Structural properties of paeonol encapsulated liposomes at physiological temperature: Synchrotron small-angle and wide-angle X-ray diffraction studies
Abstract
Structural properties of paeonol-encapsulated liposomes containing cholesterol or stigmasterol at 37°C have been investigated by synchrotron small-angle X-ray scattering (SAXS) and wide-angle X-ray scattering (WAXS) techniques. We compared the structural properties of pure dipalmitoylphosphatidylcholine (DPPC) liposomes, sterol–DPPC liposomes, and those of paeonol–sterol–DPPC liposomes at different molar ratios. Three conclusions can be drawn: First, phase separation occurs in both sterol–DPPC and paeonol–sterol–DPPC liposomes. Second, the incorporation of paeonol molecules into sterol–DPPC liposomes weakens the membrane order. Third, cholesterol has a stronger tendency to interact with DPPC as compared to its counterpart in plant, stigmasterol.
1.Introduction
Liposomes are of considerable interest as carriers for controlled delivery of drugs because many substances can be encapsulated in the aqueous or lipid phases of liposomes [9,16,25]. Drugs encapsulated in liposomes are sufficiently protected from enzymatic attack and immune recognition [14]. Paeonol (see Fig. 1) is the major active ingredient of the root cortex Moutan peony (Chinese name: Mudan, which is the national flower of China) [24,31,32]. Numerous pharmacological studies showed that paeonol is a volatile compound with analgesic, antipyretic, sedative, anti-inflammatory, anti-oxidative, and antibacterial properties [7,13,31–33]. However, the bioavailability of paeonol in vivo is low because it is poorly soluble in water. Encapsulating paeonol into liposomes can increase its bioavailability in vivo.
Fig. 1.
Molecular structures of cholesterol, stigmasterol, paeonol, and 1,2-dipalmitoyl-sn-glycero-3-phosphocholine.
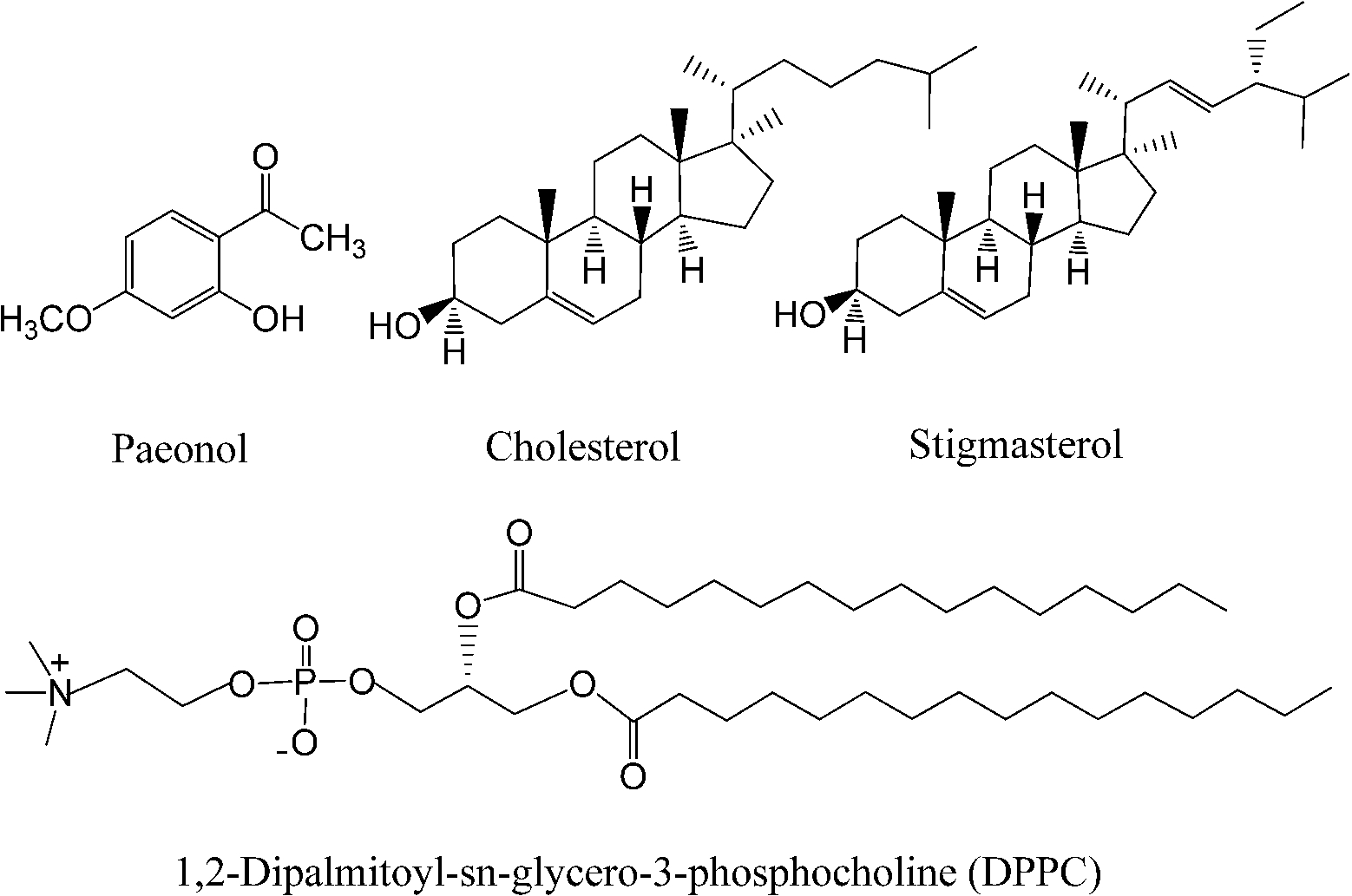
Phospholipids and sterols are typical amphiphilic molecules for the preparation of liposomes. As a major component of lecithins (L-α-phosphatidylcholines), 1,2-dipalmitoyl-sn-glycero-3-phosphocholine (DPPC, as shown in Fig. 1) has often been chosen to prepare liposomes both because lecithins are the major components in mammalian membranes and also because they show a sharp, strong, thermotropic transition near the physiological temperature [5,11,26,27,31,32]. Cholesterol (as shown in Fig. 1), which was first named 200 years ago [6], has often been chosen to prepare liposomes together with phospholipids because it can provide a number of formulation benefits, including good retention of encapsulated drug, reduced plasma protein binding and resilience to macrophage attack [4,31].
