The Association Between Anemia of Chronic Inflammation and Alzheimer’s Disease and Related Dementias
Abstract
Background:
Dementia is a spectrum of neurological diseases characterized by memory impairment and cognitive decline with the pathogenesis and effective management remaining elusive. Several studies have identified a correlation between anemia and Alzheimer’s disease and related dementias (ADRD); however, anemia subtypes and association with ADRD have yet to be studied conclusively.
Objective:
To study an association between ADRD and anemia of chronic inflammation.
Methods:
We conducted a retrospective case-control study of the patients, diagnosed with ADRD at Brookdale Hospital. Pair-wise comparisons between means of controls and cases in terms of iron studies and laboratory results were performed using a Mann–Whitney U test. Pair-wise comparisons between anemia subgroups (moderate and severe) were performed using a Two Sample proportion Z-Test, where for each couple of normally distributed population.
Results:
There was a total of 4,517 (1,274 ADRD group; 3,243 Control group) patients. There was significant difference in hemoglobin 10.15 versus 11.04 [p-value <0.001]. Iron studies showed a significant difference in ferritin 395±488.18 versus 263±1023.4 [p < 0.001], total iron binding capacity 225±84.08 versus 266±82.30 [p < 0.001] and serum iron level 64±39.34 versus 53±41.83 [p < 0.001]. Folic acid and vitamin B12 levels were normal in both groups. Severe and moderate anemia in the ADRD group were respectively 6.2% [95% CI: 4.2–8.4] and 13% [95% CI: 9.8–16.2] higher. Overall, incidence of moderate-to-severe anemia was found to be 19% higher in ADRD group [95% CI: 15.8–22.1].
Conclusion:
We demonstrated an association between ADRD and anemia of chronic inflammation independent of age, renal function, and HgbA1C levels.
INTRODUCTION
Dementia consists of a broad spectrum of neurological diseases characterized by memory impairment and cognitive decline which disproportionately affects those in later stages of life. The pathologies under the umbrella of dementia include, but are not limited to, Lewy body dementia (LBD), frontotemporal degeneration (FTD), vascular dementia, and mixed dementias with the most common being Alzheimer’s disease (AD), accounting for up to two-thirds of the cases of dementia [1]. AD currently affects 5.7 million Americans, and with an ever-growing aging population it is expected to affect as many as 13.8 million by 2050 [1, 2]. Death rates from Alzheimer’s disease and related dementia (ADRD) have more than doubled from 30.5 deaths per 100,000 in 2000 to 66.7 in 2017, with a precise pathogenesis and effective treatment remaining unclear [3].
Current evidence suggests a significant overlap between the phenotypes of dementia and their underlying pathoetiologies. For example, there exists a vascular component present on histology in patients with AD, LBD, and FTD, and amyloid-β neuritic plaques (Aβ), neurofibrillary tangles, and Lewy bodies may be found in any type of dementia. Due to a similar clinical picture and pathophysiology AD, vascular dementia, LBD, FTD, and mixed dementia are included under the umbrella of ADRD [4, 5]. Current pharmacotherapies have been unsuccessful in the reduction of morbidity and mortality associated with ADRD, and at best, only temporarily improve symptoms without successfully targeting the degenerative process [2]. Other treatment modalities, such as dietary/nutritional supplements and lifestyle modifications, have also been investigated, but there still remains insufficient evidence to support efficacy [2].
Investigation into other etiologic factors has revealed a possible correlation between anemia and ADRD [7–9]. Despite the wide-ranging severities of anemia and its multiple subtypes, there is minimal literature to define this association. We proposed that there exists an association between ADRD and anemia of chronic inflammation (ACI), and decided to investigate the association between ADRD and different subtypes of anemia, including ACI, iron deficiency anemia (IDA), and vitamin B12 and folic acid deficiency anemia.
MATERIAL AND METHODS
Patient population
To define the relationship between anemia and dementia, we conducted a retrospective case-control observational study. A review of chart data was performed on the electronic medical record at Brookdale University Hospital Medical Center (Epic© EMR Wisconsin, USA) on all patients that were seen within the healthcare system as an outpatient at one point in their clinical history from January 1, 2016 through January 1, 2019. Inclusion criteria were defined as patients who had a diagnosis that fell under the umbrella of ADRDs [Alzheimer’s disease (G30.0), vascular dementia (F01), dementia with Lewy bodies (G31.83), frontotemporal dementia (G31.0), or unspecified dementia (F03.0)]. Patients with any other causes of symptomatology similar to that of dementia due to trauma, infection, and anoxic/metabolic causes which included patients with a diagnosis of diffuse traumatic brain injury (S06.2), anoxic brain damage, not elsewhere classified (G93.1), Other and unspecified encephalopathy (G93.4), metabolic encephalopathy (G93.41), Parkinson’s disease (G20), Creutzfeldt-Jakob disease, unspecified (A81.00), and Huntington’s disease (G10) were excluded from this study.
Fig.1
Charts showing graphical representation of pair-wise comparison of laboratory data between clinical groups with respective p-values shown in Table 1. A) Difference in Hg between two clinical groups is statistically significant. B) Difference in TIBC is statistically significant. C) Difference in serum iron level is statistically significant. D) Difference in ferritin level is statistically significant. E. Difference in HgbA1C is not statistically significant. F. Difference in Cr is statistically, but not clinically significant. G) Folic acid level was normal in both clinical groups. H) B12 level was normal in both clinical groups.
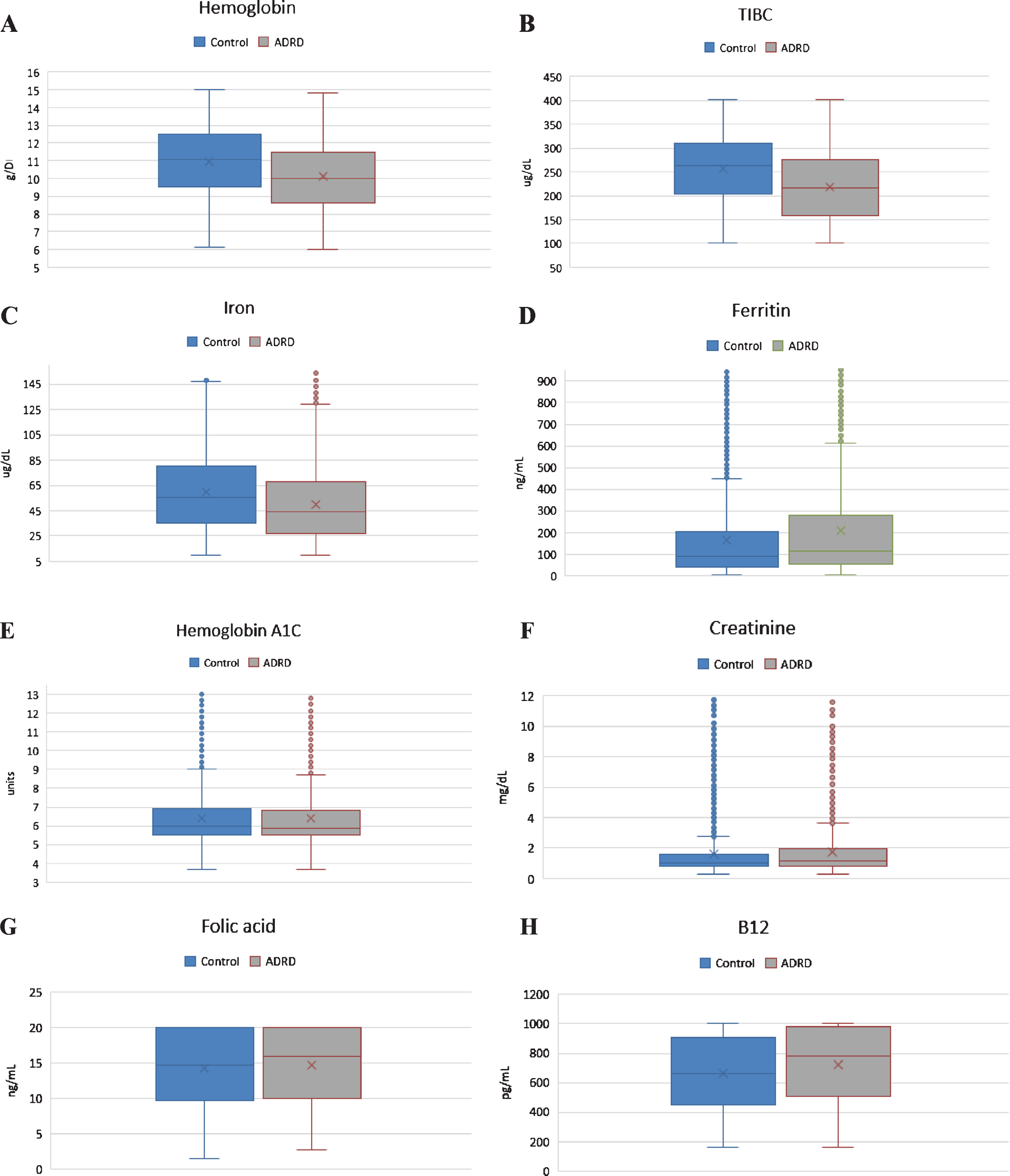
In efforts to ensure patients with anemia secondary to acute blood loss anemia were not considered anyone with a diagnosis of upper and/or lower gastrointestinal bleeding, genitourinary and/or obstetrical bleeding, and traumatic bleeding were not considered for the study. Patients with a diagnosis of malignancy were also not considered for the study due to a high prevalence of malignancy and/or chemotherapy related anemia in this patient population [10]. Patients with hematological disorders such as aplastic anemia, myelodysplastic syndrome, thalassemia, or sickle cell anemia were also not included in the study as presence of these pathologies may act as a cofounder. Patients with a diagnosis of HIV were also not considered for the study due to higher prevalence of the anemia and dementia in this population due to a different pathomechanism [11, 12].
