Exercise Improves Vascular Function, but does this Translate to the Brain?
Abstract
The number of adults with Alzheimer’s disease (AD) or related dementia is expected to increase exponentially. Interventions aimed to reduce the risk and progression of AD and dementia are critical to the prevention and treatment of this devastating disease. Aging and cardiovascular disease risk factors are associated with reduced vascular function, which can have important clinical implications, including brain health. The age-associated increase in blood pressure and impairment in vascular function may be attenuated or even reversed through lifestyle behaviors. Greater volumes of habitual exercise and higher cardiorespiratory fitness are associated with beneficial effects on vascular health and cognition. Exercise and cardiorespiratory fitness may be most important during midlife, as physical activity and cardiorespiratory fitness during the middle-aged years are associated with future cognitive function. The extent to which exercise, and more specifically aerobic exercise, influences the cerebral circulation is not well established. In this review, we present our working hypothesis showing how cerebrovascular function may be a mediating factor underlying the association between exercise and cognition, as well as discuss recent studies evaluating the effect of exercise interventions on the cerebral circulation.
INTRODUCTION
According to the Alzheimer’s Association, one in three adults over 65 years of age die with Alzheimer’s disease (AD) or another related dementia [1]. Between the years 2000 and 2014, mortality from cardiovascular disease, stroke, and HIV declined; however, the number of deaths attributed to AD increased by 89% [1]. By 2025, an estimated 7.1 million adults in the U.S. will have AD, not including other dementias [1]. Because no cure for AD exists, there is a critical need to develop interventions to delay or prevent AD and related dementias.
Adults with cardiovascular disease have a greater incidence of having cognitive impairment compared with adults without cardiovascular disease [2]. Furthermore, even in the absence of established cardiovascular disease, vascular risk factors such as hypertension, diabetes, and obesity during midlife are associated with lower brain volumes and impaired cognitive function 10 years later [3]. The idea that cardiovascular health is relevant to brain structure and function has been highlighted by the American Heart Association and American Stroke Association who stated that the same risk factors for stroke are also likely risk factors for AD [4]. Better vascular health during midlife is associated with higher cognitive function [5]. An effective way to improve vascular health and promote healthy vascular aging is through lifestyle modifications, including habitual aerobic exercise, which may act to support cerebrovascular health. Epidemiological evidence has demonstrated that physical activity and exercise are associated with preserved cognitive function [6–8]. In support of this, Barnes & Yaffe calculated population attributable risks that included the prevalence of the risk factor and the strength of association of that risk factor with AD diagnosis. Of all of the modifiable risk factors for AD (including diabetes, hypertension, obesity, smoking, depression, physical inactivity, and cognitive inactivity), increasing the proportion of the population who are physically active by 25% was statistically the most effective measure in countering AD [9]. Thus, although cardiovascular risk factors may be detrimental to brain health, habitual aerobic exercise may be a promising intervention to improve vascular health and in turn prevent or delay the onset of age-related cognitive decline. This review will discuss the hypothesis that regular exercise, and specifically regular exercise associated with improved cardiorespiratory fitness, enhances cognition through the beneficial effects of exercise on vascular health. While we are not the only group of investigators who are actively working on this hypothesis, this area is relatively undeveloped, with many unanswered research questions. Therefore, this review is limited to the available literature and relies on the previous correlative and cross-sectional studies showing associations between exercise and vascular health, as systematic, randomized control trials are currently ongoing and the results are not yet available [10–12].
PHYSICAL ACTIVITY, FITNESS AND COGNITION
To a large extent, the work establishing the link between physical activity and cognition is dependent on subjective information regarding participants’ recall of the volume of leisure time physical activity performed or on objective physiological information quantifying the level of cardiorespiratory fitness. Both subjective measures of physical activity participation and objectively measured cardiorespiratory fitness have been positively associated with cognition. For example, older adults who reported walking more blocks per week had a lower risk of cognitive impairment 9 years later [13]. Similarly, cardiorespiratory fitness measured during an incremental exercise test was correlated with executive function test scores [14, 15].
Lifelong physical activity and high levels of cardiorespiratory fitness are important for brain health as humans age. In a recent study by Horder, et al., higher cardiorespiratory fitness in women at midlife was associated with lower risk of dementia approximately 44 years later [16]. In addition, Nyberg et al. reported that greater cardiorespiratory fitness levels at age 18 were associated with a lower hazard ratio for development of mild cognitive impairment (MCI) or dementia [17]. Taken together, these results suggest that cardiorespiratory fitness and lifestyle behaviors during the young and middle-aged years are important in determining future risk of cognitive decline.
Several studies have performed randomized control trials in sedentary adults or adults with low initial physical activity levels in order to measure the effect of increased physical activity participation on cognition. The results of these trials have shown no change [18, 19] or improvements [20–22] in cognition, with improvements occurring specifically in adults with high adherence rates. While there is discrepancy in the literature in regard to the effectiveness of exercise interventions on improving cognition, the majority of studies that measure cardiorespiratory fitness and demonstrate an increase in cardiorespiratory fitness as a result of the intervention, have reported improvement in cognitive outcomes [23, 24]. In fact, a study by Vironi, et al. has shown that the magnitude of change in cardiorespiratory fitness, rather than the dose of exercise administered during the intervention, was a better predictor of cognitive benefit [23]. Thus, while the volume of exercise employed in randomized control trials is important, the improvement in cardiorespiratory fitness may be crucial to achieving improvements in cognitive function.
As mentioned above, greater volumes of physical activity and higher levels of cardiorespiratory fitness are associated with improved cognitive function and decreased risk of cognitive decline. One factor underlying the beneficial effects of physical activity or fitness on cognition is the maintenance of brain volume or reduced atrophy rates [14]. Longitudinal assessments of brain volumes have demonstrated that atrophy occurs with advancing age [25], particularly after 65 years of age [26], and this brain atrophy is accelerated in adults who progress to MCI or AD [27]. Importantly, older adults who reported walking more than 72 blocks per week demonstrated greater grey matter volume compared with adults who reported walking less [13]. Similarly, higher cardiorespiratory fitness in older adults was positively associated with greater hippocampal volume [28–30] and lower rates of age-related decline in grey matter, particularly in the prefrontal, superior parietal, and temporal cortices [30]. Therefore, physical activity and exercise appear to mitigate the atrophy rates of grey matter, hippocampal, and whole brain volumes, which are considered biomarkers of cognitive decline.
Another factor that could explain some of the beneficial effects of regular exercise on cognition is the maintenance of cerebral blood flow (CBF). Global CBF declines with age [31–33], which may be due to regional atrophy (although reduced CBF has been shown independent of regional atrophy [31]), lower cerebral metabolism, increased cerebrovascular resistance, or vascular dysfunction [33]. In older adults, there is a positive association between self-reported volumes of physical activity and CBF in grey matter regions [34]. Similarly, studies investigating blood flow parameters in the middle cerebral artery (MCA) also report an association between cardiorespiratory fitness and MCA blood velocity [35]. CBF declines with age, and greater global CBF or MCA blood velocity may be protective in reducing the negative impact of aging on brain health. This hypothesis is based on the idea that: 1) greater levels of CBF may prevent or slow the accumulation of neuropathology, in particular amyloid-β [36, 37]; and 2) greater levels of CBF may allow for higher cognitive reserve for a given level of neuropathological burden [38, 39].
While physical activity recall, physical activity tracking (e.g. steps per day using physical activity trackers), and cardiorespiratory fitness (e.g. VO2max) are used in quantifying physical activity and exercise, the mechanisms underlying the cognitive effects of physical activity vs. fitness may be different. Increasing physical activity participation and reducing sedentary behaviors have a variety of cardiovascular and metabolic benefits; however, higher cardiorespiratory fitness typically observed in lifelong exercisers may provide additional advantages. When comparing healthy middle-aged and older adults who met physical activity guidelines to adults with a history of lifelong endurance exercise, grey matter volumes were statistically higher in lifelong endurance exercisers [40]. Additionally, cortical thickness in these lifelong endurance exercisers was higher in several regions of the brain and cortical thickness was positively correlated with cardiorespiratory fitness [40]. In addition, Voss, et al. has shown that higher cardiorespiratory fitness, but not physical activity, was associated with functional connectivity in several brain regions [41]. Taken together, these results suggest that interventions designed to increase cardiorespiratory fitness, rather than simply increasing physical activity volumes or reducing sedentary behavior, may offer the greatest neuroprotection in middle-aged and older adults.
An important consideration of the aforementioned studies is that cardiorespiratory fitness has substantial variability and heritability, with maximal heritability estimates of ∼50% in sedentary individuals [42]. Thus, the positive association between cardiorespiratory fitness may be partially driven by genetic predisposition in sedentary individuals as cardiorespiratory fitness is not only influenced by the volume and intensity of exercise one participates in. Accordingly, many well-designed studies involving both sedentary and habitually exercising individuals quantify both exercise training volume and cardiorespiratory fitness levels. Even if an individual has a genetic predisposition for a high cardiorespiratory fitness, this potential advantage could be lost in the absence of regular physical activity, especially over the lifespan. In fact, studies that examine trainability of cardiorespiratory fitness have shown that, even in individuals with “low trainability”, once a greater volume of exercise is added, cardiorespiratory fitness improves to the levels of individuals with “high trainability” (see reference [43] for review). Therefore, exercise interventions should aim to increase both exercise training volume and cardiorespiratory fitness in adults with a range of initial fitness levels and determine the potential effect on brain health.
One of the hallmarks of regular endurance exercise and endurance exercise training in previously sedentary individuals is enhanced cardiovascular function [44]. Enhanced cardiovascular function is associated with a range of benefits to many organ systems and it is likely that the cardiovascular adaptations associated with exercise also influence brain health. Therefore, the enhancement in cardiovascular function may mediate the relationship between fitness, brain structure, cerebral blood flow and cognition [45].
