Efficacy and Safety of the Immunization with DNA for Alzheimer’s Disease in Animal Models: A Systematic Review from Literature
Abstract
Background:
Alzheimer’s disease (AD) is a neurodegenerative disease that does not have a proven cure; however, one of the most promising strategies for its treatment has been DNA vaccines.
Objective:
The present review is aimed to report the new developments of the efficacy and safety of DNA vaccines for AD in animal models.
Method:
The method PRISMA was used for this review. The article search was made in the electronic databases PubMed, LILACS, and Scopus using the descriptors ‘’Alzheimer disease” and ‘’Vaccine, DNA”. Articles published between January 2001 and September 2017 in English, Portuguese, and Spanish were included.
Results:
Upon the consensus, the researchers identified 28 original articles. The studies showed satisfying results as for the decrease of amyloid plaques in mouse, rabbits, and monkeys brains using mostly the DNA Aβ42 vaccine, AV-1955, and AdPEDI-(Aβ1-6)11, mainly with a gene gun. In addition to a reduction in tau by the first DNA vaccine (AV-1980D) targeting this protein. The use of adjuvants and boosters also had positive results as they increased the destruction of the amyloid plaques and induced an anti-inflammatory response profile without side effects.
Conclusion:
The results of DNA vaccines targeting the amyloid-β and the tau protein with or without adjuvants and boosters were promising in reducing amyloid plaques and tau protein without side effects in animals. Although there are many vaccines being tested in animals, few reach clinical trials. Thus, as a future perspective, we suggest that clinical studies should be conducted with vaccines that have been promising in animal models (e.g., DNA Aβ42 vaccine, AV-1955, and AdPEDI-(Aβ1-6)11).
INTRODUCTION
Alzheimer’s disease (AD) is a neurodegenerative disease responsible for the major cases of dementia in the elderly [1, 2]. It is believed that the disease is caused by the accumulation of amyloid-β proteins (Aβ) on the surface of neurons due to a misbalance between its production and clearance. Aβ protein is formed by 42 amino acids capable of aggregating themselves and, their buildup provokes toxic effects that lead to the formation of senile plaques, oxidative damage and hyperphosphorylation of the tau protein [3–6]. These alterations result in neuronal death, leading to progressive memory, judgment, cognition, language, and time-space orientation declines [4, 7]. The current indicated treatment for AD are anticholinesterases drugs (e.g., rivastigmine) that are able to lessen disease progression; however, until the present moment, there is not a proven and safe cure for AD [8].
Considering the toxic effect of the Aβ protein, scientists have been looking for strategies to destroy these plaques as a therapeutic target of AD. For the past years, the immunization of patients with AD has been considered [9, 10]. The first immunotherapy strategy had the goal of using an anti-Aβ vaccine to eliminate Aβ. However, the treatment was interrupted during the clinical phase due to the develop of meningoencephalitis by some patients [11], suggesting that the vaccine, along with the T helper (Th) type 1 adjuvant, might have induced a Th1 cellular response against Aβ [12]. An alternative found was the use of peptide vaccines, although they also presented risks of meningoencephalitis and microhemorrhages, since they also need adjuvants to be efficient [13, 14]. A new gene therapy has been analyzed in animal models using a DNA vaccine which has a simpler production technology, higher stability and does not need adjuvants to be effective [15, 16].
DNA vaccines (i.e., genetic vaccines) are considered third generation vaccines; they have the genetic information to express proteins that will stimulate an immune response against a specific target. The interest gene target (Aβ) is inserted into an expression vector and cloned, the formed complex is inoculated in the host, whose cells will then internalize the gene and express the interest protein, inducing the activation of an immune response [17]. Until 2014, 25 clinical studies were been carried out using DNA vaccines (e.g., Human Immunodeficiency Virus) and four were been commercialized for veterinary use [18].
Prior to population gaining access to immunobiological therapies, tests in animals (non-clinical phase) are needed to prove their efficacy, protection and safety [19, 20]. In animals, cellular and humoral immune responses are induced by DNA vaccination and are mainly characterized by the production of IgG1 antibodies and T helper 2 cells (Th2) [9, 21]. For this reason, researchers have investigated the effects of a variety of DNA vaccines for AD, a lot of these studies are still in the animal (e.g., mouse, rabbits, monkeys) experimentation phase and their results have been promising [9, 14, 21]. Thus, this study is a systematic revision of literature on the efficacy, safety and other effects of the DNA immunization on AD in animal models.
MATERIALS AND METHODS
Research Strategies
To develop the systematic review the PRISMA method was used [22]. The article search was made in the electronic databases PubMed (U.S. National Library of Medicine), LILACS, and Scopus, using the combination of the descriptors ‘’Alzheimer disease” and ‘’Vaccines, DNA” on the Medical Subject Headings (MeSH) or in the form of Health Science (DeCS, LILACS) descriptors. The non-found descriptors in the specific databases were searched in word forms: Alzheimer’s disease, animal models, and DNA vaccine. In this step, the researchers, independently, went through the databases in order to identify the descriptors that best identify the highest number of publications. After many simulations, the authors gathered and, by consensus, defined the adequate descriptors for this review.
Inclusion and Exclusion Criteria
Were included original articles published in the past 16 years during 01/01/2001 and 09/01/2017. The selected articles were published in Spanish, Portuguese, or English, and had available abstracts. Were excluded review articles, commentaries, editorials, errata publications, interviews, guidelines, and human studies.
Quality Evaluation of the Articles
Independently, three researchers belonging to Group I (YAM, CJT, PA) searched the article’s abstracts for a qualitative synthesis. The selection of potential publications was made through the reading of titles and abstracts, and the ones that did not follow the selection criteria were excluded. The articles chosen, by consensus among authors, were randomly divided for data extraction.
Data Extraction
The data extraction was made by structuring the relevant information from the articles in the form of tables. The analysis of each article was based on author/year/country, objective, animal model (species, gender, age), number of animals, groups, DNA vaccine (type, dosage), administration route, statistical analysis, parameters analyzed, results, bias, and conclusion.
From the selected articles, Group I extracted the data and made tables. They checked the data in three steps. The first consisted in randomly distributing the publications among the judges that promoted the first corrections. In the second one, a judge confirmed the information, previously trusted to the other, in a way that each article and data extraction were reviewed again. The third step was comprised by the correction of the judges in pairs.
RESULTS AND DISCUSSION
The research of articles relating to the use of a DNA vaccine for AD in animal models on the databases resulted in 124 studies that, after applying the exclusion criteria, resulted in 105 potential papers of which, only 28 were selected for this review (Fig. 1).
Fig.1
PRISMA flowchart with selection criteria of included articles in the systematic review of DNA immunotherapy in Alzheimer’s disease in animal models.
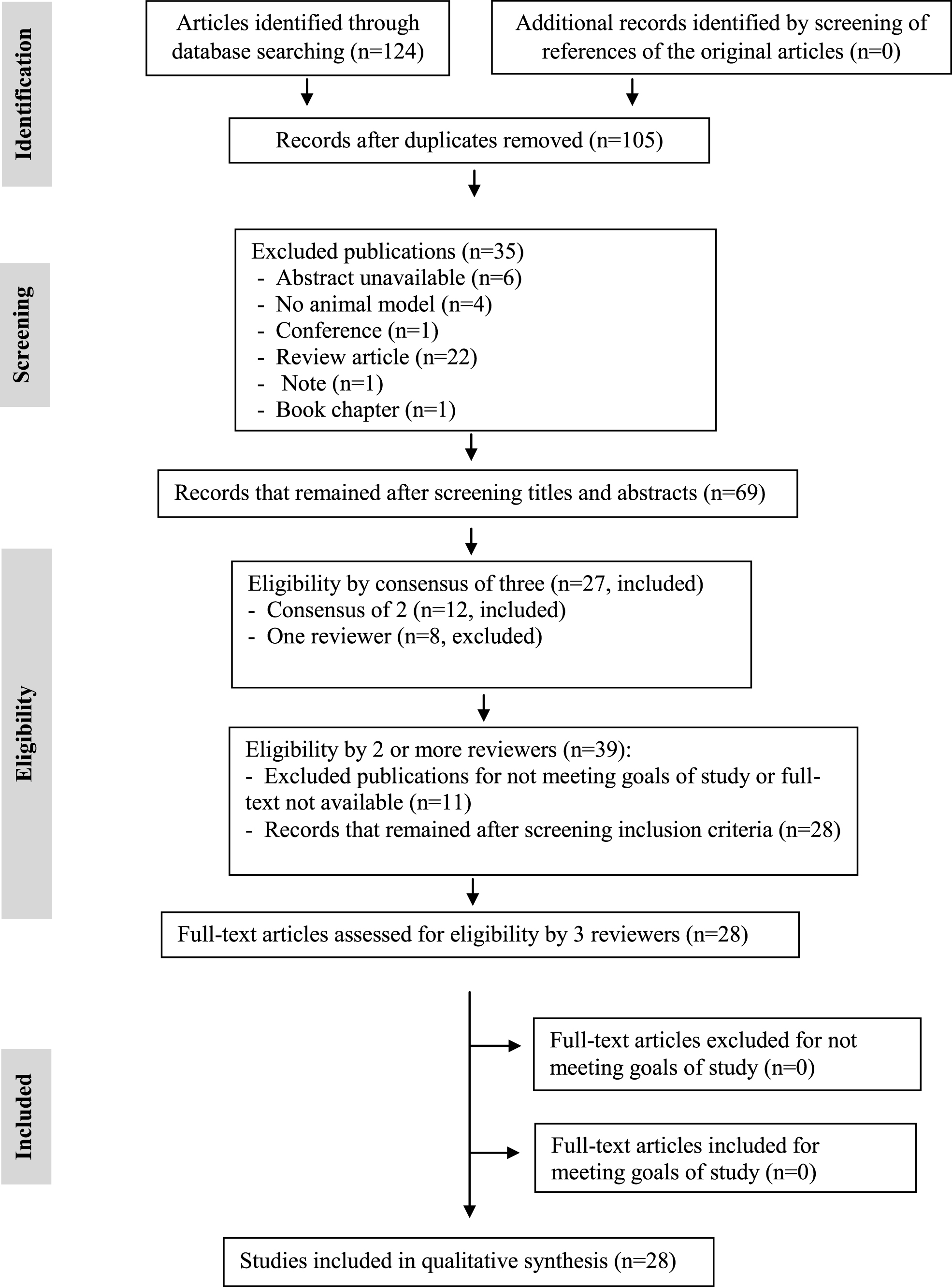
Most studies were based on the use of the Aβ protein for the development of the DNA vaccine, some in the absence of adjuvants (Table 1) [9, 21, 23–35], others used adjuvants in an attempt to amplify the vaccine’s efficacy (Table 2) [36–42], others compared DNA and protein vaccines (Table 2) [43] and some evaluated protein [44–46], adenovirus [47] and antibodies [48] boost regimens expecting to improve the immune response induced by the DNA vaccines (Table 2).
Table 1
General characteristics of DNA vaccine studies without adjuvants for Alzheimer’s disease
Reference; country | Objective | DNA vaccine | Animal model: species (n) | Gender (n) | Age | Statistical analysis |
Davtyan et al., [35]; USA | Report the immunological and therapeutic potency of the first anti-tau DNA vaccination. | AV-1980D | THY-Tau22 tg mice (6) | F | 3 m | Yes |
Evans et al., [21]; USA | Develop a DNA vaccine that induces anti-β antibody production without generating specific T cells against Aβ, and evaluate its efficacy in non-human primates. | AV-1955 | Rhesus Monkey (13) | M (13) | 2–8 m and 3–9 m | Yes |
Ghochikyan et al., [9]; USA | Verify the efficacy of a modified pre-existing DNA vaccine using an electroporation device in rabbits. | pN-3Aβ11-PADRE-Thep (AV-1955) | New Zealand White rabbits (23) | F (23) | NR | Yes |
Hara et al., [23]; Japan | Develop a new nasal DNA vaccine for AD, using the SeV recombinant vector, and evaluate its effects in mice. | rSeV-Aβ | APP-tg mice (Tg2576) | (24) | 12 and 24 m | Yes |
Kima et al., [47]; USA | Evaluate the efficacy of a DNA vaccine in preventing Aβ deposition in the brain and its improvement in behavioral deficits. | AdPEDI-(Aβ1-6)11 | Mo/Hu APPswe PS1dE9 (12) and C57BL/6 (8) mice | F (8) | 2 m | No |
Lambracht-Washington et al., [33]; USA | Show similar results found in mouse models on the efficacy and safety of the DNA Aβ42 trimer vaccine in rabbits. | DNA Aβ42 trimer | New Zealand White rabbits (16) | F (10) M (6) | 4–5 y | Yes |
Lambracht-Washington et al., [34]; USA | Test and show the effects of a DNA Aβ42 vaccination protocol in adult Rhesus macaques. | DNA Aβ42 trimer | Rhesus monkey (8) | F (4) M (4) | 6–10 y | Yes |
Matsumoto et al., [25]; Japan | Develop a new DN vaccine that acts in different Aβ types; evaluate the abilities of produced antibodies and its effects in Aβ reduction. | YM3711 | B6C3-Tg mice (9), New Zealand (6) White rabbits, and Cynomolgus monkey (4) | Mice and rabbit: NR Monkey: M (3) F (3) | Mice: 13–15 w; Monkey: 3–5 y | Yes |
Mouri et al., [26]; Japan | Investigate AAV/Aβ effects in mice. | AAV/Aβ | Non-transgenic mice (non-tg) (28) and APP (Tg2576) (28) | F (56) | 6–10 m | Yes |
Okura et al., [27]; Japan | Develop a safe and effective non-viral DNA vaccine against AD. | K-Aβ, IgL-Aβ; Aβ-Fc | Transgenic mice APP23 | NR | 3–4 m | Yes |
Okura et al., [28]; Japan | Evaluate vaccinated and non-vaccinated mice. | IgL-Aβ; Aβ-Fc | Transgenic mice APP23 and wild-type B6 | NR | 4 m | Yes |
Olkhanud et al., [29]; USA | Develop a DNA vaccine with hepatitis B virus components. | Aβ-CoreS | BALB/c, C57BL/6 and 3xTg-AD mice | NR | 4 m, 1 and 1.5 y | Yes |
Qu et al., [30]; USA | Develop an effective vaccination regimen for AD’s treatment and prevention without generating an autoimmune response. | Aβ42 plasmid | BALb/c wild-type (6) and (APPswe/PSEN1[A246E]) double Tg (6) mice | (12) | 2 m | No |
Qu et al., [32]; USA | Demonstrate that gene gun vaccination with Aβ gene generates high titles of anti-Aβ antibodies. | Aβ42 gene | APPswe/PS1DE9 mice (8) | (8) | 7–10 w | Yes |
Qu et al., [31]; USA | Compare three plasmid systems using a gene gun. | Gal4/UAS-Aβ42 monomer; Gal4/UAS-Aβ42 and CMV-Luc-Aβ42 trimer | BALB/c mice (20) | F (20) | 4–7 w | Yes |
AD, Alzheimer’s disease; w, weeks; m, months; y, years; Ig, immunoglobulin; SeV, Sendai virus; F, female; M, male; NR, not reported.
