Cancer associated fibroblasts modulate the cytotoxicity of anti-cancer drugs in breast cancer: An in vitro study
Abstract
BACKGROUND:
Tumour microenvironment (TME) contributes to resistance to anti-cancer drugs through multiple mechanisms including secretion of pro-survival factors by cancer associated fibroblasts (CAFs). In this study, we determined the chemotherapy resistance producing potential of CAFs in molecular subtypes of breast cancer.
METHODS:
The CAFs were isolated from fresh lumpectomy/mastectomy specimens of different molecular subtypes of breast cancer. The CAFs were cultured and secretome was collected from each breast cancer subtype. Breast cancer cell lines MCF-7, SK-BR3, MDA-MB-231, and MDA-MB-468 were treated with different doses of tamoxifen, trastuzumab, cisplatin, and doxorubicin alone respectively and in combination with secretome of CAFs from respective subtypes. MTT assay was done to check cell death after drug treatment. Liquid chromatography–mass spectrometry (LCMS) analysis of CAF secretome was also done.
RESULTS:
MTT assay showed that anti-cancer drugs alone had growth inhibitory effect on the cancer cells however, presence of CAF secretome reduced the anti-cancer effect of the drugs. Resistant to drugs in the presence of secretome, was determined by increased cell viability i.e., MCF-7, 51.02% to 63.02%; SK-BR-3, 34.22% to 44.88%; MDA-MB-231, 52.59% to 78.63%; and MDA-MB-468, 48.92% to 55.08%. LCMS analysis of the secretome showed the differential abundance of CAFs secreted proteins across breast cancer subtypes.
CONCLUSIONS:
The treatment of breast cancer cell lines with anti-cancer drugs in combination with secretome isolated from molecular subtype specific CAFs, reduced the cytotoxic effect of the drugs. In addition, LCMS data also highlighted different composition of secreted proteins from different breast cancer associated fibroblasts. Thus, TME has heterogenous population of CAFs across the breast cancer subtypes and in vitro experiments highlight their contribution to chemotherapy resistance which needs further validation.
1.Introduction
Breast cancer displays cellular and molecular heterogeneity and is classified based on gene expression signatures into: Luminal A, Luminal B, Her-2 enriched, and TNBC subtypes [1–4]. Due to this cellular and molecular heterogeneity, despite the availability of chemotherapy and targeted therapy, increased mortality has been witnessed in breast cancer due to therapy resistance. To uncover resistant mechanisms, research is being done on tumour component, however, the role of tumour microenvironment (TME) in therapy resistance remains unexplored. The TME includes: cellular components, like fibroblasts, vascular endothelial cells, tumor- associated immune cells, and a non-cellular component in the form of network of various matrix proteins such as glycosaminoglycans, proteoglycans, and glycoproteins [5]. In TME, the most dominant are fibroblasts, also called cancer-associated fibroblasts (CAFs) [6,7]. The proposed resistance contributing mechanisms of TME to various drugs includes: presence of dense ECM (extracellular matrix), limited blood supply, reduced drug availability to tumor cells, and interactions of tumor cells with ECM that may activate survival pathways or secretion of pro-survival factors by stromal cells [8]. A few studies have shown that TME secreted factors protect breast cancer cells against platinum-based chemotherapeutics. The CAF in TME induces epithelial–mesenchymal transition (EMT) which causes resistance to tamoxifen drug via the production of hyaluronan [9,10]. It has also been found that patients with more amount of tumor stroma have worst prognosis [11].
In this study, we isolated primary CAFs from different molecular subtypes of breast cancers and evaluated their role in modulating the sensitivity or resistance to chemotherapy in in-vitro experiments on different breast cancer cell lines.
2.Material and methods
The present study was approved by Institute Ethics Committee (IEC) wide No. NK/4116/PhD/97. Fresh breast cancer tissues were obtained from the lumpectomy/mastectomy specimens of female patients of breast carcinoma who had not undergone prior neoadjuvant chemotherapy (NACT). All cases were also processed for formalin fixed paraffin embedded sections for pathological diagnosis and molecular subtyping of breast cancer using ER, PR, Her-2/neu, and Ki-67 antibodies. Written informed consent was taken from each patient included in the study.
2.1.Isolation of breast cancer associated fibroblasts
Fibroblasts were isolated from fresh breast cancer tissue of each molecular subtypes as well as from normal breast tissue. The normal breast tissues were taken 5 cm away from cancer tissue in mastectomy/lumpectomy specimens. Collected fresh breast cancer tissue was processed for CAFs isolation through enzymatic method [12]. Briefly, 100 mg of tissue was chopped in enzyme mix solution containing 200 unit/mL of Collagenase Type I (17018029, Gibco®, Thermo Fisher Scientific) and 500 unit/mL of Hyaluronidase (Cat No. H3506, Merk, Sigma Aldrich, Missouri, United States) mixed in growth media containing Dulbecco’s modified eagle medium (DMEM)/F-12 (11320033 Gibco®, Thermo Fisher Scientific) supplemented with 10% foetus bovine serum (16000044, Gibco®, Thermo Fisher Scientific), 1% antibiotic/anti-mycotic solution (A002 Hi-Media), and incubated at 37 °C for 1.5 hour. After incubation, tissue lysate was filtered through 40 μm pore size cell strainer and collected in 15 mL sterile flask. Flask with filtrate was centrifuged at 400 × g for 5 minutes. Obtained CAFs pellet was washed with PBS and resuspended in growth media as mentioned above, in addition to supplemented with basic fibroblast growth factor (10 ng/mL) (13256-029, GibcoTM, Life Technologies). The CAFs were seeded in 6-well plate and incubated at 37 °C with 5% CO2 constant supply. After 1.5 hour, the attached cells were washed gently with PBS and nourished with fresh growth media. During cell growth, the media was changed on each alternative day and trypsinized at >90% confluency. All primary CAFs cultures were maintained up to 5 passages.