However, high levels of blood cholesterol will increase the risk of many diseases, such as coronary heart disease, stroke, especially the risk of atherosclerosis [3]. Thus, plant sterols such as stigmasterol (as shown in Fig. 1) have also been used to prepare liposomes by many research groups [12,17,34]. In our earlier study, the thermotropic phase behavior of stigmasterol–DPPC liposomes was investigated and the binary phase diagram of this binary system was constructed with the help of differential scanning calorimetry (DSC), synchrotron X-ray diffraction (XRD) and freeze-fracture electron microscopy (FFEM) techniques [30]. The results show that the thermotropic phase behavior and the binary phase diagram of stigmasterol–DPPC liposomes are very similar to those of cholesterol–DPPC liposomes. Muramatsu et al. reported that the bioavailability of insulin loaded liposomes containing stigmasterol is a little higher than that containing cholesterol [18].
The structural properties of liposomes such as the information on long range bilayer organisation and on hydrocarbon chain packing can help to understand the mechanism of molecular interactions in liposomes. There are few reports about structural properties of paenol-sterol–DPPC liposomes as yet. In this work, we employed synchrotron small-angle X-ray scattering (SAXS) and wide-angle X-ray scattering (WAXS) techniques to investigate the structural characters of paeonol–cholesterol–DPPC liposomes and that of paeonol–stigmasterol–DPPC liposomes at physiological temperature of 37°C.
2.Materials and methods
2.1.Sample preparation
1,2-Dipalmitoyl-sn-glycero-3-phosphocholine (DPPC) and, 2-hydroxyl-4-methoxyacetophone (paeonol) were purchased from Sigma Chemicals (99%, St. Louis, MO, USA). Cholesterol and stigmasterol were from MP Biomedicals Inc. (95%, Aurora, OH, Germany). They were used without further purification. All organic solvents were of analytical grade. Double deionized water with a resistivity of 18.2 MΩ · cm was used for the preparation of buffers. Liposomes were prepared following Lichtenberger et al. [15]: paeonol–sterol–DPPC mixtures with designated molar ratios were dissolved in chloroform, dried under nitrogen, and then stored in vacuum overnight. The obtained lipid films were hydrated with excess Tris-HCl buffers (50 mM Tris-HCl, 150 mM NaCl, 0.1 mM CaCl2, pH = 7.2) with repeated vortexing and heating-cooling treatment between 65°C and 20°C for at least three times, and then were stored at −20°C at least for 24 hrs before experiments. The molar ratio of sterol to DPPC was 1:24 and that of paeonol to sterol was 0:1, 0.2:1, 0.4:1, 0.8:1, or 1:1. All samples have been cooled from 65°C to 20°C at a cooling rate of 0.5°C min−1 before measurement to achieve phase equilibrium. It should be noted that the liposomes we prepared in this work are actually multilamellar vesicles, which are most suitable to XRD experiments.
2.2.Synchrotron X-ray diffraction
Synchrotron small-angle X-ray scattering (SAXS) and wide-angle X-ray scattering (WAXS) experiments were performed at the beamline 1W2A of the Beijing Synchrotron Radiation Facility (BSRF) (
Deconvolution of the multicomponent SAXS and WAXS patterns was carried out using PeakFit software (Aisn Software Inc). The baseline was created by the two-point linear method and peak type was Lorentz for all the deconvolution treatments.
3.Results and discussion
In excess water the zwitterionic molecules of DPPC spontaneously form bilayers/multilayers with temperature-dependent structure and long-range organization [21,35]. As temperature is increased from room temperature, DPPC is known to display a low enthalpic pretransition (from the lamellar gel phase
3.1.Structural properties of pure DPPC liposomes
The SAXS and WAXS patterns of paeonol–sterol–DPPC liposomes at 37°C are shown in Fig. 2 and Fig. 3, respectively, and the values of d-spacing (d) and correlation length (ξ) are summarized in Table 1. As shown in the table, in the absence of sterols and drugs, the d-spacing of SAXS and WAXS of pure DPPC liposomes at 37°C are 7.02 nm and 0.423 nm, respectively. These data are in good agreement with other studies and represent a typical lamellar-gel phase [20,22,35].
Fig. 2.
The SAXS patterns of paeonol–cholesterol–DPPC (solid lines) and paeonol–stigmasterol–DPPC (dotted lines) liposomes at different molar ratios of paeonol:sterol:DPPC collected at 37°C.
