Balance in patients with Marfan syndrome
Abstract
Background:
Marfan syndrome (MFS) is an inherited, autosomal dominant disorder that targets the connective tissue. As in other similar disorders, joint hypermobility can contribute to abnormal proprioceptive information from joint receptors, faulty reflex patterns and impairment in balance control.
Objectives:
Aim of this study was to analyse static and dynamic balance and the effect of vision in patients with MFS.
Methods:
Twenty-seven patients with a proven genetic diagnosis of MFS were enrolled. Twenty-nine healthy subjects were the control group. Balance evaluations (eyes open and eyes closed) were performed by using a computerized force platform that recorded Sway Path (SP) and Sway Area (SA) of centre of feet pressure (CoP) displacement under static and dynamic conditions produced by mobilizing the support base.
Results:
All control subjects performed the static and dynamic trials regardless of the visual conditions. Two thirds of the Marfan patients gave results superimposable to those of normal subjects’ eyes open. However, sway area measures were significantly larger under eyes closed conditions. One third of the patients did not complete the dynamic trials eyes closed (some of these even with eyes open).
Conclusions:
Vision is critical for patients with MFS because of abnormal proprioceptive information, possibly connected to confounding mechanical reflex effects from joints and muscle receptors. It would seem appropriate to plan a rehabilitative approach centred on proprioception in order to prevent the risk of fall.
1Introduction
Marfan syndrome (MFS) (OMIM #154700) is an inherited, autosomal dominant disorder, with typical clinical manifestations involving skeletal, ocular and cardiovascular system [1], although a phenotypic heterogeneity is observed [2]. MFS is caused by mutations of the FBN1 gene that maps on chromosome 15 (15q21.1) and encodes fibrillin-1, a large, extracellular matrix glycoprotein. Protein abnormalities affect the extracellular matrix with exaggerated elastolysis [3].
Patient with MFS usually present with joint hypermobility, skin hyper-extensibility, tall stature, thin body habitus, long limbs, arachnodactyly, scoliosis, ectopia lentis, aortic root dilatation, mitral valve prolapse. Diagnosis is made in accordance with the revised Ghent diagnostic criteria [4], based on clinical manifestations, family history, and genetic testing demonstrating mutations in FBN1 [5, 6].
In MFS, as in other connective tissue disorders [7], joint hypermobility and mild myopathy [8] could contribute to faulty reflex patterns [9]. An erroneous proprioceptive information from joint receptors, in addition to laxity of the postural kinematic chain, might result in an impairment in postural control.
We therefore analysed balance in patients with MFS with genetically established diagnosis, under both static and challenging postural conditions, and compared the results with those obtained in a group of healthy subjects. The effect of vision on their balance performance was also addressed, in order to understand the reliance on vision of patients with MFS for equilibrium control.
We hypothesized that patients with MFS would have a poor control of standing balance, both under quiet stance and under difficult conditions created by an unpredictably unstable base of support. We were expecting that absence of vision would have further deranged their standing capacity, due to inadequate proprioceptive input.
2Materials and methods
2.1Participants
We investigated 27 patients with a clinically and genetically proven diagnosis of Marfan Syndrome (MFS). This cohort was a convenience sample based on all patients referred for follow up to the Centre of Genetic Cardiovascular Diseases of the Foundation IRCCS Policlinico San Matteo in Pavia in the period from September 2014 to May 2015. Exclusion criteria were: history of neurological or vestibular disease or consumption of neuroleptic drugs, scoliosis with Cobb grade≥30, or spine or leg surgery, aortic dissection diagnosed by echocardiogram before the evaluation, non-corrected visual defects. There were 19 males and 8 females (BMI 24.1±5.1 kg/m2; age 34.6±13.4 years). Most of them were myopic with a normal anterior eye segment, 3 had bilateral lens subluxation, 3 bilateral aphakia, 2 bilateral pseudophakia, 1 bilateral phacosclerosis. They were wearing appropriate eyeglasses. No additional neurological symptoms were detected and there were no clinical signs of lower limbs sensory or motor neuropathy or history of traumatism. Most patients were under beta-blocker medication. Twenty-nine healthy subjects, 23 males and 6 females (BMI 20.0±2.3 kg/m2; age 24.3±4.1 years) were recruited among the staff of the hospital and included as control group. They had no major visual problems, some were myopic and wearing eyeglasses. The two groups were matched for sex, height, weight, and shoe size.
Table 1 summarizes the principal characteristics of the patients with MFS and of the control subjects. All subjects provided written informed consent to the experiment as conformed to the Declaration of Helsinki. Evaluations were performed at the Rehabilitation Unit of the Policlinico San Matteo in Pavia.
Table 1
Characteristics of the patients with MFS and of the healthy subjects (Control Group)
Patients with MFS (N 27) | Control Group (N 29) | |
N (%) | N (%) | |
Sex (M) | 19 (70.4) | 23 (79.3) |
Medicated w. beta-blocker | 23 (85.2) | 0 (0) |
Wearing eyeglasses | 15 (55.6) | 12 (41.4) |
Mean±SD | Mean±SD | |
Age (years) | 34.6±13.4 | 24.3±4.1 |
Height (m) | 1.8±0.1 | 1.8±0.2 |
BMI (kg/m2) | 24.1±5.1 | 22.5±2.3 |
Weight (kg) | 81.8±19.3 | 80.8±10.4 |
Shoe size (EUR) | 43.4±2.3 | 42.6±1.7 |
N, Number of subjects; SD, standard deviation; M, males.
