Lectins as a promising therapeutic agent for breast cancer: A review
Abstract
Efficient treatment of cancer has been a subject of research by scientists for many years. Current treatments for cancer, such as radiotherapy, chemotherapy and surgery have been used in traditional combination therapy, but they have major setbacks like non-specificity, non-responsiveness in certain cancer types towards treatment, tumor recurrence, etc. Epidemiological data has shown that breast cancer accounts for 14% of cancer cases occurring in Indian women. In recent years, scientists have started to focus on the use of natural compounds like lectins obtained from various sources to counter the side effects of traditional therapy. Lectins like Sambucus nigra Agglutinin, Maackia amurensis lectin, Okra lectins, Haliclona caerulea lectin, Sclerotium rolfsii lectin, etc., have been discovered to have both diagnostic and therapeutic potential for breast cancer patients. Lectins have been found to have inhibitory effects on various cancer cell activities such as neo-angiogenesis, causing cell cycle arrest at the G1 phase, and inducing apoptosis. The major idea behind the use of lectins in cancer diagnostics and therapeutics is their capability to bind to glycosylated proteins that are expressed on the cell surface. This review focuses on an exploration of the roles of post-translational modification in cancer cells, especially glycosylation, and the potential of lectins in cancer diagnosis and therapeutics.
1.Introduction
Cancer refers to a disease characterized by the uncontrolled proliferation of cells and their spreading into surrounding tissues [1]. Cancer cells are abnormal cells that develop as a result of mutations in the DNA or disruptions in the RNA processing of normal cells [2,3]. Cancer cells often closely resemble normal cells [4] and they can evade detection by the immune system by displaying a T-cell inflamed phenotype, which helps them avoid immune system suppressive pathways [5], by disrupting phagocytic clearance [6], by co-expressing CD200 which is a tolerogenic glycoprotein with stem cell markers [7], by downregulating antigen presentation, which helps in evading immune surveillance and makes them undetectable by antitumor T-cells [8], by reprogramming energy metabolism and changing mevalonate metabolism by activating SERBP-2 and its regulating enzymes [9], by suppressing major histocompatibility complex class 1 antigens [9], and various other mechanisms. Various studies suggest as cells age or become damaged due to various stimuli, they typically undergo apoptosis. But when this process does not take place in a controlled manner, it leads to the accumulation of damaged cells which proliferate and form a colony of their own which can invade and metastasize to other regions [10].
Breast cancer is the most prevalent type of cancer among women, affecting 1 in 29 Indian women [11]. It is alarming that an Indian woman is diagnosed with breast cancer approximately every two minutes and every nine minutes a woman dies [12]. The incidence of breast cancer is increasing in both rural and urban areas of India. According to the Globocan 2020 data, breast cancer constituted almost 13.5% (178,361 cases) of all cancer cases in India and was responsible for 10.6% (90,408 cases) of all cancer-related deaths. Studies project that the worldwide burden of breast cancer is anticipated to surpass nearly two million cases by the year 2030 [13].
It is projected that the disease will affect 1.57 million people in 2025, up from 1.46 million this year, according to a bi-annual report by the Indian Council of Medical Research (ICMR) [14]. The national average of cancer cases for 2022 stands at 100.4 per 100,000 people, with a significant number of women (105.4 per 100,000) being diagnosed with preventable breast cancer [15]. However, the data analysis does not include populous states like Uttar Pradesh and Bihar due to data unavailability [16].
In major cities like Mumbai, Delhi, Bengaluru, Bhopal, Kolkata, Chennai, and Ahmedabad, breast cancer accounts for 25% to 32% of all female cancers, representing more than a quarter of cases [17]. Globally, according to WHO data from 2020, there were 2.3 million women diagnosed with breast cancer, resulting in 685,000 deaths. By the end of 2020, there were 7.8 million women who had been diagnosed with breast cancer within the past 5 years, making it the most prevalent cancer worldwide [18]. Additionally, female breast cancer has surpassed lung cancer as the most commonly diagnosed cancer [19]. Belgium had the highest rate of breast cancer in women in 2020, followed by the Netherlands. In terms of breast cancer mortality, Barbados had the highest rate among women in 2020, followed by Fiji [20].
Various factors like aging, late menopause, early beginning of menstruation, family history of cancer, and genetic mutation increase the risk of breast cancer [21].
Fig. 1.
Depicting the categories based on which breast cancer can be divided.
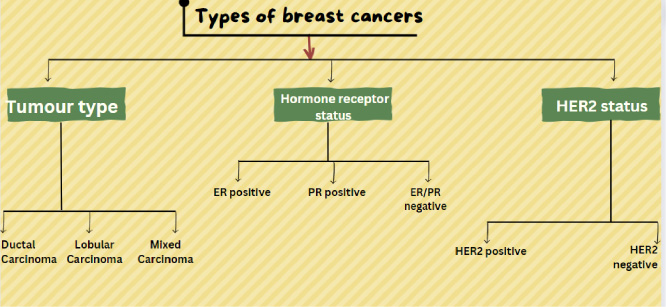
Breast cancer can be classified into three categories (Fig. 1):
Based on tumor type:
• Ductal Carcinoma: Cancer that begins in the epithelial cells lining the lactiferous ducts of the breast, which are responsible for transporting milk.
• Lobular Carcinoma: Cancer that originates in the epithelial cells of the mammary lobules, where milk-producing alveoli are found.
• Mixed Carcinoma: Cancer characterized by a histological combination of features from both ductal and lobular carcinomas, often displaying a mixture of cellular characteristics from these two types [22].
Based on hormone receptor status:
• ER-positive breast cancer: This means that the cancer cells have receptors for the hormone estrogen. When estrogen binds to these receptors, it can promote the growth and division of cancer cells. Breast cancers that are ER-positive are more likely to respond to hormone therapy, which is designed to block the effects of estrogen on the cancer cells. This type of breast cancer is more common among postmenopausal women [23].
• PR-positive breast cancer: This indicates that the cancer cells have receptors for the hormone progesterone. Similar to ER-positive cancer, when progesterone binds to these receptors, it can influence the growth of cancer cells. PR-positive breast cancer is often treated with hormone therapy targeting progesterone receptors [24].
• ER/PR-negative breast cancer: In this case, the cancer cells lack both estrogen and progesterone receptors. This means that hormones like estrogen and progesterone do not significantly drive the growth of these cancer cells. Typicallly, hormone therapy is not typically effective in treating ER/PR-negative breast cancer. Other treatment options, such as chemotherapy or targeted therapies, are often considered for ER/PR-negative breast cancer [25].
Based on HER2 status:
• HER2-positive breast cancer features an abundance of HER2 receptors, which can lead to aggressive cell growth.
• HER2-negative breast cancer lacks an excess of HER2 receptors, generally resulting in a less aggressive cancer [26].
2.Staging of breast cancer
The TNM system, which stands for Tumor, Node, and Metastasis, is a widely used staging approach for breast cancer [27]. The T classification, which ranges from Tis, T0 (no indication of a tumor), T1 (smaller tumor size and has not spread extensively), T2 (intermediate tumor size and has invaded nearby tissues), T3 (larger tumor size with extensive invasion in nearby tissues compared to T1, T2 and T3) and T4 (big tumor or substantial invasion), indicates the size and scope of the original tumor.
Tis or tumor in situ indicates cancer that remains localized, not spreading or invading nearby tissues. It is typically an early stage, making early detection and treatment crucial for better outcomes [28].
With N0 denoting no lymph node involvement, N1 denotes lymph node involvement to a limited number, N2 denotes extensive lymph node involvement indicating spread of cancer beyond initial tumor site and N3 denoting considerable lymph node involvement, including distant lymph nodes. The N category identifies the involvement of neighboring lymph nodes. The M category indicates whether distant metastasis is present or absent; M0 implies the absence of distant metastasis, while M1 suggests the existence of metastatic dissemination [27].
Breast cancer is categorized from stage 0 (non-invasive or in situ cancer) to stage IV (metastatic cancer) by combining the T, N, and M categories giving a more thorough evaluation of the malignancy, the staging method also considers additional elements such as hormone receptor status, HER2/neu status, and histological grade.
Breast cancers can also be classified based on the molecular composition of the tumor cells. One of the most widely embraced molecular classification tool in breast cancer research is the PAM50 (Prediction Analysis of Microarray 50) gene expression assay. This assay assesses the expression levels of 50 genes in a sample of breast tumor and assigns it to one of four intrinsic subtypes: Luminal A, Luminal B, HER2-enriched, or Basal-like [29].
Luminal A: The PAM50 classifier identifies the Luminal A subtype, which is linked to a more favorable prognosis in comparison to other subtypes. The luminal A subtype is defined by the expression of hormone receptors, specifically estrogen receptor (ER) and progesterone receptor (PR), as well as low levels of human epidermal growth factor receptor 2 (HER2) [30]. Individuals with Luminal A tumors typically experience slower tumor growth and have a greater probability of responding positively to hormonal treatments like tamoxifen or aromatase inhibitors [31]. Nonetheless, it is important to note that not all Luminal A tumors exhibit identical clinical characteristics, and when determining treatment options, additional factors such as tumor size, lymph node involvement, and histological grade should also be taken into consideration. The primary components of treatment for luminal A breast cancer encompass surgery, radiation therapy, hormonal therapy, and targeted therapy [32].
Luminal B: The PAM50 classifier identifies the Luminal B subtype, characterized by the overexpression of genes associated with cell proliferation and hormone signaling pathways. Luminal B tumors typically have a higher proliferation rate compared to other luminal subtypes and are associated with a poorer prognosis [33]. Therefore, patients with luminal B breast cancer may benefit from more aggressive treatment strategies, such as chemotherapy, in addition to hormone therapy. This is because luminal B tumors have a higher risk of recurrence and are less responsive to hormone therapy alone [34].
HER-2 enriched: The PAM50 classification system identifies the HER-2 enriched subtype by assessing the expression patterns of genes associated with HER-2 amplification and activation, rendering it a valuable tool for molecular categorization [30]. This classification assists in the identification of individuals who may benefit from precision therapies and provides significant insights into the fundamental biology of HER-2 positive breast cancer. The HER-2 enriched subtype is characterized by the overexpression of the human epidermal growth factor receptor 2 (HER-2) gene [35]. Breast cancers with HER-2 positivity tend to be more aggressive and carry a higher risk of recurrence in comparison to other subtypes. However, the introduction of targeted treatments such as trastuzumab (Herceptin) has notably improved outcomes for patients with HER-2 positive breast cancer [36].
Basal-like: The basal-like subtype, commonly known as triple-negative breast cancer (TNBC) because it lacks the expression of estrogen receptor (ER), progesterone receptor (PR), and human epidermal growth factor receptor 2 (HER2), is associated with an elevated risk of recurrence and metastasis. The PAM50 basal-like molecular classification not only provides insights into the biology of basal-like breast cancer but also has implications for personalized medicine. It helps identify patients who are more likely to benefit from individualized treatments such as chemotherapy or targeted therapies [37].
