Exosomes in the visual system: New avenues in ocular diseases
Abstract
Exosomes are a subgroup of membrane-bound extracellular vesicles secreted by all cell types and present virtually in all biological fluids. The composition of exosomes in the same cell type varies in healthy and disease conditions. Hence, exosomes research is a prime focus area for clinical research in cancer and numerous age-related metabolic syndromes. Functions of exosomes include crucial cell-to-cell communication that mediates complex cellular processes, such as antigen presentation, stem cell differentiation, and angiogenesis. However, very few studies reported the presence and role of exosomes in normal physiological and pathological conditions of specialized ocular tissues of the eye and ocular cancers. The eye being a protected sense organ with unique connectivity with the rest of the body through the blood and natural passages, we believe that the role of exosomes in ocular tissues will significantly improve our understanding of ocular diseases and their interactions with the rest of the body. We present a review that highlights the existence and function of exosomes in various ocular tissues, their role in the progression of some of the neoplastic and non-neoplastic conditions of the eyes.
1Introduction
Extracellular vesicles (EVs) are diverse groups of membrane-bound nano-sized vesicles found abundantly in all body fluids such as blood, milk, cerebrospinal fluid, urine including ocular tissues, and ocular fluids like tears, aqueous, and vitreous humor (VH). Wolf first identified EVs in plasma and termed them “platelet dust” [1]. Earlier, EVs were considered waste-removing vehicles released by cells. But recent studies have established them as effectors of cellular communication in normal and pathological conditions. However, over the last decade, there has been an enormous amount of knowledge gained about EVs with specific reference to small EVs (< 200 nm) with endosomal origin called exosomes. From being considered as dust, they are now believed to be contributing to the disease progression. Evidence from research studies shows that exosomes promote tumor progression by promoting angiogenesis and tumor cell migration in metastasis [2, 3]. While the role of exosomes is important in every organ, it is of unique importance in the eye, a specialized sense organ capable of receiving visual images, which are then carried to the brain. It is not only uniquely situated in a bony orbit, connected directly with a cranial nerve to the brain and meninges, enjoys immune privilege, and has a blood-retinal barrier in the retina that regulates the nutrient flux between the circulating blood and the retina. Because of our interest in ocular pathology and ophthalmic research, and the emerging evidence of the role of exosomes, in this review, we present a brief overview of EVs, the classification of different types of EVs, and the most well-described entity “exosomes” with specific reference to ocular tissues in health and disease conditions. As clarified in the subsequent paragraphs, there is a slight ambiguity regarding small EVs vs exosomes in using terminology. Therefore, we have included the keywords “EVs”, “microvesicles (MVs)”, “small EVs” and “exosomes” for the literature search.
2Classification of EVs
Three main subtypes of EVs are described based on their subcellular origin, release pathway, vesicle size, content (cargos: DNA, RNA, and proteins), and function [4]. The largest vesicles are apoptotic bodies having sizes varying from 1–5 μm in diameter, that are released during apoptotic processes through budding directly from the plasma membrane and then discharged into extracellular space [5]. MVs’ size ranges from 100–1000 nm. MVs are formed by direct budding of the cell’s plasma membrane. Exosomes are the smallest subpopulation among all EVs with a diameter of 30–150 nm. Exosomes are released into the extracellular space after fusion with late endosomes or multi-vesicular bodies with the plasma membrane. The characteristics of three different types of EVs are shown inTable 1.
Table 1
Characteristics of different types of Extracellular Vesicles
Properties | Apoptotic bodies | Microvesicles | Exosomes |
Approximate size | 1–5 μm | 100–1,000 nm | 30–150 nm |
Biogenesis | Cellular, Plasma membrane fragment | Cellular contents | Intraluminal vesicles within multivesicular bodies |
Release | Apoptosis, Cell necrosis | Blebbing of the plasma membrane | Fusion of multivesicular bodies with the plasma membrane |
Activation | Apoptosis | Constitutive and/or induced by specific signal | Constitutive and/or induced by specific signal |
Pathways used to secrete | Apoptosis | Cell-dependent Ca2+-dependent stimuli, PH, dependent | ESCRT-dependent, independent, Tetraspanin-dependent Ceramide-dependent stimuli-dependent |
Lipid composition | ————- | Phosphatidylserine, enriched in cholesterol and diacylglycerol, | Enriched in cholesterol and ceramide, phosphatidylcholine, phosphatidylethanolamine, serine |
Cargos | Proteins, lipids, DNA, miRNAs Cell organelles, fragments | Proteins, mRNA, miRNA, lipids | Proteins, mRNA, miRNA, lipids, DNA Glycoproteins |
Protein Marker | Caspase 3, histones, | Integrin, CD40, Selectins | CD63, CD81, Alix, Tsg101 |
Techniques EVs for isolation | Poorly standardized protocols | Ultracentrifugation, Differential centrifugation | Ultracentrifugation, Differential centrifugation and Sucrose gradient, Commercial kit, PEG precipitation method |
Characterization techniques | Flow cytometry, Microscopy, SEM | Flow cytometry, FE-SEM capture based assays, Microscopy | TEM, FE-SEM, SEM, flow cytometry, western blotting, Mass spectrometry |
SEM: Scanning electron microscopy), FE-SEM: Field emission electron microscopy), TEM: Transmission electron microscopy.
As evident in Table 1, there is an overlap of size between exosomes and MVs and the same is reflected in various publications. Some of the authors used the size of 30–100 nm [4, 6] as the criterion of distinguishing exosomes, while others have used the size of 40–120 nm [7], 30–150 nm [8], 50–150 nm [9], and 40–200 nm [10]. One may speculate that the differences in sizes of exosomes may result from different types of methods and principles used for measuring; the hydrodynamic diameter measured by nanoparticle tracking analysis (NTA) and dynamic light scattering (DLS) is in the range of 119 and 182 nm, whereas geometric sizes of hydrated and desiccated exosomes measured by differential mobility analyzer (DMA), and scanning electron microscopy (SEM) are 37–71 nm [11]. Moreover, it became a general practice that the term “exosome” is used for all the vesicles of less than 200 nm, even when their origin is unknown/ not proved as endosomal [12]. Because of this, the International Society for Extracellular Vesicles (ISEV) framed Minimal Information for Studies of Extracellular Vesicles (“MISEV”) guidelines for uniforming nomenclature and functionally characterize different groups of EVs. As per MISEV 2014 and updated 2018 guidelines it is recommended to name the EV subtypes that refer to a) physical properties of EVs, like size (small EVs for < 100 nm and < 200 nm vesicles; large/medium for > 200 nm vesicles) or density (low, middle, high, with each range defined; b) cell surface marker/biochemical composition (CD63 + /CD81+- EVs, Annexin A5-stained EVs, etc.); or c) based on the cell of origin (podocyte EVs, hypoxic EVs, large oncosomes, apoptotic bodies) instead of terms such as “exosome” and “MV” [13]. Literature review on EVs’ role in ocular health and disease also revealed the ambiguity of using the term “exosomes”. The majority of the authors quoted small vesicles (< 200 nm) as exosomes, some of them referred to them as “small EVs” or “EVs” as such without addressing their origin. We have included all of them in the review as they fall into the definition/criteria of the size of exosomes. We also believe that the work should be quoted as such without any change from our end. Thus, the terminology used for subtypes of EVs in this review is as per source publication. Numerous methods are available for the isolation of EVs, but none of them offer substantial yields with reliable quality. Common methods used for EV isolation are density gradient centrifugation techniques, size exclusion chromatography, ultrafiltration, and microfluidics-based sorting mechanisms. Immunoaffinity methods using anti-CD63, -CD9, and -CD81 antibodies are used to isolate exosomes from mixed cell populations such as in cell cultures, tissues, and biological fluids (plasma, serum, and urine) [14, 15]. EV characterization involves the determination of vesicle size, shape, surface charge, porosity, particle concentration, stability, yield, and purity which are determined by several biophysical and molecular biology methods [16].
3Exosomes
Exosomes originating from late endosomes along with their cargo are considered of great importance due to their key role in cell-cell communication, neovascularization, stem cell differentiation, in maintaining cellular integrity and homeostasis in normal and diseased conditions such as cancers, neurodegenerative diseases, cardiovascular illnesses, dry eye syndrome, age-related macular degeneration (AMD) and glaucoma [17]. Exosomes were first described by two different research groups led by Harding and Pan in 1983 and found that transferrin receptors moved from the plasma membrane to the reticulocytes in a ∼50 nm-sized vesicle and then released the receptors from the maturing reticulocytes which then repacked with ∼50 nm-sized vesicles inside them. Afterward, they are released from maturing blood reticulocytes into the extracellular space without undergoing its usual process, the lysosomal destruction [18, 19]. Rose Johnstone named these vesicles as exosomes in 1987 [20]. Since then, exosomes derived from different types of cultured cells such as reticulocytes, immune cells, neurons, fibroblasts, primary tumors, and also from above-stated disease conditions have been isolated and characterized [21–23]. In addition to this, exosomes have also been purified from several body fluids such as saliva, blood, urine, breast milk, cerebrospinal fluid, bronchoalveolar fluid, epididymal fluid, amniotic fluid, and in aqueous humor (AH), suggesting their key role in normal physiological processes [24–28].
It is interesting to note that also vesicles derived from different kinds of stem cells (pluripotent, embryonic, mesenchymal, and cardiac progenitor cells) are being evaluated, and given the nature of these vesicles, they are emerging as new therapeutic aids in the treatment of cardiovascular, neurodegenerative, and inflammatory ocular diseases and are being studied in various disease models [29–31]. For instance, exosomes derived from mesenchymal stem cells (MSCs) can improve cognition by repairing oxidative damage in neurons and astrocytes in cognitively impaired diabetic animals [32]. Another study reported that exosomes secreted from MSCs delivered into a rodent model of optic nerve crush can protect retinal ganglion cells (RGC) from death and preserve its function by exerting their neuroprotective and neurogenic effects [33]. The clinical significance of these vesicles is due to the following properties: a) significant difference in vesicle content during normal and disease conditions b) non-cytotoxicity, c) low immunogenicity, d) easy to determine their cellular origin as numerous cells express specific cell surface molecules, e) non-invasive specimen collection because of their presence in biofluids (saliva, tears, blood, and urine, etc), f) stable and can be stored for longer periods for analysis, g) high cargo loading capacity [34–36]. Based on these properties, exosomes are being studied for their role in corneal wound healing and regeneration, immune modulation, potential biomarkers, and drug delivery vehicles. The therapeutic applications of exosomes are depicted in Fig. 1.
Fig. 1
Therapeutic Applications of Exosomes.