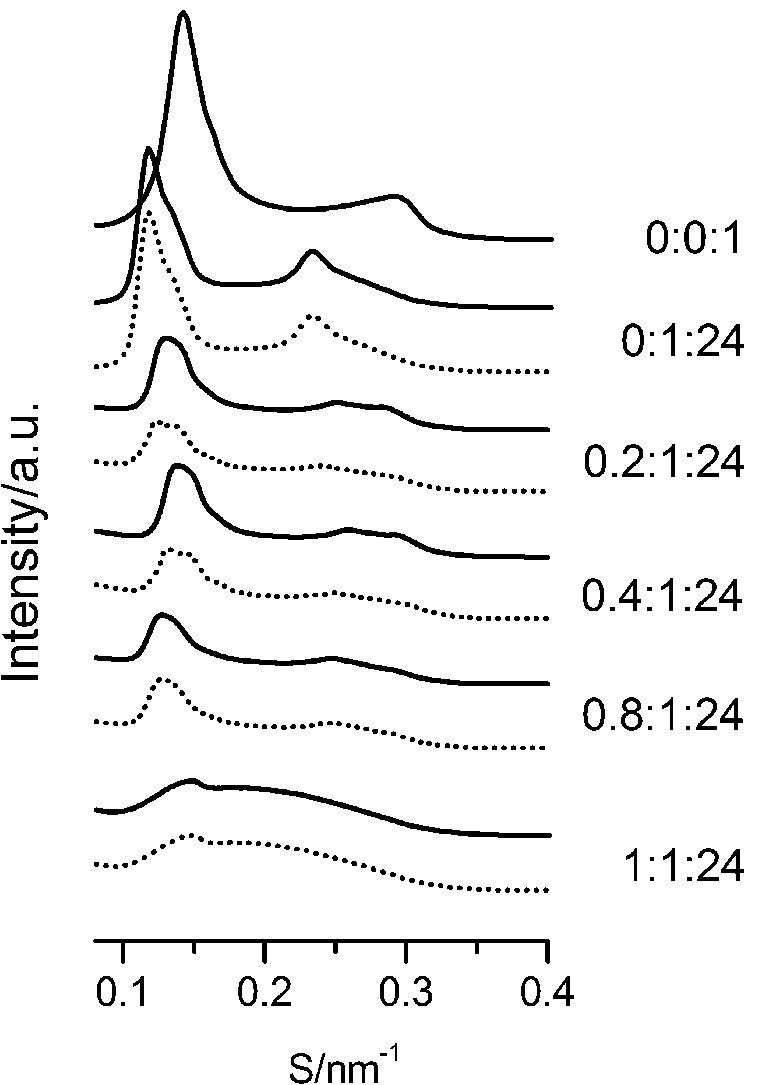
Fig. 3.
The WAXS patterns of paeonol–cholesterol–DPPC (solid lines) and paeonol–stigmasterol–DPPC (dotted lines) at different molar ratios of paeonol:sterol:DPPC collected at 37°C.
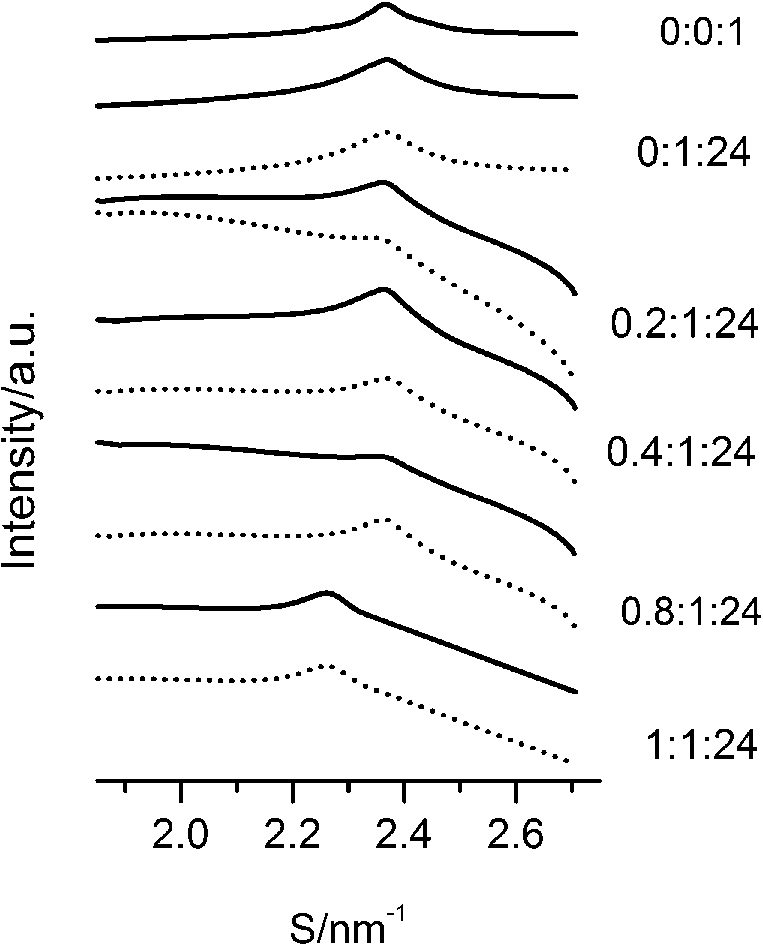
Table 1
The d-spacings (d) and the correlation length (ξ) of paeonol–sterol–DPPC liposomes at different molar ratios at 37°C
Molar ratio of paeonol:sterol:DPPC | Sterol | Domain | d-spacing of SAXS (nm) | ξ of SAXS (nm) | d-spacing of WAXS (nm) |
0:0:1 | 7.02 | 42.6 | 0.423 | ||
0:1:24 | cholesterol | sterol-rich | 7.68 | 40.5 | 0.458 |
DPPC-rich | 8.56 | 42.3 | 0.422 | ||
stigmasterol | sterol-rich | 7.76 | 39.2 | 0.459 | |
DPPC-rich | 8.57 | 43.0 | 0.424 | ||
0.2:1:24 | cholesterol | sterol-rich | 7.01 | 35.7 | 0.423 |
paeonol-rich | 7.95 | 34.9 | 0.442 | ||
stigmasterol | sterol-rich | 6.94 | 38.8 | 0.425 | |
paeonol-rich | 8.27 | 37.7 | 0.444 | ||
0.4:1:24 | cholesterol | sterol-rich | 6.82 | 34.4 | 0.420 |
paeonol-rich | 7.69 | 32.2 | 0.443 | ||
stigmasterol | sterol-rich | 6.77 | 33.2 | 0.423 | |
paeonol-rich | 7.97 | 29.8 | 0.446 | ||
0.8:1:24 | cholesterol | sterol-rich | 7.00 | 38.6 | 0.411 |
paeonol-rich | 7.99 | 36.2 | 0.432 | ||
stigmasterol | sterol-rich | 7.04 | 38.8 | 0.413 | |
paeonol-rich | 8.04 | 33.4 | 0.434 | ||
1:1:24 | cholesterol | sterol-rich | 7.03 | 22.8 | 0.408 |
paeonol-rich | 4.85 | 9.40 | 0.445 | ||
stigmasterol | sterol-rich | 7.13 | 20.0 | 0.409 | |
paeonol-rich | 4.90 | 9.10 | 0.448 |
3.2.Structural properties of sterol–DPPC liposomes
Many research groups have reported that phase separation occurs in both cholesterol–DPPC and stigmasterol–DPPC liposomes [10,29,30]. As shown in Fig. 2, the SAXS peaks of cholesterol–DPPC and stigmasterol–DPPC liposomes seem to consist of two overlapping peaks. This implies that there are two domains coexisting in these liposomes at 37°C, indicating phase separation. Most likely, one phase reflects a sterol-rich domain and the other a DPPC-rich domain.