3.Etiology of breast cancer
Although the cause of breast cancer is not much of a well-understood concept [38]. With the help of epidemiological studies, researchers have been able to identify some key factors that increase the chances of a person or make them more susceptible to breast cancer. The understanding of the root cause or the factors that contribute to the occurrence of breast cancer is of utmost importance when a breast cancer susceptible gene is present in an individual and preventive measures must be understood and taken by them to avoid the occurrence of the disease.
Some of the factors which are known to cause cancer are as follows:
Genetic clustering: The evidence of genetic clustering dates to 100AD in the medical scriptures of Rome [39]. It has been suggested, after taking into consideration various medical evidence, that a woman’s chance of getting breast cancer increases when she has a first-degree relative who has a history of breast cancer. This probability of occurrence of the disease increases more when two first-degree relatives show involvement with breast cancer. The risk was known to be higher when the occurrence of cancer was before menopause and the disease was bilateral [39,40]. It has also been identified can be transmitted through both maternal and paternal lines in an autosomal dominant pattern. Not much has been further studied to understand the pattern, Usually, the risk associated with cancer is related to the maternal line, but the paternal line inheritance is known to elevate it [41].
Intrinsic factors: A womans age at which the integral processes took place has also known to affect the occurrence of breast cancer [42]. Chances of getting breast cancer are known to increase if a woman has an early menarche, has never given birth, gave birth to a child after entering her 30s, or got her menopause late [43]. In all these cases, the breast tissues will be exposed to estrogen for a longer time, thus increasing the risk [44].
Obesity: Many studies have shown that obesity contributes to the occurrence of breast cancer. In the case of obese people, the formation of 2-OH estrogenic compound is much less that causing an increase in the effect of estrogen [45]. It has also been stated that the people who have high-fat consumption and obesity have reduced amounts of globulins (sex hormone-binding globulin (SHBG) Corticosteroid-binding globulin (CBG)) to which the sex hormones bind resulting in a higher amount of sex hormones circulating freely thus highly available to exert their effect on tissues of the breasts [46].
Radiation: Breasts are very sensitive organs to any kind of stimuli. The high sensitivity of breast tissue to radiation is because of its numerous dividing cells and high metabolic rate. It is crucial to grasp the impact of radiation on breast tissue and the potential risks it poses. Thus, exposing breasts to radiation for a longer period and or to a higher frequency can be damaging to them and can result in mutations which can ultimately increase the risk of breast cancer [47].
Contraceptives: They are known to contain synthetic forms of hormones of a female. Estrogen and progesterone act as promotors of the growth of cancer cells. Consuming birth control pills, causes increased exposure to hormones in the body thus increasing the risk of breast cancer [48].
Dietary factors: Although the relationship between diet and the incidence of breast cancer is not well understood it has been identified that consumption of higher amounts of fat increases the risk of breast cancer [49]. Also, the people who consume a good amount of omega-3 i.e., ∼1.1g per day for adult females and ∼1.6g for adult males have a lower risk of getting the disease [50,51]. Consumption of reduced carotene and fibers has been shown to increase the chances of occurrence of cancer [52].
4.Current cancer treatments
Some of the current cancer treatments include:
Chemotherapy: Certain anti-cancer agents such as cyclophosphamide, methotrexate, 5-fluorouracil, vinorelbine, and doxorubicin are used to treat breast cancer [53]. They may be used to cure the cancer using combination therapy or to suppress the symptoms (depending on the case). Along with the therapeutic effects chemotherapeutics agents also exhibit adverse side effects like immunosuppression, myelosuppression, neutropenic enterocolitis, diarrhea, anemia, vomiting, nausea, loss of hair, and in some cases, development of secondary neoplasm. [54–61]
Immunotherapy: This therapy is employed to make our immune system strong enough to fight cancer [62]. Immunotherapy can cause some side effects like it can cause Immune-Related Adverse Events (irAEs) [63], Cytokine Release syndrome [64], and allergic reactions [65]
Surgery: The part where the cancer cells proliferate is removed from the body [66]. It can cause side effects like pain, swelling and bruising, scarring, infection, bleeding, nerve damage, impaired function, lymphedema, digestive problems, respiratory issues, blood clots, adverse reactions to anesthesia and delayed healing [67].
Stem cell transplant: The stem cells are taken from the matching donor; the stem cell converts into blood cells and replaces the blood cells that were lost due to radiation or chemotherapy [68]. Some side effects of this are Graft-Versus-Host Disease (GVHD), infection, organ damage, bone health, and gonadal dysfunction [69].
Radiation therapy: The size of the tumor shrinks and the tumor cells are killed due to the high dose of radiation in this therapy [70]. Some of the side effects of radiation therapy are mucositis, xerostomia, dermatitis, fatigue, and osteoradionecrosis [71].
Hormone therapy: Some cancers such as prostate cancer and breast cancer require hormones to grow, this therapy either stops the tumor growth or slows it down [72]. The side effects are hot flashes, loss of libido, breast tenderness or growth, decreased muscle mass, weight gain, memory and cognitive changes, and increased risk of cardiovascular disease [73].
Photodynamic therapy: Drugs which are sensitive to light and gets activated by them to kill the tumor cells [74]. Some of the side effects of Photodynamic therapy are Skin Sensitivity and sunburn, pain or discomfort, swelling and inflammation, hypersensitivity reactions, and pain or discomfort during light exposure [75].
Hyperthermia treatment: In this treatment the body tissue that has cancer is heated up to 45 degree celcius to kill the tumor cells without effecting the function of normal cells present in the tissue [76]. Side effects of this treatment are redness, blistering, or burns, pain and discomfort, nausea and vomiting, dehydration, blood pressure changes and muscle or joint pain [77]
Targeted cell therapy: In this, the changes in the tumor cells that help them to proliferate and mature are targeted [78]. Side effects of this treatment are hair loss (alopecia), nail changes, including nail dystrophy, paronychia, or onycholysis, skin discoloration, and Hand-Foot Skin Reaction (HFSR) [79].
Cryoablation- The tumor cell tissue is frozen with the help of the gas and then the tissue is thawed, this step is done multiple times to kill the tumor cells [80]. Some of the side effects of this treatment are urinary symptoms, erectile dysfunction, pain or discomfort, and swelling and bruising [81].
5.Protein modification and its role in cancer
Protein modification refers to the protein structure alteration after its translation, i.e., post-translational modification (PTM) of proteins. These modifications are important because they help in various functions required in regulating cell homeostasis, cell signaling, etc. Several types of PTMs that happen in our body are phosphorylation, glycosylation, acetylation, methylation, and ubiquitination [82]. These modifications help the cells to cope with stresses like oxidative stress or DNA damage [83]. PTMs can modify the protein-protein interactions by altering the existing binding sites so that a specific molecule cannot bind to cells or creating binding sites having affinity to some molecule [84]. This can happen by modifying the signal transduction pathway or the gene regulating the receptor present on the cell surface [85].
Ubiquitination: An important post-translational modification which helps in maintaining the activity of protein. In this process, ubiquitin molecules are attached to amino acids like lysine and degrade the damaged protein molecules. So, it also helps in clearing the accumulated protein debris [86]. Dysregulation in ubiquitination plays a significant role in promoting breast cancer by regulating the function of oncogenic proteins. One such example is the oncogenic protein c-Myc (also known as cellular myc), which can be ubiquitinated at specific lysine sites. This ubiquitination process increases c-Myc’s stability and activity, resulting in elevated c-Myc levels observed in various cancer types, including breast cancer. This increase in c-Myc levels is associated with enhanced cell proliferation, angiogenesis, and tumor development. Similarly, the oncogenic protein HER2 also undergoes ubiquitination, which can enhance its activity and contribute to the growth of cancerous tumors [83].
Phosphorylation: It is the process in which a phosphate group is added to a protein molecule to change its structure and function with the help of enzyme kinase. It is a reversible modification and usually occurs in amino acids like serine, threonine, and tyrosine. This process is very crucial in signaling pathways to activate or deactivate the cascade. Likewise, the phosphatase enzyme is responsible for removing the phosphate group (i.e., dephosphorylation). In almost 30% of the cells phosphorylation occurs, so, any mutation in a protein phosphate site can lead to cancer and other diseases [87,88]. Many signaling pathways like PI3K/AKT/mTOR pathway, and tyrosine kinase pathway which play an important role in maintaining the cell cycle need the phosphorylation process [89]. In cancer cells these pathways are unregulated and faults in the phosphorylation process are a reason for it. The progression and inhibition of breast cancer are significantly related to the phosphorylation of upstream and downstream regulatory factors of nitric oxide (NO) [90].
Acetylation: Acetylation is the process in which the acetyl group is added to the amino acid residues. Histone acetylation is one of the most crucial processes that occur in a cell. Acetyl, with the help of the acetyltransferase enzyme gets attached to the lysine residue present in the histone (binding protein) and gives a positive charge to the molecule. The electrostatic force between the positively charged histone and negatively charged DNA helps in loosening up the chromatin providing easier access for the DNA repair process to occur. Acetylation dysregulation can cause cancer because of a disrupted DNA repair process which can lead to the accumulation of damaged DNA and leading to genomic instability and over time it can progress into cancer. Recent studies have shown that the use of histone deacetylase (HDAC) can help in the re-expression of Estrogen receptors in Triple negative breast cancer in which there is an absence or low level of ER due to gene alteration [91]. With the help of this theory, the TNBC patient can be treated with hormonal therapy by re-expressing estrogen receptors.
Glycosylation: Glycosylation is a major co- & post-translational modification of proteins that is primarily catalyzed by glycosyltransferases. It is important as it leads to protein folding by acting as molecular chaperones and preventing faulty protein folding and degradation. Glycan protein also helps in transferring the protein molecules through the cell membrane and to their destined location where they are needed- sorting of protein [92]. Glycan protein attached to receptors present on the cell surface helps in more specific binding of ligands. Protein glycosylation changes alter the roles of proteins involved in cell-cell interactions that lead to tumor cell dissemination & local invasion, immune response regulation, and growth regulation in a variety of organ niches. In drug sensitivity cases, the role of glycoproteins is very prominent as the altered glycosylation of the ATP binding cassette transport proteins has been linked to drug-resistant tumor cells shown by many patients of TNBC who suffered a short relapse due to ineffective chemotherapy [93].
Glycosylation is altered during the progression and metastasis of breast cancer. Previous research into the relationship between both the malignant behavior of TNBC cells & glycosylation yielded some intriguing results. According to Zhou and colleagues [94], bindings of the RCA-I (Ricinus communis agglutinin I) to TNBC cell lines were found to correlate with their ability to metastasize, as well as RCA-I inhibited many processes in TNBC cells like cell adhesion, cell migration and invasion with high metastatic capacity. The mechanism of RCA-I lectin in the inhibition of cell adhesion, metastasis and invasion is not fully understood. Although it has been speculated by Zhou and colleagues that RCA-I inhibits the motility of TNBC cells by blocking the metastasis- associated surface glycans. It is worth noting that the majority of previous studies on this topic were conducted in vitro.