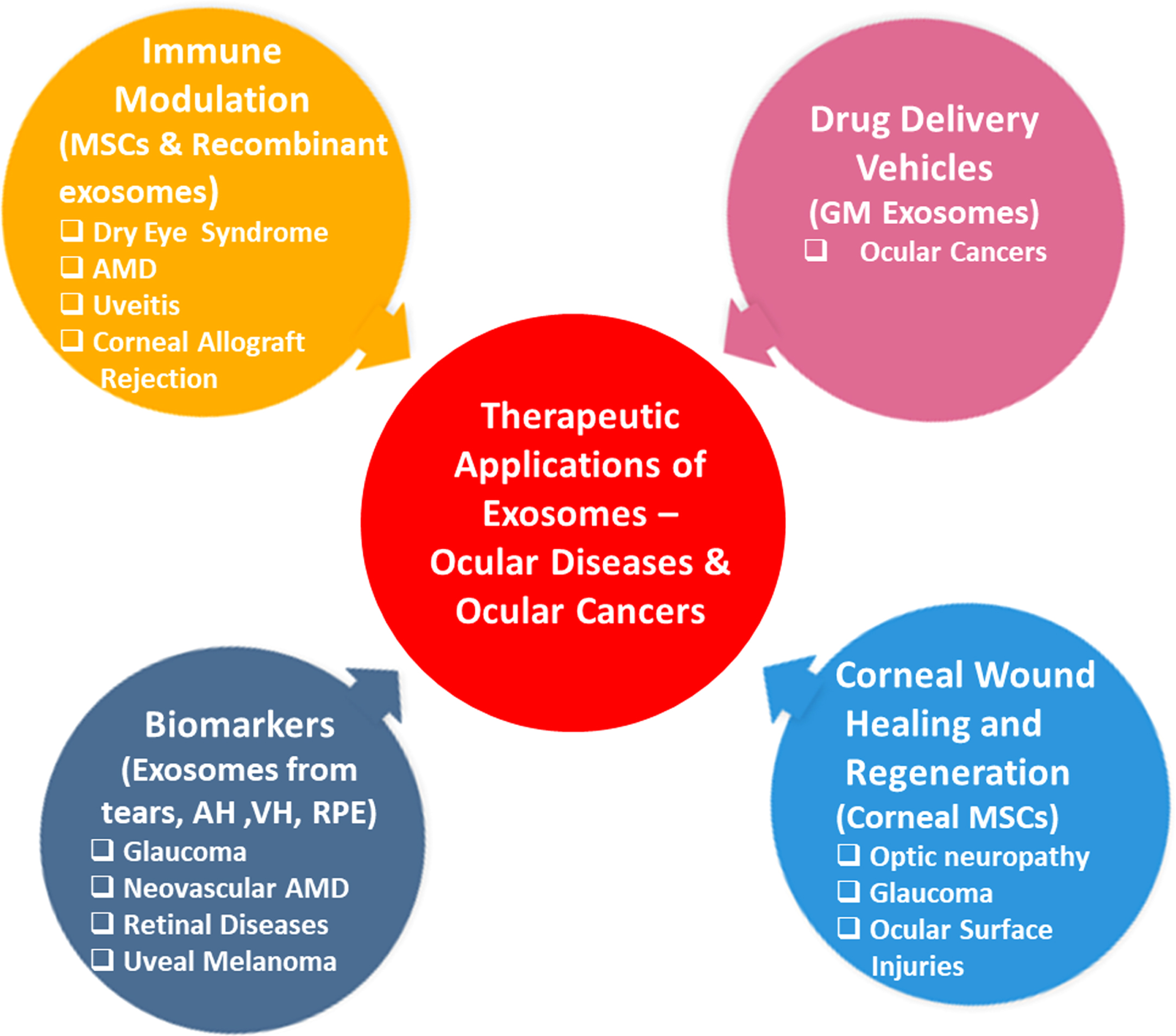
Recent reports suggested that the delivery of exosomes into the eye can produce promising therapeutic applications in several models of retinal disease, which includes preventing retinal injury and restoration of RGC that are lost during optic neuropathy and glaucoma [37, 38]. It has been shown that the corneal wound healing and neovascularization properties of epithelial cell-derived exosomes are mediated via intercellular communication between corneal epithelial cells, corneal keratocytes, and vascular endothelial cells. As a result, exosomes can be considered as ideal targets for possible therapeutic interventions [39]. Samaeekia et al. reported that exosomes isolated from corneal MSCs could elicit corneal epithelial wound healing and may serve as a therapeutic approach for ocular surface injuries [40]. In a recent study, Tao et al. isolated EVs having a size within the range of exosomes from human placenta-derived MSCs (hP-MSCs) and showed their corneal wound healing properties in a mouse alkali-burn corneal injury model. hP-MSCs effectively enhanced wound healing by inhibiting inflammation and angiogenesis [41]. Exosomes can also be genetically modified so that they can easily pass through the biological barriers, the blood-brain barrier, penetrate tissues, help in cell-specific targeting through their cargo, and efficiently carry synthetic drugs and thus are considered natural nanocarriers [42, 43]. Some of the clinically tested drugs or entities that are delivered through exosomes are curcumin, doxorubicin, miR-122, etc. for the treatment of various cancers, ulcers and type I diabetes mellitus [42, 44].
4Exosomes in ocular tissues
The eye is composed of three layers- the outer protective sclera with cornea and conjunctiva, middle vascular uveal tissues (iris, ciliary body, and choroid), and the inner retinal layer that receives the visual signals. Some of the unique features of these ocular tissues are transparency and avascularity of cornea, transparent media and lens, and neurosensory retina that carries the images to the brain for interpretation. Added to this are an immune privilege, blood-retinal barriers, and direct visualization of retinal blood vessels which are believed to be the window to the body. This unique feature makes the eye susceptible to a wide variety of diseases and each part of the eye can give important clues to the diagnosis of systemic diseases, eg. diabetes, hypertension, jaundice, anemia. Hence, it is apparent that the exosome research in the eye is an exciting field, as exosomes have a potential as drug delivery vehicles, biomarkers, and therapeutic agents. Several research groups have identified exosomes in different eye structures and ascertained their role in normal physiology and diseased conditions.
Exosomes have been reported in various ocular tissues such as cornea (corneal epithelial cells), ciliary body (CB), lacrimal gland (LG), retina (retinal pigment epithelial cells (RPE), trabecular meshwork (TM), and ocular fluids like AH, VH, and also in tears [45, 46]. The presence of exosomes in different ocular tissues/fluids is depicted in Fig. 2. Exosomes were identified in vitreous samples of normal humans (vitrectomized), cadavers, and mouse eyes. It is hypothesized that the interaction between the vitreous, and retina is mediated through exosomes present in the vitreous [47].
Fig. 2
The presence of exosomes in different ocular tissues: Exosomes are present in the ocular tissues such as cornea (corneal epithelial cells), ciliary body, lacrimal gland, retina (retinal pigment epithelial cells), trabecular meshwork, and ocular fluids like aqueous humor, vitreous humor, and tears.
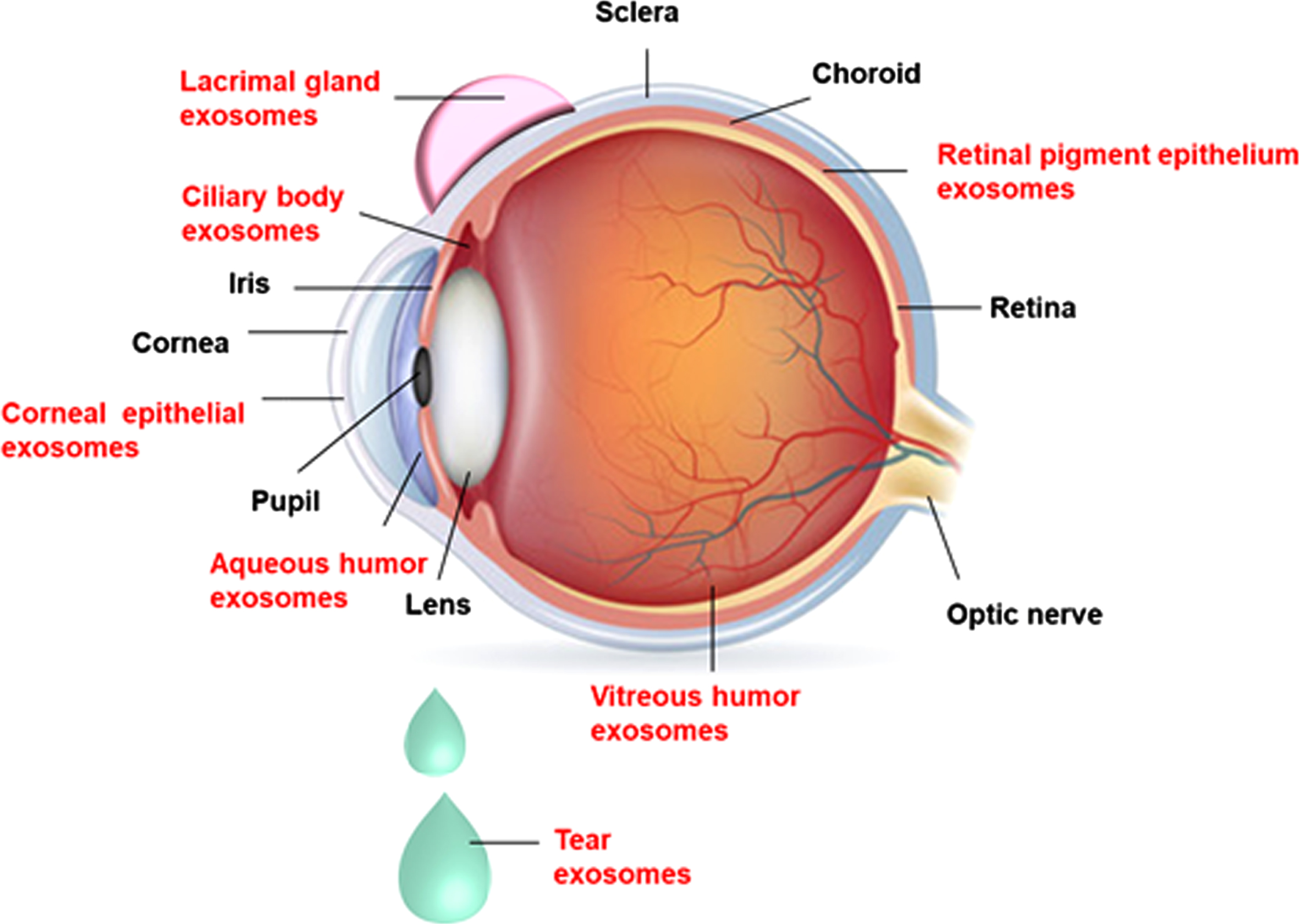
4.1Exosomes in the anterior segment
Han et al. identified the corneal wound healing and neovascularization properties of corneal epithelial cell-derived exosomes in rat epithelial debridement model and rabbit anterior stromal keratectomy model. After 18 hours of epithelial debridement in rat cornea, exosomes were observed between corneal epithelial cells and the stroma during wound healing. Likewise, exosomes were also visible in the rabbit cornea after 48 hours of anterior stromal keratectomy. Further, proliferation and transformation of stromal fibroblasts into myofibroblasts and endothelial cell proliferation were observed after exposure of mouse corneal epithelial cell-derived exosomes. The angiogenic properties of exosomes are also confirmed by in vitro and ex vivo assays. In addition, proteomic analysis of epithelial cell-derived exosomes confirmed that these vesicles harbor proteins related to wound healing and neovascularization [39]. A study by Leszczynska et al. confirmed the role of exosomes in the limbal niche in limbal stromal – limbal epithelial cell communications in healthy and diabetic corneas. The molecular content (RNA) present in exosomes derived from normal and diabetic limbal stromal cells (LSC) are different and exosomes derived from normal LSC showed superior paracrine effects on limbal epithelial cells (LEC), such as proliferation and wound healing, compared to diabetic exosomes. These effects are mediated via AKT (protein kinase B) signaling pathway. The differential expression profiles of small RNAs in exosomes derived from normal and diabetic LSCs suggest an involvement of miRNAs in diabetic corneas. These results will pave the way for the development of novel therapeutic strategies that specifically target the limbal niche and its cell constituents for treating corneal diseases [48]. Exosomes were also identified in human tears and confirmed by the expression of exosome-specfic markers, such as CD63, CD9, and CD24 receptors [49–51]. In a recent study, Han et al. proved the presence of exosomes in tear fluid by the expression of CD81 antibody and reported that elevated levels of exosomal proteins were recovered from tears of thyroid eye disease (TED) patients compared to healthy controls. Furthermore, cultured orbital fibroblasts expressed higher levels of interleukins (IL-6 & IL-8) and monocyte chemoattractant protein-1 after exposing to tear exosomes from TED patients indicating that exosomes and their proteins may be involved in TED pathogenesis [52]. These studies show that small EVs particularly exosomes are found in various ocular tissues and they play an important role in intercellular communication, inflammation responses and cause alterations in signaling pathways. Different types of exosomal cargos present in ocular tissues/fluids and their functions in normal and disease conditions of the eye are summarized in Table 2.