BLOOD PRESSURE AND COGNITION
There is significant evidence that elevated cardiovascular risk is linked to cognitive decline, highlighting the importance of vascular contributions to development of AD and dementia. Hypertension, and specifically hypertension during the middle-aged years, is a major vascular risk factor for cognitive decline [4, 46]. Hypertensive adults have a higher propensity for developing cognitive impairment compared with normotensive adults [47, 48]. A murine model of hypertension (transverse aortic coarctation) demonstrated that hypertension led to amyloid- deposition, and reduced learning capacity, likely through oxidative stress pathways [49]. Even in the absence of hypertension, elevated diastolic blood pressure is associated with cognitive impairment in humans [48, 50]. Similarly, pulse pressure (the difference between systolic and diastolic blood pressure) is also linked to cognitive decline [51, 52]. In an angiotensin II (ANG II) model of hypertension in mice, the effect of hypertension on myogenic autoregulation and disruption of the blood-brain barrier is further exacerbated by aging, which suggests a synergistic effect of hypertension and aging on brain health [49, 53].
The association between blood pressure characteristics and cognition illustrates the importance of the structure and function of the vasculature in the development of cognitive decline. Autoregulatory mechanisms should maintain adequate blood flow to the brain while protecting the delicate cerebral microvessels, despite exposure to a high-pressure environment. Yet, isolated systolic hypertension and high pulse pressure may surpass the autoregulatory curve, preventing the necessary protection of the cerebral microvessels [52]. In an ANG II mouse model of hypertension, high blood pressure augmented the progression of brain pathologies [54]. In humans, higher brachial blood pressure is associated with lower CBF, a potential biomarker of cognitive decline [55]. The presence of hypertension also increases cerebral microbleeds [56, 57] and white matter hyperintensities [58, 59], which are also important biomarkers of cognitive risk. Blood pressure measured in the aorta and carotid arteries may be more indicative of the pulsatile exposure experienced by the cerebral circulation, when compared to brachial blood pressure. We and others have shown an association between aortic blood pressure characteristics and white matter hyperintensities in middle-aged adults [60], normotensive postmenopausal women [61] and elderly adults [62].
Importantly, the relationship between blood pressure and cognition is not consistent throughout the lifespan. For example, pulse pressure and its association with cognitive function is highly dependent on both age and baseline systolic blood pressure [63]. Managing blood pressure during midlife is associated with improved cognitive outcomes [64]. Yet, low blood pressure, often due to aggressive pharmacological intervention, is associated with cognitive impairment in older adults [65–67]. The widening of pulse pressure and isolated systolic hypertension with increasing age is due in part to an increase in central arterial stiffness. In young adults, there is a mismatch of vascular impedance between the central elastic arteries and the peripheral muscular arteries [68]. As humans age, the increase in central arterial stiffness reduces the central-to-peripheral impedance mismatch, resulting in greater transmission of the pulsatile energy to the peripheral vasculature. With age-associated increases in arterial stiffness, higher pulsatile flow will reach the microvessels in vulnerable high flow organs such as the brain and kidneys [69, 70], potentially causing damage. Aggressive blood pressure lowering therapy in the elderly, without a concomitant reduction in arterial stiffness, may not have a beneficial effect on cognitive function. Thus, this highlights the complex relationship between blood pressure control and the effect on brain structure and function.
ARTERIAL STIFFNESS AND COGNITION
An increase in age-related blood pressure at rest may be due to a parallel increase in the rigidity of the large arteries. As the structure of the large central arteries stiffens (due to structural characteristics like increased collagen deposition, elastin degeneration, and advanced glycation end-product cross-linking, and/or functional characteristics like increased sympathetic nerve activity or reduced nitric oxide (NO) bioavailability; see [71] for review), the large arteries are less capable of dampening the pulsatile flow from the heart. Thus, with increased arterial stiffness and arteriosclerosis, which may precede clinically-relevant increases in blood pressure, higher pulsatile flow will reach the microvessels [69, 70]. If this happens in highly vascularized tissue like the brain, damage to the microcirculation will ultimately impair organ function, as mentioned above.
Aortic pulse wave velocity (PWV) is the gold standard technique to measure arterial stiffness of the large central arteries in humans [72]. Large epidemiology studies, like Framingham, have shown that higher aortic PWV is associated with lower working memory scores [73] and associated with the progression of cognitive decline [74]. Changes in brain structure and cerebrovascular function have been suggested to mediate the relationship between arterial stiffness and cognition. Cooper, et al. demonstrated that vascular resistance in the cerebral vessels and white matter hyperintensity fraction explained 41% of the relationship between aortic PWV and memory scores [75]. Thus, interventions aimed to improve or reduce arterial stiffness may be important in mitigating the risk of cognitive decline.
VASCULAR FUNCTION AND COGNITION
Endothelial dysfunction may underlie the age-related increases in arterial stiffness. The endothelial layer of the blood vessels is responsible for producing vasoactive substances, or endothelial-derived relaxing factors (EDRFs). For example, when endothelial cells experience an increase in shear stress, this initiates a signaling cascade resulting in the release of EDRFs, including NO and prostacyclin, which diffuse into the adjacent vascular smooth muscle cells (VSMCs) to cause VSMC relaxation/vasodilation [76]. Peripheral endothelial function is commonly quantified from the magnitude of endothelium-dependent vasodilation (EDD) either using intra-arterial infusions of vasodilating substances (i.e. acetylcholine) and measuring the change in blood flow [77] or by occlusion of blood flow for several minutes and quantifying the magnitude of vasodilation upon release of blood flow restriction (flow-mediated dilation; FMD) [78]. Because endothelial dysfunction is likely systemic, previous studies have evaluated EDD (often in the arm or leg) and determined its association with biomarkers of cognitive decline. In adults with cardiovascular disease, brachial artery FMD was inversely associated with white matter hyperintensities such that individuals with worse FMD demonstrated greater white matter hyperintensity volume [79]. FMD was also positively associated with working memory activation in middle-aged adults [80]. Other studies have shown that patients with AD demonstrated lower brachial artery FMD compared with healthy controls, and that FMD was associated with clinical severity of AD [81]. Similarly, adults with MCI also demonstrated impaired FMD compared with age-matched controls [82]. Taken together, these studies suggest that systemic endothelial dysfunction is present in patients with MCI and AD, and may be important in both the development and progression of cognitive decline.
Although several studies have shown impaired FMD in MCI or AD patients or a relationship between FMD and cognitive variables, this is not consistent across all cognitive domains. In fact, a recent systematic review demonstrated that worsening FMD is associated with lower executive function, but not correlated with global cognition, memory, or processing speed [83]. Some limitations noted in this review state that the studies often are not powered to investigate the confounding effect of medications or traditional cardiovascular disease risk factors. Future studies should evaluate the impact of improving endothelial function, and thereby FMD (via pharmacological or lifestyle interventions), and determine the effect on neuropathology and/or cognition.
While the literature strongly suggests the role of vascular and hemodynamic influences in the development and progression of cognitive decline, studies reporting associations between vascular function or hemodynamic variables and cognition have several notable limitations. First, causality cannot be determined by these correlative relationships. Second, as noted above, many of these studies are underpowered to adjust for the confounding variables, making it difficult to interpret these results. Third, the few studies that have longitudinal data on cognition are subject to practice effects and test-retest variability, which could either underestimate or overestimate the effects of vascular health on cognition. Finally, a single measure of cognitive function is often used to represent a cognitive domain, which may result in an inaccurate or incomplete assessment of cognitive abilities. These limitations highlight the need for systematic, randomized control trials to further establish the role of vascular dysfunction in cognitive decline.
EXERCISE AND VASCULAR FUNCTION
Age-associated impairments in vascular function may be attenuated or even reversed through changing lifestyle behaviors. The association between habitual exercise or cardiorespiratory fitness and cognition may be mediated by the beneficial effect on vascular function. Thus, exercise is a promising potential intervention for individuals at risk for AD or dementia.
Age-associated vascular dysfunction primarily manifests as increased central arterial stiffness and lower EDD, which can have wide-ranging effects on organ function. Fortunately, there is evidence that habitual exercise is able to reduce central arterial stiffness and improve EDD, in addition to attenuating the age-associated impairment in vascular function. For example, exercise-trained middle-aged and older adults demonstrate favorable aortic PWV [84, 85] and EDD [86, 87] when compared to age-matched sedentary adults. Additionally, both aortic PWV and EDD are improved after a short-term exercise intervention in middle-aged adults [86, 88]. Importantly, greater cardiorespiratory fitness is associated with lower aortic PWV [84, 85] and higher EDD [87, 89] in adults throughout the lifespan (Fig. 1).
Fig. 1.
The relationship between cardiorespiratory fitness (VO2max) and aortic pulse wave velocity (PWV), a measure of central arterial stiffness, in 53 healthy women. Adapted with permission from Tanaka, et al. [85].
