Is There a Characteristic Autonomic Response During Outbursts of Combative Behavior in Dementia Patients?
Abstract
We sought to determine whether skin conductance level could warn of outbursts of combative behavior in dementia patients by using a wristband device. Two outbursts were captured and are reported here. Although no physiologic parameter measured by the wristband gave advance warning, there is a common pattern of parasympathetic withdrawal (increased heart rate) followed approximately 30 seconds later by sympathetic activation (increased skin conductance). In the literature, a similar pattern occurs in psychogenic non-epileptic seizures. We hypothesize that similar autonomic responses reflect similarities in pathophysiology and that physical activity may partially account for the time course of skin conductance.
INTRODUCTION
The emergence of behavioral disturbances in a dementia patient greatly increases the burden on the caregiver [1]. In addition to disorders of mood, sleep, and thought in dementia, there is a class associated with general agitation that includes wandering, inappropriate sexual behavior, and aggression [2]. Some neurologic disinhibition must be involved in this last class in order for the patient to exhibit previously restrained behavior [3], and removing provocative stimuli seems a better remedy than trying to revive inhibition in a failing brain. However, behavioral disturbances often occur without apparent triggers, and for this reason we have explored physiologic measurements that might warn of an impending disturbance, especially an outburst of combative behavior.
We have evaluated wearable devices that monitor skin conductance in order to determine how well it may predict an impending disturbance. This study captured an outburst of combative behavior in two subjects, both of which we report here. Neither skin conductance nor other physiological parameters measured by this device suggest any way to anticipate the outburst; however, an autonomic pattern common to both outbursts emerged that may have a more general medical interest.
MATERIALS AND METHODS
These two cases were recorded as part of a study of electrodermal activity in Alzheimer’s disease and other forms of dementia. The data reported here were acquired through a wristband [4] (e4, Empatica, Inc., Boston, MA, USA). Skin conductance was measured by injecting a small current through two electrodes on the ventral wrist surface. A 3-axis accelerometer monitored activity. Heart rate was determined by photoplethysmography using two wavelengths in order to compensate for motion artifact. Skin temperature was also measured but not used.
The Institutional Review Board approved the procedures used, all of which conformed to the Declaration of Helsinki. Legal representatives gave informed consent for all participants. Participants were patients in the Geriatric Psychiatry Unit of the Edith Nourse Rogers Memorial Veterans Hospital and would go freely about their activities while wearing the wristband. A research staff member would observe from various distances and record significant target behaviors. Time-stamped data were stored on the device, uploaded to the manufacturer’s server for display, and compared afterwards with the behavioral notes. Data exported as a spreadsheet were read into R [5] for further analysis and display. Seven participants were observed for over 200 hours. The wristband captured only two outbursts of combative behavior which arose suddenly in a patient who had previously been calm, i.e., for whom there was no escalation to the outburst. These two cases are reported here. The Supplementary Material contains details of their medical histories and drug regimens.
RESULTS
Case 1
A 67-year-old African American male veteran presented to the Geriatric Psychiatry Unit with increasingly aggressive behavior and homicidal ideation at home. He suffered from memory loss for ten years prior to admission and was diagnosed by neurology with vascular dementia. The patient had served in Vietnam for one year. Although there was discussion of post-traumatic stress disorder in his medical record, he had not seen combat and had never been diagnosed with post-traumatic stress disorder by a psychiatrist. He had several confrontations with other patients on the unit.
While wearing the wristband, the patient sat quietly in the day room watching television. A veteran standing behind him addressed him using a racial slur. The patient quickly rose to his feet and threatened to kill the other veteran. Staff separated the two and a psychiatric emergency was called. There were no injuries, but the patient was transferred to a more acute psychiatric unit. Figure 1 shows the data recorded during this incident.
Fig. 1
Case 1 physiologic measurements. Panel A shows the time course for total acceleration (norm of vector), skin conductance (scale on left axis), and heart rate (scale on right axis). The provocation occurred at the time of the sharp increase of all three measures around 6350 s (marked by vertical line). Panel B is a magnified view of the time around the provocation, with the heart rate tracing shifted downward. The increase in heart rate begins about 35 s before the change in skin conductance. Note that there is no change in skin conductance prior to the onset.
