EMP3: A promising biomarker for tumor prognosis and targeted cancer therapy
Abstract
Epithelial membrane protein 3 (EMP3) belongs to the peripheral myelin protein 22 kDa (PMP22) gene family, characterized by four transmembrane domains and widespread expression across various human tissues and organs. Other members of the PMP22 family, including EMP1, EMP2, and PMP22, have been linked to various cancers, such as glioblastoma, laryngeal cancer, nasopharyngeal cancer, gastric cancer, breast cancer, and endometrial cancer. However, few studies report on the function and relevance of EMP3 in tumorigenicity. Given the significant structural similarities among members of the PMP22 family, there are likely potential functional similarities as well. Previous studies have established the regulatory role of EMP3 in immune cells like T cells and macrophages. Additionally, EMP3 is found to be involved in critical signaling pathways, including HER-2/PI3K/Akt, MAPK/ERK, and TGF-beta/Smad. Furthermore, EMP3 is associated with cell cycle regulation, cellular proliferation, and apoptosis. Hence, it is likely that EMP3 participates in cancer development through these aforementioned pathways and mechanisms. This review aims to systematically examine and summarize the structure and function of EMP3 and its association to various cancers. EMP3 is expected to emerge as a significant biological marker for tumor prognosis and a potential target in cancer therapeutics.
1.Introduction
The peripheral myelin protein 22 kDa (PMP22) gene family encompasses several members, including epithelial membrane protein1, 2, and 3 (EMP1, EMP2, EMP3), PMP22, p53 apoptosis effect related to PMP22 (PERP), lens fiber cell protein MP20 (also named LIM2), and brain cell membrane protein [1]. Genes within this family are implicated in cellular proliferation, migration, and invasion and have active roles in tumor development [2]. Members of the PMP22 family share significant structural similarities and homology, suggesting potential overlap in function and involvement in cancer. PMP22 proteins are hydrophobic and approximately 160 amino acids long, containing two extracellular domains, four transmembrane domains, and small intracellular regions [3]. The amino acid sequence homology of EMP3 with PMP22, EMP1, EMP2 and MP20 is 41%, 33%, 38% and 23%, respectively. EMP3, PMP22, and EMP2 share the same C-terminal residue, suggesting that this motif may have functional importance as it closely resembles the retrieval signal of endoplasmic reticulum proteins [4]. Within the EMP protein family, all members feature N-glycosylation sites and share a highly conserved transmembrane domain [3]. There are notable structural differences between EMPs. EMP3 lacks a functional site for phosphorylation by casein kinase 2, whereas EMP1 and EMP2 contain the target site. EMP2 and EMP3 share similar molecular structures, both featuring an N-terminal glycosylation site in their first hydrophilic domain and a protein kinase C (PKC) phosphorylation site, both of which are absent in EMP1. EMP2 does not contain an N-myristoylation region. Moreover, expression of PMP22 proteins varies across organs and tissues. EMP1 mRNA levels are high in the esophagus, followed by adipose tissue, and the gallbladder, while EMP1 protein levels are high in the stomach but low elsewhere. EMP2 is most commonly expressed in the lung, skin, and esophagus and least commonly expressed in the pancreas and brain. A higher transcript level of EMP2 is found in the fetal lung and kidney but not in the adult thymus or peripheral leukocytes. In contrast to EMP2, EMP3 mRNA levels are high in peripheral leukocytes of adults and relatively high in fetal lungs, liver, and kidneys [4].
Many studies have confirmed the relationship between PMP22 family genes and cancer. Cañellas et al. [5] used EMP1 as a marker for highly recurrent cells in colon cancer. The group tracked and selectively eliminated this cell population and found that eliminating cells with high EMP1 expression could prevent metastasis and recurrence of colorectal cancer. In prostate cancer, EMP1 is downregulated and believed to negatively regulate tumor cells through modulation of caspase-9 and vascular endothelial growth factor (VEGF) levels [6]. A reduction in EMP1 expression is also observed in other cancers, such as laryngeal cancer, oral squamous cell carcinoma, nasopharyngeal carcinoma, and gastric cancer. EMP1 overexpression was found to inhibit tumor cell proliferation in esophageal cancer. On the other hand, EMP1 levels are higher in non-small-cell lung carcinoma than in tissues from benign controls, possibly due to the aberrant activation of the phosphatidylinositol 3-kinase (PI3K)/protein kinase B (Akt) signaling pathway [7, 8, 9, 10, 11]. EMP2 has been identified as an oncogene in human endometrial and ovarian cancers, promoting tumor formation through focal adhesion kinase (FAK) and steroid receptor coactivator (SRC) activation [1]. A study quantifying EMP2 expression levels in breast cancer cell lines found a 2.56-fold higher expression in MDA-MB-231 cells than in MCF7 cells. Importantly, EMP2 outperformed epithelial cell adhesion molecule in identifying breast cancer cells in patient blood samples, suggesting its potential as a novel biomarker and target [12]. While patients with normal brain tissue typically have low and undetectable levels of EMP2 expression, these levels are significantly elevated in 95% of patients with glioblastoma (GBM). EMP2 enhances glioma tumor growth in vivo by promoting cell migration and invasion. EMP2 upregulates
Studies looking into EMP3 have been relatively limited, yet the protein’s unique characteristics warrant further investigation. The EMP3 gene is located on chromosome 19q13.3, a genomic locus characterized by high gene density. This region carries mediator29 (MED29), formerly known as IXL, polymerase-associated factor1 (PAF1), dual specificity tyrosine phosphorylation regulated kinase1B (DYRK1B), p21-activated kinase4 (PAK4), cyclin E3, which have undergone functional validation and are suggested to be putative amplicon targets [17, 18, 19]. Alaminos et al. [20] compared the expression profiles of 89 neuroblastoma tumors with that of benign ganglioneuromas through microarray analysis. The group found EMP3 was the only gene whose expression is down-regulated by more than threefold and contains CpG islands. While the exact biological function of EMP3 remains incompletely understood, some studies have employed EMP3 as a marker for tumor proliferation. EMP3 is associated with various cellular activities, including cell migration, antiviral and antitumor responses, and inflammatory processes, thereby influencing disease onset and progression [21, 22]. Notably, EMP3 is considered a tumor suppressor gene in certain tumor types and has also emerged as a potential target for cancer therapy [23, 24]. Therefore, a comprehensive investigation into the role of EMP3 in the context of tumorigenesis is timely.
Figure 1.
The structure of EMP3. The main part of EMP3 consists of four hydrophobic transmembrane structural domains, each containing 21 amino acids. The extracellular domain (ECD) consists of 41 and 18 amino acids, and the three intracellular domains consist of short cytoplasmic N- and C-terminal tails and a 13-amino acid loop. The reported variants and glycosylation modification sites have been color-coded.
