Course of objectively measured physical activity and sleep in postmenopausal breast cancer survivors during the COVID-19 pandemic: A 1-year follow-up
Abstract
BACKGROUND:
As physical inactivity and poor sleep quality may impose additional risk for cancer recurrence and overall mortality in postmenopausal breast cancer (PMBC) survivors, it is important to gain insight into the effect of the COVID-19 pandemic on their physical activity (PA) and sleep level.
OBJECTIVE:
This study aimed to assess the course of their physical activity (PA) and sleep throughout governmental measures against COVID-19 during 12 months of the COVID-19 pandemic.
METHODS:
PMBC survivors (n = 96) wore an ActiGraph wGT3X-BT for seven consecutive days at 12 and 18 months after diagnosis and additional measurements were taken after onset of the second (partial) COVID-19 lockdown. Longitudinal data was categorized into four timepoints: before onset of COVID-19 (T1), during the initial lockdown (T2), in between initial and second lockdown (T3), and during the second lockdown (T4). General linear mixed effects models assessed differences in moderate-to-vigorous physical activity (MVPA) per day, total minutes of PA per day, average acceleration, intensity gradient, sleep efficiency, and sleep duration over time.
RESULTS:
Levels of MVPA per day before COVID-19 were low (Median = 20.9 min/day (IQR = 10.8;36.2)), and time spent physically active was most often in light intensity, which remained stable throughout the pandemic. Sleep duration (Median = 442.8 min/night (IQR = 418.3;478.0)) and efficiency (85.9% (IQR = 79.6;88.4)) was sufficient before COVID-19 and showed stability over time.
CONCLUSIONS:
Low levels of PA with mostly light intensity, and adequate sleep efficiency and duration were observed before COVID in PMBC survivors. This was not further affected by COVID-19 governmental measures.
1.Introduction
The majority of postmenopausal breast cancer (PMBC) survivors have suboptimal lifestyle and body weight [1–3], poorer health-related quality of life, and an increased risk of type II diabetes mellitus, cardiovascular disease, second primary cancers, cancer recurrences, and all-cause mortality as compared with a healthy population [4–6]. These adverse health outcomes may partly be prevented by maintenance of healthy levels of physical activity (PA; i.e., at least 150 minutes spent on moderate intensity PA per week or at least 75 minutes spent on vigorous PA per week, or equivalent [7,8]). In addition, higher levels of PA are associated with lower risk of cancer recurrence and higher cancer survival [7,9]; reduced symptoms of anxiety and depression, and improved self-confidence [8,10].
In addition to being sufficiently physically active, it is important for PMBC survivors to maintain a healthy sleep duration (i.e., sleeping 7–9 hours a night [11]) and sleep quality. Both chronic sleep deprivation and excess sleep have been associated with early mortality in the general population [12]. Sleep deprivation may result in metabolic changes (e.g., altered endocrine function and glucoregulation, and increased inflammation) that may predispose women to breast cancer [13]. The influence of sleep on breast cancer prognosis is inconclusive, however it has been shown that sleeping nine or more hours per night is associated with an increased risk of breast cancer recurrence, and increased risks for both breast cancer-specific mortality and all-cause mortality [14]. In addition, poor sleep quality has been linked to negative emotions and mood, and an unhealthy lifestyle [15,16].
The Coronavirus disease 2019 (COVID-19) pandemic has a substantial impact on the lives of breast cancer survivors worldwide [17]. Until a sufficient degree of vaccination has been reached, hygienic means and physical distancing, including ‘intelligent’ lockdowns, are the most used strategies to minimize the progression and severity of the COVID-19 pandemic. In addition, the World Health Organization (WHO) strongly recommends social isolation for groups at risk of severe illness such as older or individuals with obesity and those with underlying health conditions [18]. In the Netherlands, the first partial lockdown took place between 23 March 2020 and 8 June 2020 and the second partial lockdown started at 15 December 2020 (see Fig. 1 for governmental measures during these partial lockdowns).
Fig. 1.
Timeline of reported COVID-19 related deaths per day, Dutch government COVID-19 measures, and study acquisition periods. Note. Timeline starting from 1 February 2019 till 1 February 2020. The number of inhabitants of the Netherlands is 17.51 million (3th of August 2021).
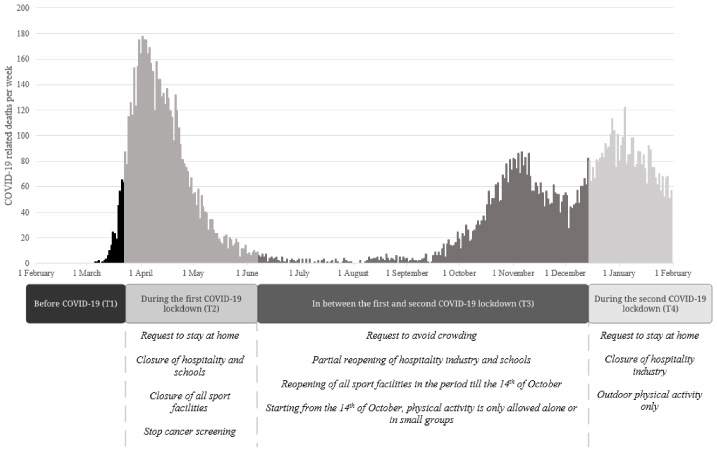
The COVID-19 pandemic, including COVID-19 lockdowns, may have impacted PA levels in PMBC survivors. Restrictions on ‘non-essential’ travel, the closure of gyms and sport courts, and the recommendation to stay at home may have decreased overall physical activity levels [19]. Moreover, the recommended social isolation for groups at risk of severe illness can make it extra challenging to meet physical activity guidelines [20].
Cancer survivors have shown to be worried about getting infected with COVID-19 more often as compared to a normative population [17]. Anxiety and uncertainties about health and economic security, may have negatively affected sleep onset and quality [21]. However, the lower number of social meetings may have resulted in longer sleep duration on weekdays and a decreased social jetlag (indicating less variable sleep timing comparing week and weekend days), positively affecting sleep quality [22]. In addition, during the second COVID-19 lockdown the COVID-19 pandemic already lasted for over 10 months, therefore possibly affecting psychological and emotional resources of cancer survivors. In contrast, in other PMBC survivors, the long period of COVID-19 pandemic may have led to adaptation and acceptance of the situation. Consequently, the effect of the initial and second COVID-19 lockdown on PA and sleep in these cancer survivors may differ.