Table 2
Role of exosomes in ocular diseases
S. No | Name of the ocular disease | Source of exosomes | Cargo Evaluated | Biological function in eye in health and disease | Ref |
Role of exosomes in human ocular diseases | |||||
1 | AMD | AH | Cytokeratin 8, cytokeratin 14, cathepsin D, Hsp70; myosin-9, and actin, aortic smooth muscle | Proteins related to the autophagy-lysosomal pathway and epithelial-mesenchymal transition | [73] |
Serum | hsa-miR (19a, 126, and 410) | Apoptosis and/or angiogenesis | [97] | ||
2 | Cataract | AH | has-miRNAs (miR-486-5p, miR-204, and miR-184miR181a, miR-191, miR-148a, miR-26a, miR-125a-5p, let-7a,, let7b) | Communication between AH inflow and outflow tissues to maintain intraocular pressure homeostasis | [68] |
3 | Myopia | AH | has-miR (582-3p, 17-5p, 885-3p, 19b-3p, and 450b-5p) | NA | [122] |
AH | Proteins (TTR and HPX) | NA | [123] | ||
Ocular cell lines/primary cells in ocular diseased condition | |||||
4 | AMD | ARPE-19 | VEGFR2 | Involved in neo angiogenesis | [85] |
ARPE-19 | has-miR -494-3p | Potential biomarkers of mitochondrial dysfunction | [124] | ||
ARPE-19 | Apaf1 | Induction of inflammation and apoptosis in normal RPE cells via Apaf1/caspase-9 axis | [125] | ||
ARPE-19 | Angiogenic factors | Promotion of the angiogenesis | [126] | ||
Primary porcine RPE cells, ARPE-19 | HDAC6 | Communicate stress messages RPE cells and contributing to RPE dysfunction | [94] | ||
ARPE-19 | Proteins (CD63 and LAMP2) | Drusen formation | [96] | ||
5 | DR | ARPE-19 | has-miR-543 | Induction of EMT in receptor RPE cells | [127] |
ARPE-19 | has-miR-202-5p | Inhibition of growth, migration and tube formation of Diabetic Retinopathy (DR)HUVEC cells | [91] | ||
6 | DR | Human retinal pericytes (ACBRI-183) | Circular RNA cPWWP2A | Regulation of retinal microvascular function by Mir-579/ingiogenin 1/occludin/SIRT1 pathway | [128] |
Human retinal astrocytes | NA | Promotion of the proliferation and migration of endothelial cells | [129] | ||
7 | RD | ARPE-19 | NA | Protect the photoreceptor and inhibit the expression of inflammatory factors, reduce the oxidative damage | [130] |
8 | POAG | NPCE primary cells | has-miR-29b | Reduces levels of COL3A1 and regulates WNT/β-catenin pathway | [131] |
Primary human (TM) cells | has-miR-182 | Associated with POAG via miR-182 | [132] | ||
Role of exosomes in ocular cancers | |||||
9 | RB | Cell culture conditioned media (CM) | Proteins | The expressed proteins related to invasion and metastasis | [113] |
Serum | hsa-miR-301b-3p and hsa-miR-216b-5p | Biomarkers | [114] | ||
RB tissues | hsa-miR-373, hsa-miR-181a | Invasion and metastasis CDKN1B-mediated cell cycle pathway | [111] | ||
HVEC | has-miR -92a -3p | Promote angiogenesis | [133] | ||
10 | UM | Vitreous humor (VH) and serum | has-miR (-21-34a, -146a) | Promotion the proliferation of tumor cells | [107] |
NA | MIF | A novel drug therapy to prevent metastasis in uveal melanoma | [102] | ||
Ocular cancer cell lines | |||||
11 | RB cell lines WERIRB | Cell culture conditioned media | has-miR (-92a,–20a, –129a –17) and proteins (C–X–C 4, thrombospondin 1- 7A) | Enhance tumour deterioration by infiltrating the microenvironment | [101] |
Cell lines derived from primitive mass and vitreous seeding | Cell culture conditioned media | Proteins (ENO3, GALK1, ASNS and SCCPDH) | Invasion and metastasis | [113] | |
12 | UM cell line MP41, MP46, 92.1, MEL 270, MEL285 and OMM2.5 | Cell culture conditioned media | HSP90, HSP70 and integrin V | Involvement of cancer progression and metastasis. | [106] |
13 | UM- melanoma cell line A375, MML-1 | Cell culture conditioned media | microRNA | Provides theoretical basis for the diagnosis of exosomes in metastatic UM | [134] |
92.1, Omm1.3 and Omm1 | Cell culture conditioned media | Pro-inflammatory cytokine | Activates cell signaling pathways and induce metastasis | [102] | |
Therapeutic applications of human stem cell-derived exosomes for ocular diseases | |||||
14 | DR | Human umbilical cord- derived exosomes | hsa-miR126 | Reduces-hyperglycemia induced retinal inflammation by inhibiting the HMGB1 pathway | [135] |
15 | Retinal ischemia-reperfusion | Human bone marrow MSCs | NA | Enhancement of the functional recovery and reduction of the neuroinflammation and apoptosis | [136] |
16 | RD | Neural stem/progenitor cell | hsa-miR (26a-5p, 21-5p, etc.), hsa-let-7a-5p | Protection of photoreceptor cells by inactivating microglia cells | [137] |
17 | Optic nerve injury | Human placenta-derived MSCs | NA | Restore the expression of regeneration markers in R28 cells injured by hypoxia damage | [138] |
18 | CNV | Human umbilical cord MSCs | hsa-miR-27b | Inhibition of EMT and subretinal fibrosis by targeting HOXC6 | [139] |
19 | AU | Human MSCs | NA | Ameliorates experimental AU through suppressing the migration of inflammatory cells | [81] |
20 | DR | Adipose MSCs | hsa-miR-192 | Negative regulation of ITGA1 | [140] |
AMD: Age-related macular degeneration; AH: Aqueous humor; NA: Not available; HPX: Hemopexin; TTR: Transthyretin; ARPE-19: A spontaneously arising retinal pigment epithelia; VEGF-2:Vascular endothelial growth factor receptor 2; DR: Diabetic Retinopathy; HDAC6: Histone deacetylase; RD: Retinal degeneration; LAMP: Lysosome-associated membrane glycoprotein; HUVEC: Human umbilical vein endothelial cells; POAG: Primary open angle glaucoma; NPCE: Non-pigmented ciliary epithelium; TM: Trabecular meshwork; RB: Retinoblastoma; UM: Uveal melanoma; CNV: Choroidal neovascularization; HoX: Homeobox protein; RPE: Retinal pigment epithelial cells; ENO3: Enolase 3; GALK1: Galactokinase ASNS: Asparagine synthetase; SCCPDH: Saccharopine dehydrogenase-like oxidoreductas; C-X-C-4:chemokine receptor type 4; MSC: Mesenchymal Stem Cell; EMT: Epithelial-mesenchymal transformation; 6;; MIF: Macrophage migration inhibitory factor; AU: Autoimmune Uveitis; ITGA1: Integrin Subunit Alpha 1.
4.1.1Corneal allograft rejection
Keratoplasty is carried out to improve the vision and to preserve corneal integrity. Although the avascular nature of the cornea enjoys immune privilege, corneal graft failure is mainly attributed to the allograft rejection caused by the activation of an adaptive immune response [53, 54]. Antigen-presenting cells (APCs) such as dendritic cells, macrophages, and B cells play an important role in graft rejection. After corneal allograft, donor APCs carrying HLA-II molecules sensitize host T cells and activate CD4+ helper T-cells and CD8 + cytotoxic T cells, which will specifically target donor cells [55]. Recent studies explored the role of exosomes in adaptive immune responses mediated in allograft rejection as well as allograft tolerance [56]. Following the progress in the use of MSC-derived exosomes in islet transplantation, there appears to be an interest in the clinical application of exosomes for successful corneal transplantation [57, 58].
4.1.2Sjögren’s syndrome (SS)
SS is an autoimmune disease affecting salivary and lacrimal glands (LGs) resulting in dry mouth and dry eyes. SS is one of the severe forms of dry eye diseases and is caused by dysfunction of the lacrimal functional unit (LFU), which is primarily due to the infiltration of immune cells [59]. Both innate and adaptive immune system pathways play a key role in SS pathogenesis [60]. Along with LFU, other components such as immune cells, matrix cells, hormones, small molecules, and even microbiome interact together to form the ocular surface microenvironment (OSM) and they regulate ocular surface homeostasis [61]. Recent studies have revealed that along with LFU, paracrine factors such as exosomes released from OSM are critical for maintaining homeostasis and for treating ocular surface diseases, as they can overcome the limitations and risks of stem cell transplantation [62].
Very few studies have reported the involvement of exosomes in pterygium, a very common benign recurrent ocular surface outgrowth of the conjunctiva that grows towards the cornea and invades it causing ocular irritation, blurred vision, and visual impairment in some patients. Previous studies reported that abnormal matrix production by fibroblasts and tissue invasion leads to this clinical condition. Analysis of secretome profile provides insights about abnormal proteins and molecular pathways involved in the disease pathobiology. Hou et al. reported that 31% of proteins identified in the secretome of combined pterygium and conjunctival fibroblasts in vitro were exosome proteins. Pathway analysis revealed that these proteins are involved in cancer-related p53-related pathways, ECM-receptor interaction, and TGF-beta signaling pathways [63].
4.1.3Chronic graft versus host disease (cGvHD)
Dry eye disease is also initiated by cGvHD condition, which is a consequence of graft rejection post bone marrow transplantation. Although a wide variety of therapeutic options are available for cGvHD, the overall efficacy is poor with significant side effects affecting the quality of life. To overcome these negative effects and to improve the therapeutic efficacy, MSCs were tested for the treatment of cGvHD-related dry eye disease and it was found that infusion of MSCs improved clinical symptoms in 54.6% of the patients [64]. A phase 1 & 2 clinical trials (NCT04213248) was initiated by the same authors in December 2019 to verify whether umbilical cord mesenchymal stem cell (UMSC)-derived exosomes can relieve dry eye symptoms in cGvHD patients, and to evaluate the clinical efficacy. The study design included recruitment of 27 patients; treating them with artificial tears for 2 weeks to get the normalized baseline, followed by UMSC-exosome intervention for 2 weeks. Alleviation of dry eye symptoms in patients with cGvHD would be measured by Ocular Surface Index Score (OSDI), tear secretion amount, tear break time, and other tissue damage parameters. These patients are to be followed up for 12 weeks. This trial is still under the recruitment phase and the results are awaited as per the latest update in February 2022 [65].
4.2Exosomes in inflammatory and autoimmune diseases of the eye
The eye is considered an immune-privileged (IP) site that prevents immune-mediated inflammation within the intraocular compartments, and this phenomenon is believed to be essential for the protection of vision. With the breach in immune privilege status several inflammatory and immune-mediated vision-threatening diseases of the eye, such as glaucoma and uveitis, and several corneal infections occur [54, 66]. In these disease conditions, as a consequence of responses from the immune cells, expression of pro-inflammatory cytokines and chemokines cause local inflammatory responses resulting in ocular tissue damage that can be repaired by exosomes derived from MSCs, and could serve as a promising cell-free therapy [54, 67].
4.2.1Glaucoma
Glaucoma is a diverse group of disorders characterized by optic neuropathy leading to loss of vision and caused by elevated intraocular pressure (IOP). IOP is based on the equilibrium between inflow and outflow of AH through the TM. The TM inducible glucocorticoid response protein (MYOC) also called myocilin is highly expressed in the TM and plays a key role in the regulation of IOP. Abnormal expression of MYOC leads to TM dysfunction resulting in increased IOP. The mechanism of AH homeostasis and release of abnormal EVs with myocilin proteins is illustrated in Fig. 3. Numerous studies have suggested that the soluble components such as hormones, growth factors, neuropeptides and exosomes present in AH play a crucial role in the crosstalk between inflow and outflow tissues [68, 69]. In 2005, Hardy et al. proved that the release of MYOC from TM cells into its conditioned medium is possible via exosome-like vesicles. They explicated the mechanism by which tissue-specific localization and pathology of MYOC is achieved, and proposed that in most cells MYOC is an intracellular protein, but is released with exosomes by the TM [70]. A similar kind of study was carried out by the Perkumas group. The authors demonstrated that MYOC-associated exosomes are present in different human ocular samples such as effluent from the perfused human anterior segments and aqueous humor from live and cadaveric human eyes. They are also present in a conditioned medium from cultured human TM cells. This study revealed that exosomes may assist the transport of MYOC into the extracellular space of human ocular cells [45]. In another study, Lerner et al. demonstrated that non-pigmented ciliary epithelium (NPCE) derived exosomes can affect the canonical Wnt signaling pathway in TM cells through down regulation of expression of phospho-GSK3 and β-catenin expression [71]. These results are consistent with previous reports that canonical Wnt signaling is involved in IOP regulation and AH homeostasis [72]. Thus, NPCE-derived exosomes affecting Wnt signaling pathway molecules could be novel targets for glaucoma treatment. In addition, Dismuke et al. hypothesized that exosomes are part of AH and they primarily originate from NPCE cells. Furthermore, the authors revealed that the communication between inflow and outflow tissues homeostasis is mediated via exosomes released from AH and their cargo molecules chiefly via miRNAs. AH exosomal miRNAs such as miR-204 and miR-148a are shown to be involved in inflow and outflow facility regulation to maintain proper IOP homeostasis [68]. It is possible that in near future the exosome profile and their cargo in AH could be used as prognostic and diagnostic markers for ocular diseases including glaucoma [73, 74].