Table 2
General characteristics of DNA vaccine studies with adjuvants for Alzheimer’s disease
Reference; country | Objective | DNA vaccine | Animal model: species (n) | Gender (n) | Age | Statistical Analysis |
Davtyan et al., [45]; USA | Develop a DNA vaccine with a recombinant protein as a booster. | DepVac | C57Bl/6 mice (82) | F (82) | 5–6 w | Yes |
Davtyan et al., [36]; USA | Test LT-IS patches abilities in increasing the effectiveness of DNA and protein vaccines. | pCMVE/MDC-3Aβ11-PADRE (DepVac) | C57BL/6, 3xTg-AD, B6SJL and Tg2576 mice | F (77) | 5–6 w, 12–16 m, 4–6 m. | Yes |
Guo et al., [42]; China | Investigate the potential of an active Aβ immunization. | p(Aβ3-10)10-C3d-p28.3 | C57BL/6J mice | F (21) | 8–10 w | Yes |
Kim et al., [24]; USA | Investigate if a new vaccination regimen increases DNA vaccine efficacy by inducing a humoral response. | pCA-PEDI-(Aβ1-6)11 | C57BL/6J mice | F (21) | 2 m | Yes |
Kou et al., [37]; USA | Evaluate Aβ and simvastatin immunization for AD. | AdPEDI-(Aβ1-6) and pCA-PEDI-(Aβ1-6) | TgAPPswe/PS1dE9 mice (53) | M (26) F (27) | 11 m | Yes |
Lambracht-Washington et al. [43]; USA | Compare immune responses after a DNA trimmer and Aβ peptide immunizations. | DNA Aβ42 trimmer | B6SJLF1/J mice (16) | F (16) | 4–8 m | Yes |
Lambracht-Washington et al., [46]; USA | Evaluate the efficacy of two vaccination regimens and its possible side effects for AD. | Aβ1 - 42 DNA prime | B6SJLF1/J mice (24) | F (24) | 4–6 m | Yes |
Lambracht-Washington and Rosenberg, [48]; USA | Test a DNA immunization as a possible active immunotherapy for AD. | Transcription factor Gal4 and human Aβ1 - 42 | BALB/c-Foxp3–EGFP mice | M F | 3–8 m | Yes |
Movsesyana et al., [38]; USA | Develop a DNA vaccine with adjuvant and investigate its potential as a molecular adjuvant. | 3Aβ1–11-PADRE and 3Aβ1-11-PADRE-3C3d | C57BL/6 mice (15) | F (15) | 8–10 w | Yes |
Movsesyana et al., [39]; USA | Develop a different and safe DNA vaccine that can induce Strong anti-Aβ antibodies without generating an autoreactive T cell response. | pMDC-3Aβ1–11-PADRE | 3xTg-AD mice (23) | (23) | 3–4 m | Yes |
Movsesyana et al., [40]; USA | Test the immunological and therapeutic efficacy of a DNA vaccine in mice. | 3Aβ1–11-PADRE-3C3d | Tg2576 and 3xTg-AD (H2b haplotype) mice (27) | F (27) | 3–4 m | Yes |
Schultz et al., [44]; Switzerland | Describe an effective vaccination using a DNA plasmid with a small dose of peptide, without adjuvants. | pVR1012tPA-Aβ42 | SWE eTg (huAPP695.SWE; SwAPP) mice | NR | 4–6 w and 16–20 m | Yes |
Xing et al., [41], China | Test if a new DNA vaccine can induce a Th2 immune response. | p(Aβ3-10)10-CpG plasmid | BALB/c mice (24) | NR | 8 w | Yes |
w, weeks; m, months; y, years; LT-IS, immunostimulatory patches with Escherichia coli thermolabile enterotoxin; F, female; M, male.
Most articles were published in the United States (n = 17) [9, 21, 24, 29–40, 43, 45–48], followed by Japan (n = 5) [23, 25–28], China (n = 2) [41, 42], and Switzerland (n = 1) [44] (Tables 1 and 2). Only two, of the 28 studies, did not present statistical analysis to validate its results (Table 1) [24, 30]. To better understand the obtained results this systematic review was divided into four sections: DNA vaccines without adjuvants, DNA vaccines with adjuvants, a comparison between DNA and protein vaccines, and DNA vaccines followed by a booster.
DNA Vaccines
10 DNA vaccines were tested in different animal models, they were: AV-1955 (3Aβ11-PADRE-Thep) [9, 21], YM3711 (IgL-Aβx4-Fc-IL-4) [25], AdPEDI-(Aβ1-6)11 [24], rSev-Aβ [26], AVV-Aβ [23], Aβ-CoreS [29], K-Aβ [27, 28], IgL-Aβ [27, 28], Aβ-Fc [27, 28], Aβ42 [30, 32–34], and AV-1980D [35] (Table 1). The animals used for the efficacy and safety study of these vaccines were: monkeys (Rhesus and Cynomolgus [25]), New Zealand rabbits [9, 25, 33], and different species of mouse, wild type and transgenics (B6C3-Tg [25], 3xTg-AD [29, 39], BALB/c [29, 30], C57BL/6 [24, 29], Tg2576 [23, 26], TgAPP23 [27, 28], Non-Tg [26], APPswe/PSEN1 [30], Mo/HuAPPswe PS1dE9 [24], APPswe/PS1dEA [32], and THY-Tau22 [35] (Table 1).
AV-1955 Vaccine
Evans et al. [21] verified the efficacy of the AV-1955 vaccine (3Aβ11-PADRE-Thep - Aβ11 gene inserted into pET11d-myc6His-Tag plasmid) in male Rhesus monkeys (n = 13; eight months to three years old) (Table 1). The animals were separated into three groups: group 1 (n = 5) received intramuscular injections by electroporation from the vaccine (0.4 mg); group 2 (n = 5) received 4 mg; and group 3 received 2 mg of a control vector (Table 3). This vaccine induced the production of long-lasting and powerful antibodies without side effects, indicating that AV-1955 can be a candidate to initiate clinical trials [21] (Table 5).
Table 3
Treatment characteristics (dose and administration route) and analyzed parameters to verify efficacy and safety of DNA vaccines for Alzheimer’s disease in animal models
Reference | Groups (n) | Treatment (dose) | Administration route | Analyzed parameters |
Davtyan et al., [35] | Control (7) | Empty MultiTEP plasmid | IM (EP) | Detection of IFN-γ producing splenocytes, antibodies response and isotyping tau tangles in human brain tissue by IHC and confocal microscopy; western blot of brain from AD cases; immunohistochemistry; confocal microscopy, quantitative and biochemical analysis. |
Vaccinated (6) | AV-1980D (40μg/im; 7 doses) | |||
Evans et al., [21] | Group 1 (5) | AV-1955 (0.4 mg/DNA/animal) | IM (EP) | Detection of Aβ-specific antibodies and isotyping, IFN-γELISPOT assay, surface plasmon resonance analysis, detection of Aβ plaques in human brain tissues, neurotoxicity assay. |
Group 2 (5) | AV-1955 (4 mg/DNA/animal) | |||
Control (3) | Vector pVAX1 (2 mg) | |||
Ghochikyan et al., [9] | P3Aβ11-PADRE | p3Aβ11-PADRE(19.4μg/ml; 4 dose) | IM (EP) | Detection of anti-Aβ antibody responses by ELISA, immunoprecipitation, western blotting, purification of anti-Aβ11 antibodies, surface plasmon resonance analysis, neurotoxicity assay, detection of Aβ plaques in human brain tissues. |
AV-1955 (9) | pN-3Aβ11PADRE-Thep(0.5 ml; 4 doses) | |||
Hara et al., [23] | Control (10) | rSeV-LacZ (0.02 ml) | Nasal e IM | Pathological study, serum antibodies against Aβ42, Aβ42 measurements in brain tissue by ELISA, detection of Aβ oligomers in soluble fraction by Western Blot analysis, immunohistochemistry, behavioral analysis. |
rSeVNasal (7) | rSeV-Aβ; (0.02 ml) | Nasal | ||
rSeV IM (7) | rSeV-Aβ; (0.02 ml) | IM | ||
Kima et al., [47] | Mo/Hu APPswe PS1dE9 control (6) | AdGM-CSF | Nasal | Determination of serum titers with ELISA, cytokine-specific ELISA, immunoreactivity of antisera to amyloid plaques in the brain. |
Mo/Hu AdPEDI-(Aβ1-6)11 (20 ml) | AdPEDI-(Aβ1-6)11 (20 ml) | |||
C57BL/6 control (4) | ||||
C57BL/6 (4) | AdPEDI-(Aβ1-6)11 (20 ml) | |||
Lambracht-Washington et al., [33] | High dose (6) | DNA Aβ42 trimer (16μg/im; 5 doses) | ID (gene gun) | Determination of antibodies and peptides, necropsy reports and histology, plasma and splenocyte collection, Antibodies ELISA, dot blot assay, ELISA and ELISPOT assay, B cell ELISPOT, analysis of cell proliferation by CFSE dilution, AD mouse model brain immunohistochemistry. |
Low dose (6) | DNA Aβ42 trimer (8μg/im; 5 doses) | |||
Lambracht-Washington et al., [34] | Control (2) | – | Gene gun | Response of antibodies and peptides, blood work, antibody enzyme-linked immunosorbent assay, cytokine enzyme-linked immunospot and ELISA assay, analysis of cell proliferation by carboxyfluorescein succinimidyl ester dilution, immunohistochemistry of mouse brain. |
High dose (3) | DNA Aβ42 trimer (16μg/im; 6 doses) | |||
Low dose (3) | DNA Aβ42 trimer (8μg/im; 6 doses) | |||
Matsumoto et al., [25] | Mice (6) | YM3711 (100μg/animal; 6 doses) | IM | Tissue amyloid plaque immunoreactivity (TAPIR) assay, measurement of brain Aβ and anti-Aβ antibodies in plasma, western blot analysis of anti-Aβ oligomer antibodies and Aβ fibrils, purification of translated YM3711 product and anti-YM3711 P antibodies, binding and competition assays using YM3711P. |
Rabbit (6) | YM3711 (1 mg/animal; 6 doses) | |||
Monkey (6) | YM3711 (4 mg/animal; 6 doses) | |||
Mouri et al., 2007 [26] | AAV/control-(non-tg) (14) | AVV/control (0.1 ml; one dose) | Oral | Behavioral analysis, novel object recognition test, spontaneous alternation in a Y-maze test, Morris water maze test, cued and contextual fear conditioning tests, biochemical analysis, ELISA for detection of insoluble and soluble Aβ, western blot analysis for detection of Aβ oligomers in the soluble fraction, Berlin blue stain to detect the hemorrhagic lesion in the mouse brain, serum antibodies against Aβ42. |
AAV/Aβ (non-tg) (14) | AVV/Aβ (0.1 ml; one dose) | |||
AAV/control- Tg2576 (14) | AVV/control (0.1 ml; one dose) | |||
AAV/Aβ-Tg2576 (14) | AVV/Aβ (0.1 ml; one dose) | |||
Okura et al., [27] | Control | IgL-Aβ (100μg; 6 doses) | IM | Immunohistochemistry, quantitative analysis of Aβ burden, ELISA, T cell proliferation. |
IgL-Aβ | Aβ-Fc (100μg; 6 doses) | |||
Aβ-Fc | K-Aβ (100μg; 6 doses) | |||
K-Aβ | ||||
Okura et al., [28] | APP23 | (100μg) | IP | Immunohistochemistry, quantitative analysis of Aβ burden and microglia, western blot, RT-PCR, tissue amyloid plaque immunoreactivity assay, quantification of TNF in the CNS tissues and plasma Aβ with ELISA. |
Control | ||||
Olkhanud et al., [29] | Control | Aβ1 - 42(10μg) or PBS (100μl) | SC | T cell activation assay, elevated plus maze, fear conditioning, detection of Aβ immunohistochemistry, negative stain immunoelectron microscopy. |
4 months | Aβ-CoreS (25μg DNA/animal) | EP | ||
1 year | Aβ-CoreS (25μg DNA/animal) | EP | ||
1,5 year | Aβ-CoreS (25μg DNA/animal) | EP | ||
Qu et al., [30] | Control (4) | Vector without Aβ42 | Gene gun | Immunoassays, histological staining and examination. |
BALB (3) | DNA plasmid (2μg; 4 im) | |||
Tg (3) | Human Aβ1 - 42 and Aβ1-16 plasmid genes | |||
Qu et al., [32] | Control (4) | Proteic vectors non-Ahou and Aβ16 (11 im) | Gene gun | Immunoassay for detection of anti-Aβ42 antibodies in serum, ELISPOT, Enzyme-linked immunosorbent assay for detection of Aβ42 peptide in brain tissues and in plasma, immunofluorescence detection of Aβ42 plaques and quantification of amyloid burden. |
APPswe-PS1DE9 (4) | Aβ42 gene (1 Ag; 11 im) | |||
Qu et al., [31] | Gal4/UAS-Luc (4) | Gal4/UAS-Luc (4μg; 6–11 im) | Gene gun | Detection of expressed proteins by Western blot and ELISA, detection of Aβ42 protein in western blots, ELISAs for detection of anti-Aβ antibody, isotyping and epitope mapping, detection of senile plaques with vaccine generated immune sera. |
Gal4/UAS-Aβ42 monomer (4) | Gal4/UAS-Aβ42 monomer (4μ g; 6–11 im) | |||
Gal4/UAS-Aβ42 trimmer (4) | Gal4/UAS-Aβ42 trimmer (4μg; 6–11 im) | |||
CMV-LUC-Aβ42 trimmer (4) | CMV-LUC-Aβ42 trimmer (4μg; 6–11 im) |
IM, intramuscular; IP, intraperitonial; im, immunizations; SC, subcutaneous; EP, electroporation; ID, intradermal; TNF, tumor necrosis factor.