![The relationship between cardiorespiratory fitness (VO2max) and aortic pulse wave velocity (PWV), a measure of central arterial stiffness, in 53 healthy women. Adapted with permission from Tanaka, et al. [85].](https://content.iospress.com:443/media/bpl/2018/4-1/bpl-4-1-bpl180075/bpl-4-bpl180075-g001.jpg)
Despite these promising results in healthy adults, exercise-derived improvements in vascular function may not be universal. Exercise-related changes in peripheral vascular function in women may be estrogen-dependent, as there is evidence that exercise does not enhance FMD in postmenopausal women without impaired endothelial function [90, 91], unless they are given supplemental estradiol [90]. Similarly, a recent review suggests that there are conflicting results on the effect of exercise interventions on vascular function in individuals with type 2 diabetes (T2D) [92]. One study reported no improvement in FMD in adults with T2D after a 6-month supervised exercise intervention, despite improvements in cardiorespiratory fitness [93]. Collectively, these studies suggest that the magnitude of improvement in vascular function may be dependent on the initial health and/or presence of comorbidities in the sample population. Finally, it is important to note that, although many studies suggest that age-associated increases in arterial stiffness precede age-associated elevations in blood pressure [94], arterial stiffness and blood pressure are inter related and could both change in a parallel manner. Due to this association, exercise-induced reductions in arterial stiffness may be partially influenced by exercise-induced reductions in blood pressure.
The majority of published studies in healthy adults demonstrate exercise-related improvements in vascular function, which are likely the result of multiple mechanisms. During exercise, the increase in muscle blood flow increases shear stress in the blood vessels supplying the skeletal muscle, which persists throughout muscle contraction. A local increase in shear stimulus experienced by the endothelial cells enhances NO production through the endothelial NO synthase (eNOS) enzyme. Repeated habitual exercise acutely increases this shear stimulus and results in upregulated NO production. Through repeated exposure, the endothelial cells adapt by increasing eNOS mRNA expression [95] and increasing eNOS phosphorylation [96]. When compared to light intensity exercise, moderate to vigorous intensity exercise results in the greatest increase in endothelial shear stress [97], and is more efficient at improving cardiorespiratory fitness [98]. In support of this idea, cardiorespiratory fitness is associated with EDD in men and women [87, 89]. Thus, changes in eNOS expression and/or function may underlie the exercise-related adaptations in the brachial artery and resistance vessels as a result of exercise training. Furthermore, this favorable adaptation to exercise is prevented when blood flow (and therefore shear stress) is occluded in one arm during an exercise training intervention [99, 100].
Another mechanism underlying exercise-related adaptations in the peripheral vasculature includes oxidative stress, which increases with advancing age [101], and reduces NO bioavailability (due to combination of NO with reactive oxygen species (ROS) and/or uncoupling the eNOS enzyme). Adults who habitually exercise and have greater cardiorespiratory fitness demonstrate lower oxidative stress and higher antioxidant enzyme expression [101, 102] which are related to EDD [101]. Therefore, the reduction in oxidative stress could be secondary to the upregulation of endogenous antioxidant enzymes, an adaptation that could protect against the excess oxidative stress produced during exercise. Structurally, oxidative stress is associated with disadvantageous arterial wall remodeling, specifically collagen I protein expression [103], which is prevented when cells are treated with an antioxidant enzyme mimetic [103]. In an animal model, aerobic exercise has been shown to reduce advanced glycation end-products, which accumulate with age to increase arterial stiffness [104]. Functionally, supraphysiologic administration of ascorbic acid (a potent antioxidant) acutely reduced arterial stiffness [105] and improved EDD [106], strongly suggesting a role of oxidative stress. Interestingly, the reduction in arterial stiffness and improvement in EDD with ascorbic acid infusion only occurred in older sedentary adults and not in young adults, or those who were already habitually active [106, 107], highlighting the role of ROS in vascular aging and the benefit of habitual exercise in mitigating this effect.
To date, there have been many published reports examining the effect of exercise (particularly aerobic exercise) on arterial stiffness and peripheral vascular function, yet there are still many unresolved questions and further studies are needed. For example, some studies report no improvement in EDD after an exercise training invention, which may be due to methodological/technical limitations in quantifying the shear stimulus. The magnitude of the vasodilatory response should account for the magnitude of the shear stimulus to properly compare the effect of an intervention. Additionally, there is evidence that light intensity exercise involving a large muscle mass may not increase shear stress in vessels of non-exercising tissue [108]. Interventions that utilize light intensity exercise may not detect a significant increase in EDD after training because the increase in shear stress during light intensity is insufficient. Yet another unresolved issue is the contrasting results regarding the effectiveness of an exercise intervention on vascular function in postmenopausal women and adults with T2D, as mentioned above. More research is necessary to determine optimal interventions to improve vascular function in these populations.
An important and often overlooked factor to consider when evaluating the role of exercise on vascular function is the mode and intensity of exercise. Many exercise interventions utilize moderate intensity aerobic exercise; however, there are contrasting results with resistance exercise interventions regarding whether they induce beneficial or maladaptive changes in vascular function. For example, several studies have reported an increase in arterial stiffness in healthy adults as a result of a high intensity resistance exercise intervention [109–111]. Even under the umbrella of aerobic exercise, the mode of aerobic exercise may also be important as arterial stiffness was lower in runners, swimmers, and rowers, compared with age-matched sedentary adults [112, 113], but FMD was not different between swimmers and sedentary adults [113]. Thus, future studies are necessary to elucidate the mechanism underlying exercise-induced adaptations and specify which exercise interventions are most effective and what populations benefit the most from these interventions.
TRANSLATION OF VASCULAR ADAPTATIONS TO THE CEREBRAL CIRCULATION
While the beneficial effects of exercise on the peripheral circulation are more established, there is also evidence that exercise in general enhances the health of the cerebral circulation. Specifically, cardiorespiratory fitness was associated with improved resting MCA blood velocity in men [35, 114]. Similarly, cardiorespiratory fitness was associated with increased MCA cerebral vasodilator responses (a measure of cerebrovascular function) in young [114] and older [114, 115] healthy adults (Fig. 2). There is also evidence that cerebral vasodilator responses to metabolic stimuli are impaired in adults with low cardiorespiratory fitness [116], demonstrating a potential mechanism underlying the association between exercise and cerebrovascular function. Along these lines, Tarumi, et al. reported correlations between measures of cerebral and peripheral vascular function (cerebral vasodilator responses and FMD) and measures of cognition (memory and executive function) [117]. Furthermore, the relationship between cardiorespiratory fitness and measures of cognition in this study were abolished after controlling for cerebral and peripheral vascular function [117], which strongly suggests the link between exercise and cognition may be mediated by improvements in cerebrovascular function.
Fig. 2.
The relationship between cardiorespiratory fitness (VO2max) and cerebrovascular function (CVRCO2) in older adults as a function of exercise training status. CVRCO2 indicates cerebral vasodilator responses to carbon dioxide. Adapted with permission from Bailey, et al. [114].
![The relationship between cardiorespiratory fitness (VO2max) and cerebrovascular function (CVRCO2) in older adults as a function of exercise training status. CVRCO2 indicates cerebral vasodilator responses to carbon dioxide. Adapted with permission from Bailey, et al. [114].](https://content.iospress.com:443/media/bpl/2018/4-1/bpl-4-1-bpl180075/bpl-4-bpl180075-g002.jpg)
The effect of higher cardiorespiratory fitness on cerebral vasodilator responses may be due, in part, to the effect of habitual aerobic exercise on the extracranial arteries supplying the brain. As mentioned above, increased arterial stiffness may result in a greater magnitude of hemodynamic forces transmitted to the delicate cerebral microvessels, resulting in microvascular damage and remodeling that ultimately impairs their function. In an animal model of arterial stiffness (mice with reduced elastin content), cerebral vasodilator responses of the MCA were lower when compared to wild type mice, indicating cerebrovascular dysfunction [118]. Over time, impaired cerebral microvessel function may impair neuronal function, limit the clearance of amyloid-β, increase neuropathology, and manifest as reduced cognitive function. Demonstrating this, increased carotid arterial stiffness is associated with increased prevalence of neuropathology and lower cognition [62], which may be mediated by impaired cerebral microvessel function. Additionally, carotid arterial stiffness is associated with the progression of cognitive decline in individuals with MCI [119]. While studies have linked aortic stiffness (PWV) to cognition [73–75], the stiffness of the carotid arteries may be a stronger predictor of cerebrovascular health and cognitive outcomes, as the common carotid arteries branch directly (left) or indirectly (right) off of the aortic arch. Due to the early branching from the aorta, the blood flow that reaches the carotid arteries is considerably pulsatile, with little elastic dampening. With increased stiffness of the carotid arteries, a greater amount of this pulsatile energy may reach the intracranial arteries. In support of this theory, carotid arterial stiffness, but not aortic stiffness, was inversely associated with cognition in adults [120]. Aerobic exercise has been shown to reduce carotid arterial stiffness [107, 121]; therefore, these beneficial adaptations may result in a more favorable hemodynamic profile, thus enhancing cerebrovascular function.
The improvements in cerebrovascular function in habitually exercising animals or adults may also be due to adaptations as a result of regular increases in blood flow to the brain. Blood flow to the brain increases during aerobic exercise up to approximately 60% VO2max, then it plateaus, and decreases with any further increases in intensity [122, 123]. The amount of the increase in blood flow is likely dependent on many other factors, such as the amount of muscle mass active, the mode of exercise, and skeletal muscle afferent feedback mechanisms. During these increases in CBF during exercise, the cerebral vessels experience increases in shear stress that parallel the increase in blood flow. With habitual exercise, the cerebrovasculature experiences repeated, acute increases in shear stress that likely result in shear-mediated intracellular signaling cascades similar to the peripheral circulation. While previous studies have shown that age is associated with unfavorable adaptations in the cerebral vessels [124, 125] and impaired cerebral vasodilator responses [126], aerobic exercise may prevent or attenuate these age-associated changes. Evidence of this is limited in humans; however, animal models have demonstrated that habitual exercise may be able to prevent age-associated cerebrovascular dysfunction. We have recently shown that habitually exercising adults do not demonstrate an age-related decline in cerebral vasodilator responses of the MCA [127]. In middle-aged mice with access to voluntary running wheels, there are distinct differences in cerebrovascular structure and function, with greater endothelial cell nuclei size (Fig. 3) compared to sedentary animals [128]. Similarly, mice with access to a running wheel also have greater EDD of the cerebral arteries [129]. If exercise directly improves cerebrovascular function, it may also lead to enhanced astrocyte function. Aging is associated with astrocyte hypertrophy and increased reactivity, which may be due to astrocytes attempting to compensate for impairments in cerebrovascular function. If exercise enhances the function of the cerebrovasculature, it may attenuate the age-associated increases in hypertrophy and reactivity, due to the synergistic relationship between cerebral microvessels and astrocytes. This could, in turn, prevent neuronal hypoperfusion and hypometabolism that is a result of impaired blood flow delivery.