2.2Data collection and analysis
All evaluations of static and dynamic balance were made by using a computerized force platform for postural assessment (ProKin 252 Type-B, Tecnobody®). In this device, the support base on which subjects stand can be stable (static condition) or unstable (dynamic condition). Instability depends on the settings chosen by the operator by selecting one amongst several coefficients, from 1 (completely unstable) to 50 (completely stable). When a given coefficient of instability is set, the system offers the same stiffness of the platform for all patients, regardless of their body weight, by means of an automatic ‘instability control’ based on force sensors. In the present study, the coefficient of instability for the dynamic condition was set at level 30. The device computed the instantaneous position of the centre of foot pressure (CoP) at an acquisition frequency of 20 Hz. The following parameters were off-line analysed: Sway Path (SP), or the total length of the CoP trajectory travelled during 60 s (in mm), and Sway Area (SA), or the area (in mm2) of the confidence ellipse encompassing the 90% of the recorded CoP data points in the same time period.
The evaluations included eight consecutive standing trials, each lasting 60 s. Trials under static condition with eyes open (EO) and eyes closed (EC) were repeated twice. Then, participants performed the trials under dynamic condition with EO and EC, again each repeated twice. The order of the trials was fixed. With EO, participants focussed on the patterned wall of the examination room at a distance of 1 m from the force platform. During all trials, subjects were instructed to maintain the upright standing position and avoid making steps. Arms were kept at their sides and feet were positioned at an angle of±15° with respect to the antero-posterior direction, with an inter-malleolar distance of 3–4 cm. Subjects and patients with visual disabilities carried out all EO tests with their glasses or contact lenses. When on the platform, the first trial started after an interval of 10 s in order to avoid the transition phase to steady-state [10, 11]. A 10-s time-interval elapsed between subsequent trials. The duration of the entire acquisition session lasted about ten minutes. During the trials, the operator recorded any discomfort (such as fatigue, perception of instability or fear of falling) that occurred during the acquisition and stopped the current trial when the participant asked to do so or touched onto the stable frame surrounding the platform or made a step. The interrupted test was not repeated.
2.3Statistical analysis
Our convenience sample of patients with Marfan syndrome was based on the subjects who accepted to participate in this study. Since, to the best of our knowledge, there are no studies in the literature that have previously addressed postural control in these patients, we could not anticipate the prospective power calculation. Therefore, a post-hoc calculation was made of the sample size that would have given 80% power based on the actual means and standard deviation values of the Control Subjects and patients with Marfan Syndrome. The present sample size proved to be well above that necessary for the comparison between Controls and Patients for the following conditions: Sway Path EO static, SP EC static, SP EO dynamic. Sample size did not reach the required 80% power for the SP EC dynamic condition. This is the condition that not all patient were able to tolerate.
In the text, continuous data are reported as mean±SD (Sway Path, Sway Area, age, height, BMI, weight, shoe size) and categorical data as absolute values and frequencies (sex, medicated w. beta-blockers, wearing eyeglasses). The mean values of sway variables and the anthropometric characteristics of the two groups were compared with the Student’s t-test for non-paired data. The Chi-squared test was used for frequencies. Sway Path and Sway Area data from each participant entered the analysis when at least one trial was completed per balance and visual condition. When data from both trials were available, the average value from the two trials was computed. All participants with MFS completed all the static trials. Not all of them succeeded in performing at least one complete (60 s) dynamic EC trial (see below). Hence, the dynamic condition trials (under both visual condition) of these patients were excluded from the statistical analysis.
A general linear model ANOVA was used to assess the differences in Sway Area and Sway Path between the two groups (MFS and Control) and between the two balance conditions (static and dynamic), with group and condition as factors. The effect of vision was assessed by repeated-measure comparison. A post-hoc analysis was performed using the Tukey’s HSD test when the main effects or the interactions were significant. The level of significance was set at p < 0.05 for all statistics. A linear regression model was used to assess the relationship between age and body sway in the two groups. Statistics were performed by means of the software Statistica (Statsoft, Tulsa, OK, USA).
3Results
All control subjects succeeded in completing all balance trials, under both static and dynamic conditions and under both visual conditions. All patients with MFS completed all static condition trials as well, both under EO and EC condition. However, seven of these patients did not complete the EC dynamic trials, even though four of them completed at least one dynamic trial EO. The trials were stopped when the perception of instability or fear of falling induced patients to touch onto the stable frame surrounding the platform or to make a step.
Statokinesigrams of one patient with Marfan syndrome and one control subject, depicting the movement of the CoP during static and dynamic trials, are shown in Fig. 1. The displacements of the CoP obtained under each balance condition with EO (red trace) and EC (green trace) are superimposed in each panel.
Fig.1
Static (left panels) and dynamic trials (right panels) of a patient with MFS (top) and a healthy subject (bottom). EO trials are shown in red, EC trials in green. The CoP displacement obviously differed between the MFS and the healthy subjects. The differences appear to be much larger under EC than EO condition, and larger under dynamic than static condition.