Fig. 3
Schematic representation of the role of exosomes in aqueous humor (AH) homeostasis under normal and disease conditions. Red arrows show the movement of exosomes from the ciliary body (CB -site of AH production) to trabecular meshwork (TM). The green arrow show the movement of exosomes from TM to CB. Based on the available literature, we speculate that exosomes control AH outflow and inflow regulation, maintain AH equilibrium and normal intraocular pressure (IOP). 1. Glaucoma: Myocilin-derived EVs (red circles) released from CB (yellow arrow) diffuse into the AH causing disrupted AH homeostasis blocking TM resulting in elevated IOP leads to impaired vision. 2. Age-related macular degeneration (AMD): In response to oxidative stress and autophagy retinal pigment epithelial (RPE) cells, the outer layer of retina cells release higher concentration of exosomes having elevated expression of VEGFR2 into the anterior chamber (pink arrow) induces new blood vessels formation and drusen leads to AMD condition. 3. Retinoblastoma (RB): Exosomes released from RB tumors enter into the systemic circulation. 4. Uveal melanoma (UM): Exosomes released from primary UM involve tumor invasion, migration, and formation of metastatic niche in liver, the most common metastatic site for UM. Exosomes and their molecular content released into the systemic circulation during the above disease conditions serve as disease signature markers and possess diverse diagnostic and prognostic potential.
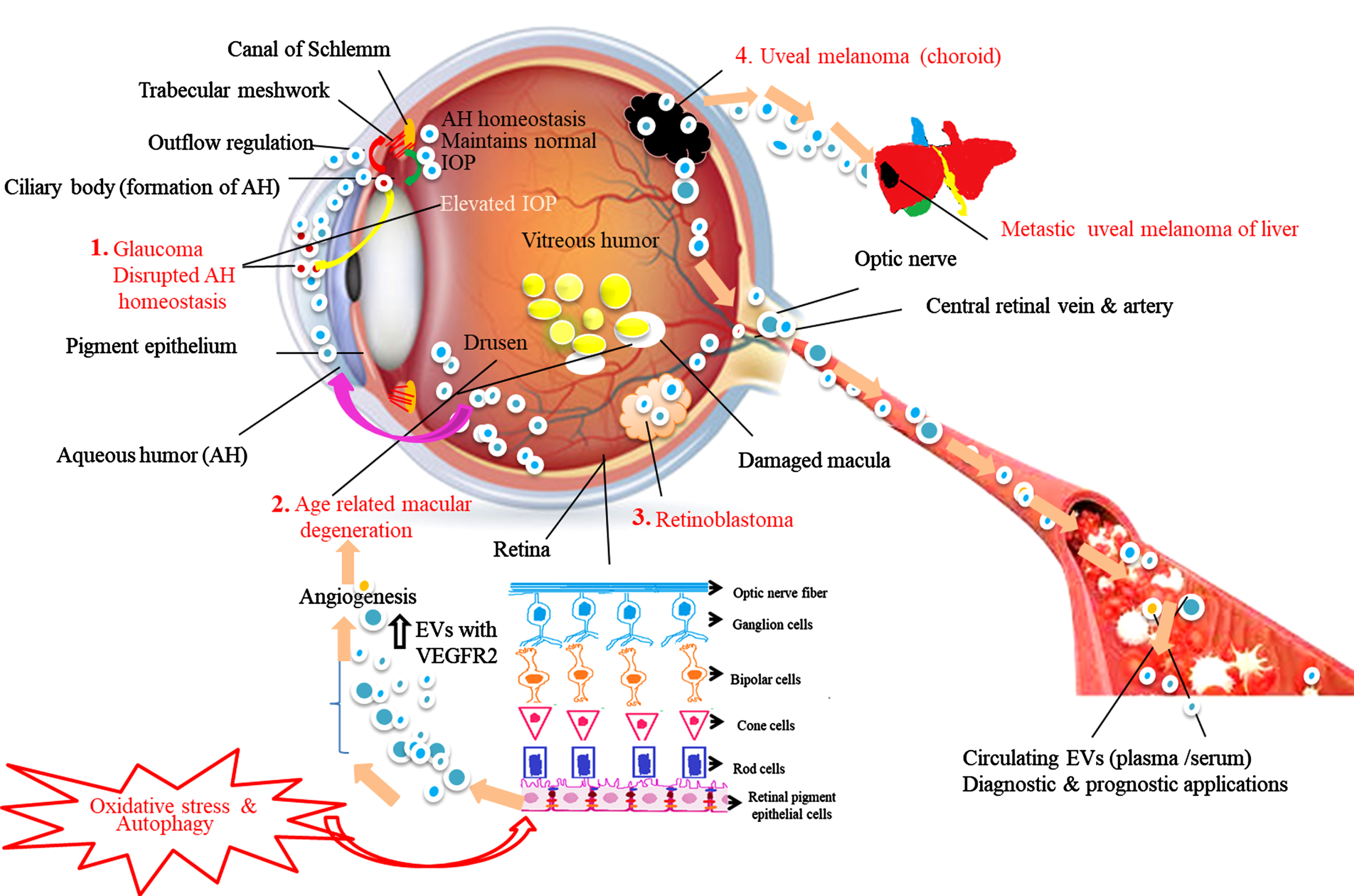
Recent animal studies have demonstrated the possible therapeutic applications of exosomes against glaucoma-related vision loss. Mead et al. compared the neuroprotective effect of BMC derived and fibroblast-derived small EVs having size within the range of exosomes (100–120 nm) among rats with ocular hypertension induced either by intracameral injection of microbeads or by laser photocoagulation of the TM and limbal vessels. The results show that injection of BMSC-derived small EVs into the vitreous provided significant therapeutic benefit to glaucomatous eyes possibly via their direct effect on RGC survival through miRNA-dependent mechanisms [75]. Similarly, the neuroprotective effects of BMSC small EVs were also reported in a chronic model of ocular hypertension [76]. Later, the same group demonstrated that BMSC small EVs can effectively elevate RGC survival in both rat retinal cultures and in human stem cell-derived RGC cultures. Their therapeutic efficacy can be elevated via TNFα priming possibly mediated through the increased levels of PEDF and VEGF-AA [77].
4.2.2Autoimmune uveitis (AU)
Inflammation of the uvea and surrounding tissues around it leads to AU. It is caused by either an abrupt immune response mediated by pathogenic Th17 cells or by the involvement of the innate inflammatory molecules in response to external stimulus [78, 79]. RPE cells are shown to have immunosuppressive properties and these cells are getting damaged in uveitis conditions. Previous studies have suggested that EVs secreted from RPE cells suppress the proliferation of T lymphocytes harvested from peripheral blood of noninfectious uveitis patients [80]. Exosomes from MSCs derived from different sources such as human bone marrow and human umbilical cord inhibit the experimental autoimmune uveoretinitis (EAU) by different anti-inflammatory effects, either by inhibiting T cell proliferation or by inhibiting inflammatory cell migration or by suppressing dendritic cell-driven Th17 responses [54, 81, 82]. The above results claim the therapeutic potential of exosomes against AU and future studies are warranted to explore their possible mechanism of action. Apart from in vitro studies and pre-clinical studies, a single clinical trial (NCT00331331) related to uveitis, vasculitis, and ocular inflammatory diseases was initiated in 2007 on patients aged above 18 years (n = 147) in order to evaluate the vitreous proteome and inflammatory mediators such as cytokines/lymphokines, biomarkers, proteome and exosomes present in the vitreous and anterior chamber. Vitreous specimens and corresponding blood samples were collected to compare inflammatory products present in both specimens. The study results of the same are awaited as per the latest update in April 2022 [83].
4.3Exosomes in the posterior segment
Along with the above-mentioned ocular components, exosomes are also identified in ocular fluids and are hypothesized to be serving as proximal biofluids of the retina. RPE is the outermost layer of the retina involved in maintaining retinal homeostasis. Different research groups revealed polarity-specific functions of exosomes in RPE cells and angiogenesis [84, 85]. The role of exosomes and their cargos in normal and disease conditions of the eye are summarized in Table 2.
4.3.1Exosomes in ocular involvement in systemic conditions: Diabetic retinopathy (DR)
DR is a group of retinal disorders caused by microvascular complications of diabetes and is the foremost reason for decreased vision and blindness worldwide [86]. Various studies have postulated that the complement system has a key role in the development of vascular damage and development of DR. Activation of the complement pathway and deregulation of complement regulatory proteins were noticed in the DR patient eyes and in vivo studies involving DR animal models [87, 88]. The pro-inflammatory changes breakdown the blood-retina barrier and initiates the pathogenesis of DR [89]. But, the exact molecular pathway responsible for the activation of the complement system and its involvement in DR is not clearly understood. Recent studies claimed that IgG-laden exosomes secreted into the plasma can activate the complement pathway, which in turn activates the inflammatory molecules leading to vascular damage. The number of exosomes is also found to be increased with diabetic conditions. Huang et al. demonstrated that a lack of IgG in exosomes in diabetic mice results in a reduction in retinal vascular damage and contributes to the development of DR [90]. In another study, miR-202-5p-containing exosomes in RPE cells (ARPE-19 cell line) are shown to regulate the TGFβ signaling pathway by targeting TGFβR2 and protecting against proliferative DR [91]. The therapeutic efficacy of rabbit adipose tissue-derived MSCs exosomes has been evaluated in retinal regeneration of experimentally induced diabetes mellitus. It was shown that the retinal repair capacity of MSC exosomes was mediated via their cargo molecules, particularly miR-222. These studies provide convincing evidence that exosomes containing miRNAs may provide a novel therapeutic approach to prevent or delay DR [92]. It would be important to understand the role of exosomes derived from the important target tissues like kidney, eye, peripheral nerves, etc. affected by diabetes, and how they influence the progress in this multi-systemic progressive disease. This would pave the way for identifying some prognostic indicators and possible therapeutic interventions in future.
4.3.2Age-related macular degeneration (AMD)
AMD is a multifactorial disease and was initially thought to be primarily a degenerative condition. Later, the role of the complement system of innate immunity has been recognized as a key regulator in AMD pathogenesis [93]. Numerous research groups have shown that low expression of membrane-bound complement molecules in RPE cells leads to RPE damage in AMD. These complement regulators are partially released via apoptotic bodies and exosomes which indicate that RPE cell-derived exosomes are one of the key regulators of complement-activated innate immune responses in AMD [94, 95]. Drusen formation is known to be one of the hallmarks of AMD. Wang et al. reported expression of exosomal proteins in AMD donor eyes. The authors suggested that drusen are formed by exosomes released from aged RPE cells. In response to the oxidative stress and autophagy, RPE cells release higher concentrations of exosomes with high expression of VEGFR2 resulting in the formation of new blood vessels [96]. The role of EVs in AMD pathology is illustrated in Fig. 3. In addition, a recent study by Elshelmani et al. provided evidence that serum-derived exosomal cargo (microRNAs (miRNAs) are involved in AMD disease pathobiology. The up-regulated miRNAs (miR-410, miR-126 and miR-19a) in AMD patients were further analyzed by bioinformatics approaches for identification of their target genes and signaling pathways. The results revealed that exosomal miRNAs are involved in apoptosis and angiogenesis. Specifically, miR-410 and miR-19-a are associated with the VEGF signaling pathway [97]. VEGF is already established to be a key factor in the development of choroidal neovascularization by increasing angiogenesis and vascular permeability [98]. Furthermore, Chen et al. demonstrated that miRNA-410 could reduce the expression levels of VEGF via interaction with the 3′UTR of the VEGFA mRNA in two weeks old mice. Similarly, eye drops having miRNA-410 effectively inhibited retinal angiogenesis and VEGF expression in oxygen-induced retinopathy mice model, suggesting that exosomal miRNA-410 could serve as an ideal therapeutic intervention for treating AMD [99]. Kang et al. in 2014 identified exosome proteins secreted by RPE cells in vivo as possible biomarkers and potential therapeutic targets for AMD treatment. These proteins are involved in the autophagy-lysosomal pathway and epithelial-mesenchymal transition (EMT) [73]. The above convincing results suggest that exosomes and their cargo molecules have potential therapeutic applications for treating AMD.
5Exosomes in ocular cancers
Emerging evidence from recent reports states that exosomes play a decisive role in the progression of ocular cancers. Tumor-derived exosomes, fibroblasts, and cancer-associated fibroblasts (CAFs) within the TM influence various cancer signaling pathways such as those linked to EMT, angiogenesis, and metastasis [100–102]. The presence of exosomes and their possible applications in diagnosis and prognosis in ocular cancers, such as uveal melanoma (UM) and retinoblastoma (RB), are well documented.