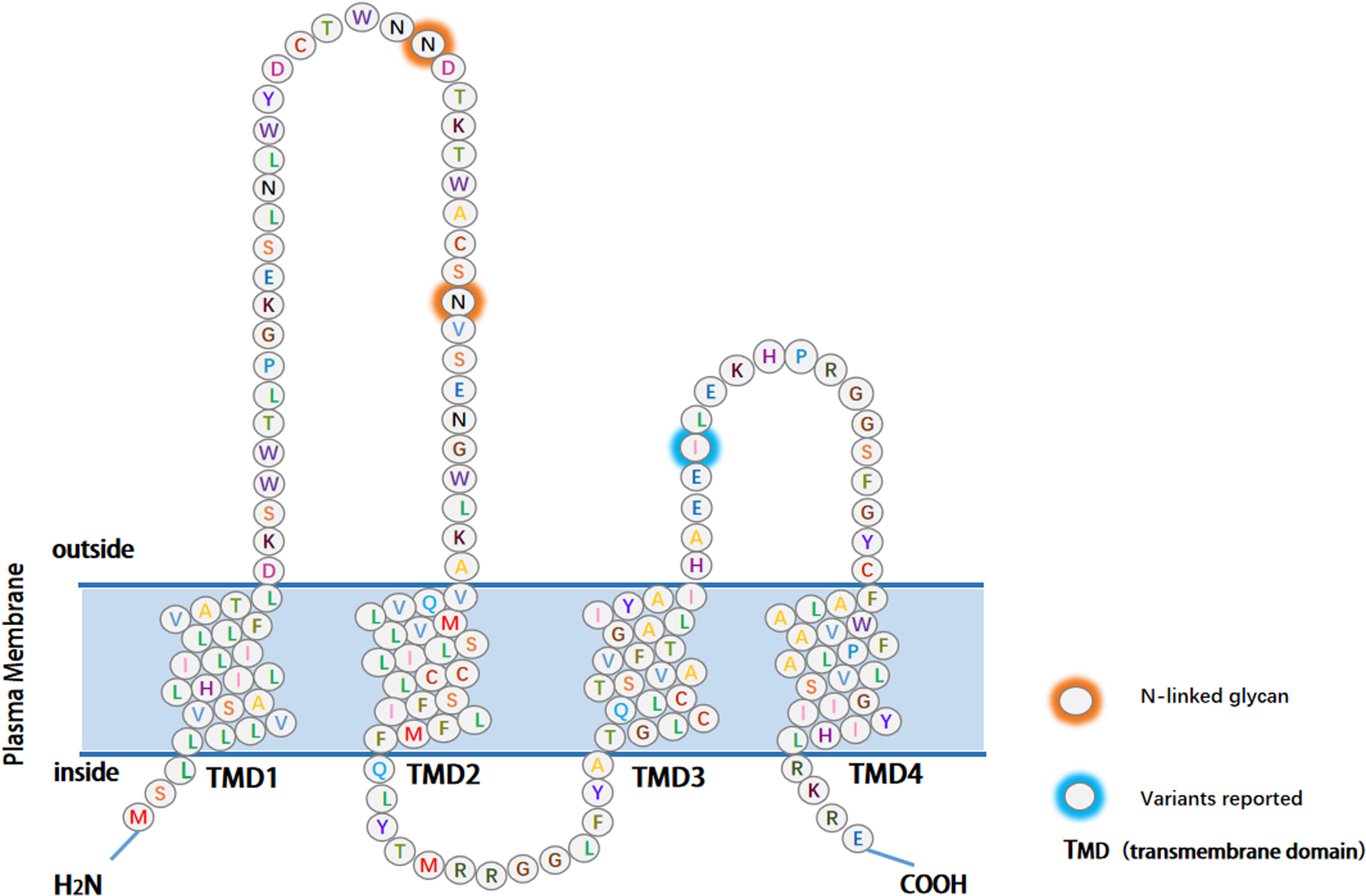
2.Structure of EMP3
The EMP3 gene is located on human chromosome 19q13.3 and mouse chromosome 7. The protein comprises 163 amino acids, encoding a hydrophobic integral membrane protein with an approximate molecular weight of 18 kDa. Its primary structural components comprise four transmembrane topological domains, two extracellular loops, and a short intracellular loop [25]. The two extracellular loops are 18 and 41 amino acids long, and the intracellular loop is composed of 13 amino acids. The N- and C-terminal domains are located within the cell [24, 26]. EMP3 is categorized as a tetraspanin membrane protein, known for its association with integrins and role in regulating integrin-mediated functions such as proliferation, survival, migration, and tumor invasion [27]. As the small size of the intracellular loop makes it unlikely to be involved in signaling pathways, the extracellular loops are free to interact with proteins and molecules, potentially holding functional significance. Post-translational modifications, including glycosylation and myristoylation, frequently occur on amino acid residues on the cell surface. Myristoylation, a lipid modification crucial for membrane targeting and signal transduction in various human tumors, is catalyzed by the enzyme N-myristoyltransferase, thereby playing a crucial role in signal transduction across different tumors [1] (Fig. 1). EMP3 contains a PKC phosphorylation site. It is well established that abnormal PKC activity is associated with cancer, as this kinase plays critical roles in cell proliferation, survival, migration, and apoptosis. The expression of the three PKC isoforms is regulated by various oncogenes such as rat sarcoma virus oncogene (RAS) and myelocytomatosis viral oncogene homolog (MYC). Different PKC isoforms mediate cell transformation, revealing its participation in cancer signaling pathways [28, 29]. Consequently, EMP3 may exert influence over the initiation and progression of tumors through its interactions with the aforementioned genes.
3.Expression of EMP3
EMP3 is expressed as both a 1.0-kb and a 1.4-kb mRNA in most tissues, with higher expression detected in the bone marrow, smooth muscle, uterus, bladder, lung, and ovary. There is lowerr expression found in tissues such as the thyroid, parathyroid gland, testis, and cerebral cortex [3]. In adult peripheral nerves, EMP3 can be detected in the axon core but not in the myelin sheath. It is also commonly expressed in Langerhans cells, monocytes, macrophages, granulocytes, and melanocytes [30, 31]. EMP3 protein is present on the surface of red blood cells and essential for the expression of the MAM blood group antigen. Individuals lacking the MAM antigen, known as MAM-negative individuals, carry inactivating mutations in the EMP3 gene. Experimental knockouts of the EMP3 gene in a human erythroid cell line resulted in the elimination of MAM antigen expression. MAM-negative erythrocytes show increased proliferation in vitro, revealing a regulatory role in erythropoiesis and cell production. Additionally, EMP3 is known to interact with and stabilize the receptor CD44 on the plasma membrane of erythrocytes. This association enables CD44 incorporation into signaling complexes such as lipid rafts [24].
4.Effect of EMP3 on immune cells and signaling pathways
4.1Effect of EMP3 on immune cells
EMP3 expression in macrophages contributes to immune suppression by increasing TNF-
Figure 2.