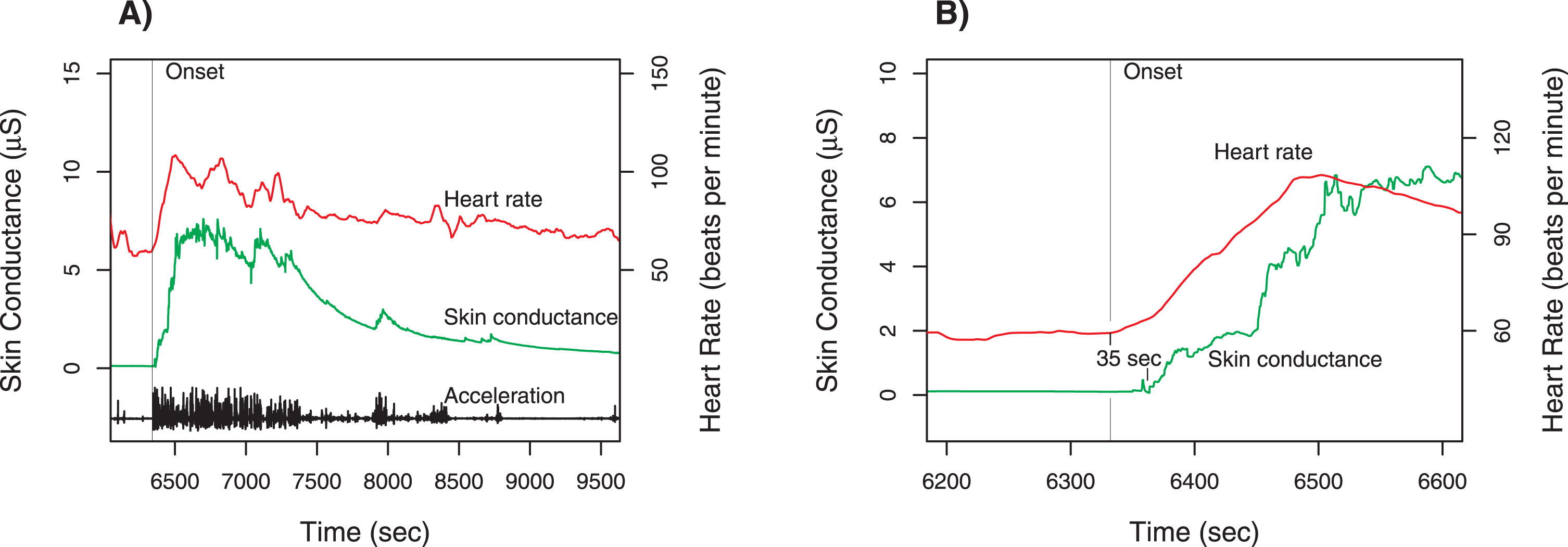
Case 2
An 83-year-old Caucasian male veteran transferred to the Geriatric Psychiatry Unit from a nursing home due to increasingly aggressive behavior. He complained of memory loss for four years and suffered a frontal lobe stroke one year before admission. Neurology diagnosed senile dementia of the Alzheimer’s type with a vascular component. There was no psychiatric history.
The patient was standing in the corridor, talking with two nurses and a research staff member. With no change in affect, he swung his fist at the investigator’s face, striking a clipboard; this outburst did not answer to any observable stimulus. The patient then calmly said to the research staff member: “Here I thought you were a nice guy—and then you go and pull a thing like that....” Although the patient glowered for a brief period afterwards, there were no significant agitated movements, and eventually he left the group to walk around the unit. The data recorded during this incident are displayed in Fig. 2.