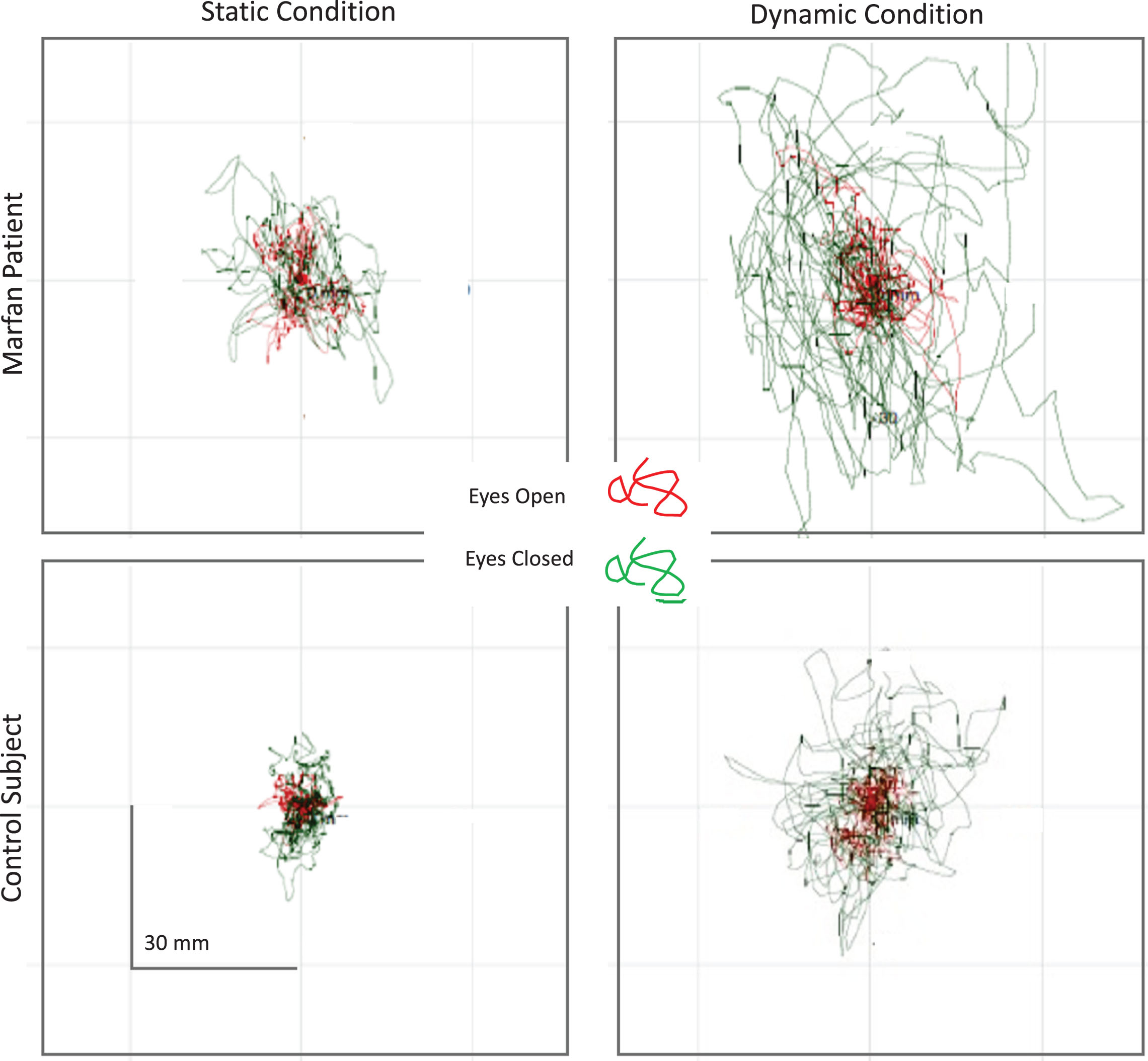
All the 27 patients were included in the analysis of the static trials. Only 20 patients were considered for the analysis of the balancing behaviour under dynamic condition, because seven patients did not complete one or both or the dynamic conditions. The Chi-squared test, applied to assess whether the two frequencies observed under dynamic conditions (Marfan 7/27; Control 29/29) were different, gave a significant result (p = 0.012). The mean values±SD of the postural parameters considered are shown in Table 2 for patients with MFS and controls.
Table 2
Sway Path and Sway Area under static and dynamic condition, with and without vision (MFS, Marfan Syndrome; Control Group). Values are reported as mean±standard deviation
Patients with MFS | Control Group | |
Sway Path EO, static (mm) | 749.4±124.6 N 27 | 600.9±142.1 N 29 |
Sway Path EC, static | 1132.7±301.9 N 27 | 865.7±211.3 N 29 |
Sway Path EO, dynamic | 1397.6±396.7 N 20 | 1066.1±319.6 N29 |
Sway Path EC, dynamic | 3545.5±1073.2 N 20 | 3069.2±1106.0 N29 |
Sway Area EO, static (mm2) | 382.1±145.2 N 27 | 207.4±81.1 N 29 |
Sway Area EC, static | 630.6±408.7 N 27 | 349.5±157.3 N 29 |
Sway Area EO, dynamic | 820.1±486.0 N 20 | 463.3±227.4 N 29 |
Sway Area EC, dynamic | 4999.5±3195.2 N 20 | 3523.7±2381.4 N 29 |
N, number of cases; mm, millimetres.