As a transmembrane protein, EMP3 is involved in signal transduction in cells. EMP3 activates the PI3K/Akt pathway and increases MMP, urokinase , RhoA and ROCK1/2 activities in hepatocellular carcinoma and uroepithelial carcinoma. EMP3 affects cell proliferation in endometrial and gallbladder cancers through regulation of the MAPK pathway, and in lung cancer and Glioblastoma through the EMP3/TGF
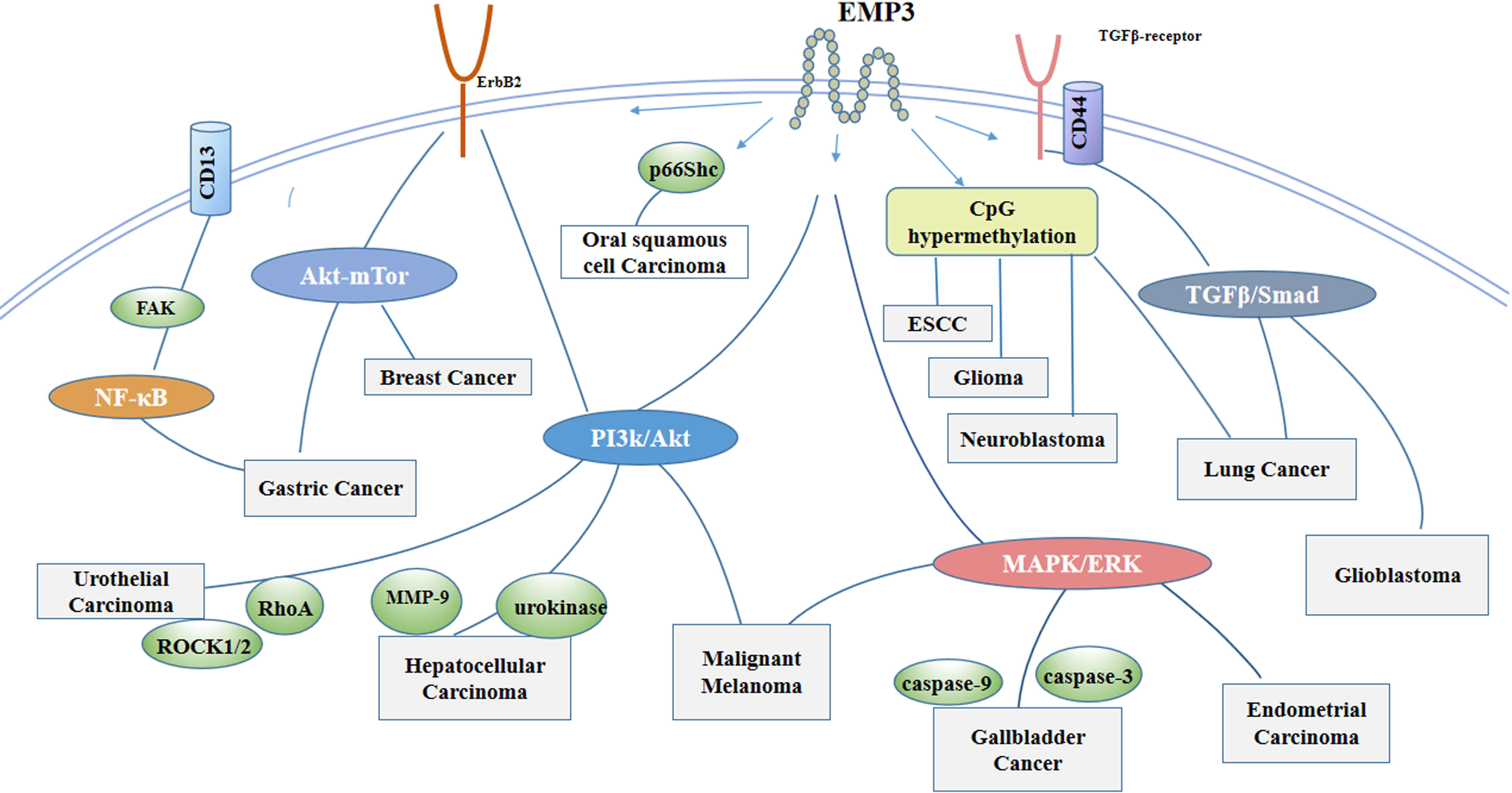
4.2Effect of EMP3 on signaling pathways
EMP3 is a transmembrane protein that can bind to various proteins within the cell membrane, including receptor tyrosine kinases, integrins, and transforming growth factor beta receptor 2 (TGF-
5.Effect of EMP3 on cell proliferation and apoptosis
EMP3 negatively correlates with a cluster of genes associated with the S phase of the cell cycle, including cyclin E2 (CCNE2), cyclin-dependent kinase 2 (CDK2), proliferating cell nuclear antigen (PCNA), replication factorC4 (RFC4), minichromosome maintenance 4 (MCM4), and GINS1 [22]. Studies suggest a significant relationship between EMP3 expression and the tumor cell cycle. Downregulation of EMP3 has been linked to decreased levels of phosphorylated Akt (p-Akt), phosphorylated epidermal growth factor receptor (p-EGFR), and p-ERK. Additionally, the absence of EMP3 expression decreases TGF-
6.EMP3 and cancers
6.1EMP3 and nervous system cancers
Frequent genomic deletions are observed in the 19q13 chromosomal region of neuroblastoma and glioma tumor samples, suggesting the presence of tumor suppressor genes in this region. Loss of heterozygosity at the EMP3 gene locus on the long arm of chromosome 19 is associated with higher rates of recurrence and mortality in patients with oligodendroglioma [20, 49]. Studies have shown that EMP3 is subject to hypermethylation in approximately 20%–40% of neuroblastoma and glioma cases. Upon demethylation, EMP3 expression is restored in neuroblasts, resulting in a decreased ability of tumor cells to form colonies. This underscores EMP3’s role in tumor suppression. Hypermethylation of EMP3 serves as an adverse prognostic marker for neuroblastoma patients [20]. Notably, the methylation frequency of EMP3 in neuroblastoma is higher than in pheochromocytoma [50]. In GBM, EMP3 is overexpressed in the primary neoplasm, especially in cases characterized by high CD44 levels, when compared to non-neoplastic white matter tissue. Inhibition of EMP3 expression suppresses tumor cell proliferation, reduces tumorigenic potential in vitro, and induces cell apoptosis. EMP3 in GBM plays a significant role in TGF-
6.2EMP3 and breast cancer
Elevated levels of EMP3 mRNA and protein are detected in primary breast carcinoma tissues, accompanied by a concomitant decrease in microRNA-765 (miR-765) levels. EMP3 is a target of miRNA-765, and its impact on breast cancer outcomes involves attenuating tumor proliferation and promoting apoptosis. Given its association with disease aggressiveness, EMP3 may play a tumor-oncogenic role in breast cancer [42, 60, 61]. Notably, EMP3 overexpression in primary breast carcinomas is strongly correlated with histological grade III, lymph node metastasis, and high HER-2 expression [62]. Transcriptomic studies in breast cancer have revealed that changes in the expression of HER-2-related signaling proteins impact MYC signaling, among which are EMP1 and EMP3. In cell lines with overexpression of HER-2, both MYC and EMP3 are upregulated, suggesting a potential interaction between EMP3 and MYC, further implicating EMP3 as an oncogene in HER-2 positive cancers [63]. EMP3 expression is notably higher in HER-2 positive breast cancers and may serve as a potential therapeutic target [64]. On the other hand, in the context of high histological grade phyllodes tumors, the expression of EMP3 across tissue is variable, with decreased expression in the epithelial component and increased expression in the stromal component. Stromal EMP3 expression may serve as an independent prognostic factor in rare breast cancers [65]. Other studies [22, 66] have shown that EMP3 inhibits breast cancer cells from entering the S phase of the cell cycle, preventing DNA replication and proliferation. EMP3 negatively regulates breast cancer susceptibility gene (BRCA1) and restriction association site DNA 51(RAD51), preventing homologous recombination repair of double-strand DNA breaks. Furthermore, EMP3 has been found to reduce resistance to chemotherapy, suppress stem cell-like properties, and block Akt/mTOR signaling activation. These findings suggest that EMP3’s action as a breast cancer tumor suppressor, enhancing sensitivity of breast cancer to chemotherapy. Depletion of EMP3 has been found to enhance organoid growth.
6.3EMP3 and lung cancer
In the context of non-small-cell lung cancer, studies consistently reveal decreased EMP3 expression levels in neoplastic tissue compared to normal lung tissue. Similarly, EMP3 is negatively correlated with TNM staging and the proliferation marker Ki67. Lung cancer patients with lower EMP3 expression levels tend to experience poorer postoperative recurrence-free survival rates. In vitro experiments have provided further insights, demonstrating that EMP3 expression can be restored in lung cancer A549 cells following their release from serum starvation. This observation suggest that EMP3 may function as a tumor suppressor gene, particularly in advanced-stage lung cancer [67]. Another study observed upregulated EMP3 expression is upregulated in non-small-cell lung cancer cells overexpressing aldehyde dehydrogenase 1. EMP3 activates the downstream TGF-
6.4EMP3 and digestive system cancers
EMP3 exhibits elevated expression levels in human oral squamous cancer. Recent research has revealed that a high dose of TNF-
6.5EMP3 and genitourinary system cancers
EMP3 is expressed in the human embryonic kidney cell line HEK-293. In clear cell renal cell carcinoma (ccRCC), EMP3 functions as an oncogene and is notably enriched in MYC Target V2 signaling pathways. It is implicated in promoting EMT, inhibiting of fatty acid degradation, and enhancing the malignant potential of tumor cells. Furthermore, EMP3 strongly correlates with the degree of immune infiltration in ccRCC. EMP3 holds promise as a biomarker for diagnosing ccRCC and predicting patient survival [73]. In the context of urothelial carcinoma, EMP3 may also function as an oncogene, as RNA levels closely correlate with the onset and progression of tumorigenesis. Co-expression of HER-2 and EMP3 emerges as a critical indicator of progression-free and metastasis-free survival in patients [1, 37]. Furthermore, EMP3 plays a pivotal role in the development of endometrial cancer. Its differential expression in endometrial cancer affects various cellular processes, including RNA splicing, noncoding RNA regulation, covalent chromatin modification, histone modification, and organelle fission. These alterations significantly impact several pathways, particularly the MAPK signaling pathway [74]. Experimental studies have shown that the inoculation of nude mice with EMP1 overexpressing prostate cells leads to lymph nodes and lung metastases. Similar evidence points to EMP3 playing a comparable role in prostate cancer, although further research is needed [3, 75, 76].
