Synthesis, Characterization, and Biological Activity of Anthraquinone-Substituted Imidazolium Salts for the Treatment of Bladder Cancer
Abstract
BACKGROUND:
Bladder cancer is one of the most common types of cancer diagnosed each year, and more than half of patients have non-muscle invasive bladder cancer (NMIBC). The standard of care for patients with high-grade NMIBC is Bacillus Calmette-Guerin (BCG). Unfortunately, multiple BCG shortages have limited access to this treatment. Available alternatives using intravesical administration of chemotherapy have some efficacy, but lack prospective validation and long-term outcomes. Development of novel intravesical therapies may provide more active alternatives to BCG for patients with high-grade NMIBC.
OBJECTIVE:
To develop an optimal imidazolium salt for the intravesical treatment of NMIBC and determine preliminary in vitro activity of anthraquinone-substituted imidazolium salts.
METHODS:
The development of the anthraquinone-substituted imidazolium salts was undertaken in an attempt to increase the potency of this class of compounds by incorporating the quinone functional group observed in the chemotherapeutics doxorubicin, valrubicin, and mitomycin. All compounds were characterized by 1H and 13C NMR spectroscopy and infrared spectroscopy. Furthermore, these imidazolium salts were tested for in vitro cytotoxicity by the Developmental Therapeutics Program (DTP) on the NCI-60 human tumor cell line screening. Additional in vitro testing was performed against diverse bladder cancer cell lines (RT112, TCCSUP, J82, and UMUC13) using CellTiter-Glo® assays and colony-forming assays.
RESULTS:
The NCI-60 cell line screening indicated that compound 7 had the highest activity and was concluded to be the optimal compound for further study. Using CellTiter-Glo® assays on bladder cancer cell lines, 50% growth inhibitory concentration (IC50) values were determined to range from 32–50μM after an exposure of 1 h, for compound 7. Further evaluation of the compound by colony-forming assays showed the complete inhibition of growth at 10 days post a 100μM dose of compound 7 for 1 h.
CONCLUSIONS:
The most active lipophilic anthraquinone imidazolium salt, compound 7, could be a viable treatment for non-muscle invasive bladder cancer as it exhibits a cell-killing effect at a 1 h time period and completely inhibits cancer regrowth in colony-forming assays.
INTRODUCTION
Bladder cancer is estimated to affect over 80,000 new patients nationally in 2020, with more than 17,000 deaths anticipated [1]. Additionally, bladder cancer is the 4th and 8th most prevalent cancer in men and women, respectively, with the most common stage at the time of diagnosis being non-muscle invasive bladder cancer (NMIBC) [2]. Many therapies are used for NMIBC, but few are effective at elimination of the disease in some patients. The current standard of care for NMIBC is intravesical treatment with Bacillus Calmette-Guerin (BCG). Although BCG is an effective therapy, it is currently underproduced, which puts many patients at risk for cancer recurrence, progression, and subsequent radical cystectomy [3, 4]. Immunotherapy isn’t the only option as intravesical chemotherapy can be used as a treatment for NMIBC. A problem with intravesical chemotherapy is that the treatment has been shown to lead to 62% recurrence in intermediate grade patients within 5 years, according to a study performed by the European Association of Urology [5, 6]. This may be circumvented with a treatment regimen of gemcitabine/docetaxel that has shown a reduction of recurrence, but lacks the evaluation of long term outcomes and prospective validation [7]. Furthermore, there are more difficult cases to treat with high-grade NMIBC such as carcinoma in situ (CIS), which have shown cancer progression when treated with intravesical chemotherapy [8]. We presume that one of the reasons for the reduced efficacy of intravesical chemotherapy is that the activity of the chemotherapeutic used is dependent on prolonged exposure in order to be incorporated into the cell in S-phase (during DNA replication) or mitosis, when most chemotherapies exert their toxicity. Prolonged exposure requires longer intravesical retention of the chemotherapy to achieve a therapeutic effect, which is not practical for most patients.
Due to the numerous BCG shortages and the reduced efficacy of intravesical chemotherapy compared to BCG, novel chemotherapeutics are needed to treat bladder cancer. Development of novel anticancer materials is a necessity to combat bladder cancer and improve patient outcomes. We recently developed an imidazolium salt (TPP1) that selectively targets and is cytotoxic toward bladder cancer in vivo [9]. This compound has somewhat low potency, with 500–1000μM concentrations needed to treat select bladder cancer models, although this is still easily achievable in an intravesical setting (for reference, gemcitabine is often given at a concentration of 2.00 g in 50.0 mL, or 152 mM). To increase the potency of imidazolium salts for the treatment of bladder cancer, quinone moieties (which are key functional groups in the highly cytotoxic chemotherapeutics doxorubicin, valrubicin, and mitomycin C (Fig. 1)) can be incorporated. Therefore, we present the synthesis and characterization of novel imidazolium salts containing anthraquinone moieties and their in vitro activity against bladder cancer.
Fig. 1
Examples of current chemotherapeutics that contain anthraquinone (3-ring system) and quinone (the basic functional group of a single conjugated cyclic dione) moieties are illustrated above and are highlighted with dashed bond lines. The structures of doxorubicin, valrubicin, and mitomycin are shown.