5.1Exosomes in UM
UM is the most common intraocular malignancy in adults that arises in the melanocytes of the eye. Most of the tumors develop at cutaneous localization (> 90%), and in the uveal tract [103]. Even after successful treatment for primary tumors, nearly one-third of patients develop metastases, primarily in the liver [104]. Eldh et al. isolated exosomes from metastatic UM and proved that miRNAs isolated from the liver originated from melanoma cells, and exosome concentration in the systemic circulation is higher in metastatic UM patients compared to healthy controls [105]. In a recent study, EVs derived from UM cell lines showed transforming and oncogenic potential in vivo, in NOD-SCID mice. Single oncogene mutated cells, human BRCA1-deficient fibroblasts (Fibro-BKO), were exposed to UM-EVs, and malignant transformation was observed in the target cells. After inoculation of these cells in SCID mice, they developed tumors of different sizes. Furthermore, histopathological evaluation of xenotransplants showed negative staining for vimentin. These results suggest that UM-EVs play a key role in target cell phenotypic switching. Proteomics profiling of UM-EVs confirmed their melanocytic origin and the majority of upregulated proteins (GNAQ, GNA11) are linked to UM tumorigenesis and integrin αV is related to liver metastasis. The proteomic profile of primary UM-EVs is distinct from that of metastaticUM-EVs. These results suggest that UM-EVs are involved in tumor cell proliferation, invasion, migration, and metastatic niche formation [106]. In another study, Ragusa et al. proposed that exosomal miRNA can be a circulating marker for UM, based on the results obtained from miRNA profiling in VH and serum from UM patients. They found that miR-146a levels were upregulated in serum, and also in formalin-fixed and paraffin-embedded UM tissues [107]. In UM patients, several other groups have also proposed tumor exosomes and their cargo (miRNA and proteins) as biomarkers for tumor progression and as potential therapeutic targets [100, 108, 109].
5.2Exosomes in RB
RB is a pediatric neuroectodermal malignancy originating in the retinal precursor cells owing to RB1 biallelic loss. Patients mostly present with leukocoria and strabismus. Eye salvage is possible for early diagnosed cases with a high survival rate ( 96%). However, enucleation and poor life expectancy is inevitable for advanced cases [110]. Regular tissue biopsy is not admissible for RB due to the risk of extraocular tumor spread and metastasis. Thus, contemporary research relies on liquid biopsies such as blood, as they are noninvasive, easy to extract, and known to be reservoirs of tumor signatures residing in circulating tumor cells and EVs. Several research groups have reported the involvement of EVs including exosomes in RB tumor progression by infiltrating the microenvironment [101]. Exosomal cargo (miRNA and proteins) is shown to be dysregulated in RB, and could serve as diagnostic, prognostic indicator, and provide potential therapeutic targets [111, 112]. In a recent study, Galardi et al. recorded the proteomic profile of exosomes released from primary RB tumors as well as vitreous seeding cell lines to find new putative biomarkers and potential therapeutic targets associated with vitreous seeding in RB. The upregulated proteins, such as TLN, ß3 integrin, and GSTM1, have been implicated in extracellular matrix (ECM) remodeling, glucose and amino acid metabolism, and associated with invasion and metastasis, maintenance of stem cell phenotype, and chemoresistance [113]. The presence of exosomal miRNAs from RB primary tissue samples was analyzed in the corresponding serum samples by Ravishankar et al. But, none of the tested miRNAs which are expressed in RB cell lines and primary RB tissue samples were expressed in serum. Probably, serum exosomal miRNAs are not ideal prognostic factors for RB, rather exosomal miRNAs derived from other ocular fluids such as AH and VH may serve the purpose [114]. Various clinical trials have been initiated for investigating exosomes as diagnostic and prognostic biomarkers for non-ocular cancers. Clinical trials’ results on prostate cancer (NCT02702856, NCT03031418, NCT03235687, NCT04720599) revealed that ExoDx Prostate IntelliScore (EPI), the urine exosome RNA-based test for risk assessment of high-grade prostate cancer, outperformed the multivariate risk calculators in a homogenous risk group of pre-biopsy men. The EPI score was associated with low-risk pathology post-RP and may guide to take active surveillance decisions [115]. Trials on pancreatic ductal adenocarcinoma PDAC (NCT03032913), gastric (NCT01779583), oropharyngeal (NCT02147418), lung (NCT05058768), sarcoma (NCT03800121), and ovarian cancers (NCT03738319) have also been initiated [116–121]. However, to the best of our knowledge, there is no trial registered for testing exosomes as diagnostic markers for ocular cancers. As exosomal research in ocular cancers is still emerging and due to their presence in normal ocular tissues, such as corneal epithelium, stromal cells, TM cells, LG, and CB. The role of these small EVs in other ocular cancers may be the focus of research in the near future.
6Summary
Exosomes are the smallest vesicles having multiple roles in intercellular communication, immune response, and tumor-associated pathways. They have a dual role as tumor progression mediators and tumor antagonist factors. Over the years, numerous exosome isolation and characterization methods have developed, which poses a challenge in comparing the data and drawing parallels. Exosome samples are often contaminated with other EVs as separation techniques rely on vesicle size and cell surface marker expression. MVs and exosomes share common cell surface markers and have overlapping cell sizes, which leads to ambiguity in the literature regarding nomenclature. Hence there is a dire need to harmonize the tools for isolation and characterization of EVs and their naming. It is even more relevant to the ocular tissue as the available tissue samples from the eye are very small and rare. Hence samples obtained by noninvasive or minimally invasive methods to diagnose, prognosticate or provide targeted therapy would be of immense value. Evaluation of exosomal cargo and their biology will aid in understanding the pathogenesis of ocular diseases such as AMD, glaucoma to restore vision by improving antiangiogenic therapies. Similarly, in ocular tumors, understanding the interactions between tumor-derived exosomes with cells of the tumor microenvironment and cells of metastatic sites would aid in unraveling the molecular mechanisms responsible for tumor development, angiogenesis, metastasis, and invasion. The cumulative knowledge obtained from the exosomal cargo would pave the way for the identification of new biomarkers and also in the development of novel therapeutics that exclusively target tumor-associated pathways.
6.1Viewpoints
We strongly believe that the exosomal profile in health and disease can pave the way for a better understanding of the disease process and prognosis. It also provides an opportunity for intervention at various stages of the disease process. Hence, it is necessary to analyze exosomes profiles for each disease and should in due course lead to being a part of clinical evaluation and armamentarium of molecular diagnosis. Given the ease with which the mobility of the exosomes is facilitated, especially for natural barriers, it would serve as an excellent choice for drug delivery and provides a ray of hope for planning personalized treatment for patients with ocular diseases.
While the prospects seem evident, the challenges are also well identified. Despite the emergence of new technology, there is a dire need to develop an ideal method of exosome isolation and characterization to understand the specific signatures within the cargo of individual cells and organs in both health and disease. Since these signatures influence various pathological processes such as angiogenesis, inflammation, immune regulation, AMD, DR, and ocular cancers, the point of potential intervention could be identified.
The knowledge on exosomes and integration of evidence obtained from various systems we believe would contribute to a better understanding of disease processes, especially in the emerging subspecialties of ophthalmic research.
Acknowledgments
The authors acknowledge all the team members of stem cells and cancer biology research laboratory, school of medical sciences, University of Hyderabad for their constant support during the preparation of this manuscript.
Author contributions
CONCEPTION: Radhika Manukonda, Jyothi Attem, Vengala Rao Yenuganti, Swathi Kaliki and Geeta K Vemuganti
DATA CURATION: Radhika Manukonda, Jyothi Attem, Vengala Rao Yenuganti, Swathi Kaliki and Geeta K Vemuganti
ANALYSIS OF DATA: Radhika Manukonda, Jyothi Attem, Vengala Rao Yenuganti, Swathi Kaliki and Geeta K Vemuganti
PREPARATION OF THE MANUSCRIPT: Radhika Manukonda, Jyothi Attem, Vengala Rao Yenuganti and Swathi Kaliki
REVISION FOR IMPORTANT INTELLECTUAL CONTENT: Radhika Manukonda, Jyothi Attem, Vengala Rao Yenuganti, Swathi Kaliki and Geeta K Vemuganti
SUPERVISION: Geeta K Vemuganti
Conflict of interest
The authors declare no potential conflicts of interest with respect to the research, authorship, and/or publication of this review article.
Funding
The authors disclose receipt of the following financial support for the research, authorship, and/or publication of this article: This study was supported by UGC-UKIERI (Thematic Partnership 2017–2020), Hyderabad Eye Research Foundation, LV Prasad Eye Institute, Science and Engineering Research Board (SERB-DST), Academic research grants from the DST-PURSE (University of Hyderabad) and UPE-II (University of Hyderabad). R.M. is supported by the UGC-UKIERI grant & HERF, LVPEI, JA is supported by the UGC, Government of India (Junior Research Fellowship), and V.R.Y is supported by DST inspire fellowship. The funding body had no role in the design of the study, collection, analysis, and interpretation of data and in writing the manuscript.
