RANK in cancer-associated fibroblasts: A valuable prognostic determinant for metastasis in early-stage breast cancer patients
Abstract
BACKGROUND:
The molecular system of receptor activator of nuclear factor kappa-
METHODS AND RESULTS:
We explored the significance of RANK expression in cancer-associated fibroblasts (CAFs) as a prognostic biomarker in early breast cancer patients (BCPs) by immunohistochemistry. Results reveal a significant correlation between high RANK expression in CAFs and an increased risk of metastasis (
CONCLUSIONS:
These results support the clinical relevance of RANK in CAFs and propose its potential as a future therapeutic target in the treatment of early BCPs.
1.Introduction
Breast cancer is a complex system composed of tumor cells and their stromal microenvironment, where CAFs play a significant role [1, 2]. These activated fibroblasts are a heterogeneous population of spindle-shaped stromal cells that do not express CD34 and CD31 but could exhibit positivity for smooth muscle actin
RANK functional activities have been clearly established by studying the phenotype of RANK knockout mice, which exhibit severe osteopetrosis characterized by a lack of mature osteoclasts and an absence of lymph node development, leading to impairment in B- and T-cell maturation [29, 30]. RANK is thus recognized as the second key player in “osteoimmunology” [31]. Furthermore, studies have demonstrated that RANK is upregulated in osteosarcoma, a mesenchymal tumor of osteoblastic origin. Additionally, this receptor is present in normal osteoblasts and plays a crucial role in controlling their migration inside the bone, which is essential for bone modeling and remodeling [32, 33, 34]. Despite advancements in understanding the RANKL-RANK system, there is still a lack of knowledge and contradictions regarding its functions in CAFs and MSCs within the primary breast tumor microenvironment. Given the background of this research field and acknowledging the limitations of classic prognostic parameters in accurately predicting outcomes in early-stage breast cancer, it becomes imperative to evaluate the potential of RANK as a prognostic biomarker. Additionally, investigate the role of RANK in the self-renewal, proliferation, and migration processes of CAFs is of vital importance.
2.Materials and methods
2.1Retrospective analysis
2.1.1Selection and characterization of breast cancer patients
A retrospective study was conducted, including 155 consecutive patients who underwent surgical treatment for breast cancer at the Hospital Italiano in Buenos Aires, Argentina. These patients had a confirmed histological diagnosis of early-stage invasive ductal carcinoma (stage I/II) based on the TNM classification system of the International Union Against Cancer [35]. A minimum follow-up period of 10 years after surgery was ensured. Patients who received neoadjuvant therapies, those with insufficient tissue samples, and those with previous primary tumors were excluded. After surgery, all patients received appropriate treatment, including hormonal therapy, radiotherapy, and/or chemotherapy. The treatment plan was determined based on the clinical and histopathological characteristics of each patient and the guidelines recommended by the European Society for Medical Oncology [36, 37]. This study was approved by the Ethics Committees of the Institute of Biology and Experimental Medicine (IBYME) and the Hospital Italiano. Informed consent was obtained from the patients or their family members (IBYME approval: CE 050 and Hospital Italiano approval: No. 5009). The research was conducted in accordance with the principles set out in the Declaration of Helsinki. To protect the privacy of the patients, medical records were anonymized using a numerical code.
The clinical characteristics of the patients, considered classical prognostic markers, were categorized according to the cutoff values specified in the Hospital Italiano protocols [38]. These characteristics included: a) Age (
Figure 1.
Expression of RANK in CAFs from the primary tumor of BCPs. Left panel: representative example of RANK immunostaining (brown chromogen) in stromal cells assessed in the primary tumor tissue of a BCP. Right panel: isotype control. Nuclei were stained with hematoxylin (purple color). Original magnification:
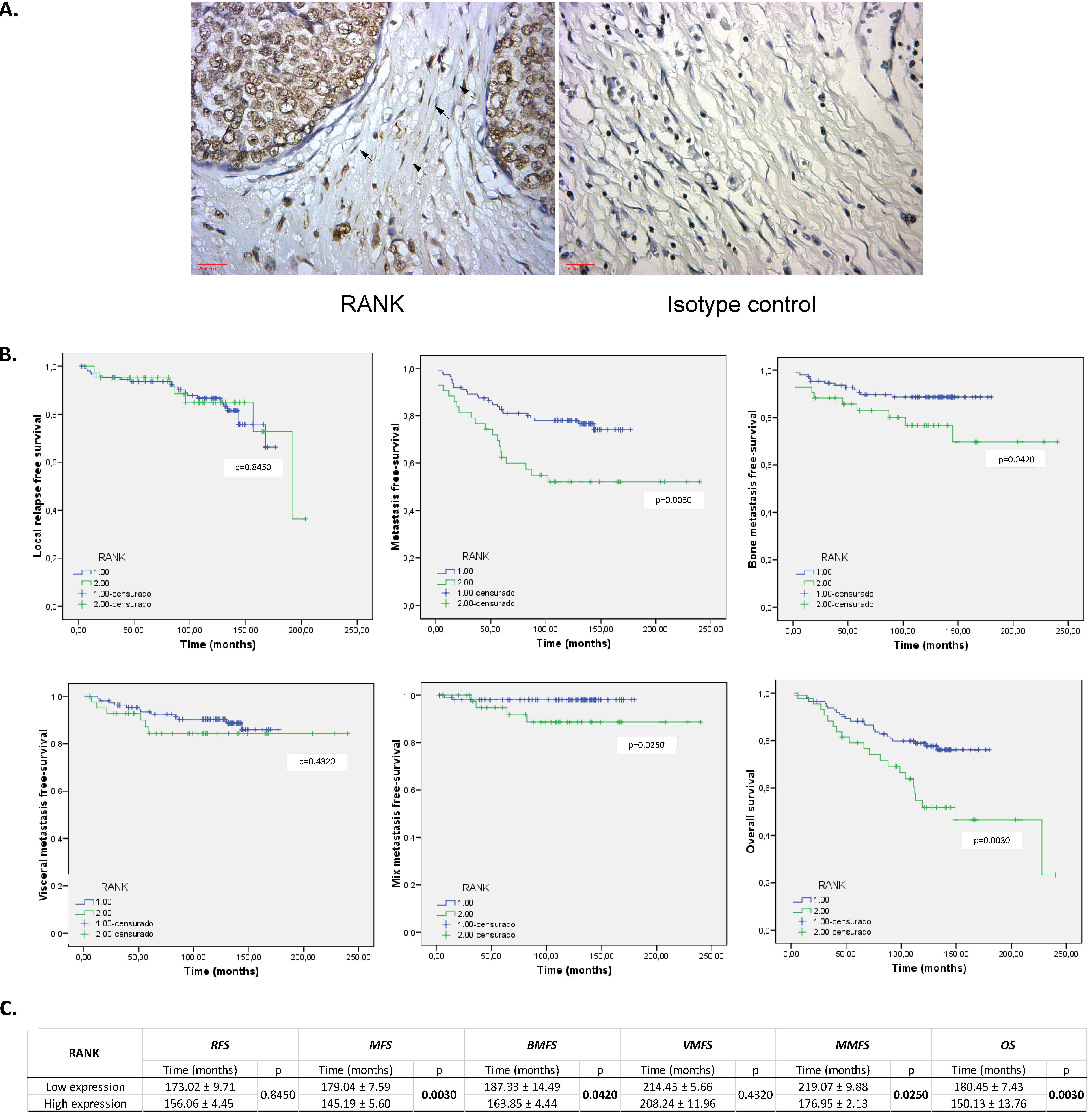
2.1.2Analysis of RANK expression
Breast tissue samples were processed following the methodology outlined by Labovsky et al. [10]. In order to assess the levels of RANK expression in spindle-shaped stromal cells not associated with vasculature, we employed an immunohistochemical protocol as described in previous studies [10]. The immunohistochemical signal was evaluated using the Allred scoring system [41]. Cells with membranous staining, nuclear counterstaining, and displaying characteristic fibroblastic morphology (spindle shape), not associated with the vasculature, were counted within the intratumoral stroma. Positive cell percentages were assigned scores according to the following categories: 0 (
2.1.3Intra-tumoral stromal characteristics
The histological characteristics of the tumor stroma, such as the percentage (%) of intratumoral stroma, the quantity of fibroblasts, collagen deposition, lymphocytic infiltration, myxoid changes, and blood and lymphatic vascularization, were assessed through hematoxylin and eosin staining. Intratumoral stroma was assessed as a percentage and categorized as low (
2.1.4Statistical analysis
The statistical analysis of RANK expression and its correlation with clinical-pathological characteristics was conducted following the methodology described by Labovsky et al. [10]. To determine the optimal cutoff value for receptor expression, we used the values of the first quartile (Q1), median, and third quartile (Q3) for sample classification. Associations between categorized RANK expression and patient OS were evaluated through univariable analysis. The cutoff value that provided the lowest p-value was selected. The optimal cutoff values for receptor expression were as follows: RANK
2.2Prospective analysis
2.2.1Patient selection
A prospective study was conducted using tumor-associated fibroblasts obtained from tumor tissue during surgery from 9 patients with early invasive ductal breast carcinoma (stages I/II, luminal type). Patients who had received neoadjuvant therapy, had a history of previous tumors, or had insufficient sample size (
2.2.2Isolation and expansion of CAFs from primary beast cancer tissue
Immediately after mastectomy, the tissues were placed in DMEM-F12 (#12500-062, Gibco). They were subsequently washed with the same medium supplemented with an antibiotic-antimycotic solution (ATB/ATM) (Gibco, Cat.15240), which contained a final concentration of 100 IU/ml of penicillin, 100
2.2.3Phenotypic characterization of CD34(-) fibroblast populations