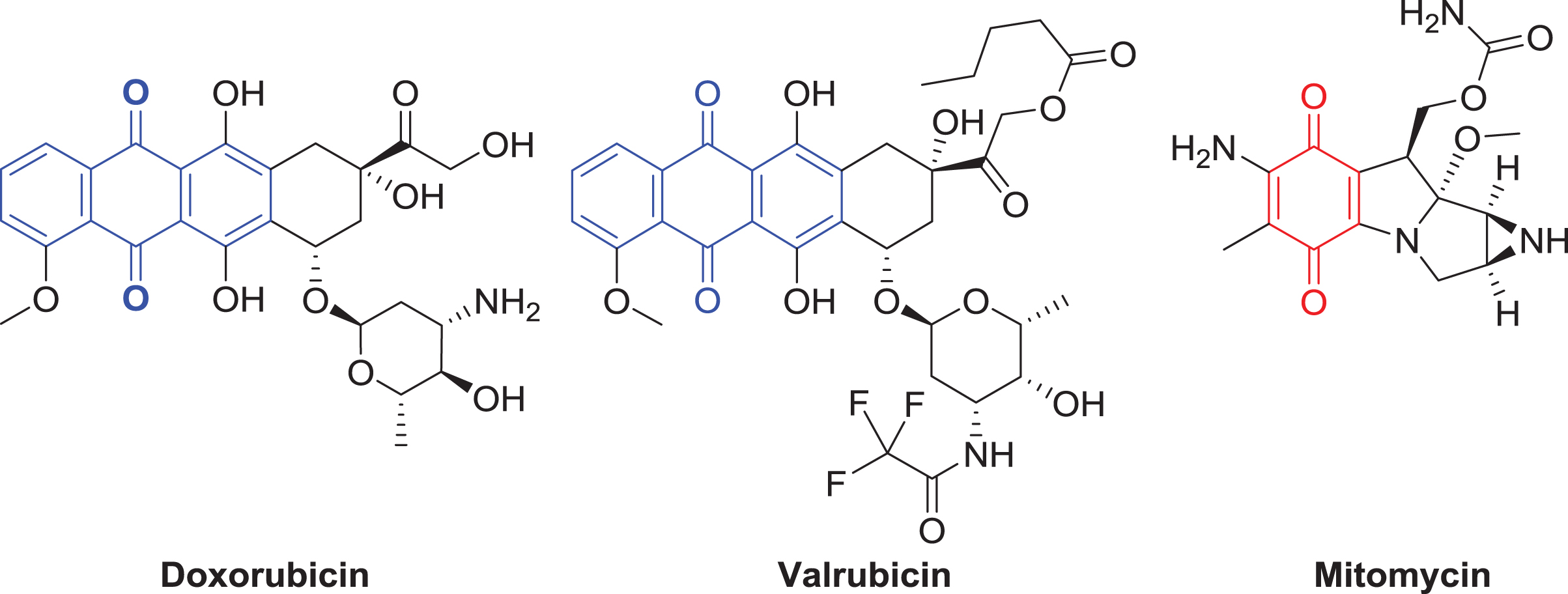
MATERIALS AND METHODS
General considerations
All reactions were performed aerobically unless otherwise stated. Solvents and chemical reagents were unmodified and purchased from VWR, Fisher Scientific, or Sigma Aldrich. The synthesis of starting material 1 was performed by a modified literature procedure [10, 11]. 1H and 13C NMR spectra were acquired on an Inova 400 MHz instrument (13C NMR 100 MHz). All NMR samples were prepared using DMSO-d6 and spectra were referenced to DMSO-d6 (1H: 2.500 ppm, 13C: 39.520 ppm). Infrared spectroscopy was performed on imidazolium salts 3, 4, 6, and 7 using a Thermo Scientific iS5 FT-IR w/ iD7 attachment.
X-ray crystallographic analysis
Crystals of the compounds were coated in Paratone oil, mounted on a CryoLoop and placed on a goniometer under a stream of nitrogen. Crystal structure data sets were collected on a Bruker Kappa APEX II Duo CCD system equipped with a Mo IμS source and a Cu IμS micro-focus source equipped with QUAZAR optics (λ= 1.54178 Å). The unit cells were determined by using reflections from three different orientations. Data sets were collected using APEX II software packages. All data sets were processed using the APEX II software suite [12, 13]. The data sets were integrated using SAINT [14]. An empirical absorption correction and other corrections were applied to the data sets using multi-scan SADABS [15]. Structure solution, refinement, and modelling were accomplished by using the Bruker SHELXTL package [16]. The structures were determined by full-matrix least-squares refinement of F2 and the selection of the appropriate atoms from the generated difference map. Hydrogen atom positions were calculated and Uiso(H) values were fixed according to a riding model.
DTP NCI-60 cell line screening
The developmental therapeutics program (DTP) treatment protocol is summarized as follows: 60 cell lines ranging from leukemia to breast cancer are treated with a 10μM concentration of the compound. The results are presented as growth percent. Continued growth is quantified from 0 to 100 and lethality is scored from –100 to 0. Further information on the experimental procedure for cell treatment can be viewed at NCI-DTP website (https://dtp.cancer.gov/discovery_development/nci-60/methodology.htm).
CellTiter-Glo® assay
Bladder cancer cell lines (RT112, TCCSUP, J82, and UMUC13) were seeded at 10,000 cells/well in black-walled 96 well plates and incubated for 24 h. Cells were treated with compound 7 in their respective media (as recommended by ATCC) at concentrations of 500 to 7.8μM dissolved in two-fold dilution increments of vehicle for 1 h, followed by replacement of treatment media with normal growth media. After 24 h, plates with cells were incubated at room temperature for 30 min, followed by the addition of 20μL of CellTiter-Glo® luminescent cell viability assay reagent (Promega) and mixing. After 2 min of incubation at room temperature, luminescence for each plate was measured using IVIS. Relative survival for each treatment group was calculated using vehicle control. All treatments were performed in quadruplicate.
