial LED (tLED) treatment [59, 60]. Fewer post-traumatic stress disorder (PTSD) symptoms were reported [58–61]. Similar improvements were reported with a Pro Ice-Hockey player (age 23) with six concussions in 5.5 years [62]. tPBM has been used safely in acute, moderate TBI; follow-up magnetic resonance imaging (MRI) after 3 months showed significant improvement in diffusion parameters of white matter tracts for real tPBM (not sham) [63].
In mild to moderately-severe dementia cases, tPBM has also improved cognition, mood, and sleep [64–66]. Cases treated for 12 weeks showed significant cognitive improvements soon after the final tPBM treatment [64]. One month later, however, decline was present. They likely had neurodegenerative disease, e.g., AD. These follow-up results with AD are in contrast to those with chronic mTBI, where improved cognition was still present 2 months after the final, 18th tLED treatment [59, 60].
tPBM has been helpful in treating chronic stroke patients. Left-hemisphere (LH) stroke patients with lasting language problems (aphasia), showed significant improvement in naming ability following 18 tPBM treatments applied to the left side of the head/scalp, e.g., same side as where the stroke occurred [67]. No improvement occurred when the LEDs were applied to both sides of the head.
tPBM has been used in acute, non-hemorrhagic stroke [68–70]. The NeuroThera Effectiveness and Safety Trial applied NIR transcranial laser once, within the first 24 h post-stroke to both sides of the head/scalp. Initial results showed significance for better outcome at day 90, for real versus sham, but analysis halfway into Phase III trials did not. Since then, other tPBM studies with humans have applied at least 18 tPBM treatments in various CNS disorders, with safety and efficacy, e.g., chronic mTBI [58–61]; dementia [64, 65]; and chronic stroke, where the tPBM was applied only to the same side as the stroke [67].
SPECT, MRI, and EEG studies with transcranial PBM
Brain imaging studies have reported increased local, regional cerebral blood flow (rCBF) post-tPBM, e.g., increased rCBF at the frontal pole after one tLED application to the forehead in major depressive disorder [71], and on brain single photon emission computed tomography (SPECT) scans after a tLED series to the forehead in a chronic, severe TBI case [72]. Significant increase in rCBF was present overall on brain SPECT following a series of tLED applications to the whole head in veterans with chronic, mTBI; improved cognition and mood followed [61].
Using resting-state functional-connectivity MRI (rs-fcMRI), increased functional connectivity (FC) has been observed between cortical nodes within specific, intrinsic neural networks after tPBM. In chronic dementia, increased FC between posterior nodes of the default mode network (DMN) was present, along with improved cognition [65]. In chronic, LH stroke patients with aphasia, application of 2-inch diameter red/NIR LED cluster heads to the LH, plus two midline nodes of DMN [mesial prefrontal cortex (mPFC), precuneus] significantly increased FC in DMN, salience network (SN), and central executive network (CEN), with improved naming ability [67].
In healthy adults (ages 18–40) undergoing rs-fcMRI during NIR laser application toward the R forehead, increased FC began within 1 min in the right hemisphere [31]. Broadband NIR spectroscopy has shown that NIR laser (1064 nm) applied to the forehead in healthy young adults significantly increased cerebral concentrations of oxidized CCO and oxygenated hemoglobin in cortical regions treated; sham had no effect [73, 74]. An EEG study with NIR tPBM in healthy, older adults (ages 61–74) showed increased power of gamma, alpha, and beta waves, and decreased power of delta and theta immediately after one, 20-min NIR tLED application to only nodes of the DMN with a 40 Hz pulse rate (gamma frequency); sham had no effect [75]. Alpha and beta frequencies are associated with attention and cognitive processes [76]. Higher power in the lower frequency bands, along with reduced power in the higher frequency bands, have been observed in disorders involving cognitive impairment, such as dementia and AD [77, 78].
Aims of study
The primary aim was to present post-tPBM changes in cognition and behavior/mood for four, American-style ex-football players who had progressive symptoms of TES, possible CTE. The secondary aim was to present post-tPBM changes on MRI, including rs-fcMRI (SN, CEN, DMN), and magnetic resonance spectroscopy (MRS) for metabolites in cingulate cortex.
This study was approved by the Institutional Review Board, VA Boston Healthcare System. The MRI scan portion was approved by the IRB, Boston University School of Medicine where MRI scans were performed. Participants signed Informed Consent Forms; all methods were performed in accordance with relevant guidelines and regulations. This was an open-protocol, pilot study.
MATERIALS AND METHODS
Participant selection
Inclusion criteria
Subjects, ages 18–75 years, with history of RHI, and appropriate results from screening neuropsychological (NP) cognitive tests and behavior/mood questionnaires:
Table 1
Participant demographics: Four ex-football players who played American football for 11 to 16 years
ID | Age at Entry (y) Race | Education (y) Highest Degree | Age First Exposure (y) Organized Football | Total years Played Football | Total years Played Pro-Football | Primary Position Played | Chief Complaints at Entry |
P1 | 65 Caucasian/White | 20 PhD, in Exercise Physiology after football | 10 Pop Warner | 14 | 1.5 CFL | Middle Linebacker, Defense | Emotional outbursts, depression, cognitive problems, poor memory, poor sleep |
P2 | 55 Caucasian/White | 16 BA | 7 Pop Warner | 15 | 0 Pop Warner, High School, College, only | Tackle, Offensive Lineman | Emotional outbursts, cognitive problems, poor memory |
P3 | 57 African American/Black | 16 BA | 14 High School | 16 | 8 NFL | Cornerback, Defense Position | Emotional outbursts, depression, cognitive problems, poor memory, poor sleep; chronic pain (15 surgeries during NFL); tinnitus |
P4 | 74 Caucasian/White | 17 BS, Engineering | 13 | 11 | 4 NFL | Defensive End, and Offensive Lineman, all positions | Emotional outbursts, cognitive problems, chronic pain (4 hip replacements, 3 shoulder surgeries; 2 knee surgeries; 3 wrist/hand surgeries; 2 biceps surgeries) |
NFL, National Football League; CFL, Canadian Football League.
Screening, NP tests, and behavior/mood questionnaires. At screening, the TOMM [79] was administered to rule out malingering. Also, the Word Reading Subtest from the Wide Range Achievement Test-4 (WRAT-4) was administered to estimate premorbid level of cognitive functioning [80]. For inclusion, a participant needed to score at least 2 SD below average on one, or 1 SD below on two, of the following NP tests/subtests (age and education-adjusted norms): 1) Trail Making Tests A & B [81]; 2) Controlled Oral Word Association Test (COWAT)/FAS Test [82, 83]; 3) California Verbal Learning Test-II (CVLT) [84]; and 4) Color-Word Interference Test for Executive Function, Stroop [85]. Screening scores were adjusted, based on estimated premorbid level of cognitive functioning from the WRAT-4 [80]. Self-reported pain questionnaires were also administered, the VAS Pain Rating (0–10), and the Short Form, McGill Pain Questionnaire (SF-MPQ) [86].
Exclusion criteria
Diagnosis of a progressive neurological disease such as amyotrophic lateral sclerosis, Parkinson’s disease, dementia; severe TBI with history of craniotomy or craniectomy; a life-threatening disease (cancer or disease requiring organ transplant); severe mental disorder (schizophrenia, bipolar); current substance abuse or active treatment; and on test for memory malingering [79], a score of less than 45 on either Part 1 or 2 indicating evidence of malingering; a pain level of greater than 7/10 on VAS pain scale, or greater than 38/50 on the SF-MPQ [86].
Participants
Table 1 provides demographics for the four ex-football players. They were under continuing medical care from their own physicians; treatable conditions were addressed [87]. Using the 2021 NIH, TES consensus criteria for CTE [7], all four ex-football players met TES criteria for “Possible CTE.” This included documented cognitive impairment (here, screening NP tests at Entry); substantial exposure to contact/collision sport, ≥5 years football (here, 11–16 years); delayed onset; and neurobehavioral dysregulation (here, behavior/mood questionnaires, self-ratings). Three cases were able to undergo MRI scanning (P1, P2, P4) (Supplementary Figures 1 and 2). A cavum septum pellucidum (possible future biomarker for CTE [7]) was present in two of these three cases (P1, P4). There were no focal lesions, although cortical atrophy was present. P3 could not undergo MRI due to shoulder pain if inside a closed-bore scanner. MRI acquisition protocols and analysis programs are in Supplementary Text Material 1.
Initial, in-office tPBM series
Each participant received an initial, In-office tPBM treatment series. This included 18 tLED treatments, each lasting approximately 22–40 min (3x/week, 6 weeks), with 48 h between treatments. Three different tPBM devices/protocols were used. This is due to availability of more advanced devices, as the pilot study progressed. Common characteristics across all devices included application of NIR wavelengths (810 nm, 850 nm, or 870 nm) to scalp locations, and 26 J/cm2 at each location. Each device, and treatment protocol is described within each case report below (Results, Part 1).
Pre-/post-testing schedule for a tPBM series
Pre- and Post- a tPBM treatment series, each participant was examined with NP cognitive tests, and self-rated, behavior/mood questionnaires (Supplementary Table 1). Participants were tested pre-treatment, and at 1 week, 1 month (4–6 weeks), and 2 months (8–12 weeks) after the final, 18th in-office tPBM treatment (6-week treatment series). If a later, at-home tPBM series was performed, one post-testing was obtained within 2 weeks following that 12-week series.
Results are presented below in two parts: Part 1, Results for NP tests and behavior/mood ratings - four cases; and Part 2, Results for MRI studies - three cases.
RESULTS, PART 1. NP TESTS AND BEHAVIOR/MOOD QUESTIONNAIRES: FOUR CASES
Case P1
P1 entered at age 65, with AFE to football at age 10. He played middle linebacker, defense position for 14 years including 1.5 years in the Canadian Football League (Table 1). He received two tPBM treatment series, e.g., initial, in-office, and later, at-home.
Initial, in-office tPBM series
P1 was treated with tPBM Protocol A (Fig. 1, column A; Supplementary Table 2). This protocol applied red/NIR LED cluster heads (2-inch diameter) over the entire scalp, using the same scalp locations as with our previous mTBI studies [59, 60].
Fig. 1
LED devices used for tPBM Protocols A, B, and C. Protocol A: In-office treatments with red/NIR, LED cluster heads (1); two sets of six, LED cluster heads were held in place with a soft, breathable, nylon cap (arrow, 2), MedX Health, Inc., Canada. Supplementary Table 2 lists all LED parameters. Protocol B: At-home treatments with single, NIR diodes (810 nm, pulsed 40 Hz) placed on scalp only over cortical nodes, Default Mode Network, including a single, NIR intranasal applicator directed towards olfactory bulbs to indirectly treat connections with hippocampal areas (3), Neuro Gamma device; plus a separate, red intranasal diode (4), Vielight, Inc., Canada. Not necessary to shave the head. Additional home treatment information is in Supplementary Text Material 2. Protocol C: In-office treatment with a helmet (5), lined with red/NIR, LED cluster heads placed in rows inside the helmet (6), THOR Photomedicine, Ltd., UK. NIR, near-infrared. Permissions: Protocol A, (2) reprinted with authors’ permission from [59]. Protocol B, (3) and (4) reprinted with permission from Vielight, Inc., in the public domain.
![LED devices used for tPBM Protocols A, B, and C. Protocol A: In-office treatments with red/NIR, LED cluster heads (1); two sets of six, LED cluster heads were held in place with a soft, breathable, nylon cap (arrow, 2), MedX Health, Inc., Canada. Supplementary Table 2 lists all LED parameters. Protocol B: At-home treatments with single, NIR diodes (810 nm, pulsed 40 Hz) placed on scalp only over cortical nodes, Default Mode Network, including a single, NIR intranasal applicator directed towards olfactory bulbs to indirectly treat connections with hippocampal areas (3), Neuro Gamma device; plus a separate, red intranasal diode (4), Vielight, Inc., Canada. Not necessary to shave the head. Additional home treatment information is in Supplementary Text Material 2. Protocol C: In-office treatment with a helmet (5), lined with red/NIR, LED cluster heads placed in rows inside the helmet (6), THOR Photomedicine, Ltd., UK. NIR, near-infrared. Permissions: Protocol A, (2) reprinted with authors’ permission from [59]. Protocol B, (3) and (4) reprinted with permission from Vielight, Inc., in the public domain.](https://content.iospress.com:443/media/adr/2023/7-1/adr-7-1-adr220022/adr-7-adr220022-g001.jpg)
Results: Initial, in-office tPBM series
NP tests: At 1 week or 1 month after the final, 18th In-office treatment (compared to pre-treatment), P1 showed significant improvement (2 SD, p < 0.05) on five tests/subtests: 1) CVLT for 16 words to remember: Short Delay Cued Recall; 2) and 3), the computer-timed Continuous Performance Test (CPT): decreased False Alarm Rate, and improved d prime, detectability; 4) and 5), Brief Visuospatial Memory Test (BVMT): Immediate Recall, and Recognition Discrimination Index (Recognition Hits minus False Alarms). At the 2-months post-testing, however, there was decline, with significant improvement remaining only on one subtest, the BVMT, Recognition Discrimination Index (graphs, Fig. 2A; full data, Supplementary Table 3A).
Fig. 2
P1, cognitive and behavior/mood results. A) Z-Score graphs for some NP tests (Stroop, CPT, BVMT) after the initial, In-office tPBM series (Protocol A); and the later, at-home series (Protocol B). B) Self-ratings for behavior/mood questionnaires after each series. These ratings showed a pattern similar to NP tests, e.g., improvements at 1 week or 1 month after initial series, but worsening 2 months later, especially, PTSD/PCL-C (a). Graphs show improved (lower) ratings after the 12-week, at-home series. See Supplementary Tables 3A and 3B. NP, neuropsychological; PTSD/PCL-C, Post-traumatic Stress Disorder Checklist, Civilian; the // refers to time period when 18 tPBM treatments were applied; *p < 0.05.
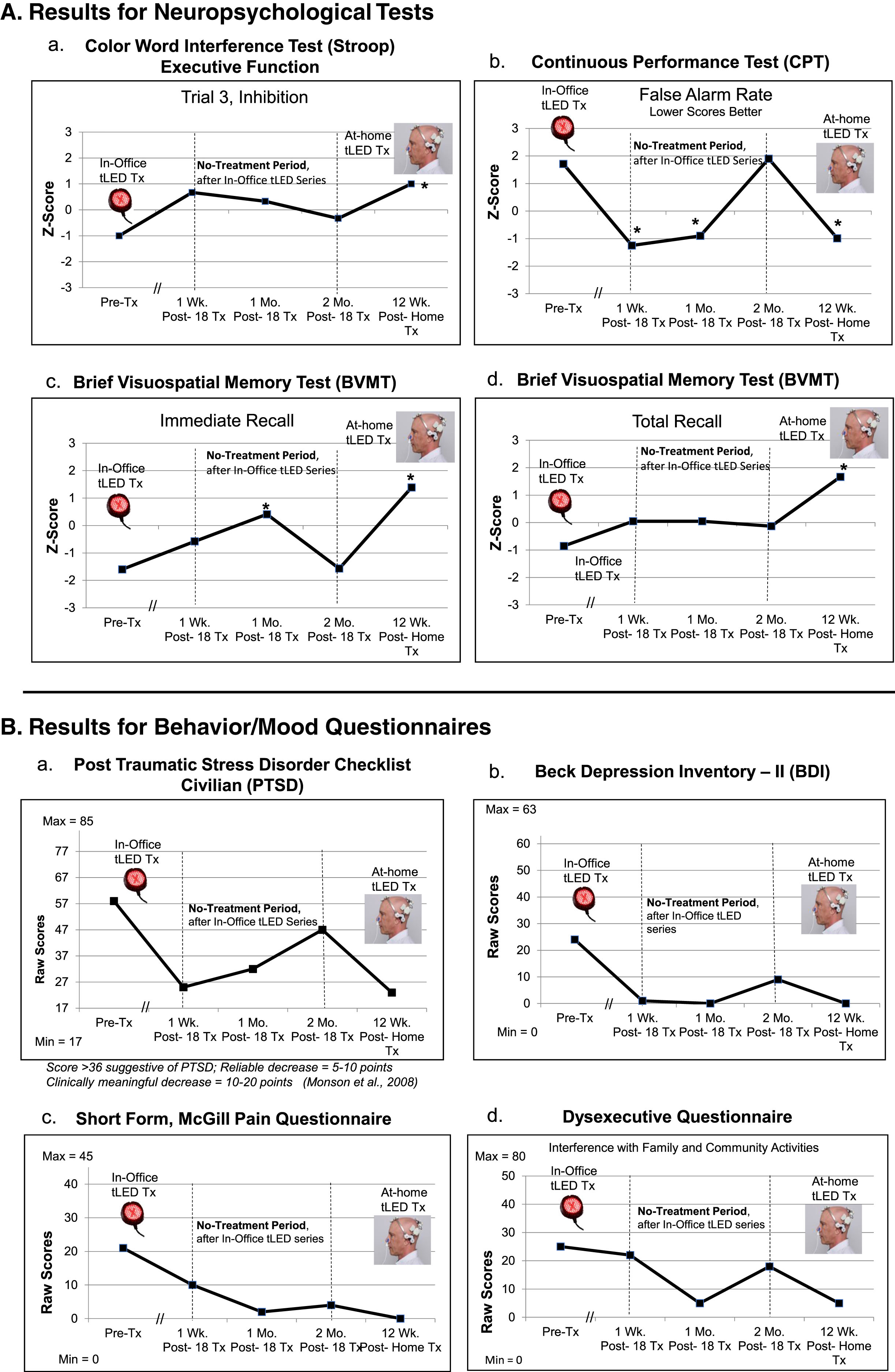
Behavior/mood questionnaires: Lower ratings indicate less impairment; there are no Z-scores. At 1 week or 1 month after the In-office series (compared to pre-treatment), P1 showed improvement in four areas: 1) PTSD/PCL-C; 2) Beck Depression Inventory (BDI); 3) pain/SF-MPQ; and 4) Dysexecutive Questionnaire (DEX, interference with family/community involvement). At the 2-months post-testing, however, these self-ratings regressed, particularly for PTSD/PCL-C [88, 89] (graphs, Fig. 2B; Supplementary Table 3B).