Fig. 3.
Scanning electron micrographs comparing cerebrovascular microstructure of sedentary and exercised middle-aged mice. A) Low magnification images of the middle cerebral artery (MCA) (single arrow) with branch (double arrow) in a sedentary (left) and exercised (right) mouse. Arteries are distinguished from veins (arrowhead) by the distinct impressions made by their endothelial cell nuclei (ECN). B) High magnification images of ECN imprints from the MCA in a sedentary (left) and exercised (right) mouse. C and D) Exercise increased the area of ECN in the MCA and its associated branches. *p < 0.005, t-test. Adapted with permission from Latimer, et al. [128].
![Scanning electron micrographs comparing cerebrovascular microstructure of sedentary and exercised middle-aged mice. A) Low magnification images of the middle cerebral artery (MCA) (single arrow) with branch (double arrow) in a sedentary (left) and exercised (right) mouse. Arteries are distinguished from veins (arrowhead) by the distinct impressions made by their endothelial cell nuclei (ECN). B) High magnification images of ECN imprints from the MCA in a sedentary (left) and exercised (right) mouse. C and D) Exercise increased the area of ECN in the MCA and its associated branches. *p < 0.005, t-test. Adapted with permission from Latimer, et al. [128].](https://content.iospress.com:443/media/bpl/2018/4-1/bpl-4-1-bpl180075/bpl-4-bpl180075-g003.jpg)
In summary, exercise may serve to preserve cerebrovascular function and neuronal function, and subsequently cognition, through multiple mechanisms. Aging is associated with increased blood pressure, arterial stiffness, and endothelial dysfunction. Enhanced vascular function or amelioration in the age-related increases in arterial stiffness and endothelial dysfunction with habitual aerobic exercise likely translates to the cerebral circulation (Fig. 4). Yet, this hypothesis has undergone limited testing.
Fig. 4.
Our working hypothesis showing that aging is associated with an adverse cardiovascular profile, including increased blood pressure, arterial stiffness, and endothelial dysfunction, which could all contribute to cerebrovascular dysfunction, and ultimately, cognitive decline. Individuals at elevated risk of cognitive decline may experience “accelerated aging”. Aerobic exercise appears to attenuate vascular aging and may be directly or indirectly associated with preserved cerebrovascular function throughout the lifespan. Solid lines represent mechanisms with strong support from the literature. Dashed lines represent mechanisms that require further research to be definitively established.
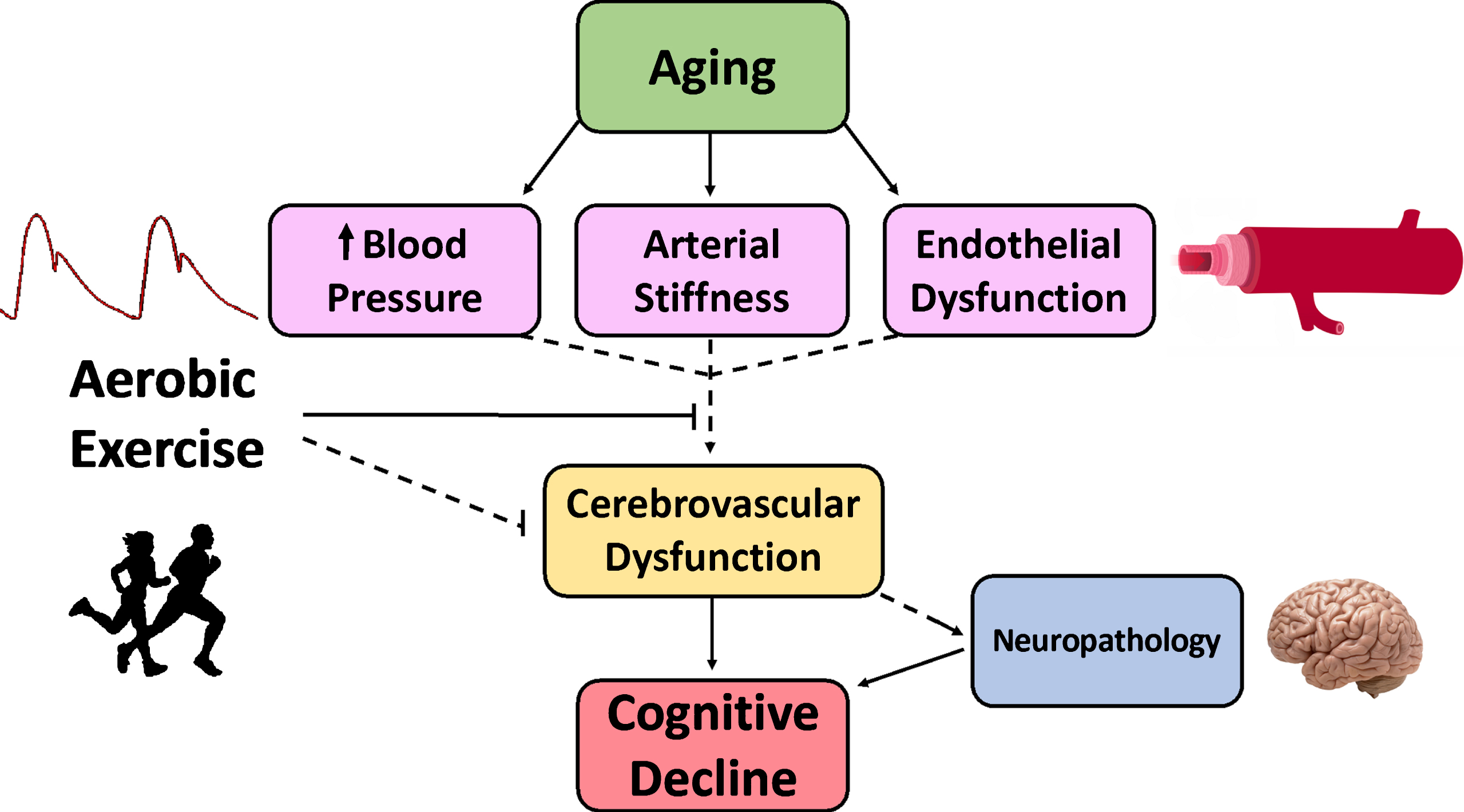
EXERCISE INTERVENTIONS AND CEREBROVASCULAR FUNCTION
Aerobic exercise and cardiorespiratory fitness may be most important during midlife, as physical activity levels and cardiorespiratory fitness during the middle-aged years are associated with future cognitive function [16, 130]. Large-scale studies are currently underway to evaluate the effect of exercise interventions in older adults without cognitive complaints on cerebrovascular function [10–12]. These will be important in understanding how increasing the volume of exercise may mitigate the effect of aging on cerebrovascular function in sedentary adults. Small-scale studies have shown that cerebral vasodilator responses to carbon dioxide increased after an aerobic training in healthy adults [131, 132] and in patients with stroke [133]. Although more randomized control trials are necessary, the results of these studies suggest that vascular function in the cerebral vessels can be improved with a short-term aerobic exercise intervention. Furthermore, initiating exercise interventions early (i.e. during midlife), before the onset of cognitive decline, may be the most effective way to lower the risk of cognitive decline.
ADDITIONAL MECHANISMS UNDERLYING THE BENEFIT OF EXERCISE
This review discussed the hypothesis that improved vascular health is one mechanism underlying the association between regular aerobic exercise and enhanced cognitive function. In addition to improvements in vascular health, regular aerobic exercise induces numerous cardiac, musculoskeletal, and metabolic adaptations that may also contribute to the brain. A consistent finding is that exercise can induces neurotrophins and growth factors, such as vascular endothelial growth factor (VEGF) or brain-derived neurotrophic factor (BDNF), but the mechanism by which these are activated and affect the cerebral circulation are less clear. During exercise, skeletal muscle contraction causes the release of numerous biologically-active factors into the circulation. These “myokines” can then affect other tissues through endocrine or paracrine mechanisms that may mediate the systemic effects of regular exercise [134–136]. These myokines may also promote neurogenesis, linking skeletal muscle contraction to brain structure and function. Recent studies have shown that cathepsin B (CTSB) mRNA and protein levels are elevated after exercise in mice, and in CTSB-deficient mice, the exercise-induced improvement in spatial memory is absent [137]. Furthermore, treadmill training increased CTSB levels in monkeys and plasma CTSB is associated with spatial memory in humans [137]. Additionally, the PGC-1α/FDNC5 pathway is activated by aerobic exercise in the hippocampus (similar to skeletal muscle) in mice, which leads to increased hippocampal BDNF [138].
During high-intensity exercise, lactate accumulates in the skeletal muscle and blood lactate concentrations increase. Thus, studies have investigated lactate as a potential link between skeletal muscle contraction and brain adaptations. High-intensity exercise in mice, sufficient to elevate blood lactate levels, increases VEGF expression [139]. Lactate injection has been shown to mimic the effect of exercise [139, 140] and increase BDNF concentration in humans [141]. Along these lines, exercise at a high enough intensity to promote lactate production in humans is associated with improved motor memory and executive function [142, 143]. Taken together, these studies demonstrate that numerous byproducts of skeletal muscle contraction may contribute to neurogenesis, cerebral angiogenesis, and enhanced brain function, if the exercise is of sufficient intensity and repeated regularly.