During glycosylation, sugars are enzymatically attached to specific amino acid residues in the protein sequence. The sugar moieties can be attached to the protein either through N-linked glycosylation, where the sugar is linked to the nitrogen atom of an asparagine residue, or through O-linked glycosylation, where the sugar is linked to the oxygen atom of a serine or threonine residue [95].
Breast cancer is a complex disease characterized by the uncontrolled proliferation of cells in breast tissue. Alterations in glycosylation have been identified as associated with breast cancer progression and metastasis, influencing multiple aspects of tumor biology [96].
Lectins can bind to the carbohydrate moiety which is present in the form of glycoprotein and glycolipids on the cell surface. As we know, altered glycosylation of the protein molecules (e.g., receptors) present on the cancer cell surface leads to cancer cell adhesion to extracellular matrix (ECM) or avoids immune responses through alteration of the stability of ligands of immune checkpoint receptors which results in cancer progression [97]. So, understanding the binding of lectins with different glycoproteins can help in treating cancer.
Some lectins like SNA and MAA have been found to identify the glycoproteins which are present in the breast cancer cells and agglutinate them by forming linkage with the sialic acid (that is the part of glycoprotein on the cancer cell surface) (Fig. 2) [98,99]. Sialic acid is overexpressed (hyper sialylation) in the tumor tissues in ovarian cancer, breast cancer, colorectal cancer, and leukemia in comparison to normal cells of these organs [100,101]. So, if there are natural compounds that can attach themselves to sialic acid and precipitate the glycoconjugates then this phenomenon can be used in targeting cancer cells. Lectins are not only able to detect cancer cells but also show anti-tumor effect (Table 1).
6.Role of glycosylation in breast cancer
The process of glycosylation has been known to be an important benefactor in various processes associated with cancer progression which includes invasion, progression, and metastasis [102]. It is interesting to know that most biomarkers that are identified in cancer include glycans and glycoproteins which thus show a significant role in cancer diagnosis and prognosis [105]. Although the exact sequential process of production of these modified glycans (e.g., mucin integrins and CD44) through the glycosylation process occurs is not well understood but can be due to mutations in genetic or epigenetics, non-regulated expressional processes [103]. Some studies even referred to glycosylation as a “hallmark of cancer” [104].
Fig. 2.
Binding of MAA and SNA lectin with sialic acid.
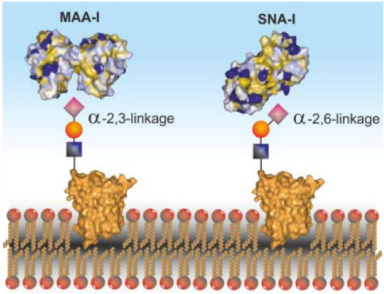
Changes in glycosylation patterns have been shown in breast cancer cells and can contribute to tumor growth and metastasis. These changes in glycosylation patterns have been found in breast cancer cells.
Assisting in signaling for proliferation: Cancer cells can multiply enormously, and it has been identified that glycosylation plays an integral role in providing the essential signals required for uncontrolled division of cells [106]. The management of the proteins that have importance in cell cycle continuation like c-MYC (Cellular myelocytomatosis oncogene) is known to be controlled by the modification of O-GlcNAc [98]. When there is an increase in the expression of modified c-MYC, it can affect the phosphorylation process stabilizing the modified protein [107]. Various growth factors like EGFR (epidermal growth factor receptor), and PDGF (platelet-derived growth factor) have also been identified that are managed by N- glycan modifications. Thus, glycosylation also controls signaling for growth factors [98].
ECM acts as an interface that contains various non- cellular components that support the various growth-related signaling processes that are taking place in the nearby cells. [108]. The modification of CD44 and regulation of growth factors dependent on integrin are also attributed to the glycosylation process that results in uncontrolled cell growth [109,110].
Escaping suppression of growth: The two of the most important tumor suppressors, p53 and retinoblastoma are also known to contain regions for glycosylation modification of O-GlcNAc or phosphorylation that is suspected to turn on their oncogenic activity resulting in the production and promotion of cancer cells [105].
p53: The p53 protein is frequently referred to as the “guardian of the genome” because of its critical function in preserving genomic integrity [111]. When p53 is activated, it acts as a transcription factor when DNA damage occurs. It promotes the production of genes related to apoptosis, DNA repair, and cell cycle arrest [112]. p53 encourages cell cycle arrest at the G1 phase in response to DNA damage, giving time for DNA repair mechanisms to rectify the damage before replication. To remove cells with potentially detrimental genetic defects where the DNA damage is too severe to be repaired, p53 causes apoptosis. However, p53 gene alterations can result in a defective p53 protein which can cause cells with damaged DNA to continue replication as mutated p53 is less efficient at causing cell cycle arrest or death. These cells may develop into cancer because of the accumulation of genetic anomalies [113]. Glycosylation of p53 can either enhance or inhibit its function, depending on the glycan structures and attachment sites. Numerous studies have demonstrated that glycosylation can enhance p53’s stability by protecting it from degradation through ubiquitin-proteasome pathways, resulting in increased p53 levels that bolster its role as a tumor suppressor. Moreover, aside from stabilizing p53, glycosylation can influence its localization within cells. Specifically, glycosylation at certain sites promotes the nuclear localization of p53, enabling more efficient regulation of gene expression and DNA repair processes. Conversely, alterations in glycosylation patterns can lead to p53 being retained in the cytoplasm or mis-localized in other cellular compartments hampering its ability to carry out its functions. Additionally, glycosylation can affect p53’s transcriptional activity, with certain glycans enhancing its DNA-binding affinity and transcriptional activation potential. These modifications enable p53 to bind more effectively to target genes and initiate processes related to cell cycle arrest or apoptosis. Breast cancer often exhibits disrupted p53 function. This disruption is frequently attributed to mutations in the TP53 gene(one of the most common genetic alterations associated with breast cancer). However, in addition to genetic mutations, changes in p53 glycosylation have also been linked to breast cancer progression. Research has shown that abnormal glycosylation patterns of p53 are connected to the development and aggressiveness of breast cancer. These alterations influence p53’s stability, cellular location, and transcriptional activity, resulting in disrupted cell cycle regulation and impaired DNA repair mechanisms. These changes contribute to the accumulation of genetic mutations and genomic instability, which are characteristic features of breast cancer [114]. In conclusion, p53’s carcinogenic activity entails the loss of its ability to repair DNA and govern the cell cycle because of mutations, allowing cells with damaged DNA to multiply and accumulate more genetic abnormalities.
Retinoblastoma (RB): The RB protein controls how cells move from the G1 phase to the S phase of the cell cycle. It suppresses the growth of tumors by preventing the E2F family of transcription factors from acting [115]. Genes involved in DNA synthesis and cell division are activated by E2F proteins. When the RB protein is in an active state act as an inhibitor for E2F proteins. RB enables regulated progression through the cell cycle by controlling E2F. Cells cannot reach the S phase unless they have received the correct signals and have replicated their DNA. E2F is no longer properly controlled when RB protein is inactive or under-expressed because of mutations or genetic changes. Unchecked E2F activity results in uncontrolled cell cycle progression and enhanced cell proliferation, which increases the risk of tumor development in the afflicted cells. The loss or inactivation of RB’s E2F inhibitory function, which results in unchecked cell cycle progression and enhanced cell proliferation, is what causes it to become carcinogenic [116]. RB glycosylation primarily targets specific asparagine residues, impacting its stability, cellular localization, and interactions with other proteins. It can enhance RB stability by safeguarding it from degradation and influencing its movement between the nucleus and cytoplasm, affecting its role in transcription. Moreover, glycosylation affects RB’s interactions with proteins involved in cell cycle control, like cyclins and cyclin-dependent kinases. Changes in RB’s glycosylation disrupts these interactions, impairing its ability to inhibit cell cycle progression. In breast cancer, dysregulated RB function is linked to the disease’s development and progression, often involving altered glycosylation patterns. Aberrant enzyme expression can modify RB glycosylation, leading to changes in stability and function. Additionally, shifts in carbohydrate structures on RB have been observed in breast cancer cells compared to normal tissue, impacting its localization and interactions, ultimately affecting its ability to regulate cell cycle progression and promote tumor growth [117].
Reducing cell energy processes: Cancer cells are known to exhibit the Warburg effect wherein; the cells shift their metabolic processes from oxidative phosphorylation to aerobic glycolysis [118]. As Cancer cells need to multiply rapidly and they need to have an increased metabolism and high energy production, this shifting is identified by a high glucose release, thus providing cancer cells with what they need. With the processes like hexosamine biosynthetic pathway, the high amount of glucose present causes an increased glycolysis and flux. The end product of this pathway is UDP-GlcNAc, an essential metabolite for the O-GlcNAc in both N and O liked modifications [120]. O-GlcNAc is identified as a “nutritional sensor” that helps regulate the nutrition processes and is capable of altering different metabolic enzymes, hence resulting in changes in metabolism [119].
Glycolysis: O-GlcNAc modification can enhance glycolysis. Increased O-GlcNAcylation of glycolytic enzymes, such as hexokinase and phosphofructokinase, promotes their activity and facilitates the diversion of glucose towards glycolysis rather than oxidative phosphorylation [121].
Pentose phosphate pathway (PPP): O-GlcNAcylation regulates the PPP, an alternative pathway of glucose metabolism that generates nucleotides and reduces equivalents. Increased O-GlcNAcylation of key enzymes in the PPP, such as glucose-6-phosphate dehydrogenase, enhances their activity and promotes the diversion of glucose towards PPP [122].
Lipid metabolism: O-GlcNAcylation affects lipid metabolism by regulating enzymes involved in fatty acid synthesis and oxidation. Increased O-GlcNAcylation of acetyl-CoA carboxylase promotes fatty acid synthesis, while decreased O-GlcNAcylation of carnitine palmitoyl transferase I inhibit fatty acid oxidation [123].
Amino acid metabolism: O-GlcNAcylation influences amino acid metabolism by modulating enzymes involved in the synthesis and degradation of amino acids. For example, increased O-GlcNAcylation of glutamine-fructose-6-phosphate transaminase 1 enhances glutamine metabolism, which is important for cancer cell growth and proliferation [124].
Signaling pathways: O-GlcNAc modification can modulate various signaling pathways involved in cancer progression, such as the PI3K/Akt/mTOR pathway and the AMP-activated protein kinase (AMPK) pathway. O-GlcNAcylation of key proteins in these pathways affects their activity and downstream signaling events [125].