At the 2-months post-testing visit, P1 mentioned that return of emotional outbursts and depression was disturbing. Within one week, he obtained his own tPBM devices for at-home, self-treatment.
Later, at-home tPBM series
P1 self-treated at home for 12 weeks with Protocol B (Fig. 1, column B; Supplementary Table 2). There were two LED devices: 1) The NIR, Neuro Gamma device was applied for 20 min, 3x/week, with 48 h between treatments. This device targeted only cortical nodes of the DMN (Supplementary Text Material 2); and 2) A red diode, nasal applicator was used for 25 min, 6x/week. The two devices were often used simultaneously; no PBM treatments were performed one day a week.
Results: Later, at-home tPBM series
NP Tests: P1 re-gained most of the previous significant improvements present at 1 week or 1 month following the In-office series (but had lost after 2 months). Six tests/subtests showed significant improvement after the at-home series (compared to pre-treatment). This included for the first time, the Stroop, Trial 3, inhibition, suggesting improved executive function; and BVMT, Total Recall (Fig. 2A; Supplementary Table 3A).
Behavior/mood questionnaires: There were also improved (lower) self-ratings on PTSD/PCL-C, BDI, SF-MPQ, and DEX. These were at near-normal levels (Fig. 2B; Supplementary Table 3B).
P1 continues to self-treat at home, to date for 4 years, and reports doing well. He has initiated tPBM treatment research with college football players at two universities with prominent football programs, as well as with retired, ex-football players experiencing cognitive and behavior/mood disturbances.
Case P2
P2 entered at age 55, with AFE to football at age 7. He played offensive lineman, tackle position for 15 years through college only (Table 1). He received two tPBM series, e.g., initial, in-office, and later, at-home.
Initial, in-office tPBM series
P2 was treated with tPBM Protocol C (Fig. 1, column C; Supplementary Table 2). The tPBM device was a helmet, lined with red/NIR LED cluster heads.
Results: Initial, in-office tPBM series
NP tests: At 1 week or 6 weeks after the final, 18th in-office treatment (compared to pre-treatment), P2 showed significant improvement on six tests/subtests: 1)–4), CVLT four subtests: Total Trials 1–5; Short Delay, Cued Recall; Long Delay (20 min) Free Recall, and Cued Recall; 5), verbal fluency on COWAT; and 6) BVMT, Immediate Recall. At 12 weeks after the in-office series, five tests/subtests (CVLT) still showed significant improvement (Fig. 3A; Supplementary Table 4A).
Fig. 3
P2, cognitive and behavior/mood results. A) Z-Score graphs for some CVLT subtests after the initial in-office tPBM series (Protocol C). B) Ratings for behavior/mood questionnaires after the initial, in-office series, and after the later, at-home series (Protocol B). Improvements (lower ratings) were present at 1 week and 6 weeks after the in-office series; however, there was some worsening (higher ratings) at 12 weeks on PTSD/PCL-C (a) and pain/SF-MPQ (c). Improved (lower) ratings for PTSD (a) and pain (c) were again present after the 12-week, at-home tPBM series. See Supplementary Tables 4A and 4B. CVLT, California Verbal Learning Test; PTSD/PCL-C, Post-traumatic Stress Disorder Checklist, Civilian; SF-MPQ, Short form, McGill Pain Questionnaire; *p < 0.05.
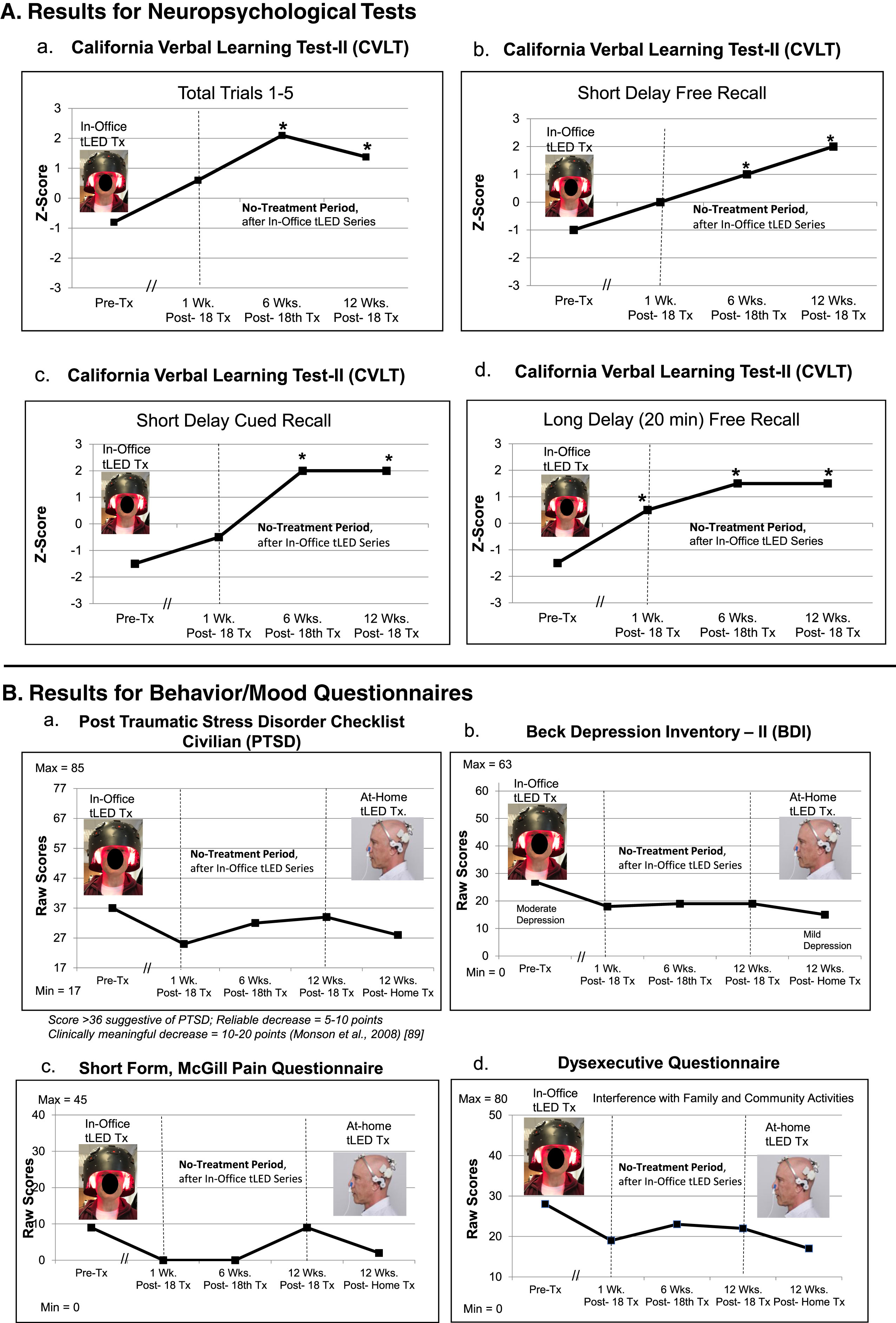
Behavior/mood questionnaires: At 1-week or 6-weeks post- the in-office tPBM series (compared to pre-treatment), improvements were present for PTSD/PCL-C, BDI, SF-MPQ, and DEX. At 12 weeks, however, there was an increase in PTSD/PCL-C, and some increased pain on the SF-MPQ rating to 9, the same as pre-treatment (Fig. 3B; Supplementary Table 4B).
At 12 weeks after the in-office series had ended, P2 was disturbed by return of emotional outbursts. One week later, he obtained his own tPBM devices for at-home, self-treatment.
Later, at-home tPBM series
P2 self-treated at home for 12 weeks using Protocol B (Fig. 1, column B; Supplementary Table 2), the same as P1.
Results: Later, at-home, tPBM series
NP tests: No in-person, NP cognitive testing could be performed after the 12-week, at-home tPBM series due to COVID restrictions.
Behavior/mood questionnaires: The questionnaires were mailed to his home after 12 weeks of home treatments. The PTSD/PCL-C, BDI, SF-MPQ, and DEX were again improved (lower ratings) (Fig. 3B; Supplementary Table 4B).
P2 reports he continues to self-treat at home, to date for two years, and is doing well. He remains employed in his own business.
Case P3
P3 entered at age 57, with AFE to football at age 14. He played cornerback, defense position for 16 years, including 8 years in the NFL (Table 1). He received only the initial, in-office tPBM series.
In-office tPBM series
P3 was treated with tPBM Protocol C (Fig. 1, column C; Supplementary Table 2). The tPBM device was a helmet, lined with red/NIR LED cluster heads.
Results: In-office tPBM series
NP tests: At 1 month after the 18th in-office treatment, P3 showed significant improvement on three tests/subtests: 1) and 2), CVLT: Total Trials 1–5, and Short Delay, Cued Recall; and 3), the computer-timed CPT, with improved d prime, detectability (Fig. 4A; Supplementary Table 5A).
Fig. 4
P3, cognitive and behavior/mood results. A) Z-Score graphs for some NP tests (CVLT, CPT) after the in-office tPBM series (Protocol C). B) Behavior/mood ratings after the in-office series. Graphs show improved (lower) ratings for pain at 1 week and at 1 month. P3 had discontinued two narcotic medications a few weeks before the 1-month post-testing time point, yet still had satisfactory pain relief (c). See Supplementary Tables 5A and 5B. NP, neuropsychological; CVLT, California Verbal Learning Test; CPT, computer-timed, Continuous Performance Test; *p < 0.05.
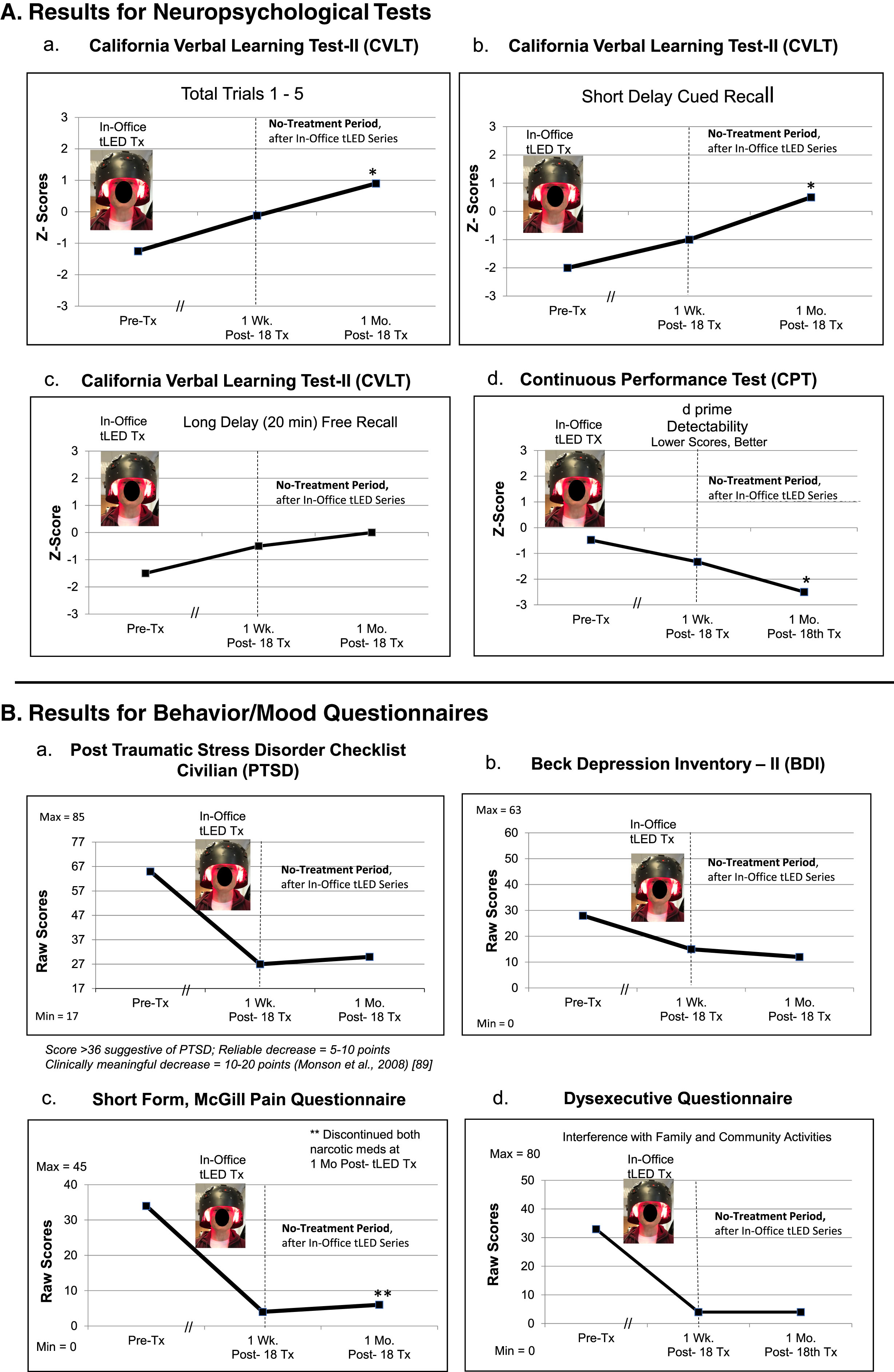
Behavior/mood questionnaires: At 1-week and at 1-month post- the in-office series, P3 improved on PTSD/PCL-C, BDI, SF-MPQ, and DEX (Fig. 4B; Supplementary Table 5B).
Additional, unexpected improvements: P3 showed two unexpected improvements at 1-month post- the tPBM series. First, he was able to discontinue two narcotic medications and still maintain satisfactory pain relief; the SF-MPQ rating was reduced by 82% . He discontinued Opana (oxymorphone) and Exalgo (hydromorphone). He continued Neurontin (gabapentin). Pre-treatment, his VAS pain rating was 7/10; at 1-week post-testing, 3/10; and at 1 month, 5.5/10 after discontinuing the narcotic medications (Supplementary Table 5B). Application of NIR tPBM to the forehead has been reported to reduce opioid cravings [90]. Second, his severe tinnitus level was reduced by 36%, to only mild. Figure 5 shows that the visible red, and hence, the non-visible NIR photons reached the sides of the neck. Application of NIR photons to sides of the neck (stellate ganglion areas) has been reported to reduce severe tinnitus [91]. No MRI scans were available for P3.
Fig. 5
P3, Tinnitus Handicap Inventory. The severe tinnitus ratings on the THI were reduced at 1 week, and at 1 month after the in-office series. White arrows suggest that the red/NIR photons reached sides of the neck with the LED-lined helmet (Protocol C). The NIR photons likely reached the left and right stellate ganglion areas, a target for NIR photons to reduce tinnitus [91]. THI, Tinnitus Handicap Inventory.