3.3.Structural properties of paeonol–sterol–DPPC liposomes
After careful analysis of the data in Table 1 and SAXS patterns of Fig. 2, we drew the following three conclusions: First, phase separation occurs in both paeonol–cholesterol–DPPC and paeonol–stigmasterol–DPPC liposomes. As shown in Fig. 2, the SAXS peaks of all the paeonol–sterol–DPPC liposomes consist of two overlapping peaks. This implies that there are two domains coexisting in these liposomes at 37°C, signaling phase separation. Most likely, one phase reflects a sterol-rich domain and the other phase a paeonol-rich domain. As the molecular structure of paeonol greatly differs from that of sterol, the phase structures of the two domains may differ markedly. Thus phase separation occurs. We deconvoluted the XRD peaks of paeonol–sterol–DPPC liposomes using Peakfit software (Aisn Software Inc), as shown in Fig. 4 for SAXS (1:1:24, paeonol:sterol:DPPC) and in Fig. 5 for WAXS (1:1:24, paeonol:sterol:DPPC), respectively. The values of d and ξ of deconvoluted peaks are summarized in Table 1. As can be seen in the table, the domain with a smaller d-spacing of WAXS and a longer correlation length (ξ) is attributed to sterol-rich phase, while the domain with a larger d-spacing of WAXS and a shorter correlation length is attributed to paeonol-rich phase. In our earlier study of competitive molecular interaction among paeonol-loaded liposomes, we concluded that sterols have much more favorable, stabilizing interactions with DPPC compared to paeonol [31]. Therefore the sterol-rich domain should show a longer correlation length (ξ) and a smaller d-spacing of WAXS compared with paeonol-rich domain.
Fig. 4.
The SAXS patterns (solid lines) of paeonol–cholesterol–DPPC (A) and paeonol–stigmasterol–DPPC (B) liposomes at molar ratio of 1:1:24 (paeonol:sterol:DPPC) and the deconvoluted patterns of sterol-rich domains (dashed lines) and paeonol-rich domains (dotted lines) at 37°C.