Fig. 2
Case 2 physiologic measurements. Panel A shows the time course for total acceleration (norm of vector), skin conductance (scale on left axis) and heart rate (scale on right axis). The unprovoked swing occurred at the time of the sharp increase in heart rate and a burst of activity around 8,550 s marked by vertical line); there is a step decrease in skin conductance, indicating a break in the contact between the skin and electrodes. Contact returns about 8,640 s, and the time course of skin conductance is displayed afterwards. Panel B is a magnified view of the time around the swing, with the heart rate tracing shifted downward. To estimate the onset of the increase in skin conductance, the time course before and after the disruption are linearly extrapolated; the increase in skin conductance is estimated to begin 27 s after the change in heart rate. Note that there is no change in skin conductance prior to the onset.
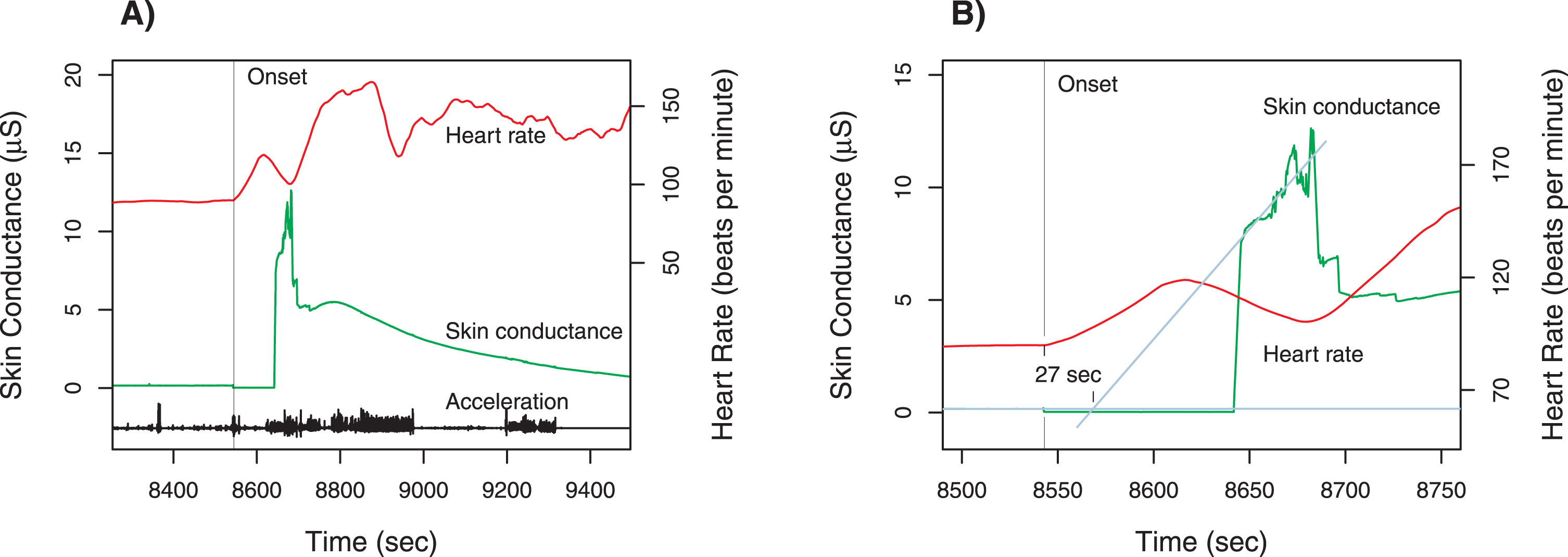
The two figure legends discuss the details of each case. We believe the most significant result is the similarity of the latency between heart rate increase and skin conductance increase (35 s and 27 s for Cases 1 and 2). Comparison of peak-to-baseline ratios further confirms that the two responses are similar for heart rate ratios (1.7 and 1.8 for Cases 1 and 2) and for skin conductance ratios (56.8 and 54.9 for Cases 1 and 2). Further details on the ratios are given in Supplementary Table 1.
DISCUSSION
In both cases, there is witnessed behavior marking an abrupt transition from a calm to an agitated state, which separates these from the more common scenario of uncooperative behavior escalating into combative behavior. These observed acts coincide with data recorded on the wearable device through the accelerometer readings and, in Case 2, through the temporary break of electrode contact. The pattern common to both cases is that a sustained increase in heart rate begins at the time of the transition to an agitated state, well before the change in skin conductance. Regarding the motivation that led to these measurements, the physiological parameters monitored by the device do not suggest any method to anticipate the outburst of Case 2. However, the existence of a common autonomic pattern for provoked and unprovoked responses is intriguing, and an exploration of what factors may be at play adds insight into these measurements.