The graph of Fig. 2 summarizes the Sway Area values for both groups, for both balance conditions and for both visual conditions (the data points refer to all normal subjects and to the twenty patients who successfully performed the dynamic trials under both visual conditions).
Fig.2
Sway Area under static and dynamic condition, with and without vision (A, Control Group, N = 29; B, patients with MFS, N = 20). Values are means±standard deviations.
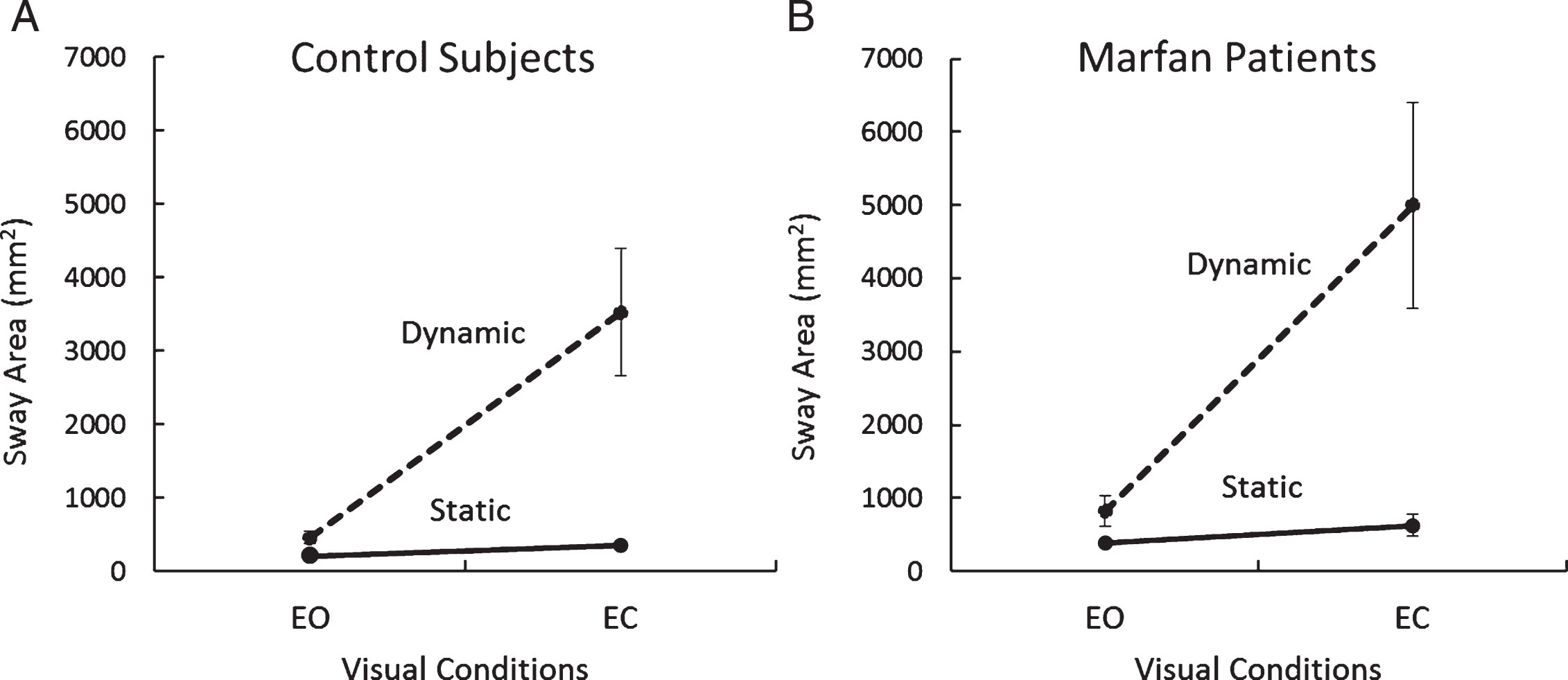
ANOVA showed a difference in Sway Area between groups (MFS > Controls; F(1,101) = 7.54, p < 0.01), between balance conditions (dynamic > static; F(1,101) = 97.63, p < 0.001) and between vision conditions (EC > EO; F(1,101) = 115.29, p < 0.001). The interaction effect of group and balance condition did not reach a significant difference (F(1,101) = 2.73, p = 0.10). The interaction of group and visual condition did not reach a significant difference, either (F(1,101) = 2.97, p = 0.09). Neither was the interaction group×vision×balance condition (static, dynamic) significant (F(1,101) = 2.03, p = 0.16). These results indicate a broadly similar effect of vision and balance condition on the subjects of both groups, who succeeded in completing both the static and the dynamic tasks. The post-hoc test showed that Sway Area under dynamic condition EC was significantly greater in the patients with MFS than in the Control subjects (p < 0.01). However, there were no significant differences between MFS and Control groups for the dynamic condition EO (p = 0.99) and for static condition, both EO and EC (p = 0.99; p = 0.99).