A total of 3
2.2.4Evaluation of RANK and CD105 co-expression in CAFs isolated from fresh breast tissue and paraffin samples by immunofluorescence
CAFs isolated from breast tumor tissue were seeded onto slides at a density of 350,000 cells/ml (from 4th subculture). They were then fixed with methanol for 15 minutes and subsequently hydrated with 0.1% TBS-TW20 for 10 minutes. To perform double-label immunofluorescence, firstly, they were incubated over-night with the primary anti-RANK Ab (#MAB683, RyD Systems). On the second day, extensive washes were carried out with 1X PBS, followed by incubation with a secondary anti-mouse Ab labeled with Alexa 488 (715545150, Jackson-immunoresearch). Then, overnight incubation was performed with the primary anti-CD105 Ab (#AF1097, RyD Systems). On the third day, two washes with 1X PBS with 0.1% Tween 20 were done, followed by incubation with a secondary anti-goat Ab labeled with Alexa-647 (705605147, Jackson Immunoresearch). Subsequently, counterstaining was done using DAPI, followed by a wash with distilled water, and finally, the samples were mounted using Vectashield. CAFs expressing RANK (+) and CD105 (+) were visualized using a confocal microscope (40X). To perform immunofluorescence on breast tumor tissue embedded in paraffin, the same procedure was followed, with the difference that the initial processing of the sample was carried out as previously mentioned in the retrospective study [10]. Each sample was performed in duplicate.
2.2.5Quantitative RT-PCR
CD34(-) stromal cells (CAF-like) for the 4th subculture was again seeded at 3,000 cells/cm2 to obtain enough cells density for the RNA isolation. The culture conditions were as follow: i) 10 ml of supplemented
2.2.6Fibroblastic colony-forming unit (CFU-F) assay
Isolated CD34(-) stromal cells (CAFs-like) were plated at a density of 100 cells of 4th subculture/cm2 in 25 cm2 culture flasks containing: i) 10 ml of supplemented
2.2.7Proliferation assay
Viable CD34(-) fibroblasts were seeded at a density of 5
Table 1
Association of RANK expression in spindle shape stromal cells, not associated to the vasculature, with clinical-pathological characteristics (classic prognostic markers), local relapse, metastasis occurrence, bone metastasis occurrence, visceral metastasis occurrence, and mix metastasis occurrence in 155 patients with early invasive ductal breast cancer. Fisher’s exact test was used for the association between variables
Clinicopathological characteristics | RANK | ||||
| High expression |
| |||
n | % | ||||
Age (years) | 42 | 12 | 28.57% | 1 | |
| 113 | 31 | 27.43% | ||
Tumor size (cm) |
| 111 | 27 | 24.32% | 0.1640 |
44 | 16 | 36.36% | |||
ER | Negative | 19 | 4 | 21.05% | 0.5930 |
Positive | 136 | 39 | 28.68% | ||
PR | Negative | 31 | 7 | 22.58% | 0.6540 |
Positive | 124 | 36 | 29.03% | ||
Her2/neu | Negative | 137 | 40 | 29.20% | 0.1570 |
Positive | 18 | 2 | 11.11% | ||
Histological grade | G1 | 12 | 4 | 33.33% | 0.6700 |
G2 | 89 | 26 | 29.21% | ||
G3 | 54 | 13 | 24.07% | ||
Regional lymph nodes | Negative | 106 | 30 | 28.30% | 0.8500 |
Positive | 49 | 13 | 26.53% | ||
Local relapse | Negative | 129 | 36 | 27.91% | 1 |
Positive | 26 | 7 | 26.92% | ||
Metastatic occurrence | Negative | 109 | 23 | 21.10% | 0.0060 |
Positive | 46 | 20 | 43.48% | ||
Bone metastatic occurrence | Negative | 133 | 33 | 24.81% | 0.0690 |
Positive | 22 | 10 | 45.45% | ||
Visceral metastatic occurrence | Negative | 137 | 37 | 27.01% | 0.5820 |
Positive | 18 | 6 | 33.33% | ||
Mix-metastatic occurrence | Negative | 149 | 39 | 26.17% | 0.0500 |
Positive | 6 | 4 | 66.67% |
*p-value
2.2.8Migration assay
Experiments were conducted using transwell membranes with an 8
2.2.9Statistical analysis
Results were presented as the mean
3.Results
3.1Retrospective study
3.1.1Expression of RANK in spindle-shaped stromal cells, not associated with vasculature, and its association with the clinical-pathological characteristics of breast cancer patients
Out of a total of 155 BCPs diagnosed with invasive ductal breast cancer (stage I/II), 43 (27.75%) samples were found to have high RANK expression while 112 (72.25%) samples were found to have low RANK expression. Within the high expression group of RANK, the average percentage of fibroblasts expressing RANK was 46.90
Table 2
Association of RANK expression in spindle shape stromal cells, not associated to the vasculature, with the number (#) of metastatic foci per organ in general. Fisher’s exact test was used for the association between variables
Characteristics of the metastatic focus |
| # metastatic foci | |
---|---|---|---|
| |||
RANK | |||
Low expression | 112 | 14 | 0.0050 |
High expression | 43 | 15 |
*p-value
There were no significant differences found regarding the association between RANK expression in spindle-shaped stromal cells, not associated with vasculature, “fibroblasts-like”, and clinical parameters such as ER, PR, Her2/neu status, tumor size, age, histological grade, and regional lymph node status (Table 1). However, it was found that RANK expression in these stromal cells was related to the occurrence of metastasis in BCPs at early stages (I/II). High RANK expression was significantly associated with a higher risk of developing metastasis (
3.1.2Expression of RANK in spindle-shaped stromal cells, not associated with vasculature, and its association with intratumoral stromal characteristics
The expression of RANK was associated with the percentage of intratumoral stroma, as well as with blood and lymphatic vascularization (
3.1.3Association between classical prognostic markers and tumor progression
The ER status, PR status, tumor size, and histological grade were significantly associated with a worse prognosis in BCPs. Patients with ER (-) had lower MFS, BMFS, VMFS, and OS (
Table 3
Association between stromal histological characteristics and RANK in spindle shape stromal cells, not associated to the vasculature, expression in a cohort of 155 early breast cancer patients. Fisher’s exact test was used for the association between variables
Characteristics of the breast tumor stroma | RANK | |||
---|---|---|---|---|
Total | High expression |
| ||
% Intratumoral Stroma |
| 88 | 18 | 0.0290 |
67 | 25 | |||
% Fibroblast | non-large amount | 140 | 38 | 0.5620 |
Large amount | 15 | 5 | ||
Collagen deposition | non-large amount | 70 | 16 | 0.2800 |
Large amount | 85 | 27 | ||
Lymphatic infiltration | non-large amount | 135 | 35 | 0.1920 |
Large amount | 20 | 8 | ||
Desmoplasia | non-large amount | 60 | 10 | 0.0850 |
Large amount | 95 | 27 | ||
Mixoid changes | non-large amount | 59 | 13 | 0.3520 |
Large amount | 96 | 30 | ||
Blood vascularization | non-large amount | 109 | 1 | 0.0130 |
Large amount | 46 | 18 | ||
Lymphatic vascularization | non-large amount | 111 | 1 | 0.0006 |
Large amount | 44 | 18 | ||
Total | 155 | 43 |
*p-value
Figure 2.