Helping cells to escape death: Under normal circumstances, cells are prevented from multiplying uncontrollably through apoptosis [126]. The intercellular signaling pathways to control the process of apoptosis are significantly regulated by glycans [127]. With the help of glycosylation glycans present on the apoptosis, receptors can be altered to become resistant to cell death signals [127]. Below are a few examples of how glycosylation helps in the regulation of apoptosis:
Apoptosis mediated by Fas/FasL: The Fas receptor (CD95) and its ligand (FasL) are essential for the initiation of apoptotic signaling [128]. Fas and FasL can be glycosylated to alter their interactions and the susceptibility of cells to apoptosis. The amplitude of the apoptotic signal might vary depending on changes in the binding affinity between Fas and FasL due to changes in glycosylation patterns [129].
Glycosylation of apoptotic receptors: Upon ligand binding, apoptotic receptors like as TNF receptor 1 (TNFR1) and TRAIL receptors are responsible for conveying apoptotic signals. The stability, location, and signaling effectiveness of these receptors can all be impacted by glycosylation. Apoptosis signaling can become dysregulated because of altered glycosylation patterns, which can affect the assembly of receptor complexes [130].
Anti-apoptotic protein glycosylation: Glycosylation can change the stability and function of anti-apoptotic proteins like the Bcl-2 family of proteins. Alterations in glycosylation patterns can impact the ratio of pro- and anti-apoptotic proteins, which in turn affects how likely it is for cells to undergo apoptosis [131].
Caspases glycosylation: Caspases are proteases that are crucial to the execution of apoptosis [132]. Caspases’ capacity to begin and spread apoptotic signaling can be affected by glycosylation, which can alter their activity and function [98].
Activation of immortality in cells-Normally the cells undergo senescence that happens due to the shortening of telomeres, but cancer cells can escape the senescence [133]. This happens due to the transcriptional reactivation of the human telomerase reverse transcriptase(hTERT). To date the direct effect of glycosylation in the activation if the telomerase has not been identified but it is thought that glycosylation has an indirect effect on the telomerase through c-MYC. The activation of telomerase is known to be mediated by c-MYC which is known to activate the hTERT gene [135]. As the c-MYC gets glycosylated and is modified, the telomerase activation is also induced through O-GlcNAc modification which has frequently been observed in many cases of cancer [134].
7.Lectins as diagnostic and therapeutic agents
Lectins are proteins capable of binding to specific sugar structures present on the surface of cells, thereby influencing cell signaling, adhesion, and immune responses [136]. Following are some of the lectins that have been studied for their effects in cancer.
Okra lectin: Abelmoschus esculentus, popularly known as okra is a member of Malvaceae, first originated in Africa. It is mostly used as a food source but also has a significant role in traditional medicines for dysentery, urinary infections, constipation, and many more disorders [137]. Although the medicinal properties of the okra fruit have been known for a while, it is only a recent discovery that lectins were isolated from the seeds of this plant and not much has been studied about this lectin. This lectin affects the cell proliferation of breast cancer cells by inhibiting it (up to 63%). It also increases the expression of pro-caspase-9 and pro-caspase-3 proteins both of which are important in the intrinsic or mitochondrial pathway of apoptosis of cells. Also, it is known to increase the BAX/BCL-2 ratio which also plays a significant role in the apoptosis process [138].
Thus, the effect that is produced by the lectin isolated from okra is due to inhibition of cell growth and apoptosis.
Helilectin-3: The cancer treatment is significant when it acts on the cancer cells and at the same time does not cause any effect on the normal cells. This is what is the mode of action of this lectin which is isolated from a marine sponge called Haliclona caerulea [139]. It is seen that this lectin on its first encounter with the cancer cells has been known to reduce the viability of cells and not produce any effect on normal and healthy cells. When this lectin is allowed to be incubated with the cancer cells for more than 24 hours, it causes an arrest in the cell cycle at the G1 phase. Further, there is a remarkable increase in the expression of caspase-9 protein which is an important part of the intrinsic or mitochondrial pathway of apoptosis of the cell. Helilectin-3 has a negative effect on the adhesiveness of the breast cancer cells which is important for cell proliferation and their survival in the body. It is known to impair the adhesion capability of the cancer cells. This lectin is also known to cause autophagic death of the cancer cell due to the increased expression of microtubule-associated protein light chain 3(LC3) with an observable conversion of LC3-I to membrane-bound LC3-II which is an autophagic death marker [140].
Thus, the effect produced by helilectin-3 on breast cancer cells is due to contribution of both apoptotic and autophagic death.
Sclerotium rolfsiilectin:Sclerotium rolfsii lectin (SRL) is obtained from a pathogenic fungal source. SRL has been found to recognize and specifically bind oncofetal Thomsen-Friedenreich (Galβ1-3GalNAc-O-ser/thr, or TF) related O-linked glycan on the tumor cell surface [141]. For the betterment of cancer patients, research has been going on to find an alternative mode of action for treatment than chemotherapy and chemoprevention. In this regard, SRL has shown many properties that can be useful in combating tumor cells [142]. In many cancer therapies, we observe that along with tumor cell destruction, some normal cells are also affected which might be detrimental to the patients body. Surprisingly SRL was found to have a more apoptotic effect on the tumor cells of benign and metastatic cancer rather than the normal mammary cells. So SRL can be used in the targeted therapy, and it is obtained from a natural source can decrease the side effects of the treatment on the body [143]. It is to be noted that although some of the chemotherapy drugs are also obtained from natural sources it is still more toxic than SRL. It is because of pharmacokinetics i.e. These medications are typically given systemically, either through oral ingestion or intravenous delivery, enabling them to travel throughout the body. This broad dissemination enhances the chances of them coming into contact with normal cells and tissues. These drugs’ therapeutic index is also very narrow so even a slight accumulation of these drugs can cause lethal effects on the body. And SRL with its highly specific nature will not cause this issue thereby decreasing its toxicity level [144].
In recent studies, it has been found that SRL was inhibiting the neo-angiogenesis which will prevent cancer cells from getting sufficient nutrients for their growth, resulting in a decrease of tumor cell proliferation. After performing an adhesion assay, it was found that SRL has adhesive property that is it has significantly decreased the adhesion of tumor cells to fibronectin and collagen of ECM [145].
On a mice model that had MCF-7xenografts, SRL efficacy was observed. It was found that SRL was inhibiting tumor proliferation [142].
Helix pomatia: Helix pomatia agglutinin (HPA) obtained from the Roman snails is part of the carbohydrate-binding protein family. It specifically binds to N- acetyl galactosamine (called GalNAc). Glycan arrays were done to find the lectin specificity of the compound so that its use as a cancer marker can be explored [145].
A study was done in which HPA and c-erbB-2 oncoprotein were used to diagnose the patient and try to make an appropriate future treatment plan. It was seen that the levels of HPA staining, and c-erb-2 expression were associated with the axillary lymph node metastasis in breast cancer patients. Their level was used to predict the incidence of axillary lymph node metastasis as well as the tumor size. This may help in generating a better treatment plan in patients who did not have the dissection of the axillary lymph node, the levels of HPA and c-erb-2 can be checked, and they can be put on aggressive therapy to prolong their survival rate [146].
MAA: The plant Maackia amurensis produces a lectin called Maackia amurensis agglutinin (MAA), which is found in its seeds. The possible involvement of MAA in the diagnosis, prognosis, and therapy of breast cancer has been investigated [147]. Compared to normal cells, breast cancer cells frequently have modifications to their glycosylation patterns. A kind of glycan, sialic acids, can change in expression or structure because of these modifications.
The agglutination test used by MAA particularly detects 2,3-linked sialic acid and aids in the identification and characterization of these glycan changes. Increased tumor aggressiveness and metastatic potential have been linked to the presence of 2,3-linked sialic acid glycans in breast cancer cells. Increased MAA agglutination reactivity has been linked in studies to a worse prognosis, which includes a greater tumor grade, lymph node involvement, and lower overall survival rates [148,149]. MAA has also been explored as a potential therapeutic tool in breast cancer treatment. But the research for this is still on going and not completely established.
Mistletoe Mistletoe species like Viscum album var. coloratum (Korean mistletoe) is one of the most significant medicinal plants in anthroposophical medicine and can treat cancer. Because of its few adverse effects and the fact that they are not life-threatening, mistletoe extract is advised for the treatment of cancer, particularly breast cancer [150]. There have been a few reports of allergic responses. Mistletoe extract was first used in oncology as a novel form of cancer treatment by Rudolf Steiner, the developer of the anthroposophical medical system, in 1920 [151].
Despite being one of the more debatable lectins when it comes to cancer treatment, extracts of lectins from mistletoe plant species have been extensively examined due to their vast efficiency on a range of neoplastic cells [152]. Numerous studies demonstrate that mistletoe lectins can either promote or inhibit apoptosis depending on their concentration [153,154]. After treatment with the lectin-containing mistletoe extracts ISCADOR, tumor growth in glioblastoma xenograft-bearing mice was inhibited, and genes associated with glioblastoma progression and malignancy, such as transforming growth factor- and matrix-metalloproteinases, displayed downregulation [155]. Mistletoe lectin I, II, and III make up the majority of mistletoe lectin extracts, while further research is needed to fully understand their variances [156].
Viscum album var. coloratum agglutinin (VCA), has been shown in tests to have beneficial effects on human breast cancer cells. The VCA lectin and doxorubicin (DOX) combination produced even more potent results for programmed cell death, but DOX’s therapeutic applications are constrained because of its hazardous side effects, which include cardiotoxicity and myelosuppression. The use of VCA and DOX together led to the stimulation of apoptosis-inducing proteins like Bax and Puma and the suppression of Bcl-2 [157].
In both SK-Hep-1 (p53-positive) and Hep 3B (p53-negative) human hepatocarcinoma cells, the Korean mistletoe lectin (Viscum album L. coloratum agglutinin) induced apoptosis through p53- and p21-independent pathways by downregulating Bcl-2 and telomerase and upregulating Bax acting upstream of caspase-3 [158].
C-type lectin-Lebectin: Lebectin is the C-type protein isolated from the venom of Macrovipera lebetina. C-type lectin-like proteins from the viper venom also known as the snaclecs do not have the sugar -calcium binding loops which are generally found in C-type lectin proteins [159]. Lebectin has 129 amino acids in the α subunit and 131 amino acids in the β subunit. Snaclecs are known to have anticancer effects [160]. Lebectin also shows the anti-aggregation activity on the platelets. In the MDA-MB-231, which is a cell line of triple-negative breast cancer that is the subtype of breast cancer, Lebectin blocks the cell migration in a dose-dependent manner and also blocks the fibronectin and fibrinogen-dependent adhesion but does not affect the viability of the cells. Lebectin can block α5β1 integrins. Hence, Lebectin prevents the proliferation, adhesion, invasion, and migration of cancer cell lines [161,162].
Sambucus nigra Agglutinin (SNA): SNA lectin is extracted from the elderberry plant, Sambucus nigra. It is isolated from the seeds or bark through a process involving crushing and purification [163].