Colony-forming assay
Cells were seeded at 500 cells/well in 6-well plates and 24 h later treated with 25μM, 50μM or 100μM of compound 7 or vehicle for 1 h. Cells were then allowed to grow in normal media for 10 days followed by staining with crystal violet (0.5% crystal violet in 20% MeOH/water) and incubation at 4°C for 5 min. The wells were then washed several times with PBS and dried prior to imaging. All the experiments were performed in triplicate.
Synthesis of 2-(bromomethyl)-9,10-anthraquinone (1)
2-(Bromomethyl)-9,10-anthraquinone (compound 1) was synthesized by a modified literature procedure [10, 11]. The radical initiator used was azobisisobutyronitrile (AIBN) instead of benzoyl peroxide (BPO). To obtain adequate purification for further reactions the product was collected from the hot reaction mixture by vacuum filtration and subsequently recrystallized with ethanol.
Synthesis of 2-((imidazol-1-yl)methyl)-9,10-anthraquinone (2)
Imidazole (0.308 g, 4.52 mmol) was stirred at reflux in acetonitrile (10 mL) with potassium hydroxide (0.281 g, 5.01 mmol). After 15 minutes compound 1 (1.361 g, 4.52 mmol) was added and the reaction mixture was allowed to reflux for 24 h. The reaction was filtered hot to remove KBr and the filtrate volatiles were removed under reduced pressure. The resulting crude solid was stirred in hot water. The product was collected via vacuum filtration, washed with ethyl ether, and dried under vacuum. The solid was dissolved in dichloromethane and impurities were precipitated with ethyl ether. Upon filtration, the filtrate volatiles were removed affording compound 2 as a yellow solid (0.963 g, 73.9%). 1H-NMR (400 MHz DMSO-d6): δ 8.18 (m, 2H, Ar), 8.08 (d, 1H, Ar), 8.00 (s, 1H, Ar), 7.91 (dd, 2H, Ar), 7.83 (s, 1H, Ar), 7.72 (m, 1H, Ar), 7.26 (s, 1H, Ar), 6.96 (s, 1H, Ar), 5.45 (s, 2H, CH2).
Synthesis of 1,3-bis((9,10-anthraquinone-2-yl)methyl)imidazolium bromide (3)
Compound 2 (0.499 g, 1.73 mmol) was refluxed in acetonitrile (8 mL) with compound 1 (0.535 g, 1.78 mmol) for 15 h. A yellow precipitate formed and was collected via vacuum filtration. The crude product was stirred in refluxing acetonitrile, collected via vacuum filtration and washed with THF and ethyl ether to produce compound 3 (0.609 g, 59.7%). 1H-NMR (400 MHz DMSO-d6): δ 9.61 (s, 1H, NCHN), 8.26 (d, 2H, Ar), 8.21 (m, 4H, Ar), 8.15 (m, 2H, Ar), 7.99 (s, 3H), 7.97 (m, 5H, Ar), 5.73 (s, 4H, CH2). 13C NMR (100 MHz DMSO-d6): δ 181.9, 141.1, 137.2, 134.6, 134.5, 134.1, 133.3, 132.9, 132.8, 127.6, 126.7, 126.5, 123.3, 51.5. ATR-IR: 3068w (CH, sp2), 3006w (CH, sp2), 1674s (C = O), 1590m (C = C) cm–1.
Crystal data for 3: C33H21BrN2O4, M = 589.43, monoclinic, a = 11.5769(8) Å, b = 11.9943(8) Å, c = 36.529(2) Å, β= 98.832(4)°, V = 5012.2(6) Å3, T = 100(2) K, space group C2/c, Z = 8, 21708 reflections measured, 5086 independent reflections (Rint = 0.0894). The final R1 values were 0.0594 (I > 2σ(I)). The final wR(F2) values were 0.1074 (I > 2σ(I)). The final R1 values were 0.1276 (all data). The final wR(F2) values were 0.1331 (all data).
Synthesis of 1-((9,10-anthraquinone-2-yl)methyl)-3-(naphthalen-2-ylmethyl)imidazolium bromide (4)
Compound 2 (0.283 g, 0.981 mmol) was refluxed in acetonitrile (2 mL) with 2-(bromomethyl)naphtha-lene (0.225 g, 1.02 mmol) for 15 h. A yellow precipitate formed and was collected via vacuum filtration (0.164 g, 32.8%). 1H NMR (400 MHz DMSO-d6) δ= 9.56 (1H, s, NCHN) 8.25 (4H, m, Ar) 7.98 (9H, m, Ar) 7.56 (3H, m, Ar) 5.71 (2H, s, CH2) 5.65 (2H, s, CH2). 13C NMR (100 MHz DMSO-d6): δ 182.0, 181.9, 141.2, 136.9, 134.63, 134.58, 134.1, 133.3, 132.9, 132.84, 132.82, 132.7, 132.6, 132.0, 128.8, 127.8, 127.7, 127.6, 127.5, 126.7, 126.6, 126.5, 125.7, 123.2, 123.0, 52.3, 51.4. ATR-IR: 3140w (CH, sp2), 3009w (CH, sp2), 2968w (CH, sp3), 1672s (C = O), 1592 m (C = C) cm–1.
Synthesis of 2-((benzimidazol-1-yl)methyl)-9,10-anthraquinone (5)
Benzimidazole (0.506 g, 4.52 mmol) was stirred at reflux in acetonitrile (10 mL) with potassium hydroxide (0.273 g, 5.01 mmol). After 30 minutes, 1 (1.271 g, 4.52 mmol) was added and the reaction mixture was allowed to reflux for 24 h. The reaction mixture was filtered hot to remove KBr and the filtrate volatiles were removed under reduced pressure. The resulting crude solid was stirred in hot water. Compound 5 was collected via vacuum filtration and washed with ethyl ether (0.520 g, 73.9%). 1H-NMR (400 MHz; DMSO-d6): δ 8.50 (s, 1H, Ar), 8.18 (m, 3H, Ar), 8.07 (s, 1H, Ar), 7.89 (m, 2H, Ar), 7.79 (d, 1H, Ar), 7.70 (m, 1H, Ar), 7.54 (m, 1H, Ar), 7.22 (m, 2H, Ar), 5.77 (s, 2H, CH2).