Sway Path measures gave similar findings. ANOVA showed a difference in Sway Path (not shown in Figure) between groups (MFS > Controls; F(1,101) = 11.17, p < 0.01), between balance conditions (dynamic > static; F(1,101) = 245.07, p < 0.001) and an effect of vision (EC > EO; F(1,101) = 321.23, p < 0.001). The interaction between group and balance condition was not significant (F(1,101) = 1.15, p = 0.29). The interaction of group and visual condition was not significant, either (F(1,101) = 0.97, p = 0.33). The interaction group×vision×balance condition was not significant (F(1,101) = 0.01, p = 0.92), indicating that the effect of vision and balance condition on the Sway Path was substantially similar in the Control subjects and in the patients with MFS, who succeeded in completing the dynamic tasks.
Of note, the differences between groups emerging from the ANOVA are underestimated because the patients with MFS that entered that analysis were those able to complete all the trials. Seven patients (26% of our sample) failed to complete the trials under dynamic conditions. Four of these did not complete the dynamic trail EO. When the Sway Area values recorded under static conditions in those patients with MFS that did not complete the dynamic trials were considered, no difference was found in their mean values with respect to the other MFS patients under static condition, for either EO or EC conditions (Student’s t-test, p > 0.8 for both comparisons).
Figure 3 A shows the relationship between Sway Area eyes closed under dynamic condition and Sway Area eyes closed under static condition in the entire population of Control subjects and in the patients with MFS, who performed successfully the dynamic trials (n = 20). Each data point corresponds to one subject. The graph shows that Sway Area is much larger under dynamic than static condition (note the different scales), and that the area under the dynamic condition increases across subjects as a function of their instability under static condition. Moreover, it appears that this relationship broadly holds for both groups.
Fig.3
A. Mean individual Sway Area (EC) values recorded under dynamic condition (vertical axis) are plotted against those recorded under static condition (horizontal axis) in the two groups (filled circles, patients with MFS; open circles, Control Subjects). B. Sway area (mm2) under dynamic condition only; EC data are plotted against EO.
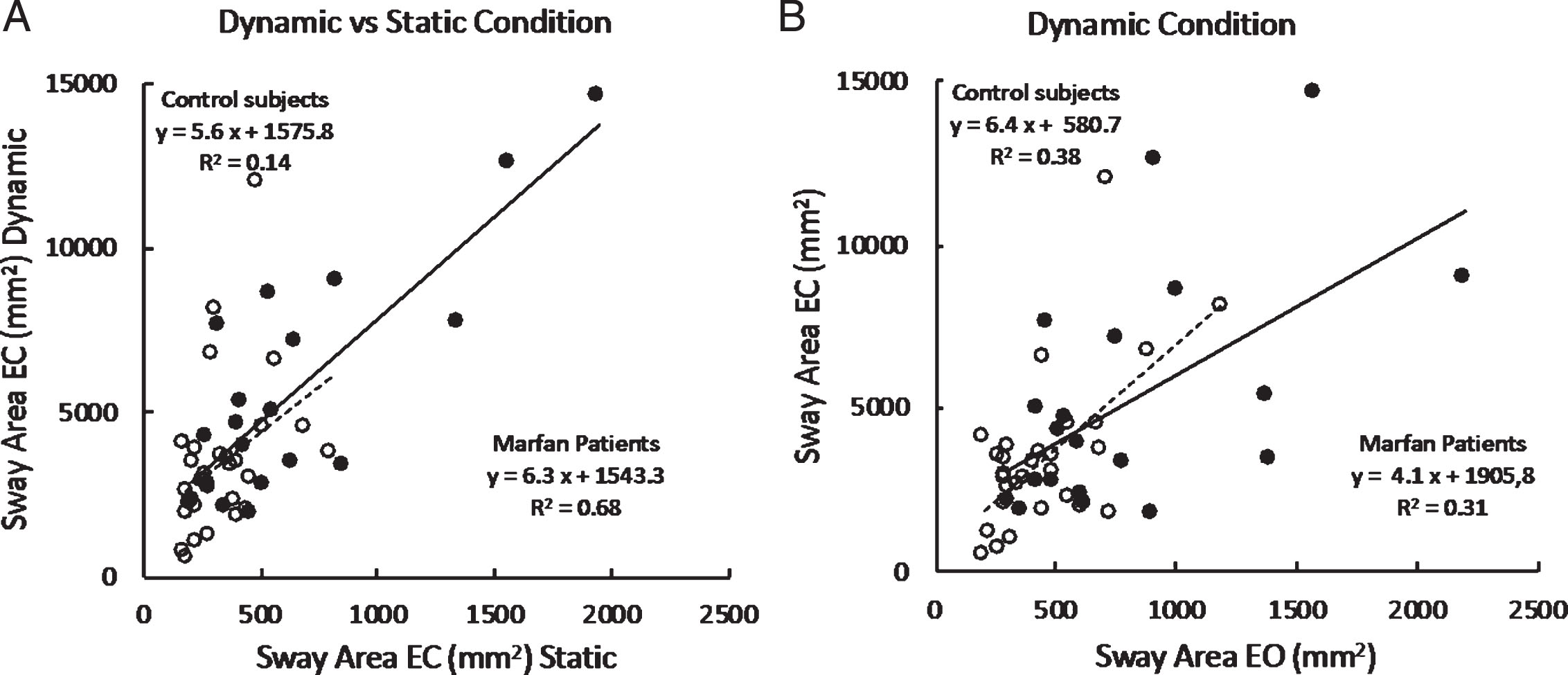
The effect of vision under dynamic conditions in the patients who succeeded in these trials under both visual conditions is highlighted in Fig. 3B. This shows the relationship between Sway Area eyes closed versus Sway Area eyes open under dynamic condition only. Each data point corresponds to one subject. Sway Area is larger with eyes closed for all the subjects of both groups. The relationship of EC to EO data broadly holds for both groups.