6.6EMP3 and other cancers
In melanoma C32 cells, the knockdown of the EMP3 gene resulted in reduced Akt phosphorylation and a subsequent decrease in cell viability, indicating EMP3’s involvement in cell survival pathways. The influence on signaling pathways, such as EGFR/Akt and MAPK, appears to be tumor-specific, suggesting a diverse role in tumorigenesis. EMP3 is implicated in both inflammation and pyroptosis processes in melanoma [77]. Conversely, uveal melanoma cells show an upregulation of EMP3 expression, while in cutaneous melanoma patients, EMP3 levels are closely correlated with survival time, making it a potential therapeutic target under investigation [34, 78, 79]. Furthermore, semi-quantitative RT-PCR experiments have found that retinoic acid-induced differentiation of F9 teratocarcinoma stem cells upregulated EMP3 expression, highlighting its role in cellular differentiation processes [80]. In acute myeloid leukemia experiments, EMP3 is linked to cell migration and adhesion and was found to be markedly expressed in CD34 + CD117dim blasts [81]. Looking at neuroblastoma and pheochromocytoma, there are differences in epigenetic modifications of the EMP3 gene, despite overlapping regions of allelic loss and tumor suppressor gene methylation in the two conditions. Methylation of EMP3 is frequent in neuroblastoma but rare in pheochromocytoma [50]. In thyroid cancers, EMP3 expression varies among subtypes, with higher expression observed in papillary carcinomas with BRAF V600E mutations [82].
Table 1
Published studies on the relationship between EMP3 and cancer
Cancer | Related pathways, proteins, gene or cytokine | Related changes | Role of EMP3 in cancer | Refs |
---|---|---|---|---|
Glioblastoma | CCL2, TGF- | Angiogenesis activated, Reducing CD4 + T-cells, CD4 + T-cells deficient | Oncogenic | [32] |
TGF- | CD44-high GBM cell proliferation | Oncogenic | [36] | |
Glioma | EMP3 associated promoter CpG island hypermethylation | Transcription inactivation of tumor suppressor gene | Anti-oncogenic | [20] |
Breast cancer | BRCA1,RAD51,Akt-mTOR | Inducing cellular autophagy | Anti-oncogenic | [22] |
Primary breast carcinoma | miR-765 | Enhancing cellular proliferation and invasion | Oncogenic | [42] |
Lung cancer | TGF- | Enhancing cellular migration and invasion | Oncogenic | [40] |
Non-small cell lung cancer | EMP3 associated promoter CpG island hypermethylation | Reducing expression of ki67 and cell proliferation | Anti-oncogenic | [67] |
Oral squamous cancer | miR-765, P66Shc | Inhibiting cellular migration | Anti-oncogenic | [68] |
Esophageal squamous cell carcinoma | EMP3 associated promoter CpG island hypermethylation | Inducing cellular growth inhibition and apoptosis | Anti-oncogenic | [69] |
Gastric cancer | CD13/EMP3/FAK/NF- | Enhancing cellular invasion | Oncogenic | [71] |
Hepatocellular carcinoma | P13K/Akt, uPA/MMP-9 | Enhancing cellular migration and invasion | Oncogenic | [38] |
Gallbladder cancer | MAPK/ERK | Inducing cellular apoptosis | Anti-oncogenic | [39] |
Clear cell renal cell carcinoma | Myc Targets V2 | Enhancing cellular migration, invasion and lipid storage | Oncogenic | [73] |
Urothelial carcinoma | HER-2/ P13K/Akt,FAK/Src | Promoting cellular growth migration | Oncogenic | [37] |
Endometrial cancer | MAPK, Rap1 | Promoting cellular proliferation and division | Oncogenic | [74] |
Melanoma | EGFR/Akt, MAPK | Reducing CD4 + T-cells, CD4 + T-cells deficient | Oncogenic | [77][34] |
Overall, EMP3 affects cancer through different signaling pathways, cytokines, and proteins, leading to changes in cancer cell proliferation, migration, and apoptosis. These findings are summarized in Table 1.
7.EMP3 and cancer therapy
EMP3 is strongly involved in cancer development and progression, playing different roles in promoting or inhibiting cancer growth across different diseases. While it may act as a tumor-suppressive protein in esophageal squamous cell carcinoma and gallbladder cancer, EMP3 functions as a tumor promotor in endometrial cancer and hepatocellular carcinoma. The function of EMP3 is still controversial in many cancers. In summary, EMP3 is involved in several pathways: methylation of dependent tumor suppressors, interference with the MAPK/ERK axis affects tumor progression, action as an oncogene by regulating the HER-2 pathway, activation of the PI3K/Akt signaling pathway; regulation of TGF-
There are limited reports on EMP3 and tumor therapy, however, some studies have noted EMP the relationship between EMP3 and drug resistance. EMP3 can act downstream of CD13 and regulate the PI3K/Akt pathway, hereby promoting EMT and cisplatin resistance in gastric cancer cells [71]. Similarly, in primary gastric cancer, EMP3 can function as a novel downstream effector target of TWIST1/2 and as a modifier gene involved in cisplatin resistance [70]. EMP3 modulates a variety of membrane receptor activities associated in IDH-WT GBM, including increasing GBM-associated receptor tyrosine kinase activity and promoting the expression, stability, or activity of several extracellular matrix receptors and matrix degraders. In addition, EMP3 interacts with purinergic receptor P2RX7, which has received increasing attention for its role in GBM. EMP3 regulates the trafficking and enhancing the stability of EGF, and EMP3 deletion renders tumor cells more sensitive to the pan-kinase inhibitor staurosporine and osimertinib, an EGFR inhibitor. The stabilizing effect of EMP3 provides tumor cells with an additional defense barrier against targeted kinase inhibition. Therefore, EMP3 may be an interesting target for therapeutic intervention [53]. In primary breast cancer, studies found the downregulation of EMP3 by miR-765 mimics or RNA-EMP3 resulted in inhibition of homologous recombination repair of DNA double-strand breaks through negative regulation of BRCA1 and RAD51 expression. Consequently, this led to a reduction in the resistance of breast cancer cells to DNA-damaging drug Adriamycin [22, 42]. As previously described, patients with overexpression of EMP3 in upper urinary tract urothelial carcinoma exhibited significantly poorer overall survival and metastasis-free survival [37]. This suggests that EMP3 serves as an important prognostic marker for patients who may benefit from more aggressive therapy. In addition, the co-expression of EMP3 and HER-2 is one of the most important indicators for progression-free and metastasis-free survival of patients, as there is a functional interaction between the two proteins. Therefore, EMP3 can be used as an innovative target for targeted therapy in cancer treatment.
The development of monoclonal antibodies targeting EMP family members is challenging due to the clinical and pathologic heterogeneity and biological complexity of cancers. Some progress has been made in the research and development of anti-EMP monoclonal antibodies. Research shows that anti-EMP2 diabody treatment demonstrated significant antiproliferative effects through enhancing caspase-3-mediated apoptosis in endometrial adenocarcinoma cell lines. It proved successful in reducing tumor size and promoting cell death in human endometrial cancer xenografts [85]. Anti-EMP2 IgG1 improves endometrial cancer survival through reduction in cancer stem cells [86]. In a model of transplanted ovarian cancer tumors, anti-EMP2 bivalent antibody therapy reduced the proliferation of ovarian cancer cells and promoted apoptosis [87]. In addition, treatment of breast cancer with anti-EMP2 IgG1 promoted tumor cell death and inhibited tumor cell invasion. In a mouse model of breast cancer, anti-EMP2 treatment reduced the number of spontaneous metastases from xenografts expressing high levels of EMP2 [88]. So far, there has been no report on the use of anti-EMP3 in clinical trials. EMP3 is of great significance in tumor drug resistance and evaluation of disease prognosis. Therefore, it is necessary to further study the specific role of EMP3 and its application value in the clinical setting.