Forest plot showed odds ratios for the multivariate association between classical prognosis factors and RANK, and metastasis-free survival (A), bone metastasis-free survival (B), visceral metastasis-free survival (C), and overall survival (D) in early invasive ductal BCPs.
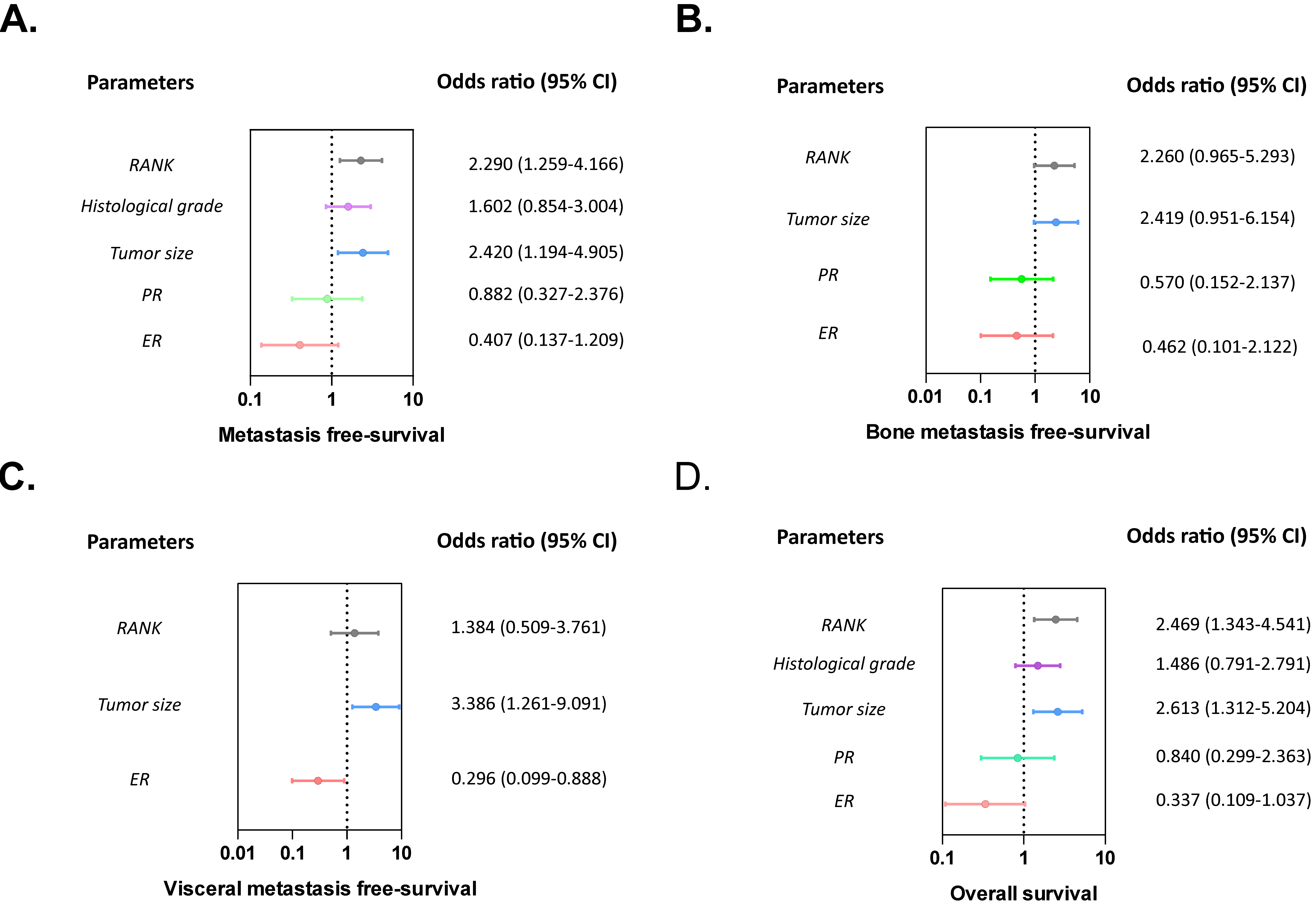
3.1.4Multivariate analysis
RANK expression was an independent prognostic factor for MFS and OS in our BCPs (
3.2Prospective study
3.2.1Phenotypic characterization of CD34(-) spindle-shaped stromal cells isolated from BCPs
Figure 3.
Phenotypic characterization of CD34(-) CAFs. A. Representative flow cytometry surface antigen histograms of CAFs from a representative BCP. Isotype control (). B. Co-expression of RANK and CD105 in CAFs of breast tumors. A representative dot plot of RANK-CD105 co-expression, CD34-CD105, and CD34-RANK in fibroblasts isolated from the primary tumors of BCPs (I/II). C. Dual staining of RANK (green) and CD105 (red) by immunofluorescence in CD34(-) fibroblasts isolated from the primary tumors of BCPs. Counterstained with DAPI. Magnification 400X. The scale corresponds to 50
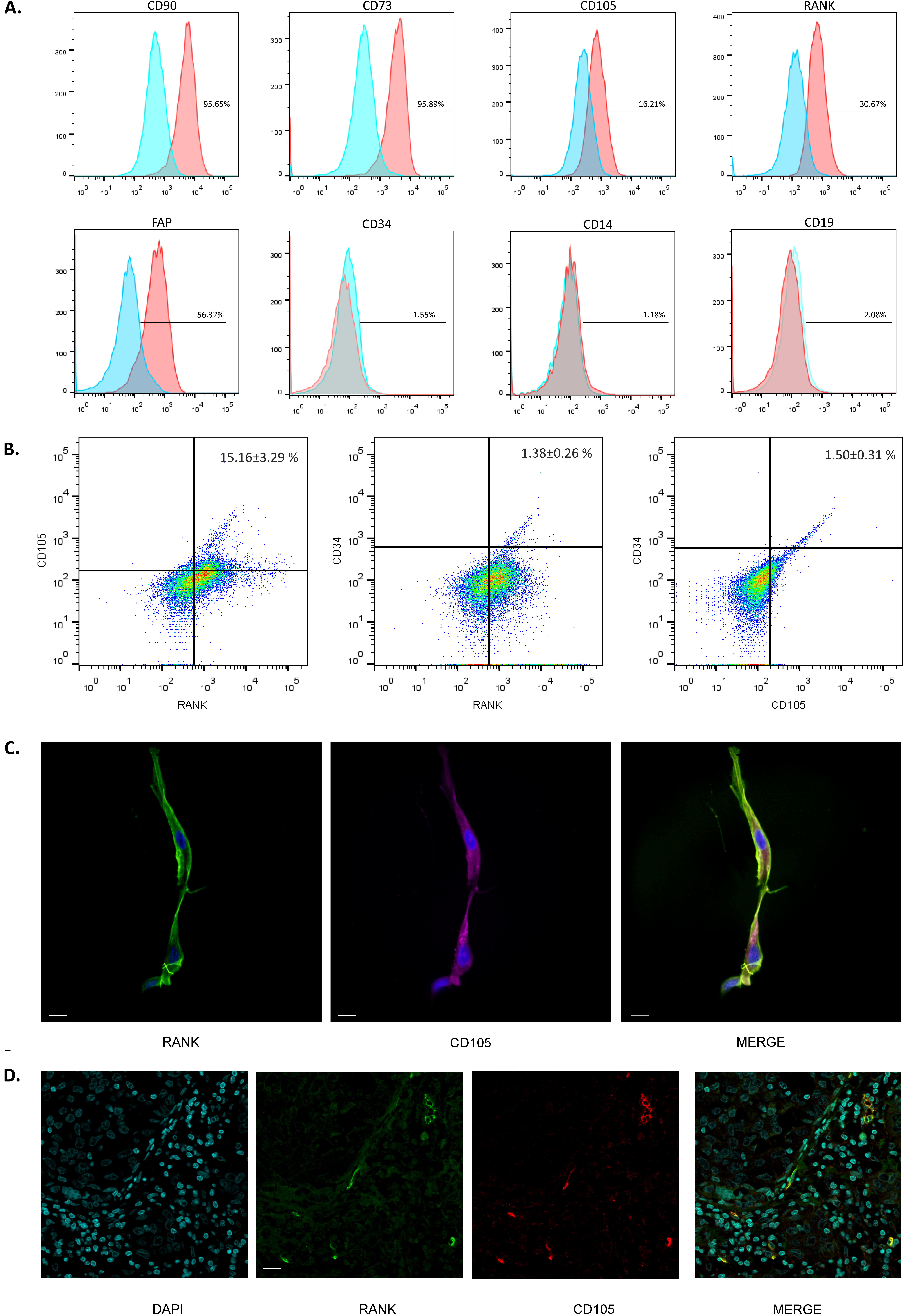
Phenotypic analysis conducted through flow cytometry revealed that the studied CD34(-) spindle-shaped stromal cells exhibited the expression of markers associated with both CAFs and MSCs. We observed that our cell population had an expression of 97.19
Figure 4.