In efforts to ensure that we conducted an age appropriate study, only patients with an age >60 were included in the study as dementia in the younger population often carried a diagnosis/history that would exclude the patient from the study. Controls were defined as patients that were seen during the above-mentioned specified time period that did not carry a diagnosis of exclusion.
Definitions
Anemia was defined in accordance with the adult World Health Organization (WHO) definition of anemia, in terms of hemoglobin (Hb) which included mild, moderate, and severe. For males greater than 15 years of age, categories were as follows: mild 11.0–12.9 g/dL; moderate 8.0–10.9 g/dL; severe <8.0 g/dL. For females, non-pregnant, greater than 15 years of age, categories were as follows: mild 11.0–11.9 g/dL, moderate 8.0–10.9 g/dL severe <8.0 g/dL [13]. Additional information was obtained from chart review to help specify category of anemia, i.e., iron deficiency, vitamin B12, folate deficiency, and anemia of ACI. For all patients with available data, serum iron, total iron binding capacity (TIBC), ferritin, vitamin B12, and folic acid was obtained. To mitigate any confounding factors, serum creatinine (Cr) level and hemoglobin A1C (HgbA1C) were obtained to rule out chronic kidney disease (CKD) and diabetes mellitus (DM) as a possible cause of ACI.
Statistical analysis
All the data are reported as sample means with standard deviations. Pair-wise comparisons between means of controls and cases in terms of Hb, iron, TIBC, folic acid, vitamin B12, Cr, and HgbA1C were performed using a Mann–Whitney U test where the null hypothesis presuming means are equal was tested. Statistical significance was defined as p value <0.05.
Pair-wise comparisons between anemia subgroups (moderate and severe) were performed using a Two Sample proportion Z-Test, where for each couple of normally distributed population, the null hypothesis that the probability for event within each group is identical was verified. Statistical significance in Two Sample proportion Z-Test was defined as a p value less than 0.05 and 95% confidence interval (CI) not including one. Brookdale University Hospital Medical Center Institutional Review Board approval was obtained for this study, IRB approval number 19/04.
RESULTS
A total of 4,517 patients (43% males; 57% females) with a mean age of 75.6±9.9 years were enrolled in the study. Of those, 1,274 patients (42% males; 58% females) with mean age of 80.8±9.4 years with diagnosis of ADRD and 3,243 patients (44% males; 56% females) with mean age of 73.5±9.4 years without a diagnosis of ADRD were included in the study based on exclusion/inclusion criteria.
In our analysis, we examined nine clinical tests relevant to the etiology of the anemia which have been shown in Table 1. Pair-wise comparison of means were analyzed using the Mann–Whitney U test, which revealed that although both groups had low Hb level, there was significant difference in Hb between the ADRD and control group (10.15 g/dL versus 11.06 g/dL, p < 0.001, n = 4517). Both serum iron level (64±39.34μg/dL versus 53±41.83μg/dL; p < 0.001) and TIBC (225±84.08μg/dL versus 266±82.30μg/dL; p < 0.001) were found to be significantly lower in the ADRD group compared to controls. Ferritin level was elevated in only in the ADRD (395±488.18 ng/mL versus 263±1023.4 ng/mL; p < 0.001). Even though statistically significant, mean folic acid and vitamin B12 levels were found to be normal in both groups, suggesting no clinical relevance. Similarly, serum creatinine was found to be statistically significant between the two groups but was without clinical relevance as the mean values from both groups fell within the same clinical range of kidney disease. There was no statistically significant difference in HgbA1C between the groups (p = 0.28). Graphical representation of pair-wise comparison of laboratory data between clinical group is shown of Fig. 1. Although both groups met the WHO defined criteria for anemia, the degree was significantly worse in the ADRD group. We compared moderate, severe, and moderate-to-severe anemia subtypes across the two groups, using a Two Sample proportion Z-Test. Analysis revealed that severe anemia in ADRD group was 6.2% higher than in controls [95% CI: 4.2–8.4], and moderate anemia was 13% higher [95% CI: 9.8–16.2]. Overall, moderate-to-severe anemia was found to be 19% higher in ADRD group [95% CI: 15.8–22.1] (Fig. 2). Giving the 7.3-year difference in age between two clinical groups, there was a concern that age would confound the comparisons. Therefore, it was decided to compare the groups on Hb and iron panel (Iron Level, ferritin, and TIBC) measures controlling for age. Iron panel was chosen to investigate if the association between ADRD and ACI is still valid after controlling for age. The correlation between age and TIBC was –0.16. and that between Age and Iron Level, was –0.12. The correlation between Age and Ferritin was –0.02. and that between Age and HGB was –0.20. It is clear that the correlations between Age and each of the measures were low. These correlations suggest that adjusting for chronological age would have little effect on the differences between groups. In order to do the analysis, a multiple regression analysis was computed using Age and Group (ADRD, Control) as predictors. A separate regression was computed for each measure (TIBC, ferritin, iron level, and Hb). This test represents the difference between the two groups, controlling for chronological age. Table 2 contains the difference between the groups without controlling for age and the difference between the two groups, controlling for age. In this way, it is clear as to what effect adjusting for Age would have on comparing the two groups on each of the four measures. The differences are computed by subtracting the mean of each measure of the Control Group from the mean of each measure of the ADRD Group. Therefore, a negative difference indicates that the mean for ADRD Group is lower than that for the Control Group. The appropriate standard errors are presented in parentheses with each difference. The results of the test for regression weights are presented in Table 2 with the means of measures mentioned above controlling for age in Table 3. The results indicate that the ADRD Group was significantly lower in TIBC, Iron Level, and Hb and higher in Ferritin than the Control Group. There are only small differences between the group difference with and without controlling for age. The pattern of results along with magnitude of difference are very similar whether one does or does not control for age.