Table 4
Treatment characteristics (dose and administration route) and analyzed parameters to verify efficacy and safety of DNA vaccines with adjuvants for Alzheimer’s disease in animal models
Reference | Groups (n) | Treatment (dose) | Administration route | Analyzed parameters |
Davtyan et al., [45] | DepVac (82) | DNA vaccine (9μg; 4 doses) | ID (gene gun) | Concentration of anti-Aβ antibody and binding avidity, detection of Aβ plaques in human brain tissues, T-cell proliferation. |
PepVac (41) | Protein vaccine (50μg) | SC | ||
DepVac/PepVac (41) | DNA (9μg) and protein (50μg) | ID (gene gun) | ||
Davtyan et al., [36] | DNA control (16) | DepVac (6 w) and placebo patches | ID (gene gun) | Detection of anti-Aβ antibody concentration using ELISA, T cell proliferation, detection of cytokine production. |
DNA vaccine (16) | DepVac (6 w) and LT-IS | ID | ||
Protein control (19) | B6SJL: Lu AF20513 (50μg; 3 doses) and placebo patches | |||
Protein vaccine (19) | Tg2576: Lu AF20513 (50μg; 5 doses) and Alhydrogel ® patches | |||
B6SJL: Lu AF20513 (50μg; 3 doses) and LT-IS patches Tg2576: Lu AF20513 (50μg; 5 doses) and LT-IS patches | ||||
Guo et al., [42] | p(Aβ3-10)10-C3d-p28.3 (7) | p(Aβ3-10)10-C3d-p28.3 (500μg of plasmid/animal; 5 doses) | IM | Detection of serum anti-Aβ antibody concentrations and isotype, detection of splenic T cell proliferation and cytokines, detection of Aβ plaques in APP/PS1 transgenic mice brain. |
pcDNA3.1 (7) | Aβ42 peptide (50μg; 5 doses) and Freund’s adjuvants | |||
Peptide Aβ42 (7) | ||||
Kim et al., [24]. | Control (7) | PBS (2 doses) | Nasal | Determination of serum titers with ELISA, cytokine-specific ELISA, immunoreactivity of antisera to amyloid plaques in the brain. |
Plasmid and Vector (7) | pCA-PEDI-(Aβ1-6)11 ou pShut-CA-PEDI-(Aβ1-6)11 (100μg/DNA; 2 doses) and AdPEDI-(Aβ1-6)11 (1×108 PFU/vector; 2 doses) boost | |||
Mice (7) | pCA-PEDI-(Aβ1-6)11, pShut-CA-PEDI-(Aβ1-6)11 or AdPEDI-(Aβ1-6)11 | |||
Kou et al., [37] | PBS (14) | PBS and food | Nasal | Determination of total cholesterol levels in plasma, determination of anti-Aβ antibodies in serum and quantification in the brain by ELISA, histochemistry, and immunohistochemistry, Prussian blue reaction, Immunoblot analysis, cytokine/chemokine microarray, assessments of potential liver and muscle injury. |
Statin (14) | Statin (50 mg/kg/day/animal) and food | Oral | ||
Vaccine (11) | pCA-PEDI-(Aβ1-6) (100μg/animal) AdPEDI-(Aβ1-6)(1×108 PFU/animal) and food Statin (50 mg/kg/day); | Nasal | ||
Vaccine-statin (14) | pCA-PEDI-(Aβ1-6): 100μg/animal); AdPEDI-(Aβ1-6) (1×108 PFU/animal) and food | Nasal | ||
Lambracht-Washington et al., [43] | Aβ42 DNA trimmer (8) | Aβ42 DNA trimmer (4μg) | ID (gene gun) | Antibody titers, mapping of binding sites (epitopes), isotype profiles of the Aβ42-specific antibodies, T-cell activation, anti-Aβ antibody enzyme-linked immunosorbent assay, splenocyte proliferation assay, cytokine ELISA. |
Aβ42 human peptide (8) | Aβ42 human peptide (100μg/animal) and Quil A (20μg/animal) | IP | ||
Lambracht-Washington et al., [46] | Aβ42 DNA trimer (4) | Aβ42 DNA trimmer (6 doses) | Gene gun | Analysis of cell proliferation by CFSE dilution, ELISA and ELISPOT assays. |
DNA-peptide (4) | Aβ42 DNA trimmer (3 doses) followed by Aβ42 peptide (3 doses) | |||
Aβ42 peptide (4) | Aβ42 peptide (6 doses) | |||
Peptide-DNA (4) | Aβ42 peptide (3 doses) followed by Aβ42 DNA trimmer (3 doses) | |||
Lambracht-Washington and Rosenberg, [48] | Mice (2 doses) | DNA vaccine (4μg DNA/im) followed by an Ac injection | ID (gene gun) | Antibodies levels in plasma, flow cytometry, T cell stimulation in cell culture, cytokine analysis by ELISA and ELISPOT. |
Mice (3 doses) | DNA vaccine (4μg DNA/im) followed by an Ac injection | ID (gene gun) | ||
Control | Control Ac or TNFRSF4/25 Ac | IP | ||
Movsesyana et al., [38] | 3Aβ1–11-PADRE-3C3d (5) | 3Aβ1–11-PADRE-3C3d (9μg/animal; 5 doses) | Gene gun | Detection of plasmid expression by Western blot, detection of anti-Aβ antibody concentration and isotypes, detection of T cell response, detection of different cytokines concentrations in splenocyte culture media, detection of cytokines production by splenocytes, testing the functionality of generated anti-Aβ antibodies. |
3Aβ1–11-PADRE (9μg/animal; 5 doses) | ||||
pSecTag2A (9μg/animal; 5 doses) | ||||
3Aβ1–11-PADRE (5) Vector (5) | ||||
Movsesyana et al., [39] | pMDC-3Aβ1–11-PADRE (13) | pMDC-3Aβ1–11-PADRE (9μg/animal) | Gene gun | Anti-Aβ antibody in serum detection and functionality, T cell proliferation, production of cytokines induced by vaccination and different cytokines concentrations in brain extracts, behavior testing, determination of Aβ and tau levels in soluble/insoluble fractions, immunohistochemistry, and quantitative and semi-quantitative image analysis. |
pMDC-control (5) | pMDC-control | |||
Non-treated (5) | ||||
Movsesyana et al., [40] | p3Aβ1–11-PADRE-3C3d (9) | p3Aβ1–11-PADRE-3C3d (10μg; 9 im) | Gene gun | Biochemical and immunohistochemical analysis of mouse brains, anti-Aβ antibody response measured by ELISA. |
p3Aβ1–11-PADRE (9) | p3Aβ1–11-PADRE (10μg; 9 im) | |||
Control (9) | ||||
Schultz et al., [44] | Control | DNA or peptide | IM | Quantification of Aβ42 in brain lysates, Aβ42 ELISA for serum antibodies, thioflavin T binding assay, generation of Aβ42-specific monoclonal antibodies, epitope mapping, immunohistochemistry. |
Peptide | Aβ42 peptide (20μg; 12 im) | |||
Aβ42 DNA | DNA vaccine (200μg; 12 im) | |||
Passive im | Serum from vaccinated mice (200–300μg; 4 im) | |||
Xing et al., [41] | p(Aβ3-10)10-CpG (7) | p(Aβ3-10)10-CpG (100μg/; 6 im) | IM | Immunohistochemistry analysis, ELISA analysis for serum anti-Aβ antibodies, splenocyte culture, cytokine ELISA. |
pcDNA3.1 (+) empty vector (7) | pcDNA3.1 (+) (100μg; 6 im) | |||
PBS (6) | ||||
Aβ42 peptide (4) | PBS (100μl; 6 im) Aβ42 peptide (50μg; 6 im) |
IM, intramuscular; SC, subcutaneous; ID, intradermic; IP, intraperitoneal; im, immunizations.
Table 5
Results, bias, and conclusion of DNA vaccine studies for Alzheimer’s disease in animal models
Reference | Results | Bias | Conclusion |
Davtyan et al., [35] | Activation of specific T cells that initiated a robust humoral immune response against the tau protein, leading to a decrease in tau and its variations in mice brain, without side effects. | NR | AV-1980D is immunogenic and therapeutic in mice, suggesting that it can be used for inducing a strong immune response against the tau protein in AD. |
Evans et al., [21] | AV-1995 induced strong and long-lasting anti-Aβ antibodies, and a specific cellular immune response to antigenic epitopes. | NR | The studies showed that a DNA vaccine might be appropriate for clinical trials. |
Ghochikyan et al., [9] | AV-1955 induced a rapid and intense anti-Aβ antibody response with binding affinity to different Aβ forms, showing its therapeutic potential. | Yes | This vaccine can be an effective option for AD patients. |
Hara et al., [23] | Nasal administration of the vaccine resulted in the decrease of amyloid burden, including Aβ oligomer, and improved cognitive functions without side effects. | NR | Vaccination protocol is safe, non-invasive and long-lasting, proving to be an alternative for AD’s immunotherapy. |
Kima et al., [47] | Vaccinated animals showed a Th2 cellular immune response, generating IgG1 isotype antibodies that were able to reduce Aβ burden in the brain. IL-4 was also found, suggesting that the addition of this cytokine as an adjuvant can potentialize the immune response. | Yes | Nasal vaccination was effective in producing therapeutic antibodies. |
Lambracht-Washington et al., [33] | Absence of skin inflammation and irritation on the injection site and in the brain of the rabbits. The larger mammals presented a similar response to the previous mouse model analyzed, showing a good response of anti-Aβ antibodies. | NR | DNA Aβ42 is highly likely to be a safe and effective prevention therapy for AD’s clinical trials. |
Lambracht-Washington et al., [34] | Production of high titers of anti-Aβ42 IgG and IgA antibodies capable of detecting a wide variety of Aβ1 - 42 peptide with no indication of an inflammatory cellular immune response. | NR | The DNA Aβ42 trimer vaccine might have a positive outcome in clinical trial patients with early AD, since it showed a good humoral immune response and no inflammation. |
Matsumoto et al., [25] | The vaccine induced high titers of antibodies against different Aβ forms, being able to reduce Aβ deposits without side effects. | NR | The decrease of Aβ in the brains suggests that this vaccine must be more studied. |
Mouri et al., [26] | Improvement in progressive cognitive loss through a decrease in Aβ burden, Aβ soluble and insoluble oligomer, microglial attraction and synaptic degeneration; absence of lymphocytic infiltration and microhemorrhages in vaccinated mice brain. | – | Vaccine was able to decrease Aβ burden and attenuate cognitive and histological impairments without side effects, suggesting that it can be an effective and safe immunotherapy for AD. |
Okura et al., [27] | Vaccine led to decrease in amyloid plaques without side effects, even after being administrated for a long period. | NR | Non-viral vaccines are safe and highly effective in reducing amyloid plaques in mice brain, therefore being a therapeutic promise against AD. |
Okura et al., [28] | Vaccine significantly increased the number of microglia around amyloid, however even when activated they did not produce significant amounts of TNF; also, increased plasmatic concentrations of Aβ and amyloid plaques immunoreactivity. | Yes | The study showed that Aβ deposits are phagocytized by microglial cells. |
Olkhanud et al., [29] | Vaccine reduced amyloid plaques, improving cognitive deficits and increased life expectancy. | NR | The vaccine can effectively fight AD’s alterations and be beneficial for patients’ survival. |
Qu et al., [30] | Aβ42 gene can effectively activate a humoral immune response without an exacerbated T cell response against Aβ. | Sim | The vaccine induced a significant humoral immune response, without exacerbated T cell response, showing that it can be an alternative for AD’s treatment and prevention. |
Qu et al., [32] | Vaccine generated high antibodies titles with a Th2 immune response and decreased Aβ42 levels in mice brain. | NR | Effective vaccine protocol to break host tolerance from Aβ, induce a Th2 immune response and decrease amyloid plaque. |
Qu et al., [31] | Aβ42 trimmer and Gal4/UAS vaccination generated high levels of anti-Aβ antibodies from IgG1 isotype, suggesting a Th2 immune response. | NR | Potential vaccination protocol for clinical trials in AD patients. |
AD, Alzheimer’s disease; Th, T helper lymphocyte.