SUMMARY
Advancing age is the largest risk factor for developing AD or other dementias. The epidemiological and experimental evidence strongly suggests that cardiovascular risk factors and/or poor vascular health is positively associated with cognitive decline. As blood vessel health is associated with both brain structure and brain function, vascular health is likely a key mechanism by which regular aerobic exercise improves cognitive function. The World Health Organization estimates that 25% of adults, globally, are not meeting the minimum physical activity recommendations. The high percentage of adults who are physically inactive will have a substantial impact on future brain health. Importantly, higher volumes of exercise and greater cardiorespiratory fitness are associated with favorable vascular function and the majority of studies show that aerobic exercise interventions improve vascular function in healthy adults. Aerobic exercise could directly increase cerebral angiogenesis, preserve the function of existing cerebral microvessels, or enhance extracranial artery function to protect the cerebral microvasculature from the unfavorable transmission of hemodynamic forces. Because direct evidence on the effect of exercise on cerebrovascular function in humans is currently limited, future studies are necessary to determine the effect of aerobic exercise on cerebrovascular function and elucidate the potential underlying mechanisms. Furthermore, additional research is necessary to optimize and implement exercise interventions early in the treatment or prevention of cognitive decline.
CONFLICTS OF INTEREST
The authors have no conflicts of interest to report.
ACKNOWLEDGMENTS
Thank you to Kathleen Miller for her critical review of the manuscript and Spencer Contreras for assistance with the figures. This work is funded by National Institute of Health grant HL118154 (JNB) and Alzheimer’s Association grant 17-499398 (JNB).
REFERENCES
[1] | Association As. 2017 Alzheimer’s Disease Facts and Figures. Alzheimer’s & Dementia: The Journal of the Alzheimer’s Association. (2017) ;13: :325–73. |
[2] | Roberts RO , Geda YE , Knopman DS , Cha RH , Pankratz VS , Boeve BF , et al. Cardiac disease associated with increased risk of nonamnestic cognitive impairment: Stronger effect on women. JAMA Neurol. (2013) ;70: (3):374–82. |
[3] | Debette S , Seshadri S , Beiser A , Au R , Himali JJ , Palumbo C , et al. Midlife vascular risk factor exposure accelerates structural brain aging and cognitive decline. Neurology. (2011) ;77: (5):461–8. |
[4] | Gorelick PB , Scuteri A , Black SE , Decarli C , Greenberg SM , Iadecola C , et al. Vascular contributions to cognitive impairment and dementia: A statement for healthcare professionals from the american heart association/american stroke association. Stroke; A Journal of Cerebral Circulation. (2011) ;42: (9):2672–713. |
[5] | Gonzalez HM , Tarraf W , Harrison K , Windham BG , Tingle J , Alonso A , et al. Midlife cardiovascular health and 20-year cognitive decline: Atherosclerosis Risk in Communities Study results. Alzheimers Dement.(2017) . |
[6] | Ahlskog JE , Geda YE , Graff-Radford NR , Petersen RC . Physical exercise as a preventive or disease-modifying treatment of dementia and brain aging. Mayo Clin Proc. (2011) ;86: (9):876–84. |
[7] | Kirk-Sanchez NJ , McGough EL . Physical exercise and cognitive performance in the elderly: Current perspectives. Clin Interv Aging. (2014) ;9: :51–62. |
[8] | Zhu N , Jacobs DR Jr , Schreiner PJ , Yaffe K , Bryan N , Launer LJ , et al. Cardiorespiratory fitness and cognitive function in middle age: The CARDIA study. Neurology. (2014) ;82: (15):1339–46. |
[9] | Barnes DE , Yaffe K . The projected effect of risk factor reduction on Alzheimer’s disease prevalence. Lancet Neurology. (2011) ;10: (9):819–28. |
[10] | Tyndall AV , Davenport MH , Wilson BJ , Burek GM , Arsenault-Lapierre G , Haley E , et al. The brain-in-motion study: Effect of a 6-month aerobic exercise intervention on cerebrovascular regulation and cognitive function in older adults. BMC Geriatr. (2013) ;13: :21. |
[11] | Leeuwis AE , Hooghiemstra AM , Amier R , Ferro DA , Franken L , Nijveldt R , et al. Design of the ExCersion-VCI study: The effect of aerobic exercise on cerebral perfusion in patients with vascular cognitive impairment. Alzheimers Dement (N Y). (2017) ;3: (2):157–65. |
[12] | Brown BM , Rainey-Smith SR , Castalanelli N , Gordon N , Markovic S , Sohrabi HR , et al. Study protocol of the Intense Physical Activity and Cognition study: The effect of high-intensity exercise training on cognitive function in older adults. Alzheimers Dement (N Y). (2017) ;3: (4):562–70. |
[13] | Erickson KI , Raji CA , Lopez OL , Becker JT , Rosano C , Newman AB , et al. Physical activity predicts gray matter volume in late adulthood: The Cardiovascular Health Study. Neurology. (2010) ;75: (16):1415–22. |
[14] | Weinstein AM , Voss MW , Prakash RS , Chaddock L , Szabo A , White SM , et al. The association between aerobic fitness and executive function is mediated by prefrontal cortex volume. Brain Behav Immun. (2012) ;26: (5):811–9. |
[15] | Brown AD , McMorris CA , Longman RS , Leigh R , Hill MD , Friedenreich CM , et al. Effects of cardiorespiratory fitness and cerebral blood flow on cognitive outcomes in older women. Neurobiology of Aging. (2010) ;31: (12):2047–57. |
[16] | Horder H , Johansson L , Guo X , Grimby G , Kern S , Ostling S , et al. Midlife cardiovascular fitness and dementia: A 44-year longitudinal population study in women. Neurology. (2018) ;90: (15):e1298–e305. |
[17] | Nyberg J , Aberg MA , Schioler L , Nilsson M , Wallin A , Toren K , et al. Cardiovascular and cognitive fitness at age 18 and risk of early-onset dementia. Brain. (2014) ;137: (Pt 5):1514–23. |
[18] | Sink KM , Espeland MA , Castro CM , Church T , Cohen R , Dodson JA , et al. Effect of a 24-Month Physical Activity Intervention vs Health Education on Cognitive Outcomes in Sedentary Older Adults: The LIFE Randomized Trial. JAMA. (2015) ;314: (8):781–90. |
[19] | Toots A , Littbrand H , Bostrom G , Hornsten C , Holmberg H , Lundin-Olsson L , et al. Effects of Exercise on Cognitive Function in Older People with Dementia: A Randomized Controlled Trial. J Alzheimers Dis. (2017) ;60: (1):323–32. |
[20] | Baker LD , Frank LL , Foster-Schubert K , Green PS , Wilkinson CW , McTiernan A , et al. Effects of aerobic exercise on mild cognitive impairment: A controlled trial. Arch Neurol. (2010) ;67: (1):71–9. |
[21] | Hoffmann K , Sobol NA , Frederiksen KS , Beyer N , Vogel A , Vestergaard K , et al. Moderate-to-High Intensity Physical Exercise in Patients with Alzheimer’s Disease: A Randomized Controlled Trial. J Alzheimers Dis. (2016) ;50: (2):443–53. |
[22] | Nishiguchi S , Yamada M , Tanigawa T , Sekiyama K , Kawagoe T , Suzuki M , et al. A 12-Week Physical and Cognitive Exercise Program Can Improve Cognitive Function and Neural Efficiency in Community-Dwelling Older Adults: A Randomized Controlled Trial. J Am Geriatr Soc. (2015) ;63: (7):1355–63. |
[23] | Vidoni ED , Johnson DK , Morris JK , Van Sciver A , Greer CS , Billinger SA , et al. Dose-Response of Aerobic Exercise on Cognition: A Community-Based, Pilot Randomized Controlled Trial. PLoS One. (2015) ;10: (7):e0131647. |
[24] | Voss MW , Heo S , Prakash RS , Erickson KI , Alves H , Chaddock L , et al. The influence of aerobic fitness on cerebral white matter integrity and cognitive function in older adults: Results of a one-year exercise intervention. Hum Brain Map. (2013) ;34: (11):2972–85. |
[25] | Resnick SM , Pham DL , Kraut MA , Zonderman AB , Davatzikos C . Longitudinal magnetic resonance imaging studies of older adults: A shrinking brain. J Neurosci. (2003) ;23: (8):3295–301. |
[26] | Crivello F , Tzourio-Mazoyer N , Tzourio C , Mazoyer B . Longitudinal assessment of global and regional rate of grey matter atrophy in 1,172 healthy older adults: Modulation by sex and age. PLoS One. (2014) ;9: (12):e114478. |
[27] | Jack CR Jr , Shiung MM , Gunter JL , O’Brien PC , Weigand SD , Knopman DS , et al. Comparison of different MRI brain atrophy rate measures with clinical disease progression in AD. Neurology. (2004) ;62: (4):591–600. |
[28] | Erickson KI , Prakash RS , Voss MW , Chaddock L , Hu L , Morris KS , et al. Aerobic fitness is associated with hippocampal volume in elderly humans. Hippocampus. (2009) ;19: (10):1030–9. |