Effect of RANKL-RANK system on the self-renewal, proliferation abilities, expression of pluripotency factors and migration of CD34(-) CAFs in BCPs. A. Gene expression of self–renewal and pluripotency factors in CAFs from BCPs treatment with and without RANKL. Expression of OCT-4, SOX-2 and DKK-1 by quantitative real-time polymerase chain reaction (RT-PCR). All the results were normalized against a set of reference genes. B. CFU-F Assay: The self-renewal capacity of CD34(-) CAFs from BCPs was assessed in
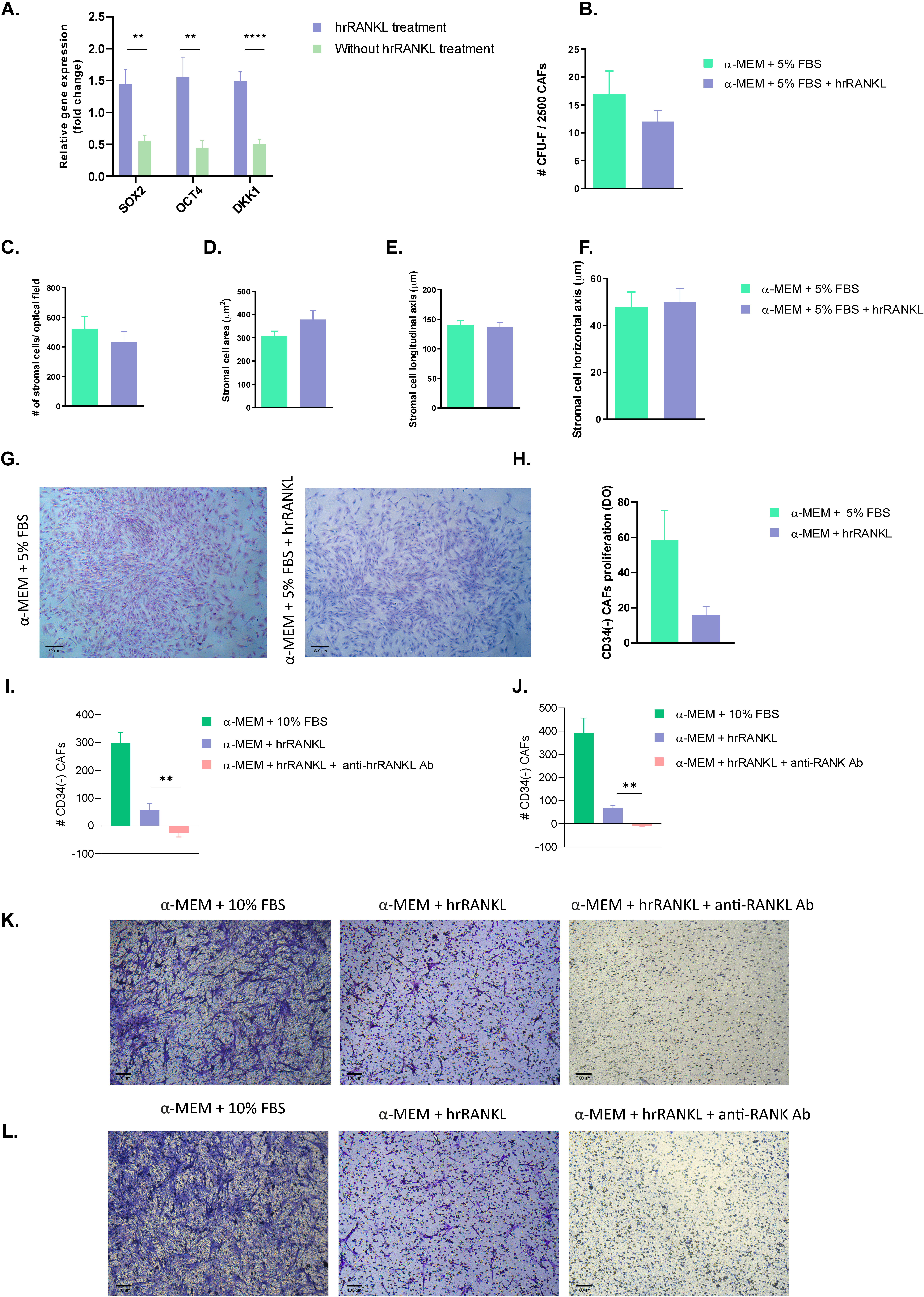
3.2.2Gene expression of self-renewal and multipotency
We observed that CD34 (-) fibroblasts treated with 25 ng/ml hrRANKL had increased expression of genes related to cell multipotentiality as well as self-renewal, such as OCT4, SOX-2 and DKK-1, compared to CD34(-) fibroblasts that did not receive treatment (Fig. 4, panel A).
3.2.3Capacity to generate fibroblastic colony-forming units
We did not find differences in the capacity to form CFU-F between CD34(-) spindle-shaped stromal cells treated with
3.2.4Proliferation capacity
Regarding the proliferation analysis performed using the MTS assay, we observed an increase in CD34(-)spindle-shaped stromal cells proliferation when we cultured these stromal cells with 25ng/ml hrRANKL alone. However, the stimulating effect of hrRANKL was lower than the values obtained with FBS (5% and 10%) (Fig. 4, panel H).
3.2.5Migration capacity
The CD34(-) spindle-shape stromal cells had migratory capacity when stimulated with 50 ng/ml of hrRANKL. Furthermore, this migratory capacity was inhibited when adding an anti-RANKL Ab to the assay (Fig. 4, panel I and K). Similarly, when the study was conducted with the blocking of the RANKL receptor, RANK, using an anti-RANK Ab, a similar reduction in migration was observed (Fig. 4, panel J and L). Thus, in our experimental conditions, hrRANKL promoted CD34(-) spindle-shaped stromal cells migration.