2.1.1.Collection of conditioned media from primary CAFs
Conditioned media (CM) or secretome was collected from CAF cultures of all breast cancer subtypes. For that, 1 × 106 fibroblasts were seeded (75 cm2 plate) in serum free media and secretome was collected after 24 hours and 48 hours. Collected CM was centrifuged at 500 × g twice and stored at −20 °C.
2.1.2.Immunohistochemistry/Immunofluorescence
Isolated primary CAFs were characterized by immunohistochemistry (IHC) and immunofluorescence (IF). Antibodies (a) α-SMA and vimentin (fibroblast cell markers) were used for positive selection, (b) cytokeratin (epithelial cell marker) and CD31 (endothelial cell) were used for negative selection of primary cultured fibroblasts. Fibroblasts (2 × 103) were seeded on sterile 10 mm glass coverslip placed at the bottom of 24 well plate for IHC and IF. For IHC, colour reaction was developed by 3,3′-Diaminobenzidine (DAB) with substrate and counter stained with haematoxylin. For IF, secondary antibody was labelled with FITC and DAPI (MB097, Hi-Media) was used to stain cell nucleus. The IHC and IF staining was analysed using light microscope (Olympus) and fluorescent microscope (EVOS® cell imaging systems) respectively.
2.1.3.Breast cancer cell lines
Breast cancer cell lines MCF-7 (estrogen positive), SK-BR3 (Her-2 positive), MDA-MB-231, and MDA-MB-468 (estrogen and Her-2 negative) were purchased from NCCS, Pune, Maharashtra, India and grown under hygienic conditions with RPMI-1640 supplemented with 10% FBS and 1% anti-biotic/anti-mycotic and incubated at 37 °C with 5% CO2 constant supply. During cell growth, media was changed every alternative day and cells were trypsinized at >90% confluency. Each cell line was grown for 10 successive passages and accordingly stored in vapour phase of liquid nitrogen in cell freezing solution.
2.2.Treatment with drugs and CAF conditioned media
Cytotoxicity of isolated CM was checked by treatment on MCF-7 and MDA-MB-468 cell lines. The CM was diluted in RPMI media at 1:1 ratio before treatment. Breast cancer cell lines were treated with different doses of anti-cancer drugs which are being used in the clinical setting i.e., MCF-7 (ER positive cell line) with tamoxifen, SK-BR3 (Her2 positive cell line) with trastuzumab, and MDA-MB-231 or MDA-MB-468 (Triple negative cell line) with cisplatin and doxorubicin. To see the subtype specific effect, respective cell lines were treated with CM isolated from the corresponding molecular subtypes (ER+, Her2+ and Triple negative) of breast cancers. Cytotoxicity/cell viability was checked with MTT assay as per the manufacturer’s instructions (M2128, Sigma Aldrich). Briefly, 5 × 103 cells were seeded in 96-well plate and allowed to grow for 24 hours in CO2 incubator at 37 °C. Next day, the cells were washed with PBS and treated with different doses of drug in 100 μL volume. IC50 value for each drug was calculated. Treatment of drug alone and drug: CM (1:1) was performed for every cell line in respective combinations. Two different time intervals i.e., 24 hours and 48 hours were selected for every drug and drug: CM treatment. Post treatment, 100 μL of 0.5× MTT was added in each well and plate was incubated in CO2 incubator at 37 °C for 3.5 hours. After incubation, MTT was discarded and formazan crystal were dissolved in 100 μL DMSO. Within next 5 minutes, amount of formazan produced was measured at 570 nm on Infinite® 200 PRO plate reader (Tecan Group Ltd.). All dugs/CM treatment experiments were done in triplicates and repeated twice to confirm the reproducibility of results and finally represented as in mean.
2.3.Liquid chromatography–mass spectrometry (LCMS)
Liquid chromatography–mass spectrometry of conditioned media of CAFs of different subtypes of breast cancers was performed on Orbitrap FusionTM TribridTM Mass Spectrometer (Thermo Fisher Scientific). Briefly, collected supernatant was concentrated on vacuum concentrator by spin at 15000 × g for 20 minutes at room temperature. Secretome (20 μl) was mixed with 10 mM dithiothreitol (DTT) and incubated for 45 minutes in dark followed by incubation with indole-3-acetic Acid (IAA) 50 mM for 45 minutes in dark. Secretome was then diluted 10 times and incubated for digestion with 1 μg of trypsin enzyme at 37 °C for 16 hours. Sample was mixed with 10% trifluoro acetic Acid and analyzed on LCMS. Comparative analysis for proteins abundance across molecular subtypes of breast cancer was done with using normal control.