In breast cancer, SNA lectin may induce changes in glycosylation, the addition of sugar molecules to proteins or lipids on the cell surface, potentially leading to significant effects on cancer progression [164]. For example, Lomax-Browne et al. conducted a study where they examined the variations in glycosylation of IgA1 in the serum of both healthy volunteers and patients with breast cancer. They employed several well-established commercial lectins, namely HPA, SNA, and MAL-II, for their analysis. The study revealed a significant alteration in N-glycosylation, particularly a notable increase in disialo-biantennary N-glycans of IgA1 in breast cancer patients [165]. These alterations in glycosylation could impact various crucial aspects of breast cancer development and behavior [166].
Firstly, changes in cell adhesion may occur, affecting how cancer cells stick to each other and the surrounding extracellular matrix. Altered adhesion properties could, in turn, influence tumor growth, invasion, and the formation of metastases [167]. Secondly, modifications in glycosylation may interfere with important signaling pathways within cancer cells. These disruptions could lead to the activation of pro-cancer pathways or the inhibition of tumor-suppressive pathways, ultimately influencing cancer cell behavior and survival [168]. Thirdly, the interactions between cancer cells and the immune system could also be affected. Changes in glycosylation patterns caused by SNA lectin binding might allow breast cancer cells to evade immune surveillance and avoid destruction by immune cells, potentially contributing to tumor immune evasion [169]. Furthermore, glycosylation changes could play a role in the metastatic process of breast cancer. The migration and invasion of cancer cells to distant organs are essential steps in metastasis, and alterations in glycosylation may impact
these metastatic properties [170].
Table 1
Summarizing the source and purpose of different lectins
No. | Name | Source | Use | References |
1 | Okra lectin | Plant source- Abelmoschus esculentus | inhibit cell proliferation, increase gene expression for apoptotic proteins | [137,138] |
2 | Haliclona caerulea (Helilectin-3) | Marine sponge- Haliclona caerulea. | causes cell arrest of cancer cells at the G1 phase, doesn’t affect surrounding cells, promotes autophagic death of cells, reduces cell adhesion | [139,140] |
3 | Sclerotium rolfsii lectin (SRL) | Pathogenic fungus | inhibit neo-angiogenesis, anti-adhesive properties, inhibit tumor proliferation | [142,143] |
4 | Helix pomatia | Roman snail | promote apoptosis, downregulate telomerase enzyme | [145,146] |
5 | Maackia amurensis agglutinin (MAA) | Maackia amurensis | used for diagnostics-cancer marker to stage and grade breast cancer | [147–149] |
6 | Viscum album var. coloratum agglutinin Viscum album L. coloratum agglutinin | Mistletoe | Used to detect tumor stage and grade, related to tumor aggressiveness in breast cancer. | [157,158] |
7 | Lebectin | venom of Macrovipera lebetina | inhibits cell migration, anti-adhesive property inhibits invasion of cancer cell | [159,161,162] |
8 | Sambucus Nigra Agglutinin (SNA) | Elderberry Plant | Affects cell adhesion, and disrupts the signaling pathway of cancer cells. | [163,168] |
However, it is crucial to emphasize that the specific role of SNA lectin in breast cancer is still an area of active research, and much remains to be elucidated [171]. Researchers continue to investigate the intricacies of lectin interactions in breast cancer cells and the implications of glycosylation changes in cancer progression [172]. A comprehensive understanding of SNA lectins role in breast cancer may potentially open up new avenues for targeted therapies and interventions to combat this devastating disease [173].
8.Conclusion
Lectins have been studied recently for identification of biomarker for prognosis and effective treatment of choice for clinicians. Natural compounds are found to be more beneficial in cancer treatment because of their ability to work synergistically with other treatment methods, enhancing efficacy, reducing side effects and provide a source of novel compounds with ability to modulate mechanisms such as apoptosis, inhibiting proliferation and metastasis. [174–176] Lectins have been found to have anticancer properties like promoting apoptosis of cancerous cells, decreasing the adhesiveness of cancer cells, maintaining the cell proliferation rate by triggering cell arrest in the G1 cycle. Lectin can also be used as an efficient cancer diagnostic tool. In short, lectins can disrupt the aberrant cell signaling pathways involving glycosylation inhibit cancer cell growth, or trigger the immune cells to detect and kill the tumor cells. Recent studies are more focused on finding treatment options for few types of aggressive breast cancer due to the absence of ER/PR receptors or HER2 protein. Glycoprotein-targeted lectin therapy is more advantageous in terms of specificity as well and may not have a problem of drug resistance in the body. With more research on elucidating lectin’s potential in diagnostics and novel therapies may lead to discovery of novel target molecules for effective therapy of relapse prone triple negative breast cancer.
Acknowledgements
The authors state that they don’t acquire any financial aid, grants, or other support for the preparation of this paper.
Author contributions
Manuscript preparation- Keerti Singh, Lokita Agrawal, Rhea Gupta; Formal analysis and investigation- Meghavi Kathpalia; Review and editing- Divyam Singh; Supervision and conceptualization- Navkiran Kaur. All authors have read and agreed to the manuscript.
Conflict of interest
Authors involved in the study assert that there are no conflicts of interest.
References
[1] | Wani SH, , Lone SA. Cancer: Diseases. . Educreation Publishing; (2018) . |
[2] | Goodall GJ, , Wickramasinghe VO, RNA in cancer, Nat Rev Cancer, 21: (1): 22–36, (2021) . |
[3] | Bertram JS, The molecular biology of cancer, Mol Aspects Med, 21: (6): 167–223, (2000) . |
[4] | Gonzalez H, , Hagerling C, , Werb Z, Roles of the immune system in cancer: From tumor initiation to metastatic progression, Genes & Development, 32: (19-20): 1267–1284, (2018) . |
[5] | Gajewski T, , Schreiber H, , Fu Y, Innate and adaptive immune cells in the tumor microenvironment, Nat Immun, 14: : 1014–1022, (2013) . doi:10.1038/ni.2703. |
[6] | Feng M, , Jiang W, , Kim B, , Zhang C, , Fu Y, , Weissman I, Phagocytosis checkpoints as new targets for cancer immunotherapy, Nat Rev Cancer, 1–19, (2019) . doi:10.1038/s41568-019-0183-z. |
[7] | Kawasaki B, , Mistree T, , Hurt E, , Kalathur M, , Farrar W, Co-expression of the toleragenic glycoprotein, CD200, with markers for cancer stem cells, Biochem Biophys Res Commun, 364: (4): 778–782, (2007) . doi:10.1016/J.BBRC.2007.10.067. |
[8] | Charette M, , Marabelle A, , Houot R, Turning tumour cells into antigen presenting cells: The next step to improve cancer immunotherapy? Eur J Cancer, 68: : 134–147, (2016) . doi:10.1016/j.ejca.2016.09.010. |
[9] | Xue L, , Qi H, , Zhang H, , Ding L, , Huang Q, , Zhao D , Targeting SREBP-2-regulated mevalonate metabolism for cancer therapy, Front Oncol, 10,: (2020) . doi:10.3389/fonc.2020.01510. |
[10] | Polyak K, Breast cancer: Origins and evolution, J Clin Invest, 117: (11): 3155–3163, (2007) . |
[11] | Geyer FC, , Rodrigues DN, , Weigelt B, , Reis-Filho JS, Molecular classification of estrogen receptor-positive/luminal breast cancers, Adv Anat Pathol, 19: (1): 39–53, (2012) . |
[12] | Prat A, , Perou CM, Deconstructing the molecular portraits of breast cancer, Mol Oncol, 5: (1): 5–23, (2011) . |
[13] | Szymiczek A, , Lone A, , Akbari MR, Molecular intrinsic versus clinical subtyping in breast cancer: A comprehensive review, Clin Genet, 99: (5): 613–637, (2021) . |
[14] | Sareyeldin RM, , Gupta I, , Al-Hashimi I, , Al-Thawadi HA, , AL Farsi HF, , Vranic S , Gene expression and miRNAs profiling: Function and regulation in human epidermal growth factor receptor 2 (HER2-positive breast cancer, Cancers, 11: (5): 646, (2019) . |
[15] | Oh DY, , Bang YJ, HER2-targeted therapies—a role beyond breast cancer, Nat Rev Clin Oncol, 17: (1): 33–48, (2020) . |
[16] | Prat A, , Adamo B, , Cheang MC, , Anders CK, , Carey LA, , Perou CM, Molecular characterization of basal-like and non-basal-like triple-negative breast cancer, Oncologist, 18: (2): 123–133, (2013) . |
[17] | Vaka AR, , Soni B, , Reddy S, Breast cancer detection by leveraging machine learning, ICT Express, 6: (4): 320–324, (2020) . |
[18] | The International Agency for Research on Cancer (IARC). Global cancer observatory [Internet]. 2021 [cited 2023 Oct 26]. Available from: https://gco.iarc.fr/. |
[19] | Sathishkumar K, , Chaturvedi M, , Das P, , Stephen S, , Mathur P, Cancer incidence estimates for 2022 & projection for 2025: Result from National Cancer Registry Programme, India, Ind J Med Res(2023) . |
[20] | Aloor LJ, , Skariyachan S, , Raghavamenon AC, , Kumar KM, , Narayanappa R, , Uttarkar A , BRCA1/TP53 tumor proteins inhibited by novel analogues of curcumin—Insight from computational modelling, dynamic simulation and experimental validation, Int J Biol Macromol, 253: : 126989, (2023) . |
[21] | McPherson K, , Steel C, , Dixon JM, Breast cancer—epidemiology, risk factors, and genetics, BMJ, 321: (7261): 624–628, (2000) . |