Scheme 1.
Syntheses of compounds 2–7 by alkylation of imidazole or benzimidazole with 1 or 2-bromomethylnaphthalene.
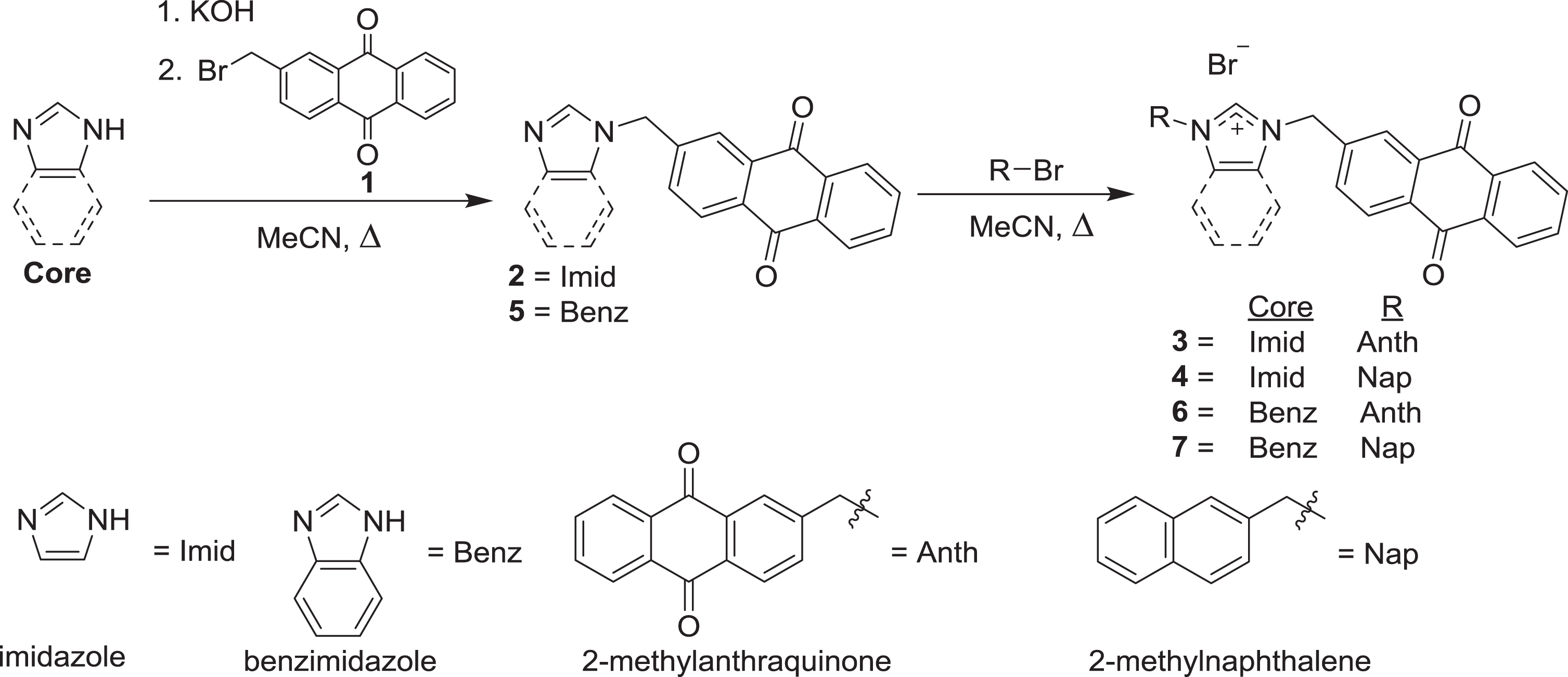
Synthesis of 1,3-bis((9,10-anthraquinone-2-yl)methyl)benzimidazolium bromide (6)
Compound 5 (0.502, 1.73 mmol) was refluxed in acetonitrile (5 mL) with compound 1 (0.456 g, 1.78 mmol) for 16 h. A tan precipitate formed and was collected via vacuum filtration. The crude product was dissolved in DMF and precipitated with ethyl ether to afford compound 6 (0.752 g, 68.1%) 1H-NMR (400 MHz DMSO-d6): δ 9.61 (s, 1H, NCHN), 8.26 (d, 2H, Ar), 8.21 (m, 4H, Ar), 8.15 (m, 2H, Ar), 7.99 (s, 3H), 7.97–7.92 (m, 5H), 5.73 (s, 4H). 13C NMR (100 MHz DMSO-d6): δ 182.0, 181.9, 143.7, 140.4, 134.6, 134.5, 134.0, 133.4, 132.9, 132.8, 131.1, 127.5, 127.1, 126.7, 126.5, 114.1, 49.6. ATR-IR: 3133w (CH, sp2), 3071w (CH, sp2), 3015w (CH, sp2), 2928w (CH, sp3), 1668s (C = O), 1603 m (C = C) cm–1.