As physical inactivity and poor sleep quality may impose an additional risk for cancer recurrence and overall mortality, it is important to gain insight into the effect of the COVID-19 pandemic on PA and sleep in PMBC survivors. To our knowledge, previous studies investigating the effect of COVID-19 lockdown on PA and sleep mainly focused on the general population, self-reported measures, and only during initial lockdown. This study aims to assess the course of accelerometer-measured PA and sleep during 12 months of the COVID-19 pandemic, and to gain insight into possible changes in PA and sleep of both the initial and second COVID-19 lockdown, in postmenopausal breast cancer (PMBC) survivors.
2.Methods
2.1.Participants
For the present analyses, data from the OPtimal TIming and Method for promoting sUstained adherence to lifestyle and body weight recommendations in postMenopausal breast cancer survivors (OPTIMUM)-study was used [23]. Details of the OPTIMUM-study are presented elsewhere [23].
Patient recruitment and data collection for the OPTIMUM-study started in September 2019. Inclusion criteria were being diagnosed with breast cancer up to 6 months ago; being postmenopausal, having not menstruated for at least 1 year, and being able to read and understand Dutch. Each eligible PMBC survivor, from one of eight participating hospitals, was invited to participate in the study. The OPTIMUM-study is a longitudinal study with accelerometer measurements at 12 and 18 months following diagnosis. For the present analyses, we included data of the first 96 participants of the OPTIMUM-study who all had a first accelerometer measurement (at 12 months following diagnosis) between September 2019 and September 2020. Patients included in the OPTIMUM study between September 2020 and September 2022 are not included in the current analysis. Due to the continuous inclusion and measurement of the OPTIMUM-study, the time-point of the accelerometer measurements during the COVID-19 pandemic varied for all participating PMBC survivors The measurements were categorized into four time-periods: before onset of COVID-19 (T1), during the first COVID-19 lockdown (T2), the time in between the first and second COVID-19 lockdown (T3), and during the second COVID-19 lockdown (T4) (Fig. 2). Of the 96 participants, 30 had their first accelerometer measurement before the onset of COVID-19 (T1), 25 had their first accelerometer measurement during COVID-19 lockdown 1, and 41 had their first accelerometer measurement between lockdown 1 and lockdown 2 (Fig. 2).
Fig. 2.
Overview of repeated measurements of physical activity and sleep using the Actigraph. Note. On the vertical axes, the number of observations per time point are depicted. On the horizontal axes, the number of participants per repeated measures timeline are depicted.
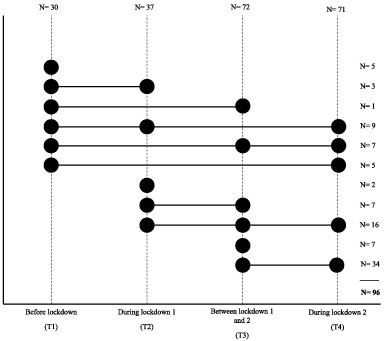
Six months following their first accelerometer measurement, the PMBC survivors who were 18 months past their diagnosis, were invited for their second accelerometer measurement (n = 43). In addition, after onset of the second COVID-19 lockdown on the 15th of December 2021, we re-invited all 96 participants for an additional measurement with the accelerometer, of whom 71 (75%) participated. Due to the continuous inclusion and measurement in the OPTIMUM-study, the last measurement during the second COVID-19 lockdown occurred at varying timepoints since diagnosis (mean = 1.7 years since diagnosis (SD = 0.3 year)).
All participants were invited to wear the accelerometer at three time-points during this COVID-19 timeline. However, due to the continuous measurement and inclusion in the OPTIMUM-study not all participants had finished all measurements at the time of analyses. Additionally, there was missing data due to physical limitations (e.g., intensive breast cancer treatment; illness; broken leg) as well as practical issues (e.g., unpractical to wear the accelerometer in work setting, cleaning, healthcare including helping someone shower). In the present analyses we were able to include complete data for three out of four time-point during the COVID-19 pandemic for 32 PMBC survivors. For 42 PMBC survivors we could include data of 2 time-points, and for 14 PMBC survivors data of 1 time-point (Fig. 2). To assess the stability of our results, a sensitivity analysis was conducted of pairwise descriptive data of full responders across consecutive timepoints: T1 vs. T2 (N = 12), T2 vs. T3 (N = 23), and T3 vs. T4 (N = 58).
All participants provided written informed consent prior to the start of the study, and prior to the additional measurement during the second COVID-19 lockdown. The study protocol was approved by a medical ethical review board, according to the Dutch Medical Research Involving Human Subjects Act (WMO).
2.2.Procedure
A baseline questionnaire was completed 4–6 months after diagnosis (T0). Participants were invited to complete either an online or paper questionnaire via the PROFILES (Patient Reported Outcomes Following Initial treatment and Long term Evaluation of Survivorship) registry [24].
At 12 months and 18 months after diagnosis (during T1 or T2 or T3; varying per patient) and during the second COVID-19 lockdown (T4), participants completed follow-up questionnaires and wore an ActiGraph wGT3X-BT accelerometer (ActiGraph; ActiGraph, LLC, Pensacola, FL, USA) on their non-dominant wrist (i.e., defined as the hand they normally do not write with) [25]. All participants received a phone call to inform them about the procedure with respect to wearing the accelerometer, and a start-date was planned. We ensured that the participants had no planned vacation or other abnormalities during the planned week of wearing the accelerometer. Following, all participants received the accelerometer together with a paper instruction concerning device placement (illustrated in pictures), a wearing log, a sleep diary, and wear instructions via mail. Participants were instructed to wear the device 24 hours a day for 7 consecutive days, except during water-based activities such as showering, bathing, and swimming. Prior to distribution, each accelerometer was initialized to capture data at 100 Hz and was synchronized to Greenwich Mean Time.
2.3.Measures
2.3.1.Sociodemographic and clinical characteristics
The baseline questionnaire assessed comorbidities in the past 12 months by use of the Self-administered Comorbidity Questionnaire (SCQ) [26]. The SCQ is a list of 14 medical conditions, with the option to list up to three additional medical conditions [26]. Follow-up questionnaires assessed body weight and height. Clinical data (i.e., cancer stage, date of diagnosis, and start- and end-date of treatment) was obtained from the Netherlands Cancer Registry (NCR). The NCR routinely collects data on all newly diagnosed cancer patients in the Netherlands [27]. Time since diagnosis was calculated by subtracting the date of diagnosis from the start day of wearing the accelerometer. Treatment during time of measurement was determined by subtracting the time since diagnosis from the number of days since treatment ended.