References
[1] | Wolf P . The nature and significance of platelet products in human plasma. Br J Haematol. (1967) ;13: (3):269–88. doi: 10.1111/j.1365-2141.1967.tb08741.x |
[2] | Rak J , editor. Microparticles in cancer. Semin Thromb Hemost. (2010) ;36: (8):888–906. doi: 10.1055/s-0030-1267043 |
[3] | Hood JL , San RS , Wickline SA . Exosomes released by melanoma cells prepare sentinel lymph nodes for tumor metastasis. Cancer Res. (2011) ;71: (11):3792–801. doi: 10.1158/0008-5472.CAN-10-4455 |
[4] | Shin S , Han D , Park MC , Mun JY , Choi J , Chun H , et al. Separation of extracellular nanovesicles and apoptotic bodies from cancer cell culture broth using tunable microfluidic systems. Sci Rep. (2017) ;7: (1):1–8. doi: 10.1038/s41598-017-08826-w |
[5] | Dignat-George F , Boulanger CM . The many faces of endothelial microparticles. Arterioscler Thromb Vasc Biol. (2011) ;31: (1):27–33. doi: 10.1161/ATVBAHA.110.218123 |
[6] | Théry C , Zitvogel L , Amigorena S . Exosomes: composition, biogenesis and function. Nat Rev Immunol. (2002) ;2: (8):569–79. doi: 10.1038/nri855 |
[7] | Zaborowski MP , Balaj L , Breakefield XO , Lai CP . Extracellular vesicles: composition, biological relevance, and methods of study. Bioscience. (2015) ;65: (8):783–97. doi: 10.1093/biosci/biv084 |
[8] | Doyle LM , Wang MZ . Overview of extracellular vesicles, their origin, composition, purpose, and methods for exosome isolation and analysis. Cells. (2019) ;8: (7):727. doi: 10.3390/cells8070727 |
[9] | Kalra H , Drummen GP , Mathivanan S . Focus on extracellular vesicles: introducing the next small big thing. Int J Mol Sci. (2016) ;17: (2):170. doi: 10.3390/ijms17020170 |
[10] | Gheinani AH , Vögeli M , Baumgartner U , Vassella E , Draeger A , Burkhard FC , et al. Improved isolation strategies to increase the yield and purity of human urinary exosomes for biomarker discovery. Sci Rep. (2018) ;8: :3945. doi: 10.1038/s41598-018-22142-x |
[11] | Chernyshev VS , Rachamadugu R , Tseng YH , Belnap DM , Jia Y , Branch KJ , et al. Size and shape characterization of hydrated and desiccated exosomes. Anal Bioanal Chem. (2015) ;407: (12):3285–301. doi: 10.1007/s00216-015-8535-3 |
[12] | Witwer KW , Théry C . Extracellular vesicles or exosomes? On primacy, precision, and popularity influencing a choice of nomenclature. J Extracell Vesicles. (2019) ;8: (1):1648167. doi: 10.1080/20013078.2019.1648167 |
[13] | Théry C , Witwer KW , Aikawa E , Alcaraz MJ , Anderson JD , Andriantsitohaina R , et al. Minimal information for studies of extracellular vesicles 2018 (MISEV2018): a position statement of the International Society for Extracellular Vesicles and update of the MISEV2014 guidelines. J Extracell Vesicles. (2018) ;7: (1):1535750. doi: 10.1080/20013078.2018.1535750 |
[14] | Patel GK , Khan MA , Zubair H , Srivastava SK , Khushman M , Singh S , et al. Comparative analysis of exosome isolation methods using culture supernatant for optimum yield, purity and downstream applications. Sci Rep. (2019) ;9: (1):1–10. doi: 10.1038/s41598-019-41800-2 |
[15] | Ludwig N , Whiteside TL , Reichert TE . Challenges in exosome isolation and analysis in health and disease. Int J Mol Sci. (2019) ;20: (19):4684. doi: 10.3390/ijms20194684 |
[16] | Gardiner C , Vizio DD , Sahoo S , Théry C , Witwer KW , Wauben M , Hill AF . Techniques used for the isolation and characterization of extracellular vesicles: results of a worldwide survey. J Extracell Vesicles. (2016) ;5: (1):32945. doi: 10.3402/jev.v5.32945 |
[17] | Klingeborn M , Stamer WD , Bowes Rickman C . Polarized exosome release from the retinal pigmented epithelium. Adv Exp Med Biol. (2018) :539–44. doi: 10.1007/978-3-319-75402-4_65 |
[18] | Pan B-T , Johnstone RM . Fate of the transferrin receptor during maturation of sheep reticulocytes in vitro: selective externalization of the receptor. Cell. (1983) ;33: (3):967–78. doi: 10.1016/0092-8674(83)90040-5 |
[19] | Harding C , Heuser J , Stahl P . Receptor-mediated endocytosis of transferrin and recycling of the transferrin receptor in rat reticulocytes. J Cell Biol. (1983) ;97: (2):329–39. doi: 10.1083/jcb.97.2.329 |
[20] | Johnstone RM , Adam M , Hammond J , Orr L , Turbide C . Vesicle formation during reticulocyte maturation. Association of plasma membrane activities with released vesicles (exosomes). J Biol Chem. (1987) ;262: (19):9412–20. doi: 10.1016/S0021-9258(18)48095-7 |
[21] | Potolicchio I , Carven GJ , Xu X , Stipp C , Riese RJ , Stern LJ , et al. Proteomic analysis of microglia-derived exosomes: metabolic role of the aminopeptidase CD13 in neuropeptide catabolism. J Immunol. (2005) ;175: (4):2237–43. doi: 10.4049/jimmunol.175.4.2237 |
[22] | Fauré J , Lachenal G , Court M , Hirrlinger J , Chatellard-Causse C , Blot B , et al. Exosomes are released by cultured cortical neurones. Mol Cell Neurosci. (2006) ;31: (4):642–8. doi: 10.1016/j.mcn.2005.12.003 |
[23] | Segura E , Théry C . Exosomes: naturally occurring minimal antigen-presenting units. Allergy frontiers: future perspectives. (2010) :305–19. doi: 10.1007/978-4-431-99365-0_20 |
[24] | Raposo G , Nijman HW , Stoorvogel W , Liejendekker R , Harding CV , Melief C , et al. B lymphocytes secrete antigen-presenting vesicles. J Exp Med. (1996) ;183: (3):1161–72. doi: 10.1084/jem.183.3.1161 |
[25] | Keller S , Ridinger J , Rupp A-K , Janssen JW , Altevogt P . Body fluid derived exosomes as a novel template for clinical diagnostics. J Transl Med. (2011) ;9: (1):1–9. doi: 10.1186/1479-5876-9-86 |
[26] | Lässer C , Seyed Alikhani V , Ekström K , Eldh M , Torregrosa Paredes P , Bossios A , et al. Human saliva, plasma and breast milk exosomes contain RNA: uptake by macrophages. J Transl Med. (2011) ;9: (1):1–8. doi: 10.1186/1479-5876-9-9 |
[27] | Admyre C , Grunewald J , Thyberg J , Gripenbäck S , Tornling G , Eklund A , et al. Exosomes with major histocompatibility complex class II and co-stimulatory molecules are present in human BAL fluid. Eur Respir J. (2003) ;22: (4):578–83. doi: 10.1183/09031936.03.00041703 |
[28] | Gatti J-L , Métayer S , Belghazi M , Dacheux F , Dacheux J-L . Identification, proteomic profiling, and origin of ram epididymal fluid exosome-like vesicles. Biol Reprod. (2005) ;72: (6):1452–65. doi: 10.1095/biolreprod.104.036426 |
[29] | Harrell CR , Simovic Markovic B , Fellabaum C , Arsenijevic A , Djonov V , Arsenijevic N , et al. Therapeutic potential of mesenchymal stem cell-derived exosomes in the treatment of eye diseases. Adv Exp Med Biol. (2018) ;1089: :47–57. doi: 10.1007/5584_2018_219 |
[30] | Wang Y , Zhang L , Li Y , Chen L , Wang X , Guo W , et al. Exosomes/microvesicles from induced pluripotent stem cells deliver cardioprotective miRNAs and prevent cardiomyocyte apoptosis in the ischemic myocardium. Int J Cardiol. (2015) ;192: :61–9. doi: 10.1016/j.ijcard.2015.05.020 |
[31] | Vrijsen KR , Maring JA , Chamuleau SA , Verhage V , Mol EA , Deddens JC , et al. Exosomes from cardiomyocyte progenitor cells and mesenchymal stem cells stimulate angiogenesis via EMMPRIN. Adv Healthc Mater. (2016) ;5: (19):2555–65. doi: 10.1002/adhm.201600308 |
[32] | Nakano M , Nagaishi K , Konari N , Saito Y , Chikenji T , Mizue Y , et al. Bone marrow-derived mesenchymal stem cells improve diabetes-induced cognitive impairment by exosome transfer into damaged neurons and astrocytes. Sci Rep. (2016) ;6: (1):1–14. doi: 10.1038/srep24805 |
[33] | Mead B , Tomarev S . Bone marrow-derived mesenchymal stem cells-derived exosomes promote survival of retinal ganglion cells through miRNA-dependent mechanisms. Stem Cells Transl Med. (2017) ;6: (4):1273–85. doi: 10.1002/sctm.16-0428 |
[34] | Tschuschke M , Kocherova I , Bryja A , Mozdziak P , Angelova Volponi A , Janowicz K , et al. Inclusion biogenesis, methods of isolation and clinical application of human cellular exosomes. J Clin Med. (2020) ;9: (2):436. doi: 10.3390/jcm9020436 |
[35] | Boukouris S , Mathivanan S . Exosomes in bodily fluids are a highly stable resource of disease biomarkers. Proteomics Clin Appl. (2015) ;9: (3-4):358–67. doi: 10.1002/prca.201400114 |
[36] | Muzykantov VR . Drug delivery by red blood cells: vascular carriers designed by mother nature. Expert Opin Drug Deliv. (2010) ;7: (4):403–27. doi: 10.1517/17425241003610633 |
[37] | Mead B , Tomarev S . Extracellular vesicle therapy for retinal diseases. Prog Retin Eye Res. (2020) ;79: :100849. doi: 10.1016/j.preteyeres.2020.100849 |
[38] | Yu B , Shao H , Su C , Jiang Y , Chen X , Bai L , et al. Exosomes derived from MSCs ameliorate retinal laser injury partially by inhibition of MCP-1. Sci Rep. (2016) ;6: (1):1–12. doi: 10.1038/srep34562 |
[39] | Han K-Y , Tran JA , Chang J-H , Azar DT , Zieske JD . Potential role of corneal epithelial cell-derived exosomes in corneal wound healing and neovascularization. Sci Rep. (2017) ;7: (1):1–14. doi: 10.1038/srep40548 |
[40] | Samaeekia R , Rabiee B , Putra I , Shen X , Park YJ , Hematti P , et al. Effect of human corneal mesenchymal stromal cell-derived exosomes on corneal epithelial wound healing. Invest Ophthalmol Vis Sci. (2018) ;59: (12):5194–200. doi: 10.1167/iovs.18-24803 |
[41] | Tao H , Chen X , Cao H , Zheng L , Li Q , Zhang K , et al. Mesenchymal stem cell-derived extracellular vesicles for corneal wound repair. Stem Cells Int. (2019) ;2019: :5738510. doi: 10.1155/2019/5738510 |
[42] | Batrakova EV , Kim MS . Using exosomes, naturally-equipped nanocarriers, for drug delivery. J Control Release. (2015) ;219: :396–405. doi: 10.1016/j.jconrel.2015.07.030 |
[43] | Morishita M , Takahashi Y , Matsumoto A , Nishikawa M , Takakura Y . Exosome-based tumor antigens–adjuvant co-delivery utilizing genetically engineered tumor cell-derived exosomes with immunostimulatory CpG DNA. Biomaterials. (2016) ;111: :55–65. doi: 10.1016/j.biomaterials.2016.09.031 |
[44] | Yang X-X , Sun C , Wang L , Guo X-L . New insight into isolation, identification techniques and medical applications of exosomes. J Control Release. (2019) ;308: :119–29. doi: 10.1016/j.jconrel.2019.07.021 |
[45] | Perkumas K , Hoffman E , McKay B , Allingham RR , Stamer W . Myocilin-associated exosomes in human ocular samples. Exp Eye Res. (2007) ;84: (1):209–12. doi: 10.1016/j.exer.2006.09.020 |
[46] | Klingeborn M , Dismuke WM , Rickman CB , Stamer WD . Roles of exosomes in the normal and diseased eye. (2017) ;59: :158–77. doi: 10.1016/j.preteyeres.2017.04.004 |
[47] | Zhao Y , Weber SR , Lease J , Russo M , Siedlecki CA , Xu L-C , et al. Liquid biopsy of vitreous reveals an abundant vesicle population consistent with the size and morphology of exosomes. Transl Vis Sci Technol. (2018) ;7: (3):6. doi: 10.1167/tvst.7.3.6 |
[48] | Leszczynska A , Kulkarni M , Ljubimov AV , Saghizadeh M . Exosomes from normal and diabetic human corneolimbal keratocytes differentially regulate migration, proliferation and marker expression of limbal epithelial cells. Sci Rep. (2018) ;8: (1):1–13. doi: 10.1038/s41598-018-33169-5 |