Table 1
Laboratory data mean values in the clinical groups
Measurement (units) (reference range) | ADRD group | Control group | p |
Hb total (g/dL) (M: 13.5 to 17.5; F: 12.0 to 15.5) | 10.15±2.08 | 11.06±2.08 | <0.001 |
Serum Iron (ug/dL) (37.0–170.0) | 64±39.34 | 53±41.83 | <0.001 |
TIBC (ug/dL) (240–450) | 225±84.08 | 266±82.30 | <0.001 |
Ferritin (ng/mL) (11.10–264.00) | 395±488.18 | 263±1023.4 | <0.001 |
Vitamin B12 (pg/mL) (200–900) | 726±252.17 | 667±248.26 | <0.001 |
Folic acid (ng/mL) (2.76–20) | 14.7±5.38 | 14.3±5.25 | <0.05 |
Serum Creatinine (mg/dL) (0.52–1.04) | 1.8±1.82 | 1.7±1.81 | <0.05 |
Hb A1C (<6.5) | 6.39±1.51 | 6.42±1.51 | 0.28 |
Fig.2
Histogram illustrating significantly higher proportion of moderate and severe anemia in ADRD group (gray) in comparison to control (blue) (95% CI: 15.8–22.1).
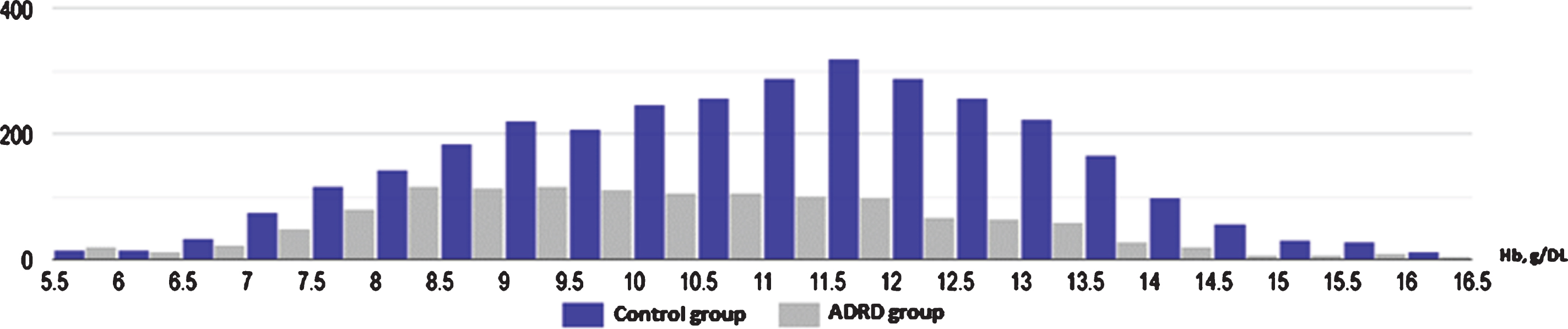
Table 2
Mean differences between groups without and with controlling for age and test of the differences between groups controlling for age
Outcome measure | Difference between groups | Difference between groups controlling for age | Test result |
TIBC | –41.86 (2.72) | –35.94 (2.88) | t(4517) = –12.48, p < 0.001 |
Iron Level | –10.63(1.35) | –7.98 (1.43) | t(4517) = –5.58, p < 0.001 |
Ferritin | 132.41(22.81) | 158.92 (24.11) | t(4449) = 6.59, p < 0.001 |
HGB | –0.91(.07) | –0.68 (.07) | t(4517) = –9.42, p < 0.001 |
Table 3
Means of the dementia group and the control group adjusting for age
Measure | Dementia group | Control group |
TIBC | 229.13 | 265.07 |
Iron Level | 54.98 | 62.96 |
Ferritin | 414.00 | 255.08 |
HGB | 10.32 | 11.00 |
DISCUSSION
Our data suggests a clear statistically significant association between ADRD and ACI, given low serum iron and TIBC levels, elevated serum ferritin with normal vitamin B12 and folic acid level in ADRD group. We did not find statistical association between HgbA1C and anemia in the ADRD group, which excluded DM as the cause of ACI. Serum creatinine also was not found to be clinically substantial, which precludes CKD as contributor of ACI. These findings suggest that there may be an independent association between ADRD and ACI.
Anemia and ADRD
Preliminary reports of a possible association between anemia and ADRD emerged in late 20th century, and later has been strongly supported by large prospective and retrospective studies [7, 8, 14–17]. In a prospective study from Hong et al., presence of anemia was associated with developing of ADRD (HR = 1.64; 95% CI: 1.30–2.07) [8]. This association was confirmed with another prospective study by Atti et al., demonstrating a two-fold increase in incidence of dementia in patients with anemia within 3 years after adjusting for confounders like inflammation, malnutrition and chronic disease (HR 1.6, 95% CI: 1.1–2.4) [15]. A large retrospective study by Jeong et al. enrolled 37,900 persons and found significant association between anemia and ADRD (HR 1.24; 95% CI: 1.02–1.51), and interestingly, hazard ratio (HR) increased to 5.7 (95% CI: 1.84–17.81) for patients with severe anemia [7]. Despite the strong evidence of the general association, the relationship between anemia subtypes and ADRD has remained largely undefined, which could limit further research for potential treatment options.
Smaller studies examining single subtypes of anemia have also been presented. A case-control study from Chung at el. found an association between IDA and ADRD in females (OR 2.02, CI: 1.43–2.84), but interestingly, no association was found in the male population [18]. The study did not investigate an association between other types of anemia aside of IDA. Weuve et al. investigated the association between ADRD and anemia with an elevated RDW, which is usually associated with both IDA and ACI; however, no statistically significant difference was found (OR, 0.99; 95% CI: 0.86–1.18) [19]. In a retrospective study by Faux et al. an Australian Imaging, Biomarkers and Lifestyle (AIBL) cohort was used to investigate an association between anemia and dementia in an Alzheimer’s population. The study included 211 patients with AD, 133 patients with mild cognitive impairment and 768 healthy adults [9, 20]. This study again showed a strong association between anemia and ADRD (OR 2.43, CI: 1.31–4.54), but more importantly, it was the first study which investigated a possible association between ADRD and certain types of anemia. No statistically significant differences were found between serum vitamin B12, folic acid, serum Cr, blood urea nitrogen, and haptoglobin, which did not support an association ADRD with some subtypes of anemia such as vitamin B12 and folic acid deficiency anemia, hemolytic anemia, and anemia of CKD. Serum iron level was normal in both groups, and ferritin was elevated in ADRD group (p = 0.004), which does not support association of IDA and ADRD. Anemia of chronic inflammation was considered by authors as candidate for association, based on high ferritin and erythrocyte sedimentation rate (ESR) (p = 0.006), but rejected based on absence of statistically significant differences in serum iron, TIBC, transferrin saturation, and interleukin-6.
Similarly, we found no association between ADRD and IDA (in both the male and female population), anemia of CKD, and vitamin B12 and folic acid deficiency anemia. In contrast to Faux at el., we found a statistically significant difference in serum iron, ferritin and TIBC between the control and study groups. Although normal in the control group, we found significant evidence of iron dyshomeostasis and ACI in ADRD group (low iron and TIBC levels, and high ferritin level) (Table 1).
Though the association between anemia and dementia has been well described in literature, the pathophysiology of this association remains not well understood. It has been hypothesized that anemia may lead to memory loss due to chronic brain hypoxia [7]. Alternatively, it has also been hypothesized that it could be a sign of underlying ADRD and a reflection of a pathologic process that is yet understood. We found a strong association with ADRD and ACI, which implies that a combination of anemia and iron dyshomeostasis play a significant role in pathogenesis of dementia.