![P3, Tinnitus Handicap Inventory. The severe tinnitus ratings on the THI were reduced at 1 week, and at 1 month after the in-office series. White arrows suggest that the red/NIR photons reached sides of the neck with the LED-lined helmet (Protocol C). The NIR photons likely reached the left and right stellate ganglion areas, a target for NIR photons to reduce tinnitus [91]. THI, Tinnitus Handicap Inventory.](https://content.iospress.com:443/media/adr/2023/7-1/adr-7-1-adr220022/adr-7-adr220022-g005.jpg)
Within a few weeks after completion of the 1-month post-testing, P3 obtained his own tPBM equipment (Neuro Gamma) and began self-treating at home, the same as P1. There are no further post-testing data. He reports anecdotally that he is doing well, and to date, has continued to treat at home for over 2 years.
Case P4
P4 entered at age 74, with AFE to football at age 13. He played 11 years, including 4 years in the NFL (Table 1). His position was defensive end, and offensive lineman, playing all linemen positions. He received only the initial, in-office tPBM series.
In-office tPBM series
P4 was treated with tPBM Protocol C (Fig. 1, column C; Supplementary Table 2). The tPBM device was a helmet, lined with red/NIR LED cluster heads.
Results: In-office tPBM treatment series
NP tests: No NP, cognitive test data were available at 1-week post- the initial, on-office tPBM series. At the 1-month post-testing, there were improvements (1.5 SD) on three tests/subtests: 1) CVLT: Long Delay (20 min) Free Recall; 2) CPT: Correct Detections; and 3) BVMT, Delayed (20 min) Recall (Fig. 6A; Supplementary Table 6A).
Fig. 6
P4, cognitive and behavior/mood results. A) Z-Score graphs for some NP tests (CVLT, CPT, BVMT, Stroop) after the initial, in-office tPBM series (Protocol C). B) Behavior/mood ratings after the In-office series. See Supplementary Tables 6A and 6B. NP, neuropsychological; CVLT, California Verbal Learning Test; CPT, Continuous Performance Test; BVMT, Brief Visuospatial Memory Test.
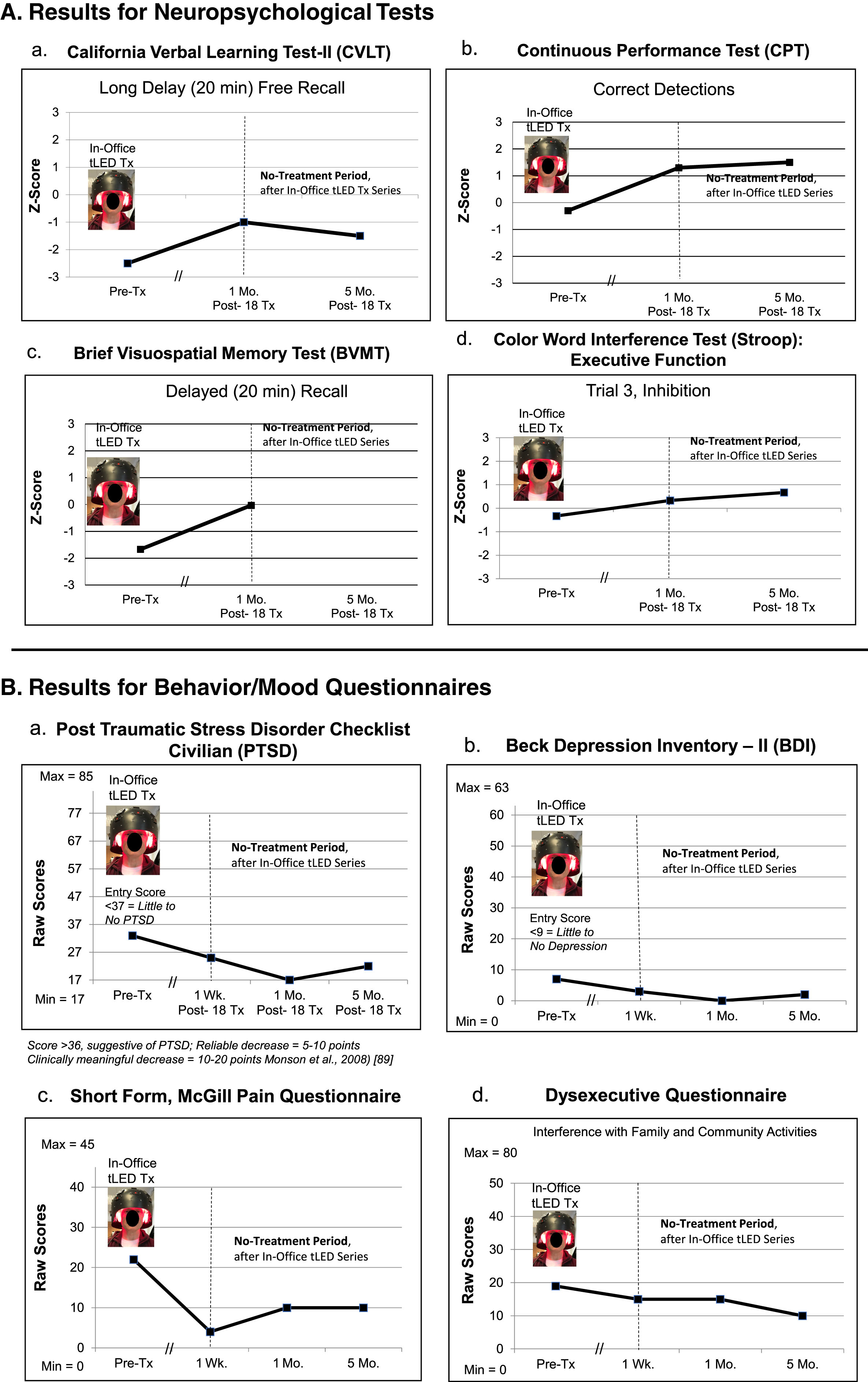
Behavior/mood questionnaires: The questionnaires were mailed to P4, for the 1-week post-testing. At 1-week and 1-month post- the final, in-office tPBM treatment, there were improved ratings for PTSD/PCL-C, BDI, SF-MPQ, and DEX, compared to pre-treatment (Fig. 6B; Supplementary Table 6B).
One year after completing study participation, P4 started tPBM home treatments, the same as P1. He has now self-treated for over 2 years and reports doing well. At age 77, he continues to work in his own business; he recently helped care for an elderly family member out of state.
SUMMARY RESULTS, PART 1. NP TESTS, AND BEHAVIOR/MOOD QUESTIONNAIRES
Table 2 shows the specific NP tests with significant improvement (2 SD, p < 0.05) for each of the three younger players (P1, P2, P3) at each time point. These three players showed significant improvement on three to six tests/subtests at 1 week or 1 month post-treatment. (P4, the older player, had shown 1.5 SD improvement on three NP tests.) Table 2 shows that the CVLT (verbal learning and memory) showed the highest number of observations of significant improvement (six), particularly at the 1-month post-testing time point. A somewhat similar pattern was present for the CPT; however, pre-treatment data on CPT for P2 were missing. Across all NP tests, the 1-month post-testing time point showed the highest number of significant improvements (twelve), compared to 1 week (seven), or 2 months (five).
Table 2
Cases with significant improvement* on specific Neuropsychological tests/subtests, at three post-testing time points. This includes only the younger cases (P1, P2, P3; ages 55, 57, 65 years); and only for the initial, In-office tPBM series
Neuropsychological Test or Subtest | 1 Week Post- | 1 Month Post-(4–6 weeks) | 2 Months Post-(8–12 weeks) |
California Verbal Learning Test II | |||
Total Trials 1–5 | – | P2, P3 | P2 |
Short Delay, Free Recall | – | – | P2 |
Short Delay, Cued Recall | P1 | P2, P3 | P2 |
Long Delay (20 min), Free Recall | P2 | P2 | P2 |
Long Delay (20 min), Cued Recall | P2 | P2 | P2 |
Total Subtests, Three Cases | 3 | 6 | 5 |
Continuous Performance Test** | |||
Correct Detections | – | – | – |
False Alarm Rate | P1 | P1 | – |
d prime | P1 | P1, P3 | – |
Total Subtests, Two Cases | 2 | 3 | 0 |
Brief Visuospatial Memory Test - Revised | |||
Trial 1 (Immediate Recall) | P2 | P1 | – |
Total Recall | – | – | – |
Delayed (20 min) Recall | – | – | – |
Recognition Discrimination Index: (Hits minus Recognition False Alarms) | P1 | P1 | P1 |
Total Subtests, Three Cases | 2 | 2 | 1 |
Controlled Oral Word Association Test | |||
For Each Letter, FAS or BHR | – | P2 | – |
Total Subtests, Three Cases | 0 | 1 | 0 |
Stroop: Color Word Interference Test | |||
Trial 3, inhibition | – | – | – |
Trial 4, inhibition/switching | – | – | – |
Total Subtests, Three Cases | 0 | 0 | 0 |
Total Tests/Subtests Improved, at Each Time Point | |||
1 Week Post-, or 1 Month Post-, or 2 Months Post- | 7 | 12 | 6 |
*≥= 2 SD (p < 0.05) Improvement; Tx, treatment. **Missing data for P2, for Continuous Performance Test.
To further analyze results for the behavior/mood questionnaires, repeated measures ANOVAs were performed with data for the four ex-football players as a group (Fig. 7; Supplementary Table 7). Significant improvements were present at 1 month after the in-office series for PTSD/PCL-C (p < 0.05); depression/BDI (p < 0.04); pain/SF-MPQ (p < 0.03); and sleep/PSQI (p < 0.04). The DEX showed borderline improvement, p < 0.097.
Fig. 7
Group statistical analyses (repeated measures ANOVAs), pre- versus post- the initial, in-office tPBM series at 1-week and 1-month post-treatment for behavior/mood questionnaires (a, b, c, d). See Supplementary Table 7.
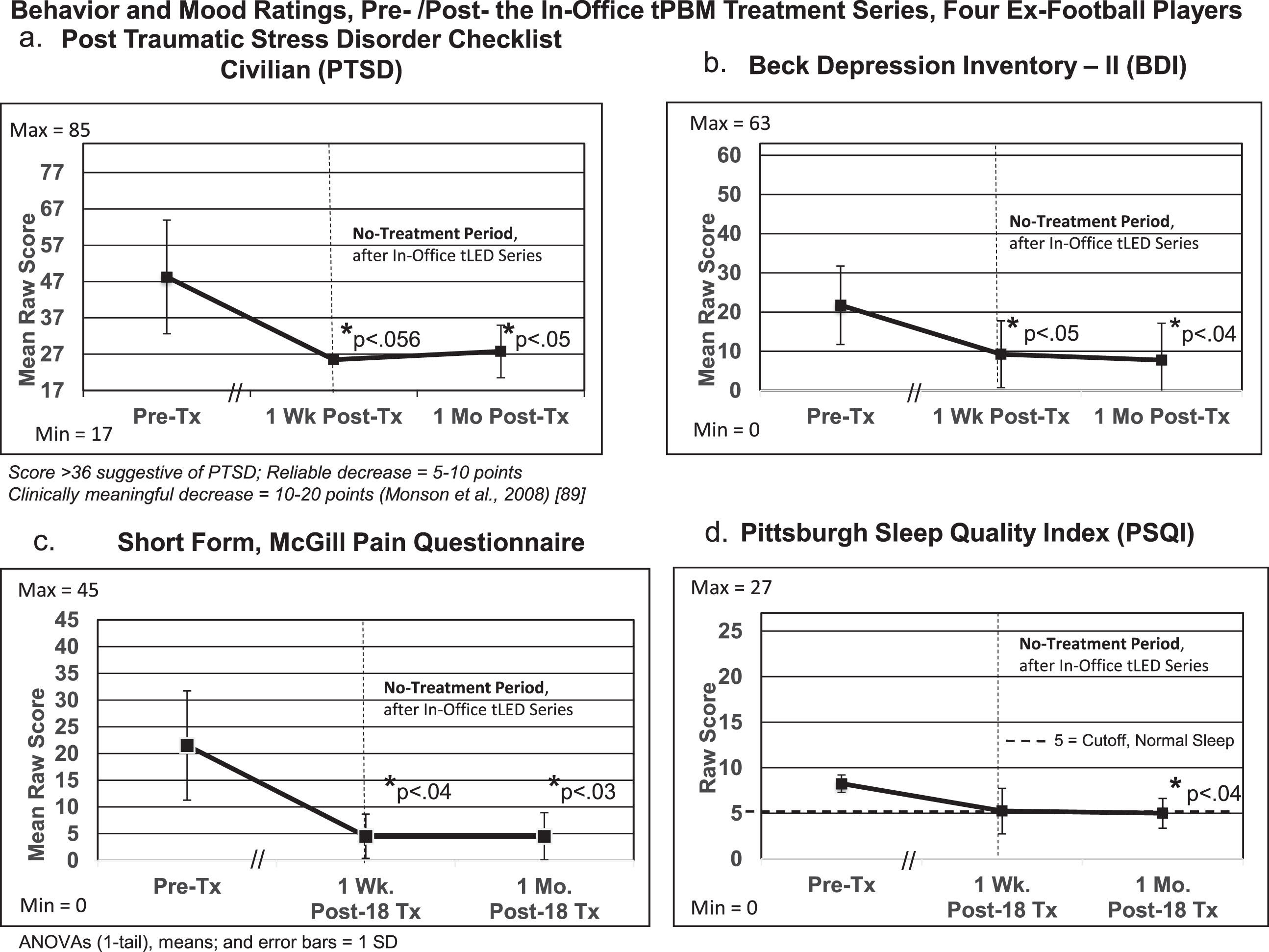
In two cases, some initial gains present at 1-month post-treatment, declined at 2 months (P1, in cognition and behavior/mood; P2, behavior/mood). After treating only the cortical nodes of the DMN at home for 12 weeks, most improvements present at 1 month (but lost at 2 months) were re-gained. All four cases have continued with tPBM home treatments for 2–4 years, with complete safety; they anecdotally report doing well.
RESULTS, PART 2. MRI STUDIES: THREE CASES
Resting-state functional-connectivity MRI: SN, CEN, DMN
Case P1
There were rs-fcMRI data for the initial, in-office series, and for the later, at-home series. P1 showed a pattern of increased FC within the SN at 1 week and at 1 month after the initial, in-office series (Fig. 8, top row, graph A). These are the same time points when he showed significant improvement on five NP tests/subtests (Fig. 2A, Supplementary Table 3A); and better (lower) ratings for PTSD/PCL-C, BDI, and SF-MPQ (Fig. 2B, Supplementary Table 3B).
Fig. 8
P1, rs-fcMRI. Top: Average FC within each network over time, pre- /post- the initial, in-office tPBM series and the later, at-home series. Compared to pre-treatment, there was a pattern of increased SN FC at 1 week and 1 month, but not at 3 months. There was return of increased SN FC after 12 weeks of home treatment. Bottom: Significant correlations were present between increased SN FC over time, and Stroop, Trials 3 and 4 (D, E), CPT (F, G), and PTSD (H); and between increased CEN FC over time, and CVLT (I, J) and BDI (K). rs-fcMRI, resting-state functional-connectivity MRI; SN, salience network; CEN, central executive network; DMN, default mode network; CPT, computer-timed, Continuous Performance Test; CVLT, California Verbal Learning Test; BDI, Beck Depression Inventory.