In opposition to protein vaccines, DNA vaccines must be inserted into the cell and cross the cytoplasm and nuclear membranes, in order to be effective and induce antigen expression, for that new technologies such as gene gun and electroporation (EP) have been explored. Gene gun uses a bombarding DNA/gold particles technique into the skin surface, allowing DNA molecules to be captured and expressed by dendritic cells (DC) for antigen processing and presentation, being capable to induce strong specific immune responses even with low doses of DNA [49]. Thereafter came the development of the electroporation that showed greater effectiveness in muscle cells when compared to gene gun [50]. Intramuscular EP increased muscle cell permeability, allowing the insertion of the DNA molecule inside the cell and subsequent protein expression [51]. Moreover, increased distribution and capture of DNA molecules at the injection site, and the use of electric pulses afterward attracted immune cells (e.g., dendritic cells). Thus, EP increased the magnitude and duration of an antigen-specific immune response [52].
Ghochikyan et al. [9] used a modified version of the AV-1955, in which the N-terminal free region of the Aβ11 gene was conjugated with an aspartic acid; the plasmid used was pVAXI. The tests were carried out in white female New Zealand rabbits; each animal received 0.5 mL of the DNA vaccine (pN-3Aβ11-PADRE-Thep) by electroporation in four doses (Table 3). This vaccine induced the production of antibodies capable of recognizing all forms of the Aβ peptide, binding with amyloid plaques in the brain and inhibiting the neurotoxicity mediate by Aβ oligomerization and fibrillation [9]. These results suggest that this vaccine represent an effective therapy for AD, but other studies must be made, since its safety was not evaluated due to the lack of laboratorial methods to detect immune cellular response in rabbits. Yet, authors believe that this vaccine will not induce an immune auto reactive response in humans, but clinical trials have yet to be carried out [9] (Table 5).
The N-terminal region of Aβ has been a target for AD treatments; however, using antibodies against this region may be harmful since it can shift non-toxic Aβ fibrils to soluble Aβ oligomers with high neurotoxicity which could induce or exacerbate the disease [53]. Healthy people have antibodies anti-Aβ oligomers, showing their importance in AD’s natural protection, and do not have anti-N terminal region antibodies [54].
Davtyan et al. [55] compared AV-1955 with a modified version of it (AV-1959) in Rhesus monkeys. In order to generate a higher immunogenicity, new T cell epitopes were added to the vaccine structure. In spite of both not stimulating autoreactive T cells, activating a variety of specific T cells and inducing the production of anti-Aβ antibodies, the new vaccine presented itself as a better therapeutical candidate. It generated stronger humoral and cellular immune responses and was able to activate naïve T cell e memory cells, something beneficial to overcome immune alterations that happen with aging [55].
YM3711 vaccine
Matsumoto et al. [25] tested the DNA vaccine YM3711 in different animal species. The vaccine was constructed from the combination of four tandem repeats of the Aβ1 - 42 gene with the genes for the constant portion of the immunoglobulin (Fc) and interleukin 4 (IL-4) (IgL-Aβx4-Fc-IL-4). B6C3-Tg mouse from 13 to 15 weeks (n = 9), New Zealand white rabbits (n = 6), and Cynomolgus monkeys (n = 4) from three to five years old (Table 1) were given the vaccine intramuscularly in the dosages 100μg, 1 mg, and 4 mg, respectively (Table 3) [25]. YM3111 induced the production of antibodies against diverse Aβ species and other amyloid peptides in all the animals, and generated a significative reduction of these molecules in mouse brains; showing that this vaccine should be further studied (Table 5) [25]. Until the present moment, no other publications were found in this DNA vaccine.
IL-4 is an anti-inflammatory cytokine produced by Th2 cells, being the greatest inducer of macrophages from the M2a phenotype immune response [56]. This response has indicated attenuations and improvements on the neurotoxicity of Aβ burden on the brain [57]. Latta et al. [58] studied the expression of IL-4 in mouse after injection with the AAV adenovirus, and their results have shown a M2a phenotype induction with increased activation of microglia and astrocytes and slight reduction of Aβ. Suggesting a controversy, because the presence of these cells tend to represent a pro-inflammatory response and they present themselves significantly higher in M2a presence, an anti-inflammatory macrophage phenotype [58].
Moreover, this cytokine has also been used as a base treatment for AD with the goal of reverting certain pathological aspects of the disease and generating a neuroprotective effect on animal models [59]. However, Li et al. [60] and Soosnabadi et al. [61] found that single nucleotide polymorphism (SNP) in the IL-4 gene can contribute to AD susceptibility. In the Chinese population, the –590C/T and –1098T/G SNPs were associated with AD [60]; in addition, –590C carriers may have diminish IL-4 levels in early stages of cerebral lesions, reducing its anti-inflammatory effects, and consequently facilitating new lesions that could possibly have increased AD susceptibility [60]. –590C/T SNP has also been associated with the development of late-onset AD in Iran, showing that this allele (IL-4 –590C/T) can be applied as an AD marker in the population [61].
AdPEDI-(Aβ1-6)11 vaccine
Kim et al. [47] evaluated the efficacy of AdPEDI-(Aβ1-6)11 vaccine in Mo/HuAPPswe PS1dE9 (n = 12) and C57BL/6 (n = 8) mouse (Table 1). The vaccine was formed by eleven tandem repeats of the Aβ1-6 gene in an adenoviral vector with a linking domain for the enterotoxin A from Pseudomonas (PEDI). All animals received nasally 20 mL of the vaccine (Table 3). This vaccine induced the production of IgG1 type antibodies, indicating a Th2 cellular immune response, that was able to react against the amyloid plaques and other Aβ species, reducing Aβ burden on mouse brains. Yet, it was capable of increasing IL-10 levels, suggesting that a Th2 response is controlled by IL-10 since this cytokine inhibits dendritic cells that are responsible for Th1 cellular immune responses (Table 5) [47].
A Th2 immune response that induces anti-inflammatory cytokine production (e.g., IL-4, IL-10) is required in neurodegenerative diseases like AD because it prevents cerebral damages caused by pro-inflammatory cytokines. This type of response can be gained by Th2 agonists or adjuvants. A Th1/Th2 response will, most likely, lead to cell death, whereas Th2 immunity will lead to protective non-cytolitic antibodies production, isotype shifts and inhibition of Th1 cells route [54]. Still, T CD4+ cells with Th1 cytokine profile support a systemic inflammatory response, which is very useful against infections and tumors, but not for degenerative diseases [54].
rSev-Aβ vaccine
Hara et al. [23] used the recombinant virus vector Sendai (Sev) with the LacZ-cDNA gene to develop a new DNA vaccine (rSev-LacZ) consisting of a cDNA from the Aβ1-43 gene and a cDNA from the interleukin-10 from mice (Table 1). The vaccine was tested in 12 to 24 month-old Tg2576 mouse who received 0.02 mL of rSev-LacZ (Table 3) [23]. The results showed a decrease of the soluble and insoluble fractions of Aβ and a reduction of its oligomers in the animal’s brain, and yet, there was an improvement of their cognitive skills. This immunotherapy might be considered as an alternative for the treatment of AD due to its advantages of being safe, relatively long-lasting, and non-invasive. Also, demonstrated that the nasal vaccine was effective in inducing the production of therapeutics antibodies [23] (Table 5).
Viral vectors have the advantage of being effective in, mostly, only one injection and dissipate easily through the cells [62], however they are originated from pathogens, thus, presenting risks of mutation and enabling by the complement system, besides being non-indicated to immunosuppressed individuals [63], needing to be used carefully. The Sendai virus is a parainfluenza virus (PIV) murine and presents a certain similarity to the human PIV, therefore was tested as a xenotropic vaccine in African green monkeys and human beings, not showing significant adverse reactions [64]. The safety of the Sendai Virus is still being established, in their study, Hara et al. [23] did not demonstrate any adverse reaction. Other studies have been evaluating the virus capacity to induce the production of pluripotent cells from peripheral human T cells [65] and its potential for treating cancer [66].
AAV-Aβ
Mouri et al. [26] evaluated the action of a recombinant adenovirus associated to an adenovirus (AAV-Aβ) with a cDNA from the Aβ1-43 gene, utilizing the pTRUF2 plasmid in non-transgenic female (n = 28) and Tg2576 (n = 28) mouse (Table 1). A single dose of this vaccine was able to improve cognitive functions and decrease soluble and insoluble deposits and Aβ oligomers, without microhemorrhages or leucocyte infiltration. Yet, attenuating histological and cognitive abnormalities, proving to be a safe and effective immunotherapy for AD (Table 5) [26].
To better improve Aβ therapy, Hara et al. [67] investigated the efficacy of the oral vaccine rAAV/Aβ (Aβ1-43 cDNA) in Cynomolgus (n = 10) and African green (n = 12) monkeys. The results showed a significant decrease of amyloid plaques and intracellular Aβ in the brain without side effects (e.g., inflammation and cerebral microhemorrhages). However, there was an increase of soluble and insoluble Aβ oligomers, demonstrating that this vaccine seems safe, but should be used carefully. rAAV/Aβ can be recommended in the early stages of AD, while there are only a few amount of Aβ deposits [67].
Vacina Aβ-CoreS
Olkhanud et al. [29] combined the surface antigen of the hepatitis B virus (HBsAg) with the Aβ1-11 gene, creating the DNA Aβ-CoreS vaccine which was inserted in the mammalian vector pVAX1 and analyzed in BALB/C, C57BL/6 and 3xTg-AD mouse (Table 1). These animals had a decrease in cerebral amyloid plaques, high titers of antibodies, improvement of cognitive functions and longer life-span. The study proposed that the vaccine against the Aβ1-11 gene can be used effectively and safely to tackle alterations generated by AD and be beneficial to patients with the disease (Table 5) [29].
In many countries, as Brazil, the immunization against the hepatitis B virus (HBV) is preconized. This procedure leads to the development of antibodies and T and B memory cells that protect individuals in case of exposure to the HBV. It is believed that using boosts containing conventional HBV epitopes (e.g., HBsAg) as a strong molecular adjuvant, might be sufficient for the rapid activation of pre-existing memory cells, resulting in a strong production of anti-Aβ antibodies in the elderly, and also increasing their defense against the formation of Aβ plaques [68].
K-Aβ, IgL-Aβ, and Aβ-Fc vaccines
Okura et al. [27] developed three DNA vaccine (K-Aβ, IgL-Aβ, and Aβ-Fc) and tested them in three and four-month-old Tg-APP23 mouse; each animal received a 100μg DNA injection (Tables 1 and 3). K-Aβ was made from the insertion of the Kozak sequence at the 5’ terminal of the Aβ1-42 gene, while at the IgL-Aβ there was the addition of the signaling sequence Igκ from mice at the 5’ terminal from the Aβ1-42 gene as a way to improve the secretory efficacy of the Aβ peptide, and Aβ-Fc was developed by the insertion of the Fc portion of human immunoglobulin to the 3’ terminal from the Aβ1-42 gene in order to stabilize the secretory protein. The expression vector used in all vaccines was the mammalian vector pTarget [27].
IgL-Aβ and Aβ-Fc were able to decrease intra and extracellular amyloid plaques and increase anti-Aβ antibodies levels without neuroinflammation and T cell proliferation (Table 5) [27]. These promising results lead Okura et al. [28] to verify the mechanisms of Aβ decrease in Tg-APP23 mouse aged four to 15 months immunized with 100μg of IgL-Aβ or Aβ-Fc (Tables 1 and 3). From these results, it was possible to observe the production of antibodies that stimulated the activation of microglia, increasing its phagocytic activity on Aβ deposits, suggesting the important role of these cells in this peptide decrease after the immunotherapy with the DNA vaccine [28]. Non-viral DNA vaccines are safe and highly effective in reducing amyloid plaques in mouse brain, being a possible therapeutically promise against AD (Table 5) [27].
Although there is strong evidence that microglial activation favors AD’s progression [69,70], there was no such association in this study, due to the production of tumor necrosis factor (TNF), an important inflammatory cytokine that was not present in significant quantities; microglial phagocytosis was increased only to Aβ deposits and not other targets, avoiding unnecessary inflammatory reactions; use of immunoglobulin attached to the Aβ vaccine, which led to a Fc mediated phagocytosis specifically to Aβ plaques [28].
Aβ42 DNA Vaccine
Qu et al. [30] and Qu et al. [32] evaluated a new immunization strategy using the Aβ gene (DNA Aβ42) in BALB/C, APPswe/PSEN1 and APPswe/PS1DE9 mouse (Table 1). The vaccine was developed with the Aβ42 gene, the secretory signaling sequence from the human α-antitrypisin and the major histocompatibility complex II (MHC-II). All animals present an efficient humoral immune response capable of decreasing the Aβ42 peptide in their brain without a significant cellular immune response (Table 5). From this vaccine, it was possible to verify the break-in host tolerance to the Aβ42 without adjuvants, and yet, inducing a Th2 immune response and decreasing amyloid plaques. This protocol is a potential candidate for clinical use in AD patients [30, 32].