[49] | Grigor’Eva A , Tamkovich S , Eremina A , Tupikin A , Kabilov M , Chernykh V , et al. Exosomes in tears of healthy individuals: Isolation, identification, and characterization. Biochem Moscow Suppl Ser B. (2016) ;10: :165–72. doi: 10.1134/S1990750816020049 |
[50] | Aass C , Norheim I , Eriksen EF , Thorsby PM , Pepaj M . Single unit filter-aided method for fast proteomic analysis of tear fluid. Anal Biochem. (2015) ;480: :1–5. doi: 10.1016/j.ab.2015.04.002 |
[51] | Matheis N , Lantz M , Grus F , Ponto K , Wolters D , Brorson H , et al. Proteomics of orbital tissue in thyroid-associated orbitopathy. J Clin Endocrinol Metab. (2015) ;100: (12):E1523–E30. doi: 10.1210/jc.2015-2976 |
[52] | Han J-S , Kim SE , Jin J-Q , Park NR , Lee J-Y , Kim HL , et al. Tear-derived exosome proteins are increased in patients with thyroid eye disease. Int. J. Mol. Sci. (2021) ;22: (3):1115. doi: 10.3390/ijms22031115 |
[53] | Gurnani B , Czyz CN , Mahabadi N , et al. Corneal Graft Rejection. [Updated 2022 Feb 22]. In: StatPearls [Internet]. Treasure Island (FL): StatPearls Publishing; 2022. https://www.ncbi.nlm.nih.gov/books/NBK519043/ |
[54] | Li N , Zhao L , Wei Y , Ea VL , Nian H , Wei R . Recent advances of exosomes in immune-mediated eye diseases. Stem Cell Res Ther. (2019) ;10: (1):1–0. doi: 10.1186/s13287-019-1372-0 |
[55] | Dua HS , Azuara-Blanco A . Corneal allograft rejection: risk factors, diagnosis, prevention, and treatment. Indian J Ophthalmol. (1999) ;47: (1):3. |
[56] | Gonzalez-Nolasco B , Wang M , Prunevieille A , Benichou G . Emerging role of exosomes in allorecognition and allograft rejection. Curr Opin Organ Transplant. (2018) ;23: (1):22. doi: 10.1097/MOT.0000000000000489 |
[57] | Aiello S , Rocchetta F , Longaretti L , Faravelli S , Todeschini M , Cassis L , et al. Extracellular vesicles derived from T regulatory cells suppress T cell proliferation and prolong allograft survival. Sci Rep. (2017) ;7: (1):1–19. doi: 10.1038/s41598-017-08617-3 |
[58] | Wen D , Peng Y , Liu D , Weizmann Y , Mahato RI . Mesenchymal stem cell and derived exosome as small RNA carrier and Immunomodulator to improve islet transplantation. J Control Release. (2016) ;238: :166–75. doi: 10.1016/j.jconrel.2016.07.044 |
[59] | López-Miguel A , Tesón M , Martín-Montañez V , Enríquez-de-Salamanca A , Stern ME , González-García MJ , Calonge M . Clinical and Molecular Inflammatory Response in Sjögren Syndrome–Associated Dry Eye Patients Under Desiccating Stress. Am J Ophthalmol. (2016) ;161: :133–41. doi: 10.1016/j.ajo.2015.09.039 |
[60] | Sandhya P , Theyilamannil Kurien B , Danda D , Hal Scofield R . Update on pathogenesis of Sjogren’s syndrome. Curr Rheumatol Rev. (2017) ;13: (1):5–22. doi: 10.2174/1573397112666160714164149 |
[61] | Zhang X , Jeyalatha MV , Qu Y , He X , Ou S , Bu J , et al. Dry eye management: targeting the ocular surface microenvironment. Int J Mol Sci. (2017) ;18: (7):1398. doi: 10.3390/ijms18071398 |
[62] | Mansoor H , Ong HS , Riau AK , Stanzel TP , Mehta JS , Yam GH-F . Current trends and future perspective of mesenchymal stem cells and exosomes in corneal diseases. Int J Mol Sci. (2019) ;20: (12):2853. doi: 10.3390/ijms20122853 |
[63] | Hou A , Law KP , Tin MQ , Lim YP , Tong L . In vitro secretomics study of pterygium-derived fibroblasts by iTRAQ-based quantitative proteomics strategy. Exp Eye Res. (2016) ;153: :14. doi: 10.1016/j.exer.2016.10.006 |
[64] | Weng J , He C , Lai P , Luo C , Guo R , Wu S , et al. Mesenchymal stromal cells treatment attenuates dry eye in patients with chronic graft-versus-host disease. Mol Ther. (2012) ;20: (12):2347–54. doi: 10.1038/mt.2012.208 |
[65] | Effect of UMSCs Derived Exosomes on Dry Eye in Patients With cGVHD. Zhongshan Ophthalmic Center, Sun Yat-sen University. NCT04213248. https://clinicaltrials.gov/ct2/show/NCT04213248 |
[66] | Williams KA , Coster DJ . Rethinking immunological privilege: implications for corneal and limbal stem cell transplantation. Mol Med Today. (1997) ;3: (11):495–501. doi: 10.1016/S1357-4310(97)01122-2 |
[67] | Ren K . Exosomes in perspective: a potential surrogate for stem cell therapy. Odontology. (2019) ;107: (3):271–84. doi: 10.1007/s10266-018-0395-9 |
[68] | Dismuke WM , Challa P , Navarro I , Stamer WD , Liu Y . Human aqueous humor exosomes. Exp Eye Res. (2015) ;132: :73–7. doi: 10.1016/j.exer.2015.01.019 |
[69] | Hoffman EA , Perkumas KM , Highstrom LM , Stamer WD . Regulation of myocilin-associated exosome release from human trabecular meshwork cells. Invest Ophthalmol Vis Sci. (2009) ;50: (3):1313–8. doi: 10.1167/iovs.08-2326 |
[70] | Hardy KM , Hoffman EA , Gonzalez P , McKay BS , Stamer WD . Extracellular trafficking of myocilin in human trabecular meshwork cells. J Biol Chem. (2005) ;280: (32):28917–26. doi: 10.1074/jbc.M504803200 |
[71] | Lerner N , Avissar S , Beit-Yannai E . Extracellular vesicles mediate signaling between the aqueous humor producing and draining cells in the ocular system. PLoS One. (2017) ;12: (2):e0171153. doi: 10.1371/journal.pone.0171153 |
[72] | Mao W , Millar JC , Wang W-H , Silverman SM , Liu Y , Wordinger RJ , et al. Existence of the canonical Wnt signaling pathway in the human trabecular meshwork. Invest Ophthalmol Vis Sci. (2012) ;53: (11):7043–51. doi: 10.1167/iovs.12-9664 |
[73] | Kang GY , Bang JY , Choi AJ , Yoon J , Lee WC , Choi S , Yoon S , Kim HC , Baek JH , Park HS , Lim HJ . Exosomal proteins in the aqueous humor as novel biomarkers in patients with neovascular age-related macular degeneration. Journal of Pproteome Rresearch. J Proteome Res. (2014) ;13: (2):581–95. doi: 10.1021/pr400751k |
[74] | Liao Z , Lou Z , Xiao Z . Isolation of rabbit aqueous humor-derived exosomes and their immunosuppression function. Journal of Zhejiang University Medical Sciences. (2012) ;41: (3):315–9. Chinese. |
[75] | Mead B , Amaral J , Tomarev S . Mesenchymal stem cell–derived small extracellular vesicles promote neuroprotection in rodent models of glaucoma. Invest Ophthalmol Vis Sci. (2018) ;59: (2):702–14. doi: 10.1167/iovs.17-22855 |
[76] | Mead B , Ahmed Z , Tomarev S . Mesenchymal stem cell–derived small extracellular vesicles promote neuroprotection in a genetic DBA/2J mouse model of glaucoma. Invest Oph Invest Ophthalmol Vis Sci. (2018) ;59: (13):5473–80. doi: 10.1167/iovs.18-25310 |
[77] | Mead B , Chamling X , Zack DJ , Ahmed Z , Tomarev S . TNFα-mediated priming of mesenchymal stem cells enhances their neuroprotective effect on retinal ganglion cells. Invest Ophthalmol Vis Sci. (2020) ;61: (2):6. doi: 10.1167/iovs.61.2.6 |
[78] | Pepple KL , Lin P . Targeting interleukin-23 in the treatment of noninfectious uveitis. Ophthalmology. (2018) ;125: (12):1977–83. doi: 10.1016/j.ophtha.2018.05.014 |
[79] | Caspi RR . A look at autoimmunity and inflammation in the eye. J Clin Invest. (2010) ;120: (9):3073–83. doi: 10.1172/JCI42440 |
[80] | Knickelbein JE , Liu B , Arakelyan A , Zicari S , Hannes S , Chen P , et al. Modulation of immune responses by extracellular vesicles from retinal pigment epithelium. Invest Oph Invest Ophthalmol Vis Sci. (2016) ;57: (10):4101–7. doi: 10.1167/iovs.15-18353 |
[81] | Shigemoto-Kuroda T , Oh JY , Kim D-K , Jeong HJ , Park SY , Lee HJ , et al. MSC-derived extracellular vesicles attenuate immune responses in two autoimmune murine models: type 1 diabetes and uveoretinitis. Stem Cell Reports. (2017) ;8: (5):1214–25. doi: 10.1016/j.stemcr.2017.04.008 |
[82] | Bai L , Shao H , Wang H , Zhang Z , Su C , Dong L , et al. Effects of mesenchymal stem cell-derived exosomes on experimental autoimmune uveitis. Sci Rep. (2017) ;7: (1):1–11. doi: 10.1038/s41598-017-04559-y |
[83] | Kodati Shilpa M . The Vitreous Proteome and Inflammatory Mediators in Ocular Inflammatory Disease. National Eye Institute, Bethesda, Maryland. NCT00331331 (last updated June 2nd 2022). https://clinicaltrials.gov/ct2/show/NCT00331331 |
[84] | Klingeborn M , Dismuke WM , Skiba NP , Kelly U , Stamer WD , Bowes Rickman C . Directional exosome proteomes reflect polarity-specific functions in retinal pigmented epithelium monolayers. Sci Rep. (2017) ;7: (1):1–16. doi: 10.1038/s41598-017-05102-9 |
[85] | Atienzar-Aroca S , Serrano-Heras G , Freire Valls A , Ruiz de Almodovar C , Muriach M , Barcia JM , et al. Role of retinal pigment epithelium-derived exosomes and autophagy in new blood vessel formation. J Cell Mol Med. (2018) ;22: (11):5244–56. doi: 10.1111/jcmm.13730 |
[86] | Stitt AW , Curtis TM , Chen M , Medina RJ , McKay GJ , Jenkins A , et al. The progress in understanding and treatment of diabetic retinopathy. Prog Retin Eye Res. (2016) ;51: :156. doi: 10.1016/j.preteyeres.2015.08.001 |
[87] | S Bora N , Jha P , V Lyzogubov V , S Bora P . Emerging role of complement in ocular diseases. Current Immunology Reviews. (2011) ;7: (3):360–7. doi: 10.2174/157339511796196656 |
[88] | Bora NS , Jha P , Bora PS . The role of complement in ocular pathology. Semin Immunopathol. (2008) ;30: (2):85–95. doi: 10.1007/s00281-008-0110-y |
[89] | Joussen AM , Murata T , Tsujikawa A , Kirchhof B , Bursell S-E , Adamis AP . Leukocyte-mediated endothelial cell injury and death in the diabetic retina. Am J Pathol. (2001) ;158: (1):147–52. doi: 10.1016/S0002-9440(10)63952-1 |
[90] | Huang C , Fisher KP , Hammer SS , Navitskaya S , Blanchard GJ , Busik JV . Plasma exosomes contribute to microvascular damage in diabetic retinopathy by activating the classical complement pathway. Diabetes. (2018) ;67: (8):1639–49. doi: 10.2337/db17-1587 |
[91] | Gu S , Liu Y , Zou J , Wang W , Wei T , Wang X , et al. Retinal pigment epithelial cells secrete miR-202-5p-containing exosomes to protect against proliferative diabetic retinopathy. Exp Eye Res. (2020) ;201: :108271. doi: 10.1016/j.exer.2020.108271 |
[92] | Safwat A , Sabry D , Ragiae A , Amer E , Mahmoud RH , Shamardan RM . Adipose mesenchymal stem cells–derived exosomes attenuate retina degeneration of streptozotocin-induced diabetes in rabbits. J Circ Biomark. (2018) ;7: :1849454418807827. doi: 10.1177/1849454418807827 |
[93] | Bora NS , Matta B , Lyzogubov VV , Bora PS . Relationship between the complement system, risk factors and prediction models in age-related macular degeneration. Mol Immunol. (2015) ;63: (2):176–83. doi: 10.1016/j.molimm.2014.07.012 |
[94] | Shah N , Ishii M , Brandon C , Ablonczy Z , Cai J , Liu Y , et al. Extracellular vesicle-mediated long-range communication in stressed retinal pigment epithelial cell monolayers. Biochim. et Biophys. Acta (BBA)-Molecular Basis of Disease. (2018) ;1864: (8):2610–22. doi: 10.1016/j.bbadis.2018.04.016 |
[95] | Ebrahimi KB , Fijalkowski N , Cano M , Handa JT . Oxidized low-density-lipoprotein-induced injury in retinal pigment epithelium alters expression of the membrane complement regulatory factors CD46 and CD59 through exosomal and apoptotic bleb release. Retinal Degenerative Diseases: Springer. (2014) . pp. 259–65. doi: 10.1007/978-1-4614-3209-8_33 |
[96] | Wang AL , Lukas TJ , Yuan M , Du N , Tso MO , Neufeld AH . Autophagy and exosomes in the aged retinal pigment epithelium: possible relevance to drusen formation and age-related macular degeneration. PloS one.e. (2009) ;4: (1):e4160. doi: 10.1371/journal.pone.0004160 |