[22] | Mobasheri A, , Barrett-Jolley R, Aquaporin water channels in the mammary gland: From physiology to pathophysiology and neoplasia, J Mammary Gland Biol Neoplasia, 19: (1): 91–102, (2014) . |
[23] | Baumgarten SC, , Frasor J, Minireview: inflammation: An instigator of more aggressive estrogen receptor (ER) positive breast cancers, Mol Endocrinol, 26: (3): 360–371, (2012) . |
[24] | Cui X, , Schiff R, , Arpino G, , Osborne CK, , Lee AV, Biology of progesterone receptor loss in breast cancer and its implications for endocrine therapy, J Clin Oncol, 23: (30): 7721–7735, (2005) . |
[25] | Allison KH, , Hammond MEH, , Dowsett M , Estrogen and progesterone receptor testing in breast cancer: ASCO/CAP guideline update, J Clin Oncol, 38: (12): 1346–1366, (2020) . doi:10.1200/JCO.19.02309. |
[26] | Goldberg J, , Pastorello RG, , Vallius T , The immunology of hormone receptor positive breast cancer, Front Immunol, 12: : 674192, (2021) . doi:10.3389/fimmu.2021.674192. |
[27] | Amin MB, , Greene FL, , Edge SB, , Compton CC, , Gershenwald JE, , Brookland RK , The eighth edition AJCC cancer staging manual: Continuing to build a bridge from a population-based to a more “personalized” approach to cancer staging, CA: A Cancer Journal for Clinicians, 67: (2): 93–99, (2017) . |
[28] | Beňačka R, , Szabóová D, , Guľašová Z, , Hertelyová Z, , Radoňák J, Classic and new markers in diagnostics and classification of breast cancer, Cancers, 14: (21): 5444, (2022) . |
[29] | Dank M, , Mühl D, , Pölhös A, , Csanda R, , Herold M, , Kovacs AK , The prediction analysis of microarray 50 (PAM50 gene expression classifier utilized in indeterminate-risk breast cancer patients in hungary: A consecutive 5-year experience, Genes, 14: (9): 1708, (2023) . |
[30] | Szymiczek A, , Lone A, , Akbari MR, Molecular intrinsic versus clinical subtyping in breast cancer: A comprehensive review, Clin Genet, 99: (5): 613–637, (2021) . |
[31] | Henry NL, , Shah PD, , Haider I, , Freer PE, , Jagsi R, , Sabel MS. Cancer of the breast. Abeloff’s Clinical Oncology. 1560–1603. (2020) . |
[32] | Liu N, , Yang Z, , Liu X, , Niu Y, Lymph node status in different molecular subtype of breast cancer: Triple negative tumors are more likely lymph node negative. Oncotarget, 8: (33): 55534, (2017) . |
[33] | Geyer FC, , Rodrigues DN, , Weigelt B, , Reis-Filho JS, Molecular classification of estrogen receptor-positive/luminal breast cancers, Adv Anat Pathol, 19: (1): 39–53, (2012) . |
[34] | Prat A, , Perou CM, Deconstructing the molecular portraits of breast cancer, Mol Oncol, 5: (1): 5–23, (2011) . |
[35] | Sareyeldin RM, , Gupta I, , Al-Hashimi I, , Al-Thawadi HA, , Al Farsi HF, , Vranic S , Gene expression and miRNAs profiling: Function and regulation in human epidermal growth factor receptor 2 (HER2-positive breast cancer, Cancers, 11: (5): 646, (2019) . |
[36] | Oh DY, , Bang YJ, HER2-targeted therapies—a role beyond breast cancer, Nat Rev Clin Oncol, 17: (1): 33–48, (2020) . |
[37] | Prat A, , Adamo B, , Cheang MC, , Anders CK, , Carey LA, , Perou CM, Molecular characterization of basal-like and non-basal-like triple-negative breast cancer, The Oncologist, 18: (2): 123–133, (2013) . |
[38] | Petrakis N, . Genetic cerumen type, breast secretory activity, and breast cancer epidemiology. Genetics of Human Cancer, . Mulvihill JJ, , Miller RW, , Fraumeni JF (eds), (New York, NY), Raven Press; 297–300. (1977) . |
[39] | Carter CL, , Jones DY, , Schatzkin A, , Brinton LA, A prospective study of reproductive, familial and socioeconomic risk factors for breast cancer using NHANES I data, Public Health Rep, 104: : 45–50, (1989) . |
[40] | Anderson DE, Some characteristics of familial breast cancer, Cancer, 28: : 1500–1504, (1979) . |
[41] | Mansfield CM, A review of the etiology of breast cancer, J Natl Med Assoc, 85: (3): 217, (1993) . |
[42] | Kampert JB, , Whittemore AS, , Paffenbarger Jr RS, Combined effect of childbearing, menstrual events, and body size on age-specific breast cancer risk, Am J Epidemiol, 128: (5): 962–979, (1988) . |
[43] | Travis RC, , Key TJ, Oestrogen exposure and breast cancer risk, Br Cancer Res, 5: : 1–9, (2003) . |
[44] | Tabar L, , Fagerberg CJ, , Gad A, Reduction in mortality from breast cancer after mass screening with mammography, Lancet, 1: : 829–832, (1985) . |
[45] | Ronckers CM, , Erdmann CA, , Land CE, Radiation and breast cancer: A review of current evidence, Br Cancer Res, 7: : 1–2, (2004) . |
[46] | Casey PM, , Cerhan JR, , Pruthi S, Oral contraceptive use and the risk of breast cancer, Mayo Clinic Proceedings, 83: (1): 86–91, (2008) . |
[47] | Greenwald P, Strengths and limitations of methodological approaches to the study of diet and cancer, Prev Med, 18: : 163–166, (1989) . |
[48] | Boyar AP, , Rose DP, , Wynder EL, Recommendations for the prevention of chronic disease, Am J Clin Nutr, 48: : 896–900, (1988) . |
[49] | Hiatt RA, , Klatsky A, , Armstrong NA, Alcohol and breast cancer, PrevMed, 17: : 683–685, (1988) . |
[50] | Sharma BS, , Prabhakaran V, , Desai AP, , Bajpai J, , Verma RJ, , Swain PK, Post-translational modifications (PTMs), from a cancer perspective: An overview, Oncogen, 2: : 12, (2019) . |
[51] | Institute of Medicine, Food and Nutrition Board. Dietary reference intakes for energy, carbohydrate, fiber, fat, fatty acids, cholesterol, protein, and amino acids (macronutrients). Washington, DC: National Academy Press, 2005. |
[52] | Boyar AP, , Rose DP, , Wynder EL, Recommendations for the prevention of chronic disease, Am J Clin Nutr, 48: : 896–900, (1988) . |
[53] | Corrie PG, , Pippa G, Cytotoxic chemotherapy: Clinical aspects, Medicine, 36: (1): 24–28. |
[54] | Kathpalia M, , Sharma A, , Kaur N, Sacituzumab govitecan as a second-line treatment in relapsed/refractory metastatic triple-negative breast cancer patients: A systematic review and meta-analysis, Ann Pharmacotherapy, 58: (1): 44–53, (2024) . |
[55] | Léger CS, , Nevill TJ, Hematopoietic stem cell transplantation: A primer for the primary care physician, Can Med Assoc J, 170: (10): 1569–1577, (2004) . |
[56] | Keidan RD, , Fanning J, , Gatenby RA, , Weese JL, Recurrent typhlitis. A disease resulting from aggressive chemotherapy, Dis Colon Rectum, 32: (3): 206–209, (1989) . |
[57] | Rodrigues FG, , Dasilva G, , Wexner SD, Neutropenic enterocolitis, World J Gastroenterol, 23: (1): 42–47, (2017) . |
[58] | Groopman JE, , Itri LM, Chemotherapy-induced anemia in adults: incidence and treatment, J Natl Cancer Inst, 91: (19): 1616–1634, (1999) . |
[59] | Sanger GJ, , Andrews PLR, A history of drug discovery for treatment of nausea and vomiting and the implications for future research, Front Pharmacol, 9: : 913, (2018) . |
[60] | Can G, , Demir M, , Erol O, , Aydiner A, A comparison of men and women’s experiences of chemotherapy-induced alopecia, Euro J Oncol Nurs, 17: (3): 255–260, (2013) . |
[61] | Hijiya N, , Hudson MM, , Lensing S, , Zacher M, , Onciu M, , Behm FG , Cumulative incidence of secondary neoplasms as a first event after childhood acute lymphoblastic leukemia, JAMA, 297: (11): 1207–1215, (2007) . |
[62] | Old LJ, Immunotherapy for cancer, Sci Am, 275: (3): 136–143, (1996) . |
[63] | Liu YH, , Zang XY, , Wang JC, , Huang SS, , Xu J, , Zhang P, Diagnosis and management of immune related adverse events (irAEs) in cancer immunotherapy, Biomed Pharmacotherapy, 120: : 109437, (2019) . |
[64] | Morris EC, , Neelapu SS, , Giavridis T, , Sadelain M, Cytokine release syndrome and associated neurotoxicity in cancer immunotherapy, Nat Rev Immunol, 22: (2): 85–96, (2022) . |
[65] | Tan S, , Li D, , Zhu X, Cancer immunotherapy: Pros, cons and beyond, Biomed Pharmacotherapy, 124: : 109821, (2020) . |
[66] | Sudhakar A, History of cancer, ancient and modern treatment methods, J Cancer Sci Therapy, 1: (2): 1, (2009) . |
[67] | Collins KK, , Liu Y, , Schootman M, , Aft R, , Yan Y, , Dean G , Effects of breast cancer surgery and surgical side effects on body image over time, Br Cancer Res Treat, 126: : 167–176, (2011) . |
[68] | Domen J, , Wagers A, , Weissman IL, Bone marrow (hematopoietic) stem cells, Regenerative Med, 2: : 14–28, (2006) . |
[69] | Mohty B, , Mohty M, Long-term complications and side effects after allogeneic hematopoietic stem cell transplantation: An update, Blood Cancer J, 1: (4): e16, (2011) . |
[70] | Saini A, , Kumar M, , Bhatt S, , Saini V, , Malik A, Cancer causes and treatments, Int J Pharmaceut Sci Res, 11: (7): 3121–3134, (2020) . |
[71] | Zackrisson B, , Mercke C, , Strander H, , Wennerberg J, , Cavallin-Ståhl E, A systematic overview of radiation therapy effects in head and neck cancer, Acta Oncologica, 42: (5-6): 443–461, (2003) . |
[72] | Jordan VC, Furr BJ, (eds) Hormone therapy in breast and prostate cancer. Humana Press; 2009. |
[73] | Hellerstedt BA, , Pienta KJ, The current state of hormonal therapy for prostate cancer, CA: A Cancer Journal for Clinicians, 52: (3): 154–179, (2002) . |
[74] | Hasan T, , Ortel B, , Solban N, , Pogue B, Photodynamic therapy of cancer, Cancer Med, 7: : 537–548, (2003) . |
[75] | Juarranz Á, , Jaén P, , Sanz-Rodríguez F, , Cuevas J, , González S, Photodynamic therapy of cancer. Basic principles and applications, Clin Translational Oncol, 10: : 148–154, (2008) . |
[76] | Suit HD, , Shwayder M, Hyperthermia: Potential as an anti-tumor agent, Cancer, 34: (1): 122–129, (1974) . |