8.Conclusion and future perspective
Rapid advancements in genomics research have catalyzed the emergence of molecular targeted therapy as a promising avenue for cancer treatment and facilitated the discovery of numerous novel tumor biomarkers. Among these proteins, EMP3, a member of the epithelial membrane protein family, has shown expression across various tissues and appears to regulate signaling pathways related to cell proliferation and cell–cell interactions. A notable advantage of using EMP3 as a potential drug target is its location on the cell membrane and its involvement in diverse signaling pathways, making it physically accessible for drug targeting. However, the precise biological function of EMP3 remains incompletely understood. Studies suggest that the protein acts as an oncogene in several tumors while suppressor function in others, highlighting the complex nature of EMP3-mediated pathways in tumorigenesis. Further research is necessary to understand the patterns of EMP3 expression and activity. Ultimately, EMP3 holds promise as a potential prognostic indicator across various cancers. There is a need to further elucidate the potential associations between EMP3 expression and tumor immunity. Therefore, it is crucial to further investigate the interplay between EMP3-mediated immune dysregulation and tumor progression, with a focus on understanding the underlying molecular mechanisms.
Acknowledgments
This works was supported by the Scientific Development Program of Jilin Province, No.20230402008GH.
Author contributions
Conception: Wenjing Zhu.
Preparation of the manuscript: Wenjing Zhu, Shu Song.
Revision for important intellectual content: Yangchun Xu, Hanyue Sheng.
Supervision: Shuang Wang.
References
[1] | Y.-W. Wang, H.-L. Cheng, Y.-R. Ding, L.-H. Chou and N.-H. Chow, EMP1, EMP 2, and EMP3 as novel therapeutic targets in human cancer, Biochim Biophys Acta (BBA Rev Cancer 1868: ((2017) ), 199–211. |
[2] | I. Ben-Porath, C.A. Kozak and N. Benvenisty, Chromosomal Mapping of Tmp(Emp1), Xmp(Emp2), and Ymp(Emp3), Genes Encoding Membrane Proteins Related to Pmp22, Genomics 49: ((1998) ), 443–447. |
[3] | A. Amin, Shimizu and Ogita, The Pivotal Roles of the Epithelial Membrane Protein Family in Cancer Invasiveness and Metastasis, Cancers 11: ((2019) ), 1620. |
[4] | V. Taylor and U. Suter, Epithelial membrane protein-2 and epithelial membrane protein-3: two novel members of the peripheral myelin protein 22 gene family, Gene 175: ((1996) ), 115–120. |
[5] | A. Cañellas-Socias, C. Cortina, X. Hernando-Momblona, S. Palomo-Ponce, E.J. Mulholland, G. Turon, L. Mateo, S. Conti, O. Roman, M. Sevillano, F. Slebe, D. Stork, A. Caballé-Mestres, A. Berenguer-Llergo, A. Álvarez-Varela, N. Fenderico, L. Novellasdemunt, L. Jiménez-Gracia, T. Sipka, L. Bardia, P. Lorden, J. Colombelli, H. Heyn, X. Trepat, S. Tejpar, E. Sancho, D.V.F. Tauriello, S. Leedham, C.S.-O. Attolini and E. Batlle, Metastatic recurrence in colorectal cancer arises from residual EMP1 cells, Nature 611: ((2022) ), 603–613. |
[6] | G.G. Sun, Y.D. Wang, D.W. Cui, Y.J. Cheng and W.N. Hu, EMP1 regulates caspase-9 and VEGFC expression and suppresses prostate cancer cell proliferation and invasion, Tumor Biol 35: ((2013) ), 3455–3462. |
[7] | H. Li, X. Zhang, X. Jiang and X. Ji, The expression and function of epithelial membrane protein 1 in laryngeal carcinoma, International Journal of Oncology 50: ((2016) ), 141–148. |
[8] | H.-T. Wang, Analysis of gene expression profile induced by EMP-1 in esophageal cancer cells using cDNA Microarray, WJG 9: ((2003) ), 392. |
[9] | Z. Abdalla, T. Walsh, N. Thakker and C.M. Ward, Loss of epithelial markers is an early event in oral dysplasia and is observed within the safety margin of dysplastic and T1 OSCC biopsies, PLoS ONE 12: ((2017) ), e0187449. |
[10] | G.G. Sun, Y.F. Lu, Z.Z. Fu, Y.J. Cheng and W.N. Hu, EMP1 inhibits nasopharyngeal cancer cell growth and metastasis through induction apoptosis and angiogenesis, Tumor Biol 35: ((2013) ), 3185–3193. |
[11] | G. Sun, G. Zhao, Y. Lu, Y. Wang and C. Yang, Association of EMP1 with gastric carcinoma invasion, survival and prognosis, International Journal of Oncology 45: ((2014) ), 1091–1098. |
[12] | Q. Chen, L. Yao, D. Burner, B. Minev, L. Lu, M. Wang and W. Ma, Epithelial membrane protein 2: a novel biomarker for circulating tumor cell recovery in breast cancer, Clin Transl Oncol 21: ((2018) ), 433–442. |
[13] | Y. Qin, M. Fu, M. Takahashi, A. Iwanami, D. Kuga, R.G. Rao, D. Sudhakar, T. Huang, M. Kiyohara, K. Torres, C. Dillard, A. Inagaki, N. Kasahara, L. Goodglick, J. Braun, P.S. Mischel, L.K. Gordon and M. Wadehra, Epithelial Membrane Protein-2 (EMP2) Activates Src Protein and Is a Novel Therapeutic Target for Glioblastoma, J Biol Chem 289: ((2014) ), 13974–13985. |
[14] | Y.-W. Wang, W.-M. Li, W.-J. Wu, C.-Y. Chai, T.-Y. Chang, Y. Sun, C.-J. Cheng, Y.-L. Shiue, S.-J. Su, H.-L. Cheng, H.-S. Liu and N.-H. Chow, Epithelial Membrane Protein 2 Is a Prognostic Indictor for Patients with Urothelial Carcinoma of the Upper Urinary Tract, Am J Pathol 183: ((2013) ), 709–719. |
[15] | D. Tong, G. Heinze, D. Pils, A. Wolf, C.F. Singer, N. Concin, G. Hofstetter, I. Schiebel, M. Rudas and R. Zeillinger, Gene expression of PMP22 is an independent prognostic factor for disease-free and overall survival in breast cancer patients, BMC Cancer 10: ((2010) ), 682. |
[16] | M. van Dartel and T.J.M. Hulsebos, Characterization of PMP22 expression in osteosarcoma, Cancer Genet Cytogenet 152: ((2004) ), 113–118. |
[17] | N. Moniaux, C. Nemos, B.M. Schmied, S.C. Chauhan, S. Deb, K. Morikane, A. Choudhury, M. VanLith, M. Sutherlin, J.M. Sikela, M.A. Hollingsworth and S.K. Batra, The human homologue of the RNA polymerase II-associated factor 1 (hPaf1), localized on the 19q13 amplicon, is associated with tumorigenesis, Oncogene 25: ((2006) ), 3247–3257. |
[18] | X. Deng, S. Li, A. Naqvi and E. Friedman, THE KINASE MIRK/DYRK1B MEDIATES CELL SURVIVAL IN PANCREATIC DUCTAL ADENOCARCINOMA, Pancreas 31: ((2005) ), 438. |