It is also important to mention that there have been no long-term studies to assess the effect of untreated anemia on the brain and memory function. We hypothesize that the brain, the most sensitive organ to hypoperfusion and hypoxia, may require a higher level of hemoglobin to maintain cortical functions as we age. We found a significant difference of almost 1 g/dL of Hb between the ADRD and control group (10.15 and 11.06, p < 0.001, N = 4,517). Prevalence of moderate-to-severe anemia in ADRD group was 19% higher as opposed to control group [95% CI: 15.8–22.1]. In fact, blood transfusion goals for anemia have been established based on guidelines determined by cardiovascular mortality and morbidity data. According to current guidelines, the transfusion goal for acute, symptomatic anemia is set at hemoglobin level of 10 g/dL, and 7–8 g/dL in asymptomatic hospitalized patients, as well as in an outpatient setting [21–24]. In a meta-analysis by Carson et al., there was no 30-day difference in cardiovascular mortality and morbidity between restrictive (Hb goal more than 8) group when compared to liberal group (Hb goal more than 10) in terms of blood transfusion [22]. These studies remain the cornerstone of transfusion guidelines and treatment of anemia, but there is strong possibility that the cerebral cortex requires a level of hemoglobin higher than that of 7–8 g/dL to prevent development of ADRD. Interestingly enough, Weiskopf et al. showed in their study that a reduction of Hb level from 6 to 5 g/dL produces subtle delays in reaction time and impaired immediate and delayed memory, which reversed after the subjects were transfused to a hemoglobin of above 7 g/dL [25].
If an acute reduction in Hb can cause such a change in cognition, it is within reason to postulate that long standing anemia may cause chronic damage to the brain, contributing to memory loss and development of dementia. Further prospective studies are needed to determine, if blood transfusion targeting a Hb level of more than 11 g/dL and/or correction of moderate-to-severe anemia reduces the incidence or progression of ADRD.
Anemia of chronic inflammation
Anemia of chronic inflammation (ACI), formerly termed anemia of chronic disease, is defined as an impaired production of erythrocytes due to inflammatory conditions such as chronic infection, cancer, autoimmune diseases or milder but persistent inflammatory states such as DM, obesity, aging, and CKD [26, 27]. A diagnosis of ACI requires normal iron stores with low circulation iron less than 60μg/dL, normal to low TIBC, serum ferritin more than 12 ng/mL, or erythrocyte protoporphyrin concentration less than 1.24 microM [26, 27]. Chronic inflammation increases ferritin levels, a marker of stored iron; however, inability to use iron for erythropoiesis in a state of chronic inflammation causes “functional iron deficiency” [28]. Anemia of chronic inflammation is frequently associated with inappropriately low levels of erythropoietin and elevated measures of inflammatory markers, such as C-reactive protein (CRP) [26].
Prevalence of anemia increases rapidly with age, and in general 11.0% of men and 10.2% of women 65 years and older, and 20% of those greater than 85 years of age are found to be anemic [29]. As was defined previously, ACI is frequently multifactorial and commonly associated with chronic diseases, including DM and CKD, and aging itself. Joosten et al. observed 191 hospitalized elderly patients with anemia, and showed that 70% of the patients had ACI. 16% of the patients with ACI had concomitant chronic renal failure, 71% of them had an acute infection, 12% had cancer, and 16% had a chronic infection, such as pressure ulcer, or a chronic autoimmune inflammatory disease [30]. In addition, a multi-center study with 4,892 ICU patients in United States revealed that ACI is particularly common in critically ill patients [31].
We investigated the association between ACI in ADRD and CKD and did not find a clinical difference in serum creatinine (1.8 mg/dL versus 1.7 mg/dL, p < 0.001). Diabetes mellitus is another chronic condition commonly associated with ACI [32]. HgbA1C levels of both clinical groups were obtained, and no significant difference was found (6.39% versus 6.42%, p = 0.28). Both conditions are among the most common causes of ACI and it was essential to investigate their role as the potential confounders. As laboratory sampling for both clinical groups were obtained from outpatient clinic visits, it makes acute illness less likely the cause of ACI. Present or past history of cancer was one of the exclusion criteria for this study making malignancy less likely to contribute to ACI picture.
Blood transfusion, intravenous iron, and erythropoietin are established standards of care in treatment of ACI [26]. Administration of erythropoietin in anemia has shown to be effective by elevating concentrations of reticulocytes and serum transferrin receptors [33]. There is also novel experimental therapy targeting IL-6 and hepcidin-ferroportin axis [26]. Tocilizumab, an anti-IL6 receptor antibody, has demonstrated efficacy in reduction of hepcidin level and improving ACI in patients with rheumatoid arthritis. In addition, therapies targeting the hepcidine-ferroportine axis include LDN-193189 and NOX-H94, these agents have shown to be effective in improvement of ACI in animal models.
Iron dyshomeostasis and ADRD
The phenomenon of iron dyshomeostasis in patients with ADRD has been reported in multiple studies, however the studies are of relatively smaller sample size [34–43]. The role iron elements play in AD could be theoretically established by trying to quantify tissue concentrations as compared to controls. In a postmortem case-control study of 16 patients by Grundke-Iqbal et al. ferritin was observed in Aβ plaques [35]. In addition, two in vivo studies totaling 167 patients reported increased ferritin storage in hippocampus and basal ganglia on of MRI imaging of AD [39, 40]. Investigations have revealed that there are increased concentrations of transferrin in the post-mortem histopathologic studies of the patients with AD [42, 43]. Interestingly, the total concentration of iron in human brains with AD was shown be to 67% higher in gray matter and 27% higher in white matter as compared to control tissue in postmortem study [43]. However, contrary to the above-mentioned studies, in the large prospective study by Ayton et al., no difference in ferritin level in CSF was found in cognitively normal patients, patients with mild cognitive impairment, or ADRD [44].
Evidence of iron dyshomeostasis in peripheral blood associated with ADRD has been reported previously. In a systematic review by Lopes da Silva et al. in 2014 with a total of 153 ADRD patients and 544 controls across five studies, only two studies showed a statistically significant decrease in plasma iron levels though the parameters of TIBC and ferritin levels were not investigated [45]. Faux et al. used the AIBL cohort to investigate the association between anemia and dementia, and as was discussed earlier, was the first study to investigate the association of all major types of anemia with ADRD [9, 20]. No statistically significant association was observed between specific types of anemia and ADRD, but the authors, based on elevated ESR and ferritin in ADRD group, proposed an association with ACI. In 2017, a prospective study by Goozee et al. showed statistically significant difference in serum ferritin in patients with preclinical dementia with high neocortical Aβ load, but serum iron, TIBC, or Hb were not investigated [46]. In our study, we were able to show a statistically significant association between ACI and ADRD, which we theorize is due to the higher power of our study compared to previous reports.
While dysregulation of iron homeostasis in patients with ADRD is evident, the exact origin of excessive iron and ferritin stores as well as its precise pathogenesis of its accumulation remain unclear. Iron induced oxidative stress has been proposed and is well supported in literature. Excess iron could promote the conversion of hydrogen peroxide (H2O2) to hydroxyl radical (–OH) with high toxic properties and trigger Aβ production, and thus, Aβ plaques [34, 37, 38, 47].