Synthesis of 1-((9,10-anthraquinone-2-yl)methyl)-3-(naphthalen-2-ylmethyl)benzimidazolium bromide (7)
Compound 5 (0.630, 1.86 mmol) was refluxed in acetonitrile (5 mL) with 2-(bromomethyl)naphthalene (0.428 g, 1.94 mmol) for 16 h. A tan precipitate formed and was collected via vacuum filtration. The crude product was triturated with dichloromethane to afford an off-white solid, compound 7 (0.643 g, 61.7 %) 1H-NMR (400 MHz DMSO-d6): δ 10.24 (s, 1H, NCHN), 8.37 (s, 1H, Ar), 8.23 (m, 3H, Ar), 8.15 (s, 1H), 8.03 (dd, 4H, Ar), 7.94 (m, 4H, Ar), 7.66 (d, 3H, Ar), 7.57 (m, 2H, Ar), 6.10 (s, 2H, CH2), 6.02 (s, 2H, CH2). 13C NMR (100 MHz DMSO-d6): δ 182.5, 182.4, 143.8, 141.0, 135.06, 135.02, 134.4, 133.8, 133.3, 133.28, 133.24, 133.2, 133.1, 131.7, 131.6, 131.5, 129.2, 128.3, 128.2, 128.1, 127.9, 127.4, 127.3, 127.2, 127.1, 126.9, 126.2, 114.7, 114.4, 50.8, 50.0. ATR-IR: 3129w (CH, sp2), 3069w (CH, sp2), 3025w (CH, sp2), 2952w (CH, sp3), 1668s (C = O), 1591 m (C = C) cm–1.
Crystal data for compound 7: C33H23BrN2O2, M = 559.44, Triclinic, a = 7.9808(4) Å, b = 11.3358(5) Å, c = 15.2282(8) Å, α= 74.908(3)°, β= 86.015(3)°, γ= 70.555(3)° V = 5012.2(6) Å3, T = 100(2) K, space group P-1, Z = 2, 20211 reflections measured, 5073 independent reflections (Rint = 0.0640). The final R1 values were 0.0601 (I > 2σ(I)). The final wR(F2) values were 0.1359 (I > 2σ(I)). The final R1 values were 0.0854 (all data). The final wR(F2) values were 0.1538 (all data).
RESULTS AND DISCUSSION
Synthesis and characterization
The syntheses of the anthraquinone-substituted imidazolium salt compounds 3, 4, 6, and 7 are outlined in the general reactions of Scheme 1. Compounds 3 and 4 were first synthesized by deprotonation of imidazole with potassium hydroxide and then adding 2-(bromomethyl)anthracene-9,10-dione. The purified mono-substituted imidazole (compound 2) was subsequently combined with either 2-(bromomethyl)anthracene-9,10-dione (compound 1) or 2-(bromomethyl)naphthalene to form compounds 3 and 4 respectively. The compounds were first evaluated by 1H NMR spectroscopy and the characteristic C2 proton resonances were observed at 9.61 ppm for 3 and 9.57 ppm for 4. Additionally a single resonance at 5.73 ppm was observed for the methylene protons of the symmetric compound 3. In contrast the asymmetric imidazolium salt (compound 4) yielded the methylene resonances at 5.71 and 5.65 ppm. Further characterization by 13C NMR spectroscopy yielded the C2 carbon resonance at 141 ppm for both compounds. The inequivalent carbonyl resonances of the 9,10-anthraquinone moieties were also observed for both compounds at 181.9 and 182.0 ppm.
The anthraquinone benzimidazolium salts, compounds 6 and 7, were synthesized by a similar route to 3 and 4, with formation of the mono-substituted benzimidazole (5) first, then a subsequent alkylation. Structure elucidation of both compounds was determined by 1H NMR spectroscopy with the methylene resonances at 6.11 ppm for the symmetric compound 6 and 6.10 and 6.02 ppm for the asymmetric benzimidazolium salt, 7. Furthermore the C2 proton resonances were observed downfield at 10.26 and 10.24 ppm for 6 and 7 respectively. Similar to the 13C spectra of compounds 3 and 4, both 6 and 7 had C2 carbon resonances at 143 ppm and the inequivalent anthraquinone carbonyl resonances at 182.0 and 181.9 ppm.
To confirm the structures of the compounds discussed above, single crystal X-ray diffraction was used. Crystals suitable for analysis were obtained from vapor diffusion of ethyl ether into N,N-dimethylformamide solutions and only structures for compounds 4 and 7 were determined (Fig. 2).
Fig. 2
Thermal ellipsoid plots of 4 and 7 with hydrogen atoms not shown for clarity. Thermal ellipsoids were drawn to 50% probability.
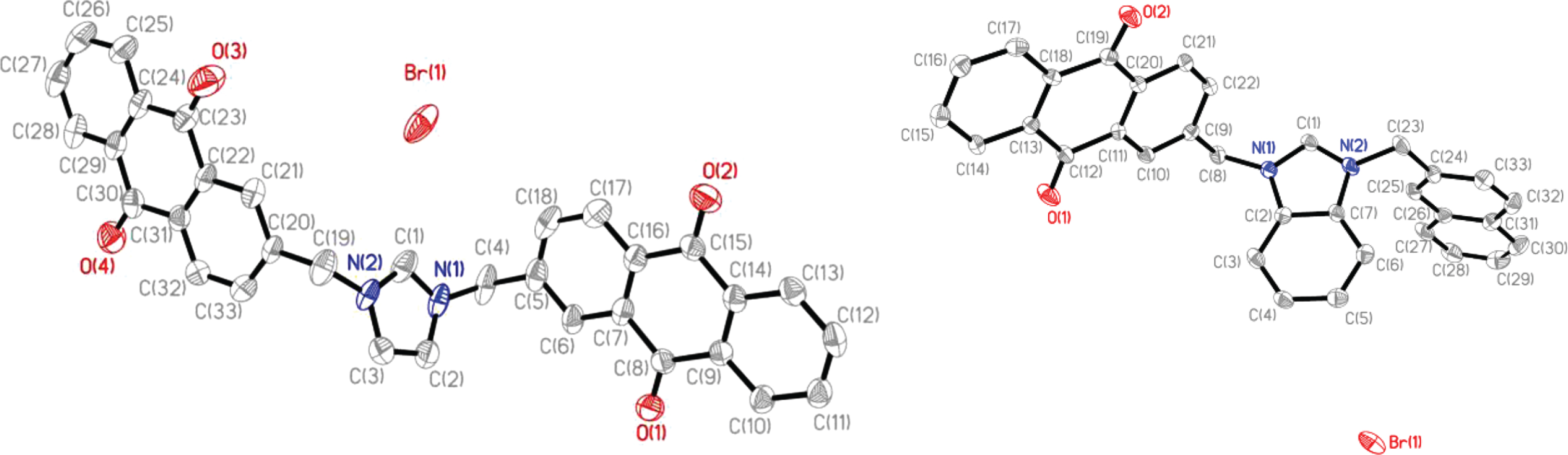
In vitro biological evaluation of anthraquinone compounds as anticancer agents
The in vitro biological activity of compounds 3, 4, 6, and 7 was first evaluated by the Developmental Therapeutics Program (DTP) using the NCI-60 cell line screening (Table 1). At 10μM the bisanthraquinone imidazolium salts, 3 and 6, had little inherent activity toward most of the 60 cell lines with a 48 h exposure.