4.Discussion
There is substantial evidence supporting the presence of RANK in various functional organs and cell types, including osteoclasts, MSCs, osteoblasts, endothelial cells, mammary and immune system cells, and certain cancer cells, such as breast and prostate cancer [28, 29, 30, 44]. Although limited studies have assessed RANK expression in primary tumors as a prognostic factor, it is worth mentioning that functional RANK expression has been reported in human breast cancer cell lines [15]. Additionally, Santini et al. found that the expression of RANK in the primary tumor of BCPs, particularly those with invasive ductal breast carcinoma, is associated with the occurrence of bone metastases and the time to this type of metastasis [45]. These findings suggest that the RANK status in breast cancer cells plays a crucial role in their tendency to metastasize to bone, particularly when RANKL is abundantly expressed [15, 45]. Our study focused on evaluated RANK expression in spindle-shape stromal cells, not associated to the vasculature, (fibroblast-like). We investigated its correlation with clinical-pathological characteristics, to gain valuable insights into the role of these cells in breast cancer progression and prognosis. Our findings revealed a clear association between RANK expression in these stromal cells and the occurrence of metastasis. Specifically, high RANK expression was significantly associated with an increased risk of developing metastases. While no significant association was established between RANK expression and the occurrence of site-specific metastasis, the trend toward an association with bone metastasis suggests a potential role in this particular type of metastatic event. Furthermore, the observed correlation between RANK expression and the number of metastatic foci per organ added implies that RANK could serve as a valuable marker for assessing bone response in metastatic patients. This could aid in the early detection of imbalances in bone homeostasis [46]. Our results showed that the significant association between RANK expression in spindle- shape stromal cells, not associated to the vasculature, and MFS, BMFS, MMFS, and OS highlights the potential role of RANK as a prognostic indicator for early BCPs (stage I/II). Patients with high RANK expression in these type of stromal cells had a shorter MFS, BMFS, and MMFS, as well as lower OS compared to those with low RANK expression. This proposes that RANK expression could serve as a valuable prognostic biomarker for predicting disease progression and survival in early BCPs. From the study of RANK expression in relation to stromal characteristics, significant associations emerged with intratumoral stromal percentage and blood and lymphatic vascularization. Patients with high RANK expression had a higher abundance of intratumoral stroma, as well as increased blood and lymphatic vascularization. These results suggest a possible role of RANK(+) CAFs in influencing the composition of the microenvironment and vascularization patterns, factors that may play a critical role in breast cancer progression and metastasis. It is known that abundance of intratumoral stroma, as well as increased blood and lymphatic vascularization are powerful prognostic factors of poorer survival in BCPs [47, 48, 49]. RANK expression emerged as an independent prognostic factor for MFS and OS in BCPs, reinforcing its potential as a prognostic indicator, which can provide additional information beyond classical prognostic markers.
When performing the phenotypic characterization of CD34(-) CAFs isolated from the primary tumor of luminal BCPs, we discovered that these stromal cells express RANK in 37.14
Previous studies demonstrate the involvement of the RANKL-RANK system in cell self-renewal and proliferation processes [27, 51]. However, in our experimental model, our results showed no significant differences in CFU-F and proliferation capacity when comparing CD34(-) fibroblasts with and without hrRANKL treatment. Nevertheless, hrRANKL treatment resulted in an upregulation of genes associated with self-renewal and multipotency. The basal expression level of OCT-4 is critical for preserving the stemness and differentiation potential of stem cells, like MSCs, by modulating the expression of SOX-2 and NANOG [52]. In this study, we observed that treatment with hrRANKL increased the expression of OCT-4 and SOX-2. These findings suggest that the RANKL-RANK system may promote MSCs-like properties in these CAFs. Furthermore, a major concern in the field of cell therapy is the potential conversion of stem cells into malignant forms, like cancer stem cells. As tumor stem cells dedifferentiate, they often reactivate specific stem cell markers, with OCT-4 levels frequently increasing in cancer stem cells [53, 54]. So the examination of RANK-RANKL expression as well as OCT-4 gene expression in stem cells such as MSCs or the CAFs that originated from them could serve as a valuable tool for predicting their potential for malignant transformation in tumor stromal microenvironment of early BCPs Also, Hiroaki K. et al. described that elevated expression of SOX2 in the stromal tissue of colorectal cancer patients is linked to increased invasiveness and a less favorable prognosis in terms of recurrence-free survival [55]. These previous observations suggest that the RANKL-RANK system, by promoting the expression of SOX-2, may induce the pro-tumoral activity of these stromal cells in early BCPs. Our results also showed that treatment with hrRANKL increased the gene expression of DKK-1. In this regard, Gregory CA. et al. discovered that human bone marrow MSCs at clonal densities, initiate the production of the Wnt inhibitor, DKK-1. This mechanism enables cells to re-enter the cell cycle by suppressing the canonical Wnt/
Metastasis are a common and significant complication in many cancer patients, contributing significantly to morbidity and mortality [58]. Although breast cancer have a marked tendency to metastasize to bones [59], the underlying reasons for this osteotropic behavior remain poorly understood. Given the high expression of RANKL in the bone marrow/bone environment, the hypothesis arises that RANKL could function as a chemoattractant for breast tumor cells [9]. In particular, our study on the migratory capacity of CD34(-) CAFs observed in response to stimulation with hrRANKL, as well as the inhibition of this migration after the addition of an anti-RANKL or anti-RANK Ab, suggests that this response to RANKL is primarily mediated by the RANK receptor. Previous results of our group, suggest a reciprocal communication between RANKL expression in breast tumor cells and spindle-shaped stromal cells, not associated to the vasculature, and an autocrine and paracrine regulation of RANK, in particular in this type of CAFs [10]. Taking into account this background information and our results from the present study, it is conceivable that the RANKL-RANK system could play a significant role in the migration of CAFs within the breast tumor microenvironment, as well as in their extravasation to future pre-metastatic niches, such as the bone. Studies of other authors, showed that CAFs can enhance the survival and establishment of cancer cells in distant parts of the body by spreading through the bloodstream as either circulating CAFs or CAF clusters, sometimes in the presence of cancer cells and sometimes independently [60, 61]. Circulating CAFs and clusters of CAFs have been detected in the peripheral blood of breast cancer patients with metastatic diseases [61].
All of these results together contribute in part to a better understanding of the role of RANK(+) CAFs in the tumor microenvironment and open avenues for further research on therapies targeting RANKL-RANK signaling in the context of breast cancer. Hence, blocking RANKL and RANK with Denosumab (RANKL Ab) or RANK Ab, respectively, could serve as preventive strategies to decrease the incidence of breast cancer initiation and metastasis, targeting not only the breast tumor cell but also the RANK (+) CAFs.
5.Conclusion
The results of both retrospective and prospective studies provide a comprehensive understanding of the influence of RANK expression in the tumor microenvironment and its impact on the progression and prognosis of early BCPs. RANK expression in CAFs emerges as a clinically relevant marker, associated with the occurrence of metastasis, the formation of multiple metastatic foci, and a poorer prognosis in terms of MFS and OS. Additionally, its involvement in the composition of the intratumoral stroma and its influence on vascularization are evident, highlighting its role in regulating these stromal cells as a potential prognostic marker and therapeutic target in the management of breast cancer. This provides a solid base for future research and clinical developments aimed at manipulating the RANKLRANK signaling pathway in this context. On the other hand, migratory and proliferative response to hrRANKL as well as the upregulation of the expression of OCT-4, SOX-2 and DKK-1 genes in CAFs indicate a complex and regulated interaction with the microenvironment. These findings expand our understanding of the versatility and function of these stromal cells in breast cancer, contributing to potential therapeutic approaches in the future.
Funding
Not applicable.
Abbreviations
RANK receptor activator of nuclear factor kappa-
RANKL receptor activator of nuclear factor kappa-
CAFs cancer-associated fibroblast.
MSCs mesenchymal stem/stromal cells.
BCPs breast cancer patients.
DKK-1 Dickkopf 1.
MFS metastasis-free survival.
BMFS bone metastasis-free survival.
VMFS visceral metastasis-free survival.
RFS local relapse-free survival.
MMFS mix metastasis-free survival.
OS overall survival.
ER estrogen receptor.
PR progesterone receptor.
FBS fetal bovine serum.
CFU-F fibroblastic colony-forming unit.
Authors’ contributions
Conception: Norma Alejandra Chasseing, María Belén Giorello.
Interpretation or analysis of data: María Belén Giorello, Francisco Raúl Borzone, María Florencia Mora, María del Rosario Padin, Alejandra Wernicke, Vivian Labovsky.
Preparation of the manuscript: María Belén Giorello, Norma Alejandra Chasseing.
Revision for important intellectual content: Norma Alejandra Chasseing, Vivian Labovsky, María Belén Giorello.
Supervision: Norma Alejandra Chasseing.
Acknowledgments
The authors would like to thank the Consejo Nacional de Investigaciones Científicas y Técnicas (CONICET), Argentina, Fondo para la Investigación Científica y Tecnológica (FONCYT), Argentina (PICT2017 #2501), Roemmers Foundation (2019-2021), René Barón Foundation, Argentina and Williams Foundation, Argentina.