Qu et al. [31] compared three plasmid systems using an Aβ42 monomer and trimer along with the transcription yeast factor Gal4 (Gal4/UAS-Aβ42 monomer and Gal4/UAS-Aβ42 trimer) and a trimer with the cytomegalovirus (CMV) promoter as an attempt to increase antibodies titers (CMV-Aβ42) (Table 1). Four to seven-week-old female BALB/C mouse (n = 20) were used (Tables 1 and 3). The Gal4/UAS-Aβ42 trimer system was able to induce high titers of IgG1 antibodies capable to identify amyloid plaques, being a possible therapeutic candidate for AD prevention (Table 5) [31].
Besides Th2 cell activation and higher production of specific IgG1 for amyloid plaques, this vaccine increased the production of regulatory T cells (CD4+, CD25+, and FoxP3+) and IL-10, resulting in the downregulation of effective T cells (Th1 and Th17) [71].
As seen in the prior study and many others present in this systematic review, the majority of animal models used are female, which can be considered a bias, since some studies show differences in efficacy related to a difference in hormonal concentration between genders [72, 73]. Llort et al. reviewed the differences in neuroimmunoendocrine pathways in AD’s etiopathogenesis of male and female 3xTg-AD mice and showed that the impairments of aging in this pathway are more evident in male, which could be responsible for the increased morbidity and mortality of these animals [74]. In many species, including humans, it is well established that females live longer than males, and a factor that can be involved in this is the levels of corticosteroids, which are more elevated in females. Estrogen has been suggested as the one responsible for female’s higher immunocompetence and stronger antibodies and cellular immune responses [72, 73]. Suggesting that the differences in gender must be taking into account both in animal and human research when analyzing the pathology and therapeutic strategies for AD [74].
Lambracht-Washington et al. [33, 34] evaluated the effects of an Aβ42 DNA trimer vaccine in New Zealand White rabbits (n = 16, four to five years old) and Rhesus monkeys (n = 8; six to ten years old), respectively. Both studies were composed of two vaccinated groups, a low and a high dose, in which each animal was immunized with 8 and 16μg of the vaccine by gene gun, the rabbits received a total of five doses, meanwhile, the monkeys received six (Tables 1 and 3). The results from these studies were promising, both species presented high antibodies titers from IgG and IgA isotope, which might indicate a collaboration among them in removing the excess of amyloid from the brain, and neither species showed signs of an inflammatory cellular immune response [33, 34] (Table 5). Therefore, this vaccination protocol seems to be effective and safe in large mammals, suggesting a positive outcome for its use in AD’s clinical trials. Another important result from Lambracht-Washington et al. was the absence of skin inflammation and irritation on the vaccination site of the rabbits, which suggests similar results in human skin since rabbit skin is a great mark for chemical agents and other stimuli that causes irritation [33, 75, 76].
AV-1980D vaccine
Davtyan et al. reported the immunological and therapeutic potency of the first anti-tau DNA vaccine (AV-1980D) in female THY-Tau22 tg mice (n = 13; three-month-old) (Table 1) [35]. AV-1980D consists of the Igκ-chain signal sequence, three copies of the tau2 - 18 B cell epitope linked to the MultiTEP platform, the 2-18 amino acids target on the vaccine were chosen due to the presence of the phosphatase-activating domain (PAD), which is exposed during tau aggregation and is important in the polymerization of tau [77]. The animals were separated into two groups, the control group (n = 7) and the vaccinated group (n = 6) and received an empty MultiTEP plasmid and 40μg of the vaccine seven times in different intervals, respectively (Table 3) [35]. The immunized animals had high titers of anti-tau antibodies that reduced total tau accumulation and were able to recognized tangles in human AD brain tissue, indicating a possible alternative for AD’s prevention and treatment (Table 5) [35].
AD is characterized not only by the accumulation of Aβ inside the neurons but also by the hyperphosphorylation and aggregation of the tau protein inside these cells. In healthy cells, tau is important for the regulation and stabilization of the microtubules; however, neurodegenerative diseases lead to its conformational change, disassociation from the microtubules and self-aggregation [78]. Also, these microtubules alterations harm the axonal transport of substances within the cells through the activation of a signaling cascade located in the PAD [79]. Tau, alone, does not inhibit this transport, only when aggregated does it happened, most likely due to its conformational change and consequent exposure of PAD [80], thus using this domain might bring great benefits for a vaccine-induced immune response.
DNA Vaccines with Adjuvants
DNA vaccines lead to the synthesis of small quantities of high-affinity antibodies and, in order to improve this response, adjuvants can be used, preferably Th2 adjuvants that will induce a safe anti-inflammatory immune response, at the same time as it inhibits Th1 and Th17 responses [81, 82]. Adjuvants can regulate the type of generated immune response against immunogenic substances containing Aβ, altering to a Th1 (pro-inflammatory) or Th2 (anti-inflammatory) response, possibly important to avoid adverse reactions due to high quantities of cytokines and chemokines. In addition, it becomes important the determination of antibody isotope in response to the vaccine. This isotope determines the various types of effector functions (complement system activation or macrophages activation from Fc receptors) that can be induced when the antibody connects with its target and form immunocomplexes [68]. In this review, the adjuvants utilized were: simvastatin [37], Escherichia coli thermolabile enterotoxin [36], C3d component [38, 40, 42], CpG sequence [41], and macrophage-derived chemokine (MDC) [39].
Simvastatin
Kou et al. [37] evaluated the efficacy of a DNA vaccine with simvastatin, a drug effective in reducing cardiovascular diseases morbidities. In this study, the simvastatin was conjugated with the Aβ1-6 gene and inserted in the pCA-PEDI(Aβ1-6)11 plasmid, and tested in Tg-APPswe/PSQdE9 mice (n = 53, 22 months old); all vaccinated animals received an adenovirus boost (AdPEDI-(Aβ1-6)11) and 50μg of Aβ-KLH, an immunostimulatory carrier protein associated with the Aβ1-8 gene (Tables 2 and 4). This immunization regime induced moderate antibodies titers that was able to increase the solubility of amyloid plaques, however not significantly [37]. This may have happened due to the ‘specific-epitope suppression phenomenon’ induced by the carrier (CIESS) [83], a phenomenon in which antibodies that were supposed to act against the Aβ protein, are actually competing with antibodies against the carrier protein [84].
After stimulating the synthesis of IL-10 and promoting a Th2 cellular immune response, at the same time as it inhibited a CD45+ cell invasion (phagocytic microglia of Aβ protein activated by the vaccination), simvastatin proved to be efficient in preventing a exacerbated immune response when associated with the DNA vaccine, therefore being a great adjuvant [37] (Table 6).
Table 6
Results, bias, and conclusion of DNA vaccine studies with adjuvants and boosts for Alzheimer’s disease in animal models
Reference | Results | Bias | Conclusion |
Davtyan et al., [45] | DNA followed by protein recombinant boost vaccinated animals presented an increase in anti-Aβ antibodies that were long lasting and presented high avidity for the Aβ42 peptide. | NR | A heterologous vaccination regime can be effective for the development of a potent AD vaccine. |
Davtyan et al., [36] | LT-IS patches significantly increased anti-Aβ antibodies response in immunized mice in both DNA and protein vaccines. | NR | LT-IS patches improved immunization protocols for both DNA and protein vaccines. |
Guo et al., [42] | Production of anti-Aβ antibodies capable of binding to brain amyloid plaques and a Th2 immune response profile. | NR | The vaccine is a promising option for AD’s immunotherapy. |
Kim et al., [24] | Antibodies produced by the DNA vaccine followed by an adenovirus boost were IgG1 isotype, showing that this vaccination protocol resulted in a Th2 immune response. | NR | Heterologous vaccination strategies can make AD’s immunotherapies more effective in reducing Aβ burden. |
Kou et al., [37] | Due to modest titles of antibodies, an additional Aβ1-8-KLH boost was administered, leading to an increase in antibodies title and Aβ protein solubility. Simvastatin was able to inhibit CD45 cell invasion, suggesting its effectiveness in reducing inflammatory process. | Yes | The results show that more studies on prevention of side effects associated with Aβ’s immunotherapy with simvastatin must be performed. |
Lambracht-Washington et al., [43] | Aβ42 DNA trimmer immunization reduced Aβ42 levels in mice brain, produced a Th2 immune response and appears to present a small potential of T cell inflammatory response. | NR | Aβ42 DNA trimmer immunization resulted in a strong Th2 immune response with great chances of a non-inflammatory profile, thus being a safe option to decrease AD’s progression |
Lambracht-Washington et al., [46] | Both regimens significantly increased antibodies production. Aβ42 DNA trimmer did not stimulate cytokine production and T cell proliferation. | NR | Peptide-DNA protocol appears to be the safest and most advantageous, as it presents a strong antibody response and a small risk of inflammatory responses. |
Lambracht-Washington et al., [48] | Immunization led to a Th2 immune response with high titers of antibodies that induced T regulatory cells, and co-stimulation with Ac amplified these cells effects. | NR | Aβ42 DNA vaccine generated a minimum inflammatory and autoimmune reaction risks. |
Movsesyana et al., [38] | Vaccine alone generated moderate titles of antibodies, with the adjuvant, however, there was an increase in antibodies and a shift to a Th2 immune cellular response. | Yes | Adjuvant presence increased the immune response and lead them to a Th2 profile response. |
Movsesyana et al., [39] | Prophylactic immunization with DNA generated a Th2 intense immune response and high titers of antibodies that inhibited Aβ accumulation in mice brain; decreased glial cell activation and prevented behavioral deficits in older animals, without side effects. | Yes | The vaccine can be used as a safe and effective AD therapeutic strategy, but more studies in bigger animals are needed. |
Movsesyana et al., [40] | Both responses were weak, even after using a molecular adjuvant able to increase antibodies response to the vaccine. | NR | Low potency of the DNA vaccine in Tg2576 mice, confirming that in small concentrations, antibodies are not able to reduce amyloid plaque. |
Schultz et al., [44] | Immune serum and monoclonal antibodies solubilized Aβ42 burden and were able to recognize amyloid plaques in mice brain, and passive immunization quickly reduced amyloid plaques from this region. | Yes | Vaccination protocol induced a Th2 response with high antibodies’ titles, suggesting that it can be a therapeutic option for early stages of AD. |
Xing et al., [41] | DNA vaccine induced high titles of antibodies from IgG1 isotype, alongside an increase in IL-4 synthesis and low IFN-γ response. | NR | Vaccine appears to be non-inflammatory and therefore safe for AD’s treatment. |
LT-IS, immunostimulatory patches with Escherichia coli thermolabile enterotoxin; IFN-λ, interferon gamma; Ig, immunoglobulin; AD, Alzheimer’s disease.
Escherichia coli thermolabile enterotoxin
Davtyan et al. [36] evaluated if patches with the Escherichia coli thermolabile enterotoxin (LT-IS) could increase the efficacy of DepVac (pCMVE/MDC-3Aβ11-PADRE, constructed with the Aβ1-11 gene inserted in the mammalian expression plasmid pCMVE-Aβ/MDC) and PepVac (MDC-3Aβ11-PADRE consisted of three copies of B cell epitopes of the Aβ1-12 gene and two copies of Th cell epitopes from the tetanus toxin) in C57BL/6, B6SJL, 3xTg-AD and Tg2576 mice (Table 2). All animals who received the LT-IS had their immune responses significantly enhanced followed by the production of specific antibodies and T cells, demonstrating that the patches increased the amplitude of anti-Aβ antibodies, thus improving the immunization protocol of the DNA and protein vaccines [36] (Table 6).
Moreover, a group of animals received Alhdrogel ®, an alum-based adjuvant, so that the difference between it and the LT-IS could be evaluated. Alum (potassium and aluminum sulfate) is the only approved adjuvant for humans vaccines use, even though it is not considered highly effective and has low efficacy when used in the elderly population [82]. Alum has been used to prevent a mix immune response (Th1/Th2) however its low activity did not improve the efficacy of vaccines in animals and elderly [85]. Another concern is its increase neurotoxicity due to its cerebral translocation and persistence [85]. Therefore, it should be carefully applied in order to avoid the unnecessary usage and consequent adverse reactions.
C3d complement
Guo et al. [42], Movsessyan et al. [38], and Movsessyan et al. [40] used the molecular adjuvant C3d complement in their DNA vaccine design. Guo et al. [42] used eight to ten-month female C57BL/6J and twelve-month transgenic mice immunized with p(Aβ3-10)10-C3d-p28.3, vaccine developed with ten tandem repeats of human Aβ3-10 gene and three tandem repeats of cDNA from the C3d-p28 mice gene (Table 4) [42]. The DNA vaccine was able to induce high IgG1 antibodies titles that were capable of strongly connecting to the amyloid plaques, suggesting a Th2 immune cell response, leading to a more efficient removal of cerebral amyloid plaques without causing an excessive inflammatory response. Thus, this vaccine may be a new safe and effective candidate for AD’s immunotherapy (Table 6) [42].
Another DNA vaccine studied was the 3Aβ1-11-PADRE (3Aβ1-11-PADRE-3C3d) which contains the Aβ1-11 gene inserted in the pSecTag2A mammalian expression vector [38, 40]. Movsessyan et al. [38] tested this vaccine in C57BL/6 mice (n = 15, aged between eight and ten weeks). All animals presented great cellular and humoral immune responses which were shifted to the Th2 phenotype, therefore a non-inflammatory immune response [38]. Tg2576 mice were analyzed by Movsessyan et al. [40], however the animals presented a low immune response, demonstrating that low concentrations of antibodies were not enough to reduce amyloid plaques in their brains and the number of antibodies must be essential to improve the AD pathology (Table 6) [40].