No relationship was found between age and body sway in the two groups standing under EO and EC static conditions (Sway Path; control subjects: R2 = 0.02, p = 0.37 and R2 = 0.006, p = 0.68, for EO and EC respectively; patients with MFS: R2 0.0009, p = 0.87 and R2 0.06, p = 0.061, for EO and EC respectively. Sway Area; control subjects: R2 0.1, p = 0.089 and R2 0.031, p = 0.35, for EO and EC respectively; patients with MFS: R2 0.001, p = 0.87 and R2 0.0016, p = 0.83, for EO and EC respectively). This analysis was not made for the dynamic conditions because many patients failed to complete the trials, so they were excluded from this part of the study.
4Discussion
This study analysed static and dynamic balance in patients with Marfan syndrome (MFS). To the best of our knowledge, no studies focused on stance stability of patients with MFS. Assessment of balance control capacity in MFS may contribute to enhance our knowledge of the pathophysiology of this disease and help prevent falls that could be complicated by fractures, considering the alteration of bone mineral density in this syndrome [12, 13]. The literature has focused so far on specific clinical features of this disease [14], such as cardiovascular or orthopaedic conditions [15–18], but no studies examined the quality of standing balance of these patients, under easy and challenging conditions [14]. Patients with MFS suffer from a neuromuscular involvement, which seems to be unrecognized at diagnosis [8], and possibly leads to the increased risk of falling that gets worse with age. We had hypothesized that impairment of postural control under static and dynamic conditions would be a hallmark of MFS patients, defined here as an alteration of balance parameters as Sway Area (as an index of the precision of the postural control system) and Sway Path (as an index of the energy expenditure to preserve the balance).
Indeed, all conditions collapsed, the body oscillation was significantly larger in the patients than in the control subjects, indicating a general poorer stability in MFS. This was true, regardless of the non-inclusion in the ANOVA of the seven out of the 27 Marfan patients that did not complete the dynamic trials. On the one hand, this simple but significant difference in the frequencies of difficult trial completion indicates that the clinical condition of the MFS can be for some a serious tax to stance stability under challenging conditions. On the other hand, it shows that not all MFS patients are unable to maintain equilibrium on an unstable base of support for a period as long as one minute.
4.1Balance control under static conditions
We were surprised in noting no major difference in body oscillation under static condition (either for length of path and area covered by the CoP displacement) in many of our patients compared to the control group, despite the patients’ joint hypermobility, which is generally most pronounced in distal joints [19]. Body oscillation in MFS patients did not significantly differ from that observed in the control group when participants stood quietly on the firm platform with eyes open. Although the mean values of the MFS population were indeed larger than in the control subjects, the difference was independent of age, and did not reach significance owing to the large inter-individual variability.
The performance was not significantly worse in patients with MFS under quiet-stance condition eyes-closed, either. Proprioception is apparently sufficient for controlling body sway when the standing conditions are not challenging. Likely, hypermobility does not express itself under static condition, so that proprioceptive information may be adequate. The finding would be also in favour of absence in these patients of a major peripheral nerve disease, a condition that might occur in MFS [8]. However, we cannot deny that the patients exhibiting the largest oscillations might be presenting with a subclinical neuropathy, which is known to affect the control of quiet stance [20]. Others have shown in Prader-Willi and Ehlers-Danlos patients that muscle weakness affects balance [7, 21].
4.2Balance control under dynamic conditions
Our data showed a significant difference between the patients with MFS who succeeded in standing under dynamic conditions and Control subjects when we compared the respective body sway area recorded during the dynamic trials with eyes closed (sway area was 42% larger in patients with MFS than in control subjects). This contrasts with the absence of a significant difference between groups in the dynamic condition eyes-open. Under dynamic conditions, vision must have an actual stabilizing effect on body oscillation, so that patients with MFS did not oscillate significantly more than healthy subjects when vision was allowed. Perhaps, the ‘instability’ setting of the platform (the amplitude of the tilts allowed by the setting) was not enough to create a challenging condition to which eyes-open MFS patients could react abnormally. Conversely, the stabilizing role of vision is emphasised by the fact that absence of vision was detrimental under dynamic conditions in some patients with MFS, since seven of them (26% of the cohort) could not even complete the 1-min trial without vision. We considered and excluded possible phenotypical characteristics (height, weight, age, gender, foot length) accountable for the inadequate postural performance of the MFS patients who did not complete the dynamic condition trials. Vision is critical for any subjects standing under dynamic conditions [22, 23], but certainly more so for the patients with MFS, likely because of the potential unreliability of proprioception derived from their joint laxity. Perhaps, proprioception is down-weighted in these patients because of the changes in connective tissues and extracellular matrix [24], since the mechanical effects of the reflexes originating from large and small-diameter spindle fibres and from Golgi tendon organs would be highly unpredictable and variable, and possibly produce uncoordinated reactions. The proprioceptive ‘unreliability’ might be a more severe impairment than muscle weakness [25], because force is not really a limitation during upright stance, even under critical conditions [26]. Inaccuracy of proprioception might in turn produce a major dependence on vision, a sense of anxiety, and an increased sense of effort in these patients in sustaining the dynamic trials.