In humans, the intrinsic rate of the sinoatrial pacemaker is about 100 beats per minute [6]. At rest parasympathetic tone usually exceeds sympathetic, and the resulting heart rate is well below 100 [6]. In animal studies, a change in parasympathetic tone may alter the heart rate in a fraction of a second, whereas sympathetic tone takes two orders of magnitude longer (20–30 s) [6, 7]; the concepts developed in animal experiments apply well to human physiology [6]. The accelerometer data associate the onset of the outburst with the increase in heart rate (Figs. 1A and 2A). This is particularly interesting in Case 2 where there was no apparent stimulus. A burst of accelerometer activity and the break in electrode contact, both associated with the swing of the fist, occurred simultaneously with the increase in heart rate (Fig. 2A). Similarly, in Case 1, the accelerometer activity corresponding to the patient’s rising to his feet coincided with the increase in heart rate (Fig. 1A). This coincidence of accelerometer activity and onset of tachycardia does not allow the 20–30 s necessary for sympathetic tone to affect the heart rate. It follows that these two combative outbursts—one with a clear trigger, the other without—both began with a withdrawal of parasympathetic tone.
In contrast, skin conductance is controlled by the sympathetic nervous system [8–10]. Electrical conductance is increased by the secretions of the sweat glands [11]. The basic physiologic purpose of this system is thermoregulation [12], and sympathetic tone answers either to an actual increase in metabolic rate or to an anticipated one. The time course of skin conductance may thus be classified as slow changes in baseline (tonic) and brief changes lasting a few seconds (phasic) [12]; phasic changes may occur spontaneously or in response to a stimulus like an unexpected loud noise. In experiments designed to evoke phasic changes, the interval between stimulus and response (latency) is less than 5 s [13]. Assuming that the autonomic response begins with the increase in heart rate, the latency of the skin conductance response (35 s and 27 s, respectively, for the two cases) is much greater than that of a phasic response. On the other hand, once initiated, the change in skin conductance occurs much faster than tonic baseline drift (Figs. 1 and 2 before onset). These considerations raise the question of what factors determine the time course of skin conductance during an outburst of combative behavior. Comparison of these data with other paroxysmal behavior can integrate these results into the literature. We will briefly consider panic attacks, startle response, and seizures.
Although panic attacks are associated with in-creased heart rate and other signs of sympathetic activation [14, 15], we were unable to find any published results concerning skin conductance during an attack. However, one report indicated that a panic attack may start before or after the onset of sinus tachycardia in the same individual [16], which makes panic attacks dissimilar to the outbursts described here.
Concerning startle response, one informative study used a simulated automobile crash to evaluate it and found that tachycardia begins at the moment of the crash and skin conductance increases with a latency of 2-3 s [17]. Therefore, startle response appears to be a special case of the paradigms that evoke phasic responses of skin conductance [18].
Many studies have demonstrated that seizures are associated with increased heart rate and skin conductance [19–27]. In particular, heart rate and skin conductance may distinguish between epileptic seizures (ES) and psychogenic non-epileptic seizures (PNES) [26]. Epileptic seizures are by definition associated with abnormal electroencephalograms (EEGs); during epileptic seizures there is a significant increase in skin conductance [19, 26]. On the other hand, for PNES there is no abnormality detected by EEG [24, 25]. Patients suffering from PNES show much more variability in skin conductance during a “seizure” than those with ES, although frequently a significant increase does occur (Zsom A, personal communication, 2021). Reinsberger and colleagues [21] studied the relationship of heart rate and seizure type and determined that a distinguishing feature of PNES is that the heart rate increases 30 s before the “seizure,” that is, before the period associated with elevated skin conductance. This is the same pattern noted in the two cases of combative behavior. The latency periods are 35 and 27 s; although approximate, they are nonetheless consistent with the heart rate in PNES patients being elevated 30 s before the “seizure”.