Table 1
One Dose NCI-60 Cell Line Screening for Compounds 3,4,6, and 7 (GI%)
![]() |
The asymmetric compounds 4 and 7 had increased activity in comparison to compounds 3 and 6 against the NCI-60 cell lines, with compound 7 exhibiting the most cytotoxicity at an average percent growth inhibition (GI%) of 47.3% over all cell lines. This potency is believed to be directly caused by the addition of the naphthalene substituent and increased lipophilicity of the benzimidazole, as similar activity has been previously reported by the Youngs group with respect to lung cancer [17].
With preliminary data indicating the increased cytotoxicity of compound 7 against the NCI-60 cell line screening, further in vitro activity was evaluated against select bladder cancer cell lines. IC50 values of 32, 35, 50, and 48μM were determined for a 1 h exposure of compound 7 to bladder cancer cell lines RT112, TCCSUP, J82, and UMUC13 respectively (Fig. 3). The shortened exposure time is necessary as intravesical treatments cannot be retained in patients for prolonged periods of time.
Fig. 3
IC50 values determined by the CellTiter-Glo® assay for a 1 h exposure of select bladder cancer cell lines to compound 7.
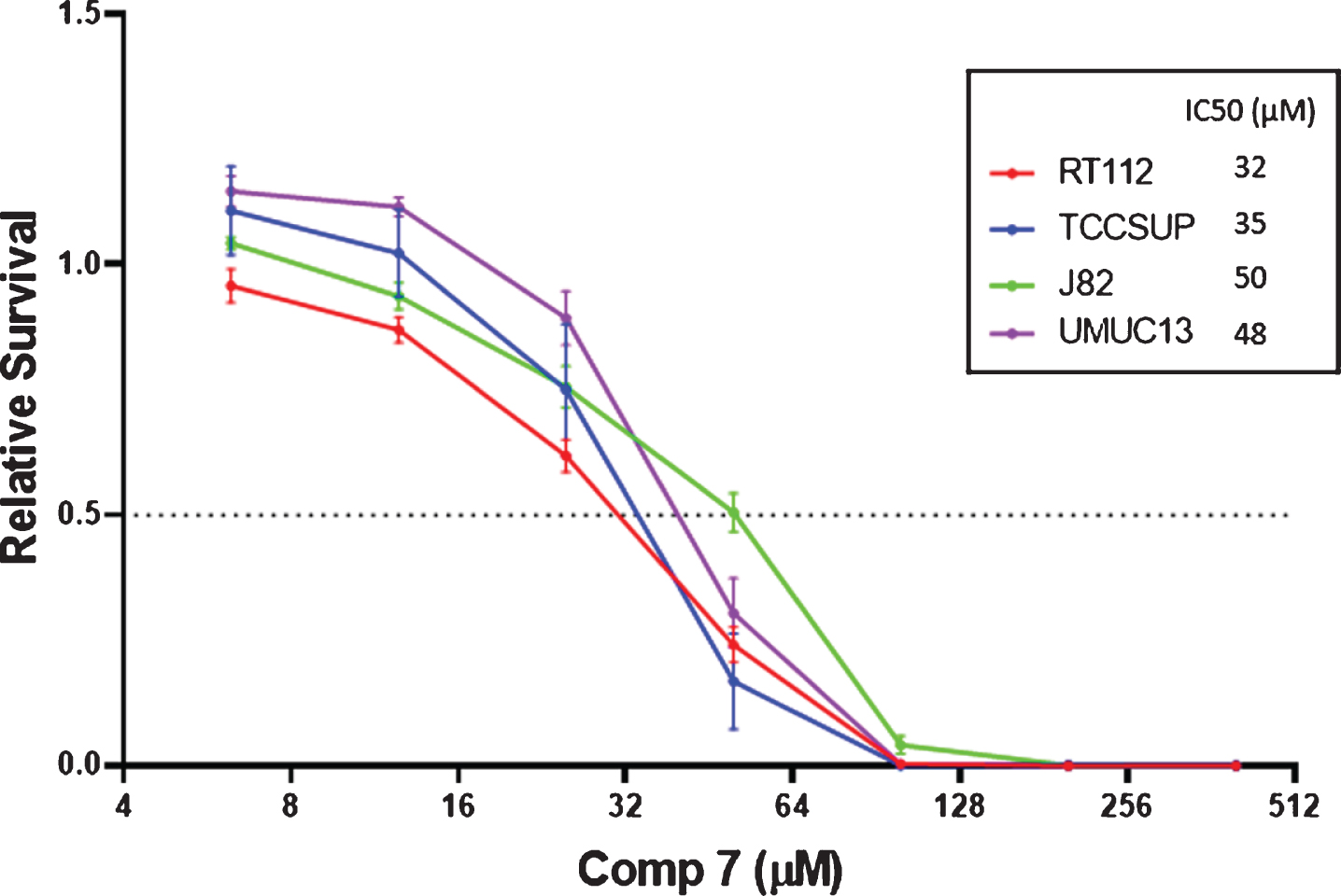
Fig. 4
Colony-forming assay of select bladder cancer cell lines 10 days after a 1 h treatment with compound 7.