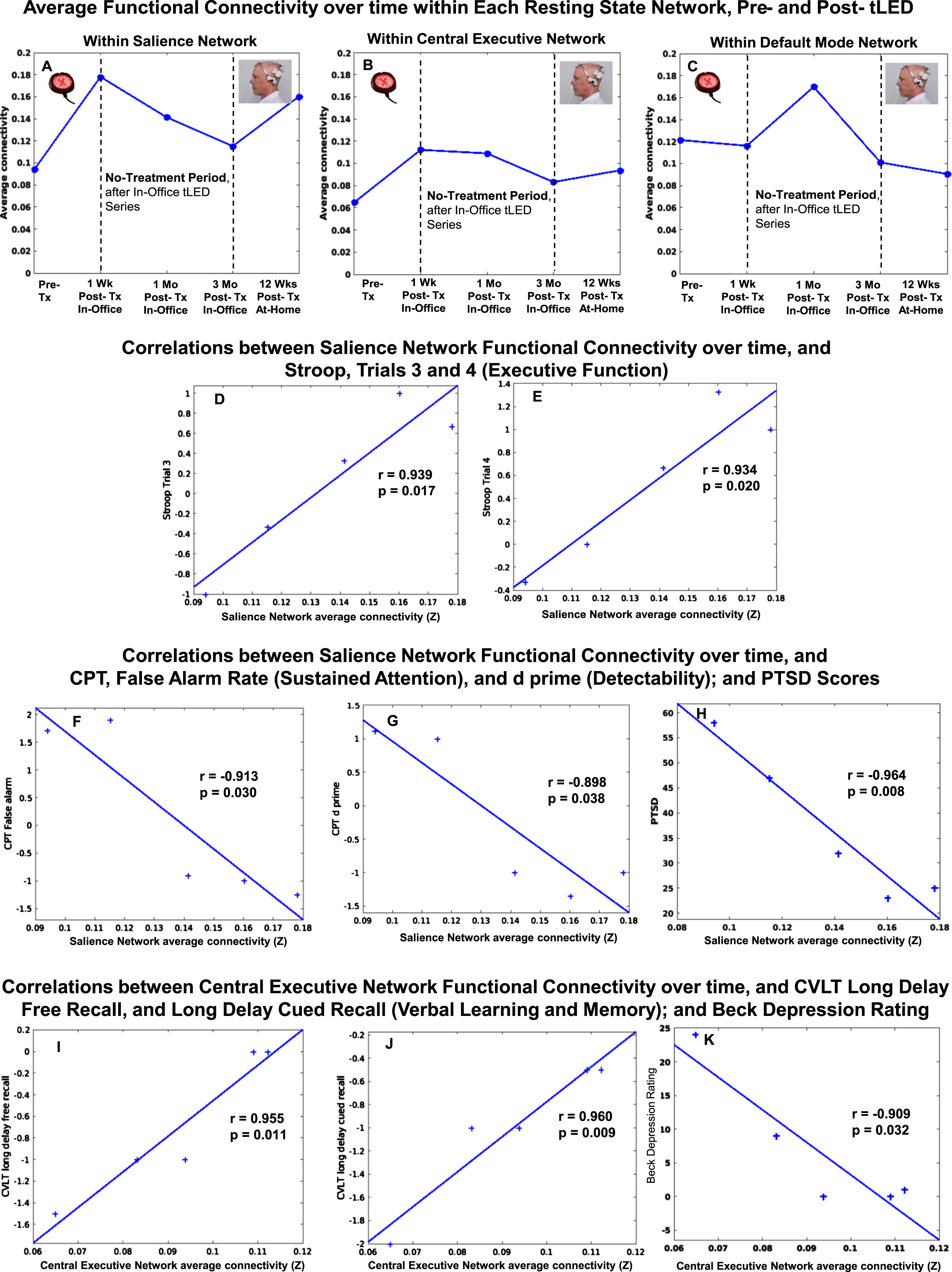
At 2-months Post-tPBM, however, his NP tests and behavior/mood ratings regressed. Likewise, the rs-fcMRI at 3 months after the in-office series showed decreased SN FC. The SN FC increased again, however, after 12 weeks of tPBM home treatment, when his cognitive tests showed significant improvement on six tests/subtests (Fig. 2A, Supplementary Table 3A); and his PTSD/PCL-C, BDI and SF-MPQ self-ratings were his best observed (Fig. 2B, Supplementary Table 3B).
Figure 8 also shows significant correlations between increased FC in two different networks (SN, CEN) over time, and specific NP tests and behavior/mood ratings over time, for P1. These include a significant positive correlation between increased SN FC and scores on the Stroop, Color-Word Interference Test (executive function), Trial 3, inhibition (graph D), and Trial 4, inhibition/switching (graph E). There was a significant negative correlation between increased SN FC, and shorter reaction times on the computer-timed CPT: False Alarm Rate, sustained attention (graph F); and d prime, detectability (graph G). In addition, significant negative correlations were present between increased SN FC and lower ratings, PTSD/PCL-C (graph H), and sleep/PQSI [p = 0.05, r = –0.88 (Supplementary Table 8)]. These significant correlations suggest a parallel relationship between increased SN FC, and better performance on objective tests (executive function, Stroop; attention, CPT), as well as subjective ratings (PTSD,sleep).
Figure 8 also shows there was a significant positive correlation with a second intrinsic network, e.g., increased CEN FC, and CVLT: Long Delay (20 min) Free Recall (graph I), and Long Delay, Cued Recall (graph J). A significant negative correlation was present between increased CEN FC and lower BDI ratings (graph K). These significant correlations also suggest a parallel relationship between increased CEN FC and better performance on objective tests (verbal learning and memory, CVLT), as well as subjective self-ratings (depression, BDI).
There were no significant correlations between DMN FC over time, and cognitive tests, or behavior/mood ratings. All correlations for FC within each intrinsic network over time, and NP tests and behavior/mood questionnaires for each case (P1, P2, P4) are listed at the end of Supplementary Tables section (Supplementary Tables 8–10).
Case P2
Resting-state fcMRI scans were available for the initial, in-office series, but not for the at-home series due to COVID restrictions. There was a pattern of increased SN FC at 1 week and at 12 weeks after the In-office series (Fig. 9, top row, graph A).
Fig. 9
P2, rs-fcMRI. Top: Average FC within each network over time, pre- /post- the initial, in-office tPBM series only. Compared to pre-treatment, there was a pattern of increased SN FC at 1 week and at 12 weeks. Bottom: Significant positive correlation between increased SN FC over time, and Stroop, Trial 3 (D). rs-fcMRI, resting-state functional-connectivity MRI; SN, salience network; CEN, central executive network; DMN, default mode network.
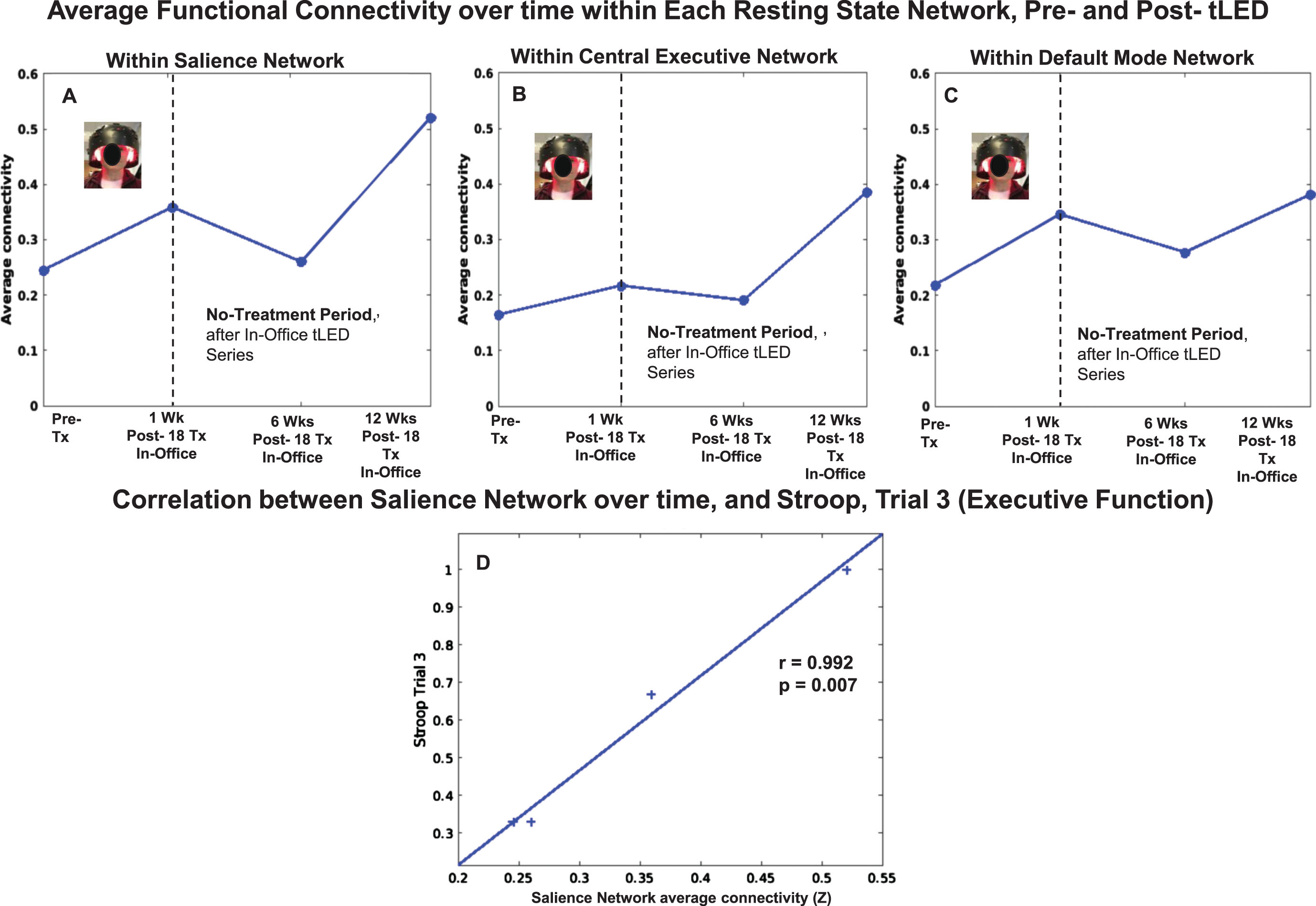
Figure 9 also shows there was a significant, positive correlation between increased SN FC over time, and performance on the Stroop, Color-Word Interference Test (executive function), Trial 3, inhibition (graph D).
Case P4
Compared to pre-treatment, the SN FC pattern for P4 showed increase at 1 week, 1 month, and 5 months (Fig. 10, top row, graph A). A significant negative correlation was present between increased SN FC, and lower ratings for pain, SF-MPQ (graph D).
Fig. 10
P4, rs-fcMRI. Top: Average FC within each network over time, pre- /post- the initial, in-office tPBM series. Compared to pre-treatment, the SN FC showed increase at 1 week, 1 month, and 5 months. Bottom: Significant negative correlation was present between increased SN FC over time, and decreased SF-MPQ pain ratings (D). rs-fcMRI, resting-state functional-connectivity MRI; SN, salience network; CEN, central executive network; DMN, default mode network.
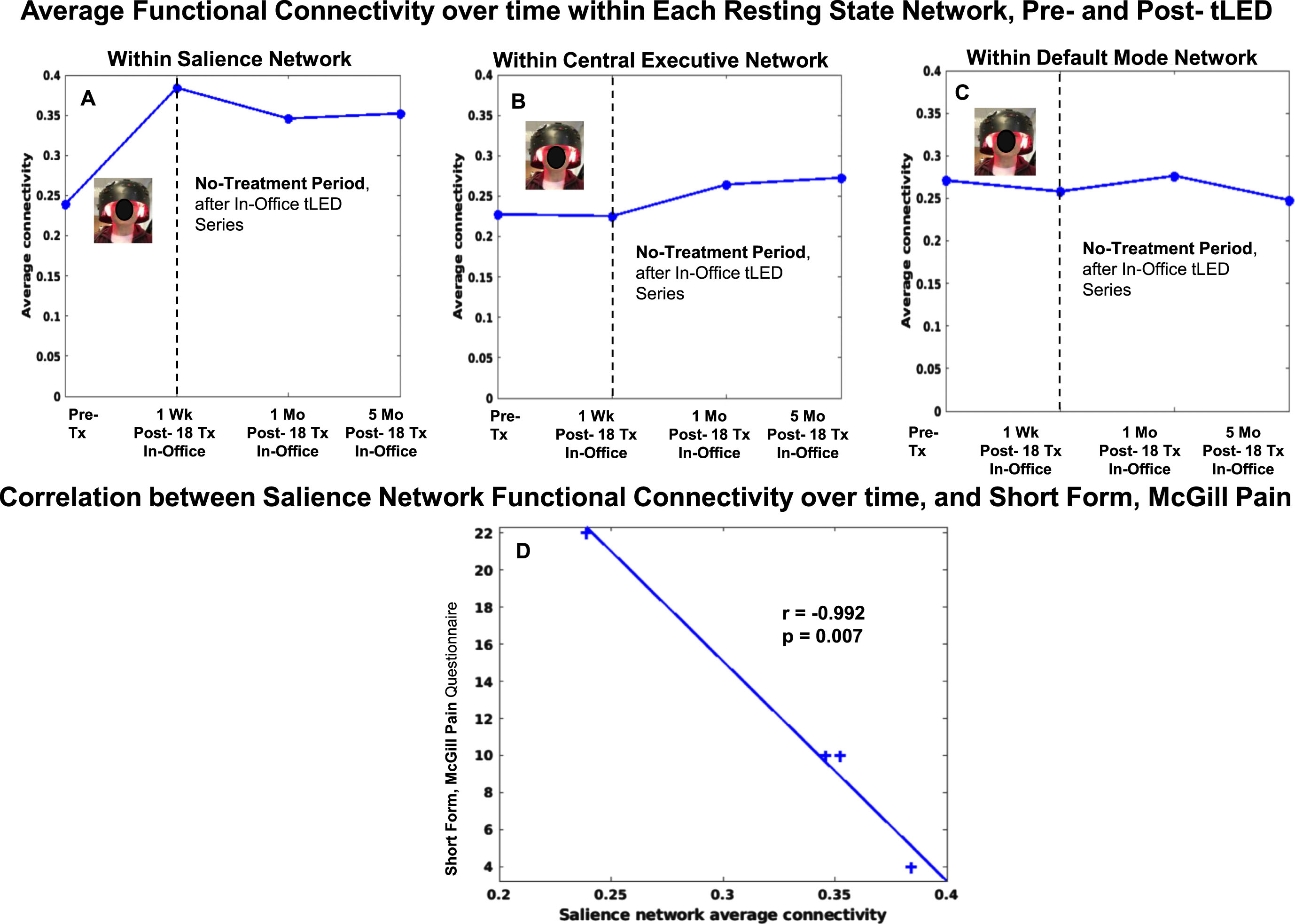
Magnetic resonance spectroscopy
Case P2
MRS metabolites were examined in ACC, and posterior cingulate cortex (PCC) separately, for the initial, in-office series only. The metabolite n-acetyl-aspartate (NAA) was examined as well as others. NAA is a neural marker present only within the body of neural cells, axons, and dendrites; it is synthesized in mitochondria and correlates with oxygen consumption [92].
At 1 week and 6 weeks after the in-office series (compared to pre-treatment), the NAA levels were higher in ACC (Fig. 11). (Changes were negligible in PCC, not shown.) At 12 weeks after the in-office series, however, the NAA levels decreased to pre-treatment levels. Increased NAA in ACC at 1-week and 6-weeks post-tPBM were parallel to his reduced pain ratings on SF-MPQ at those times, e.g., at 1 week, 0; at 6 weeks, 0, compared to pre-treatment, 9. At 12 weeks, however, the pain rating worsened to 9, same as pre-treatment (Fig. 3B; Supplementary Table 4B). A similar pattern was present for PTSD/PCL-C. The SF-MPQ and PTSD ratings were again improved, however, after the at-home series (questionnaires were mailed back), where SF-MPQ pain was 2, and PTSD/PCL-C was 28, suggesting no presence of PTSD symptomatology. There are no MRS data following the at-home tPBM series due to COVID restrictions.
Fig. 11
P2, MRS results for metabolite levels in anterior cingulate cortex over time, pre- /post- the initial, in-office tPBM series (Protocol C). Increased NAA (a correlate of oxygen consumption in mitochondria) was present at 1 week and 6 weeks after the in-office tPBM series, but not at 12 weeks, when NAA returned to pre-treatment level. P2 had rated less pain and PTSD at 1 week and 6 weeks; however, these self-ratings returned towards, or at pre-, at 12 weeks (Fig. 3B, graphs a and c). The PTSD and pain ratings again improved after the at-home tLED series. No MRS data are available then, due to COVID restrictions. MRS, magnetic resonance spectroscopy; NAA, n-acetyl-aspartate; MI, myo-inositol; Cho, choline; Cr, creatine; PPM, parts per million; PTSD, post-traumatic stress disorder.