We hypothesize that physical activity may partially account for the time course of skin conductance in both combative outbursts and PNES. Exercise not only increases heart rate [28] but also leads to increased skin conductance [29, 30], clearly a thermoregulatory response to increased metabolic demand. Published data [30] confirm an expected positive correlation between level of exercise and skin conductance; however, we could find no literature concerning the latency between a change in level of activity and resultant change in skin conductance. Nonetheless, it is plausible that increased activity accounts for the increased skin conductance in Case 1 and the variability in the responses of patients with PNES. However, this explanation would not readily account for all aspects of Case 2 because there was a significant increase in skin conductance but minimal change in physical activity.
Even if incomplete, the hypothesis that combative outbursts in dementia patients and PNES share a common autonomic pattern may nonetheless be useful. Although we examined PNES because of its paroxysmal nature, the hypothesis leads to a broader perspective that includes movement disorders and seizures in neurodegenerative diseases [31]. For example, in juvenile Huntington’s disease, one study reported that 16 patients had seizures but 7 of these had no epileptiform activity on EEG [32]; it would be interesting to learn what their autonomic response is during a seizure in this genetically determined disease. Many genes have been linked to cellular pathways that are central to the pathologic mechanisms of neurodegenerative diseases [33]. It is well established that different genes may lead to the highly similar phenotypes, on both pathologic and psychiatric levels [34, 35]. Genetic studies may also suggest a variety of candidate drugs as possible treatments [33]. Although the study of the genetics of PNES has only recently begun [36], our hypothesis provides a framework for integrating results with those of neurodegenerative diseases. From a practical perspective, wristband devices that monitor skin conductance and heart rate are ideal for monitoring this autonomic response. However, accelerometer activity may not accurately capture the amount of energy expended by physical activity. Video monitoring may be necessary. In a different clinical setting, analysis of skin conductance has successfully classified behavioral disturbances of autistic children [37]; therefore, it is plausible that analysis of heart rate and skin conductance may lead to a subclassification of PNES.
In conclusion, we have studied combative outbursts in two dementia patients and found a common autonomic pattern in both. Review of the literature suggests that there is a similar autonomic pattern in PNES. We posit that the autonomic responses in combative outbursts and PNES are the same and that physical activity may partly account for this pattern. The mechanism proposed is that a trigger (external in Case 1 but internal in Case 2 and PNES) causes an increased heart rate within a fraction of a second (parasympathetic withdrawal); increased skin conductance (sympathetic activation) follows with a delay which measurement shows to be longer than that of a phasic response.
ACKNOWLEDGMENTS
The research described here was supported by the Department of Veterans Affairs, Veterans Health Administration, Rehabilitation Research and Development Service, Award number RX001932-01, to Frank A. Greco, MD, PhD at the VA Bedford Healthcare System. Any opinions expressed are those of the authors alone. The authors thank Dr. A. Mandell for his critical review of the manuscript.
CONFLICT OF INTEREST
The authors have no conflict of interest to report.
SUPPLEMENTARY MATERIAL
[1] The supplementary material is available in the electronic version of this article: https://dx.doi.org/10.3233/ADR-210007.