C3d complement is an adjuvant with the potential to induce B cell response against a specific antigen from the vaccine [86]. The C3d fragment connects with the receptor 2 from the complement (CD21), inducing the antigenic recognition and, consequently, the innate and adaptive immunity [86]. This connection, alongside the linkage with the B lymphocyte antigen receptor (BCR), increases the antigenic recognition strength and induces a strong specific activation of B lymphocytes [86]. For these reasons, this fragment is one of the most promising adjuvants to be used in vaccines.
CpG sequence
Xing et al. [41] developed a DNA vaccine (p(Aβ3 - 10)10-CpG) using the CpG adjuvant (immunostimulatory sequence from the bacterial DNA) and ten tandem repeats of the Aβ3-10 gene inserted in the eukaryotic expression vector pcDNA3.1(+). BALB/c (n = 24, eight weeks old) mice were inoculated with 100μg of the vaccine in each immunization protocol (Tables 2 and 4). The animals produced IgG1 isotope antibodies, indicating a Th2 immune cellular response with minor chances of an inflammatory reaction, that is, the vaccine seems to be non-inflammatory and, therefore, a safe therapy for AD’s treatment [41] (Table 6).
CpG adjuvant is involved with promoters of DNA ribosomal methylation in human brains. This activity promotes an antigenic recognition and a target immune response to this antigen. Thus, Pietrzak et al. [87] evaluated the methylation capacity in human brains and verified that CpG promoted a co-methylation highly amplified, but not equally methylated. These modifications can determine the rDNA human transcription, being a regulatory influence for rDNA [87]. Piaceri et al. [88] evaluated 708 autopsied brains as to DNA methylation related to Alzheimer, and found an association between CpG and ANK1, CDH23, DIP2A, RHBDF2, RPL13, SERPINF1, and SERPINF2 genes, suggesting that DNA methylation might have an important role on the beginning of AD once researchers observed a genetic susceptibility for AD and pre-symptomatic individuals [88].
Antigen-presenting cell (APC) recognize strange DNA by its connection with Toll-like receptors (TLRs) and, as consequence, there is a release of cytokines that stimulate an acquired immune response [89]. CpG effects are influenced by antigen presence and DNA release [90, 91]. These genetic sequences may be present in recombinant plasmids in order to increase vaccine effectiveness [89]. There are controversies on CpG effectiveness as an adjuvant because it has not always shown improvements in immune response. However, there are results that demonstrate an increase in vaccine immunogenicity when used as plasmid adjuvant [92].
Macrophage-derived chemokine (MDC)
Movsessyan et al. [39] studied the effects of the DNA vaccine pMDC-3Aβ1-11-PADRE in 3xTg-AD (n = 23, three and four-month-old) mice. The vaccine was made by the insertion of Aβ1-11 gene in a mammalian expression vector (pCMVE-Aβ/MDC). The vaccine induced a Th2 immune cellular response that was able to stimulate high titers of antibodies capable of diminishing amyloid plaques and reduce glial cell activation without any behavioral deficits and increased adverse effects. The authors suggested that more studies must be conducted in bigger animals [39] (Table 6). Microglial cells and genetic associations have been strongly implicated in AD’s pathology, APOE, TREM2, CD33 and other polymorphisms genes modified the risks for AD and might be considered targets for therapeutic interventions. These are the first cells in an immune response, and from Fc receptor mediation, they facilitate antibodies functions. They are also involved in complement signaling proteins, antigen presentation, lysosomal degradation, ROS generation, therefore increasing AD’s progression [69, 70]. Thus, vaccines that inhibit glial cells activation are a good strategy to reduce or prevent AD.
Comparison between DNA and Protein Vaccines
Lambracht-Washington et al. [43] compared the trimer DNA vaccine Aβ42 with human peptide Aβ1 - 42 and Quil A adjuvant. For this, they used female B6SJLF1/J mice aging from four to eight months (Table 2). A yeast transcription factor gene (GAL4) was used as an activator plasmid and as a responding plasmid, an expression binder for GAL4 to UAS gene was utilized [43]. The animals were divided into two groups of eight animals each, one group received six DNA immunizations (4μg) with a gene gun and the other group was vaccinated six times with the peptide vaccine (100μg) [43] (Table 4). Despite the antibodies titers being lower in the animals who received the DNA vaccine, both groups showed a reduction on Aβ cerebral plaques, and those vaccinated with the Aβ42 DNA trimer presented IgG1 isotope antibodies. These isotopes demonstrate an activation of a Th2 immune response, which presents a lower probability of an inflammatory reaction. Therefore, its therapeutic potential must be considered, as this vaccine may be able to decrease AD’s progression [43] (Table 6).
Quil A is considered a Th1/Th2 adjuvant that can induce a strong systemic inflammatory reaction capable of dissipating through cytokine stimulation, this induced response by adjuvant cam increase an inflammatory reaction in the elderly [54]. A Phase II study in Japan used the ACC-001 vaccine with the QS-21 adjuvant (fraction of Quil A) and saw small and moderates adverse reactions due to the adjuvant’s inflammatory action in order to increase an anti-Aβ response [54]. Another clinical trial also using this adjuvant was the AN1792 in which the patients presented meningoencephalitis as result of Th1 cell activation [68].
It is known that there are quantitative and qualitative differences among DNA and protein vaccines immune reactions. On one hand, protein vaccine activated effector T cells, there is no stimulation for regulatory T cells, and on the other hand, DNA vaccines lead to an increase in regulatory T cell and IL-10 and a downregulation of effector T cell. This downregulation reduces, significantly, cytotoxic T cell migration to brain tissue, which was evident in AN1792 study [68].
DNA Vaccines followed by a Booster
Knowing that DNA vaccines are capable of generating moderate antibody titers, the use of boosters through a subsequent immunization protocol may be helpful to induce a more effective immune response [71]. In this revision, the boosters used were proteins, adenovirus, and antibodies.
Protein boost
Davtyan et al. [45], Lambracht-Washington et al. [46], and Shultz et al. [44] studied different vaccination protocols with a DNA and protein vaccine. One of the regimens analyzed was made with female C57BL/6 mice, ages of five and six weeks, that received a DNA vaccine (DepVac or pCMVE/MDC-3Aβ11-PADRE, developed with the Aβ11 gene cloned from a mammalian expression vector and inserted into the expression E. coli plasmid pET11d/MDC-myc/6xHis) followed by a protein boost (PepVac or MDC-3Aβ11-PADRE, in which the recombinant protein was purified from BL21(DE3) E. coli cells and transformed by the pET11d/MDC-3Aβ11-PADRE plasmid) [45] (Table 4). In another set of experiments, it was made a comparison between a protein boost (human peptide Aβ) after a DNA vaccine (Aβ42 trimer) and a DNA boost after a protein vaccine. For that, four to six months B6SJLF1/J mice were utilized [46] (Table 2). The results obtained showed that the DNA vaccine prior to a protein boost protocol was more effective in mice since it generated high IgG1 antibodies titles with high avidity [45] (Table 6). Lambracht-Washington et al. [46], on the contrary, observed that a DNA boost after a protein vaccine presents safer results, seeing that there was not T cell activation and inflammatory cytokine production (Table 6).
Shultz et al. [44] analyzed two immunization protocol, an active one using a DNA vaccine (pVR1012tPA-Aβ42) developed with the Aβ42 gene and an expression vector pVR1012-tPA, followed by a small dosage of a protein boost (Aβ42 peptide); and a passive one, in which the animals received the serum of previously vaccinated animals (Table 4). Animals actively immunized presented monoclonal IgG1 antibodies that were effective in the quick solubilization of amyloid plaques from passively immunized animals [44]. These results suggest that a Th2 immune response can be important in preventing an autoimmune response in AD patients and that monoclonal antibodies can be studied as a therapeutic vaccine in the early stages of the disease (Table 6) [44].
Passive immunization studies with anti-Aβ antibodies identified anti-Aβ antibodies presence and a great decrease in amyloid plaques on mice brains. The only adverse reactions observed were brain microhemorrhages in those who received higher doses of monoclonal antibodies [93, 94].
Adenovirus boost
Kim et al. [24] evaluated the combination of a DNA vaccine (pCA-PEDI-(Aβ1-6)11) followed by an adenovirus boost (AdPEDI-(Aβ1-6)11) in female C57BL/6J mice (n = 21, two months old) (Table 2). The vaccine and the boost were developed with 11 tandem repeats of the Aβ1-6 gene, a cDNA and a domain for the Pseudomonas exotoxin A receptor (PEDI) [24]. The animals presented high IgG1 antibodies titers with greater affinity for amyloid plaques, suggesting that a heterologous protocol may be effective in reducing Aβ burden in AD patients [24] (Table 6).
Adenoviruses have been quite explored as vaccine vectors in the prevention of infectious diseases and cancer treatment, considering that they induce a Th1 profile response [95]. However, when combined with Th2 cytokine, they are able to induce a Th2 immune response (81). Kim et al. [24] concluded that nasally vaccinated animals with the AdPEDI-(Aβ1-6)11 adenovirus had an increase in IL-10 production, demonstrating that the adjuvant, the antigen, and the vaccine’s delivery system contribute to cytokine production.
Antibodies boost
Lambracht-Washington and Rosenberg (2015) [48] evaluated the action of the TNFRSF antibody, with the goal of stimulating regulatory T cells (Tregs), in association with a DNA Aβ42 vaccine, in BALB//c-Foxp3-EGFP mice (Table 2). The vaccine was developed using a two-plasmid system, an activator which had the Gal4 transcription gene, and a responder which had three copies of the Aβ1 - 42 human gene [48]. Vaccinated animals presented a Th2 cellular immune response that produces IL-4, an important cytokine on the production of new Tregs (CD4+ CD25+ Foxp3+). These cells are associated with an increase of IL-10, a cytokine involved in the decrease of T cells, therefore, this vaccination protocol induced a non-inflammatory (Th2) response with high antibodies titers and, the antibody boost was able to amplify Tregs activities [48] (Table 6).
In humans, monoclonal antibodies and protein vaccines have already made into clinical trials. Solanezumab, a monoclonal antibody, is in Phase III studies and has presented beneficial effects on moderate levels of AD [96] yet, passive immunization presents a very high cost, suggesting that an active protocol would be more accessible for the population. Protein vaccines have been analyzed in Phase II studies [97, 98], but they have not reached the expected results and have presented adverse reactions. Another vaccine that got into a Phase I clinical trial, was conjugated with the tau protein and presented a safe and effective profile [99]. DNA vaccines, however, have limitations in clinical trials due to the low delivery rate of DNA to the host cells [100].
DNA vaccines have been highly investigated in the past two decades in small and medium-sized animal models (e.g., mice, rabbits, and monkeys) and some presented positive outcomes, however, when applied to humans, its low immunogenicity poses the biggest issue when compared to other strategies (protein vaccines). To the best of our knowledge, there are no clinical trials on DNA vaccines in humans. Although, protein vaccines with or without adjuvants have shown promising effects, but clinical trials are still in Phase I and II and have presented numerous biases and adverse effects. Novak et al. tested AADvac1 in a Phase I clinical trial, and observed a favorable safety profile and excellent immunogenicity in the first-in-man study, however, further studies are needed [101]. Pasquier et al. and Arai et al. tested vanutide cridificar (ACC-001) and the QS-21 adjuvant in a Phase II clinical trial, the results had acceptable safety profile in patients with mild to moderate AD and more investigations with anti-Aβ vaccines therapy are required [98,102]. Thus, the small number of clinical trials make room for research on protein and DNA vaccines for the discovery of new therapies for AD, and becoming a promising scientific and technological field.
In order to overcome the immunogenicity problem, strategies using conventional and molecular adjuvants and boosters have been tested. Even though many vaccines in this review did not use these strategies, their results were also promising. The most investigated vaccine was Aβ42 DNA, presenting favorable results in mice, rabbits, and monkeys with and without adjuvants, and followed by or prior to a protein boost. In addition, only one study did not target the Aβ gene, but the tau protein one that also presented great effectiveness. After analyzing the efficacy and safety of the vaccines presented, it is established that DNA vaccines for AD must induce a Th2 immune response (e.g., generate IgG1 isotope antibodies and anti-inflammatory cytokines) and not exacerbate Th1 and Th17 immune responses, respectively. Most studies did not present adverse reactions similar to the ones seeing in protein vaccines studies (e.g., hemorrhages and meningoencephalitis). However, a better investigation of this factor must be developed, since the studies presented here did not explore it deeply.
Taking into consideration that animal models’ studies do not guarantee a safe and effective translocation for clinical trials, they are still of major importance and must be performed. As presented in the current review, the results of DNA vaccines targeting the Aβ and the tau protein with or without adjuvants and boosters were promising to be tested in patients with AD.
CONFLICT OF INTEREST
The authors have no conflict of interest to report.