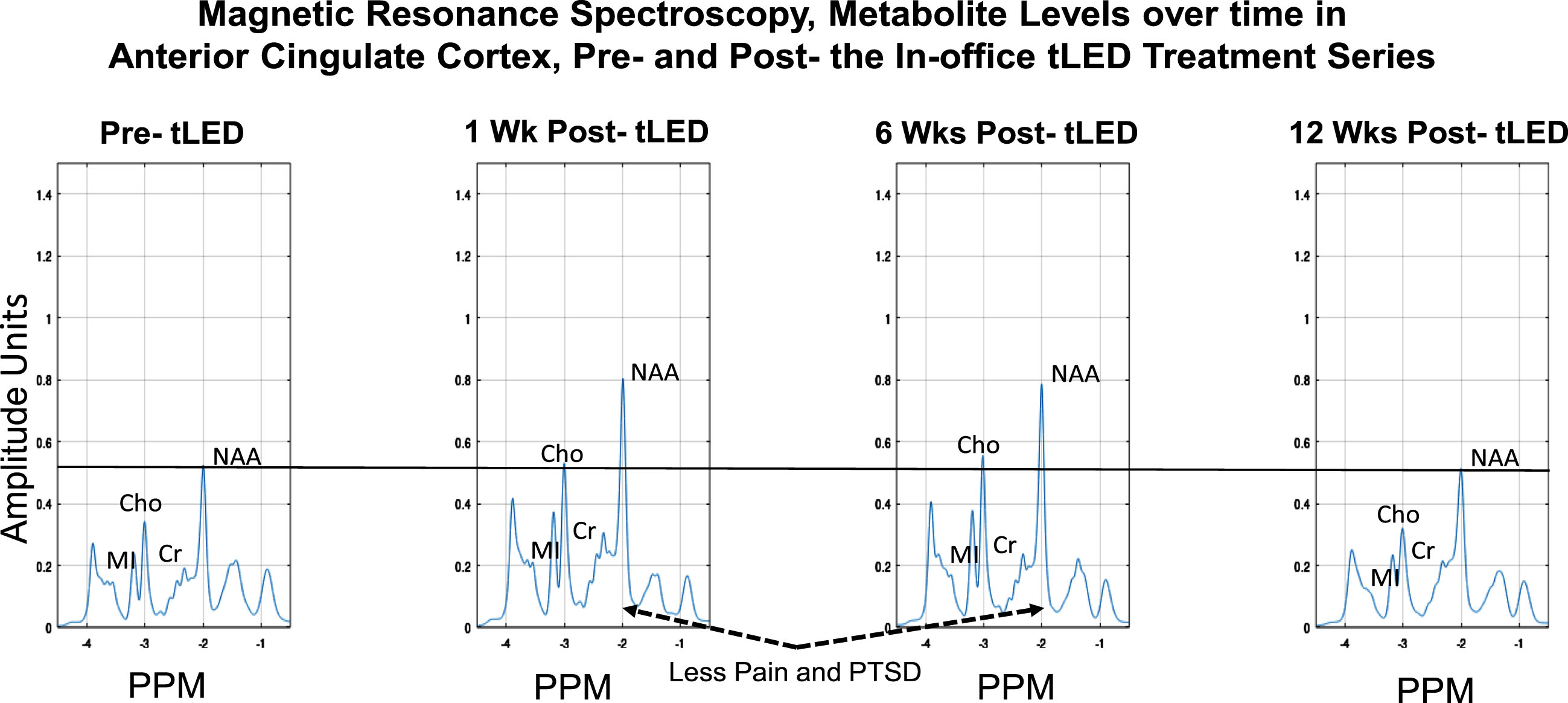
SUMMARY RESULTS, PART 2. MRI STUDIES
There was a pattern of increased SN FC at 1-week post- the in-office tPBM series for all three cases (P1, P2, P4, Figs. 8–10). Table 3 shows significant correlations between increased SN FC and CEN FC over time, with specific NP tests and behavior/mood questionnaires for each of the three cases. The SN showed the highest number of significant correlations with NP tests and behavior/mood questionnaires. There were eight instances of significant correlations between increased SN FC and the following: Stroop, Trial 3 (P1, P2); Stroop, Trial 4; CPT: False Alarm Rate and d-prime; PTSD/PCL-C (all, P1); SF-MPQ (P4); and PSQI (P1). There were three instances of significant correlations between increased CEN FC and CVLT: Long Delay, Free Recall and Cued Recall; and BDI (all, P1).
Table 3
Significant correlations between increased resting-state functional-connectivity in Salience Network, and in Central Executive Network over time, with Neuropsychological Tests and Behavior/Mood Questionnaires, Pre- /Post-tPBM for each case with rs-fcMRI data (P1, P2, P4). CVLT, California Verbal Learning Test
Intrinsic Network | Neuropsychological Tests | |||||
Stroop, | Stroop, Trial 4 | Continuous Performance Test | Continuous Performance Test | CVLT | CVLT | |
Trial 3 Inhibition | Inhibition/switching | False Alarm Rate | d-prime, Detect. | Long Delay Free Recall | Long Delay Cued Recall | |
Salience Network | P1 | P1 | P1 | P1 | ||
r = 0.94 | r = 0.93 | r = – 0.91 | r = – 0.90 | |||
p = 0.017 | p = 0.020 | p = 0.030 | p = 0.038 | |||
P2 | ||||||
r = 0.99 | ||||||
p = 0.007 | ||||||
Central Executive Network | P1 | P1 | ||||
r = 0.96 | r = 0.96 | |||||
p = 0.011 | p = 0.009 | |||||
Behavior/Mood Questionnaires | ||||||
PTSD PCL-C | Short Form McGill Pain Q. | Pittsburgh Sleep Quality Index | Beck Depression Inventory-II | |||
Salience Network | P1 | P4 | P1 | |||
r = – 0.96 | r = – 0.99 | r = – 0.88 | ||||
p = 0.008 | p = 0.007 | p = 0.05 | ||||
Central Executive Network | P1 | |||||
r = – 0.91 | ||||||
p = 0.03 |
On MRS, increased levels of NAA (correlated with oxygen consumption in mitochondria) in ACC were present at 1 week and 6 weeks after the in-office tPBM series (P2). These NAA increases were parallel to his reduced pain and PTSD, at those times. At 12 weeks, however, the NAA level, along with the pain and PTSD ratings, returned towards (or at) pre-treatment levels. This suggests an inverse relationship where higher NAA levels in ACC were associated with less pain and PTSD.
DISCUSSION
To our knowledge, this is the first treatment study (open-protocol) reporting significant improvements (cognition, behavior/mood) in ex-football players with a level of TES compatible with the category of Possible CTE [7]. Post-tPBM results and possible mechanisms are discussed in relationship to previous tPBM studies with chronic mTBI, and dementia.
Improvement in cognition
Significant cognitive improvements here are similar to areas of cognitive improvement in tPBM studies with chronic mTBI, e.g., executive function, attention, verbal learning and memory, visuospatial memory, and verbal fluency. In those studies, either the whole head/scalp had been treated [59–61] or five nodes of the DMN [62].
Improvement in behavior/mood
Behavior/mood ratings after the in-office tPBM series for the four ex-football players as a group, showed significant improvement at 1-month post-tPBM, e.g., PTSD/PCL-C, depression/BDI, pain/SF-MPQ, and sleep/PSQI. Reduced PTSD symptoms following tPBM with tLEDs were previously reported in chronic mTBI [58–62]. A recent tPBM study including 49 athletes with RHI reported significant improvement in PTSD, depression, adjustment/adaptability, and sleep after 8 weeks of tLED treatment to DMN with the Neuro Gamma [93]. Another study reported less mood dysregulation, anxiety, and irritability symptoms after tPBM with a Class IV, high-power NIR laser in chronic mTBI [94].
Ex-football players’ pattern of response over time, similar to neurodegenerative disease
The pattern of improvement at 1 month after the initial, in-office tPBM series, but followed by decline 2 months later (P1, P2), is similar to the pattern of response to tPBM in dementia [64], but not in chronic mTBI [59, 60]. When dementia cases were tested within 1 week after 12 weeks of tPBM, there were significant improvements on Alzheimer’s Disease Assessment Scale-Cognitive Subscale (p < 0.032) and Mini-Mental State Examination (p < 0.003). When LED equipment was withdrawn for the next month, however, these gains regressed. The dementia cases likely had neurodegenerative disease (AD). Thus, the pattern of response following tPBM treatment in the present study with ex-football players (P1, P2) was similar to the dementia cases. Later decline was not observed in mTBI cases, where improvements continued out to 2 months after the final tPBM treatment [59, 60]. The pattern of initial improvement (at 1 week, 1 month), but later decline (after 2-3 month) in the present study (P1, P2) suggests presence of a neurodegenerative disease, e.g., possible CTE. Each case improved again, after tPBM home treatments.
MRI changes post-tPBM
rs-fcMRI
Average FC was examined in three intrinsic networks (SN, CEN, DMN), across the pre- /post-tPBM time points available for each player (P1, P2, P4). The SN and CEN are discussed.
Salience network (cingulo-opercular network)
The SN showed the most consistent pattern of increased FC among the three networks examined, with increased FC at 1 week after the in-office tPBM series for all three cases (P1, P2, P4; Figs. 8–10), compared to pre-treatment. The SN FC also showed the highest number of significant correlations over time, with cognitive tests/subtests (Stroop, CPT); and behavior/mood (PTSD, pain, sleep); total of eight cognitive and behavior/mood measures.
Significant correlations between SN FC and cognition are compatible with the literature. The SN is necessary for efficient regulation of activity in the DMN; failure leads to inefficient cognitive control [95]. Significant positive correlation for increased SN FC and performance on Stroop, Trial 3, executive function (P1, P2) supports the role of SN in regulation of cognitive control for DMN. Significant negative correlation between SN FC and performance on the reaction-time, CPT also supports the role of the SN (particularly ACC, within SN) in facilitating sustained attention (P1). Significant correlations between increased SN FC over time, and improved performance on these tests of executive function and attention, suggests that tPBM supported these improvements.
It is noteworthy that in the Chao et al. case report [62] with a professional ice-hockey player, age 23 (6 concussions), only nodes of the DMN were treated, yet no change was observed in DMN FC, only in SN FC, similar to the present study. The SN and the DMN are closely linked and dysfunction in SN impairs DMN after TBI [96, 97].
There was a significant negative correlation between SN FC and pain ratings on SF-MPQ (P4). P4 had had 14 surgeries, associated with football. Dysfunction of ACC (a node of SN) has been reported in college football players with concussion studied up to 19 weeks post-injury [98]. A relationship between SN and chronic pain has been reported [99, 100]. There are more frequent shifts between SN and DMN in chronic pain, possibly indicating greater attention to pain [100]. There was significant reduction in pain (SF-MPQ) for the four ex-football players as a group at 1 month after the initial tPBM series. Pain-related neurons are present in the human ACC [101, 102]. Placement of tPBM over the mPFC (target area of DMN) may have also delivered NIR photons to ACC, in close anatomical proximity. Targeting the ACC in SN with tPBM in chronic pain cases could be beneficial.
A significant negative correlation between increased SN FC, and lower PTSD ratings was also present (P1). Neurocircuitry of PTSD includes deficiency in top-down regulation of amygdala by ACC, mPFC, and hippocampus [103–105]. Direct tPBM treatment of mPFC and indirect treatment of ACC in the present study may have improved top-down control of amygdala, thus reducing PTSD as also observed in other tPBM studies [58–62, 93, 94]. A role for SN in active duty US Army soldiers with PTSD has been reported [106]. Treatment of the SN with tPBM could reduce symptoms of PTSD in the military, as well as civilians.
To our knowledge, SN FC has not been previously reported in TES/Possible CTE. Here, significant correlations between SN FC over time, and improvements in executive function/Stroop (P1, P2), attention/CPT (P1), PTSD (P1), pain (P4), and sleep (P1) suggest that scalp application of NIR tPBM affected SN FC in a beneficial manner for cognition, as well as behavior/mood. The midline frontal locations of mPFC and ACC (anatomically close) suggest they are both vulnerable to damage following RHI to the forehead in American-style football, especially linemen positions [6, 22, 24]. Delivering NIR photons to both the mPFC and ACC could be especially important in treating ex-football players with RHI and possible CTE.
Central executive network (fronto-parietal network)
A significant, positive correlation was observed between increased CEN FC and verbal learning and memory on CVLT: Long Delay Free Recall, and Cued Recall (P1). Cortical nodes of the CEN are primarily the L and R DLPFC, plus the L and R intraparietal cortex (angular gyrus area). The CEN is important in initiating and adjusting cognitive control that enables an immediate transfer of knowledge to novel tasks [107]. Increased CEN FC for P1 at 1 week and 1 month after the in-office tPBM series supports his improved CVLT scores at those times on the two most difficult subtests, Long Delay (20 min) Free Recall and Cued Recall for 16 words previously heard.
The CVLT scores for P1 declined, however, at 2 months after the in-office tPBM series, as did CEN FC near that time (3 months). His later scores on these two CVLT subtests after the 12-week at-home series, however, did not rebound, nor did the CEN FC. The at-home series had treated only cortical nodes of DMN; these did not include L and R DLPFC nodes of CEN, only the L and R intraparietal cortex nodes of both networks (DMN, CEN). The initial, in-office tPBM series had treated the whole head/scalp including the L and R DLPFC, as well as intraparietal cortex. Single diodes over L and R, DLPFC have been added to the new Neuro Pro device, which still includes all DMN nodes.
There was a significant negative correlation between increased CEN FC and less depression/BDI (P1). Depression ratings were reduced from 24 at pre-treatment, to 1 at 1 week, and 0 at 1 month after the In-office series; but had increased to 9, at 2 months after that series. The CEN FC at 3 months was also decreased to almost pre-treatment level. There was little change, however, in CEN FC after the at-home tPBM series, mentioned above. Nevertheless, the depression rating had improved to 0 after the at-home series, but the contributing factors are unknown. Studies have reported a relationship between CEN FC and depression. In subthreshold depression, there was dysfunction in the cognitive control network (similar to CEN nodes), particularly with decreased FC between DLPFC and temporo-parietal junction [108]. Decreased connectivity between CEN and the rest of the brain has been related to increased depression [109]. tPBM treatment to both sets of nodes in CEN (DLPFC and intraparietal cortex) could be helpful to treat depression.
MRS: Increased NAA in ACC post-tPBM
Increased NAA was present in ACC at 1 week and 6 weeks after the initial, in-office series, compared to pre-treatment (P2). His pain ratings (SF-MPQ) had improved at those two times (lower, rated 0). At 12 weeks, however, his pain rating returned to pre-treatment (rated 9), and NAA also returned to pre-treatment level. Decreased NAA in ACC has been reported in chronic pain [110]. The importance of ACC (node in SN), and relevance of ACC and SN to pain were reviewed above (99–102).
There was also a similar pattern for NAA levels in ACC over time, and PTSD ratings for P2. Ratings were lower at 1-week and 6-weeks post-tPBM, when NAA levels in ACC were higher. A relationship between presence of PTSD and reduced NAA in ACC has been reported [111, 112].
To our knowledge, this is the first report of increasing NAA in the cortex (ACC) following a series of red/NIR tPBM treatments. Reduced NAA in the brain has been reported in aging, mild cognitive impairment, AD [113], blast-TBI with memory problems (in hippocampus) [114, 115], retired NFL players symptomatic for behavior/mood disturbances (parietal white matter) [116], and in all levels of TBI [117]. In athletes with a recent mTBI (one concussion), reduced NAA was present in frontal WM after 3 days, with return to normal at 30 days; however, at 45 days, if a second concussion had occurred within 2 weeks [118]. These athletes self-reported “ready to play” at 3 days. Increase towards normal NAA levels was slower the first two weeks, and faster, the second two weeks [119]. In animal studies with repeat mTBIs, low ATP and ADP were reported [120]; PBM is known to increase ATP in hypoxic/stressed cells [33, 34]. tPBM should be considered in treatment of acute (non-hemorrhagic) TBI.
Application of tPBM to increase NAA in ACC may be especially important for ex-football players with RHI primarily to frontal lobes, where p-tau deposits are present even in early-stage CTE [1, 2, 6, 20]. Indirect RHI to ACC could have occurred, considering the manner football was played 35–55 years ago. For P2, it may be particularly relevant that he played tackle, offensive lineman, e.g., a position with higher incidence of severe CTE pathology postmortem [6]. P2 also had AFE to tackle football at less than age 12 (age 7, Pop Warner), and he played American football for 15 years; both factors posit increased risk for CTE postmortem [21, 25].
Possible cellular changes
There was likely increased production of ATP by mitochondria in cortical neurons exposed to NIR photons during a tPBM treatment series, thus improving cellular oxygenation and respiration [27, 28, 33, 34]. In addition, there was likely release of NO from CCO in the mitochondria of hypoxic/compromised cells [32], thus increasing vasodilation in local blood and lymphatic vessels. Reduced inflammation and oxidative damage would be expected [46].