2.3.2.Data processing and accelerometer-measurement of physical activity and sleep
Upon return of the accelerometer, the data was downloaded using the accompanying software ActiLife (Version 6.13.3; ActiGraph, Pensacola, FL, USA) and saved in raw format as .gt3x files. Subsequently, the .gt3x files were converted to time-stamp free .csv files which could be exported into R v.3.6.0. The .csv files were processed using the R-package ‘GGIR: Raw Accelerometer Data Analysis’ v.2.1-0 [28]. Data processing included detection of sustained abnormally high values, detection of nonwear time defined as the time not wearing the accelerometer in minutes [29], and auto-calibration of the raw tri-axial accelerometer signals using local gravity as reference [30]. In addition, within GGIR the Euclidean Norm Minus One (ENMO) (1g) was calculated averaged over 5 seconds epochs and expressed in milli-gravitational units (mg) as previously described by Rowlands and colleagues (2018) [31].
Data of participants was excluded from subsequent analysis if their accelerometer files demonstrated a post-calibration error larger than 0.01 g (10 mg); or if there were less than 3 valid wear-days (defined as ≥16 h per day) [31]. Detection of nonwear time has previously been described in detail (see “Procedure for nonwear detection” as supplementary document to van Hees et al. [29]). The default nonwear setting was used imputing invalid data by the average of similar time points on different days of the week [29], which means that for each valid wear day outcome variables were based on the complete 24 h cycle (1440 minutes) for all participants. PA level was expressed as average acceleration across the day (ENMO, mg) [31], average time accumulated in light intensity PA (LPA) per day (min/day), average time accumulated in moderate-to-vigorous PA (MVPA) per day (min/day), and time spent inactive per day (min/day). Time spent in LPA and MVPA was the accumulated time above an acceleration of respectively 50 mg and 100 mg [30]. In contrast, time spent inactive was defined as the time accumulated below an acceleration of 50 mg [31]. To determine the distribution of physical activity intensity by use of the intensity gradient (IG), the argument “iglevels = TRUE” was used in GGIR. The IG reflects the negative curvilinear relation between PA intensity and time spent in that intensity [31]. Periods of physical inactivity, defined as no changes in arm angle greater than 5 degrees for 5 minutes or more during a predefined nocturnal sleep window (e.g., from the participants’ sleep log), were classified as sleep [29,32]. Sleep quality was expressed as the sleep efficiency (i.e., proportion of the sleep span actually sleeping (%)), the frequency of long sleep interruptions (i.e., the number of long wake episodes ≥ 5 minutes), and average total number of minutes spent sleeping [32,33].
2.4.Statistical analysis
Descriptive information for the total group and for the participants at each time-point of normally distributed variables is presented as mean and standard deviation, otherwise the median and interquartile range are used.
Generalized linear mixed-effects models were built, using 3,000 parametric bootstraps, to investigate the changes in PA (MVPA per day; total min physical activity per day; average acceleration; intensity gradient) and sleep (sleep efficiency and sleep duration) over time. Time, the variable of main interest, was handled as categorical fixed effect including the four time points (i.e., before COVID-19 (T1); during the first lockdown (T2); in between the first and second lockdown (T3); during the second lockdown (T4)) in all models. All models included a random intercept to adjust for clustering of observations within participants. Time since diagnosis was entered as covariate in the basic mixed-effects models to adjust for the course of PA and sleep rehabilitation in the time since diagnosis till the first accelerometer measurement. In addition, adjusted mixed-effect models were built, extending previous models by adjusting for the following potential confounding background variables: age, receiving any active treatment versus no active treatment during the time of measurement (i.e., chemotherapy, radiotherapy, hormonal therapy or, targeted treatment; yes vs. no), number of comorbidities (0; 1; 2>), and employment. The models’ regression coefficients, 95% confidence intervals (CI), and p-values are presented.
All analyses were performed using R version 4.1.0 (https://cran.r-project.org).
3.Results
Participants (N = 96) were on average 64.3 (SD = 7.6) years old, 39% were employed, and mean BMI was 26.9 (SD = 4.3) (Table 1).
Table 1
Socio-demographic and clinical characteristics
Overall | Before COVID2 | During first COVID lockdown3 | In between first and second COVID lockdown4 | During second COVID lockdown5 | |
(T1) | (T2) | (T3) | (T4) | ||
N 1 | 96 | 30 | 37 | 72 | 71 |
Age, mean (SD) | 64.3 (7.6) | 62.4 (7.5) | 65.0 (8.2) | 64.7 (7.2) | 64.9 (7.9) |
Partner (yes), N (%) | 72 (67%) | 20 (74%) | 29 (85%) | 55 (80%) | 50 (75%) |
Education level, N (%) 6 | |||||
Low | 2 (2%) | 2 (7%) | 2 (6%) | 0 (0%) | 2 (3%) |
Medium | 55 (59%) | 16 (59%) | 24 (71%) | 43 (62%) | 39 (58%) |
High | 36 (39%) | 12 (44%) | 10 (29%) | 26 (38%) | 27 (40%) |
Employment (yes), N (%) | 36 (39%) | 11 (41%) | 13 (38%) | 27 (39%) | 24 (36%) |
BMI, mean (SD) | 26.9 (4.3) | 26.8 (4.0) | 26.6 (4.5) | 27.1 (4.4) | Unknown9 |
Comorbidities, N (%) | |||||
0 | 27 (28%) | 11 (37%) | 12 (32%) | 19 (26%) | 22 (31%) |
1 | 28 (29%) | 7 (23%) | 10 (27%) | 23 (32%) | 18 (26%) |
≥2 | 41 (43%) | 12 (40%) | 15 (41%) | 30 (42%) | 31 (44%) |
Tumor stage, N (%) | |||||
0 | 4 (4%) | 2 (7%) | 3 (8%) | 3 (4%) | 4 (6%) |
I | 47 (49%) | 15 (50%) | 17 (46%) | 36 (50%) | 36 (51%) |
II | 40 (42%) | 12 (40%) | 14 (38%) | 29 (40%) | 27 (38%) |
III | 4 (4%) | 1 (3%) | 3 (8%) | 3 (4%) | 3 (4%) |
IV | 1 (1%) | 0 (0%) | 0 (0%) | 1 (1%) | 1 (1%) |
Time since diagnosis (days), mean (SD) | 504.3 (128.7) | 392.7 (57.7) | 430.3 (80.5) | 467.6 (91.6) | 626.0 (108.4) |
Treatment during time of measurement 7 | |||||
No treatment, N (%) | N/A8 | 8 (27%) | 16 (43%) | 27 (38%) | 26 (37%) |
Surgery (yes), N (%) | N/A | 0 (0%) | 0 (0%) | 0 (0%) | 0 |
Chemotherapy (yes), N (%) | N/A | 0 (0%) | 2 (5%) | 0 (0%) | 0 |
Radiotherapy (yes), N (%) | N/A | 0 (0%) | 0 (0%) | 0 (0%) | 0 |
Hormonal therapy (yes), N (%) | N/A | 21 (70%) | 19 (51%) | 38 (53%) | 38 (53%) |
Targeted therapy (yes), N (%) | N/A | 1 (3%) | 1 (3%) | 10 (14%) | 9 (13%) |
[i] Note: Descriptives of socio-demographic and clinical characteristics for the total group and for the participants at each time-point. Due to repeated measures (see Fig. 2), the number of participants at the different time points does not add up to 96 (i.e., Total N).1 N = number of participants assessed by accelerometer per time point. Variables may deviate from 100% due to rounding off. SD standard deviation. BMI Body Mass Index. 2Measurement before 23 March 2020. 3Measurement between 23 March till 9 June 2020. 4Measurement between 9 June till 15 December 2020. 5Measurements between 15 December 2020 and 1 February 2021. 6Education level: Low (no or primary school); medium (lower general secondary education or vocational training); high (pre-university education, high vocational training, university). 7Treatment during the time of measurement with the Actigraph. Due to combined treatment in certain participants, percentages do not add up to 100%. 8N/A = not applicable. 9BMI was not measured during the second COVID lockdown.