Remarkably, the effect of vision is larger, and significantly so, when balance is evaluated by sway area than by sway path. Large amplitude CoP oscillations (sway area) in patients showing just a minor increase in the total length of the CoP path under dynamic conditions eyes closed indicate ample, low frequency body oscillations, and can be compatible with impaired moment-to-moment control of the stiffness of the postural muscles [27], as if proprioception would be down-weighted or impaired motor reactions to proprioceptive input would occur as a consequence of the joint hypermobility, or both. Interestingly, Sakellari et al. [28] found comparable results in normal subject (sway area increased, but sway path increased only marginally) in which dizziness was induced by hyperventilation, and attributed this to altered proprioception. Proportionally larger values for sway area than sway path have been observed recently in patients with multiple sclerosis standing eyes closed, and attributed to their impaired reliance on somatosensory information [29].
4.3Balance in hypermobility syndromes
Other investigators focused on postural control in another heritable connective tissue disorders, the Ehlers-Danlos Syndrome (EDS) [21, 30, 31]. All found balance impairment in these patients. In particular, Galli et al. [21] assumed that hypotonia and joint hypermobility in EDS could lead to a motor dysfunction, based mainly on faulty reflex sensory patterns originated from joint sensors and tendon organs of those patients. Rombaut et al. [30] compared balance and gait of a group of EDS adults to that of control subjects, and showed an increased body sway in EDS patients, both during EO and EC trials. Rigoldi et al. [32] investigated the postural control in children, teenagers and adults with Down Syndrome, which are also characterized by hypotonia and ligament laxity (a feature common with MFS), and found impaired postural control among all these patients, with the onset of some postural abnormalities during the childhood. Our study suggests that investigating dynamic conditions and the effect of vision can enhance our knowledge about equilibrium control in hypermobility syndromes.
4.4Limitations
There was considerable variability in the sway data within our participants with MFS. The patients were just older than the subjects of the control group (significantly so, p < 0.001). In this population, age might have a so far unknown effect, whereas no significant age-related increase in sway is to be expected in a population of healthy subjects, for either static [33] or dynamic conditions [34]. However, when the same age range was considered for both groups (age < 40 yrs), no significant difference was observed. Further, within the whole patients' group, we found no significant relationship between sway and age (for both static EO and EC conditions).
Absence of instrumental evaluation of nerve conduction velocity or muscle force is a limitation of this study. Conduction velocity of sensory nerves was not tested in these patients, nor reflex excitability was tested by eliciting the H-reflex. The results of these tests would have allowed a finer evaluation of subclinical peripheral neuropathy and postural muscle reflex excitability in these patients with MFS.
4.5Prospective intervention
Vision is critical for MFS patients because of their potential alterations in proprioception, possibly connected to confounding mechanical reflex effects from abnormally activated joints and muscle receptors. Patients with MFS would exploit vision as an adaptive or compensatory mechanism tending to restore normal balance control in spite of the unreliability of the proprioceptive control. Any intervention aimed at enhancing their ability to integrate multisensory inputs and to reduce anxiety connected with the effort of maintaining balance under critical conditions [35, 36] would have important translational impact [37].
By considering the risk of falls in these patients, we would suggest to plan an appropriate rehabilitative approach, focused on the improvement of their muscular and proprioceptive performance, with exercises performed both eyes open and eyes closed. To our knowledge, no studies focused on this issue, even if studies on rehabilitation of patients with Marfan syndrome exist [38]. Because of the high frailty of these patients, it is difficult to locate the best rehabilitative approach to improve their balance performance without inducing cardio-vascular effort and muscle fatigue [39]. However, a regular aerobic exercise appears to be useful to improve motor function and reduce risk of fall in different syndromic diseases such as, for instance, Parkinson’s Disease [40, 41]. Simple stretching and limbering exercises as well as exercises to improve balance and body awareness would lead to reweighting of proprioceptive information, allowing more reliance on proprioceptive sense in order to favour the control of equilibrium under both static and dynamic conditions, much as it is suggested for post-stroke patients [42] and elderly people [43].
5Conclusions
Balance is critical under challenging conditions in patients with Marfan syndrome, and vision availability plays an important role in equilibrium control. On the one hand, the findings enforce appropriate eyesight correction [44], on the other they encourage rehabilitation by proprioceptive training, which can yield meaningful improvements in somatosensory and sensorimotor function [45].
Acknowledgments
We would like to sincerely thank all the participants in this research.