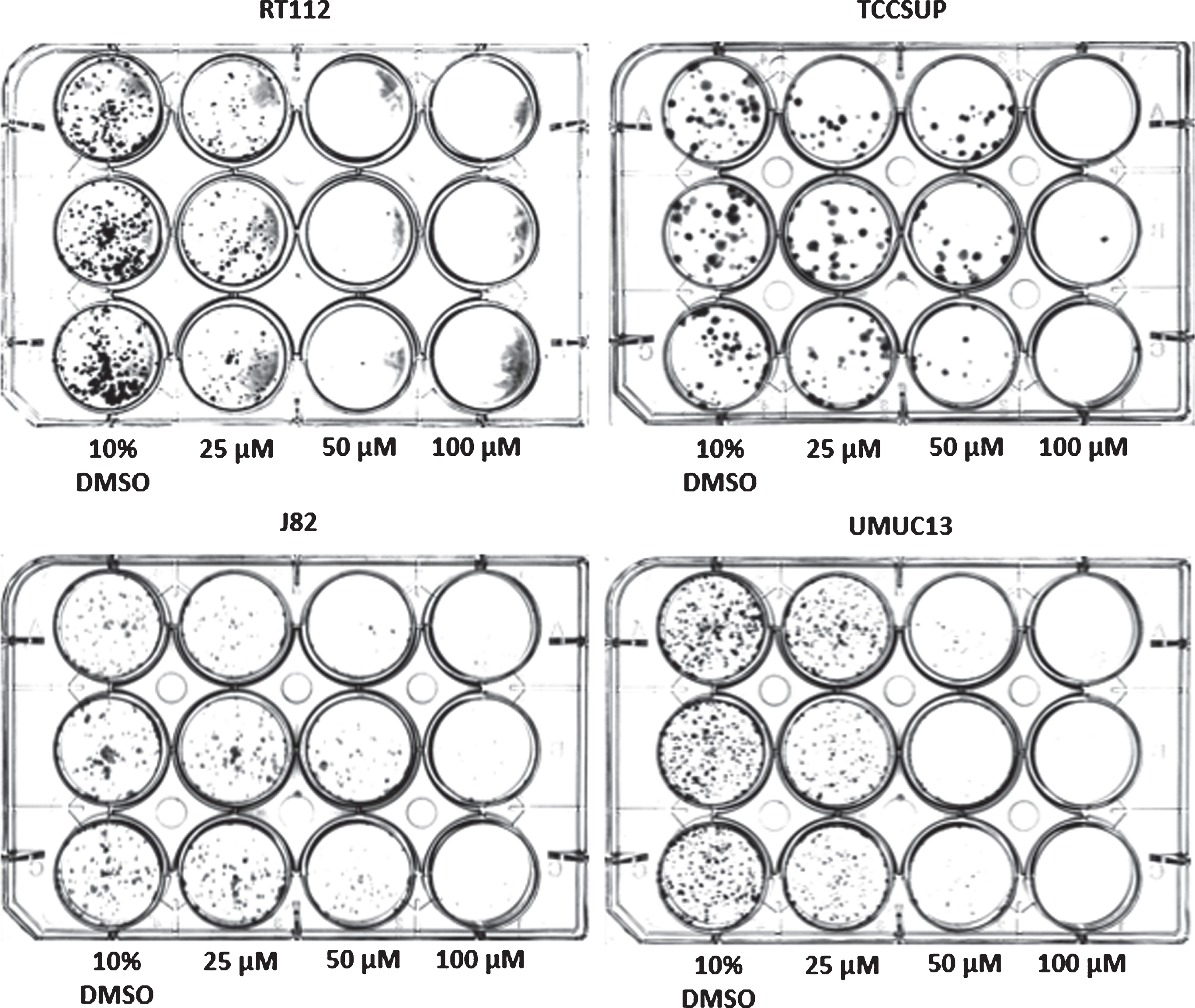
The long-term cytotoxicity of short-term exposure to compound 7 was evaluated next. Using the colony-forming assay, compound 7 was shown to inhibit cell growth against RT112 and UMUC13 at 50μM (1 h exposure) after a recovery period of 10 days. When increasing the concentration to 100μM, compound 7 inhibited cancer regrowth in all tested cell lines after 10 days recovery.
CONCLUSION
In this work, a series of anthraquinone-substituted imidazolium salts was synthesized. The bisanthraquinone imidazolium and benzimidazolium salts, when tested by DTP on the NCI-60 cell line screening, yielded little inherent activity against all cell lines at the 10μM test concentration. Even though these compounds are lipophilic, the correlation between the naphthalene substituent and activity is apparent, as compounds 4 and 7 both exhibited activity against the cell lines in the screening. Moreover it was determined that replacement of the imidazole (4) with benzimidazole (7) resulted in a significant increase in cytotoxicity, believed to be due to the increase in lipophilicity. With preliminary data from the NCI-60 showing compound 7 to be the most cytotoxic of the compounds, it was chosen for further study. Compound 7 exhibited significant in vitro activity against select bladder cancer cell lines after a 1 h exposure time. Further, compound 7 prevented bladder cancer regrowth in the colony-forming assay against all tested cell lines over a period of 10 days. With these results compound 7 exhibits a cell-killing effect in an acceptable time period against bladder cancer cell lines and may be a highly effective treatment for NMIBC.