There was also likely increased BDNF following a tPBM series. BDNF is a neurotrophin associated with neural regeneration, dendritic sprouting, reconnection, and improved synaptic efficacy [48]. Dysregulation of BDNF has been observed both in TBI and PTSD in humans [121]. A significantly lower level of plasma BDNF has been reported in PTSD, suggesting involvement of BDNF in the pathophysiology of PTSD [122]. Here, there was a significant decrease in symptoms of PTSD at 1 month after the initial, in-office tPBM series in the four, ex-football players as a group. It is possible increased BDNF was present at that time.
In addition, there may have been improved function of “fast-spiking interneurons,” post- tPBM. Gamma oscillations (approximately 30 to 100 Hz) are present in many brain regions, including hippocampus and neocortex, with rhythmic and synchronous fluctuations common to most or all neurons in a neuronal network [123]. These fluctuations are associated with precise synaptic interactions of excitatory pyramidal cells and inhibitory GABAergic interneurons. Action potentials generated at high frequency in these fast-spiking interneurons require high oxygen consumption. They are particularly enriched with mitochondria and CCO to accommodate this. Nakazono et al. [124] have suggested that gamma oscillations may offer a future therapeutic target. In the present study, it is possible that mitochondrial function in these critical, fast-spiking interneurons was improved following each series of tPBM treatments. The addition of at-home tPBM treatments (with 40-Hz gamma frequency) long-term would have provided continued delivery of NIR photons to compromised cortex, including interneurons. This may have contributed to continued well-being for 2–4 years thus far, in these ex-football players who have continued home treatments with tPBM.
Potential for tPBM application to superior sagittal sinus, to drain lymphatics and p-tau
The potential for tPBM to augment brain glymphatic drainage has been reviewed [125]. In the present study, it is likely there was dilation of lymphatic vessels, as well as blood vessels in the dura, within the superior sagittal sinus area (Fig. 12). Meningeal lymphatic vessels participate in waste clearance [36, 39, 40]. In advancing CTE, there is increasing accumulation of p-tau [2]. There are direct vascular channels connecting skull bone marrow and the brain surface enabling myeloid cell migration [126].
Fig. 12
A) Frontal, coronal diagram of blood vessels including valveless, emissary veins in superior sagittal sinus. Emissary veins connect the extracranial venous system with intracranial venous sinuses, including direct passage from external scalp to meninges. Application of NIR photons promotes vasodilation of local blood vessels [61,71,72]. B) Frontal, coronal MRI of lymphatic vessels in superior sagittal sinus [134]. C) Sagittal MRI showing location of lymphatic vessels along superior sagittal sinus, rostral-to-caudal [134]. Application of NIR photons delivered here, are hypothesized to promote vasodilation of lymphatic vessels in dura mater [125]. This could assist in removing abnormal protein deposits, including p-tau present in CTE. Permissions: A) reprinted with authors’ permission [58]; schematic diagram by C. Foltz, based on plates from Grey’s Anatomy, Oxford University Press, public domain; B) and C) reprinted from open-access article [134].
![A) Frontal, coronal diagram of blood vessels including valveless, emissary veins in superior sagittal sinus. Emissary veins connect the extracranial venous system with intracranial venous sinuses, including direct passage from external scalp to meninges. Application of NIR photons promotes vasodilation of local blood vessels [61,71,72]. B) Frontal, coronal MRI of lymphatic vessels in superior sagittal sinus [134]. C) Sagittal MRI showing location of lymphatic vessels along superior sagittal sinus, rostral-to-caudal [134]. Application of NIR photons delivered here, are hypothesized to promote vasodilation of lymphatic vessels in dura mater [125]. This could assist in removing abnormal protein deposits, including p-tau present in CTE. Permissions: A) reprinted with authors’ permission [58]; schematic diagram by C. Foltz, based on plates from Grey’s Anatomy, Oxford University Press, public domain; B) and C) reprinted from open-access article [134].](https://content.iospress.com:443/media/adr/2023/7-1/adr-7-1-adr220022/adr-7-adr220022-g012.jpg)
During the initial in-office series, the entire midline, superior sagittal sinus was treated with red/NIR photons (Fig. 1, Protocol A or C). Thus, focal vasodilation of lymphatic and blood vessels likely occurred there. This would be compatible with results from a study with AD mice, where clearance of amyloid-β via the lymphatic system of the brain and the neck was observed following application of NIR laser (1267 nm, 32 J/cm2) to the frontal scalp area [41].
When the ex-football players later used only the Neuro Gamma device at home, treating only cortical nodes of the DMN, they continued to improve (P1, P2). The Neuro Gamma uses only two NIR diode placements on the midline, superior sagittal sinus area, e.g., 1) midline, bilateral mPFC, and 2) midline, bilateral precuneus. It is possible that placement of only two LEDs over the superior sagittal sinus - anterior (frontal) and posterior (high parietal), was adequate to dilate lymphatic and blood vessels within the dura to promote continued waste clearance. Application of tPBM to the confluence of sinuses area (midline, near occipital protuberance) could have an additive effect to further promote waste removal.
Ex-football players who played 35–55 years ago are increasing in age. Increasing age has been associated with a dramatic decline in the efficiency of exchange between subarachnoid cerebrospinal fluid and brain parenchyma as part of waste removal. This contributes to impaired glymphatic clearance, cognitive decline, and dementia among the elderly [127, 128]. It has been suggested that improving glymphatic clearance “is a novel therapeutic target for treatment of neurodegenerative diseases with accumulation of misfolded protein aggregates” [127]. The glymphatic system is more effective during sleep [129]; a recent study with mice has reported an associated circadian rhythm for this [130]. Impaired sleep was a major complaint for the four ex-football players at entry into this tPBM study. There was significant improvement in sleep at 1 month after the in-office tPBM series. Their improved sleep may have been associated with improved glymphatic clearance of waste products. Treating in sync with a person’s own circadian rhythm, perhaps even at night, might have a greater beneficial effect [131].
Conclusions
Overall results are encouraging from this first, case-series report with four ex-football players meeting TES criteria for Possible CTE. Their cognitive and behavior/mood disturbances were well-managed and significantly improved following NIR tPBM treatments. To the authors’ knowledge, this is the first report of a potential treatment modality to mitigate symptoms of Possible CTE. Instead of continuing to worsen over time, these cases improved with tPBM. Without sham-control, a possible placebo effect is unknown. Significant correlations on rs-fcMRI with increased SN FC and improvements in executive function, attention, PTSD, pain, and sleep; and increased CEN FC with verbal learning and memory, less depression; plus increased NAA in ACC on MRS along with less pain and PTSD, all support a beneficial effect from tPBM.
The NINDS Consensus Workshop regarding TES criteria for CTE in vivo, suggested MRI might offer future biomarkers [7]. The present study supports this notion in three areas of MRI: 1) structural MRI showed presence of cavum septum pellucidum; reported in former pro-football players with CTE postmortem [1, 132, 133]; 2) rs-fcMRI showed changes/increased FC in SN and CEN post-tPBM, parallel to improvements; 3) MRS showed changes/increased NAA in ACC.
Limitations and future directions
This was a small, open-protocol case series (n = 4). Future studies with sham and real NIR tPBM devices for self-treatment at home are suggested; NIR photons >700 nm, e.g., 810 nm, are not visible. MRI studies can be used to monitor changes post-tPBM, including FC of intrinsic networks, and MRS metabolites (especially, NAA). There was likely dilation of lymphatic vessels in superior sagittal sinus. Change in lymphatic vessels could be monitored with post- gadobutrol, T2-FLAIR MRI, and subtraction images [134]. Dilation may assist in removal of abnormal protein deposits (p-tau). Reduction in pain medications (narcotic) and tinnitus should be studied. More than one post-testing time point is recommended. Here, there were more instances of significant improvement on cognitive tests after 1 month (twelve), than after 1 week (seven). This pattern was observed in previous tPBM studies with mTBI [59, 60] and Gulf War Illness [135]. Here, decline was present after 2 months in two cases (where data were available); this prompted tPBM home treatments. No negative side effects, adverse or serious adverse events occurred. tLED treatments are safe and can be self-administered at home—here for 2–4 years, to date. Not all concussion cases recover, even after three years [136]. tPBM could be considered for very chronic TBI.
ACKNOWLEDGMENTS
The authors thank Marc Kaufman, PhD, Translational Imaging Laboratory, McLean Hospital for consultation, interneurons; Hannah Lovato, MS for assistance with manuscript preparation; and Anita Saltmarche, BScN, MHSc, MedX Health, Inc.; Lew Lim, PhD, Vielight, Inc.; and James Carroll, THOR Photomedicine, Ltd., for assistance with equipment.
FUNDING
This research was funded in part, by Vielight, Inc., Toronto, Canada; THOR Photomedicine, Ltd., London, UK; VA Boston Healthcare System; and Boston VA Research Institute (BVARI). The research also used LED equipment from the Department of Veterans Affairs, Office of Research and Development, Merit Review Grant Project ID: IO1CX000524, to MN.
MRH was supported by US NIH Grants R01AI050875 and R21AI121700.
CONFLICT OF INTEREST
MRH declares the following potential conflicts of interest: Scientific Advisory Boards: Transdermal Cap, Inc., Cleveland, OH; Hologenix, Inc., Santa Monica, CA; Vielight, Inc., Toronto, Canada; JOOVV, Inc., Minneapolis-St. Paul, MN; Consulting: USHIO Corp., Japan; Sanofi-Aventis Deutschland, GmbH, Frankfurt am Main, Germany.
The other authors have no potential conflicts of interest to declare.
SUPPLEMENTARY MATERIAL
[1] The supplementary material is available in the electronic version of this article: https://dx.doi.org/10.3233/ADR-220022.
REFERENCES
[1] | McKee AC , Stern RA , Nowinski CJ , Stein TD , Alvarez VE , Daneshvar DH , Lee H-S , Wojtowicz SM , Hall G , Baugh CM , Riley DO , Kubilus CA , Cormier KA , Jacobs MA , Martin BR , Abraham CR , Ikezu T , Reichard RR , Wolozin BL , Budson AE , Goldstein LE , Kowall NW , Cantu RC ((2013) ) The spectrum of disease in chronic traumatic encephalopathy. Brain 136: , 43–64. |
[2] | McKee AC , Cairns NJ , Dickson DW , Folkerth RD , Keene CD , Litvan I , Perl DP , Stein TD , Vonsattel J-P , Stewart W , Tripodis Y , Crary JF , Bieniek KF , Dams-O’Connor K , Alvarez VE , Gordon WA ((2016) ) The first NINDS/NIBIB consensus meeting to define neuropathological criteria for the diagnosis of chronic traumatic encephalopathy. Acta Neuropathol 131: , 75–86. |
[3] | Stern RA , Daneshvar DH , Baugh CM , Seichepine DR , Montenigro PH , Riley DO , Fritts NG , Stamm JM , Robbins CA , McHale L , Simkin I , Stein TD , Alvarez VE , Goldstein LE , Budson AE , Kowall NW , Nowinski CJ , Cantu RC , McKee AC ((2013) ) Clinical presentation of chronic traumatic encephalopathy. Neurology 81: , 1122–1129. |
[4] | Stein TD , Alvarez VE , McKee AC ((2014) ) Chronic traumatic encephalopathy: A spectrum of neuropathological changes following repetitive brain trauma in athletes and military personnel. Alzheimers Res Ther 6: , 4. |
[5] | Montenigro PH , Baugh CM , Daneshvar DH , Mez J , Budson AE , Au R , Katz DI , Cantu RC , Stern RA ((2014) ) Clinical subtypes of chronic traumatic encephalopathy: Literature review and proposed research diagnostic criteria for traumatic encephalopathy syndrome. Alzheimers Res Ther 6: , 68. |
[6] | Mez J , Daneshvar DH , Kiernan PT , Abdolmohammadi B , Alvarez VE , Huber BR , Alosco ML , Solomon TM , Nowinski CJ , McHale L , Cormier KA , Kubilus CA , Martin BM , Murphy L , Baugh CM , Montenigro PH , Chaisson CE , Tripodis Y , Kowall NW , Weuve J , McClean MD , Cantu RC , Goldstein LE , Katz DI , Stern RA , Stein TD , McKee AC ((2017) ) Clinicopathological evaluation of chronic traumatic encephalopathy in players of American football. JAMA 318: , 360–370. |
[7] | Katz DI , Bernick C , Dodick DW , Mez J , Mariani ML , Adler CH , Alosco ML , Balcer LJ , Banks SJ , Barr WB , Brody DL , Cantu RC , Dams-O’Connor K , Geda YE , Jordan BD , McAllister TW , Peskind ER , Petersen RC , Wethe JV , Zafonte RD , Foley ÉM , Babcock DJ , Koroshetz WJ , Tripodis Y , McKee AC , Shenton ME , Cummings JL , Reiman EM , Stern RA ((2021) ) National Institute of Neurological Disorders and Stroke Consensus Diagnostic Criteria for Traumatic Encephalopathy Syndrome. Neurology 96: , 848–863. |
[8] | Martland HS ((1928) ) Punch drunk. JAMA 91: , 1103–1107. |
[9] | Omalu BI , DeKosky ST , Minster RL , Kamboh MI , Hamilton RL , Wecht CH ((2005) ) Chronic traumatic encephalopathy in a National Football League player. Neurosurgery 57: , 128–134. |
[10] | McKee AC , Cantu RC , Nowinski CJ , Hedley-Whyte ET , Gavett BE , Budson AE , Santini VE , Lee H-S , Kubilus CA , Stern RA ((2009) ) Chronic traumatic encephalopathy in athletes: Progressive tauopathy after repetitive head injury. J Neuropathol Exp Neurol 68: , 709–735. |
[11] | Omalu BI , Bailes J , Hammers JL , Fitzsimmons RP ((2010) ) Chronic traumatic encephalopathy, suicides and parasuicides in professional American athletes: The role of the forensic pathologist. Am J Forensic Med Pathol 31: , 130–132. |
[12] | Omalu B , Bailes J , Hamilton RL , Kamboh MI , Hammers J , Case M , Fitzsimmons R ((2011) ) Emerging histomorphologic phenotypes of chronic traumatic encephalopathy in American athletes. Neurosurgery 69: , 173–183 discussion 183. |
[13] | McKee AC , Daneshvar DH , Alvarez VE , Stein TD ((2014) ) The neuropathology of sport. Acta Neuropathol 127: , 29–51. |
[14] | Maroon JC , Winkelman R , Bost J , Amos A , Mathyssek C , Miele V ((2015) ) Chronic traumatic encephalopathy in contact sports: A systematic review of all reported pathological cases. PLoS One 10: , 0117338. |