Conflict of interest
The authors declare there are no potential conflicts of interest with respect to the research, authorship and/or publication of this article. Consent to participate Informed consent was obtained from all individual participants included in the study.
References
[1] | G. Lorusso and C. Rüegg, The tumor microenvironment and its contribution to tumor evolution toward metastasis, Histochem. Cell Biol. 130: ((2008) ), 1091–1103. doi: 10.1007/s00418-008-0530-8. |
[2] | M.J. Bissell, D.C. Radisky, A. Rizki, V.M. Weaver and O.W. Petersen, The organizing principle: Microenvironmental influences in the normal and malignant breast, Differentiation 70: ((2002) ), 537–546. doi: 10.1046/j.1432-0436.2002.700907.x. |
[3] | R. Kalluri and M. Zeisberg, Fibroblasts in cancer, Nat. Rev. Cancer 6: ((2006) ), 392–401. doi: 10.1038/nrc1877. |
[4] | M.B. Giorello, F.R. Borzone, V. Labovsky, F.V. Piccioni and N.A. Chasseing, Cancer-Associated Fibroblasts in the Breast Tumor Microenvironment, J. Mammary Gland Biol. Neoplasia 26: ((2021) ), 135–155. doi: 10.1007/s10911-020-09475-y. |
[5] | M. Sarkar, T. Nguyen, E. Gundre, O. Ogunlusi, M. El-Sobky, B. Giri and T.R. Sarkar, Cancer-associated fibroblasts: The chief architect in the tumor microenvironment, Front. Cell Dev. Biol. 11: ((2023) ), 1–24. doi: 10.3389/fcell.2023.1089068. |
[6] | E. Elwakeel and A. Weigert, Breast Cancer CAFs: Spectrum of Phenotypes and Promising Targeting Avenues, Int. J. Mol. Sci. 22: ((2021) ), 11636. doi: 10.3390/ijms222111636. |
[7] | Y. Raz, N. Cohen, O. Shani, R.E. Bell, S. V Novitskiy, L. Abramovitz, C. Levy, M. Milyavsky, L. Leider-Trejo, H.L. Moses, D. Grisaru and N. Erez, Bone marrow – derived fibroblasts are a functionally distinct stromal cell population in breast cancer, J. Exp. Med. 215: ((2018) ), 3075–3093. doi: 10.1084/jem.20180818. |
[8] | A.E. Karnoub, A.B. Dash, A.P. Vo, A. Sullivan, M.W. Brooks, G.W. Bell, A.L. Richardson, K. Polyak, R. Tubo and R.A. Weinberg, Mesenchymal stem cells within tumour stroma promote breast cancer metastasis, Nature. 449: ((2007) ), 557–563. doi: 10.1038/nature06188. |
[9] | P. Barcellos-de-souza, V. Gori, F. Bambi and P. Chiarugi, Tumor microenvironment: Bone marrow-mesenchymal stem cells as key players, BBA – Rev. Cancer ((2013) ). doi: 10.1016/j.bbcan.2013.10.004. |
[10] | V. Labovsky, L.M. Martinez, K.M. Davies, H. García-rivello, M.D.L. Calcagno, A. Matas, V. Beatriz, F. Vallone, A. Wernicke, H. Choi and N.A. Chasseing, Association Between Ligands and Receptors Related to the Progression of Early Breast Cancer in Tumor Epithelial and Stromal Cells, Clin. Breast Cancer. 15: ((2015) ), e13–e21. doi: 10.1016/j.clbc.2014.05.006. |
[11] | L. Kiesel and A. Kohl, Role of the RANK/RANKL pathway in breast cancer, Maturitas 86: ((2016) ), 10–16. doi: 10.1016/j.maturitas.2016.01.001. |
[12] | V. Sigl and J.M. Penninger, RANKL/RANK – From bone physiology to breast cancer, Cytokine Growth Factor Rev. 25: ((2014) ), 205–214. doi: 10.1016/j.cytogfr.2014.01.002. |
[13] | V. Sigl, L.P. Jones and J.M. Penninger, RANKL/RANK: from bone loss to the prevention of breast cancer, Open Biol. 6: ((2016) ), 160230. doi: 10.1098/rsob.160230. |
[14] | E. Gonzalez-Suarez, A.P. Jacob, J. Jones, R. Miller, M.P. Roudier-Meyer, R. Erwert, J. Pinkas, D. Branstetter and W.C. Dougall, RANK ligand mediates progestin-induced mammary epithelial proliferation and carcinogenesis, Nature 468: ((2010) ), 103–107. doi: 10.1038/nature09495. |
[15] | D.H. Jones, T. Nakashima, O.H. Sanchez, I. Kozieradzki, S. V Komarova, I. Sarosi, S. Morony, E. Rubin, R. Sarao, C. V Hojilla, V. Komnenovic, Y. Kong, M. Schreiber, S.J. Dixon, S.M. Sims, R. Khokha, T. Wada and J.M. Penninger, Regulation of cancer cell migration and bone metastasis by RANKL, Nature. 440: ((2006) ), 692–696. doi: 10.1038/nature04524. |
[16] | D. Schramek, A. Leibbrandt, V. Sigl, L. Kenner, J.A. Pospisilik, H.J. Lee, R. Hanada, P.A. Joshi, A. Aliprantis, L. Glimcher, M. Pasparakis, R. Khokha, C.J. Ormandy, M. Widschwendter, G. Schett and J.M. Penninger, Osteoclast differentiation factor RANKL controls development of progestin-driven mammary cancer, Nature 468: ((2010) ), 98–102. doi: 10.1038/nature09387. |
[17] | G. Tonini, M. Imperatori, B. Vincenzi, A.M. Frezza and D. Santini, Rechallenge therapy and treatment holiday: different strategies in management of metastatic colorectal cancer, J. Exp. Clin. Cancer Res. 32: ((2013) ), 92. doi: 10.1186/1756-9966-32-92. |
[18] | V. Sigl, K. Owusu-Boaitey, P.A. Joshi, A. Kavirayani, G. Wirnsberger, M. Novatchkova, I. Kozieradzki, D. Schramek, N. Edokobi, J. Hersl, A. Sampson, A. Odai-Afotey, C. Lazaro, E. Gonzalez-Suarez, M.A. Pujana, F. CIMBA, H. Heyn, E. Vidal, J. Cruickshank, H. Berman, R. Sarao, M. Ticevic, I. Uribesalgo, L. Tortola, S. Rao, Y. Tan, G. Pfeiler, E.Y. Lee, Z. Bago-Horvath, L. Kenner, H. Popper, C. Singer, R. Khokha, L.P. Jones and J.M. Penninger, RANKL/RANK control Brca1 mutation-driven mammary tumors, Cell Res. 26: ((2016) ), 761–774. doi: 10.1038/cr.2016.69. |
[19] | M. Infante, A. Fabi, F. Cognetti, S. Gorini, M. Caprio and A. Fabbri, RANKL/RANK/OPG system beyond bone remodeling: involvement in breast cancer and clinical perspectives, J. Exp. Clin. Cancer Res. 38: ((2019) ), 12. doi: 10.1186/s13046-018-1001-2. |
[20] | M. Palafox, I. Ferrer, P. Pellegrini, S. Vila, S. Hernandez-Ortega, A. Urruticoechea, F. Climent, M.T. Soler, P. Muñoz, F. Viñals, M. Tometsko, D. Branstetter, W.C. Dougall and E. González-Suárez, RANK Induces Epithelial – Mesenchymal Transition and Stemness in Human Mammary Epithelial Cells and Promotes Tumorigenesis and Metastasis, Cancer Res. 72: ((2012) ), 2879–2888. doi: 10.1158/0008-5472.CAN-12-0044. |
[21] | R.M. Dwyer, S.M. Potter-Beirne, K.A. Harrington, A.J. Lowery, E. Hennessy, J.M. Murphy, F.P. Barry, T. O’Brien and M.J. Kerin, Monocyte Chemotactic Protein-1 Secreted by Primary Breast Tumors Stimulates Migration of Mesenchymal Stem Cells, Clin. Cancer Res. 13: ((2007) ), 5020–5027. doi: 10.1158/1078-0432.CCR-07-0731. |