[97] | ElShelmani H , Wride MA , Saad T , Rani S , Kelly DJ , Keegan D . The role of deregulated microRNAs in age-related macular degeneration pathology. Transl Vis Sci Technol. (2021) ;10: (2):12. doi: 10.1167/tvst.10.2.12 |
[98] | Penn J , Madan A , Caldwell RB , Bartoli M , Caldwell R , Hartnett M . Vascular endothelial growth factor in eye disease. Prog Retin Eye Res. (2008) ;27: (4):331–71. doi: 10.1016/j.preteyeres.2008.05.001 |
[99] | Chen N , Wang J , Hu Y , Cui B , Li W , Xu G , et al. MicroRNA-410 reduces the expression of vascular endothelial growth factor and inhibits oxygen-induced retinal neovascularization. PLoS One. (2014) ;9: (4):e95665. doi: 10.1371/journal.pone.0095665 |
[100] | Angi M , Kalirai H , Prendergast S , Simpson D , Hammond DE , Madigan MC , et al. In-depth proteomic profiling of the uveal melanoma secretome. Oncotarget. (2016) ;7: (31):49623. doi: 10.18632/oncotarget.10418 |
[101] | Chen S , Chen X , Qiu J , Chen P , Han X , Wu Y , et al. Exosomes derived from retinoblastoma cells enhance tumour deterioration by infiltrating the microenvironment. Oncol Rep. (2021) ;45: (1):278–90. doi: 10.3892/or.2020.7858 |
[102] | Ambrosini G , Rai AJ , Carvajal RD , Schwartz GK . Uveal melanoma exosomes induce a pro-metastatic microenvironment through macrophage migration inhibitory factor (MIF). Mol Cancer Res. (2022) . doi: 10.1158/1541-7786.MCR-21-0526 |
[103] | Shannan B , Perego M , Somasundaram R , Herlyn M . Heterogeneity in melanoma. Melanoma. (2016) :1–15. doi: 10.1007/978-3-319-22539-5_1 |
[104] | Diener-West M , Reynolds SM , Agugliaro DJ , Caldwell R , Cumming K , Earle JD , et al. Development of metastatic disease after enrollment in the COMS trials for treatment of choroidal melanoma: Collaborative Ocular Melanoma Study Group Report No. 26. Arch. of ophthalmol. (Chicago, Ill: 1960). (2005) ;123: (12):1639–43. doi: 10.1001/archopht.123.12.1639 |
[105] | Eldh M , Bagge RO , Lässer C , Svanvik J , Sjöstrand M , Mattsson J , et al. MicroRNA in exosomes isolated directly from the liver circulation in patients with metastatic uveal melanoma. BMC cancer. (2014) ;14: (1):1–10. doi: 10.1186/1471-2407-14-962 |
[106] | Tsering T , Laskaris A , Abdouh M , Bustamante P , Parent S , Jin E , et al. Uveal melanoma-derived extracellular vesicles display transforming potential and carry protein cargo involved in metastatic niche preparation. Cancers. (2020) ;12: (10):2923. doi: 10.3390/cancers12102923 |
[107] | Ragusa M , Barbagallo C , Statello L , Caltabiano R , Russo A , Puzzo L , et al. miRNA profiling in vitreous humor, vitreal exosomes and serum from uveal melanoma patients: Pathological and diagnostic implications. Cancer Biol Ther. (2015) ;16: (9):1387–96. doi: 10.1080/15384047.2015.1046021 |
[108] | Tucci M , Passarelli A , Mannavola F , Stucci LS , Ascierto PA , Capone M , et al. Serum exosomes as predictors of clinical response to ipilimumab in metastatic melanoma. Oncoimmunology. (2018) ;7: (2):e1387706. doi: 10.1080/2162402X.2017.1387706 |
[109] | Isola AL , Eddy K , Chen S . Biology, therapy and implications of tumor exosomes in the progression of melanoma. Cancers. (2016) ;8: (12):110. doi: 10.3390/cancers8120110 |
[110] | Dimaras H , Dimba EA , Gallie BL . Challenging the global retinoblastoma survival disparity through a collaborative research effort. BMJ Publishing Group Ltd; (2010) . pp. 1415–6. doi: 10.1136/bjo.2009.174136 |
[111] | Yang Y , Mei Q . miRNA signature identification of retinoblastoma and the correlations between differentially expressed miRNAs during retinoblastoma progression. Mol Vis. (2015) ;21: :1307. |
[112] | Lande K , Gupta J , Ranjan R , Kiran M , Torres Solis LF , Solís Herrera A , et al. Exosomes: insights from retinoblastoma and other eye cancers. Int J Mol Sci. (2020) ;21: (19):7055. doi: 10.3390/ijms21197055 |
[113] | Galardi A , Colletti M , Lavarello C , Di Paolo V , Mascio P , Russo I , et al. Proteomic profiling of retinoblastoma-derived exosomes reveals potential biomarkers of vitreous seeding. Cancers. (2020) ;12: (6):1555. doi: 10.3390/cancers12061555 |
[114] | Ravishankar H , Mangani AS , Moses GLP , Mani SP , Parameswaran S , Khetan V , et al. Serum exosomal miRNA as biomarkers for retinoblastoma. ExEye Res. (2020) ;199: :108184. doi: 10.1016/j.exer.2020.108184 |
[115] | Kretschmer A , Tutrone R , Alter J , Berg E , Fischer C , Kumar S , et al. Pre-diagnosis urine exosomal RNA (ExoDx EPI score) is associated with post-prostatectomy pathology outcome. World J. Urol. (2022) :1–7. doi: 10.1007/s00345-022-03937-0 |
[116] | Diagnostic Accuracy of Circulating Tumor Cells (CTCs) and Onco-exosome Quantification in the Diagnosis of Pancreatic Cancer - PANC-CTC (PANC-CTC). NCT03032913. University Hospital, Bordeaux. 2018. https://clinicaltrials.gov/ct2/show/NCT03032913 |
[117] | Pazo-Cid Roberto A . Circulating Exosomes As Potential Prognostic And Predictive Biomarkers In Advanced Gastric Cancer Patients (“EXO-PPP Study”). Medical oncology Department, Miguel Servet University, Hospital Zaragoza, Spain. 2015 Mar. NCT01779583. https://clinicaltrials.gov/ct2/show/NCT01779583 |
[118] | Exosome Testing as a Screening Modality for Human Papillomavirus-Positive Oropharyngeal Squamous Cell Carcinoma. 2014. University of New Mexico Cancer Center, Albuquerque, New Mexico, United States. 2022 May. NCT02147418. https://clinicaltrials.gov/ct2/show/NCT02147418 |
[119] | Omics Sequencing of Exosomes in Body Fluids of Patients With Acute Lung Injury Nanfang Hospital. Southern Medical University, Guangzhou, Guangdong, China. 2021 Sep. NCT05058768. https://clinicaltrials.gov/ct2/show/NCT05058768 |
[120] | Study of Exosomes in Monitoring PatientsWith Sarcoma (EXOSARC). CHU de BesançonBesançon, France. Centre Georges François Leclerc. Dijon, France. CHU de Poitiers Poitiers, France. 2022 Apr. NCT03800121. https://clinicaltrials.gov/ct2/show/NCT03800121 |
[121] | Lei Li . Non-coding RNA in the Exosome of the Epithelia Ovarian Cancer. Beijing, Beijing, China. 2018 Nov. NCT03738319. https://clinicaltrials.gov/ct2/show/NCT03738319 |
[122] | Chen C-F , Hua K , Woung L-C , Lin C-H , Chen C-T , Hsu C-H , et al. Expression profiling of exosomal miRNAs derived from the aqueous humor of myopia patients. The Tohoku J Exp Med. (2019) ;249: (3):213–21. doi: 10.1620/tjem.249.213 |
[123] | Tsai C-Y , Chen C-T , Lin C-H , Liao C-C , Hua K , Hsu C-H , et al. Proteomic analysis of Exosomes derived from the Aqueous Humor of Myopia Patients. Int J Med Sci. (2021) ;18: (9):2023–9. doi: 10.7150/ijms.51735 |
[124] | Ahn J , Datta S , Bandeira E , Cano M , Mallick E , Rai U , et al. Release of extracellular vesicle miR-494-3p by ARPE-19 cells with impaired mitochondria. Biochim Biophys Acta. (BBA)-General Subjects.98. (2021) ;1865: (4):129598. doi: 10.1016/j.bbagen.2020.129598 |
[125] | Ke Y , Fan X , Rui H , Xinjun R , Dejia W , Chuanzhen Z , et al. Exosomes derived from RPE cells under oxidative stress mediate inflammation and apoptosis of normal RPE cells through Apaf1/caspase-9 axis. J Cell Biochem. (2020) ;121: (12):4849–61. doi: 10.1002/jcb.29713 |
[126] | Fukushima A , Takahashi E , Saruwatari J , Tanihara H , Inoue T . The angiogenic effects of exosomes secreted from retinal pigment epithelial cells on endothelial cells. Biochem Biophys Rep. (2020) ;22: :100760. doi: 10.1016/j.bbre2020.100760 |
[127] | Zhang Y , Wang K , Pan J , Yang S , Yao H , Li M , et al. Exosomes mediate an epithelial-mesenchymal transition cascade in retinal pigment epithelial cells: Implications for proliferative vitreoretinopathy. J Cell Mol Med. (2020) ;24: (22):13324–35. doi: 10.1111/jcmm.15951 |
[128] | Liu C , Ge H-M , Liu B-H , Dong R , Shan K , Chen X , et al. Targeting pericyte–endothelial cell crosstalk by circular RNA-cPWWP2A inhibition aggravates diabetes-induced microvascular dysfunction. Proc Natl Acad Sci. (2019) ;116: (15):7455–64. doi: 10.1073/pnas.1814874116 |
[129] | Zhu L , Zang J , Liu B , Yu G , Hao L , Liu L , Zhong J . Oxidative stress-induced RAC autophagy can improve the HUVEC functions by releasing exosomes. J Cell Physiol. (2020) ;235: (10):7392–409. doi: 10.1002/jc29641 |
[130] | Wang Y , Zhang Q , Yang G , Wei Y , Li M , Du E , et al. RPE-derived exosomes rescue the photoreceptors during retina degeneration: an intraocular approach to deliver exosomes into the subretinal space. Drug Deliv. (2021) ;28: (1):218–28. doi: 10.1080/10717544.2020.1870584 |
[131] | Lerner N , Schreiber-Avissar S , Beit-Yannai E . Extracellular vesicle-mediated crosstalk between NPCE cells and TM cells result in modulation of Wnt signalling pathway and ECM remodelling. J Cell Mol Med. (2020) ;24: (8):4646–58. doi: 10.1111/jcmm.15129 |
[132] | Wang Y , Niu L , Zhao J , Wang M , Li K , Zheng Y . An update: mechanisms of microRNA in primary open-angle glaucoma. Brief Funct Genomics. (2021) ;20: (1):19–27. doi: 10.1093/bfgp/elaa020 |
[133] | Chen S , Chen X , Luo Q , Liu X , Wang X , Cui Z , et al. Retinoblastoma cell-derived exosomes promote angiogenesis of human vesicle endothelial cells through microRNA-92a-3p. Cell Death & Dis. (2021) ;12: (7):1–11. doi: 10.1038/s41419-021-03986-0 |
[134] | Gencia I , Baderca F , Avram S , Gogulescu A , Marcu A , Seclaman E , et al. A preliminary study of microRNA expression in different types of primary melanoma. Bosn J Basic Med Sci. (2020) ;20: (2):197. doi: 10.17305/bjbms.2019.4271 |
[135] | Zhang W , Wang Y , Kong Y . Exosomes derived from mesenchymal stem cells modulate miR-126 to ameliorate hyperglycemia-induced retinal inflammation via targeting HMGB1. Investig Ophthalmol Vis Sci. (2019) ;60: (1):294–303. doi: 10.1167/iovs.18-25617 |
[136] | Mathew B , Ravindran S , Liu X , Torres L , Chennakesavalu M , Huang C-C , et al. Mesenchymal stem cell-derived extracellular vesicles and retinal ischemia-reperfusion. Biomaterials. (2019) ;197: :146–60. doi: 10.1016/j.biomaterials.2019.01.016 |
[137] | Bian B , Zhao C , He X , Gong Y , Ren C , Ge L , et al. Exosomes derived from neural progenitor cells preserve photoreceptors during retinal degeneration by inactivating microglia. J Extracell Vesicles. (2020) ;9: (1):1748931. doi: 10.1080/20013078.2020.1748931 |
[138] | Seyedrazizadeh S-Z , Poosti S , Nazari A , Alikhani M , Shekari F , Pakdel F , et al. Extracellular vesicles derived from human ES-MSCs protect retinal ganglion cells and preserve retinal function in a rodent model of optic nerve injury. Stem Cell Res Ther. (2020) ;11: (1):1–13. doi: 10.1186/s13287-020-01702-x |
[139] | Li D , Zhang J , Liu Z , Gong Y , Zheng Z . Human umbilical cord mesenchymal stem cell-derived exosomal miR-27b attenuates subretinal fibrosis via suppressing epithelial–mesenchymal transition by targeting HOXC6. Stem Cell Res Ther. (2021) ;12: (1):1–17. doi: 10.1186/s13287-020-02064-0.3 |
[140] | Gu C , Zhang H , Gao Y . Adipose mesenchymal stem cells-secreted extracellular vesicles containing microRNA-192 delays diabetic retinopathy by targeting ITGA1. J Cell Physiol. (2021) ;236: (7):5036–51. doi: 10.1002/jc30213 |