3.Results
3.1.Morphology and phenotypic characterization of fibroblasts
Under the light microscope, primary cultured fibroblasts showed spindled to oval shape morphology. The fibroblasts isolated from breast cancer tissue were larger with more cytoplasm compared to the fibroblasts isolated from normal breast tissue (Fig. 1). No epithelial or endothelial were observed in the culture plates and showed the pure fibroblasts only. The characterization of primary fibroblasts was done with IHC and IF. Fibroblasts showed positive staining for vimentin and α-SMA antibodies and negative staining for cytokeratin, E-Cadherin and CD31 antibodies. Staining for vimentin and α-SMA was stronger on cancer associated fibroblasts compared to fibroblasts isolated from normal breast tissue, due to the activated nature of cancer associated fibroblasts (Fig. 1). Similarly on IF, CAFs had showed positive staining for vimentin and were negative for E-cadherin and cytokeratin (Supplementary Fig. S1). Both these immunophenotyping techniques confirmed that isolated cells were pure fibroblasts and not contaminated by epithelial and endothelial cells.
Fig. 1.
Immunohistochemistry images showing characterization of primary of fibroblasts. α-SMA and vimentin antibodies showed positive staining and cytokeratin and CD31 antibodies showed negative staining on fibroblasts isolated from breast cancer tissue and normal breast tissue. Moreover, α-SMA and vimentin antibodies had strong positive staining on fibroblasts isolated from breast cancer tissue and weaker staining on fibroblasts isolated from normal breast tissue.
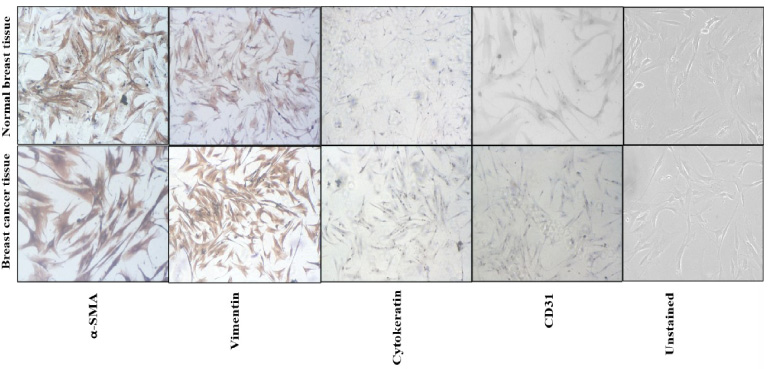
3.2.Treatment of MCF-7 cells with tamoxifen and conditioned media from CAFs of ER+ breast cancer
The percentage cell viability of the breast cancer cell lines after treatment with secretome was similar to that of control (treated with RPMI media only) thus suggesting that CM had no cytotoxic effect on the breast cancer cell lines. The MCF-7 breast cancer cell line was treated with tamoxifen alone and tamoxifen in combination with CM (collected from CAFs isolated from ER+/PR+ breast cancer tissue). MCF-7 cells were treated with 5 μg, 10 μg, 20 μg, 40 μg, and 60 μg doses of tamoxifen alone and same drug doses in combination with CM. Tamoxifen treatment reduced the MCF-7 cells viability. MCF-7 cell death after 48 hours tamoxifen treatment was slightly more (51.02%) compared to treatment for 24 hours (48.22%). The IC50 value of tamoxifen was 21.08 μg and 20 μg at 24 hours and 48 hours respectively (Fig. 2A and B). The IC50 value of tamoxifen in combination with CM was becomes 38.06 μg. Moreover, the presence of CM reduced the cytotoxic effect of tamoxifen drug and resulted in increased cell viability from 51.02% to 63.02% (24 hours treatment) and 48.22% to 59.22% (48 hours treatment) at same IC50 values (Fig. 2A & B).
Fig. 2.
(A&B) MCF-7 breast cancer cells treatment with tamoxifen and tamoxifen plus CM for 24 hours (A) and 48 hours (B). Tamoxifen dose of 20 μg reduced the cell viability of MCF-7 cells by 50% at both time interval. The tamoxifen treatment with CM reduced the cytotoxic effect of tamoxifen and increased the MCF-7 cell viability. The calculated IC50 value of tamoxifen was 21.08 μg and 19.82 μg at 24 hours and 48 hours respectively.