[22] | J. Dittmer, I. Oerlecke and B. Leyh, Involvement of Mesenchymal Stem Cells in Breast Cancer Progression, in: Breast Cancer – Focus. Tumor Microenviron. Stem Cells Metastasis, InTech, 2011. doi: 10.5772/21325. |
[23] | M. Palafox, I. Ferrer, P. Pellegrini, S. Vila, S. Hernandez-Ortega, A. Urruticoechea, F. Climent, M.T. Soler, P. Muñoz, F. Viñals, M. Tometsko, D. Branstetter, W.C. Dougall and E. González-Suárez, RANK induces epithelial-mesenchymal transition and stemness in human mammary epithelial cells and promotes tumorigenesis and metastasis, Cancer Res. 72: ((2012) ), 2879–2888. doi: 10.1158/0008-5472.CAN-12-0044. |
[24] | S.M. Potter, R.M. Dwyer, M.C. Hartmann, S. Khan, M.P. Boyle, C.E. Curran and M.J. Kerin, Influence of stromal–epithelial interactions on breast cancer in vitro and in vivo, Breast Cancer Res. Treat. 131: ((2012) ), 401–411. doi: 10.1007/s10549-011-1410-9. |
[25] | M. Mimeault and S.K. Batra, Altered gene products involved in the malignant reprogramming of cancer stem/progenitor cells and multitargeted therapies, Mol. Aspects Med. 39: ((2014) ), 3–32. doi: 10.1016/j.mam.2013.08.001. |
[26] | J. Xiong, M. Onal, R.L. Jilka, R.S. Weinstein, S.C. Manolagas and C.A. O’Brien, Matrix-embedded cells control osteoclast formation, Nat. Med. 17: ((2011) ), 1235–1241. doi: 10.1038/nm.2448. |
[27] | F. Schena, C. Menale, E. Caci, L. Diomede, E. Palagano, C. Recordati, M. Sandri, A. Tampieri, I. Bortolomai, V. Capo, C. Pastorino, A. Bertoni, M. Gattorno, A. Martini, A. Villa, E. Traggiai and C. Sobacchi, Murine Rankl-/- Mesenchymal Stromal Cells Display an Osteogenic Differentiation Defect Improved by a RANKL-Expressing Lentiviral Vector, Stem Cells. 35: ((2017) ), 1365–1377. doi: 10.1002/stem.2574. |
[28] | X. Chen, X. Zhi, J. Wang and J. Su, RANKL signaling in bone marrow mesenchymal stem cells negatively regulates osteoblastic bone formation, Bone Res. 6: ((2018) ), 34. doi: 10.1038/s41413-018-0035-6. |
[29] | J. Li, I. Sarosi, X.-Q. Yan, S. Morony, C. Capparelli, H.-L. Tan, S. McCabe, R. Elliott, S. Scully, G. Van, S. Kaufman, S.-C. Juan, Y. Sun, J. Tarpley, L. Martin, K. Christensen, J. McCabe, P. Kostenuik, H. Hsu, F. Fletcher, C.R. Dunstan, D.L. Lacey and W.J. Boyle, RANK is the intrinsic hematopoietic cell surface receptor that controls osteoclastogenesis and regulation of bone mass and calcium metabolism, Proc. Natl. Acad. Sci. 97: ((2000) ), 1566–1571. doi: 10.1073/pnas.97.4.1566. |
[30] | W.C. Dougall, M. Glaccum, K. Charrier, K. Rohrbach, K. Brasel, T. De Smedt, E. Daro, J. Smith, M.E. Tometsko, C.R. Maliszewski, A. Armstrong, V. Shen, S. Bain, D. Cosman, D. Anderson, P.J. Morrissey, J.J. Peschon and J. Schuh, RANK is essential for osteoclast and lymph node development, Genes Dev. 13: ((1999) ), 2412–2424. doi: 10.1101/gad.13.18.2412. |
[31] | M. Baud’huin, F. Lamoureux, L. Duplomb, F. R’edini and D. Heymann, RANKL, RANK, osteoprotegerin: key partners of osteoimmunology and vascular diseases, Cell. Mol. Life Sci. 64: ((2007) ), 2334–2350. doi: 10.1007/s00018-007-7104-0. |
[32] | Y. Wittrant, F. Lamoureux, K. Mori, A. Riet, A. Kamijo, D. Heymann and F. Redini, RANKL directly induces bone morphogenetic protein-2 expression in RANK-expressing POS-1 osteosarcoma cells. Int. J. Oncol. 28: ((2006) ), 261–9. http://wwwncbi.nlm.nih.gov/pubmed/16328004. |
[33] | Y. Ikebuchi, S. Aoki, M. Honma, M. Hayashi, Y. Sugamori, M. Khan, Y. Kariya, G. Kato, Y. Tabata, J.M. Penninger, N. Udagawa, K. Aoki and H. Suzuki, Coupling of bone resorption and formation by RANKL reverse signalling, Nature. 561: ((2018) ), 195–200. doi: 10.1038/s41586-018-0482-7. |
[34] | D. Golden, E.A. Saria and M.F. Hansen, Regulation of Osteoblast Migration Involving Receptor Activator of Nuclear Factor-kappa B (RANK) Signaling, J. Cell. Physiol. 230: ((2015) ), 2951–2960. doi: 10.1002/jcp.25024. |
[35] | J.D. Brierley, M.K. Gospodarowicz and C. Wittekind, TNM Classification of Malignant Tumours, 8th ed., (2017) . |
[36] | B.C. Pestalozzi, E. Luporsi-Gely, L.M. Jost and J. Bergh, ESMO Minimum Clinical Recommendations for diagnosis, adjuvant treatment and follow-up of primary breast cancer, Ann. Oncol. 16: ((2005) ), i7–i9. doi: 10.1093/annonc/mdi825. |
[37] | E. Senkus, S. Kyriakides, F. Penault-Llorca, P. Poortmans, A. Thompson, S. Zackrisson and F. Cardoso, Primary breast cancer: ESMO clinical practice guidelines for diagnosis, treatment and follow-up, Ann. Oncol. 26: ((2015) ), 8–30. doi: 10.1093/annonc/mdv298. |
[38] | M. Wernicke, P. Roitman, D. Manfre and R. Stern, Breast cancer and the stromal factor. The “prometastatic healing process” hypothesis, Medicina (B. Aires). 71: ((2011) ), 15–21. |
[39] | H.J.G. Bloom and W.W. Richardson, Histological Grading and Prognosis in Breast Cancer, Br. J. Cancer. 11: ((1957) ), 359–377. doi: 10.1038/bjc.1957.43. |
[40] | L.M. Martinez, V. Labovsky, M. de Luján Calcagno, K.M. Davies, H.G. Rivello, M.S. Bianchi, A. Wernicke, V.B.F. Vallone and N.A. Chasseing, CD105 Expression on CD34-Negative Spindle-Shaped Stromal Cells of Primary Tumor Is an Unfavorable Prognostic Marker in Early Breast Cancer Patients, PLoS One. 10: ((2015) ), e0121421. doi: 10.1371/journal.pone.0121421. |
[41] | M.E.H. Hammond, D.F. Hayes, M. Dowsett, D.C. Allred, K.L. Hagerty, S. Badve, P.L. Fitzgibbons, G. Francis, N.S. Goldstein, M. Hayes, D.G. Hicks, S. Lester, R. Love, P.B. Mangu, L. McShane, K. Miller, C.K. Osborne, S. Paik, J. Perlmutter, A. Rhodes, H. Sasano, J.N. Schwartz, F.C.G. Sweep, S. Taube, E.E. Torlakovic, P. Valenstein, G. Viale, D. Visscher, T. Wheeler, R.B. Williams, J.L. Wittliff and A.C. Wolff, American society of clinical oncology/college of american pathologists guideline recommendations for immunohistochemical testing of estrogen and progesterone receptors in breast cancer, J. Clin. Oncol. 28: ((2010) ), 2784–2795. doi: 10.1200/JCO.2009.25.6529. |