SUPPLEMENTARY INFORMATION
One dose NCI-60 human tumor cell line screening data for compounds 3, 4, 6, and 7 as presented from DTP. NMR spectroscopy data for compounds 2–7. IR spectroscopy data for compounds 3, 4, 6, and 7
Crystallographic Information File for 4 (CCDC # 1988214) and 7 (CCDC # 1988213) can be found free of charge on the Cambridge Crystallographic Data Center website.
ACKNOWLEDGMENTS
The authors would like to thank NCI’s DTP for performing the 60 human tumor cell line screening with their one dose assay for compounds 3, 4, 5, and 7. We are also grateful for the funding provided by the University of Akron’s Chemistry Department for the purchase of chemical reagents.
FUNDING
This work was funded by the following: PHA-BCAN Young Investigator Award, US-Avery Postdoctoral Fellowship, and the Fox Chase Center Core Grant (P30 CA006927). Additional funding for instrumentation was provided by the National Science Foundation (NSF) for NMR spectrometers (Nos. CHE-0341701 and DMR-0414599) and the X-ray diffractometer (CHE-0840446) used in this work. Also supported by Fox Chase Cancer Center Core grant P30 CA006927.
AUTHOR CONTRIBUTIONS
Conceptualization, M.S. and W.Y.; development and structural characterization, M.S.; biological investigation, D.W., U.S., and P.A.; writing-original draft preparation, M.S.; writing-review and editing, M.S., D.W., U.S., P.A., and W.Y. All authors have read and agreed to the published version of the manuscript.
CONFLICTS OF INTEREST
The authors declare no conflict of interest.
REFERENCES
[1] | Cancer Facts & Figures 2020. Am Cancer Soc. 2020. |
[2] | Anastasiadis A , de Reijke TM . Best practice in the treatment of nonmuscle invasive bladder cancer. Ther Adv Urol. (2012) ;4: :13–32. |
[3] | du Preez K , Seddon JA , Schaaf HS , Hesseling AC , Starke JR , Osman M , Lombard CJ , Solomons R . Global shortages of BCG vaccine and tuberculous meningitis in children. Lancet Glob Heal. (2019) ;7: :e28–e29. |
[4] | Messing EM . The BCG Shortage. Bl. cancer (Amsterdam, Netherlands). (2017) ;3: :227–28. |
[5] | Porten SP , Leapman MS , Greene KL . Intravesical chemotherapy in non-muscle-invasive bladder cancer. Indian J Urol. (2015) ;31: :297–303. |
[6] | Kamat AM , Lamm DL . Current trends in intravesical therapy for bladder cancer. Contemp Urol. (2000) ;55: :161–8. |
[7] | Steinberg RL , Thomas LJ , O’Donnell MA , Nepple KG . Sequential Intravesical Gemcitabine and Docetaxel for the Salvage Treatment of Non-Muscle Invasive Bladder Cancer. Bl. cancer (Amsterdam, Netherlands). (2015) ;1: :65–72. |
[8] | van Rhijn BWG , Burger M , Lotan Y , Solsona E , Stief CG , Sylvester RJ , Witjes JA , Zlotta AR . Recurrence and Progression of Disease in Non-Muscle-Invasive Bladder Cancer: From Epidemiology to Treatment Strategy. Eur Urol. (2009) ;56: :430–42. |
[9] | Stromyer ML , Southerland MR , Satyal U , Sikder RK , Weader DJ , Baughman JA , Youngs WJ , Abbosh PH . Synthesis, characterization, and biological activity of a triphenylphosphonium-containing imidazolium salt against select bladder cancer cell lines. Eur J Med Chem. (2020) ;185: :111832. |
[10] | Zheng Y , Zhu L , Fan L , Zhao W , Wang J , Hao X , Zhu Y , Hu X , Yuan Y , Shao J , Wang W . Synthesis, SAR and pharmacological characterization of novel anthraquinone cation compounds as potential anticancer agents. Eur J Med Chem. (2017) ;125: :902–13. |
[11] | Ellis LT , Perkins DF , Turner P , Hambley TW . The preparation and characterisation of cyclam/anthraquinone macrocyle/intercalator complexes and their interactions with DNA. J Chem Soc Dalt Trans. (2003) ;3: :2728–36. |
[12] | Bruker SMART (Version 5.625). Madison, Wisconsin, USA: Buker AXS inc.; 2012. |
[13] | Bruker APEX II. Madison, Wisconsin, USA: Bruker ACS Inc.; 2012. |
[14] | Bruker SAINT. Madison, Wisconsin, USA. Bruker ACS Inc.; 2012. |
[15] | Bruker SADABS. Madison, Wisconsin, USA: Bruker AXS Inc.; 2012. |
[16] | Sheldrick GM . Crystal structure refinement with SHELXL. ACTA Crystallogr C. (2015) ;71: :3–8. |
[17] | Wright BD , Deblock MC , Wagers PO , Duah E , Robishaw NK , Shelton KL , Southerland MR , DeBord MA , Kersten KM , McDonald LJ , Stiel Ja , Panzner MJ , Tessier Ca , Paruchuri S , Youngs WJ . Anti-tumor activity of lipophilic imidazolium salts on select NSCLC cell lines. Med Chem Res. (2015) ;24: :2838–61. |