All PA measures (min MVPA/day; total min spent physically active/day; average acceleration (mg); Intensity gradient) showed mainly engagement in low intensity PA and stable levels over the four time points (see Tables 2 and 3). The largest difference in min MVPA was observed between the first lockdown (T2) and the second lockdown (T4) (estimated difference 5.91 minutes per day; see Table 3). However, this difference was not statistically significant. A sensitivity analysis, conducted through pairwise descriptive analysis of full responders across consecutive timepoints (see Appendix), demonstrated consistent and stable results.
Table 2
Overview of physical activity and sleep parameters at all study time-points
Before COVID2 | During COVID lockdown 13 | In between COVID lockdown 1 and 24 | During COVID lockdown 25 | |
(T1) | (T2) | (T3) | (T4) | |
N | 30 | 37 | 72 | 71 |
Physical activity | ||||
MVPA per day (min/day), median (IQR) | 20.9 (10.8, 36.2) | 14.8 (3.8, 31.1) | 18.6 (5.1, 32.9) | 26.8 (11.3, 37.1) |
MVPA per week (min/week), median (IQR) | 146.0 (75.7, 253.4) | 103.6 (25.8, 217.5) | 130.1 (35.4, 230.2) | 187.3 (79.2, 259.9) |
Light intensity physical activity (min/day), median (IQR) | 117.7 (93.9, 140.6) | 110.3 (90.3, 143.1) | 113.2 (88.0, 142.8) | 105.9 (82.5, 135.2) |
Inactive time (min/day), mean (SD) | 724.0 (93.3) | 725.8 (89.0) | 744.8 (75.0) | 748.6 (69.0) |
Average acceleration (mg), median (IQR) | 22.0 (17.2, 25.0) | 19.8 (16.1, 26.1) | 21.3 (16.3, 25.3) | 21.3 (18.2, 24.3) |
Intensity gradient, mean (SD) | −3.4 (.3) | −3.4 (.4) | −3.4 (.4) | −3.4 (.4) |
Total physical activity (min/day), mean (SD) | 181.3 (60.8) | 183.6 (79.4) | 183.2 (69.3) | 172.0 (55.6) |
Sleep | ||||
Sleep duration (min/night), median (IQR) | 442.8 (418.3, 478.0) | 454.4.0 (425.8, 489.9) | 444.1 (396.4, 463.6) | 453.2 (414.2, 484.7) |
Sleep efficiency (%), median (IQR) | 85.9 (79.6, 88.4) | 87.5 (83.5, 91.5) | 85.8 (82.8, 88.8) | 86.3 (83.8, 90.6) |
Frequency of sleep interruptions (n/night), median (IQR) | 1.9 (1.5, 3.1) | 1.8 (0.8, 3.5) | 1.9 (1.4, 3.5) | 1.9 (1.0, 3.4) |
[i] 1Measurement before 23 March 2020. 2Measurement between 23 March till 9 June 2020. 3Measurement between 9 June till 15 December 2020. 4Measurements between 15 December 2020 and 1 February 2021. MVPA = moderate to vigorous physical activity; SD = standard deviation; IQR = interquartile range.
Table 3
Summary of mixed-effects analyses
MVPA per day (min/day) | ||||||
Basic modela | Corrected modelb | |||||
Time | B | LL – UL 95% CI | p | B | LL - UL 95%CI | p |
T2 vs. T1 (N = 67) | −.49 | −7.13; 6.55 | .89 | .93 | −6.17; 7.53 | .80 |
T3 vs. T1 (N = 102) | −.43 | −8.76; 7.95 | .92 | 1.07 | −7.32; 8.76 | .80 |
T4 vs. T1 (N = 101) | 5.42 | −6.23; 16.85 | .36 | 7.76 | −3.38; 18.76 | .17 |
Total physical activity (min/day) | ||||||
Basic modela | Corrected modelb | |||||
Time | B | LL – UL 95% CI | p | B | LL – UL 95% CI | p |
T2 vs. T1 (N = 67) | 12.23 | −8.14; 31.93 | .25 | 10.15 | −9.12; 29.63 | .30 |
T3 vs. T1 (N = 102) | 14.77 | −11.64; 41.95 | .27 | 15.10 | −11.98; 41.03 | .26 |
T4 vs. T1 (N = 101) | 10.00 | −26.06; 47.64 | .57 | 11.07 | −26.45; 46.85 | .55 |
Average acceleration (mg) | ||||||
Basis modela | Corrected modelb | |||||
Time | B | LL – UL 95% CI | p | B | LL – UL 95% CI | p |
T2 vs. T1 (N = 67) | −0.31 | −2.64; 2.06 | .80 | −0.49 | −2.91; 1.94 | .68 |
T3 vs. T1 (N = 102) | −0.05 | −2.42; 2.50 | .96 | −0.23 | −2.89; 2.35 | .86 |
T4 vs. T1 (N = 101) | 0.06 | −3.16; 3.37 | .97 | −0.10 | −3.51; 3.39 | .95 |
Intensity gradient | ||||||
Basic modela | Corrected modelb | |||||
Time | B | LL – UL 95% CI | p | B | LL – UL 95% CI | p |
T2 vs. T1 (N = 67) | .04 | −0.14; 0.22 | .70 | .01 | −0.17; 0.19 | .90 |
T3 vs. T1 (N = 102) | .04 | −0.14; .21 | .68 | .03 | −0.14; 0.20 | .75 |
T4 vs. T1 (N = 101) | .10 | −0.12; 0.32 | .37 | .07 | −0.16; 0.29 | .52 |
Sleep duration (min/night) | ||||||
Basic modela | Corrected modelb | |||||
Time | B | LL – UL 95% CI | p | B | LL – UL 95% CI | p |
T2 vs. T1 (N = 67) | −5.70 | −27.55; 15.80 | .63 | −2.91 | −25.00; 20.48 | .81 |
T3 vs. T1 (N = 102) | −16.01 | −39.15; 6.36 | .17 | −11.76 | −35.50; 12.76 | .33 |
T4 vs. T1 (N = 101) | −13.19 | −41.79; 15.58 | .38 | −6.27 | −37.91; 23.88 | .69 |
Sleep efficiency (%) | ||||||
Basic modela | Corrected modelb | |||||
Time | B | LL – UL 95% CI | p | B | LL – UL 95% CI | p |
T2 vs. T1 (N = 67) | 1.66 | −0.84; 4.00 | .19 | 1.46 | −1.11; 4.08 | .26 |
T3 vs. T1 (N = 102) | 0.42 | −2.16; 3.05 | .75 | .44 | −2.14; 3.06 | .75 |
T4 vs. T1 (N = 101) | 1.41 | −2.07; 4.94 | .40 | 1.51 | −1.83; 4.99 | .39 |
[i] Note: T1 = Before COVID; T2 = During first COVID lockdown; T3 = In between first and second COVID lockdown; T4 = During second COVID lockdown; MVPA = moderate to vigorous physical activity.a Basic mixed-effect model for each of the main outcome measures, controlling for time since diagnosis (using 3000 bootstraps). bCorrected model: adjusted for time since diagnosis, age, receiving active treatment at the time of measurement (chemotherapy, radiotherapy, hormonal therapy, targeted treatment), number of comorbidities (0; 1; 2>), employment.