Another intriguing hypothesis is that excessive iron interferes with protein transcription and translation in neurons and glial cells [38]. The cerebral cortex has the largest iron storage in the body and iron-regulation gene expression only second to the liver as iron serves an essential role in myelin formation, neurotransmitter production and metabolic activity of the neurons [43]. There exists several proteins involved in brain iron metabolism, including transferrin, transferrin receptor 1, ferritin, iron-regulatory proteins (IRPs), ferroportin 1 (FPN1), lactoferrin, divalent metal ion transporter, and brain-specific ceruloplasmin [38, 44, 48, 49]. Iron and IRPs controls transcription of these proteins in the brain via iron regulatory element (IRE) located on mRNAs [50]. Interestingly, IRE was also found on amyloid-β protein precursor (AβPP) mRNA, the direct progenitor of Aβ, and it has been postulated than AβPP itself can further regulate iron metabolism, inducing influx of the iron into the cell via FPN1 [50, 51]. Further excess of iron accumulation leads to increase of aggregation of AβPP, α-synuclein, and Aβ, matching the exact pathogenesis that we see in ADRD.
Blocking IRE on AβPP, α-synuclein, and Aβ could potentially prevent aggregation of misfolded proteins. Several clinical trials are currently investigating the role of IRE-inhibitors in ADRD and other neurodegenerative disorders [49, 50]. Other promising treatment molecule, targeting iron metabolism, is lactoferrin, which was shown to be effective in reduction of Aβ deposition and cognitive improvement in mice in recent study [52]. Iron chelators, such as desferoxamine, deferasirox, and deferiprone, were shown to be effective in mice and one small single-blinded human study; however, potential significant neurotoxicity is the major concern for using these agents as a treatment for ADRD [53].
Inflammation, infection, and ADRD
Neuroinflammation plays a major role in the pathogenesis of ADRD with involvement of microglia, cytokines and complement complex [54]. One hypothesis is that Aβ peptides along with CD40 co-stimulation trigger the inflammatory response [55]. Others have suggested that acute and/or chronic inflammation can be transmitted to the brain via cytokines acting on endothelial cells of the blood-brain barrier. Subsequently a pro-inflammatory state triggers Aβ-dependent or Aβ-independent inflammation inside the CNS, eventually leading to cognitive impairment [56, 57]. Our study revealed changes in the peripheral blood affecting complete blood count and iron panel, suggesting chronic inflammation in the patients affected by ADRD.
Association between inflammation and ADRD is also well-supported in prior studies. In 2002, Schmidt et al. published a large prospective study which showed an association between elevated high-sensitive CRP) and ADRD, and proposed that inflammation precedes manifestation of dementia [58]. In 2004, Engelhart et al. discovered that other inflammatory proteins such as α1-antichymotrypsin and IL-6 are associated with increased risk of ADRD; relative risks were 1.49 (95% CI: 1.23–1.81) and 1.28 (95% CI: 1.06–1.55), respectively [59]. Yaffe et al. observed a strong association between metabolic syndrome combined with inflammatory state (evident by elevated IL-6 and CRP) and ADRD with RR of 1.69 [95% CI: 1.19–2.32] [60]. In a study by Holmes et al. increases of tumor necrosis factor alpha, another pro-inflammatory cytokine, in patients with ADRD was associated with a fourfold increase in the rate of cognitive decline over six months as compared to the control group [61]. There also exists evidence of the long-term association of a septic shock and respiratory failure with cognitive impairment [62, 63]. Summary of the studies that elucidated the evidence of association between inflammation and ADRD are illustrated in the Table 4.
Table 4
Summary of the studies of association of the inflammation and ADRD
Study | N | Study design | Findings |
Schmidt et al., 2002 [58] | 1,050 | Prospective cohort study | Association between elevated hsCRP and development of ADRD (p < 0.0001) |
Engelhart et al., 2004 [59] | 915 | Prospective cohort study | Association between elevated alpha1-antichymotrypsin, interleukin-6 and ADRD (RR 1.49, 95% CI: 1.23–1.81; RR 1.28, 95% CI: 1.06–1.55) |
Yaffe et al., 2004 [60] | 2,632 | Prospective cohort study | Association between metabolic syndrome with inflammation and ADRD (RR 1.66; 95% CI, 1.19–2.32) |
Holmes et al., 2009 [61] | 300 | Prospective cohort study | Association with progression of ADRD in group with high baseline TNF-levels and presence of systemic inflammatory events (p = 0.02) |
Wallin et al., 2012 [77] | 1,449 | Prospective cohort study | Presence of any joint disorder in midlife associated with worse cognitive status later in life (OR 1.96; CI: 1.17–3.28) |
Pandharipande et al., 2013 [63] | 821 | Prospective cohort study | Longer duration of delirium during ICU stay is associated with worse global cognition and executive function (p = 0.004) |
hsCRP, high-sensitivity C-reactive protein; ADRD, Alzheimer’s disease and related dementia; TNF, tumor necrosis factor; CI, confidence intervals; RR, relative risk.
Aβ deposition has been considered the major force behind ADRD pathophysiology, and, specifically, AD [64]. However, there is a growing body of literature that suggests that role of Aβ in neuronal damage might be misunderstood as new evidence is suggestive of a protective role [57, 65]. Recent in vitro and in vivo studies have revealed antibacterial and antifungal properties of Aβ [66]. Therapies targeting Aβ reduction have not produced clinical improvement in cognition or successfully decreased Aβ level [67, 68]. Adverse effects of anti-Aβ therapy included increased infection rates, including meningioencephalitis after active immunotherapy against Aβ, and relapse of herpes infection in patients received β-site AβPP cleaving enzyme and γ-secretase inhibitors [65].
Evidence of inflammation and antimicrobial properties Aβ have led to further research in this area. Emerging data strongly point to the evidence of infection etiologies of the ADRD, including viral, bacterial, and fungal origin [65, 69, 70]. Recent in vitro studies have shown that Aβ demonstrates antimicrobial activity against at least eleven microbial pathogen, including Herpes Simplex Virus 1 and 2 (HSV1 and HSV2), Influenza A, Escherichia coli, Enterococcus faecalis, Staphylococcus aureus, and Streptococcus pneumonie. In 2017, Emery at al. published a study using 16S ribosomal gene-specific next generation sequencing (NGS) of postmortem brains, which revealed five to ten-fold increase in bacterial load in patients with ADRD in comparison to controls. A large retrospective cohort study by Tzeng et al. elucidated significant risk reduction in ADRD development in patients affected by HSV treated with antiherpetic medication (adjusted HR = 0.092, 95% CI: 0.079–0.108, p < 0.001) [71]. In 2015, Maheshwari et al. conducted a meta-analysis, which found five-fold increase occurrence of ADRD in patients with Chlamydia pneumonia in comparison to control groups (OR: 5.66; 95% CI: 1.83–17.51) [72]. In addition, majority of the patients with ADRD die from pneumonia as an immediate cause of death [69]. There is a growing body of evidence to support an association between ADRD and fungal infection; fungal Enolase, β-Tubulin, and Chitin was identified in the brain samples of the patients with ADRD, and this evidence was recently advanced by detection of the fungal DNA the frozen brain tissue of the patients with ADRD using NGS [73, 74]. Summary of the studies that elucidated evidence of association with microbial infection are presented in the Table 5.
Table 5
Summary of the studies of association of the infection and ADRD
Study | Study design | Findings |
Pisa, 2015 [70] | Postmortem | Immunohistochemical analyses of the brain tissue from AD patients showed intracellular fungal material. Similar staining did not reveal fungal material in the control tissue of the patients without AD. |
Maheshwari, 2015 [72] | Meta-analysis | Ten-fold increase in risk of occurrence of AD with presence of spirochetal infection (OR: 10.61; 95% CI: 3.38–33.29). Five-fold increase in rick of occurrence of AD with presence of Chlamydophila infection (OR: 5.66; 95% CI: 1.83–17.51) |
Pisa, 2016 [73] | Postmortem | Presence of fungal proteins, enolase and β-tubulin, and polysaccharide chitin in tissue sample from AD patients |
Alonso, 2017 [74] | Postmortem | Next-generation sequencing revealed fungal species Alternaria, Botrytis, Candida, Cladosporium, and Malassezia in the tissue of entorhinal cortex and hippocampus of the patient with ADRD |
Tzeng, 2018 [71] | Retrospective cohort study | Adjusted HR of 2.564 (95% CI: 2.351–2.795, p < 0.001) for development of ADRD in HSV-infected cohort versus non-HSV cohort. Treatment with anti-herpetic medication showed reduction of dementia development (adjusted HR = 0.092 [95% CI 0.079–0.108], p < 0.001). |
AD, Alzheimer’s disease; HSV, herpes simplex virus; OR, odds ratio; HR, hazard ratio; CI, confidence intervals.