REFERENCES
[1] | Cheng ST ((2017) ) Dementia caregiver burden: A research update and critical analysis. Curr Psychiatry Rep 19: , 64. |
[2] | Desai AK , Schwartz L , Grossberg GT ((2012) ) Behavioral disturbance in dementia. Curr Psychiatry Rep 14: , 298–309. |
[3] | Lesser JM , Hughes S ((2006) ) Psychosis-related disturbances. Psychosis, agitation, and disinhibition in Alzheimer’s disease: Definitions and treatment options. Geriatrics 61: , 14–20. |
[4] | van Lier HG , Pieterse ME , Garde A , Postel MG , de Haan HA , Vollenbroek-Hutten MMR , Schraagen JM , Noordzij ML ((2020) ) A standardized validity assessment protocol for physiological signals from wearable technology: Methodological underpinnings and an application to the E4 biosensor. Behav Res Methods 52: , 607–629. |
[5] | R Development Core Team (2019) R foundation for Statistical Computing, Vienna, Austria. |
[6] | Lahiri MK , Kannankeril PJ , Goldberger JJ ((2008) ) Assessment of autonomic function in cardiovascular disease: Physiological basis and prognostic implications. J Am Coll Cardiol 51: , 1725–1733. |
[7] | Tuttle RS , Kelts AP ((1964) ) Periodic versus continuous stimulation of cat sympathetic preganglionic nerves on heaert rate and contraction of the nictitating membrane. Life Sci (1962) 3: , 421–430. |
[8] | Boucsein W , Fowles DC , Grimnes S , Ben-Shakhar G , roth WT , Dawson ME , Filion DL ((2012) ) Publication recommendations for electrodermal measurements. Psychophysiology 49: , 1017–1034. |
[9] | Koss MC , Davison MA ((1976) ) Characteristics of the electrodermal response. A model for analysis of central sympathetic reactivity. Naunyn Schmiedebergs Arch Pharmacol 295: , 153–158. |
[10] | Vetrugno R , Liguori R , Cortelli P , Montagna P ((2003) ) Sympathetic skin response: Basic mechanisms and clinical applications. Clin Auton Res 13: , 256–270. |
[11] | Sugenoya J , Iwase S , Mano T , Ogawa T ((1990) ) Identification of sudomotor activity in cutaneous sympathetic nerves using sweat expulsion as the effector response. Eur J Appl Physiol Occup Physiol 61: , 302–308. |
[12] | Boucsein W ((2012) ) Electrodermal Activity, Springer, New York. |
[13] | Maulsby RL , Edelberg R ((1960) ) The interrelationship between the galvanic skin response, basal resistance, and temperature. J Comp Physiol Psychol 53: , 475–479. |
[14] | Wilkinson DJ , Thompson JM , Lambert GW , Jennings GL , Schwarz RG , Jefferys D , Turner AG , Esler MD ((1998) ) Sympathetic activity in patients with panic disorder at rest, under laboratory mental stress, and during panic attacks. Arch Gen Psychiatry 55: , 511–520. |
[15] | Barsky AJ ((1992) ) Palpitations, cardiac awareness, and panic disorder. Am J Med 92: , 31s–34s. |
[16] | Taylor CB , Sheikh J , Agras WS , Roth WT , Margraf J , Ehlers A , Maddock RJ , Gossard D ((1986) ) Ambulatory heart rate changes in patients with panic attacks. Am J Psychiatry 143: , 478–482. |
[17] | Mang DW , Siegmund GP , Blouin JS ((2014) ) Whiplash evokes descending muscle recruitment and sympathetic responses characteristic of startle. J Can Chiropr Assoc 58: , 109–118. |
[18] | Blain S , Power SD , Sejdic E , Mihailidis A , Chau T ((2010) ) A cardiorespiratory classifier of voluntary and involuntary electrodermal activity. Biomed Eng Online 9: , 11. |
[19] | Poh MZ , Loddenkemper T , Swenson NC , Goyal S , Madsen JR , Picard RW ((2010) ) Continuous monitoring of electrodermal activity during epileptic seizures using a wearable sensor. Annu Int Conf IEEE Eng Med Biol Soc 2010: , 4415–4418. |
[20] | Opherk C , Hirsch LJ ((2002) ) Ictal heart rate differentiates epileptic from non-epileptic seizures. Neurology 58: , 636–638. |