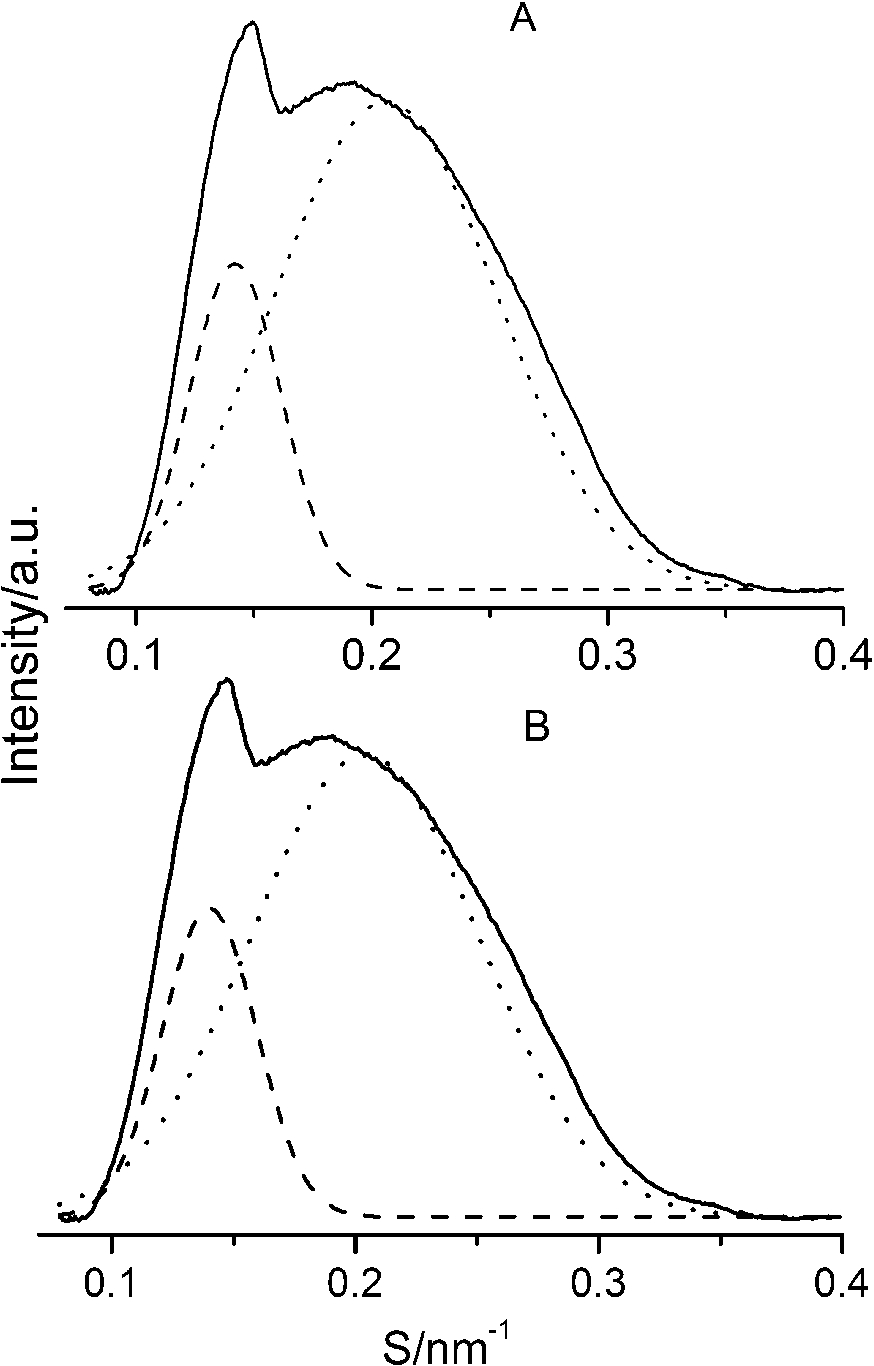
Fig. 5.
The WAXS patterns (solid lines) of paeonol–cholesterol–DPPC (A) and paeonol–stigmasterol–DPPC (B) liposomes at molar ratio of 1:1:24 (paeonol:sterol:DPPC) and the deconvoluted patterns of sterol-rich domains (dashed lines) and paeonol-rich domains (dotted lines) at 37°C.
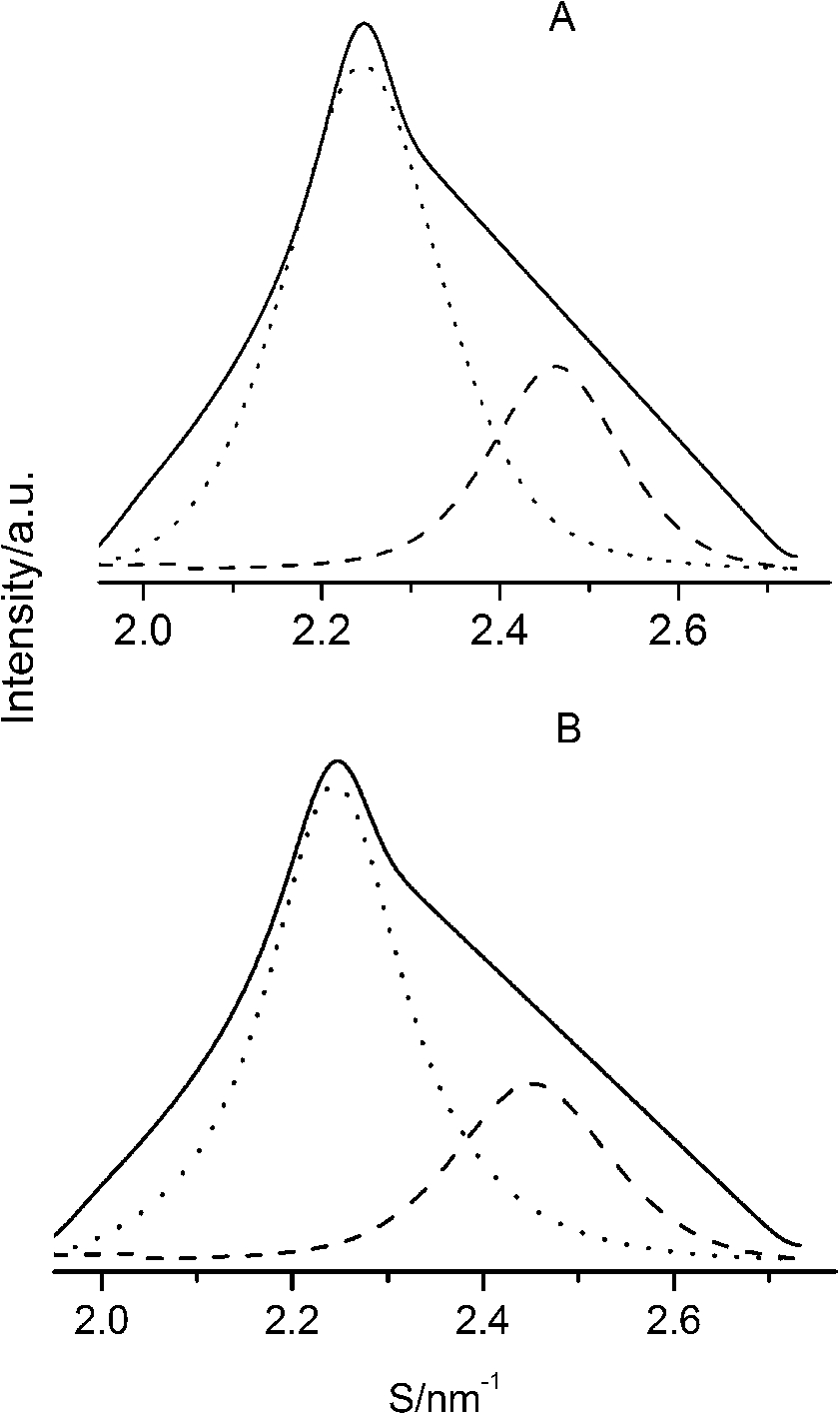
Second, the incorporation of paeonol molecules into sterol–DPPC liposomes disturbs the membrane order. Nunes et al. reported that a reduction of the correlation length of the SAXS induced by adding exogenous molecules could manifest the disturbing effect of exogenous molecules in the membrane order [19,20]. As shown in Table 1, all the correlation lengths (ξ) of SAXS of sterol–DPPC liposomes encapsulating paeonol are shorter than that of sterol–DPPC liposomes in the absence of paeonol. Therefore, the incorporation of paeonol molecules into sterol–DPPC liposomes disturbs the membrane order.
Third, cholesterol is different from stigmasterol in interacting with DPPC. As shown in Table 1, the cholesterol-rich domain shows a smaller d-spacing of WAXS compared with the stigmasterol-rich domain. Thus cholesterol has a stronger tendency to interact with DPPC as compared to stigmasterol. This conclusion can be explained as follows. The difference between the molecular structure of stigmasterol and that of cholesterol lies in that the stigmasterol has an additional ethyl group and a trans double bond in the alkyl tail (as shown in Fig. 1). The additional ethyl group of stigmasterol will suppress