[19] | R. Kuuselo, K. Savinainen, D.O. Azorsa, G.D. Basu, R. Karhu, S. Tuzmen, S. Mousses and A. Kallioniemi, Intersex-like (IXL) Is a Cell Survival Regulator in Pancreatic Cancer with 19q13 Amplification, Cancer Res 67: ((2007) ), 1943–1949. |
[20] | M. Alaminos, V. Dávalos, S. Ropero, F. Setién, M.F. Paz, M. Herranz, M.F. Fraga, J. Mora, N.-K.V. Cheung, W.L. Gerald and M. Esteller, EMP3, a Myelin-Related Gene Located in the Critical 19q13.3 Region, Is Epigenetically Silenced and Exhibits Features of a Candidate Tumor Suppressor in Glioma and Neuroblastoma, Cancer Res 65: ((2005) ), 2565–2571. |
[21] | O. Gluz, C. Kolberg-Liedtke, A. Prat, M. Christgen, D. Gebauer, R. Kates, L. Paré, E. Grischke, H. Forstbauer, M. Braun, M. Warm, J. Hackmann, C. Uleer, B. Aktas, C. Schumacher, S. Kuemmel, R. Wuerstlein, E. Pelz, U. Nitz, H.H. Kreipe and N. Harbeck, Efficacy of deescalated chemotherapy according to PAM50 subtypes, immune and proliferation genes in triple-negative early breast cancer: Primary translational analysis of the WSG-ADAPT-TN trial, Int J Cancer 146: ((2019) ), 262–271. |
[22] | K. Zhou, Y. Sun, D. Dong, C. Zhao and W. Wang, EMP3 negatively modulates breast cancer cell DNA replication, DNA damage repair, and stem-like properties, Cell Death Dis 12: ((2021) ), 844. |
[23] | Y. Kusumoto, H. Okuyama, T. Shibata, K. Konno, Y. Takemoto, D. Maekawa, T. Kononaga, T. Ishii, S. Akashi-Takamura, S. Saitoh, R. Ikebuchi, T. Moriya, M. Ueda, K. Miyake, S. Ono and M. Tomura, Epithelial membrane protein 3 (Emp3) downregulates induction and function of cytotoxic T lymphocytes by macrophages via TNF-α production, Cell Immunol 324: ((2018) ), 33–41. |
[24] | N. Thornton, V. Karamatic Crew, L. Tilley, C.A. Green, C.L. Tay, R.E. Griffiths, B.K. Singleton, F. Spring, P. Walser, A.G. Alattar, B. Jones, R. Laundy, J.R. Storry, M. Möller, L. Wall, R. Charlewood, C.M. Westhoff, C. Lomas-Francis, V. Yahalom, U. Feick, A. Seltsam, B. Mayer, M.L. Olsson and D.J. Anstee, Disruption of the tumour-associated EMP3 enhances erythroid proliferation and causes the MAM-negative phenotype, Nat Commun 11: ((2020) ), 3569. |
[25] | N. Ashki, L. Gordon and M. Wadehra, Review of the GAS3 Family of Proteins and their Relevance to Cancer, Crit Rev Oncog 20: ((2015) ), 435–447. |
[26] | T. Liehr, G. Kuhlenbäumer, P. Wulf, V. Taylor, U. Suter, C. Van Broeckhoven, J.R. Lupski, U. Claussen and B. Rautenstrauss, Regional Localization of the Human Epithelial Membrane Protein Genes 1, 2, and 3 (EMP1, EMP2, EMP3) to 12p12.3, 16p13.2, and 19q13.3, Genomics 58: ((1999) ), 106–108. |
[27] | S.A. Morales, D.G. Telander, S. Mareninov, A. Nagy, M. Wadehra, J. Braun and L.K. Gordon, Anti-EMP2 diabody blocks epithelial membrane protein 2 (EMP2) and FAK mediated collagen gel contraction in ARPE-19 cells, Exp Eye Res 102: ((2012) ), 10–16. |
[28] | I.B. Weinstein, Nonmutagenic mechanisms in carcinogenesis: role of protein kinase C in signal transduction and growth control, Environ Health Perspect 93: ((1991) ), 175–179. |
[29] | C. Borner, S.N. Guadagno, W.W. Hsiao, D. Fabbro, M. Barr and I.B. Weinstein, Expression of four protein kinase C isoforms in rat fibroblasts. Differential alterations in ras-, src-, and fos-transformed cells, J Biol Chem 267: ((1992) ), 12900–12910. |
[30] | F.O. Bagger, D. Sasivarevic, S.H. Sohi, L.G. Laursen, S. Pundhir, C.K. Sønderby, O. Winther, N. Rapin and B.T. Porse, BloodSpot: a database of gene expression profiles and transcriptional programs for healthy and malignant haematopoiesis, Nucleic Acids Res 44: ((2015) ), D917–D924. |
[31] | A.M. Jetten and U. Suteri, The peripheral myelin protein 22 and epithelial membrane protein family, in: Progress in Nucleic Acid Research and Molecular Biology, Elsevier, (2000) , pp. 97–129. |
[32] | Q. Chen, J. Jin, X. Huang, F. Wu, H. Huang and R. Zhan, EMP3 mediates glioblastoma-associated macrophage infiltration to drive T cell exclusion, J Exp Clin Cancer Res 40: ((2021) ), 160. |
[33] | J. Li, Q. Guo and R. Xing, Construction and validation of an immune infiltration-related risk model for predicting prognosis and immunotherapy response in low grade glioma, BMC Cancer 23: ((2023) ), 727. |
[34] | A. Christians, E. Poisel, C. Hartmann, A. Deimling and S. Pusch, Characterization of the epithelial membrane protein 3 interaction network reveals a potential functional link to mitogenic signal transduction regulation, Int J Cancer 145: ((2019) ), 461–473. |
[35] | C.Y. Lin, C.H. Lee, Y.H. Chuang, J.Y. Lee, Y.Y. Chiu, Y.H. Wu Lee, Y.J. Jong, J.K. Hwang, S.H. Huang, L.C. Chen, C.H. Wu, S.H. Tu, Y.S. Ho and J.M. Yang, Membrane protein-regulated networks across human cancers, Nat Commun 10: ((2019) ), 3131. |
[36] | F. Jun, J. Hong, Q. Liu, Y. Guo, Y. Liao, J. Huang, S. Wen and L. Shen, Epithelial membrane protein 3 regulates TGF-β signaling activation in CD44-high glioblastoma, Oncotarget 8: ((2016) ), 14343–14358. |
[37] | Y.-W. Wang, W.-M. Li, W.-J. Wu, C.-Y. Chai, H.-S. Liu, M.-D. Lai and N.-H. Chow, Potential Significance of EMP3 in Patients with Upper Urinary Tract Urothelial Carcinoma: Crosstalk with ErbB2-PI3K-Akt Pathway, J Urol 192: ((2014) ), 242–251. |
[38] | Y.-H. Hsieh, S.-C. Hsieh, C.-H. Lee, S.-F. Yang, C.-W. Cheng, M.-J. Tang, C.-L. Lin, C.-L. Lin and R.-H. Chou, Targeting EMP3 suppresses proliferation and invasion of hepatocellular carcinoma cells through inactivation of PI3K/Akt pathway, Oncotarget 6: ((2015) ), 34859–34874. |
[39] | Q. Ma, Y. Zhang, H. Liang, F. Zhang, F. Liu, S. Chen, Y. Hu, L. Jiang, Y. Hao, M. Li and Y. Liu, EMP3 as a key downstream target of miR-663a regulation interferes with MAPK/ERK signaling pathway to inhibit gallbladder cancer progression, Cancer Lett 575: ((2023) ), 216398. |
[40] | Y.-J. Kahm, R.-K. Kim, U. Jung and I.-G. Kim, Epithelial membrane protein 3 regulates lung cancer stem cells via the TGFβ signaling pathway, Int J Oncol 59: ((2021) ), 80. |
[41] | A.W. Lambert, D.R. Pattabiraman and R.A. Weinberg, Emerging Biological Principles of Metastasis, Cell 168: ((2017) ), 670–691. |