REFERENCES
[1] | Anand R , Gill KD , Mahdi AA ((2014) ) Therapeutics of Alzheimer’s disease: Past, present and future. Neuropharmacology 76: , 27–50. |
[2] | Hebert LE , Weuve J , Scherr PA , Evans DA ((2013) ) Alzheimer disease in the United States (2010-2050) estimated using the 2010 census. Neurology 80: , 1778–1783. |
[3] | Noguchi A , Matsumura S , Dezawa M , Tada M , Yanazawa M , Ito A , Akioka M , Kikuchi S , Sato M , Ideno S , Noda M , Fukunari A , Muramatsu S , Itokazu Y , Sato K , Takahashi H , Teplow DB , Nabeshima Y , Kakita A , Imahori K , Hoshi M ((2009) ) Isolation and characterization of patient-derived, toxic, high mass amyloid β-protein (Aβ) assembly from Alzheimer disease brains. J Biol Chem 284: , 32895–32905. |
[4] | Hardy J , Allsop D ((1991) ) Amyloid deposition as the central event in the aetiology of Alzheimer’s disease. Trends Pharmacol Sci 12: , 383–388. |
[5] | Kumar A , Dogra S ((2008) ) Neuropathology and therapeutic management of Alzheimer’s disease - An update. Drugs Future 33: , 433. |
[6] | Hardy J ((2009) ) The amyloid hypothesis for Alzheimer’s disease: A critical reappraisal. J Neurochem 110: , 1129–1134. |
[7] | Price DL , Sisodia SS ((1994) ) Cellular and molecular biology of Alzheimer’s disease and animal models. Annu Rev Med 45: , 435–446. |
[8] | Hansen RA , Gartlehner G , Webb AP , Morgan LC , Moore CG , Jonas DE ((2008) ) Efficacy and safety of donepezil, galantamine, and rivastigmine for the treatment of Alzheimer’s disease: A systematic review and meta-analysis. Clin Interv Aging 3: , 211–225. |
[9] | Ghochikyan A , Davtyan H , Petrushina I , Hovakimyan A , Movsesyan N , Davtyan A , Kiyatkin A , Cribbs DH , Agadjanyan MG ((2013) ) Refinement of a DNA based Alzheimer disease epitope vaccine in rabbits. Hum Vaccines Immunotharapeutics 9: , 1002–1010. |
[10] | Wu W , Lei P , Liu Q , Hu J , Gunn AP , Chen M , Rui Y , Su X , Xie Z , Zhao Y-F , Bush AI , Li Y ((2008) ) Sequestration of copper from β-amyloid promotes selective lysis by cyclen-hybrid cleavage agents. J Biol Chem 283: , 31657–31664. |
[11] | Orgogozo J-M , Gilman S , Dartigues J-F , Laurent B , Puel M , Kirby LC , Jouanny P , Dubois B , Eisner L , Flitman S , Michel BF , Boada M , Frank A , Hock C ((2003) ) Subacute meningoencephalitis in a subset of patients with AD after Abeta42 immunization. Neurology 61: , 46–54. |
[12] | Nicoll JAR , Wilkinson D , Holmes C , Steart P , Markham H , Weller RO ((2003) ) Neuropathology of human Alzheimer disease after immunization with amyloid-β peptide: A case report. Nat Med 9: , 448–452. |
[13] | Okura Y , Matsumoto Y ((2008) ) DNA vaccine therapy for Alzheimer’s disease: Present status and future direction. Rejuvenation Res 11: , 301–308. |
[14] | Petrushina I , Ghochikyan A , Mktrichyan M , Mamikonyan G , Movsesyan N , Davtyan H , Patel A , Head E , Cribbs DH , Agadjanyan MG ((2007) ) Alzheimer’s disease peptide epitope vaccine reduces insoluble but not soluble/oligomeric Abeta species in amyloid precursor protein transgenic mice. J Neurosci 27: , 12721–12731. |
[15] | Babiuk LA , Pontarollo R , Babiuk S , Loehr B , van Drunen Littel-van den Hurk S ((2003) ) Induction of immune responses by DNA vaccines in large animals. Vaccine 21: , 649–658. |
[16] | Li L , Saade F , Petrovsky N ((2012) ) The future of human DNA vaccines. J Biotechnol 162: , 171–182. |
[17] | Robinson HL , Pertmer TM ((2000) ) DNA Vaccines for viral infections: Basic studies and applications. Adv Virus Res 55: , 1–74. |
[18] | Braz L , Guimarães DT , Vaz MRF , Nóbrega FF , de F ((2014) ) Contribuições da biotecnologia nodesenvolvimento e de vacinas de primeira, segunda e terceira gerações. Rev Saúde Ciência 3: , 189–206. |
[19] | Agência Nacional de Vigilância Sanitária ((2011) ) Registro de produtos biológicos, 462. |
[20] | Ukelis U , Kramer P-J , Olejniczak K , Mueller SO ((2008) ) Replacementof in vivo acute oral toxicity studies by in vitro cytotoxicitymethods: Opportunities, limits and regulatory status. Regul Toxicol Pharmacol 51: , 108–118. |
[21] | Evans CF , Davtyanb H , Petrushinac I , Hovakimyanb A , Davtyanb A , Hannamana D , Cribbsc DH , Agadjanyanb MG , Ghochikyan A ((2014) ) Epitope-based DNA vaccine for Alzheimer’s disease: Translational study in macaques. Alzheimers Dement 10: , 284–295. |
[22] | Beller EM , Glasziou PP , Altman DG , Hopewell S , Bastian H , Chalmers I , Gøtzsche PC , Lasserson T , Tovey D , PRISMA for Abstracts Group ((2013) ) PRISMA for abstracts: Reporting systematic reviews in journal and conference abstracts. PLoS Med 10: , e1001419. |
[23] | Hara H , Mouri A , Yonemitsu Y , Nabeshima T , Tabira T ((2011) ) Mucosal immunotherapy in an Alzheimer mouse model by recombinant Sendai virus vector carrying Aβ1–43/IL-10 cDNA. Vaccine 29: , 7474–7482. |
[24] | Kim H , Jin J , Maxwell JA , Fukuchi K ((2007) ) Enhancing Th2 immune responses against amyloid protein by a DNA prime-adenovirus boost regimen for Alzheimer’s disease. Immunol Lett 112: , 30–38. |
[25] | Matsumoto Y , Niimi N , Kohyama K ((2013) ) Development of a new DNA vaccine for Alzheimer disease targeting a wide range of Aβ species and amyloidogenic peptides. PLoS One 8: , e75203. |
[26] | Mouri A , Noda Y , Hara H , Mizoguchi H , Tabira T , Nabeshima T ((2007) ) Oral vaccination with a viral vector containing Aβ cDNA attenuates age-related Aβ accumulation and memory deficits without causing inflammation in a mouse Alzheimer model. FASEB J 21: , 2135–2148. |
[27] | Okura Y , Miyakoshi A , Kohyama K , Park I , Staufenbiel M , Matsumoto Y ((2006) ) Nonviral Aβ DNA vaccine therapy against Alzheimer’s disease: Long-term effects and safety. Proc Natl Acad Sci U S A 103: , 9619–9624. |
[28] | Okura Y , Kohyama K , Park I ((2008) ) Nonviral DNA vaccination augments microglial phagocytosis of beta-amyloid deposits as a major clearance pathway in an Alzheimer disease mouse model. J Neuropathol Exp Neurol 67: , 1063–1071. |
[29] | Olkhanud PB , Mughal M , Ayukawa K , Malchinkhuu E , Okun E , Nagashima K , Mattson MP , Biragyn A ((2013) ) DNA immunization with HBsAg-based particles expressing a B cell epitope of amyloid β-peptide attenuates disease progression and prolongs survival in a mouse model of Alzheimer’s disease. Vaccine 30: , 1650–1658. |
[30] | Qu B , Rosenberg RN , Li L , Boyer PJ , Johnston SA ((2004) ) Gene vaccination to bias the immune response to amyloid-β peptide as therapy for Alzheimer disease. Arch Neurol 61: , 1859–1864. |
[31] | Qu B-X , Lambracht-Washington D , Fu M , Eagar TN , Stüve O , Rosenberg RN ((2010) ) Analysis of three plasmid systems for use in DNA Aβ42 immunization as therapy for Alzheimer’s disease. Vaccine 28: , 5280–5287. |
[32] | Qu B , Boyer PJ , Johnston SA , Hynan LS , Rosenberg RN ((2006) ) Abeta42 gene vaccination reduces brain amyloid plaque burden in transgenic mice. J Neurol Sci 244: , 151–158. |
[33] | Lambracht-Washington D , Fu M , Wight-Carter M , Riegel M , Rosenberg RN ((2017) ) Evaluation of a DNA Aβ42 vaccine in aged NZW rabbits: Antibody kinetics and immune profile after intradermal immunization with full-length DNA Aβ42 trimer. J Alzheimers Dis 57: , 97–112. |
[34] | Lambracht-Washington D , Fu M , Frost P , Rosenberg RN ((2017) ) Evaluation of a DNA Aβ42 vaccine in adult rhesus monkeys (Macaca mulatta): Antibody kinetics and immune profile after intradermal immunization with full-length DNA Aβ42 trimer. Alzheimers Res Ther 9: , 1–14. |
[35] | Davtyan H , Chen WW , Zagorski K , Davis J , Petrushina I , Kazarian K , Cribbs DH , Agadjanyan MG , Blurton-Jones M , Ghochikyan A ((2017) ) MultiTEP platform-based DNA epitope vaccine targeting N-terminus of tau induces strong immune responses and reduces tau pathology in THY-Tau22 mice. Vaccine 35: , 2015–2024. |
[36] | Davtyan H , Ghochikyan A , Hovakimyan A , Petrushina I , Agadjanyan MG ((2014) ) Immunostimulant patches containing Escherichia coli LT enhance immune responses to DNA- and recombinant protein- based Alzheimer’s disease vaccines. J Neuroimmunol 268: , 50–57. |
[37] | Kou J , Song M , Pattanayak A , Lim J-E , Yang J , Cao D , Li L , Fukuchi K ((2012) ) Combined treatment of Aβ immunization with statin in a mouse model of Alzheimer’s disease Jinghong. J Neuroimmunol 244: , 70–83. |
[38] | Movsesyana N , Mkrtichyana M , Petrushinab I , Rossc TM , Cribbsb DH , Agadjanyana MG , Ghochikyan A ((2008) ) DNA epitope vaccine containing complement component C3d enhances anti-amyloid-β antibody production and polarizes the immune response towards a Th2 phenotype. J Neuroimmunol 205: , 57–63. |
[39] | Movsesyan N , Ghochikyan A , Mkrtichyan M , Petrushina I , Davtyan H , Olkhanud PB , Head E , Biragyn A , Cribbs DH , Michael G ((2008) ) Reducing AD-like pathology in 3xTg-AD mouse model by DNA epitope vaccine — a novel immunotherapeutic strategy. PLoS One 3: , e2124. |
[40] | Movsesyan N , Davtyan H , Mkrtichyan M , Petrushina I , Tiraturyan T , Ross T , Agadjanyan MG , Ghochikyan A , Cribbs DH ((2010) ) Low concentrations of anti-Aβ antibodies generated in Tg2576 mice by DNA epitope vaccine fused. Hum Gene Tharapy 1576: , 1569–1576. |
[41] | Xing X , Zhang W , Sha S , Li Y , Guo R , Wang C , Cao Y ((2011) ) Amyloid β3-10 DNA vaccination suggests a potential new treatment for Alzheimer’s disease in BALB/c mice. Chin Med 124: , 2636–2641. |
[42] | Guo W , Sha S , Jiang T , Xing X , Cao Y ((2013) ) A new DNA vaccine fused with the C3d-p28 induces a Th2 immune response against amyloid-beta. Neural Regen Res 8: , 2581–2590. |
[43] | Lambracht-Washington D , Qu B-X , Fu M , Eagar TN , Stüve O , Rosenberg RN ((2009) ) DNA β-amyloid1–42 trimer immunization for Alzheimer disease in a wild-type mouse model. JAMA 302: , 1796–1802. |
[44] | Schultz JG , Salzer U , Mohajeri MH , Franke D , Heinrich J , Pavlovic J , Wollmer MA , Nitsch RM , Moelling K ((2004) ) Antibodies from a DNA peptide vaccination decrease the brain amyloid burden in a mouse model of Alzheimer s disease. J Mol Med 82: , 706–714. |
[45] | Davtyan H , Mkrtichyan M , Movsesyan N , Petrushina I , Mamikonyan G , Cribbs DH , Agadjanyan MG , Ghochikyan A ((2010) ) DNA prime–protein boost increased the titer, avidity and persistence of anti-Aβ antibodies in wild-type mice. Gene Ther 17: , 1–20. |
[46] | Lambracht-Washington D , B-X Q , Fu M , Anderson LL Jr , Eagar TN , Stüve O , Rosenberg RN ((2013) ) A peptide prime-DNA boost immunization protocol provides significant benefits as a new generation Aβ42 DNA vaccine for Alzheimer disease. J Neuroimmunol 254: , 63–68. |
[47] | Kima H-D , Taharaa K , Maxwella JA , Lalondeb R , Fukuiwac T , Fujihashic K , Van Kampend KR , Kongd F-K , Tangd DC , Fukuch K ((2008) ) Nasal inoculation of an adenovirus vector encoding 11 tandem repeats of Aβ1-6 upregulates IL-10 expression and reduces amyloid load in a Mo/Hu APPswe PS1dE9 mouse model of Alzheimer disease. J Gene Med 9: , 88–98. |
[48] | Lambracht-Washington D , Rosenberg RN ((2015) ) Co-stimulation with TNF receptor superfamily 4/25 antibodies enhances in-vivo expansion of CD4+CD25+Foxp3+T cells (Tregs) in a mouse study for active DNA Aβ42 immunotherapy. J Neuroimmunol 278: , 90–99. |
[49] | Porgador A , Irvine KR , Iwasaki A , Barber BH , Restifo NP , Germain RN ((1998) ) Predominant role for directly transfected dendritic cells in antigen presentation to CD8+T cells after gene gun immunization. J Exp Med 188: , 1075–1082. |