the formation of ordered arrangements in the acyl chains of DPPC due to the effect of steric hindrance [1,12,23]. That is to say, the additional ethyl group will cause a less favorable interaction with phospholipids. As to the double bond, although it may cause a favorable interaction with acyl chains of DPPC due to the decreased volume fluctuations in the sterol alkyl chain region, this effect is very limited [1,10,12]. These imply that the destabilizing effect of ethyl group of stigmasterol is more effective than the stabilizing effect of double bond in the molecule. As a result, cholesterol has a stronger affinity for DPPC as compared to stigmasterol.
4.Conclusions
Structural properties of paeonol-encapsulated liposomes containing cholesterol or stigmasterol at physiological temperature of 37°C have been investigated by synchrotron SAXS and WAXS techniques. Three conclusions can be drawn from the results of present experiments: First, phase separation occurs in both sterol–DPPC and paeonol–sterol–DPPC liposomes. Second, the incorporation of paeonol molecules into sterol–DPPC liposomes disturbs the order of the membrane. Third, cholesterol has a stronger tendency to interact with DPPC in comparison with stigmasterol.
Acknowledgements
This work was supported by the Natural Science Foundation of China (Grant No. 21273130) and Beijing Natural Science Foundation (Grant No. 7153171). Assistance of Dr. Zhihong Li and Dr. Guang Mo from Beijing Synchrotron Radiation Facility (BSRF) in the synchrotron station facility setup is gratefully acknowledged.