Also, sleep duration and sleep efficiency was stable over the four time points (see Table 3). The largest difference in sleep duration was observed between the time before COVID-19 (T1) and the time following the first COVID-19 lockdown (T3) (estimated difference −16.01 min per day; 95%CI = −39.15;6.36; see Table 3).
Overall average relative nonwear time during waking hours was 1.2 % (SD = 1.9) and it was 12.7% (SD = 3.5) during the hours between sleep onset and wake onset. During the whole day average relative nonwear time was 5.5% (SD = 1.3). The average number of valid wear days was 5.8 (SD = .6), with an average of 1.1 weekend days (SD = .42) (data not shown).
Sensitivity analysis excluding the participants with only one Actigraph measurement in the COVID-19 timeline, provided comparable results.
4.Discussion
This study evaluated changes in PA and sleep during the COVID-19 pandemic in PMBC survivors. Overall, we observed that both PA and sleep seem stable over time. Although PA was relatively low at the first lock-down, no statistically significant differences were found over time. The stable PA levels and intensity we observed are in line with a previous study in the general population in the United Kingdom, showing that those aged >65 were able to remain physically active during and after the initial COVID-19 restrictions [34]. Similarly, in our sample with an average age of 64.3 years, the PMBC survivors maintained, on average, a stable level of minutes spent in MVPA per day after onset of COVID-19 restrictions.
However, as physical inactivity may impose an additional risk in the population of PMBC survivors for cancer recurrence and overall mortality, the observed low levels of PA spent in MVPA a day are alarming.
The low level of PA is comparable to other studies investigating cancer survivors prior to the pandemic [35]. Pooled data of eight studies in cancer survivors (using hip-worn Actigraphs) showed on average 26 min time spent in MVPA a day [35]. Our results indicated an average of 22.1 min before COVID-19 until the second COVID-19 lockdown, and 27.9 min a day during the second COVID-19 lockdown. Previous studies indicated that being older, having a higher BMI, and being female are associated with lower MVPA levels in cancer survivors [36]. In addition, compared to other studies, the measurements in our sample were relatively shortly following their diagnosis, and even included some individuals still receiving treatment during time of measurement (e.g., chemotherapy, hormonal therapy, and targeted therapy). This also may explain the low level of MVPA per day [37].
In addition, the intensity gradient of our sample indicates mainly engagement in low intensity PA. Most previous studies that demonstrated a health benefit of PA encouraged time spent in moderate or vigorous intensity PA [37,38]. It is uncertain whether engaging mainly in light PA could also be associated with lower levels of all-cause mortality and breast cancer recurrence, especially in case of high levels of sedentary behavior, which was shown to be common (66% of waking time) in breast cancer survivors [38,39].
As the majority of PMBC survivors have suboptimal lifestyle and bodyweight, they are at increased risk for second primary cancers and comorbid conditions compared to women without cancer [4–6,40]. The stability in the level and intensity of PA in our sample during the COVID-19 governmental measurements indicates that it did not worsen, yet their PA levels remained consistently low. Consequently, it is warranted to develop strategies to improve PA level in PMBC survivors. Hence, there is a need to investigate how to effectively and sustainably change PA behavior in this population.
The norm for sleep duration in Caucasian women aged 60 to 69 years old is 423.8 minutes a night [41]. In comparison, the average minutes spent sleeping in our study sample (mostly Caucasian women) was 445.6 per night indicating that overall, the participants did not experience major problems in sleep duration. Sleep duration and sleep quality were stable and sufficient in our sample of PMBC survivors throughout the COVID-19 pandemic. In relation to COVID-19, previous studies attributed poorer sleep quality partially to those experiencing higher levels of anxiety or depression during lockdown [42]. Even though it may be expected that cancer survivors are at higher risk for feelings of anxiety and depression during lockdown, it has been shown that cancer survivors reported almost similar levels of anxiety and depression as compared to a normative population [17]. This may contribute to the stable sleep parameters found in our study.