[29] | Erickson KI , Voss MW , Prakash RS , Basak C , Szabo A , Chaddock L , et al. Exercise training increases size of hippocampus and improves memory. Proceedings of the National Academy of Sciences of the United States of America. (2011) ;108: (7):3017–22. |
[30] | Colcombe SJ , Erickson KI , Raz N , Webb AG , Cohen NJ , McAuley E , et al. Aerobic fitness reduces brain tissue loss in aging humans. The Journals of Gerontology Series A, Biological Sciences and Medical Sciences. (2003) ;58: (2):176–80. |
[31] | Chen JJ , Rosas HD , Salat DH . Age-associated reductions in cerebral blood flow are independent from regional atrophy. Neuroimage. (2011) ;55: (2):468–78. |
[32] | Liu W , Lou X , Ma L . Use of 3D pseudo-continuous arterial spin labeling to characterize sex and age differences in cerebral blood flow. Neuroradiology. (2016) ;58: (9):943–8. |
[33] | Tarumi T , Ayaz Khan M , Liu J , Tseng BY , Parker R , Riley J , et al. Cerebral hemodynamics in normal aging: Central artery stiffness, wave reflection, and pressure pulsatility. J Cereb Blood Flow Metab. (2014) ;34: (6):971–8. |
[34] | Zimmerman B , Sutton BP , Low KA , Fletcher MA , Tan CH , Schneider-Garces N , et al. Cardiorespiratory fitness mediates the effects of aging on cerebral blood flow. Front Aging Neurosci. (2014) ;6: :59. |
[35] | Ainslie PN , Cotter JD , George KP , Lucas S , Murrell C , Shave R , et al. Elevation in cerebral blood flow velocity with aerobic fitness throughout healthy human ageing. J Physiol. (2008) ;586: (16):4005–10. |
[36] | Mattsson N , Tosun D , Insel PS , Simonson A , Jack CR Jr , Beckett LA , et al. Association of brain amyloid-beta with cerebral perfusion and structure in Alzheimer’s disease and mild cognitive impairment. Brain. (2014) ;137: (Pt 5):1550–61. |
[37] | Cselenyi Z , Farde L . Quantification of blood flow-dependent component in estimates of beta-amyloid load obtained using quasi-steady-state standardized uptake value ratio. J Cereb Blood Flow Metab. (2015) ;35: (9):1485–93. |
[38] | Stern Y . Cognitive reserve in ageing and Alzheimer’s disease. Lancet Neurology. (2012) ;11: (11):1006–12. |
[39] | Davenport MH , Hogan DB , Eskes GA , Longman RS , Poulin MJ . Cerebrovascular reserve: The link between fitness and cognitive function? Exercise and Sport Sciences Reviews. (2012) ;40: (3):153–8. |
[40] | Wood KN , Nikolov R , Shoemaker JK . Impact of Long-Term Endurance Training vs. Guideline-Based Physical Activity on Brain Structure in Healthy Aging. Front Aging Neurosci. (2016) ;8: :155. |
[41] | Voss MW , Weng TB , Burzynska AZ , Wong CN , Cooke GE , Clark R , et al. Fitness, but not physical activity, is related to functional integrity of brain networks associated with aging. Neuroimage. (2016) ;131: :113–25. |
[42] | Bouchard C , Daw EW , Rice T , Perusse L , Gagnon J , Province MA , et al. Familial resemblance for VO2max in the sedentary state: The HERITAGE family study. Med Sci Sports Exerc. (1998) ;30: (2):252–8. |
[43] | Joyner MJ , Lundby C . Concepts About V O2max and Trainability Are Context Dependent. Exerc Sport Sci Rev. (2018) ;46: (3):138–43. |
[44] | Hellsten Y , Nyberg M . Cardiovascular Adaptations to Exercise Training. Compr Physiol. (2015) ;6: (1):1–32. |
[45] | Gauthier CJ , Lefort M , Mekary S , Desjardins-Crepeau L , Skimminge A , Iversen P , et al. Hearts and minds: Linking vascular rigidity and aerobic fitness with cognitive aging. Neurobiol Aging. (2015) ;36: (1):304–14. |
[46] | Iadecola C , Yaffe K , Biller J , Bratzke LC , Faraci FM , Gorelick PB , et al. Impact of Hypertension on Cognitive Function: A Scientific Statement From the American Heart Association. Hypertension. (2016) ;68: (6):e67–e94. |
[47] | Hajjar I , Quach L , Yang F , Chaves PH , Newman AB , Mukamal K , et al. Hypertension, white matter hyperintensities, and concurrent impairments in mobility, cognition, and mood: The Cardiovascular Health Study. Circulation. (2011) ;123: (8):858–65. |
[48] | Novak V , Hajjar I . The relationship between blood pressure and cognitive function. Nat Rev Cardiol. (2010) ;7: (12):686–98. |
[49] | Carnevale D , Mascio G , D’Andrea I , Fardella V , Bell RD , Branchi I , et al. Hypertension induces brain beta-amyloid accumulation, cognitive impairment, and memory deterioration through activation of receptor for advanced glycation end products in brain vasculature. Hypertension. (2012) ;60: (1):188–97. |
[50] | Tsivgoulis G , Alexandrov AV , Wadley VG , Unverzagt FW , Go RC , Moy CS , et al. Association of higher diastolic blood pressure levels with cognitive impairment. Neurology. (2009) ;73: (8):589–95. |
[51] | Yasar S , Ko JY , Nothelle S , Mielke MM , Carlson MC . Evaluation of the effect of systolic blood pressure and pulse pressure on cognitive function: The Women’s Health and Aging Study II. PLoS One. (2011) ;6: (12):e27976. |
[52] | Thorin-Trescases N , de Montgolfier O , Pincon A , Raignault A , Caland L , Labbe P , et al. The impact of pulse pressure on cerebrovascular events leading to age-related cognitive decline. Am J Physiol Heart Circ Physiol. (2018) . |
[53] | Toth P , Tucsek Z , Tarantini S , Sosnowska D , Gautam T , Mitschelen M , et al. IGF-1 deficiency impairs cerebral myogenic autoregulation in hypertensive mice. J Cereb Blood Flow Metab. (2014) ;34: (12):1887–97. |
[54] | Cifuentes D , Poittevin M , Dere E , Broqueres-You D , Bonnin P , Benessiano J , et al. Hypertension accelerates the progression of Alzheimer-like pathology in a mouse model of the disease. Hypertension. (2015) ;65: (1):218–24. |
[55] | Deverdun J , Akbaraly TN , Charroud C , Abdennour M , Brickman AM , Chemouny S , et al. Mean arterial pressure change associated with cerebral blood flow in healthy older adults. Neurobiol Aging. (2016) ;46: :49–57. |
[56] | Klarenbeek P , van Oostenbrugge RJ , Rouhl RP , Knottnerus IL , Staals J . Higher ambulatory blood pressure relates to new cerebral microbleeds: 2-year follow-up study in lacunar stroke patients. Stroke. (2013) ;44: (4):978–83. |
[57] | van Dooren M , Staals J , de Leeuw PW , Kroon AA , Henskens LH , van Oostenbrugge RJ . Progression of brain microbleeds in essential hypertensive patients: A 2-year follow-up study. Am J Hypertens. (2014) ;27: (8):1045–51. |
[58] | Verhaaren BF , Vernooij MW , de Boer R , Hofman A , Niessen WJ , van der Lugt A , et al. High blood pressure and cerebral white matter lesion progression in the general population. Hypertension. (2013) ;61: (6):1354–9. |
[59] | Aribisala BS , Morris Z , Eadie E , Thomas A , Gow A , Valdes Hernandez MC , et al. Blood pressure, internal carotid artery flow parameters, and age-related white matter hyperintensities. Hypertension. (2014) ;63: (5):1011–8. |
[60] | Shrestha I , Takahashi T , Nomura E , Ohtsuki T , Ohshita T , Ueno H , et al. Association between central systolic blood pressure, white matter lesions in cerebral MRI and carotid atherosclerosis. Hypertens Res. (2009) ;32: (10):869–74. |
[61] | Barnes JN , Harvey RE , Zuk SM , Lundt ES , Lesnick TG , Gunter JL , et al. Aortic hemodynamics and white matter hyperintensities in normotensive postmenopausal women. J Neurol. (2017) ;264: (5):938–45. |
[62] | Kearney-Schwartz A , Rossignol P , Bracard S , Felblinger J , Fay R , Boivin JM , et al. Vascular structure and function is correlated to cognitive performance and white matter hyperintensities in older hypertensive patients with subjective memory complaints. Stroke; A Journal of Cerebral Circulation. (2009) ;40: (4):1229–36. |
[63] | McDade E , Sun Z , Lee CW , Snitz B , Hughes T , Chang CC , et al. The association between pulse pressure change and cognition in late life: Age and where you start matters. Alzheimers Dement (Amst). (2016) ;4: :56–66. |
[64] | Swan GE , DeCarli C , Miller BL , Reed T , Wolf PA , Jack LM , et al. Association of midlife blood pressure to late-life cognitive decline and brain morphology. Neurology. (1998) ;51: (4):986–93. |
[65] | Mossello E , Pieraccioli M , Nesti N , Bulgaresi M , Lorenzi C , Caleri V , et al. Effects of low blood pressure in cognitively impaired elderly patients treated with antihypertensive drugs. JAMA Intern Med. (2015) ;175: (4):578–85. |
[66] | Foster-Dingley JC , van der Grond J , Moonen JE , van den Berg-Huijsmans AA , de Ruijter W , van Buchem MA , et al. Lower Blood Pressure Is Associated With Smaller Subcortical Brain Volumes in Older Persons. Am J Hypertens. (2015) ;28: (9):1127–33. |