[77] | Kaur P, , Hurwitz MD, , Krishnan S, , Asea A, Combined hyperthermia and radiotherapy for the treatment of cancer, Cancers, 3: (4): 3799–3823, (2011) . |
[78] | Yang L, , Shi P, , Zhao G, , Xu J, , Peng W, , Zhang J , Targeting cancer stem cell pathways for cancer therapy, Signal Transduction Targeted Therapy, 5: (1): 8, (2020) . |
[79] | Kroschinsky F, , Stölzel F, , von Bonin S, , Beutel G, , Kochanek M, , Kiehl M , Intensive Care in Hematological and Oncological Patients (iCHOP) Collaborative Group. New drugs, new toxicities: Severe side effects of modern targeted and immunotherapy of cancer and their management, Critical Care, 21: : 1–1, (2017) . |
[80] | Baust JG, , Bischof JC, , Jiang-Hughes S, , Polascik TJ, , Rukstalis DB, , Gage AA , Re-purposing cryoablation: A combinatorial ‘therapy’ for the destruction of tissue. Prostate Cancer and Prostatic Diseases, 18: (2): 87–95, (2015) . |
[81] | Badalament RA, , Bahn DK, , Kim H, , Kumar A, , Bahn JM, , Lee F, Patient-reported complications after cryoablation therapy for prostate cancer, Urology, 54: (2): 295–300, (1999) . |
[82] | Sharma BS, , Prabhakaran V, , Desai AP, , Bajpai J, , Verma RJ, , Swain PK, Post-translational modifications (PTMs), from a cancer perspective: An overview, Oncogen, 2: : 12, (2019) . |
[83] | Huen M, , Chen J, The DNA damage response pathways: At the crossroad of protein modifications, Cell Res, 18: : 8–16, (2008) . doi:10.1038/cr.2007.109. |
[84] | Nussinov R, , Tsai C, , Xin F, , Radivojac P, Allosteric post-translational modification codes, Trends Biochem Sci, 37: (10): 447–455, (2012) . doi:10.1016/j.tibs.2012.07.001. |
[85] | Bai M, , Ti D, , Mei Q, , Liu J, , Yan X, , Chen D , The role of posttranslational modifications in DNA repair, BioMed Res Int, 2020: : 1–3, (2020) . |
[86] | Hiatt RA, , Klatsky A, , Armstrong NA, Alcohol and breast cancer, PrevMed, 17: : 683–685, (1988) . |
[87] | Chen J, Ubiquitination in breast cancer: A review of the current understanding and future perspectives, J Hematol Oncol, 11: (1): 1–13, (2018) . |
[88] | Liu X, , Zhang Y, , Wang Y, , Yang M, , Hong F, , Yang S, Protein phosphorylation in cancer: Role of nitric oxide signaling pathway, Biomolecules, 11: (7): 1009, (2021) . |
[89] | Arrington JV, , Hsu CC, , Elder SG, , Tao WA, Recent advances in phosphoproteomics and application to neurological diseases, Analyst, 142: (23): 4373–4387, (2017) . |
[90] | Vadlakonda L, , Pasupuleti M, , Reddanna P, Role of PI3K-AKT-mTOR and Wnt signaling pathways in transition of G1-S phase of cell cycle in cancer cells, Front Oncol, 3: : 46481, (2013) . |
[91] | Mishra D, , Patel V, , Banerjee D, Nitric oxide and S-nitrosylation in cancers: emphasis on breast cancer, Breast Cancer: Basic and Clinical Research1178223419882688, (2020) . |
[92] | Ediriweera MK, , Tennekoon KH, , Samarakoon SR, Emerging role of histone deacetylase inhibitors as anti-breast-cancer agents, Drug Discov Today, 24: (3): 685–702, (2019) . |
[93] | Mereiter S, , Balmaña M, , Campos D, , Gomes J, , Reis CA, Glycosylation in the era of cancer-targeted therapy: Where are we heading? Cancer Cell, 36: (1): 6–16, (2019) . |
[94] | Zhou SM, , Cheng L, , Guo SJ, , Wang Y, , Czajkowsky DM, , Gao H , Lectin RCA-I specifically binds to metastasis-associated cell surface glycans in triple-negative breast cancer, Br Cancer Res, 17: (1): 1–4, (2015) . |
[95] | Liu H, , Ma L, , Lin J, , Cao B, , Qu D, , Luo C , Advances in molecular mechanisms of drugs affecting abnormal glycosylation and metastasis of breast cancer, Pharmacol Res, 155: : 104738, (2020) . |
[96] | Dwek RA, Glycobiology: Toward understanding the function of sugars, Chem Rev, 96: (2): 683–720, (1996) . |
[97] | Scott DA, , Drake RR, Glycosylation and its implications in breast cancer, Expert Rev Proteomics, 16: (8): 665–680, (2019) . |
[98] | Munkley J, , Elliott DJ, Hallmarks of glycosylation in cancer, Oncotarget, 7: (23): 35478, (2016) . |
[99] | Shibuya N, , Goldstein IJ, , Broekaert WF, , Nsimba-Lubaki M, , Peeters B, , Peumans WJ, The elderberry (Sambucus nigra L.) bark lectin recognizes the Neu5Ac (alpha 2-6 Gal/GalNAc sequence, J Biol Chem, 262: (4): 1596–1601, (1987) . |
[100] | Wang WC, , Cummings RD, The immobilized leukoagglutinin from the seeds of Maackia amurensis binds with high affinity to complex-type Asn-linked oligosaccharides containing terminal sialic acid-linked alpha-2, 3 to penultimate galactose residues, J Biol Chem, 263: (10): 4576–4585, (1988) . |
[101] | Büll C, , Stoel MA, , den Brok MH, , Adema GJ, Sialic acids sweeten a tumor’s life, Cancer Res, 74: (12): 3199–3204, (2014) . |
[102] | Navkiran K, , Parul J, Abstract A27: Proteomic study of the of posttranslational modifications (phosphorylation and glycosylation) and its significance in human breast cancer, Mol Cancer Ther12, (2013) . |
[103] | Rodrigues JG, , Balmaña M, , Macedo JA, , Poças J, , Fernandes Â, , de-Freitas-Junior JC , Glycosylation in cancer: Selected roles in tumor progression, immune modulation and metastasis, Cellular Immunol, 333: : 46–57, (2018) . |
[104] | Stowell SR, , Ju T, , Cummings RD, Protein glycosylation in cancer, Ann Rev of Pathol: Mechanisms Dis, 10: : 473–510, (2015) . |
[105] | Pinho SS, , Reis CA, Glycosylation in cancer: Mechanisms and clinical implications, Nat Rev Cancer, 15: (9): 540–555, (2015) . |
[106] | Kirwan A, , Utratna M, , O’Dwyer ME, , Joshi L, , Kilcoyne M, Glycosylation-based serum biomarkers for cancer diagnostics and prognostics, BioMed Res Int2015, (2015) . |
[107] | Hanahan D, , Weinberg RA, The hallmarks of cancer, Cell, 100: (1): 57–70, (2000) . |
[108] | Boscher C, , Dennis JW, , Nabi IR, Glycosylation, galectins and cellular signaling, Curr Opin Cell Biol, 23: (4): 383–392, (2011) . |
[109] | Kim SH, , Turnbull J, , Guimond S, Extracellular matrix and cell signalling: The dynamic cooperation of integrin, proteoglycan and growth factor receptor, J Endocrinol, 209: (2): 139–151, (2011) . |
[110] | English NM, , Lesley JF, , Hyman R, Site-specific de-N-glycosylation of CD44 can activate hyaluronan binding, and CD44 activation states show distinct threshold densities for hyaluronan binding, Cancer Res, 58: (16): 3736–3742, (1998) . |
[111] | Paszek MJ, , Dufort CC, , Rossier O, , Bainer R, , Mouw JK, , Godula K , The cancer glycocalyx mechanically primes integrin-mediated growth and survival, Nature, 511: (7509): 319–325, (2014) . |
[112] | Janus F, , Albrechtsen N, , Dornreiter I, , Wiesmüller L, , Grosse F, , Deppert W, The dual role model for p53 in maintaining genomic integrity, Cell Mol Life Sci CMLS, 55: : 12–27, (1999) . |
[113] | Meek DW, The p53 response to DNA damage, DNA Repair, 3: (8-9): 1049–1056, (2004) . |
[114] | Pellegata NS, , Antoniono RJ, , Redpath JL, , Stanbridge EJ, DNA damage and p53-mediated cell cycle arrest: A reevaluation, Proc e Natl Acad Sci, 93: (26): 15209–15214, (1996) . |
[115] | Liu YY, Resuscitating wild-type p53 expression by disrupting ceramide glycosylation: A novel approach to target mutant p53 tumors, Cancer Res, 71: (20): 6295–6299, (2011) . |
[116] | Giacinti C, , Giordano A, RB and cell cycle progression, Oncogene, 25: (38): 5220–5227, (2006) . |
[117] | Dick FA, , Rubin SM, Molecular mechanisms underlying RB protein function, Nat Rev Mol Cell Biol, 14: (5): 297–306, (2013) . |
[118] | Knudsen ES, , Pruitt SC, , Hershberger PA, , Witkiewicz AK, , Goodrich DW, Cell cycle and beyond: Exploiting new RB1 controlled mechanisms for cancer therapy, Trends Cancer, 5: (5): 308–324, (2019) . |
[119] | Warburg O, On the origin of cancer cells, Science, 123: (3191): 309–314, (1956) . |
[120] | Slawson C, , Hart GW, O-GlcNAc signalling: Implications for cancer cell biology, Nat Rev Cancer, 11: (9): 678–684, (2011) . |
[121] | Wells L, , Vosseller K, , Hart GW, Glycosylation of nucleocytoplasmic proteins: Signal transduction and O-GlcNAc, Science, 291: (5512): 2376–2378, (2001) . |
[122] | Wang H, , Vant J, , Wu Y, , Sanchez R, , Micou ML, , Zhang A , Glycolytic metabolon assembly on mitochondria via hexokinase O-GlcNAcylation promotes metabolic efficiency, bioRxiv, 2023: : 2023–2008. |
[123] | O’Brien CM, , Mulukutla BC, , Mashek DG, , Hu WS, Regulation of metabolic homeostasis in cell culture bioprocesses. Trends Biotechnol, 38: (10): 1113–1127, (2020) . |
[124] | Pang Y, , Xu X, , Xiang X, , Li Y, , Zhao Z, , Li J , High fat activates O-GlcNAcylation and affects AMPK/ACC pathway to regulate lipid metabolism, Nutrients, 13: (6): 1740, (2021) . |
[125] | Makwana V, , Ryan P, , Patel B, , Dukie SA, , Rudrawar S, Essential role of O-GlcNAcylation in stabilization of oncogenic factors, Biochimica et Biophysica Acta (BBA)-General Subjects, 1863: (8): 1302–1317, (2019) . |
[126] | Robles-Flores M, , Moreno-Londoño AP, , Castañeda-Patlán MC, Signaling pathways involved in nutrient sensing control in cancer stem cells: An overview, Front Endocrinol, 12: : 627745, (2021) . |
[127] | Hale AJ, , Smith CA, , Sutherland LC, , Stoneman VE, , Longthorne VL, , Culhane AC , Apoptosis: Molecular regulation of cell death, Eur J Biochem, 236: (1): 1–26, (1996) . |
[128] | Sharma K, , Wang RX, , Zhang LY, , Yin DL, , Luo XY, , Solomon JC , Death the fas way: Regulation and pathophysiology of CD95 and its ligand, Pharmacol Therapeutics, 88: (3): 333–347, (2000) . |