An important strength of this study is the use of accelerometer-measurement of PA and sleep. This may have circumvented reporting errors typically found while using self-reported measures (i.e., errors due to recall bias, misinterpretation, and social desirability) [43]. In addition, there was high compliance with daily wear time of the accelerometer. As a consequence, very little time needed to be imputed by the average of similar time points on different days of the week to add up to a complete 24-h cycle. Nevertheless, the study has several limitations. First, as the COVID-19 pandemic set in unexpectedly we could not control the number of observations at the different time points during the COVID-19 pandemic. This resulted in different trajectories of repeated measures across participants. The number of observations between the first and second COVID-19 lockdown was relatively high due to the long time span of this period in comparison to the other time points. In addition, the relatively large number of observations during the second COVID-19 lockdown can be explained by the invitation of all OPTIMUM-participants with a previous accelerometer measurement during the COVID-19 time span for this additional measurement. The different number of observations for the four time points and the different trajectories of repeated measures is taken into account by the use of mixed models but does result in relatively broad CI’s for periods with fewer participants. Our sample also precluded investigations into different trajectories of PA and sleep in subgroups of PMBC survivors. Second, some participants received active treatment (chemotherapy, hormonal therapy or targeted therapy) during any of the time points. Although we adjusted for active treatment in our analyses this may have affected the results. Specifically, PMBC survivors may have gradually increased their PA due to recovery from the diagnosis and subsequent treatment possibly balancing out a decrease in PA caused by COVID-19. Finally, only the baseline questionnaire included questions regarding the number of comorbidities.
The recommended level of PA holds spending at least 150 minutes on moderate PA per week or at least 75 minutes spent on vigorous PA per week. We found an average of 141.8 minutes spent on MVPA per week, indicating that a large proportion of the participants did not meet the PA recommendations. Additionally, a high proportion of PA was performed in lower intensity PA. As levels of PA were already low prior to the COVID-19 pandemic, it is not likely that the pandemic would have changed this behavior. Previous studies have shown that favorable lifestyle change following the cancer diagnosis is supported with evidence-based lifestyle counselling by oncology clinicians and health care practitioners concerning the benefits of sufficient PA for the health and prognosis of their cancer survivors [44]. Moreover, thus far oncology clinicians are found to be the most powerful catalysts for the promotion of health behavior [45,46]. Due to COVID-19 the number of follow-up consultations has diminished, and ongoing follow-up consultation was often replaced by telephonic or video consultation [17,47]. Consequently, possibly less counseling and advice to increase PA level may have occurred. Besides counselling, also referral to appropriate exercise-programs in line with clinical possibilities and preference may be challenging during the COVID-19 pandemic. Nevertheless, inactive patients could be advised to progressively replace sitting behavior with active breaks of walking at home and active patients to adopt physical activities to the home setting depending on ongoing COVID-19 measures.
In conclusion, on average, the PMBC survivors showed low levels of PA before COVID-19, and time spent physically active was mostly of light intensity. Sleep duration and sleep quality was sufficient before the COVID-19 pandemic in our sample of PMBC survivors. Our results point to stable levels of PA and sleep during the COVID-19 pandemic in PMBC survivors. As inactive and overweight PMBC survivors are at risk of worse progression of COVID-19, and at risk of cancer recurrence and worse prognosis following the diagnosis of cancer [20,47], it is important that health care professionals play a role in promotion of an active lifestyle for these patients, both during pandemics and thereafter.
Acknowledgements
We would like to thank all participants for their participation in the study.
Ethics approval
The study protocol was approved by the medical ethical review board METC Brabant (the Netherlands), according to the Dutch Medical Research Involving Human Subjects Act (WMO).
Consent
All participants provided written informed consent prior to the start of the study.
Conflict of interest
The authors declare that they have no conflict of interest.
Data
The data that support the findings of this study are available on request from the corresponding author. The data are not publicly available due to privacy or ethical restrictions.
Funding
The research leading to these results received funding from the Dutch Cancer Society under grant agreement number 10960, and an Investment Subsidy Large of the Netherlands Organization for Scientific Research (The Hague, The Netherlands). The funder played no role in the design, conduct, or reporting of this study.
Authors’ contributions
All authors contributed to the study conception and design. Material preparation and data collection were performed by S.J.M. van Cappellen-van Maldegem, I.L. Nijman, and H.M.W. van Valenberg. Analysis were performed by S.J.M. van Cappellen-van Maldegem, L.M. Buffart, M.R. de Boer, and B.H. de Rooij. The first draft of the manuscript was written by S.J.M van Cappellen-van Maldegem and all authors commented on previous versions of the manuscript. All authors read and approved the final manuscript.
Appendices
Appendix
See Table A1.
Table A1
Sensitivity analysis: pairwise descriptive analysis of physical activity and sleep data of full responders for consecutive timepoints, T1 vs. T2 (N = 12); T2 vs. T3 (N = 23); and T3 vs. T4 (N = 58)
Before COVID2 | During COVID lockdown 13 | During COVID lockdown 13 | In between COVID lockdown 1 and 24 | In between COVID lockdown 1 and 24 | During COVID lockdown 25 | |
(T1) | (T2) | (T2) | (T3) | (T3) | (T4) | |
N | N = 12 | N = 23 | N = 58 | |||
Physical activity | ||||||
MVPA per day (min/day), median (IQR) | 19.2 (13.5, 30.0) | 6.0 (2.4, 25.2) | 18.3 (8.9, 26.8) | 16.4 (2.5, 33.3) | 18.2 (9.7, 31.6) | 29.0 (13.3, 37.5) |
MVPA per week (min/week), median (IQR) | 134.5 (94.2, 209.9) | 42.0 (16.7, 176.1) | 127.9 (62.0, 187.5) | 114.9 (17.4, 233.3) | 127.4 (68.0, 221.5) | 203.3 (39.0, 262.8) |
Light intensity physical activity (min/day), median (IQR) | 117.9 (96.3, 140.6) | 120.4 (90.8, 127.6) | 99.3 (80.4, 155.5) | 99.8 (84.4, 123.3) | 118.1 (93.3, 144.7) | 102.1 (81.2, 135.3) |
Inactive time (min/day), mean (SD) | 729.9 (84.9) | 717.3 (84.9) | 732.8 (94.9) | 757.5 (97.0) | 739.1 (72.2) | 753.0 (65.0) |
Average acceleration (mg), median (IQR) | 22.2 (17.7, 24.8) | 19.4 (16.2, 23.2) | 19.5 (15.7, 26.3) | 21.2 (16.2, 23.6) | 22.7 (17.7, 25.4) | 21.1 (18.2, 24.3) |
Intensity gradient, mean (SD) | −3.4 (0.4) | −3.5 (0.5) | −3.4 (0.3) | −3.5 (0.4) | −3.4 (0.4) | −3.4 (0.4) |
Total physical activity (min/day), mean (SD) | 176.4 (56.6) | 173.5 (70.9) | 186.7 (86.9) | 174.4 (76.7) | 187.2 (70.1) | 172.9 (55.8) |
Sleep | ||||||
Sleep duration (min/night), median (IQR) | 447.4 (422.1, 483.0) | 447.3 (423.7, 455.5) | 457.4 (428.2, 491.4) | 454.3 (422.2, 487.1) | 443.1 (396.4, 461.5) | 444.8 (409.2, 473.7) |
Sleep efficiency (%), median (IQR) | 83.7 (81.0, 86.0) | 85.9 (80.3, 88.0) | 88.9 (83.9, 92.2) | 86.7 (85.3, 87.8) | 86.1 (82.8, 88.8) | 86.2 (83.8, 90.0) |
Frequency of sleep interruptions (n/night), median (IQR) | 2.0 (1.5, 3.7) | 1.9 (1.4, 3.9) | 1.4 (0.4, 2.9) | 1.9 (1.6, 2.5) | 1.9 (1.4, 3.4) | 1.9 (1.0, 3.4) |
[i] Note: T1 = Before COVID; T2 = During first COVID lockdown; T3 = In between first and second COVID lockdown; T4 = During second COVID lockdown; MVPA = moderate to vigorous physical activity. aBasic mixed-effect model for each of the main outcome measures, controlling for time since diagnosis (using 3000 bootstraps). bCorrected model: adjusted for time since diagnosis, age, receiving active treatment at the time of measurement (chemotherapy, radiotherapy, hormonal therapy, targeted treatment), number of comorbidities (0; 1; 2>), employment.