[42] | X.C. Hong, Y.J. Fen, G.C. Yan, H. Hong, C.H. Yan, L.W. Bing and Y.H. Zhong, Epithelial membrane protein 3 functions as an oncogene and is regulated by microRNA-765 in primary breast carcinoma, Mol Med Report 12: ((2015) ), 6445–6450. |
[43] | A. Karimian, S.M. Mir, H. Parsian, S. Refieyan, M. Mirza-Aghazadeh-Attari, B. Yousefi and M. Majidinia, Crosstalk between Phosphoinositide 3-kinase/Akt signaling pathway with DNA damage response and oxidative stress in cancer, J Cell Biochem 120: ((2018) ), 10248–10272. |
[44] | F. Villafañez, I.A. García, S. Carbajosa, M.F. Pansa, S. Mansilla, M.C. Llorens, V. Angiolini, L. Guantay, H. Jacobs, K.P. Madauss, I. Gloger, V. Gottifredi, J.L. Bocco and G. Soria, AKT inhibition impairs PCNA ubiquitylation and triggers synthetic lethality in homologous recombination-deficient cells submitted to replication stress, Oncogene 38: ((2019) ), 4310–4324. |
[45] | K. Matsumoto, T. Arao, K. Tanaka, H. Kaneda, K. Kudo, Y. Fujita, D. Tamura, K. Aomatsu, T. Tamura, Y. Yamada, N. Saijo and K. Nishio, mTOR Signal and Hypoxia-Inducible Factor-α Regulate CD133 Expression in Cancer Cells, Cancer Res 69: ((2009) ), 7160–7164. |
[46] | J. Sunayama, K.-I. Matsuda, A. Sato, K. Tachibana, K. Suzuki, Y. Narita, S. Shibui, K. Sakurada, T. Kayama, A. Tomiyama and C. Kitanaka, Crosstalk Between the PI3K/mTOR and MEK/ERK Pathways Involved in the Maintenance of Self-Renewal and Tumorigenicity of Glioblastoma Stem-Like Cells, Stem Cells 28: ((2010) ), 1930–1939. |
[47] | L.M. Bolin, T. McNeil, L.A. Lucian, B. DeVaux, K. Franz-Bacon, D.M. Gorman, S. Zurawski, R. Murray and T.K. McClanahan, HNMP-1: A Novel Hematopoietic and Neural Membrane Protein Differentially Regulated in Neural Development and Injury, J Neurosci 17: ((1997) ), 5493–5502. |
[48] | H.L. Wilson, S.A. Wilson, A. Surprenant and R.A. North, Epithelial Membrane Proteins Induce Membrane Blebbing and Interact with the P2X Receptor C Terminus, J Biol Chem 277: ((2002) ), 34017–34023. |
[49] | A. Pasini, P. Iorio, E. Bianchi, S. Cerasoli, A.M. Cremonini, M. Faedi, C. Guarnieri, G. GUiducci, L. Ricioni, C. Molinari, C. Rengucci, D. Calistri and E. GiordanoI, LOH 19q indicates shorter disease progression-free interval in low-grade oligodendrogliomas with EMP3 methylation, Oncol Rep 28: ((2012) ), 2271–2277. |
[50] | C.D.E. Margetts, M. Morris, D. Astuti, D.C. Gentle, A. Cascon, F.E. McRonald, D. Catchpoole, M. Robledo, H.P.H. Neumann, F. Latif and E.R. Maher, Evaluation of a functional epigenetic approach to identify promoter region methylation in phaeochromocytoma and neuroblastoma, Endocr Relat Cancer 15: ((2008) ), 777–786. |
[51] | C.A. Scrideli, C.G. Carlotti, O.K. Okamoto, V.S. Andrade, M.A.A. Cortez, F.J.N. Motta, A.K. Lucio-Eterovic, L. Neder, S. Rosemberg, S.M. Oba-Shinjo, S.K.N. Marie and L.G. Tone, Gene expression profile analysis of primary glioblastomas and non-neoplastic brain tissue: identification of potential target genes by oligonucleotide microarray and real-time quantitative PCR, J Neurooncol 88: ((2008) ), 281–291. |
[52] | L. Li, S. Xia, Z. Zhao, L. Deng, H. Wang, D. Yang, Y. Hu, J. Ji, D. Huang and T. Xin, EMP3 as a prognostic biomarker correlates with EMT in GBM, BMC Cancer 24: ((2024) ), 89. |
[53] | A.A. Martija and S. Pusch, The Multifunctional Role of EMP3 in the Regulation of Membrane Receptors Associated with IDH-Wild-Type Glioblastoma, IJMS 22: ((2021) ), 5261. |
[54] | A. Ernst, S. Hofmann, R. Ahmadi, N. Becker, A. Korshunov, F. Engel, C. Hartmann, J. Felsberg, M. Sabel, H. Peterziel, M. Durchdewald, J. Hess, S. Barbus, B. Campos, A. Starzinski-Powitz, A. Unterberg, G. Reifenberger, P. Lichter, C. Herold-Mende and B. Radlwimmer, Genomic and Expression Profiling of Glioblastoma Stem Cell – Like Spheroid Cultures Identifies Novel Tumor-Relevant Genes Associated with Survival, Clin Cancer Res 15: ((2009) ), 6541–6550. |
[55] | A. Kunitz, M. Wolter, J. van den Boom, J. Felsberg, B. Tews, M. Hahn, A. Benner, M. Sabel, P. Lichter, G. Reifenberger, A. von Deimling and C. Hartmann, DNA hypermethylation and Aberrant Expression of the EMP3 Gene at 19q13.3 in Human Gliomas, Brain Pathol 17: ((2007) ), 363–370. |
[56] | W.J. Zeng, Y.L. Yang, Z.Z. Liu, Z.P. Wen, Y.H. Chen, X.L. Hu, Q. Cheng, J. Xiao, J. Zhao and X.P. Chen, Integrative Analysis of DNA Methylation and Gene Expression Identify a Three-Gene Signature for Predicting Prognosis in Lower-Grade Gliomas, Cell Physiol Biochem 47: ((2018) ), 428–439. |
[57] | J. Dong, F. Wang, X. Gao, H. Zhao, J. Zhang, N. Wang, Z. Liu, X. Yan, J. Jin, Y. Ba, S. Ma, J. Du, H. Ji and S. Hu, Integrated analysis of genome-wide DNA methylation and cancer-associated fibroblasts identified prognostic biomarkers and immune checkpoint blockade in lower grade gliomas, Front Oncol 12: ((2023) ), 977251. |
[58] | Y.F. Gao, T. Zhu, C.X. Mao, Z.X. Liu, Z.B. Wang, X.Y. Mao, L. Li, J.Y. Yin, H.H. Zhou and Z.Q. Liu, PPIC, EMP3 and CHI3L1 Are Novel Prognostic Markers for High Grade Glioma, IJMS 17: ((2016) ), 1808. |
[59] | C. Shu, Q. Wang, X. Yan and J. Wang, Whole-Genome Expression Microarray Combined with Machine Learning to Identify Prognostic Biomarkers for High-Grade Glioma, J Mol Neurosci 64: ((2018) ), 491–500. |
[60] | S. Li, H. Peng and J. Li, MiR-765 Regulates Breast Cancer Cell Proliferation, Apoptosis, and Adriamycin Resistance Through Targeting Epithelial Membrane Protein 3, J Biomater Tissue Eng 10: ((2020) ), 1193–1198. |
[61] | V. Evtimova, R. Zeillinger and U.H. Weidle, Identification of Genes Associated with the Invasive Status of Human Mammary Carcinoma Cell Lines by Transcriptional Profiling, Tumor Biol 24: ((2003) ), 189–198. |
[62] | W. Zhou, Z. Jiang, X. Li, F. Xu, Y. Liu, P. Wen, L. Kong, M. Hou and J. Yu, EMP3 Overexpression in Primary Breast Carcinomas is not Associated with Epigenetic Aberrations, J Korean Med Sci 24: ((2009) ), 97–103. |
[63] | A. Mackay, C. Jones, T. Dexter, R.L.A. Silva, K. Bulmer, A. Jones, P. Simpson, R.A. Harris, P.S. Jat, A.M. Neville, L.F.L. Reis, S.R. Lakhani and M.J. O’Hare, cDNA microarray analysis of genes associated with ERBB2 (HER2/neu) overexpression in human mammary luminal epithelial cells, Oncogene 22: ((2003) ), 2680–2688. |