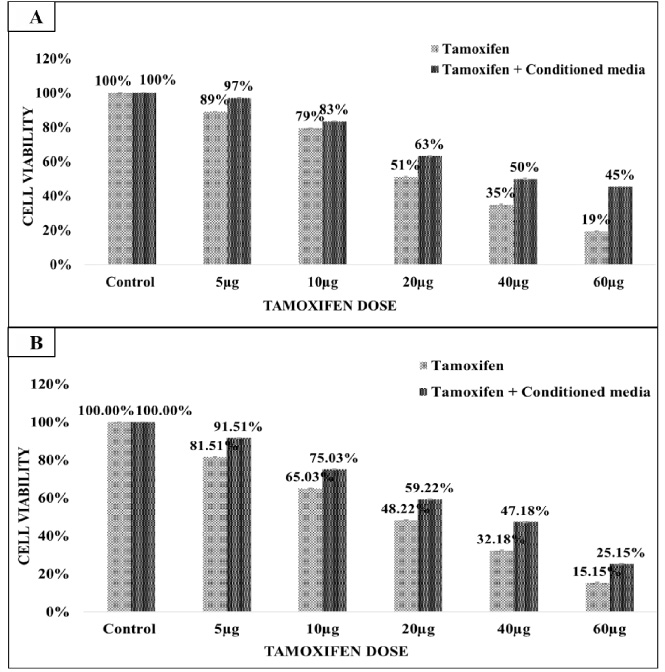
3.3.Treatment of SK-BR3 cells with trastuzumab and conditioned media from CAFs of Her-2 positive breast cancer
The SK-BR3 breast cancer cell were treated with trastuzumab alone and trastuzumab in combination with CM (collected from CAFs isolated from Her-2 + breast cancer tissue). SK-BR3 cells were treated with 10 μg, 20 μg, 30 μg, 40 μg, 50 μg, 60 μg, 70 μg, 80 μg, and 90 μg doses of trastuzumab alone and in combination with CM for 24 hours (Fig. 3). Trastuzumab treatment reduced the SK-BR3 cells viability significantly. The calculated IC50 value of trastuzumab drug was 40 μg. The presence of CM along with trastuzumab reduced the cytotoxic effect of trastuzumab drug on SK-BR3 cells. The viability of SK-BR3 cells increased from 45% (trastuzumab alone) to 48% (trastuzumab and CM) at 40 μg trastuzumab dose and 38% (trastuzumab alone) to 44% (trastuzumab and CM) at 50 μg trastuzumab dose. At 60 μg trastuzumab dose treatment, SK-BR3 cells viability further increased from 34.22% (trastuzumab alone) to 44.88% (trastuzumab and CM). The calculated IC50 values for trastuzumab was 40 μg and for trastuzumab plus CM combination was 44.7 μg (Table 1). However, difference in IC50 values under trastuzumab alone and trastuzumab and CM conditions is not significant.
Fig. 3.
SK-BR3 cells treatment with trastuzumab alone and trastuzumab plus CM for 24 hours. A trastuzumab dose of 40 μg reduced the cell viability by 50%. The IC50 value of trastuzumab changed from 40 μg to 44.7 μg in the presence of CM along with trastuzumab.
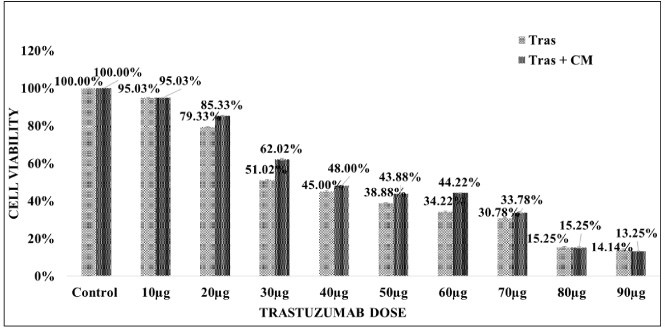
Table 1
The IC50 values of all used drugs alone and drugs in combination with CM at different time points
MCF-7 | MDA-MB-468 | MDA-MB-231 | SK-BR-3 | |||||||||||
Name of drug | Tamoxifen | Doxorubicin | Cisplatin | Herceptin | ||||||||||
24 hrs | 48 hrs | 24 hrs | 48 hrs | 24 hrs | 48 hrs | 24 hrs | ||||||||
IC50 drug only | 21.08 μg | P < 0.00 | 19.82 μg | P < 0.00 | 75 nM | P < 0.00 | 75 nM | P < 0.01 | 11.08 μg | P < 0.02 | 8.12 μg | P < 0.00 | 40 μg | P = 0.10 |
IC50 drug + CM | 38.6 μg | 38 μg | 128 nM | 100 nM | 18.75 μg | 18.75 μg | 44.7 μg |
3.4.Treatment of MDA-MB-231 cells with cisplatin and conditioned media from CAFs of triple negative breast cancer
MDA-MB-231 cells were treated with cisplatin anti-cancer drug alone and in combination with CM (collected from CAFs isolated from triple negative breast cancer tissue). MDA-MB-231 cells were treated with 5 μg, 10 μg, 20 μg, 40 μg, and 60 μg doses of cisplatin for 24 hours and 48 hours (Fig. 4A & B). Cisplatin treatment for 24 hours and 48 hours reduced the viability of MDA-MB-231 cells significantly. MDA-MB-231 cell death after 48 hours cisplatin treatment was slightly more (52.59%) compared to 24 hours cisplatin treatment (48.59%). Calculated IC50 value of cisplatin was 11.08 μg and 8.12 μg at 24 hours and 48 hours treatment respectively. Further, the presence of CM reduced the cytotoxic effect of cisplatin on MDA-MB-231 cells. At the same IC50 (11.08 μg and 8.12 μg) values the cell viability of MDA-MB-231 cells improved from 48.59% to 78.63% and 52.59% to 78.63% after 24 hours and 48 hours treatment respectively. The calculated IC50 of cisplatin in combination with CM was 18.75 μg at both time points (Table 1).
Fig. 4.
(A&B) MDA-MB-231 cells treatment with cisplatin for 24 hours and 48 hours. A cisplatin dose of IC50 11.08 μg and 8.12 μg had 50% cytotoxic effect on MDA-MB-231 at 24 hours and 48 hours. The presence CM along with cisplatin reduced the cytotoxic effect of cisplatin drug. The IC50 value of cisplatin drug was enhanced to 18.75 μg in the presence of CM along with cisplatin.