[67] | Shang S , Li P , Deng M , Jiang Y , Chen C , Qu Q . The Age-Dependent Relationship between Blood Pressure and Cognitive Impairment: A Cross-Sectional Study in a Rural Area of Xi’an, China. PLoS One. (2016) ;11: (7):e0159485. |
[68] | Nichols WW , O’Rourke MF. McDonalds’s Blood Flow in Arteries: Theoretical, Experimental and Clinical Principles. 5th ed. London: Hodder Arnold Publishing; (2005) . |
[69] | O’Rourke MF , Safar ME . Relationship between aortic stiffening and microvascular disease in brain and kidney: Cause and logic of therapy. Hypertension. (2005) ;46: (1):200–4. |
[70] | Mitchell GF , van Buchem MA , Sigurdsson S , Gotal JD , Jonsdottir MK , Kjartansson O , et al. Arterial stiffness, pressure and flow pulsatility and brain structure and function: The Age, Gene/Environment Susceptibility–Reykjavik study. Brain. (2011) ;134: (Pt 11):3398–407. |
[71] | Lacolley P , Regnault V , Segers P , Laurent S . Vascular Smooth Muscle Cells and Arterial Stiffening: Relevance in Development, Aging, and Disease. Physiol Rev. (2017) ;97: (4):1555–617. |
[72] | Townsend RR , Wilkinson IB , Schiffrin EL , Avolio AP , Chirinos JA , Cockcroft JR , et al. Recommendations for Improving and Standardizing Vascular Research on Arterial Stiffness: A Scientific Statement From the American Heart Association. Hypertension. (2015) ;66: (3):698–722. |
[73] | Tsao CW , Seshadri S , Beiser AS , Westwood AJ , Decarli C , Au R , et al. Relations of arterial stiffness and endothelial function to brain aging in the community. Neurology. (2013) ;81: (11):984–91. |
[74] | Tsao CW , Himali JJ , Beiser AS , Larson MG , DeCarli C , Vasan RS , et al. Association of arterial stiffness with progression of subclinical brain and cognitive disease. Neurology. (2016) ;86: (7):619–26. |
[75] | Cooper LL , Woodard T , Sigurdsson S , van Buchem MA , Torjesen AA , Inker LA , et al. Cerebrovascular Damage Mediates Relations Between Aortic Stiffness and Memory. Hypertension. (2016) ;67: (1):176–82. |
[76] | Vanhoutte PM , Shimokawa H , Feletou M , Tang EH . Endothelial dysfunction and vascular disease - a 30th anniversary update. Acta Physiol (Oxf). (2017) ;219: (1):22–96. |
[77] | Panza JA , Quyyumi AA , Brush JE Jr , Epstein SE . Abnormal endothelium-dependent vascular relaxation in patients with essential hypertension. N Engl J Med. (1990) ;323: (1):22–7. |
[78] | Anderson EA , Mark AL . Flow-mediated and reflex changes in large peripheral artery tone in humans. Circulation. (1989) ;79: (1):93–100. |
[79] | Hoth KF , Tate DF , Poppas A , Forman DE , Gunstad J , Moser DJ , et al. Endothelial function and white matter hyperintensities in older adults with cardiovascular disease. Stroke. (2007) ;38: (2):308–12. |
[80] | Gonzales MM , Tarumi T , Tanaka H , Sugawara J , Swann-Sternberg T , Goudarzi K , et al. Functional imaging of working memory and peripheral endothelial function in middle-aged adults. Brain Cogn. (2010) ;73: (2):146–51. |
[81] | Dede DS , Yavuz B , Yavuz BB , Cankurtaran M , Halil M , Ulger Z , et al. Assessment of endothelial function in Alzheimer’s disease: Is Alzheimer’s disease a vascular disease? J Am Geriatr Soc. (2007) ;55: (10):1613–7. |
[82] | Vendemiale G , Romano AD , Dagostino M , de Matthaeis A , Serviddio G . Endothelial dysfunction associated with mild cognitive impairment in elderly population. Aging Clin Exp Res. (2013) ;25: (3):247–55. |
[83] | Naiberg MR , Newton DF , Goldstein BI . Flow-Mediated Dilation and Neurocognition: Systematic Review and Future Directions. Psychosom Med. (2016) ;78: (2):192–207. |
[84] | Vaitkevicius PV , Fleg JL , Engel JH , O’Connor FC , Wright JG , Lakatta LE , et al. Effects of age and aerobic capacity on arterial stiffness in healthy adults. Circulation. (1993) ;88: (4 Pt 1):1456–62. |
[85] | Tanaka H , DeSouza CA , Seals DR . Absence of age-related increase in central arterial stiffness in physically active women. Arterioscler Thromb Vasc Biol. (1998) ;18: (1):127–32. |
[86] | DeSouza CA , Shapiro LF , Clevenger CM , Dinenno FA , Monahan KD , Tanaka H , et al. Regular aerobic exercise prevents and restores age-related declines in endothelium-dependent vasodilation in healthy men. Circulation. (2000) ;102: (12):1351–7. |
[87] | Rinder MR , Spina RJ , Ehsani AA . Enhanced endothelium-dependent vasodilation in older endurance-trained men. J Appl Physiol. (2000) ;88: (2):761–6. |
[88] | Hayashi K , Sugawara J , Komine H , Maeda S , Yokoi T . Effects of aerobic exercise training on the stiffness of central and peripheral arteries in middle-aged sedentary men. Jpn J Physiol. (2005) ;55: (4):235–9. |
[89] | Lippincott MF , Carlow A , Desai A , Blum A , Rodrigo M , Patibandla S , et al. Relation of endothelial function to cardiovascular risk in women with sedentary occupations and without known cardiovascular disease. Am J Cardiol. (2008) ;102: (3):348–52. |
[90] | Moreau KL , Stauffer BL , Kohrt WM , Seals DR . Essential role of estrogen for improvements in vascular endothelial function with endurance exercise in postmenopausal women. The Journal of Clinical Endocrinology and Metabolism. (2013) ;98: (11):4507–15. |
[91] | Swift DL , Weltman JY , Patrie JT , Saliba SA , Gaesser GA , Barrett EJ , et al. Predictors of improvement in endothelial function after exercise training in a diverse sample of postmenopausal women. J Womens Health (Larchmt). (2014) ;23: (3):260–6. |
[92] | Way KL , Keating SE , Baker MK , Chuter VH , Johnson NA . The Effect of Exercise on Vascular Function and Stiffness in Type 2 Diabetes: A Systematic Review and Meta-analysis. Curr Diabetes Rev. (2016) ;12: (4):369–83. |
[93] | Barone Gibbs B , Dobrosielski DA , Bonekamp S , Stewart KJ , Clark JM . A randomized trial of exercise for blood pressure reduction in type 2 diabetes: Effect on flow-mediated dilation and circulating biomarkers of endothelial function. Atherosclerosis. (2012) ;224: (2):446–53. |
[94] | Laurent S , Boutouyrie P . The structural factor of hypertension: Large and small artery alterations. Circ Res. (2015) ;116: (6):1007–21. |
[95] | Woodman CR , Price EM , Laughlin MH . Shear stress induces eNOS mRNA expression and improves endothelium-dependent dilation in senescent soleus muscle feed arteries. J Appl Physiol (2005) ;98: (3):940–6. |
[96] | Casey DP , Ueda K , Wegman-Points L , Pierce GL . Muscle contraction induced arterial shear stress increases endothelial nitric oxide synthase phosphorylation in humans. Am J Physiol Heart Circ Physiol. (2017) ;313: (4):H854–H9. |
[97] | Birk GK , Dawson EA , Batterham AM , Atkinson G , Cable T , Thijssen DH , et al. Effects of exercise intensity on flow mediated dilation in healthy humans. Int J Sports Med. (2013) ;34: (5):409–14. |
[98] | Milanovic Z , Sporis G , Weston M . Effectiveness of High-Intensity Interval Training (HIT) and Continuous Endurance Training for VO2max Improvements: A Systematic Review and Meta-Analysis of Controlled Trials. Sports Med. (2015) ;45: (10):1469–81. |
[99] | Tinken TM , Thijssen DH , Hopkins N , Dawson EA , Cable NT , Green DJ . Shear stress mediates endothelial adaptations to exercise training in humans. Hypertension. (2010) ;55: (2):312–8. |
[100] | Birk GK , Dawson EA , Atkinson C , Haynes A , Cable NT , Thijssen DH , et al. Brachial artery adaptation to lower limb exercise training: Role of shear stress. J Appl Physiol (2012) ;112: (10):1653–8. |
[101] | Donato AJ , Eskurza I , Silver AE , Levy AS , Pierce GL , Gates PE , et al. Direct evidence of endothelial oxidative stress with aging in humans: Relation to impaired endothelium-dependent dilation and upregulation of nuclear factor-kappaB. Circ Res. (2007) ;100: (11):1659–66. |
[102] | Pierce GL , Donato AJ , LaRocca TJ , Eskurza I , Silver AE , Seals DR . Habitually exercising older men do not demonstrate age-associated vascular endothelial oxidative stress. Aging Cell. (2011) ;10: (6):1032–7. |
[103] | Fleenor BS , Marshall KD , Durrant JR , Lesniewski LA , Seals DR . Arterial stiffening with ageing is associated with transforming growth factor-beta1-related changes in adventitial collagen: Reversal by aerobic exercise. J Physiol. (2010) ;588: (Pt 20):3971–82. |
[104] | Gu Q , Wang B , Zhang XF , Ma YP , Liu JD , Wang XZ . Contribution of receptor for advanced glycation end products to vasculature-protecting effects of exercise training in aged rats. Eur J Pharmacol. (2014) ;741: :186–94. |