Limitations of the study
There were certain limitations of this study. The retrospective nature of the study allowed us to show an association between ACI and ADRD but not causation. Elevations in ESR and CRP are laboratory markers of the ACI in addition to Hb and iron profile, but these markers were not available for investigation. Autoimmune disease, such as rheumatoid arthritis and systemic lupus erythematosus can lead to ACI; however, association with ADRD, ACI, and rheumatologic disorders were not investigated in this study due to relatively low prevalence in the population (prevalence of rheumatoid arthritis in the US is 0.55% [75]; prevalence of systemic lupus erythematosus is 0.006% [76]).
Conclusion
There exists a fair amount of literature examining the role of anemia in ADRD though not one study encompasses a global view of anemias in their analysis. We chose to investigate the subtypes of anemia and its role in dementia. We found a statistically and clinically significant decrease in the Hb level between ADRD group and control groups (p < 0.001). We also observed the proportion of moderate and severe anemia was significantly higher in ADRD group (95% CI: 15.8–22.1). Analysis of iron studies revealed that ACI has the strongest association with ADRD, independent of CKD and DM. We did not find association between ADRD and IDA, vitamin B12 or folic acid deficiency anemia. These findings suggest blood transfusion to target Hb of ≥11 mg/dL and/or correction of moderate-to-severe anemia could be investigated as potential therapeutic interventions. Correction of ACI with erythropoietin and/or intravenous iron administration, and treating chronic infections also warrant consideration in future studies. Further prospective studies to establish a causative link in addition to clinical trials are required to corroborate out findings.
CONFLICT OF INTEREST
The authors have no conflict of interest to report.
REFERENCES
[1] | Kramarow EA , Tejada-Vera B ((2019) ) Dementia mortality in the United States, 2000-2017. Natl Vital Stat Rep 68: , 1–29. |
[2] | Alzheimer’s Association ((2016) ) 2016 Alzheimer’s disease facts and figures. Alzheimers Dement 12: , 459–509. |
[3] | Larson EB ((2018) ) Prevention of late-life dementia: No magic bullet. Ann Intern Med 168: , 77–79. |
[4] | Iadecola C ((2010) ) The overlap between neurodegenerative and vascular factors in the pathogenesis of dementia. Acta Neuropathol 120: , 287–296. |
[5] | Corriveau RA , Koroshetz WJ , Gladman JT , Jeon S , Babcock D , Bennett DA , Carmichael ST , Dickinson SL , Dickson DW , Emr M , Fillit H , Greenberg SM , Hutton ML , Knopman DS , Manly JJ , Marder KS , Moy CS , Phelps CH , Scott PA , Seeley WW , Sieber BA , Silverberg NB , Sutherland ML , Taylor A , Torborg CL , Waddy SP , Gubitz AK , Holtzman DM ((2017) ) Alzheimer’s Disease-Related Dementias Summit 2016: National research priorities. Neurology 89: , 2381–2391. |
[6] | Ngandu T , Lehtisalo J , Solomon A , Levalahti E , Ahtiluoto S , Antikainen R , Backman L , Hanninen T , Jula A , Laatikainen T , Lindstrom J , Mangialasche F , Paajanen T , Pajala S , Peltonen M , Rauramaa R , Stigsdotter-Neely A , Strandberg T , Tuomilehto J , Soininen H , Kivipelto M ((2015) ) A 2 year multidomain intervention of diet, exercise, cognitive training, and vascular risk monitoring versus control to prevent cognitive decline in at-risk elderly people (FINGER): A randomised controlled trial. Lancet 385: , 2255–2263. |
[7] | Jeong SM , Shin DW , Lee JE , Hyeon JH , Lee J , Kim S ((2017) ) Anemia is associated with incidence of dementia: A national health screening study in Korea involving 37,900 persons. Alzheimers Res Ther 9: , 94. |
[8] | Hong CH , Falvey C , Harris TB , Simonsick EM , Satterfield S , Ferrucci L , Metti AL , Patel KV , Yaffe K ((2013) ) Anemia and risk of dementia in older adults: Findings from the Health ABC study. Neurology 81: , 528–533. |
[9] | Faux NG , Rembach A , Wiley J , Ellis KA , Ames D , Fowler CJ , Martins RN , Pertile KK , Rumble RL , Trounson B , Masters CL , Group AR , Bush AI ((2014) ) An anemia of Alzheimer’s disease. Mol Psychiatry 19: , 1227–1234. |
[10] | Maccio A , Madeddu C , Gramignano G , Mulas C , Tanca L , Cherchi MC , Floris C , Omoto I , Barracca A , Ganz T ((2015) ) The role of inflammation, iron, and nutritional status in cancer-related anemia: Results of a large, prospective, observational study. Haematologica 100: , 124–132. |
[11] | Kreuzer KA , Rockstroh JK ((1997) ) Pathogenesis and pathophysiology of anemia in HIV infection. Ann Hematol 75: , 179–187. |
[12] | Gartner S ((2000) ) HIV infection and dementia. Science 287: , 602–604. |
[13] | ((1968) ) Nutritional anaemias. Report of a WHO scientific group. World Health Organ Tech Rep Ser 405: , 5–37. |
[14] | Beard CM , Kokmen E , O’Brien PC , Ania BJ , Melton LJ 3rd ((1997) ) Risk of Alzheimer’s disease among elderly patients with anemia: Population-based investigations in Olmsted County, Minnesota. Ann Epidemiol 7: , 219–224. |
[15] | Atti AR , Palmer K , Volpato S , Zuliani G , Winblad B , Fratiglioni L ((2006) ) Anaemia increases the risk of dementia in cognitively intact elderly. Neurobiol Aging 27: , 278–284. |
[16] | Lucca U , Tettamanti M , Mosconi P , Apolone G , Gandini F , Nobili A , Tallone MV , Detoma P , Giacomin A , Clerico M , Tempia P , Guala A , Fasolo G , Riva E ((2008) ) Association of mild anemia with cognitive, functional, mood and quality of life outcomes in the elderly: The “Health and Anemia” study. PLoS One 3: , e1920. |
[17] | Peters R , Burch L , Warner J , Beckett N , Poulter R , Bulpitt C ((2008) ) Haemoglobin, anaemia, dementia and cognitive decline in the elderly, a systematic review. BMC Geriatr 8: , 18. |
[18] | Chung SD , Sheu JJ , Kao LT , Lin HC , Kang JH ((2014) ) Dementia is associated with iron-deficiency anemia in females: A population-based study. J Neurol Sci 346: , 90–93. |
[19] | Weuve J , Mendes de Leon CF , Bennett DA , Dong X , Evans DA ((2014) ) The red cell distribution width and anemia in association with prevalent dementia. Alzheimer Dis Assoc Disord 28: , 99–105. |
[20] | Ellis KA , Bush AI , Darby D , De Fazio D , Foster J , Hudson P , Lautenschlager NT , Lenzo N , Martins RN , Maruff P , Masters C , Milner A , Pike K , Rowe C , Savage G , Szoeke C , Taddei K , Villemagne V , Woodward M , Ames D , AIBL Research Group ((2009) ) The Australian Imaging, Biomarkers and Lifestyle (AIBL) study of aging: Methodology and baseline characteristics of 1112 individuals recruited for a longitudinal study of Alzheimer’s disease. Int Psychogeriatr 21: , 672–687. |