[50] | Best SR , Peng S , Juang C-M , Hung C-F , Hannaman D , Saunders JR , Wu T-C , Pai SI ((2009) ) Administration of HPV DNA vaccine via electroporation elicits the strongest CD8+T cell immune responses compared to intramuscular injection and intradermal gene gun delivery. Vaccine 27: , 5450–5459. |
[51] | Grønevik E , von Steyern FV , Kalhovde JM , Tjelle TE , Mathiesen I ((2005) ) Gene expression and immune response kinetics using electroporation-mediated DNA delivery to muscle. J Gene Med 7: , 218–227. |
[52] | Dupuis M , Denis-Mize K , Woo C , Goldbeck C , Selby MJ , Chen M , Otten GR , Ulmer JB , Donnelly JJ , Ott G , McDonald DM ((2000) ) Distribution of DNA vaccines determines their immunogenicity after intramuscular injection in mice. J Immunol 165: , 2850–2858. |
[53] | Liu Y-H , Bu X-L , Liang C-R , Wang Y-R , Zhang T , Jiao S-S , Zeng F , Yao X-Q , Zhou H-D , Deng J , Wang Y-J ((2015) ) An N-terminal antibodypromotes the transformation of amyloid fibrils into oligomers andenhances the neurotoxicity of amyloid-beta: The dust-raisingeffect. J Neuroinflammation 12: , 153. |
[54] | Marciani DJ ((2016) ) A retrospective analysis of Alzheimer’s disease vaccine progress - The critical need for new development strategies. J Neurochem 137: , 687–700. |
[55] | Davtyan H , Ghochikyan A , Petrushina I , Hovakimyan A , Davtyan A , Cribbs DH , Agadjanyan MG ((2006) ) MultiTEP platform-based AD epitope vaccine activates broad repertoire of T helper cells in non-human primates Hayk. Alzheimers Dement 244: , 151–158. |
[56] | Gordon S , Martinez FO ((2010) ) Alternative activation of macrophages: Mechanism and functions. Immunity 32: , 593–604. |
[57] | Kiyota T , Okuyama S , Swan RJ , Jacobsen MT , Gendelman HE , Ikezu T ((2010) ) CNS expression of anti-inflammatory cytokine interleukin-4 attenuates Alzheimer’s disease-like pathogenesis in APP+PS1 bigenic mice. FASEB J 24: , 3093–3102. |
[58] | Latta CH , Sudduth TL , Weekman EM , Brothers HM , Abner EL , Popa GJ , Mendenhall MD , Gonzalez-oregon F , Braun K , Wilcock DM ((2015) ) Determining the role of IL-4 induced neuroinflammation inmicroglial activity and amyloid- β using BV2 microglialcells and APP / PS1 transgenic mice. J Neuroinflammation 12: , 1–13. |
[59] | Frazer ME , Hughes JE , Mastrangelo MA , Tibbens JL , Federoff HJ , Bowers WJ ((2008) ) Reduced pathology and improved behavioral performance in Alzheimer’s disease mice vaccinated with HSV amplicons expressing amyloid-β and interleukin-4. Mol Ther 16: , 845–853. |
[60] | Li W , Qian X , Teng H , Ding Y , Zhang L ((2014) ) Association of interleukin-4 genetic polymorphisms with sporadic Alzheimer’s disease in Chinese Han population. Neurosci Lett 563: , 17–21. |
[61] | Soosanabadi M , Bayat H , Kamali K , Saliminejad K , Banan M , Khorram Khorshid H ((2015) ) Association study of IL-4 -590C/T and DDX39B -22G/C polymorphisms with the risk of late-onset Alzheimer’s disease in Iranian population. Curr Aging Sci 8: , 276–281. |
[62] | Sedegah M , Jones TR , Kaur M , Hedstrom R , Hobart P , Tine JA , Hoffman SL ((1998) ) Boosting with recombinant vaccinia increases immunogenicity and protective efficacy of malaria DNA vaccine. Proc Natl Acad Sci U S A 95: , 7648–7653. |
[63] | Glenting J , Wessels S ((2005) ) Ensuring safety of DNA vaccines. Microb Cell Fact 4: , 26. |
[64] | Slobod KS , Shenep JL , Luján-Zilbermann J , Allison K , Brown B , Scroggs RA , Portner A , Coleclough C , Hurwitz JL ((2004) ) Safety and immunogenicity of intranasal murine parainfluenza virus type 1 (Sendai virus) in healthy human adults. Vaccine 22: , 3182–3186. |
[65] | Kishino Y , Seki T , Yuasa S , Fujita J , Fukuda K ((2015) ) Generation of induced pluripotent stem cells from human peripheral T cells using sendai virus in feeder-free conditions. J Vis Exp 105: , 1–5. |
[66] | Saga K , Kaneda Y ((2015) ) Oncolytic Sendai virus-based virotherapy for cancer: Recent advances. Oncolytic Virotherapy 2015: , 141–147. |
[67] | Hara H , Ono F , Nakamura S , Matsumoto S , Jin H ((2016) ) An oral Aβ vaccine using a recombinant adeno-associated virus vector in aged monkeys: Reduction in plaque amyloid and increase in Aβ oligomers. J Alzheimers Dis 54: , 1047–1059. |
[68] | Cribbs DH ((2011) ) Abeta DNA vaccination for Alzheimer’s disease: Focus on disease prevention. CNS Neurol Disord Drug Targets 9: , 207–246. |
[69] | Wes PD , Sayed FA , Bard F , Gan L ((2016) ) Targeting microglia for the treatment of Alzheimer’s disease. Glia 64: , 1710–1732. |
[70] | Holtman IR , Raj DD , Miller JA , Schaafsma W , Yin Z , Brouwer N , Wes PD , Möller T , Orre M , Kamphuis W , Hol EM , Boddeke EWGM , Eggen BJL ((2015) ) Induction of a common microglia gene expression signature by aging and neurodegenerative conditions: A co-expression meta-analysis. Acta Neuropathol Commun 3: , 31. |
[71] | Rosenberg RN , Lambracht-Washington D , Yu G , Xia W ((2016) ) Genomics of Alzheimer disease: A review. JAMA Neurol 73: , 867–874. |
[72] | Aspinall R ((2000) ) Longevity and the immune response. Biogerontology 1: , 273–278. |
[73] | Fuente MD , La Baeza I , Guayerbas N , Puerto M , Castillo C , Salazar V , Ariznavarreta C , F-tresguerres JA ((2004) ) Changes with ageing in several leukocyte functions of male and female rats. Biogerontology 5: , 389–400. |
[74] | Giménez-Llort L , Arranz L , Maté I , De La Fuente M ((2008) ) Gender-specific neuroimmunoendocrine aging in atriple-transgenic 3×Tg-AD mouse model for Alzheimer’s disease and its relation with longevity. Neuroimmunomodulation 15: , 331–343. |
[75] | Khan IA , Aziz A , Bashir S , Raza MA , Fatima G ((2016) ) Dermatological evaluation of counter irritant effect of methanol leaf extract of Rumexvesicarius Linn. in rabbits. J Pak Med Assoc 66: , 49–52. |
[76] | Chandra SA , Peterson RA , Melich D , Merrill CM , Bailey D , Mellon-Kusibab K , Adler R ((2014) ) Dermal irritation of petrolatum in rabbits but not in mice, rats or minipigs. J Appl Toxicol 34: , 857–861. |
[77] | Ward SM , Himmelstein DS , Lancia JK , Binder LI ((2012) ) Tau oligomers and tau toxicity in neurodegenerative disease. Biochem Soc Trans 40: , 667–671. |
[78] | Ballatore C , Lee VM-Y , Trojanowski JQ ((2007) ) Tau-mediated neurodegeneration in Alzheimer’s disease and related disorders. Nat Rev Neurosci 8: , 663–672. |
[79] | LaPointe NE , Morfini G , Pigino G , Gaisina IN , Kozikowski AP , Binder LI , Brady ST ((2009) ) The amino terminus of tau inhibits kinesin-dependent axonal transport: Implications for filament toxicity. J Neurosci Res 87: , 440–451. |
[80] | Kanaan NM , Morfini GA , LaPointe NE , Pigino GF , Patterson KR , Song Y , Andreadis A , Fu Y , Brady ST , Binder LI ((2011) ) Pathogenic forms of tau inhibit kinesin-dependent axonal transport through a mechanism involving activation of al phosphotransferases. J Neurosci 31: , 9858–9868. |
[81] | Marciani DJ ((2014) ) New Th2 adjuvants for preventive and active immunotherapy of neurodegenerative proteinopathies. Drug Discov Today 19: , 912–920. |
[82] | Marciani DJ ((2015) ) Is fucose the answer to the immunomodulatory paradox of Quillaja saponins? Int Immunopharmacol 29: , 908–913. |
[83] | Schutze MP , Leclerc C , Jolivet M , Audibert F , Chedid L ((1985) ) Carrier-induced epitopic suppression, a major issue for future synthetic vaccines. J Immunol 135: , 2319–2322. |
[84] | Sad S , Gupta HM , Talwar GP , Raghupathy R ((1991) ) Carrier-induced suppression of the antibody response to a “self” hapten. Immunology 74: , 223–227. |
[85] | Gherardi RK , Eidi H , Crépeaux G , Authier F , Cadusseau J ((2015) ) Biopersistence and brain translocation ofaluminum adjuvants of vaccines. Front Neurol 6: , 4. |
[86] | Toapanta FR , Ross TM ((2006) ) Complement-mediated activation of the adaptive immune responses: Role of C3d in linking the innate and adaptive immunity. Immunol Res 36: , 197–210. |
[87] | Pietrzak M , Rempala GA , Nelson PT , Hetman M ((2016) ) Non-random distribution of methyl-CpG sites and non-CpG methylation in the human rDNA promoter identified by next generation bisulfite sequencing. Gene 585: , 35–43. |
[88] | Piaceri I , Raspanti B , Tedde A , Bagnoli S , Sorbi S , Nacmias B ((2015) ) Epigenetic modifications in Alzheimer’s disease: Cause or effect? J Alzheimers Dis 43: , 1169–1173. |
[89] | Singh M , O’Hagan DT ((2002) ) Recent advances in vaccine adjuvants. Pharm Res 19: , 715–728. |
[90] | Singh M , Briones M , Ott G , O’Hagan D ((2000) ) Cationic microparticles: A potent delivery system for DNA vaccines. Proc Natl Acad Sci U S A 97: , 811–816. |
[91] | Coban C , Ishii KJ , Gursel M , Klinman DM , Kumar N ((2005) ) Effect of plasmid backbone modification by different human CpG motifs on the immunogenicity of DNA vaccine vectors. J Leukoc Biol 78: , 647–655. |
[92] | Ulmer JB , Wahren B , Liu MA ((2006) ) Gene-based vaccines: Recent technical and clinical advances. Trends Mol Med 12: , 216–222. |
[93] | Janus C , Pearson J , McLaurin J , Mathews PM , Jiang Y , Schmidt SD , Chishti MA , Horne P , Heslin D , French J , Mount HTJ , Nixon RA , Mercken M , Bergeron C , Fraser PE , St George-Hyslop P , Westaway D ((2000) ) A beta peptide immunization reduces behavioural impairment and plaques in a model of Alzheimer’s disease. Nature 408: , 979–982. |
[94] | Pfeifer M , Boncristiano S , Bondolfi L , Stalder A , Deller T , Staufenbiel M , Mathews PM , Jucker M ((2002) ) Cerebral hemorrhage after passive anti-Abeta immunotherapy. Science 298: , 1379–1379. |
[95] | Bruna-Romero O , Rocha CD , Tsuji M , Gazzinelli RT ((2004) ) Enhancedprotective immunity against malaria byvaccination with a recombinant adenovirus encoding thecircumsporozoite protein of Plasmodium lacking the GPI-anchoringmotif. Vaccine 22: , 3575–3584. |
[96] | Tabira T ((2016) ) [Immunotherapy for Alzheimer’s disease targeting Aβ]. Nihon Rinsho 74: , 423–426. |
[97] | Mandler M , Santic R , Gruber P , Cinar Y , Pichler D ((2015) ) Tailoring the antibody response to aggregated Aβ using novel Alzheimer-vaccines. PLoS One 10: , 1–22. |
[98] | Arai H , Suzuki H , Yoshiyama T ((2015) ) Vanutide cridificar and the QS-21 adjuvant in Japanese subjects with mild to moderate Alzheimer’s disease: Results from two phase 2 studies. Curr Alzheimer Res 12: , 242–254. |
[99] | Kontsekova E , Zilka N , Kovacech B , Novak P , Novak M ((2014) ) First-in-man tau vaccine targeting structural determinants essential for pathological tau–tau interaction reduces tau oligomerisation and neurofibrillary degeneration in an Alzheimer’s disease model. Alzheimers Res Ther 6: , 44. |
[100] | Flingai S , Czerwonko M , Goodman J , Kudchodkar SB , Muthumani K , Weiner DB ((2013) ) Synthetic DNA vaccines: Improved vaccine potency by electroporation and co-delivered genetic adjuvants. Front Immunol 4: , 354. |
[101] | Novak P , Schmidt R , Kontsekova E , Zilka N , Kovacech B , Skrabana R , Vince-Kazmerova Z , Katina S , Fialova L , Prcina M , Parrak V , Dal-Bianco P , Brunner M , Staffen W , Rainer M , Ondrus M , Ropele S , Smisek M , Sivak R , Winblad B , Novak M ((2017) ) Safety and immunogenicity of the tau vaccine AADvac1 in patients with Alzheimer’s disease: A randomised, double-blind, placebo-controlled, phase 1 trial. Lancet Neurol 16: , 123–134. |
[102] | Pasquier F , Sadowsky C , Holstein A , Leterme GLP , Peng Y , Jackson N , Fox NC , Ketter N , Liu E , Ryan JM , ACC-001 (QS-21) Study Team ((2016) ) Two Phase 2 multiple ascending–dose studies of vanutide cridificar (ACC-001) and QS-21 adjuvant in mild-to-moderate Alzheimer’s disease. J Alzheimers Dis 51: , 1131–1143. |