[42] | J. Schindelin, I. Arganda-Carreras, E. Frise, V. Kaynig, M. Longair, T. Pietzsch, S. Preibisch, C. Rueden, S. Saalfeld, B. Schmid, J.-Y. Tinevez, D.J. White, V. Hartenstein, K. Eliceiri, P. Tomancak and A. Cardona, Fiji: an open-source platform for biological-image analysis, Nat. Methods 9: ((2012) ), 676–682. doi: 10.1038/nmeth.2019. |
[43] | F.R. Borzone, M.B. Giorello, L.M. Martinez, M.C. Sanmartin, L. Feldman, F. Dimase, E. Batagelj, G. Yannarelli and N.A. Chasseing, Senescent mesenchymal stem/stromal cells in pre-metastatic bone marrow of untreated advanced breast cancer patients, Oncol. Res. 31: ((2023) ), 361–374. doi: 10.32604/or.2023.028104. |
[44] | V. Labovsky, L.M. Martinez, K.M. Davies, H. García-Rivello, M. De Luján Calcagno, A. Matas, V.B. Fernández Vallone, A. Wernicke, H. Choi and N.A. Chasseing, Association between ligands and receptors related to the progression of early breast cancer in tumor epithelial and stromal cells, Clin. Breast Cancer. 15: ((2015) ), e13–e21. doi: 10.1016/j.clbc.2014.05.006. |
[45] | D. Santini, G. Schiavon, B. Vincenzi, L. Gaeta, F. Pantano, A. Russo, C. Ortega, C. Porta, S. Galluzzo, G. Armento, N. La Verde, C. Caroti, I. Treilleux, A. Ruggiero, G. Perrone, R. Addeo, P. Clezardin, A.O. Muda and G. Tonini, Receptor activator of NF-kB (rank) expression in primary tumors associates with bone metastasis occurrence in breast cancer patients, PLoS One 6: ((2011) ). doi: 10.1371/journal.pone.0019234. |
[46] | M. Sisay, G. Mengistu and D. Edessa, The RANK/RANKL/ OPG system in tumorigenesis and metastasis of cancer stem cell: potential targets for anticancer therapy, Onco. Targets. Ther. 10: ((2017) ), 3801–3810. doi: 10.2147/OTT.S135867. |
[47] | E.M. de Kruijf, J.G.H. van Nes, C.J.H. van de Velde, H. Putter, V.T.H.B.M. Smit, G.J. Liefers, P.J.K. Kuppen, R.A.E.M. Tollenaar and W.E. Mesker, Tumor-stroma ratio in the primary tumor is a prognostic factor in early breast cancer patients, especially in triple-negative carcinoma patients, Breast Cancer Res. Treat. 125: ((2011) ), 687–696. doi: 10.1007/s10549-010-0855-6. |
[48] | C.J.H. Kramer, K.M.H. Vangangelt, G.W. van Pelt, T.J.A. Dekker, R.A.E.M. Tollenaar and W.E. Mesker, The prognostic value of tumour–stroma ratio in primary breast cancer with special attention to triple-negative tumours: a review, Breast Cancer Res. Treat. 173: ((2019) ), 55–64. doi: 10.1007/s10549-018-4987-4. |
[49] | G. Van den Eynden, I. Van der Auwera, S. Van Laere, X. Trinh, C. Colpaert, P. van Dam, L. Dirix, P. Vermeulen and E. Van Marck, Comparison of molecular determinants of angiogenesis and lymphangiogenesis in lymph node metastases and in primary tumours of patients with breast cancer, J. Pathol. 213: ((2007) ), 56–64. doi: 10.1002/path.2211. |
[50] | Y. Raz, N. Cohen, O. Shani, R.E. Bell, S.V. Novitskiy, L. Abramovitz, C. Levy, M. Milyavsky, L. Leider-Trejo, H.L. Moses, D. Grisaru and N. Erez, Bone marrow-derived fibroblasts are a functionally distinct stromal cell population in breast cancer, J. Exp. Med. 215: ((2018) ), 3075–3093. doi: 10.1084/jem.20180818. |
[51] | V. Duheron, E. Hess, M. Duval, M. Decossas, B. Castaned, J.E. Klöpper, L. Amoas, J.B. Barbaroux, I.R. Williams, H. Yagita, J. Penninger, Y. Choi, F. Lézot, R. Groves, R. Paus and C.G. Mueller, Receptor activator of NF-κB (RANK) stimulates the proliferation of epithelial cells of the epidermo-pilosebaceous unit, Proc. Natl. Acad. Sci. U.S.A. 108: ((2011) ), 5342–5347. doi: 10.1073/pnas.1013054108. |
[52] | R. Malvicini, D. Santa-Cruz, N. Pacienza and G. Yannarelli, OCT4 Silencing Triggers Its Epigenetic Repression and Impairs the Osteogenic and Adipogenic Differentiation of Mesenchymal Stromal Cells, Int. J. Mol. Sci. 20: ((2019) ), 3268. doi: 10.3390/ijms20133268. |
[53] | Y. Bu and D. Cao, The origin of cancer stem cells, Front Biosci (Schol Ed) 4: ((2012) ), 819–830. doi: 10.2741/s302. |
[54] | X.Q. Wang, W.M. Ongkeko, L. Chen, Z.F. Yang, P. Lu, K.K. Chen, J.P. Lopez, R.T.P. Poon and S.T. Fan, Octamer 4 (Oct4) Mediates Chemotherapeutic Drug Resistance in Liver Cancer Cells Through a Potential Oct4–AKT–ATP-Binding Cassette G2 Pathway, Hepatology 52: ((2010) ), 528–539. doi: 10.1002/hep.23692. |
[55] | H. Kasashima, A. Duran, A. Martinez-Ordoñez, Y. Nakanishi, H. Kinoshita, J.F. Linares, M. Reina-Campos, Y. Kudo, A. L’Hermitte, M. Yashiro, M. Ohira, F. Bao, D.V.F. Tauriello, E. Batlle, M.T. Diaz-Meco and J. Moscat, Stromal SOX2 Upregulation Promotes Tumorigenesis through the Generation of a SFRP1/2-Expressing Cancer-Associated Fibroblast Population, Dev. Cell 56: ((2021) ), 95–110.e10. doi: 10.1016/j.devcel.2020.10.014. |
[56] | C.A. Gregory, H. Singh, A.S. Perry and D.J. Prockop, The Wnt Signaling Inhibitor Dickkopf-1 Is Required for Reentry into the Cell Cycle of Human Adult Stem Cells from Bone Marrow, J. Biol. Chem. 278: ((2003) ), 28067–28078. doi: 10.1074/jbc.M300373200. |
[57] | M. Li, H. Cai, Y. Yang, J. Zhang, K. Sun, Y. Yan, H. Qu, W. Wang, J. Wang and X. Duan, Perichondrium mesenchymal stem cells inhibit the growth of breast cancer cells via the DKK-1/Wnt/β-catenin signaling pathway, Oncol. Rep. 36: ((2016) ), 936–944. doi: 10.3892/or.2016.4853. |
[58] | G. Christofori, New signals from the invasive front, Nature 441: ((2006) ), 444–450. doi: 10.1038/nature04872. |
[59] | F. Macedo, K. Ladeira, F. Pinho, N. Saraiva, N. Bonito, L. Pinto and F. Gonçalves, Bone metastases: an overview, Oncol. Rev. ((2017) ), doi: 10.4081/oncol.2017.321. |
[60] | N. Ortiz-Otero, A.B. Clinch, J. Hope, W. Wang, C.A. Reinhart-King and M.R. King, Cancer associated fibroblasts confer shear resistance to circulating tumor cells during prostate cancer metastatic progression, Oncotarget. 11: ((2020) ), 1037–1050. doi: 10.18632/oncotarget.27510. |
[61] | Z. Ao, S.H. Shah, L.M. Machlin, R. Parajuli, P.C. Miller, S. Rawal, A.J. Williams, R.J. Cote, M.E. Lippman, R.H. Datar and D. El-Ashry, Identification of cancer-associated fibroblasts in circulating blood from patients with metastatic breast cancer, Cancer Res. 75: ((2015) ), 4681–4687. doi: 10.1158/0008-5472.CAN-15-1633. |