[64] | Y.J. Cha and J.S. Koo, Expression and Role of Epithelial Membrane Proteins in Tumorigenesis of Hormone Receptor-Positive Breast Cancer, J Breast Cancer 23: ((2020) ), 385. |
[65] | Y.J. Cha and J.S. Koo, Expression of EMP1, EMP2, and EMP3 in breast phyllodes tumors, PLoS ONE 15: ((2020) ), e0238466. |
[66] | M.C. Passarelli, A.M. Pinzaru, H. Asgharian, M.V. Liberti, S. Heissel, H. Molina, H. Goodarzi and S.F. Tavazoie, Leucyl-tRNA synthetase is a tumour suppressor in breast cancer and regulates codon-dependent translation dynamics, Nat Cell Biol 24: ((2022) ), 307–315. |
[67] | Q. Xue, Y. Zhou, C. Wan, L. Lv, B. Chen, X. Cao, G. Ju, Y. Huang, R. Ni and G. Mao, Epithelial membrane protein 3 is frequently shown as promoter methylation and functions as a tumor suppressor gene in non-small cell lung cancer, Exp Mol Pathol 95: ((2013) ), 313–318. |
[68] | Z. Zheng, X. Luan, J. Zha, Z. Li, L. Wu, Y. Yan, H. Wang, D. Hou, L. Huang, F. Huang, H. Zheng, L. Ge and H. Guan, TNFα inhibits the migration of oral squamous cancer cells mediated by miR-765-EMP3-p66Shc axis, Cell Signal 34: ((2017) ), 102–109. |
[69] | S. Fumoto, K. Hiyama, K. Tanimoto, T. Noguchi, J. Hihara, E. Hiyama, T. Noguchi and M. Nishiyama, EMP3 as a tumor suppressor gene for esophageal squamous cell carcinoma, Cancer Lett 274: ((2009) ), 25–32. |
[70] | M. Han and W. Xu, EMP3 is induced by TWIST1/2 and regulates epithelial-to-mesenchymal transition of gastric cancer cells, Tumour Biol 39: ((2017) ), 101042831771840. |
[71] | Q. Guo, F.J. Jing, W. Xu, X. Li, X. Li, J.L. Sun, X.M. Xing, C.K. Zhou and F.B. Jing, Ubenimex induces autophagy inhibition and EMT suppression to overcome cisplatin resistance in GC cells by perturbing the CD13/EMP3/PI3K/AKT/NFκB axis, Aging 12: ((2019) ), 80–105. |
[72] | J. Iovanna and N. Dusetti, Speeding towards individualized treatment for pancreatic cancer by taking an alternative road, Cancer Lett 410: ((2017) ), 63–67. |
[73] | Q. Lv, W. Xiao, Z. Xiong, J. Shi, D. Miao, X. Meng, H. Yuan, H. Yang and X. Zhang, Identification of candidate biomarker EMP3 and its prognostic potential in clear cell renal cell carcinoma, Front Biosci (Landmark Ed) 26: ((2021) ), 1176–1190. |
[74] | A. Liu, D. Zhang, X. Yang and Y. Song, Estrogen receptor alpha activates MAPK signaling pathway to promote the development of endometrial cancer, J Cell Biochem 120: ((2019) ), 17593–17601. |
[75] | J.K. Burmester, B.K. Suarez, J.H. Lin, C.H. Jin, R.D. Miller, K.Q. Zhang, S.A. Salzman, D.J. Reding and W.J. Catalona, Analysis of Candidate Genes for Prostate Cancer, Hum Hered 57: ((2004) ), 172–178. |
[76] | T. Habuchi, O. Ogawa, S. Satoh, S. Matsuura, M. Saito, T. Yuasa, T. Inoue, Y. Horikawa, T. Kumazawa, S. Narita, N. Tsuchiya and H. Fukuda, Clinical implication of vascular endothelial growth factor T-460C polymorphism in the risk and progression of prostate cancer, Oncol Rep 18: ((2007) ), 1155–1163. |
[77] | Y. Xu, Y. Chen, Z. Niu, J. Xing, Z. Yang, X. Yin, L. Guo, Q. Zhang, H. Qiu and Y. Han, A Novel Pyroptotic and Inflammatory Gene Signature Predicts the Prognosis of Cutaneous Melanoma and the Effect of Anticancer Therapies, Front Med 9: ((2022) ), 841568. |
[78] | Y. Liu, J. Sun, D. Han, S. Cui and X. Yan, Identification of Potential Biomarkers and Small Molecule Drugs for Cutaneous Melanoma Using Integrated Bioinformatic Analysis, Front Cell Dev Biol 10: ((2022) ), 858633. |
[79] | S. Kaochar, J. Dong, M. Torres, K. Rajapakshe, F. Nikolos, C.M. Davis, E.A. Ehli, C. Coarfa, N. Mitsiades and V. Poulaki, ICG-001 Exerts Potent Anticancer Activity Against Uveal Melanoma Cells, Invest Ophthalmol Vis Sci 59: ((2018) ), 132. |
[80] | D. Su and L.J. Gudas, Gene expression profiling elucidates a specific role for RARγ in the retinoic acid-induced differentiation of F9 teratocarcinoma stem cells, Biochem Pharmacol 75: ((2008) ), 1129–1160. |
[81] | L. Jiang, X.P. Li, Y.T. Dai, B. Chen, X.Q. Weng, S.M. Xiong, M. Zhang, J.Y. Huang, Z. Chen and S.J. Chen, Multidimensional study of the heterogeneity of leukemia cells in t(8;21) acute myelogenous leukemia identifies the subtype with poor outcome, Proc Natl Acad Sci USA 117: ((2020) ), 20117–20126. |
[82] | E.K. Kim and J. Koo, Expression of epithelial membrane protein (EMP) 1, EMP 2, and EMP 3 in thyroid cancer, Histology and Histopathology 37: ((2021) ), 51–61. |
[83] | A. Shrivastav, A. Sharma, G. Bajaj, C. Charavaryamath, W. Ezzat, P. Spafford, R. Gore-Hickman, B. Singh, M. Copete and R. Sharma, Elevated N-myristoyltransferase activity and expression in oral squamous cell carcinoma, Oncol Rep 18: ((2007) ), 93–97. |
[84] | P. Selvakumar, A. Lakshmikuttyamma, A. Shrivastav, S.B. Das, J.R. Dimmock and R.K. Sharma, Potential role of N-myristoyltransferase in cancer, Prog Lipid Res 46: ((2007) ), 1–36. |
[85] | K. Shimazaki, E.J. Lepin, B. Wei, A.K. Nagy, C.P. Coulam, S. Mareninov, M. Fu, A.M. Wu, J.D. Marks, J. Braun, L.K. Gordon and M. Wadehra, Diabodies Targeting Epithelial Membrane Protein 2 Reduce Tumorigenicity of Human Endometrial Cancer Cell Lines, Clin Cancer Res 14: ((2008) ), 7367–7377. |
[86] | M.H. Kiyohara, C. Dillard, J. Tsui, S.R. Kim, J. Lu, D. Sachdev, L. Goodglick, M. Tong, V.F. Torous, C. Aryasomayajula, W. Wang, P. Najafzadeh, L.K. Gordon, J. Braun, S. McDermott, M.S. Wicha and M. Wadehra, EMP2 is a novel therapeutic target for endometrial cancer stem cells, Oncogene 36: ((2017) ), 5793–5807. |
[87] | M. Fu, E.L. Maresh, R.A. Soslow, M. Alavi, V. Mah, Q. Zhou, A. Iasonos, L. Goodglick, L.K. Gordon, J. Braun and M. Wadehra, Epithelial Membrane Protein-2 Is a Novel Therapeutic Target in Ovarian Cancer, Clin Cancer Res 16: ((2010) ), 3954–3963. |
[88] | M. Fu, E.L. Maresh, G.F. Helguera, M. Kiyohara, Y. Qin, N. Ashki, T.R. Daniels-Wells, N. Aziz, L.K. Gordon, J. Braun, Y. Elshimali, R.A. Soslow, M.L. Penichet, L. Goodglick and M. Wadehra, Rationale and Preclinical Efficacy of a Novel Anti-EMP2 Antibody for the Treatment of Invasive Breast Cancer, Mol Cancer Ther 13: ((2014) ), 902–915. |