References
[1] | C. Bernsdorff and R. Winter, Differential properties of the sterols cholesterol, ergosterol, β-sitosterol, trans-7-dehydrocholesterol, stigmasterol and lanosterol on DPPC bilayer order, J. Phys. Chem. B 107: ((2003) ), 10658–10664. doi:10.1021/jp034922a. |
[2] | N.F. Bouxsein, C. Leal, C.S. McAllister, K.K. Ewert, Y. Li, C.E. Samuel and C.R. Safinya, Two-dimensional packing of short DNA with nonpairing overhangs in cationic liposome-DNA complexes: From onsager nematics to columnar nematics with finite-length columns, J. Am. Chem. Soc. 133: ((2011) ), 7585–7595. doi:10.1021/ja202082c. |
[3] | Y.H. Chan, B.H. Chen, C.P. Chiu and Y.F. Lu, The influence of phytosterols on the encapsulation efficiency of cholesterol liposomes, Int. J. Food Sci. Tech. 39: ((2004) ), 985–995. doi:10.1111/j.1365-2621.2004.00867.x. |
[4] | A. Chaudhury, S. Das, R.F.S. Lee, K.B. Tan, W.K. Ng, R.B.H. Tan and G.N.C. Chiu, Lyophilization of cholesterol-free PEGylated liposomes and its impact on drug loading by passive equilibration, Int. J. Pharm. 430: ((2012) ), 165–175. doi:10.1016/j.ijpharm.2012.04.036. |
[5] | J. Chen, C.Q. He, A.H. Lin, W. Gu, Z.P. Chen, W. Li and B.C. Cai, Thermosensitive liposomes with higher phase transition temperature for targeted drug delivery to tumor, Int. J. Pharm. 475: ((2014) ), 408–415. doi:10.1016/j.ijpharm.2014.09.009. |
[6] | M.E. Chevreul, Recherches chimiques sur les corps gras, et particulièrement sur leurs combinaisons avec les alcalis. Sixième mémoire. Examen des graisses d’homme, de mouton, de boeuf, de jaguar et d’oie, Annales de Chimie et de Physique 2: ((1816) ), 339–372. |
[7] | T.C. Chou, Anti-inflammatory and analgesic effects of paeonol in carrageenan-evoked thermal hyperalgesia, Brit. J. Pharmacol. 139: ((2003) ), 1146–1152. doi:10.1038/sj.bjp.0705360. |
[8] | Y.D. Dong and B.J. Boyd, Applications of X-ray scattering in pharmaceutical science, Int. J. Pharm. 417: ((2011) ), 101–111. doi:10.1016/j.ijpharm.2011.01.022. |
[9] | M. Elmowafy, T. Viitala, H.M. Ibrahim, S.K. Abu-Elyazid, A. Samy, A. Kassem and M. Yliperttula, Silymarin loaded liposomes for hepatic targeting: In vitro evaluation and HepG2 drug uptake, Eur. J. Pharm. Sci. 50: ((2013) ), 161–171. doi:10.1016/j.ejps.2013.06.012. |
[10] | W.Y. Gao, L. Chen, F.G. Wu and Z.W. Yu, Liquid ordered phase of binary mixtures containing dipalmitoylphosphatidylcholine and sterols, Acta Phys.-Chim. Sin. 24: ((2008) ), 1149–1154. doi:10.1016/S1872-1508(08)60050-9. |
[11] | D. Gmajner and N.P. Ulrih, Thermotropic phase behaviour of mixed liposomes of archaeal diether and conventional diester lipids, J. Therm. Anal. Calorim. 106: ((2011) ), 255–260. doi:10.1007/s10973-011-1596-4. |
[12] | K.K. Halling and J.P. Slotte, Membrane properties of plant sterols in phospholipid bilayers as determined by differential scanning calorimetry, resonance energy transfer and detergent-induced solubilization, Biochim. Biophys. Acta 1664: ((2004) ), 161–171. doi:10.1016/j.bbamem.2004.05.006. |
[13] | S.H. Kim, S.A. Kim, M.K. Park, S.H. Kim, Y.D. Park, H.J. Na, H.M. Kim, M.K. Shin and K.S. Ahn, Paeonol inhibits anaphylactic reaction by regulating histamine and TNF-alpha, Int. Immunopharmacol. 4: ((2004) ), 279–287. doi:10.1016/j.intimp.2003.12.013. |
[14] | M.A. Kisel, L.N. Kulik, I.S. Tsybovsky, A.P. Vlasov, M.S. Vorob’yov, E.A. Kholodova and Z.V. Zabarovskaya, Liposomes with phosphatidylethanol as a carrier for oral delivery of insulin: Studies in the rat, Int. J. Pharm. 216: ((2001) ), 105–114. doi:10.1016/S0378-5173(01)00579-8. |
[15] | L.M. Lichtenberger, Z.M. Wang, J.J. Romero, C. Ulloa, J.C. Perez, M.N. Giraud and J.C. Barreto, Non-steroidal anti-inflammatory drugs (NSAIDs) associated with zwitterionic phospholipids: Insight into the mechanism and reversal of NSAID-induced gastrointestinal injury, Nat. Med. 1: ((1995) ), 154–158. doi:10.1038/nm0295-154. |
[16] | J.H. Lin, X.L. Wang, Q. Wu, J.D. Dai, H.D. Guan, W.Y. Cao, L.Y. He and Y.R. Wang, Development of salvianolic acid B-tanshinone II A-glycyrrhetinic acid compound liposomes: Formulation optimization and its effects on proliferation of hepatic stellate cells, Int. J. Pharm. 462: ((2014) ), 11–18. doi:10.1016/j.ijpharm.2013.12.040. |
[17] | D. McKersie and J.E. Thompson, Influence of plant sterols on the phase properties of phospholipid bilayers, Plant. Physiol. 63: ((1979) ), 802–805. doi:10.1104/pp.63.5.802. |