[129] | Shatnyeva OM, , Kubarenko AV, , Weber CE, , Pappa A, , Schwartz-Albiez R, , Weber AN , Modulation of the CD95-induced apoptosis: The role of CD95 N-glycosylation, PloS One, 6: (5): e19927, (2011) . |
[130] | Wagner KW, , Punnoose EA, , Januario T, , Lawrence DA, , Pitti RM, , Lancaster K , Death-receptor O-glycosylation controls tumor-cell sensitivity to the proapoptotic ligand Apo2L/TRAIL, Nat Med, 13: (9): 1070–1077, (2007) . |
[131] | Liu FT, , Yang RY, , Hsu DK, Galectins in acute and chronic inflammation, Ann New York Acad Sci, 1253: (1): 80–91, (2012) . |
[132] | Cohen GM, Caspases: The executioners of apoptosis, Biochem J, 326: (1): 1–6, (1997) . |
[133] | Wynford-Thomas D, Cellular senescence and cancer, J Pathol, 187: (1): 100–111, (1999) . |
[134] | Greenberg RA, , O’Hagan RC, , Deng H, , Xiao Q, , Hann SR, , Adams RR , Telomerase reverse transcriptase gene is a direct target of c-Myc but is not functionally equivalent in cellular transformation, Oncogene, 18: (5): 1219–1226, (1999) . |
[135] | Chou TY, , Hart GW, , Dang CV, c-Myc is glycosylated at threonine 58, a known phosphorylation site and a mutational hot spot in lymphomas, J Biol Chem, 270: (32): 18961–18965, (1995) . |
[136] | Mishra A, , Behura A, , Mawatwal S, , Kumar A, , Naik L, , Mohanty SS , Structure-function and application of plant lectins in disease biology and immunity, Food Chem Toxicol, 134: : 110827, (2019) . |
[137] | Kyriakopoulou OG, , Arens P, , Pelgrom KT, , Karapanos I, , Bebeli P, , Passam HC, Genetic and morphological diversity of okra (Abelmoschus esculentus [L.] Moench.) genotypes and their possible relationships, with particular reference to Greek landraces, Sci Horticulturae, 171: : 58–70, (2014) . |
[138] | Monte LG, , Santi-Gadelha T, , Reis LB, , Braganhol E, , Prietsch RF, , Dellagostin OA , Lectin of Abelmoschus esculentus (okra) promotes selective antitumor effects in human breast cancer cells, Biotechnol Lett, 36: : 461–469, (2014) . |
[139] | Carneiro RF, , de Melo AA, , de Almeida AS, , da Mata Moura R, , Chaves RP, , de Sousa BL , H-3, a new lectin from the marine sponge Haliclona caerulea: Purification and mass spectrometric characterization, Int J Biochem Cell Biol, 45: (12): 2864–2873, (2013) . |
[140] | do Nascimento-Neto LG, , Cabral MG, , Carneiro RF, , Silva Z, , Arruda FV, , Nagano CS , Halilectin-3, a lectin from the marine sponge Haliclona caerulea, induces apoptosis and autophagy in human breast cancer MCF7 cells through caspase-9 pathway and LC3-II protein expression, Anti-Cancer Agents Med Chem (Formerly Current Medicinal Chemistry-Anti-Cancer Agents), 18: (4): 521–528, (2018) . |
[141] | Wu AM, , Wu JH, , Tsai MS, , Hegde GV, , Inamdar SR, , Swamy BM , Carbohydrate specificity of a lectin isolated from the fungus Sclerotium rolfsii, Life Sci, 69: (17): 2039–2050, (2001) . |
[142] | Hegde P, , Narasimhappagari J, , Swamy BM, , Inamdar SR, Efficacy studies of Sclerotium rolfsii lectin on breast cancer using NOD SCID mouse model, Chem Biol Drug Des, 92: (2): 1488–1496, (2018) . |
[143] | Savanur MA, , Eligar SM, , Pujari R, , Chen C, , Mahajan P, , Borges A , Sclerotium rolfsii lectin induces stronger inhibition of proliferation in human breast cancer cells than normal human mammary epithelial cells by induction of cell apoptosis, PLoS One, 9: (11): 110107, (2014) . e . |
[144] | Vanti GL, , Vishwanathreddy H, , Venkat H, , Bhat GG, , Padmanabhan V, , Jayaprakash NS , Sclerotium rolfsii lectin expressed in tobacco confers protection against Spodoptera litura and Myzus persicae, J Pest Sci, 89: : 591–602, (2016) . |
[145] | Rambaruth ND, , Greenwell P, , Dwek MV, The lectin Helix pomatia agglutinin recognizes O-GlcNAc containing glycoproteins in human breast cancer, Glycobiology, 22: (6): 839–848, (2012) . |
[146] | Thomas M, , Noguchi M, , Fonseca L, , Kitagawa H, , Kinoshita K, , Miyazaki I, Prognostic significance of Helix pomatia lectin and c-erbB-2 oncoprotein in human breast cancer, British Journal of Cancer, 68: (3): 621–626, (1993) . |
[147] | Hellmig TJ, , Brace EJ, , Greenspan A, , Laugier C, , Aradhya A, , Basu S Amur maackia (Maackia amurensis) seed lectin history and potential effect on cancer progression, inflammation and viral infection. Ancient and Traditional Foods, Plants, Herbs and Spices used in Cancer. . CRC Press; 89–102. (2023) . |
[148] | Büll C, , Stoel MA, , den Brok MH, , Adema GJ, Sialic acids sweeten a tumor’s life, Cancer Res, 74: (12): 3199–3204, (2014) . |
[149] | Zhang Z, , Wuhrer M, , Holst S, Serum sialylation changes in cancer, Glycoconjugate J, 35: : 139–160, (2018) . |
[150] | Heusser PE, , Kienle GS. Anthroposophic Medicine, Integrative Oncology, and Mistletoe Therapy of Cancer. . (New York, NY, USA), Oxford University Press; (2009) . |
[151] | Dhanens C, NARRATIVE REVIEW ON THE USE OF MISTLETOE AS A TREATMENT FOR CANCER (Doctoral dissertation, Ghent University). |
[152] | Saha C, Unravelling the therapeutic intervention of inflammation and cancer by Viscum album: understanding its anti-inflammatory and immunostimulatory properties (Doctoral dissertation, Université de Technologie de Compiègne). |
[153] | Hoessli DC, , Ahmad I, Mistletoe lectins: Carbohydrate-specific apoptosis inducers and immunomodulators, Curr Org Chem, 12: (11): 918–925, (2008) . |
[154] | Majeed M, , Hakeem KR, , Rehman RU, Mistletoe lectins: From interconnecting proteins to potential tumor inhibiting agents, Phytomedicine Plus, 1: (3): 100039, (2021) . |
[155] | Thies A, , Nugel D, , Pfüller U, , Moll I, , Schumacher U, Influence of mistletoe lectins and cytokines induced by them on cell proliferation of human melanoma cells in vitro, Toxicology, 207: (1): 105–116, (2005) . |
[156] | Bah CS, , Fang EF, , Ng TB, Medicinal applications of plant lectins. Antitumor potential and other emerging medicinal properties of natural compounds, 2013: : 55–74. |
[157] | Park WB, , Lyu SY, , Kim JH, , Choi SH, , Chung HK, , Ahn SH , Inhibition of tumor growth and metastasis by Korean mistletoe lectin is associated with apoptosis and antiangiogenesis, Cancer Biotherapy and Radiopharmaceuticals, 16: (5): 439–447, (2001) . |
[158] | Lyu SY, , Choi SH, , Park WB, Korean mistletoe lectin-induced apoptosis in hepatocarcinoma cells is associated with inhibition of telomerase via mitochondrial controlled pathway independent of p53, Arch Pharmacal Res, 25: : 93–101, (2002) . |
[159] | Sarray S, , Berthet V, , Calvete JJ, , Secchi J, , Marvaldi J, , Ayeb ME , Lebectin, a novel C-type lectin from Macrovipera lebetina venom, inhibits integrin-mediated adhesion, migration and invasion of human tumor cells, Lab Invest, 84: (5): 573–581, (2004) . |
[160] | Jebali J, , Fakhfekh E, , Morgen M, , Srairi-Abid N, , Majdoub H, , Gargouri A , Lebecin, a new C-type lectin like protein from Macrovipera lebetina venom with anti-tumor activity against the breast cancer cell line MDA-MB231, Toxicon, 86: : 16–27, (2014) . |
[161] | Akef HM, Snake venom: Kill and cure, Toxin Rev, 38: (1): 21–40, (2019) . |
[162] | Jebali J, , Fakhfekh E, , Morgen M, , Srairi-Abid N, , Majdoub H, , Gargouri A , Lebecin, a new C-type lectin like protein from Macrovipera lebetina venom with anti-tumor activity against the breast cancer cell line MDA-MB231, Toxicon, 86: : 16–27, (2014) . |
[163] | Knudsen BF, , Kaack KV. A review of human health and disease claims for elderberry (Sambucus nigra) fruit. International Symposium on Elderberry 1061. 121–131. (2013) . |
[164] | Lastovickova M, , Strouhalova D, , Bobalova J, Use of lectin-based affinity techniques in breast cancer glycoproteomics: A review, J Proteome Res, 19: (5): 1885–1899, (2020) . |
[165] | Lomax-Browne HJ, , Robertson C, , Antonopoulos A, , Leathem AJ, , Haslam SM, , Dell A , Serum IgA1 shows increased levels of α 2, 6-linked sialic acid in breast cancer, J Roy Soc Interface Focus, 9: (2): 20180079, (2019) . |
[166] | Scott DA, , Drake RR, Glycosylation and its implications in breast cancer, Exp Rev Proteomics, 16: (8): 665–680, (2019) . |
[167] | Walker C, , Mojares E, , del Río Hernández A, Role of extracellular matrix in development and cancer progression, Int J Mol Sci, 19: (10): 3028, (2018) . |
[168] | Chaudhary PK, , Kim S, An insight into GPCR and G-proteins as cancer drivers, Cells, 10: (12): 3288, (2021) . |
[169] | Zhou X, , Yang G, , Guan F, Biological functions and analytical strategies of sialic acids in tumor, Cells, 9: (2): 273, (2020) . |
[170] | Josic D, , Martinovic T, , Pavelic K, Glycosylation and metastases, Electrophoresis, 40: (1): 140–150, (2019) . |
[171] | Dan X, , Liu W, , Ng TB, Development and applications of lectins as biological tools in biomedical research, Med Res Rev, 36: (2): 221–247, (2016) . |
[172] | Dobie C, , Skropeta D, Insights into the role of sialylation in cancer progression and metastasis, Br J Cancer, 124: (1): 76–90, (2021) . |
[173] | Van Damme EJ, , Peumans WJ, , Pusztai A, , Bardocz S. Handbook of Plant Lectins: Properties and Biomedical Applications. . John Wiley & Sons; (1998) . |
[174] | Sauter E, Cancer prevention and treatment using combination therapy with natural compounds, Exp Rev Clin Pharmacol, 13: : 265–285, (2020) . |
[175] | Demain A, , Vaishnav P, Natural products for cancer chemotherapy, Microbial Biotechnol, 4: : 687–699, (2011) . |
[176] | Mann J, Natural products in cancer chemotherapy: Past, present and future, Nat Rev Cancer, 2: : 143–148, (2002) . |