References
[1] | Blanchard CM, , Courneya KS, , Stein K, Cancer survivors’ adherence to lifestyle behavior recommendations and associations with health-related quality of life: results from the American Cancer Society’s SCS-II, J Clin Oncol, 26: (13): 2198–2204, (2008) . |
[2] | Underwood JM, , Townsend JS, , Stewart SL, , Buchannan N, , Ekwueme DU, , Hawkins NA , Surveillance of demographic characteristics and health behaviors among adult cancer survivors—Behavioral Risk Factor Surveillance System, United States, 2009, MWRS Summaries, 61: (1): 1–23, (2012) . |
[3] | Zhao G, , Li C, , Okoro CA, , Li J, , Wen XJ, , White A , Trends in modifiable lifestyle-related risk factors following diagnosis in breast cancer survivors, J Cancer Surviv, 7: (4): 563–569, (2013) .. |
[4] | Ng AK, , Travis LB, Second primary cancers: an overview, Hematol Oncol Clin North Am, 22: (2): 271–289, (2008) . |
[5] | Lipscombe L, , Chan W, , Yun L, , Austin P, , Anderson G, , Rochon PJD, Incidence of diabetes among postmenopausal breast cancer survivors, Diabetologia, 56: (3): 476–483, (2013) .. |
[6] | Brandt A, , Thomsen H, , Hemminki K, , Riihimäki M, , Sundquist J, Death causes in breast cancer patients, Ann Oncol, 23: (3): 604–610, (2011) . |
[7] | Patel AV, , Friedenreich CM, , Moore SC, , Hayes SC, , Silver JK, , Campbell KL , American College of sports medicine roundtable report on physical activity, sedentary behavior, and cancer prevention and control, Med Sci Sports Exerc, 51: (11): 2391–2402, (2019) . |
[8] | Campbell KL, , Winters-Stone KM, , Wiskemann J, , May AM, , Schwartz AL, , Courneya KS , Exercise guidelines for cancer survivors: consensus statement from international multidisciplinary roundtable, Med Sci Sports Exerc, 51: (11): 2375–2390, (2019) . |
[9] | McTiernan A, , Friedenreich CM, , Katzmarzyk PT, , Powell KE, , Macko R, , Buchner D , Physical activity in cancer prevention and survival: a systematic review, Med Sci Sports Exerc, 51: (6): 1252, (2019) . |
[10] | Taylor CB, , Sallis JF, , Needle R, The relation of physical activity and exercise to mental health, Public Health Rep, 100: (2): 195, (1985) . |
[11] | Watson NF, , Badr MS, , Belenky G, , Bliwise DL, , Buxton OM, , Buysse D , Recommended amount of sleep for a healthy adult: a joint consensus statement of the American Academy of Sleep Medicine and Sleep Research Society, Sleep, 38: (6): 843–844, (2015) . |
[12] | Patel SR, , Ayas NT, , Malhotra MR, , White DP, , Schernhammer ES, , Speizer FE , A prospective study of sleep duration and mortality risk in women, Sleep, 27: (3): 440–444, (2004) . |
[13] | Spiegel K, , Leproult R, , Van Cauter E, Impact of sleep debt on metabolic and endocrine function, The Lancet, 354: (9188): 1435–1439, (1999) . |
[14] | Marinac CR, , Nelson SH, , Flatt SW, , Natarajan L, , Pierce JP, , Patterson RE, Sleep duration and breast cancer prognosis: perspectives from the Women’s Healthy Eating and Living Study, Breast Cancer Res Treat, 162: (3): 581–589, (2017) . |
[15] | Baglioni C, , Spiegelhalder K, , Lombardo C, , Riemann D, Sleep and emotions: a focus on insomnia, Sleep Med Rev, 14: (4): 227–238, (2010) . |
[16] | Kruger AK, , Reither EN, , Peppard PE, , Krueger PM, , Hale L, Do sleep-deprived adolescents make less-healthy food choices? Br J Nutr, 111: (10): 1898–1904, (2014) . |
[17] | van de Poll-Franse LV, , de Rooij BH, , Horevoorts NJ, , May AM, , Vink GR, , Koopman M , Perceived care and well-being of patients with cancer and matched norm participants in the COVID-19 crisis: results of a survey of participants in the Dutch PROFILES Registry, JAMA Oncol, 7: (2): 279–284, (2021) . doi:10.1001/jamaoncol.2020.6093. |
[18] | W.H. Organization, Coronavirus disease (COVID-19) pandemic, 2019. |
[19] | Avancini A, , Trestini I, , Tregnago D, , Wiskemann J, , Lanza M, , Milella M , Physical activity for oncological patients in COVID-19 Era: no time to relax, JNCI Cancer Spectrum, 4: (6): pkaa071, (2020) . |
[20] | Rezende LF, , Lee DH, , Ferrari G, , Eluf-Neto J, , Giovannucci EL, Physical activity for cancer patients during COVID-19 pandemic: a call to action, Cancer Causes Control, 32: (1): 1–3, (2021) . |
[21] | Cellini N, , Canale N, , Mioni G, , Costa S, Changes in sleep pattern, sense of time and digital media use during COVID-19 lockdown in Italy, J Sleep Res, 29: (4): e13074, (2020) . doi:10.1111/jsr.13074. |
[22] | Leone MJ, , Sigman M, , Golombek DA, Effects of lockdown on human sleep and chronotype during the COVID-19 pandemic, Curr Biol, 30: (16): R930–R931, (2020) . |
[23] | van Cappellen-van Maldegem SJ, , Mols F, , Horevoorts N, , de Kruif A, , Buffart LM, , Schoormans D , Towards OPtimal TIming and Method for promoting sUstained adherence to lifestyle and body weight recommendations in postMenopausal breast cancer survivors (the OPTIMUM-study): protocol for a longitudinal mixed-method study, BMC Women’s Health, 21: (1): 1–14, (2021) . |
[24] | van de Poll-Franse LV, , Horevoorts N, , van Eenbergen M, , Denollet J, , Roukema JA, , Aaronson NK , The patient reported outcomes following initial treatment and long term evaluation of survivorship registry: scope, rationale and design of an infrastructure for the study of physical and psychosocial outcomes in cancer survivorship cohorts, Eur J Cancer, 47: (14): 2188–2194, (2011) . |