[21] | Carson JL , Terrin ML , Noveck H , Sanders DW , Chaitman BR , Rhoads GG , Nemo G , Dragert K , Beaupre L , Hildebrand K , Macaulay W , Lewis C , Cook DR , Dobbin G , Zakriya KJ , Apple FS , Horney RA , Magaziner J , FOCUS Investigators ((2011) ) Liberal or restrictive transfusion in high-risk patients after hip surgery. N Engl J Med 365: , 2453–2462. |
[22] | Carson JL , Brooks MM , Abbott JD , Chaitman B , Kelsey SF , Triulzi DJ , Srinivas V , Menegus MA , Marroquin OC , Rao SV , Noveck H , Passano E , Hardison RM , Smitherman T , Vagaonescu T , Wimmer NJ , Williams DO ((2013) ) Liberal versus restrictive transfusion thresholds for patients with symptomatic coronary artery disease. Am Heart J 165: , 964–971 e961. |
[23] | Cooper HA , Rao SV , Greenberg MD , Rumsey MP , McKenzie M , Alcorn KW , Panza JA ((2011) ) Conservative versus liberal red cell transfusion in acute myocardial infarction (the CRIT Randomized Pilot Study). Am J Cardiol 108: , 1108–1111. |
[24] | Carson JL , Stanworth SJ , Alexander JH , Roubinian N , Fergusson DA , Triulzi DJ , Goodman SG , Rao SV , Doree C , Hebert PC ((2018) ) Clinical trials evaluating red blood cell transfusion thresholds: An updated systematic review and with additional focus on patients with cardiovascular disease. Am Heart J 200: , 96–101. |
[25] | Weiskopf RB , Kramer JH , Viele M , Neumann M , Feiner JR , Watson JJ , Hopf HW , Toy P ((2000) ) Acute severe isovolemic anemia impairs cognitive function and memory in humans. Anesthesiology 92: , 1646–1652. |
[26] | Fraenkel PG ((2015) ) Understanding anemia of chronic disease. Hematology Am Soc Hematol Educ Program 2015: , 14–18. |
[27] | Malyszko J , Mysliwiec M ((2007) ) Hepcidin in anemia and inflammation in chronic kidney disease. Kidney Blood Press Res 30: , 15–30. |
[28] | Thomas DW , Hinchliffe RF , Briggs C , Macdougall IC , Littlewood T , Cavill I , British Committee for Standards in H ((2013) ) Guideline for the laboratory diagnosis of functional iron deficiency. Br J Haematol 161: , 639–648. |
[29] | Guralnik JM , Eisenstaedt RS , Ferrucci L , Klein HG , Woodman RC ((2004) ) Prevalence of anemia in persons 65 years and older in the United States: Evidence for a high rate of unexplained anemia. Blood 104: , 2263–2268. |
[30] | Joosten E , Lioen P ((2015) ) Iron deficiency anemia and anemia of chronic disease in geriatric hospitalized patients: How frequent are comorbidities as an additional explanation for the anemia? Geriatr Gerontol Int 15: , 931–935. |
[31] | Corwin HL , Gettinger A , Pearl RG , Fink MP , Levy MM , Abraham E , MacIntyre NR , Shabot MM , Duh MS , Shapiro MJ ((2004) ) The CRIT Study: Anemia and blood transfusion in the critically ill–current clinical practice in the United States. Crit Care Med 32: , 39–52. |
[32] | Sherwani SI , Khan HA , Ekhzaimy A , Masood A , Sakharkar MK ((2016) ) Significance of HbA1c test in diagnosis and prognosis of diabetic patients. Biomark Insights 11: , 95–104. |
[33] | van Iperen CE , Gaillard CA , Kraaijenhagen RJ , Braam BG , Marx JJ , van de Wiel A ((2000) ) Response of erythropoiesis and iron metabolism to recombinant human erythropoietin in intensive care unit patients. Crit Care Med 28: , 2773–2778. |
[34] | Altamura S , Muckenthaler MU ((2009) ) Iron toxicity in diseases of aging: Alzheimer’s disease, Parkinson’s disease and atherosclerosis. J Alzheimers Dis 16: , 879–895. |
[35] | Grundke-Iqbal I , Fleming J , Tung YC , Lassmann H , Iqbal K , Joshi JG ((1990) ) Ferritin is a component of the neuritic (senile) plaque in Alzheimer dementia. Acta Neuropathol 81: , 105–110. |
[36] | Quintana C , Bellefqih S , Laval JY , Guerquin-Kern JL , Wu TD , Avila J , Ferrer I , Arranz R , Patino C ((2006) ) Study of the localization of iron, ferritin, and hemosiderin in Alzheimer’s disease hippocampus by analytical microscopy at the subcellular level. J Struct Biol 153: , 42–54. |
[37] | Smith MA , Zhu X , Tabaton M , Liu G , McKeel DW Jr. , Cohen ML , Wang X , Siedlak SL , Dwyer BE , Hayashi T , Nakamura M , Nunomura A , Perry G ((2010) ) Increased iron and free radical generation in preclinical Alzheimer disease and mild cognitive impairment. J Alzheimers Dis 19: , 363–372. |
[38] | Huat TJ , Camats-Perna J , Newcombe EA , Valmas N , Kitazawa M , Medeiros R ((2019) ) Metal toxicity links to Alzheimer’s disease and neuroinflammation. J Mol Biol 431: , 1843–1868. |
[39] | Raven EP , Lu PH , Tishler TA , Heydari P , Bartzokis G ((2013) ) Increased iron levels and decreased tissue integrity in hippocampus of Alzheimer’s disease detected in vivo with magnetic resonance imaging. J Alzheimers Dis 37: , 127–136. |
[40] | Bartzokis G , Tishler TA ((2000) ) MRI evaluation of basal ganglia ferritin iron and neurotoxicity in Alzheimer’s and Huntington’s disease. Cell Mol Biol (Noisy-le-grand) 46: , 821–833. |
[41] | Graham SF , Nasaruddin MB , Carey M , Holscher C , McGuinness B , Kehoe PG , Love S , Passmore P , Elliott CT , Meharg AA , Green BD ((2014) ) Age-associated changes of brain copper, iron, and zinc in Alzheimer’s disease and dementia with Lewy bodies. J Alzheimers Dis 42: , 1407–1413. |
[42] | Loeffler DA , Connor JR , Juneau PL , Snyder BS , Kanaley L , DeMaggio AJ , Nguyen H , Brickman CM , LeWitt PA ((1995) ) Transferrin and iron in normal, Alzheimer’s disease, and Parkinson’s disease brain regions. J Neurochem 65: , 710–724. |
[43] | Connor JR , Menzies SL , St Martin SM , Mufson EJ ((1992) ) A histochemical study of iron, transferrin, and ferritin in Alzheimer’s diseased brains. J Neurosci Res 31: , 75–83. |
[44] | Ayton S , Faux NG , Bush AI , Alzheimer’s Disease Neuroimaging Initiative ((2015) ) Ferritin levels in the cerebrospinal fluid predict Alzheimer’s disease outcomes and are regulated by APOE. Nat Commun 6: , 6760. |
[45] | Lopes da Silva S , Vellas B , Elemans S , Luchsinger J , Kamphuis P , Yaffe K , Sijben J , Groenendijk M , Stijnen T ((2014) ) Plasma nutrient status of patients with Alzheimer’s disease: Systematic review and meta-analysis. Alzheimers Dement 10: , 485–502. |