[18] | K. Muramatsu, Y. Maitani and T. Nagai, Dipalmitoylphosphatidylcholine liposomes with soybean derived sterols and cholesterol as a carrier for the oral administration of insulin in rats, Biol. Pharm. Bull. 19: ((1996) ), 1055–1058. doi:10.1248/bpb.19.1055. |
[19] | C. Nunes, G. Brezesinski, J.L. Lima, S. Reisa and M. Lúcio, Effects of non-steroidal anti-inflammatory drugs on the structure of lipid bilayers: Therapeutical aspects, Soft Matter. 7: ((2011) ), 3002–3010. doi:10.1039/c0sm00686f. |
[20] | C. Nunes, G. Brezesinski, J.L. Lima, S. Reisa and M. Lúcio, Synchrotron SAXS and WAXS study of the interactions of NSAIDs with lipid membranes, J. Phys. Chem. B 115: ((2011) ), 8024–8032. doi:10.1021/jp2025158. |
[21] | B. Pili, C. Bourgaux, F. Meneau, P. Couvreur and M. Ollivon, Interaction of an anticancer drug, gemcitabine, with phospholipid bilayers, J. Therm. Anal. Calorim. 98: ((2009) ), 19–28. doi:10.1007/s10973-009-0229-7. |
[22] | P.J. Quinn, Characterisation of clusters of a-tocopherol in gel and fluid phases of dipalmitoylglycerophosphocholine, Eur. J. Biochem. 233: ((1995) ), 916–925. doi:10.1111/j.1432-1033.1995.916_3.x. |
[23] | Y.L. Su, Q.Z. Li, L. Chen and Z.W. Yu, Condensation effect of cholesterol, stigmasterol, and sitosterol on dipalmitoylphosphatidylcholine in molecular monolayers, Colloid Surf. A-Physicochem. Eng. Asp. 293: ((2007) ), 123–129. doi:10.1016/j.colsurfa.2006.07.016. |
[24] | D.Z. Sun, L. Li, X.M. Qiu, F. Liu and B.L. Yin, Isothermal titration calorimetry and 1H NMR studies on host–guest interaction of paeonol and two of its isomers with β-cyclodextrin, Int. J. Pharm. 316: ((2006) ), 7–13. doi:10.1016/j.ijpharm.2006.02.020. |
[25] | S.H. Takano, Y. Aramaky and S. Tsuchiya, Physicochemical properties of liposomes affecting apoptosis induced by cationic liposomes in macrophages, Pharm. Res. 20: ((2003) ), 962–968. doi:10.1023/A:1024441702398. |
[26] | V.A.D. Tiera, F.M. Winnik and M.J. Tiera, Interaction of amphiphilic derivatives of chitosan with DPPC (1,2-dipalmitoyl-sn-glycero-3-phosphocholine), J. Therm. Anal. Calorim. 100: ((2010) ), 309–313. doi:10.1007/s10973-009-0375-y. |
[27] | F.G. Wu, Q. Jia, R.G. Wu and Z.W. Yu, Regional cooperativity in the phase transitions of dipalmitoylphosphatidylcholine bilayers: The lipid tail triggers the isothermal crystallization process, J. Phys. Chem. B 115: ((2011) ), 8559–8568. doi:10.1021/jp200733y. |
[28] | F.G. Wu, N.N. Wang, L.F. Tao and Z.W. Yu, Acetonitrile induces nonsynchronous interdigitation and dehydration of dipalmitoylphosphatidylcholine bilayers, J. Phys. Chem. B 114: ((2010) ), 12685–12691. doi:10.1021/jp104190z. |
[29] | R.G. Wu, L. Chen and Z.W. Yu, Structural properties of the liquid ordered phase of phosphatidylcholine/stigmasterol liposomes: A synchrotron X-ray diffraction study, Acta Phys.-Chim. Sin. 28: ((2012) ), 2008–2013. |
[30] | R.G. Wu, L. Chen, Z.W. Yu and P.J. Quinn, Phase diagram of stigmasterol–dipalmitoylphosphatidylcholine mixtures dispersed in excess water, Biochim. Biophys. Acta 1758: ((2006) ), 764–771. doi:10.1016/j.bbamem.2006.04.017. |
[31] | R.G. Wu, J.D. Dai, F.G. Wu, X.H. Zhang, W.F. Li and Y.R. Wang, Competitive molecular interaction among paeonol-loaded liposomes: Differential scanning calorimetry and synchrotron X-ray diffraction studies, Int. J. Pharm. 438: ((2012) ), 91–97. doi:10.1016/j.ijpharm.2012.08.052. |
[32] | R.G. Wu, Y.R. Wang, F.G. Wu, H.W. Zhou, X.H. Zhang and J.L. Hou, A DSC study of paeonol-encapsulated liposomes, comparison of the effect of cholesterol and stigmasterol on the thermotropic phase behavior of liposomes, J. Therm. Anal. Calorim. 109: ((2012) ), 311–316. doi:10.1007/s10973-012-2331-5. |
[33] | X.A. Wu, H.L. Chen, X.G. Chen and Z.D. Hu, Determination of paeonol in rat plasma by high-performance liquid chromatography and its application to pharmacokinetic studies following oral administration of Moutan cortex decoction, Biomed. Chromatogr. 17: ((2003) ), 504–508. doi:10.1002/bmc.259. |
[34] | X.L. Xu, R. Bittman, G. Duportail, D. Heissler, C. Vilcheze and E. London, Effect of the structure of natural sterols and sphingolipids on the formation of ordered sphingolipid/sterol domains (rafts), J. Biol. Chem. 276: ((2001) ), 33540–33546. doi:10.1074/jbc.M104776200. |
[35] | Z.W. Yu and P.J. Quinn, Phase stability of phosphatidylcholines in dimethylsulfoxide solutions, Biophys. J. 69: ((1995) ), 1456–1463. doi:10.1016/S0006-3495(95)80015-9. |