[25] | Buchan DS, , Boddy LM, , McLellan G, Comparison of free-living and laboratory activity outcomes from ActiGraph accelerometers worn on the dominant and non-dominant wrists, Meas Phys Educ Exerc Sci, 24: (4): 247–257, (2020) . |
[26] | Sangha O, , Stucki G, , Liang MH, , Fossel AH, , Katz JN, The Self-Administered Comorbidity Questionnaire: a new method to assess comorbidity for clinical and health services research, Arthritis Rheum, 49: (2): 156–163, (2003) . |
[27] | Kankerregistratie N, Cijfers over Kanker. www.cijfersoverkanker.nl. (Accessed November 9, 2021). |
[28] | Migueles JH, , Rowlands AV, , Huber F, , Sabia S, , van Hees VT, GGIR: A research community–driven open source R package for generating physical activity and sleep outcomes from multi-day raw accelerometer data, J Meas Phys Behav, 2: (3): 188–196, (2019) . |
[29] | Van Hees VT, , Gorzelniak L, , Leon ECD, , Eder M, , Pias M, , Taherian S , Separating movement and gravity components in an acceleration signal and implications for the assessment of human daily physical activity, PLoS ONE, 8: (4): e61691, (2013) . |
[30] | Van Hees VT, , Fang Z, , Langford J, , Assah F, , Mohammad A, , da Silva IC , Autocalibration of accelerometer data for free-living physical activity assessment using local gravity and temperature: an evaluation on four continents, J Appl Physiol, 117: (7): 738–744, (2014) . |
[31] | Rowlands A, , Edwardson C, , Davies M, , Khunti K, , Harrington D, , Yates T, Beyond cut points: accelerometer metrics that capture the physical activity profile, Med Sci Sports Exerc, 50: (6): 1323–1332, (2018) . |
[32] | van Hees VT, , Sabia S, , Jones SE, , Wood AR, , Anderson KN, , Kivimäki M , Estimating sleep parameters using an accelerometer without sleep diary, Sci Rep, 8: (1): 1–11, (2018) . |
[33] | van Hees VT, , Fang Z, , Zhao JH, , Heywood J, , Mirkes E, , Sabia S, GGIR: A research community–driven Open Source R Package for generating physical activity and sleep outcomes from multi-day raw accelerometer data, J Meas Phys Behav, 2(3): 188--196, 2019. doi:10.1123/jmpb.2018-0063. |
[34] | McCarthy H, , Potts HW, , Fisher A, Physical activity behavior before, during, and after COVID-19 restrictions: longitudinal smartphone-tracking study of adults in the United Kingdom, J Med Internet Res, 23: (2): e23701, (2021) . |
[35] | Sweegers M, , Boyle T, , Vallance J, , Chinapaw M, , Brug J, , Aaronson N , Which cancer survivors are at risk for a physically inactive and sedentary lifestyle? Results from pooled accelerometer data of 1447 cancer survivors, Int J Behav Nutr Phys Act, 16: (1): 1–15, (2019) . |
[36] | Kampshoff CS, , Stacey F, , Short CE, , van Mechelen W, , Chinapaw MJ, , Brug J. , Demographic, clinical, psychosocial, and environmental correlates of objectively assessed physical activity among breast cancer survivors, Support Care Cancer, 24: (8): 3333–3342, (2016) . |
[37] | Irwin ML, Physical activity interventions for cancer survivors, Br J Sports Med, 43: (1): 32–38, (2009) . |
[38] | Holmes MD, , Chen WY, , Feskanich D, , Kroenke CH, , Colditz GA, Physical activity and survival after breast cancer diagnosis, JAMA, 293: (20): 2479–2486, (2005) . |
[39] | Kesaniemi YA, , Danforth E, , Jensen MD, , Kopelman PG, , Lefèbvre P, , Reeder BA, Dose-response issues concerning physical activity and health: an evidence-based symposium, Med Sci Sports Exerc, 33: (6): S351–S358, (2001) . |
[40] | Cardinale D, , Bacchiani G, , Beggiato M, , Colombo A, , Cipolla CM, Strategies to prevent and treat cardiovascular risk in cancer patients, Semin Oncol, 40: (2): 186–198, (2013) . |
[41] | Alfano CM, , Lichstein KL, , Vander Wal GS, , Smith AW, , Reeve BB, , McTiernan A , Sleep duration change across breast cancer survivorship: associations with symptoms and health-related quality of life, Breast Cancer Res Treat, 130: (1): 243–254, (2011) . |
[42] | Zhou ES, , Michaud AL, , Owens J, , Recklitis CJ, Sleep in young-adult cancer survivors during the COVID-19 pandemic, J Clin Sleep Med, 16: (11): 1991, (2020) . |
[43] | Janz K, Physical activity in epidemiology: moving from questionnaire to objective measurement, Br J Sports Med, 40: (3): 191–192, (2006) . |
[44] | Demark-Wahnefried W, , Aziz NM, , Rowland JH, , Pinto BM, Riding the crest of the teachable moment: promoting long-term health after the diagnosis of cancer, J Clin Oncol, 23: (24): 5814–5830, (2005) . |
[45] | Mandelblatt J, , Kanetsky PA, Effectiveness of interventions to enhance physician screening for breast cancer, J Fam Pract, 40: (2): 162–172, (1995) . |
[46] | Jones LW, , Courneya KS, , Fairey AS, , Mackey JR, Effects of an oncologist’s recommendation to exercise on self-reported exercise behavior in newly diagnosed breast cancer survivors: a single-blind, randomized controlled trial, Ann Behav Med, 28: (2): 105–113, (2004) . |
[47] | Koczwara B, Cancer survivorship care at the time of the COVID-19 pandemic, Med J Australia, 213: (3)(2020) . 107–108.e1. |