[46] | Hare DJ , Doecke JD , Faux NG , Rembach A , Volitakis I , Fowler CJ , Grimm R , Doble PA , Cherny RA , Masters CL , Bush AI , Roberts BR ((2015) ) Decreased plasma iron in Alzheimer’s disease is due to transferrin desaturation. ACS Chem Neurosci 6: , 398–402. |
[47] | Castellani RJ , Moreira PI , Liu G , Dobson J , Perry G , Smith MA , Zhu X ((2007) ) Iron: The Redox-active center of oxidative stress in Alzheimer disease. Neurochem Res 32: , 1640–1645. |
[48] | Liu JL , Fan YG , Yang ZS , Wang ZY , Guo C ((2018) ) Iron and Alzheimer’s disease: From pathogenesis to therapeutic implications. Front Neurosci 12: , 632. |
[49] | Crielaard BJ , Lammers T , Rivella S ((2017) ) Targeting iron metabolism in drug discovery and delivery. Nat Rev Drug Discov 16: , 400–423. |
[50] | Zhou ZD , Tan EK ((2017) ) Iron regulatory protein (IRP)-iron responsive element (IRE) signaling pathway in human neurodegenerative diseases. Mol Neurodegener 12: , 75. |
[51] | Kawahara M , Kato-Negishi M , Tanaka K ((2017) ) Cross talk between neurometals and amyloidogenic proteins at the synapse and the pathogenesis of neurodegenerative diseases. Metallomics 9: , 619–633. |
[52] | Guo C , Yang ZH , Zhang S , Chai R , Xue H , Zhang YH , Li JY , Wang ZY ((2017) ) Intranasal lactoferrin enhances alpha-secretase-dependent amyloid precursor protein processing via the ERK1/2-CREB and HIF-1alpha pathways in an Alzheimer’s disease mouse model. Neuropsychopharmacology 42: , 2504–2515. |
[53] | Crapper McLachlan DR , Dalton AJ , Kruck TP , Bell MY , Smith WL , Kalow W , Andrews DF ((1991) ) Intramuscular desferrioxamine in patients with Alzheimer’s disease. Lancet 337: , 1304–1308. |
[54] | Rubio-Perez JM , Morillas-Ruiz JM ((2012) ) A review: Inflammatory process in Alzheimer’s disease, role of cytokines. ScientificWorldJournal 2012: , 756357. |
[55] | Town T , Nikolic V , Tan J ((2005) ) The microglial “activation” continuum: From innate to adaptive responses. J Neuroinflammation 2: , 24. |
[56] | Holmes C ((2013) ) Review: Systemic inflammation and Alzheimer’s disease. Neuropathol Appl Neurobiol 39: , 51–68. |
[57] | Cunningham C , Hennessy E ((2015) ) Co-morbidity and systemic inflammation as drivers of cognitive decline: New experimental models adopting a broader paradigm in dementia research. Alzheimers Res Ther 7: , 33. |
[58] | Schmidt R , Schmidt H , Curb JD , Masaki K , White LR , Launer LJ ((2002) ) Early inflammation and dementia: A 25-year follow-up of the Honolulu-Asia Aging Study. Ann Neurol 52: , 168–174. |
[59] | Engelhart MJ , Geerlings MI , Meijer J , Kiliaan A , Ruitenberg A , van Swieten JC , Stijnen T , Hofman A , Witteman JC , Breteler MM ((2004) ) Inflammatory proteins in plasma and the risk of dementia: The Rotterdam study. Arch Neurol 61: , 668–672. |
[60] | Yaffe K , Kanaya A , Lindquist K , Simonsick EM , Harris T , Shorr RI , Tylavsky FA , Newman AB ((2004) ) The metabolic syndrome, inflammation, and risk of cognitive decline. JAMA 292: , 2237–2242. |
[61] | Holmes C , Cunningham C , Zotova E , Woolford J , Dean C , Kerr S , Culliford D , Perry VH ((2009) ) Systemic inflammation and disease progression in Alzheimer disease. Neurology 73: , 768–774. |
[62] | Widmann CN , Heneka MT ((2014) ) Long-term cerebral consequences of sepsis. Lancet Neurol 13: , 630–636. |
[63] | Pandharipande PP , Girard TD , Jackson JC , Morandi A , Thompson JL , Pun BT , Brummel NE , Hughes CG , Vasilevskis EE , Shintani AK , Moons KG , Geevarghese SK , Canonico A , Hopkins RO , Bernard GR , Dittus RS , Ely EW , BRAIN-ICU Study Investigators ((2013) ) Long-term cognitive impairment after critical illness. N Engl J Med 369: , 1306–1316. |
[64] | Hardy JA , Higgins GA ((1992) ) Alzheimer’s disease: The amyloid cascade hypothesis. Science 256: , 184–185. |
[65] | Gosztyla ML , Brothers HM , Robinson SR ((2018) ) Alzheimer’s amyloid-beta is an antimicrobial peptide: A review of the evidence. J Alzheimers Dis 62: , 1495–1506. |
[66] | Kumar DK , Choi SH , Washicosky KJ , Eimer WA , Tucker S , Ghofrani J , Lefkowitz A , McColl G , Goldstein LE , Tanzi RE , Moir RD ((2016) ) Amyloid-beta peptide protects against microbial infection in mouse and worm models of Alzheimer’s disease. Sci Transl Med 8: , 340ra372. |
[67] | Slomski A ((2018) ) Abeta-clearing drug fails to slow Alzheimer disease. JAMA 319: , 2470. |
[68] | Panza F , Lozupone M , Logroscino G , Imbimbo BP ((2019) ) A critical appraisal of amyloid-beta-targeting therapies for Alzheimer disease. Nat Rev Neurol 15: , 73–88. |
[69] | Hill JM , Clement C , Pogue AI , Bhattacharjee S , Zhao Y , Lukiw WJ ((2014) ) Pathogenic microbes, the microbiome, and Alzheimer’s disease (AD). Front Aging Neurosci 6: , 127. |
[70] | Pisa D , Alonso R , Juarranz A , Rabano A , Carrasco L ((2015) ) Direct visualization of fungal infection in brains from patients with Alzheimer’s disease. J Alzheimers Dis 43: , 613–624. |
[71] | Tzeng NS , Chung CH , Lin FH , Chiang CP , Yeh CB , Huang SY , Lu RB , Chang HA , Kao YC , Yeh HW , Chiang WS , Chou YC , Tsao CH , Wu YF , Chien WC ((2018) ) Anti-herpetic medications and reduced risk of dementia in patients with herpes simplex virus infections-a nationwide, population-based cohort study in Taiwan. Neurotherapeutics 15: , 417–429. |
[72] | Maheshwari P , Eslick GD ((2015) ) Bacterial infection and Alzheimer’s disease: A meta-analysis. J Alzheimers Dis 43: , 957–966. |
[73] | Pisa D , Alonso R , Rabano A , Horst MN , Carrasco L ((2016) ) Fungal enolase, beta-tubulin, and chitin are detected in brain tissue from Alzheimer’s disease patients. Front Microbiol 7: , 1772. |
[74] | Alonso R , Pisa D , Aguado B , Carrasco L ((2017) ) Identification of fungal species in brain tissue from Alzheimer’s disease by next-generation sequencing. J Alzheimers Dis 58: , 55–67. |
[75] | Hunter TM , Boytsov NN , Zhang X , Schroeder K , Michaud K , Araujo AB ((2017) ) Prevalence of rheumatoid arthritis in the United States adult population in healthcare claims databases, 2004-2014. Rheumatol Int 37: , 1551–1557. |
[76] | Rees F , Doherty M , Grainge MJ , Lanyon P , Zhang W ((2017) ) The worldwide incidence and prevalence of systemic lupus erythematosus: A systematic review of epidemiological studies. Rheumatology (Oxford) 56: , 1945–1961. |
[77] | Wallin K , Solomon A , Kåreholt I , Tuomilehto J , Soininen H , Kivipelto M ((2012) ) Midlife rheumatoid arthritis increases the risk of cognitive impairment two decades later: A population-based study. J Alzheimers Dis 31: , 669–76. |