[15] | McKee AC , Alosco ML , Huber BR ((2016) ) Repetitive head impacts and chronic traumatic encephalopathy. Neurosurg Clin N Am 27: , 529–535. |
[16] | Stewart W , McNamara PH , Lawlor B , Hutchinson S , Farrell M ((2016) ) Chronic traumatic encephalopathy: A potential late and under recognized consequence of rugby union? QJM 109: , 11–15. |
[17] | Omalu B , Hammers JL , Bailes J , Hamilton RL , Kamboh MI , Webster G , Fitzsimmons RP ((2011) ) Chronic traumatic encephalopathy in an Iraqi war veteran with posttraumatic stress disorder who committed suicide. Neurosurg Focus 31: , E3. |
[18] | Goldstein LE , Fisher AM , Tagge CA , Zhang X-L , Velisek L , Sullivan JA , Upreti C , Kracht JM , Ericsson M , Wojnarowicz MW , Goletiani CJ , Maglakelidze GM , Casey N , Moncaster JA , Minaeva O , Moir RD , Nowinski CJ , Stern RA , Cantu RC , Geiling J , Blusztajn JK , Wolozin BL , Ikezu T , Stein TD , Budson AE , Kowall NW , Chargin D , Sharon A , Saman S , Hall GF , Moss WC , Cleveland RO , Tanzi RE , Stanton PK , McKee AC ((2012) ) Chronic traumatic encephalopathy in blast-exposed military beterans and a blast neurotrauma mouse model. Sci Transl Med 4: , 134ra60. |
[19] | Tagge CA , Fisher AM , Minaeva OV , Gaudreau-Balderrama A , Moncaster JA , Zhang X-L , Wojnarowicz MW , Casey N , Lu H , Kokiko-Cochran ON , Saman S , Ericsson M , Onos KD , Veksler R , Senatorov VVJ , Kondo A , Zhou XZ , Miry O , Vose LR , Gopaul KR , Upreti C , Nowinski CJ , Cantu RC , Alvarez VE , Hildebrandt AM , Franz ES , Konrad J , Hamilton JA , Hua N , Tripodis Y , Anderson AT , Howell GR , Kaufer D , Hall GF , Lu KP , Ransohoff RM , Cleveland RO , Kowall NW , Stein TD , Lamb BT , Huber BR , Moss WC , Friedman A , Stanton PK , McKee AC , Goldstein LE ((2018) ) Concussion, microvascular injury, and early tauopathy in young athletes after impact head injury and an impact concussion mouse model. Brain 141: , 422–458. |
[20] | Alosco ML , Cherry JD , Huber BR , Tripodis Y , Baucom Z , Kowall NW , Saltiel N , Goldstein LE , Katz DI , Dwyer B , Daneshvar DH , Palmisano JN , Martin B , Cantu RC , Stern RA , Alvarez VE , Mez J , Stein TD , McKee AC ((2020) ) Characterizing tau deposition in chronic traumatic encephalopathy (CTE): Utility of the McKee CTE staging scheme. Acta Neuropathol 140: , 495–512. |
[21] | Alosco ML , Mez J , Tripodis Y , Kiernan PT , Abdolmohammadi B , Murphy L , Kowall NW , Stein TD , Huber BR , Goldstein LE , Cantu RC , Katz DI , Chaisson CE , Martin B , Solomon TM , McClean MD , Daneshvar DH , Nowinski CJ , Stern RA , McKee AC ((2018) ) Age of first exposure to tackle football and chronic traumatic encephalopathy. Ann Neurol 83: , 886–901. |
[22] | Alosco ML , Tripodis Y , Jarnagin J , Baugh CM , Martin B , Chaisson CE , Estochen N , Song L , Cantu RC , Jeromin A , Stern RA ((2016) ) Repetitive head impact exposure and later-life plasma total tau in former National Football League players. Alzheimers Dement 7: , 33–40. |
[23] | Alosco ML , Tripodis Y , Fritts NG , Heslegrave A , Baugh CM , Conneely S , Mariani M , Martin BM , Frank S , Mez J , Stein TD , Cantu RC , McKee AC , Shaw LM , Trojanowski JQ , Blennow K , Zetterberg H , Stern RA ((2018) ) Cerebrospinal fluid tau, Aβ, and sTREM2 in Former National Football League Players: Modeling the relationship between repetitive head impacts, microglial activation, and neurodegeneration. Alzheimers Dement 14: , 1159–1170. |
[24] | Baugh CM , Kiernan PT , Kroshus E , Daneshvar DH , Montenigro PH , McKee AC , Stern RA ((2015) ) Frequency of head-impact-related outcomes by position in NCAA division I collegiate football players. J Neurotrauma 32: , 314–326. |
[25] | Mez J , Daneshvar DH , Abdolmohammadi B , Chua AS , Alosco ML , Kiernan PT , Evers L , Marshall L , Martin BM , Palmisano JN , Nowinski CJ , Mahar I , Cherry JD , Alvarez VE , Dwyer B , Huber BR , Stein TD , Goldstein LE , Katz DI , Cantu RC , Au R , Kowall NW , Stern RA , McClean MD , Weuve J , Tripodis Y , McKee AC ((2020) ) Duration of American football play and chronic traumatic encephalopathy. Ann Neurol 87: , 116–131. |
[26] | Stern RA , Adler CH , Chen K , Navitsky M , Luo J , Dodick DW , Alosco ML , Tripodis Y , Goradia DD , Martin B , Mastroeni D , Fritts NG , Jarnagin J , Devous MD , Mintun MA , Pontecorvo MJ , Shenton ME , Reiman EM ((2019) ) Tau positron-emission tomography in former national football league players. N Engl J Med 380: , 1716–1725. |
[27] | Karu TI , Pyatibrat LV , Afanasyeva NI ((2005) ) Cellular effects of low power laser therapy can be mediated by nitric oxide. Lasers Surg Med 36: , 307–314. |
[28] | Wong-Riley MTT , Liang HL , Eells JT , Chance B , Henry MM , Buchmann E , Kane M , Whelan HT ((2005) ) Photobiomodulation directly benefits primary neurons functionally inactivated by toxins: Role of cytochrome c oxidase. J Biol Chem 280: , 4761–4771. |
[29] | Uozumi Y , Nawashiro H , Sato S , Kawauchi S , Shima K , Kikuchi M ((2010) ) Targeted increase in cerebral blood flow by transcranial near-infrared laser irradiation. Lasers Surg Med 42: , 566–576. |
[30] | Ferraresi C , Parizotto NA , Pires de Sousa MV , Kaippert B , Huang Y-Y , Koiso T , Bagnato VS , Hamblin MR ((2015) ) Light-emitting diode therapy in exercise-trained mice increases muscle performance, cytochrome c oxidase activity, ATP and cell proliferation. J Biophotonics 8: , 740–754. |
[31] | Dmochowski GM , Shereen A (Duke) , Berisha D , Dmochowski JP ((2020) ) Near-infrared light increases functional connectivity with a non-thermal mechanism. Cereb Cortex Commun 1: , tgaa004. |
[32] | Ayuk SM , Houreld NN , Abrahamse H ((2018) ) Effect of 660 nm visible red light on cell proliferation and viability in diabetic modelsunder stressed conditions. Lasers Med Sci 33: , 1085–1093. |
[33] | Karu T , Pyatibrat L , Kalendo G ((1995) ) Irradiation with He-Ne laser increases ATP level in cells cultivated. J Photochem Photobiol B 27: , 219–223. |
[34] | Eells JT , Henry MM , Summerfelt P , Wong-Riley MTT , Buchmann EV , Kane M , Whelan NT , Whelan HT ((2003) ) Therapeutic photobiomodulation for methanol-induced retinal toxicity. Proc Natl Acad Sci U S A 100: , 3439. |
[35] | Semyachkina-Glushkovskaya O , Abdurashitov A , Klimova M , Dubrovsky A , Shirokov A , Fomin A , Terskov A , Agranovich I , Mamedova A , Khorovodov A ((2020) ) Photostimulation of cerebral and peripheral lymphatic functions. Transl Biophotonics 2: , e201900036. |
[36] | Louveau A , Smirnov I , Keyes TJ , Eccles JD , Rouhani SJ , Peske JD , Derecki NC , Castle D , Mandell JW , Lee KS ((2015) ) Structural and functional features of central nervous system lymphatic vessels. Nature 523: , 337–341. |
[37] | Aspelund A , Antila S , Proulx ST , Karlsen TV , Karaman S , Detmar M , Wiig H , Alitalo K ((2015) ) A dural lymphatic vascular system that drains brain interstitial fluid and macromolecules. J Exp Med 212: , 991–999. |
[38] | Louveau A , Herz J , Alme MN , Salvador AF , Dong MQ , Viar KE , Herod SG , Knopp J , Setliff JC , Lupi AL ((2018) ) CNS lymphatic drainage and neuroinflammation are regulated by meningeal lymphatic vasculature. Nat Neurosci 21: , 1380–1391. |
[39] | Raper D , Louveau A , Kipnis J ((2016) ) How do meningeal lymphatic vessels drain the CNS? Trends Neurosci 39: , 581–586. |
[40] | Kipnis J ((2016) ) Multifaceted interactions between adaptive immunity and the central nervous system. Science 353: , 766–771. |
[41] | Zinchenko E , Navolokin N , Shirokov A , Khlebtsov B , Dubrovsky A , Saranceva E , Abdurashitov A , Khorovodov A , Terskov A , Mamedova A ((2019) ) Pilot study of transcranial photobiomodulation of lymphatic clearance of beta-amyloid from the mouse brain: Breakthrough strategies for non-harmacologic therapy of Alzheimer’s disease. Biomed Opt Express 10: , 4003–4017 . |
[42] | Iliff JJ , Chen MJ , Plog BA , Zeppenfeld DM , Soltero M , Yang L , Singh I , Deane R , Nedergaard M ((2014) ) Impairment of glymphatic pathway function promotes tau pathology after traumatic brain injury. J Neurosci 34: , 16180–16193. |
[43] | Sun BL , Wang L , Yang T , Sun J , Mao L , Yang M , Yuan H , Colvin RA , Yang X ((2018) ) Lymphatic drainage system of the brain: A novel target for intervention of neurological diseases. Prog Neurobiol 163-164: , 118–143 |
[44] | Khuman J , Zhang J , Park J , Carroll JD , Donahue C , Whalen MJ ((2012) ) Low-level laser light therapy improves cognitive deficits and inhibits microglial activation after controlled cortical impact in mice. J Neurotrauma 29: , 408–417. |
[45] | Thunshelle C , Hamblin MR ((2016) ) Transcranial low-level laser (light) therapy for brain injury. Photomed Laser Surg 34: , 587–598. |
[46] | Hamblin MR ((2017) ) Mechanisms and applications of the anti-inflammatory effects of photobiomodulation. AIMS Biophys 4: , 337–361. |
[47] | Xuan W , Vatansever F , Huang L , Hamblin MR ((2014) ) Transcranial low-level laser therapy enhances learning, memory, and neuroprogenitor cells after traumatic brain injury in mice. Journal Biomed Opt 19: , 108003. |
[48] | Xuan W , Agrawal T , Huang L , Gupta GK , Hamblin MR ((2015) ) Low-level laser therapy for traumatic brain injury in mice increases brain derived neurotrophic factor (BDNF) and synaptogenesis. J Biophotonics 8: , 502–511. |
[49] | Hamblin MR , Demidova TN ((2006) ) Mechanisms of low level light therapy. Proc SPIE 6140: 614001;https://doi.org/10.1117/12.646294"> . |
[50] | de Freitas LF , Hamblin MR ((2016) ) Proposed mechanisms of photobiomodulation or low-level light therapy. IEEE J Sel Top Quantum Electron 22: , 348–364. |
[51] | Salehpour F , Mahmoudi J , Kamari F , Sadigh-Eteghad S , Rasta SH , Hamblin MR ((2018) ) Brain photobiomodulation therapy: A narrative review. Mol Neurobiol 55: , 6601–6636. |
[52] | Wan S , Anderson RR , Parrish JA ((1981) ) Analytical modeling for the optical properties of the skin withandapplications. Photochem Photobiol 34: , 493–499. |
[53] | Tedford CE , DeLapp S , Jacques S , Anders J ((2015) ) Quantitative analysis of transcranial and intraparenchymal light penetration in human cadaver brain tissue. Lasers Surg Med 47: , 312–322. |
[54] | Jagdeo JR , Adams LE , Brody NI , Siegel DM ((2012) ) Transcranial red and near infrared light transmission in a cadaveric model. PLoS One 7: , e47460. |
[55] | Cassano P , Tran AP , Katnani H , Bleier BS , Hamblin MR , Yuan YS , Fang QQ ((2019) ) Selective photobiomodulation for emotion regulation: Model-based dosimetry study. Neurophotonics 6: , 015004. |
[56] | Yuan YS , Cassano P , Pias M , Fang QQ ((2020) ) Transcranial photobiomodulation with near-infrared light from childhood to elderliness: Simulation of dosimetry. Neurophotonics 7: , 015009. |
[57] | Mester E , Spiry T , Szende B , Tota JG ((1971) ) Effect of laser rays on wound healing. Am J Surg 122: , 532–535. |
[58] | Naeser MA , Saltmarche A , Krengel MH , Hamblin MR , Knight JA ((2011) ) Improved cognitive function after transcranial, light-emitting diode treatments in chronic, traumatic brain injury: Two case reports. Photomed Laser Surg 29: , 351–358. |
[59] | Naeser MA , Zafonte R , Krengel MH , Martin PI , Frazier J , Hamblin MR , Knight JA , Meehan III WP , Baker EH ((2014) ) Significant improvements in cognitive performance post-transcranial, red/near-infrared light-emitting diode treatments in chronic, mild traumatic brain injury: Open-protocol study. J Neurotrauma 31: , 1008–1017. |
[60] | Naeser MA , Martin PI , Ho MD , Krengel MH , Bogdanova Y , Knight JA , Yee MK , Zafonte R , Frazier J , Hamblin MR ((2016) ) Transcranial, red/near-infrared light-emitting diode therapy to improve cognition in chronic traumatic brain injury. Photomed Laser Surg 34: , 610–626. |
[61] | Hipskind SG , Grover FL Jr , Fort TR , Helffenstein D , Burke TJ , Quint SA , Bussiere G , Stone M , Hurtado T ((2019) ) Pulsed transcranial red/near-infrared light therapy using light-emitting diodes improves cerebral blood flow and cognitive function in veterans with chronic traumatic brain injury: A case series. Photomed Laser Surg 37: , 77–84. |
[62] | Chao LL , Barlow C , Karimpoor M , Lim L ((2020) ) Changes in brain function and structure after self-administered home photobiomodulation treatment in a concussion case. Front Neurol 11: , 952. |
[63] | Figueiro Longo MG , Tan CO , Chan S-T , Welt J , Avesta A , Ratai E , Mercaldo ND , Yendiki A , Namati J , Chico-Calero I , Parry BA , Drake L , Anderson R , Rauch T , Diaz-Arrastia R , Lev M , Lee J , Hamblin M , Vakoc B , Gupta R ((2020) ) Effect of transcranial low-level light therapy vs sham therapy among patients with moderate traumatic brain injury: A randomized clinical trial. JAMA Netw Open 3: , e2017337. |
[64] | Saltmarche AE , Naeser MA , Ho KF , Hamblin MR , Lim L ((2017) ) Significant improvement in cognition in mild to moderately severe dementia cases treated with transcranial plus intranasal photobiomodulation: Case series report. Photomed Laser Surg 35: , 432–441. |
[65] | Chao LL ((2019) ) Effects of home photobiomodulation treatments on cognitive and behavioral function, cerebral perfusion, and resting-state functional connectivity in patients with dementia: A pilot trial. Photobiomodul Photomed Laser Surg 37: , 133–141. |
[66] | Salehpour F , Hamblin MR , DiDuro JO ((2019) ) Rapid reversal of cognitive decline, olfactory dysfunction, and quality of life using multi-modality photobiomodulation therapy: Case report. Photobiomodul Photomed Laser Surg 37: , 159–167. |
[67] | Naeser MA , Ho MD , Martin PI , Hamblin MR , Koo B-B ((2020) ) Increased functional connectivity within intrinsic neural networks in chronic stroke following treatment with red/near-infrared transcranial photobiomodulation: Case series with improved naming in aphasia. Photobiomodul Photomed Laser Surg 38: , 115–131. |
[68] | Lampl Y , Zivin JA , Fisher M , Lew R , Welin L , Dahlof B , Borenstein P , Andersson B , Perez J , Caparo C ((2007) ) Infrared laser therapy for ischemic stroke: A new treatment strategy: Results of the NeuroThera Effectiveness and Safety Trial-1 (NEST-1). Stroke 38: , 1843–1849. |
[69] | Zivin JA , Albers GW , Bornstein N , Chippendale T , Dahlof B , Devlin T , Fisher M , Hacke W , Holt W , Ilic S , Kasner S , Lew R , Nash M , Perez J , Rymer M , Schellinger P , Schneider D , Schwab S , Veltkamp R , Walker M , Streeter J ((2009) ) Effectiveness and safety of transcranial laser therapy for acute ischemic stroke. Stroke 40: , 1359–1364. |
[70] | Stemer AB , Huisa BN , Zivin JA ((2010) ) The evolution of transcranial laser therapy for acute ischemic stroke, including a pooled analysis of NEST-1 and NEST-2. Curr Cardiol Rep 12: , 29–33. |