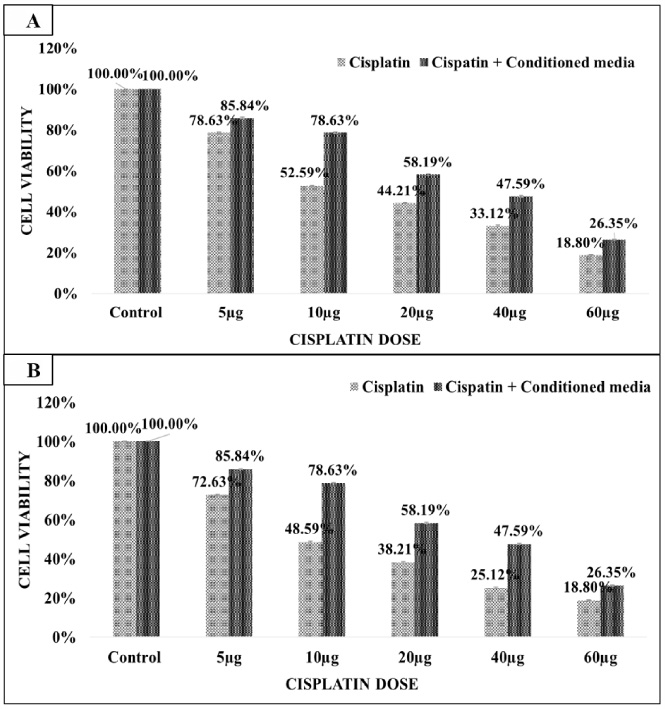
3.5.Treatment of MDA-MB-468 cells with doxorubicin and conditioned media from CAFs of triple negative breast cancer
MDA-MB-468 cells were treated with doxorubicin drug alone and in combination with CM (collected from CAFs isolated from triple negative breast cancer tissue). MDA-MB-468 cells were treated with 25 nM, 50 nM, 75 nM, 100 nM, 125 nM, and 150 nM doses of doxorubicin for 24 hours and 48 hours (Fig. 5A & B). Doxorubicin treatment reduced the MDA-MB-468 cell viability at both times points. MDA-MB-468 cell death at 48 hours was slightly more (52.98%) than at 24 hours (48.92%). Calculated IC50 value of doxorubicin was 75 nM at 24 hours and 48 hours. Further, the presence of CM along with doxorubicin reduced its cytotoxic effect on MDA-MB-468. At the 75 nM IC50 values the cell viability of cell lines was increased from 52.92% to 75.18% after 24 hours and 48.92% to 55.08% at 48 hours treatments. The calculated IC50 of doxorubicin plus CM combination was 128 nM and 100 nM at 24 hours and 48 hours (Table 1).
Fig. 5.
(A&B) MDA-MB-486 cells treatment with doxorubicin for 24 hours and 48 hours. A doxorubicin dose of IC50 75 nM had 50% cytotoxic effect on MDA-MB-468. The presence CM along with doxorubicin reduced the cytotoxic effect of doxorubicin drug. The IC50 value of doxorubicin drug alone was 75 nM and IC50 of doxorubicin and CM combination was 128 nM and 100 nM at 24 hours and 48 hours respectively.
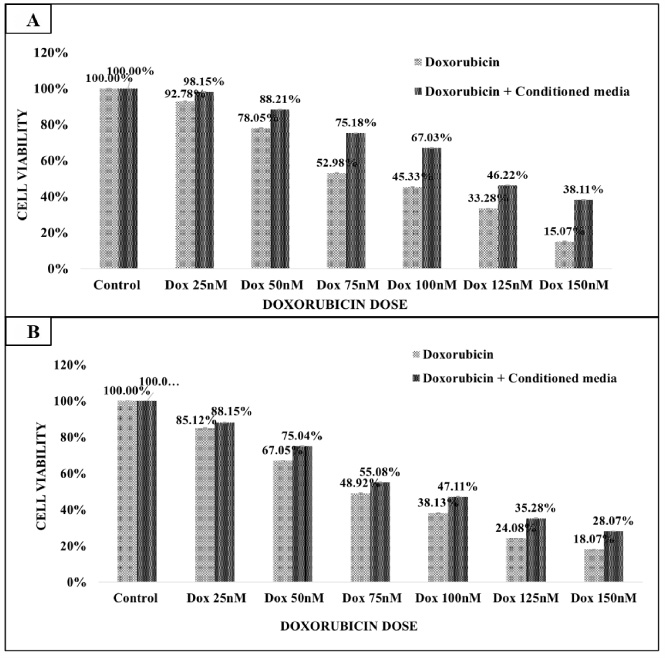
3.6.Identification of constituents of secretome
Liquid chromatography–mass spectrometry identified different proteins in secretome collected from CAFs of different molecular subtypes. Comparative analysis showed differential protein expression between molecular subtypes (Fig. 6, Supplementary Table S1). Heatmap was generated with the top 26 differentially expressed proteins, which showed distinct abundance of proteins in different subtypes. Luminal A subtype had high abundance of thrombospondin-1 and apolipoprotein A-IV. Inter-alpha-trypsin inhibitor heavy chain H1 and H2 had high abundance in secretome of Luminal B subtype. Similarly, prothrombin, laminin subunit beta-2, and collagen alpha-1 (III) chain were highly expressed in Her-2 positive subtype. Inter-alpha-trypsin inhibitor heavy chain H1, histone H2A type 3 and alpha-2-HS-glycoprotein proteins had high expression in the secretome of TNBC CAFs. Protein-protein network interaction with string analysis showed that few of the proteins from Luminal A/B and Her-2 positive subtypes had functional association networks (Supplementary Fig. S2a–c).