[105] | Moreau KL , Gavin KM , Plum AE , Seals DR . Ascorbic acid selectively improves large elastic artery compliance in postmenopausal women. Hypertension. (2005) ;45: (6):1107–12. |
[106] | Eskurza I , Monahan KD , Robinson JA , Seals DR . Effect of acute and chronic ascorbic acid on flow-mediated dilatation with sedentary and physically active human ageing. J Physiol. (2004) ;556: (Pt 1):315–24. |
[107] | Moreau KL , Gavin KM , Plum AE , Seals DR . Oxidative stress explains differences in large elastic artery compliance between sedentary and habitually exercising postmenopausal women. Menopause. (2006) ;13: (6):951–8. |
[108] | Tanaka H , Shimizu S , Ohmori F , Muraoka Y , Kumagai M , Yoshizawa M , et al. Increases in blood flow and shear stress to nonworking limbs during incremental exercise. Med Sci Sports Exerc. (2006) ;38: (1):81–5. |
[109] | Cortez-Cooper MY , DeVan AE , Anton MM , Farrar RP , Beckwith KA , Todd JS , et al. Effects of high intensity resistance training on arterial stiffness and wave reflection in women. Am J Hypertens. (2005) ;18: (7):930–4. |
[110] | Miyachi M , Kawano H , Sugawara J , Takahashi K , Hayashi K , Yamazaki K , et al. Unfavorable effects of resistance training on central arterial compliance: A randomized intervention study. Circulation. (2004) ;110: (18):2858–63. |
[111] | DeVan AE , Anton MM , Cook JN , Neidre DB , Cortez-Cooper MY , Tanaka H . Acute effects of resistance exercise on arterial compliance. J Appl Physiol. (2005) ;98: (6):2287–91. |
[112] | Cook JN , DeVan AE , Schleifer JL , Anton MM , Cortez-Cooper MY , Tanaka H . Arterial compliance of rowers: Implications for combined aerobic and strength training on arterial elasticity. Am J Physiol Heart Circ Physiol. (2006) ;290: (4):H1596–600. |
[113] | Nualnim N , Barnes JN , Tarumi T , Renzi CP , Tanaka H . Comparison of central artery elasticity in swimmers, runners, and the sedentary. Am J Cardiol. (2011) ;107: (5):783–7. |
[114] | Bailey DM , Marley CJ , Brugniaux JV , Hodson D , New KJ , Ogoh S , et al. Elevated aerobic fitness sustained throughout the adult lifespan is associated with improved cerebral hemodynamics. Stroke; A Journal of Cerebral Circulation. (2013) ;44: (11):3235–8. |
[115] | Barnes JN , Taylor JL , Kluck BN , Johnson CP , Joyner MJ . Cerebrovascular reactivity is associated with maximal aerobic capacity in healthy older adults. J Appl Physiol (2013) ;114: (10):1383–7. |
[116] | Fabiani M , Gordon BA , Maclin EL , Pearson MA , Brumback-Peltz CR , Low KA , et al. Neurovascular coupling in normal aging: A combined optical, ERP and fMRI study. Neuroimage. (2014) ;85 Pt 1: :592–607. |
[117] | Tarumi T , Gonzales MM , Fallow B , Nualnim N , Lee J , Pyron M , et al. Cerebral/Peripheral Vascular Reactivity and Neurocognition in Middle-Age Athletes. Med Sci Sports Exerc. (2015) ;47: (12):2595–603. |
[118] | Walker AE , Henson GD , Reihl KD , Morgan RG , Dobson PS , Nielson EI , et al. Greater impairments in cerebral artery compared with skeletal muscle feed artery endothelial function in a mouse model of increased large artery stiffness. J Physiol. (2015) ;593: (8):1931–43. |
[119] | Viticchi G , Falsetti L , Vernieri F , Altamura C , Bartolini M , Luzzi S , et al. Vascular predictors of cognitive decline in patients with mild cognitive impairment. Neurobiol Aging. (2012) ;33: (6):1127 e1–9. |
[120] | Geijselaers SL , Sep SJ , Schram MT , van Boxtel MP , van Sloten TT , Henry RM , et al. Carotid stiffness is associated with impairment of cognitive performance in individuals with and without type 2 diabetes. The Maastricht Study. Atherosclerosis. (2016) ;253: :186–93. |
[121] | Tanaka H , Dinenno FA , Monahan KD , Clevenger CM , DeSouza CA , Seals DR . Aging, habitual exercise, and dynamic arterial compliance. Circulation. (2000) ;102: (11):1270–5. |
[122] | Ide K , Secher NH . Cerebral blood flow and metabolism during exercise. Prog Neurobiol. (2000) ;61: (4):397–414. |
[123] | Moraine JJ , Lamotte M , Berre J , Niset G , Leduc A , Naeije R . Relationship of middle cerebral artery blood flow velocity to intensity during dynamic exercise in normal subjects. Eur J Appl Physiol Occup Physiol. (1993) ;67: (1):35–8. |
[124] | Fonck E , Feigl GG , Fasel J , Sage D , Unser M , Rufenacht DA , et al. Effect of aging on elastin functionality in human cerebral arteries. Stroke. (2009) ;40: (7):2552–6. |
[125] | Diaz-Otero JM , Garver H , Fink GD , Jackson WF , Dorrance AM . Aging is associated with changes to the biomechanical properties of the posterior cerebral artery and parenchymal arterioles. Am J Physiol Heart Circ Physiol. (2016) ;310: (3):H365–75. |
[126] | Barnes JN , Schmidt JE , Nicholson WT , Joyner MJ . Cyclooxygenase inhibition abolishes age-related differences in cerebral vasodilator responses to hypercapnia. J Appl Physiol (1985) ;112: (11):1884–90. |
[127] | Miller KB , Howery AJ , Harvey RE , Eldridge MW , Barnes JN . Cerebrovascular reactivity and central arterial stiffness in habitually exercising healthy adults. Front Physiol.(2018) . |
[128] | Latimer CS , Searcy JL , Bridges MT , Brewer LD , Popovic J , Blalock EM , et al. Reversal of glial and neurovascular markers of unhealthy brain aging by exercise in middle-aged female mice. PLoS One. (2011) ;6: (10):e26812. |
[129] | Leblond F , Nguyen A , Bolduc V , Lambert J , Yu C , Duquette N , et al. Postnatal exposure to voluntary exercise but not the antioxidant catechin protects the vasculature after a switch to an atherogenic environment in middle-age mice. Pflugers Arch. (2013) ;465: (2):197–208. |
[130] | Singh-Manoux A , Hillsdon M , Brunner E , Marmot M . Effects of physical activity on cognitive functioning in middle age: Evidence from the Whitehall II prospective cohort study. Am J Public Health. (2005) ;95: (12):2252–8. |
[131] | Murrell CJ , Cotter JD , Thomas KN , Lucas SJ , Williams MJ , Ainslie PN . Cerebral blood flow and cerebrovascular reactivity at rest and during sub-maximal exercise: Effect of age and 12-week exercise training. Age (Dordr). (2012) . |
[132] | Vicente-Campos D , Mora J , Castro-Pinero J , Gonzalez-Montesinos JL , Conde-Caveda J , Chicharro JL . Impact of a physical activity program on cerebral vasoreactivity in sedentary elderly people. J Sports Med Phys Fitness. (2012) ;52: (5):537–44. |
[133] | Ivey FM , Ryan AS , Hafer-Macko CE , Macko RF . Improved Cerebral Vasomotor Reactivity After Exercise Training in Hemiparetic Stroke Survivors. Stroke; A Journal of Cerebral Circulation. (2011) . |
[134] | Febbraio MA , Hiscock N , Sacchetti M , Fischer CP , Pedersen BK . Interleukin-6 is a novel factor mediating glucose homeostasis during skeletal muscle contraction. Diabetes. (2004) ;53: (7):1643–8. |
[135] | Whitham M , Febbraio MA . The ever-expanding myokinome: Discovery challenges and therapeutic implications. Nat Rev Drug Discov. (2016) ;15: (10):719–29. |
[136] | Fiuza-Luces C , Garatachea N , Berger NA , Lucia A . Exercise is the real polypill. Physiology (Bethesda). (2013) ;28: (5):330–58. |
[137] | Moon HY , Becke A , Berron D , Becker B , Sah N , Benoni G , et al. Running-Induced Systemic Cathepsin B Secretion Is Associated with Memory Function. Cell Metab. (2016) ;24: (2):332–40. |
[138] | Wrann CD , White JP , Salogiannnis J , Laznik-Bogoslavski D , Wu J , Ma D , et al. Exercise induces hippocampal BDNF through a PGC-1alpha/FNDC5 pathway. Cell Metab. (2013) ;18: (5):649–59. |
[139] | E L , Lu J , Selfridge JE , Burns JM , Swerdlow RH . Lactate administration reproduces specific brain and liver exercise-related changes. J Neurochem. (2013) ;127: (1):91–100. |
[140] | Morland C , Andersson KA , Haugen OP , Hadzic A , Kleppa L , Gille A , et al. Exercise induces cerebral VEGF and angiogenesis via the lactate receptor HCAR1. Nat Commun. (2017) ;8: :15557. |
[141] | Schiffer T , Schulte S , Sperlich B , Achtzehn S , Fricke H , Struder HK . Lactate infusion at rest increases BDNF blood concentration in humans. Neurosci Lett. (2011) ;488: (3):234–7. |
[142] | Skriver K , Roig M , Lundbye-Jensen J , Pingel J , Helge JW , Kiens B , et al. Acute exercise improves motor memory: Exploring potential biomarkers. Neurobiol Learn Mem. (2014) ;116: :46–58. |
[143] | Hashimoto T , Tsukamoto H , Takenaka S , Olesen ND , Petersen LG , Sorensen H , et al. Maintained exercise-enhanced brain executive function related to cerebral lactate metabolism in men. FASEB J. (2018) ;32: (3):1417–27. |