[71] | Schiffer F , Johnston AL , Ravichandran C , Polcari A , Teicher MH , Webb RH , Hamblin MR ((2009) ) Psychological benefits 2 and 4 weeks after a single treatment with near infrared light to the forehead: A pilot study of 10 patients with major depression and anxiety. Behav Brain Funct 5: , 46. |
[72] | Nawashiro H , Wada K , Nakai K , Sato S ((2012) ) Focal increase in cerebral blood flow after treatment with near-infrared light to the forehead in a patient in a persistent vegetative state. Photomed Laser Surg 30: , 231–233. |
[73] | Tian F , Hase SN , Gonzalez-Lima F , Liu H ((2016) ) Transcranial laser stimulation improves human cerebral oxygenation. Lasers Surg Med 48: , 343–349. |
[74] | Wang X , Tian F , Reddy DD , Nalawade SS , Barrett DW , Gonzalez-Lima F , Liu H ((2017) ) Up-regulation of cerebral cytochrome-c-oxidase and hemodynamics by transcranial infrared laser stimulation: A broadband near-infrared spectroscopy study. J Cereb Blood Flow Metab 37: , 3789–3802. |
[75] | Zomorrodi R , Loheswaran G , Pushparaj A , Lim L ((2019) ) Pulsed near infrared transcranial and intranasal photobiomodulation significantly modulates neural oscillations: A pilot exploratory study. Sci Rep 9: , 6309. |
[76] | Ray WJ , Cole HW ((1985) ) EEG alpha activity reflects attentional demands, and beta activity reflects emotional and cognitive processes. Science 228: , 750–752. |
[77] | Coben LA , Danziger W , Storandt M ((1985) ) A longitudinal EEG study of mild senile dementia of Alzheimer type: Changes at 1 year and at 2.5 years. Electoencephalogr Clin Neurophysiol 61: , 101–112. |
[78] | Meghdadi AH , Stevanović Karić M , McConnell M , Rupp G , Richard C , Hamilton J , Salat D , Berka C ((2021) ) Resting state EEG biomarkers of cognitive decline associated with Alzheimer’s disease and mild cognitive impairment. PLoS One 16: , e0244180. |
[79] | Tombaugh TN ((1996) ) Test of memory malingering: TOMM. Multy-Health Systems. |
[80] | Wilkinson GS , Robertson GJ ((2006) ) WRAT 4: Wide range achievement test. Psychological Assessment Resources, Lutz, FL. |
[81] | Reynolds CR ((2002) ) Comprehensive trail making test (CTMT). Pro-Ed, Austin, TX. |
[82] | Spreen O , Benton AL ((1977) ) Neurosensory center comprehensive examination for aphasia (NCCEA), 1977 revision: Manual of instructions. Neuropsychology Laboratory, University of Victoria, Victoria, B.C. |
[83] | Benton A , Hamsher K , Sivan AB ((1989) ) Multilingual Aphasia Examination. AJA Associates, Iowa City, IA. |
[84] | Delis D , Kramer J , Kaplan E , Ober B ((2007) ) California verbal learning test–second edition. Adult version Manual. The Psychological Corporation, San Antonio, TX. |
[85] | Delis DC , Kaplan E , Kramer JH ((2001) ) Delis-Kaplan executive function system: Technical manual. Harcourt Assessment Company, San Antonio, TX. |
[86] | Melzack R ((1987) ) The short-form McGill pain questionnaire. Pain 30: , 191–197. |
[87] | Grashow R , Weisskopf MG , Baggish A , Speizer FE , Whittington AJ , Nadler L , Connor A , Keske R , Taylor H , Zafonte R ((2020) ) Premortem chronic traumatic encephalopathy diagnoses in professional football. Ann Neurol 88: , 106–112. |
[88] | Blanchard EB , Jones–Alexander J , Buckley TC , and Forneris CA ((1996) ) Psychometric properties of the PTSD Checklist (PCL). Behav Res Ther 34: , 669–673. |
[89] | Monson CM , Gradus JL , Young-Xu Y , Schnurr PP , Price JL , Schumm JA ((2008) ) Change in posttraumatic stress disorder symptoms: Do clinicians and patients agree? Psychol Assess 20: , 131. |
[90] | Schiffer F , Reichmann W , Flynn E , Hamblin MR , McCormack H ((2020) ) A novel treatment of opioid cravings with an effect size of.73 for unilateral transcranial photobiomodulation over sham. Front Psychiatry 11: , 827. |
[91] | Shimizu M , Matsuzuka T , Matsumi F , Ogawa H , Murono S ((2018) ) Change of tinnitus with xenon phototherapy of the stellate ganglion. Photomed Laser Surg 36: , 468–471. |
[92] | Simmons M , Frondoza C , Coyle J ((1991) ) Immunocytochemical localization of N-acetyl-aspartate with monoclonal antibodies. Neuroscience 45: , 37–45. |
[93] | Liebel SW , Johnson PK , Lindsey HM , Russell HA , Hovenden ES , Velez C , Carr LS , Wilde EA , Tate DF ((2022) ) Abstract-25. Transcranial photobiomodulation treatment effects in former athletes with repetitive head hits. Arch Clin Neuropsychol. 37: , 1066. |
[94] | Morries LD , Cassano P , Henderson TA ((2015) ) Treatments for traumatic brain injury with emphasis on transcranial near-infrared laser phototherapy. Neuropsychiatr Dis Treat 11: , 2159. |
[95] | Menon V , Uddin LQ ((2010) ) Saliency, switching, attention and control: A network model of insula function. Brain Struct Funct 214: , 655–667. |
[96] | Bonnelle V , Leech R , Kinnunen KM , Ham TE , Beckmann CF , De Boissezon X , Greenwood RJ , Sharp DJ ((2011) ) Default mode network connectivity predicts sustained attention deficits after traumatic brain injury. J Neurosci 31: , 13442–13451. |
[97] | Bonnelle V , Ham TE , Leech R , Kinnunen KM , Mehta MA , Greenwood RJ , Sharp DJ ((2012) ) Salience network integrity predicts default mode network function after traumatic brain injury. Proc Natl Acad Sci U S A 109: , 4690–4695. |
[98] | Stevens MC , Lovejoy D , Kim J , Oakes H , Kureshi I , Witt ST ((2012) ) Multiple resting state network functional connectivity abnormalities in mild traumatic brain injury. Brain Imaging Behav 6: , 293–318. |
[99] | Borsook D , Edwards R , Elman I , Becerra L , Levine J ((2013) ) Pain and analgesia: The value of salience circuits. Prog Neurobiol 104: , 93–105. |
[100] | Otti A , Guendel H , Wohlschläger A , Zimmer C , Noll-Hussong M ((2013) ) Frequency shifts in the anterior default mode network and the salience network in chronic pain disorder. BMC Psychiatry 13: , 84. |
[101] | Hutchison WD , Davis KD , Lozano AM , Tasker RR , Dostrovsky JO ((1999) ) Pain-related neurons in the human cingulate cortex. Nat Neurosci 2: , 403–405. |
[102] | Vogt BA ((2005) ) Pain and emotion interactions in subregions of the cingulate gyrus. Nat Rev Neurosci 6: , 533–544. |
[103] | Shin LM , Whalen PJ , Pitman RK , Bush G , Macklin ML , Lasko NB , Orr SP , McInerney SC , Rauch SL ((2001) ) An fMRI study of anterior cingulate function in posttraumatic stress disorder. Biol Psychiatry 50: , 932–942. |
[104] | Nutt DJ , Malizia AL ((2004) ) Structural and functional brain changes in posttraumatic stress disorder. J Clin Psychiatry 65: (Suppl 1), 11–17. |
[105] | Shin LM , Wright CI , Cannistraro PA , Wedig MM , McMullin K , Martis B , Macklin ML , Lasko , NB , Cavanagh SR , Krangel TS , Orr SP , Pitman RK , Whalen PJ , Rauch SL ((2005) ) A functional magnetic resonance imaging study of amygdala and medial prefrontal cortex responses to overtly presented fearful faces in posttraumatic stress disorder. Arch Gen Psychiatry 62: , 273–281. |
[106] | Abdallah CG , Averill CL , Ramage AE , Averill LA , Goktas S , Nemati S , Krystal JH , Roache JD , Resick PA , Young-McCaughan S ((2019) ) Salience network disruption in US Army soldiers with posttraumatic stress disorder. Chronic Stress (Thousand Oaks) 3: , 2470547019850467. |
[107] | Zanto TP , Gazzaley A ((2013) ) Fronto-parietal network: Flexible hub of cognitive control. Trends Cogn Sci 17: , 602–603. |
[108] | Hwang J , Egorova N , Yang X , Zhang W , Chen J , Yang X , Hu L , Sun S , Tu Y , Kong J ((2015) ) Subthreshold depression is associated with impaired resting-state functional connectivity of the cognitive control network. Transl Psychiatry 5: , e683. |
[109] | Schultz DH , Ito T , Solomyak LI , Chen RH , Mill RD , Anticevic A , Cole MW ((2018) ) Global connectivity of the fronto-parietal cognitive control network is related to depression symptoms in the general population. Netw Neurosci 3: , 107–123. |
[110] | Zhao X , Xu M , Jorgenson K , Kong J Neurochemical changes in patients with chronic low back pain detected by proton magnetic resonance spectroscopy: A systematic review. Neuroimage Clin ((2017) ) 13: , 33–38. |
[111] | Ham B-J , Chey J , Yoon SJ , Sung Y , Jeong D-U , Ju Kim S , Sim ME , Choi N , Choi I-G , Renshaw PF , Lyoo IK ((2007) ) Decreased N-acetyl-aspartate levels in anterior cingulate and hippocampus in subjects with post-traumatic stress disorder: A proton magnetic resonance spectroscopy study. Eur J Neurosci 25: , 324–329. |
[112] | Swanberg KM , Campos L , Abdallah CG , Juchem C Proton magnetic resonance spectroscopy in post-traumatic stress disorder – updated systematic review and meta-analysis. Chronic Stress ((2022) ) 6: , 1–23. |
[113] | Minati L , Grisoli M , Bruzzone M ((2007) ) MR spectroscopy, functional MRI, and diffusion-tensor imaging in the aging brain: A concetual review. J Geriatr Psychiatry Neurol 20: , 3–21 . |
[114] | Hetherington HP , Hamid H , Kulas J , Ling G , Bandak F , de Lanerolle NC , Pan JW ((2014) ) MRSI of the medial temporal lobe at 7 T in explosive blast mild traumatic brain injury. Magn Reson Med 71: , 1358–1367. |
[115] | Ng TSC , Lin AP , Koerte IK , Pasternak O , Liao H , Merugumala S , Bouix S , Shenton ME ((2014) ) Neuroimaging in repetitive brain trauma. Alzheimers Res Ther 6: , 10. |
[116] | Alosco ML , Tripodis Y , Rowland B , Chua AS , Liao HJ , Martin B , Jarnagin J , Chaisson CE , Pasternak O , Karmacharya S , Koerte IK , Cantu RC , Kowall NK , McKee AC , Shenton ME , Greenwald R , McClean M , Stern RA , Lin , A ((2020) ) A magnetic resonance spectroscopy investigation in symptomatic former NFL players. Brain Imaging Behav 14: , 1419–1429. |
[117] | Joyce JM , Parker LL , Walker R , Harris AD ((2022) ) Magnetic resonance spectroscopy of traumatic brain injury and subconcussive hits: A systematic review and meta-analysis. J Neuotrauma 39: , 1455–1476. |
[118] | Vagnozzi R , Signoretti S , Tavazzi B , Floris R , Ludovici A , Marziali S , Tarascio G , Amorini AM , Di Pietro V , Delfini R , Lazzarino G ((2008) ) Temporal window of metabolic brain vulnerability to concussion: A pilot 1H-magnetic resonance spectroscopic study in concussed athletes-part III. Neurosurgery 62: , 1286–1296. |
[119] | Vagnozzi R , Signoretti S , Cristofori L , Alessandrini F , Floris R , Isgro E , Ria A , Marziale S , Zoccatelli G , Tavazzi B , Del Bolgia F , Sorge R , Broglio SP , McIntosh TK , Lazzarino G ((2010) ) Assessment of metabolic brain damage and recovery following mild traumatic brain injury: A multicentre, proton magnetic resonance spectroscopic study in concussed patients. Brain 133: , 3232–3242. |
[120] | Vagnozzi R , Signoretti S , Tavazzi B , Cimatti M , Amorini AM , Donzelli S , Delfini R , Lazzarino G ((2005) ) Hypothesis of the postconcussive vulnerable brain: Exerimental evidence of its metabolic occurrence. Neurosurgery 57: , 164–171. |
[121] | Kaplan GB , Vasterling JJ , Vedak PC ((2010) ) Brain-derived neurotrophic factor in traumatic brain injury, post-traumatic stress disorder, and their comorbid conditions: Role in pathogenesis and treatment. Behav Pharmacol 21: , 427–437. |
[122] | Dell’Osso L , Carmassi C , Del Debbio A , Dell’Osso MC , Bianchi C , Da Pozzo E , Origlia N , Domenici L , Massimetti G , Marazziti D ((2009) ) Brain-derived neurotrophic factor plasma levels in patients suffering from post-traumatic stress disorder. Prog Neuropsychopharmacol Biol Psychiatry 33: , 899–902. |
[123] | Kann O , Papageorgiou IE , Draguhn A ((2014) ) Highly energized inhibitory interneurons are a central element for information processing in cortical networks. J Cereb Blood Flow Metab 34: , 1270–1282. |
[124] | Nakazono T , Jun H , Blurton-Jones M , Green KN , Igarashi KM ((2018) ) Gamma oscillations in the entorhinal-hippocampal circuit underlying memory, dementia. Neurosci Res 129: , 40–46. |
[125] | Salehpour F , Khademi M , Bragin DE , Diduiro JO ((2022) ) Photobiomodulation therapy and the glymphatic system: Promising applications for augmenting the brain lymphatic drainage system. Int J Mol Sci 23: , 2975. |
[126] | Herisson F , Frodermann V , Courties G , Rohde D , Sun Y , Vandoorne K , Wojtkiewicz GR , Masson GS , Vinegoni C , Kim J , Kim DE , Weissleder R , Swirski FK , Moskowitz MA , Nahrendorf M ((2018) ) Direct vascular channels connect skull bone marrow and the brain surface enabling myeloid cell migration. Nat Neurosci 21: , 1209–1217. |
[127] | Kress BT , Iliff JJ , Xia M , Wang M , Wei HS , Zeppenfeld D , Xie L , Kang H , Xu Q , Liew JA , Plog BA , Ding F , Deane R , Nedergaard M ((2014) ) Impairment of paravascular clearance pathways in the aging brain. Ann Neurol 76: , 845–861. |
[128] | Nedergaard M , Goldman SA ((2020) ) Glymphatic failure as a final common pathway to dementia. Science 370: , 50–56. |
[129] | Xie L , Kang H , Xu Q , Chen MJ , Liao Y , Thiyagarajan M , O’Donnell J , Christensen DJ , Nicholson C , Iliff JJ , Takano T , Deane R , Nedergaard M ((2013) ) Sleep drives metabolic clearance from the adult brain. Science 374: , 373–377. |
[130] | Hablitz LM , Plá V , Giannetto M , Vinitsky HS , Stæger FF , Metcalfe T , Nguyen R , Benrais A , Nedergaard M ((2020) ) Circadian control of brain glymphatic and lymphatic fluid flow. Nat Commun 11: , 4411. |
[131] | Moro C , Valverde A , Dole M , Hoh Kam J , Hamilton C , Liebert A , Bicknell B , Benabid AL , Magistretti P , Mitrofanis J ((2022) ) The effect of photobiomodulation on the brain during wakefulness and sleep. Front Neurosci 16: , 942536. |
[132] | Koerte IK , Hufschmidt J , Muehlmann M , Tripodis Y , Stamm JM , Pasternak O , Giwerc MY , Coleman MJ , Baugh CM , Fritts NG , Heinen F , Lin A , Stern RA , Shenton ME ((2016) ) Cavum septi pellucidi in symptomacic former professional football players. J Neurotrauma 33: , 346–353. |
[133] | Gardner RC , Hess CP , Brus-Ramer M , Possin KL , Cohn-Sheehy BI , Kramer JH , Berger JS , Yaffe K , Miller B , Rabinovici GD ((2016) ) Cavum septum pellucidum in retired American pro-football players. J Neurotrauma 33: , 157–161. |
[134] | Absinta M , Ha S-K , Nair G , Sati P , Luciano NJ , Palisoc M , Louveau A , Zaghloul KA , Pittaluga S , Kipnis J ((2017) ) Human and nonhuman primate meninges harbor lymphatic vessels that can be visualized noninvasively by MRI. Elife 6: , 29738. |
[135] | Martin PI , Chao L , Krengel MH , Ho MD , Yee M , Lew R , Knight J , Hamblin MR and Naeser MA ((2021) ) Transcranial photobiomodulation to improve cognition in Gulf War Illness. Front Neurol 11: , 574386. |
[136] | Hiploylee C , Dufort PA , Davis HS , Wennberg RA , Tartaglia MC , Mikulis D , Hazrati L-N , Tator CH ((2017) ) Longitudinal study of postconcussion syndrome: Not everyone recovers. J Neurotrauma 34: , 1511–1523. |