Fig. 6.
Heatmap generated with top 26 proteins from CAFs secretome of different molecular subtypes. Breast cancer molecular subtypes had differential expression of proteins.
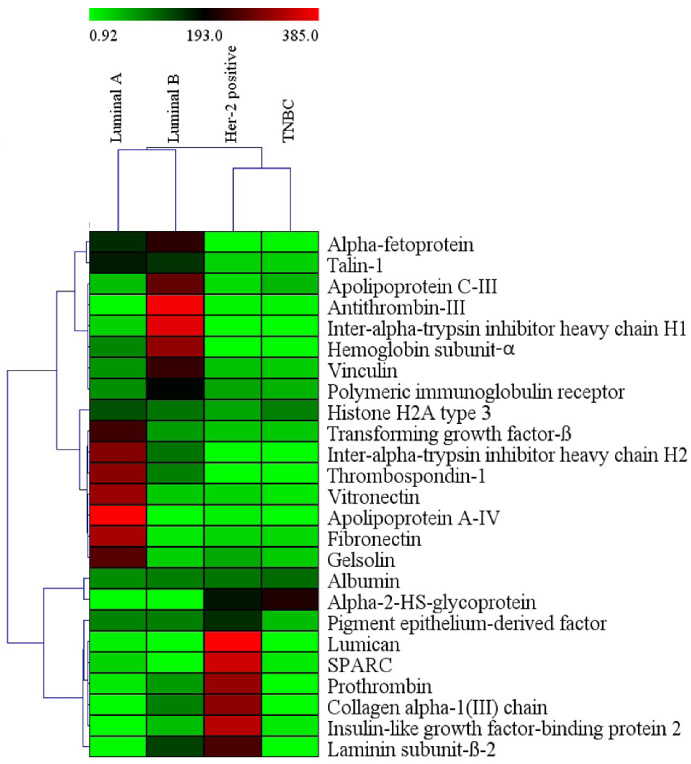
4.Discussion
The CAFs which are the dominant component in TME, has tumor-supportive roles and induce cancer cell invasion, metastasis, and chemoresistance [13]. CAFs can induce the therapeutic resistance, which is one of the main causes for high mortality in breast cancer, however this role has never been elucidated before. Anti-cancer drugs, impair the cancer cell activities through multiple pathways which also alters the TME. The CAFs secrete numerous cytokines that activate signalling cascades to prevent the elimination of cancer cells and possibly cause resistance and recurrence. In the present study, in-vitro experiments were performed to decipher the chemoresistance effect of CAFs across the different molecular subtypes of breast cancer. Conditioned media (CM) or secretome collected from cultured CAFs isolated from each subtype of breast cancer was used along with the drugs in the molecular subtype specific breast cancer cell lines to evaluate the cytotoxic inhibitory effect of CM on anti-cancer drugs. Breast cancer cell lines including MCF-7 (estrogen receptor positive), SK-BR3 (Her-2 positive), MDA-MB-231, MDA-MB-468 (TNBC) were treated with tamoxifen, trastuzumab, cisplatin, and doxorubicin respectively as done in the clinical settings. The results of in-vitro experiments showed that the treatment with anti-cancer drugs had growth inhibitory effect on breast cancer cell lines, however in the presence of CM the anti-cancer effects of each drug was reduced. CAFs secretome reduced 15–20% of anti-tumor activity of used anti-cancer drugs. These findings suggesting that CAFs secretome exhibited tumorigenic role probably through drug resistance mechanism.
Previous studies have determined that co-culturing of breast cancer cells and CAFs, increased proliferation rate of cancer cells by regulating the metabolism of CAFs [14]. Orimo et al. [15] observed that CAFs derived from breast cancers remarkably increase the tumor proliferation and angiogenesis. Brechbuhl et al. [16] indicated that subtypes specific CAFs impact the sensitivity of Luminal breast cancer to tamoxifen or estrogen therapy. In their study, the co-culture of tumor cells with breast cancer CAFs reduced the sensitivity of cancer cells towards tamoxifen. The decreased tamoxifen sensitivity was due to interaction between fibronectin, FGF, and β1 integrin and activation of MAPK/ERK 1/2 and PI3K/AKT cascades [17,18]. CAF derived IL6 activates JAK/STAT3 signalling and downregulates PTEN, stimulating stemness property, leading to trastuzumab resistance in HER-2 positive subtype [19–23]. CAFs secretome also affects the other constituent of the TME; like stromal VEGF can drive angiogenesis [24,25]. Several cytokines and chemokines released by CAFs, act on a variety of CD8+T cells, Treg cells, and macrophages and lead to immunosuppressive and immune-promoting consequences [26].