[21] | Reinsberger C , Perez DL , Murphy MM , Dworetzky BA ((2012) ) Pre- and postictal, not ictal, heart rate distinguishes complex partial and psychogenic nonepileptic seizures. Epilepsy Behav 23: , 68–70. |
[22] | Poh MZ , Loddenkemper T , Reinsberger C , Swenson NC , Goyal S , Madsen JR , Picard RW ((2012) ) Autonomic changes with seizures correlate with postictal EEG suppression. Neurology 78: , 1868–1876. |
[23] | Reinsberger C , Sarkis R , Papadelis C , Doshi C , Perez DL , Baslet G , Loddenkemper T , Dworetzky BA ((2015) ) Autonomic changes in psychogenic nonepileptic seizures: Toward a potential diagnostic biomarker? Clin EEG Neurosci 46: , 16–25. |
[24] | Sundararajan T , Tesar GE , Jimenez XF ((2016) ) Biomarkers in the diagnosis and study of psychogenic nonepileptic seizures: A systematic review. Seizure 35: , 11–22. |
[25] | Perez DL , LaFrance WC Jr. ((2016) ) Nonepileptic seizures: An updated review. CNS Spectr 21: , 239–246. |
[26] | Zsom A , Tsekhan S , Hamid T , Levin J , Truccolo W , LaFrance WC , Blum AS , Li P , Wahed LA , Shaikh MA , Sharma G , Ranieri R , Zhang L ((2019) ) Ictal autonomic activity recorded via wearable-sensors plus machine learning can discriminate epileptic and psychogenic nonepileptic seizures. Annu Int Conf IEEE Eng Med Biol Soc 2019: , 3502–3506. |
[27] | Vieluf S , Reinsberger C , El Atrache R , Jackson M , Schubach S , Ufongene C , Loddenkemper T , Meisel C ((2020) ) Autonomic nervous system changes detected with peripheral sensors in the setting of epileptic seizures. Sci Rep 10: , 11560. |
[28] | Fagraeus L , Linnarsson D ((1976) ) Autonomic origin of heart rate fluctuations at the onset of muscular exercise. J Appl Physiol 40: , 679–682. |
[29] | Carter JR , Ray CA ((2015) ) Sympathetic neural adaptations to exercise training in humans. Auton Neurosci 188: , 36–43. |
[30] | Posada-Quintero HF , Reljin N , Mills C , Mills I , Florian JP , VanHeest JL , Chon KH ((2018) ) Time-varying analysis of electrodermal activity during exercise. PLoS One 13: , e0198328. |
[31] | Freitas ME , Ruiz-Lopez M , Dalmau J , Erro R , Privitera M , Andrade D , Fasano A ((2019) ) Seizures and movement disorders: Phenomenology, diagnostic challenges and therapeutic approaches. J Neurol Neurosurg Psychiatry 90: , 920–928. |
[32] | Cloud LJ , Rosenblatt A , Margolis RL , Ross CA , Pillai JA , Corey-Bloom J , Tully HM , Bird T , Panegyres PK , Nichter CA , Higgins DS Jr. , Helmers SL , Factor SA , Jones R , Testa CM ((2012) ) Seizures in juvenile Huntington’s disease: Frequency and characterization in a multicenter cohort. Mov Disord 27: , 1797–1800. |
[33] | Gan L , Cookson MR , Petrucelli L , La Spada AR ((2018) ) Converging pathways in neurodegeneration, from genetics to mechanisms. Nat Neurosci 21: , 1300–1309. |
[34] | Deutsch CK , McIlvane WJ ((2012) ) Non-Mendelian etiologic factors in neuropsychiatric illness: Pleiotropy, epigenetics, and convergence. Behav Brain Sci 35: , 363–364. |
[35] | Frega M , Selten M , Mossink B , Keller JM , Linda K , Moerschen R , Qu J , Koerner P , Jansen S , Oudakker A , Kleefstra T , van Bokhoven H , Zhou H , Schubert D , Nadif Kasri N ((2020) ) Distinct pathogenic genes causing intellectual disability and autism exhibit a common neuronal network hyperactivity phenotype. Cell Rep 30: , 173–186.e176. |
[36] | Leu C , Bautista JF , Sudarsanam M , Niestroj LM , Stefanski A , Ferguson L , Daly MJ , Jehi L , Najm IM , Busch RM , Lal D ((2020) ) Neurological disorder-associated genetic variants in individuals with psychogenic nonepileptic seizures. Sci Rep 10: , 15205. |
[37] | Ferguson BJ , Hamlin T , Lantz JF , Villavicencio T , Coles J , Beversdorf DQ ((2019) ) Examining the association between electrodermal activity and problem behavior in severe autism spectrum disorder: A feasibility study. Front Psychiatry 10: , 654. |