To determine the composition CAF secretome, LCMS analysis was performed which identified more than 200 proteins. Differential expression/comparative analysis was done using fibroblast secretome from normal breast tissue and then within the molecular subtypes. We noted that each molecular subtype of breast cancer had distinct protein profile. For example, Luminal A had enrichment of vitronectin, fibronectin, apolipoprotein, and TGF-β; Luminal B had antithrombin-III, apolipoprotein C-III, and Vinculin; HER-2 positive had Lumican, SPARC, collagen-α-I, and insulin like growth factor-binding protein 2 enrichment. The presence of different proteins in the CAFs secretome confirmed the heterogenous nature of TME and hence phenotypic heterogeneity across breast cancer subtypes. Most of CAF secretome proteins were ECM components, cell to matrix adhesion proteins, and matrix to matrix adhesion proteins. CAFs can affect multiple aspects of tumor progression either by secreting soluble stimuli or by remodeling the ECM. Fibronectin has been considered a CAFs hallmarkers which supports the tumor growth and invasion by regulating the ECM contractility. One of the components of scretome was TGF-𝛽1 which is known to induce EMT in tumour cells by paracrine signalling and promote aggressive phenotype of breast cancer cells. This might be one of the mechanisms for acquiring metastatic potential and drug resistance in breast cancer cells with different biological characteristics [27]. Other component of secretome was vitronectin which prominently accumulates in the ECM of tumor cells of various carcinomas, especially breast cancer cells and binds with Integrin αv𝛽3, to enhance angiogenesis and neovascularization of tissues [28]. High serum levels of vitronectin in Her-2 positive breast cancer has been proposed as a blood marker in breast cancer and indicator of relapse [29]. Further, collagen an abundant ECM protein has been associated with aggressive behaviour of tumor [30].
5.Conclusion
In vitro studies, showed that tamoxifen, herceptin, cisplatin, and doxorubicin anti-cancer drugs had a significant cytotoxic effect on subtype specific breast cancer cell line. However, treatment of anti-cancer drugs in combination with conditioned media isolated from molecular subtype specific CAFs, reduced the cytotoxic effect of the drugs. Conditioned media treatment resulted in more IC50 value of each anti-cancer drug, thus highlighting the effect of tumour microenvironment especially CAFs on drug resistance. Further, LCMS data highlighted different composition of secretome from different breast cancer associated fibroblasts, thus highlighting the heterogeneity of the CAFs. Thus, present study determined chemoresistance effect of CAFs and also highlighted their functional heterogeneity across the molecular subtypes of breast cancer based on distinct composition of their secretome.
Conflict of interest
Author(s) declared no conflict of interest.
Funding
This study was funded by Indian Council of Medical Research (ICMR), New Delhi, India (5/13/14/2020-NCD-III).
Author contributions
All authors contributed to study conception and design of the work. All authors commented on previous versions of the manuscript. All authors read and approved the final manuscript.
Dr. Dharambir Kashyap: Contributed in data collection, analysis and manuscript writing.
Dr. Shalomoli Bhattacharya: Planning the experiments, interpretation of results, and Data analysis.
Dr. Ashim Das: Interpretation of results and critically reviewing the manuscript.
Dr. Gurpreet Singh, Dr. Santosh Irinike and Dr. Siddhant Khare: Interpretation of results and critically reviewing the manuscript.
Dr. Amanjit Bal: Study conception, data analysis and manuscript writing.
References
[1] | Sung H, , Ferlay J, , Siegel RL, , Laversanne M, , Soerjomataram I, , Ahmedin DMV , Global Cancer Statistics 2020: GLOBOCAN estimates of incidence and mortality worldwide for 36 cancers in 185 countries, CA: A Can J Clin, 71: : 209–249, (2020) . |
[2] | Luond F, , Tiede S, , Christofori G, Breast cancer as an example of tumour heterogeneity and tumour cell plasticity during malignant progression, Br J Cancer, 125: : 164–175, (2021) . |
[3] | Turner KM, , Yeo SK, , Holm TM, , Shaughnessy E, , Guan JL, Heterogeneity within molecular subtypes of breast cancer, Am J Physiol - Cell Physiology, 321: : C343–C354, (2021) . |
[4] | Fragomeni SM, , Sciallis A, , Jeruss JS, Molecular subtypes and local-regional control of breast cancer, Surgical Oncology Clinics of North America, 27: : 95–120, (2018) . |
[5] | Lu P, , Weaver VM, , Werb Z, The extracellular matrix: a dynamic niche in cancer progression, J Cell Biol, 196: : 395–406, (2012) . |
[6] | Sugimoto H, , Mundel TM, , Kieran MW, , Kalluri R, Identification of fibroblast heterogeneity in the tumor microenvironment, Cancer Biol Ther, 5: : 1640–1646, (2006) . |
[7] | Xing F, , Saidou J, , Watabe K, Cancer associated fibroblasts (CAFs) in tumor microenvironment, Front Biosci-Landmark, 15: : 166–179, (2010) . |
[8] | Senthebane DA, , Rowe A, , Thomford NE, , Shipanga H, , Munro D, , Mazeedi MAM, The role of tumor microenvironment in chemoresistance: To survive, keep your enemies closer, Int J Mol Sci, 18: : 1586, (2017) . |
[9] | Skolekova S, , Matuskova M, , Bohac M, , Toro L, , Demkova L, , Gursky J, Cisplatin-induced mesenchymal stromal cells-mediated mechanism contributing to decreased antitumor effect in breast cancer cells, Cell Commun Signal, 14: (2016) . |
[10] | Shiga K, , Hara M, , Nagasaki T, , Sato T, , Takahashi H, , Takeyama H, Cancer-associated fibroblasts: Their characteristics and their roles in tumor growth, Cancers (Basel), 7: : 2443–2458, (2015) . |
[11] | Van der Spek YM, , Kroep JR, , Tollenaar RAEM, , Mesker WE, Chemotherapy resistance and stromal targets in breast cancer treatment: A review, Mol Biol Rep, 47: : 8169–8177, (2020) . S Waise, R Parker, MJJ Rose-Zerilli, DM Layfield. |
[12] | Wood O, , West J, , Ottensmeier CH, , Thomas GJ, , Hanley CJ, An optimized method to isolate human fibroblasts from tissue for ex vivo analysis, Bio Protoc, 9: : e3440, (2019) . |
[13] | Yoshihiro M, , Akira O, Phenotypic heterogeneity, stability and plasticity intumor-promoting carcinoma-associated fibroblasts, The FEBS J, 289: : 2429–2447, (2022) . |
[14] | McCuaig R, , Wu F, , Dunn J, , Rao S, , Dahlstrom JE, The biological and clinical significance of stromal-epithelial interactions in breast cancer, Pathology, 49: : 133–140, (2017) . |
[15] | Orimo A, , Gupta PB, , Sgroi DC, , Arenzana SF, , Delaunay T, , Naeem R , Stromal fibroblasts present in invasive human breast carcinomas promote tumor growth and angiogenesis through elevated SDF-1/CXCL12 secretion, Cell, 121: : 335–348, (2005) . |
[16] | Brechbuhl HM, , Finlay SJ, , Yamamoto TM, , Gillen AE, , Cittelly DM, , Tan AC , Fibroblast subtypes regulate responsiveness of luminal breast cancer to estrogen, Clin Cancer Res, 23: : 1710–1721, (2017) . |
[17] | Loeffler M, , Krüger JA, , Niethammer AG, , Reisfeld RA, Targeting tumor-associated fibroblasts improves cancer chemotherapy by increasing intratumoral drug uptake, J Clin Invest, 116: : 1955–1962, (2006) . |
[18] | Pontiggia O, , Sampayo R, , Raffo D, , Motter A, , Xu R, , Bissell MJ , The tumor microenvironment modulates tamoxifen resistance in breast cancer: A role for soluble stromal factors and fibronectin through β1 integrin, Breast Cancer Res Treat, 133: : 459–471, (2012) . |
[19] | Mao Y, , Zhang Y, , Qu Q, , Zhao M, , Lou Y, , Liu J , Cancer-associated fibroblasts induce trastuzumab resistance in HER2 positive breast cancer cells, Mol BioSystems, 11: : 1029–1040, (2015) . |
[20] | Shi Y, , Gao W, , Lytle NK, , Huang P, , Yuan X, , Dann AM , Targeting LIF-mediated paracrine interaction for pancreatic cancer therapy and monitoring, Nature, 569: : 131–135, (2019) . |
[21] | Cazet AS, , Hui MN, , Elsworth BL, , Wu SZ, , Roden D, , Chan CL , Targeting stromal remodeling and cancer stem cell plasticity overcomes chemoresistance in triple negative breast cancer, Nat Commun, 9: : 1–18, (2018) . |
[22] | Tape CJ, , Ling S, , Dimitriadi M, , McMahon KM, , Worboys JD, , Leong HS , Oncogenic KRAS regulates tumor cell signaling via stromal reciprocation, Cell, 165: : 910–920, (2016) . |
[23] | Bruzzese F, , Hagglof C, , Leone A, , Sjöberg E, , Roca MS, , Kiflemariam S , Local and systemic protumorigenic effects of cancer-associated fibroblast-derived GDF15, Cancer Res, 274: : 3408–3417, (2014) . |
[24] | O’Connell JT, , Sugimoto H, , Cooke VG, , MacDonald BA, , Mehta AI, , LeBleu VS , VEGF-A and Tenascin-C produced by S100A4+ stromal cells are important for metastatic colonization, PNAS, 108: : 16002–16007, (2011) . |
[25] | Bin HMM, , Manping HMM, , Qin LBS, Cancer associated fibroblasts promote angiogenesis of hepatocellular carcinoma by VEGF-mediated EZH2/VASH1 pathway, Tech Cancer Res Treat, 18: (2019) . 1533033819879905. |
[26] | Monteran L, , Erez N, The dark side of fibroblasts: Cancer-associated fibroblasts as mediators of immunosuppression in the tumor microenvironment, Front Immunol, 10: : 1835, (2019) . |
[27] | Yu YY, , Xiao CH, , Tan LD, , Wang QS, , Li XQ, , Feng YM, Cancer-associated fibroblasts induce epithelial-mesenchymal transition of breast cancer cells through paracrine TGF-β signalling, Br J Cancer, 110: : 724–732, (2014) . |
[28] | Qiu X, , Tan H, , Fu D, , Zhu Y, , Zhang J, Laminin is over expressed in breast cancer and facilitate cancer cell metastasis, J Cancer Res Therapy S1170–S1172, (2018) . |
[29] | Bera A, , Subramanian M, , Karaian J, , Eklund M, , Radhakrishnan S, , Gana N , Functional role of vitronectin in breast cancer, PLoS One, 15: : e0242141, (2020) . |
[30] | Jansson MM, , Lindberg J, , Rask G, , Svensson J, , Billing O, , Nazemroaya A , Prognostic value of stromal type IV collagen expression in small invasive breast cancers, Front Molecular Biosci, 9: : 904526, (2022) . |