References
[1] | A. Kumar , S. Agarwal . Marfan syndrome: An eyesight of syndrome, Meta Gene 2: ((2014) ), 96–105. |
[2] | N.M. Ammash , T.M. Sundt , H.M. Connolly . Marfan syndrome-diagnosis and management, Current Problems in Cardiology 33: ((2008) ), 7–39. |
[3] | I. El-Hamamsy , M.H. Yacoub . Cellular and molecular mechanisms of thoracic aortic aneurysms, Nature Reviews Cardiology 6: ((2009) ), 771–786. |
[4] | B.L. Loeys , H.C. Dietz , A.C. Braverman , B.L. Callewaert , J. De Backer , R.B. Devereux , Y. Hilhorst-Hofstee , G. Jondeau , L. Faivre , D.M. Milewicz , R.E. Pyeritz , P.D. Sponseller , P. Wordsworth and A.M. De Paep . The revised Ghent nosology for the Marfan syndrome, Journal of Medical Genetics 47: ((2010) ), 476–485. |
[5] | T. Geva , J. Hegesh , M. Frand . The clinical course and echocardiographic features of Marfan’s syndrome in childhood, American Journal of Diseases of Children 141: ((1987) ), 1179–1182. |
[6] | H. Dietz. (2001) (2017) . Marfan Syndrome. In: M Adam , HH Ardinger , RA Pagon , SE Wallace , LJH Bean , K Stephens , A Amemiya editors. GeneReviews© [Internet]. Seattle (WA): University of Washington, Seattle; 1993-2018. http://www.ncbi.nlm.nih.gov/books/NBK1335/PubMed PMID: 20301510. |
[7] | M. Galli , V. Cimolin , L. Vismara , G. Grugni , F. Camerota , C. Celletti , G. Albertini , C. Rigoldi , P. Capodaglio . The effects of muscle hypotonia and weakness on balance: A study on Prader-Willi and Ehlers-Danlos syndrome patients, Research in Developmental Disabilities 32: ((2011) ), 1117–1121. |
[8] | N.C. Voermans , J. Timmermans , N. van Alfen , S. Pillen , J. op den Akker , M. Lammens , M.J. Zwarts , I.A. van Rooij , B.C. Hamel and B.G. van Engele . Neuromuscular features in Marfan syndrome, Clinical Genetics 76: ((2009) ), 25–37. |
[9] | H. Røgind , J.J. Lykkegaard , H. Bliddal and B. Danneskiold-Samsø . Postural sway in normal subjects aged 20-70 years, Clinical Physiology and Functional Imaging 23: ((2003) ), 171–176. |
[10] | C. Rigoldi , V. Cimolin , F. Camerota , C. Celletti , G. Albertini , L. Mainardi , M. Galli . Measuring regularity of human postural sway using approximate entropy and sample entropy in patients with Ehlers-Danlos syndrome hypermobility type, Research in Developmental Disabilities 34: ((2013) ), 840–846. |
[11] | J. Tarantola , A. Nardone , E. Tacchini , M. Schieppati . Human stance stability improves with the repetition of the task: Effect of foot position and visual condition, Neuroscience Letters 228: ((1997) ), 75–78. |
[12] | N. Carter , E. Duncan , P. Wordsworth . Bone mineral density in adults with Marfan syndrome, Rheumatology (Oxford) 39: ((2000) ), 307–309. |
[13] | P.F. Giampietro , M. Peterson , R. Schneider , J.G. Davis , C. Raggio , E. Myers , S.W. Burke , O. Boachie-Adjei , C.M. Mueller . Assessment of bone mineral density in adults and children with Marfan syndrome, Osteoporosis International 14: ((2003) ), 559–563. |
[14] | E. Ferrante , A. Citterio , A. Savino , P. Santalucia . Postural headache in a patient with Marfan’s syndrome, Cephalalgia 23: ((2003) ), 552–555. |
[15] | P.F. Giampietro , C. Raggio , J.G. Davis . Marfan syndrome: Orthopedic and genetic review, Current Opinion in Pediatrics 14: ((2002) ), 35–41. Review. Erratum in (2002): Current Opinion in Pediatrics 14, 286. |
[16] | Y. Glard , V. Pomero , P. Collignon , W. Skalli , J.L. Jouve , G. Bollini . Three-dimensional analysis of the vertebral rotation associated with the lateral deviation in Marfan syndrome spinal deformity, Journal of Pediatric Orthopaedics B 18: ((2009) ), 51–56. |
[17] | J.C. Dean . Marfan syndrome: Clinical diagnosis and management, European Journal of Human Genetics 15: ((2007) ), 724–733. |
[18] | Y. Von Kodolitsch and P.N. Robinso . Marfan syndrome: An update of genetics, medical and surgical management, Heart 93: ((2007) ), 755–760. |
[19] | S. Donkervoort , C.G. Bonnemann , B. Loeys , H. Jungbluth , N.C. Voermans . The neuromuscular differential diagnosis of joint hypermobility, American Journal of Medical Genetics Part C: Seminars in Medical Genetics 169C: ((2015) ), 23–42. |
[20] | A. Nardone , M. Grasso , M. Schieppati . Balance control in peripheral neuropathy: Are patients equally unstable under static and dynamic conditions? Gait & Posture 23: ((2006) ), 364–373. |
[21] | M. Galli , C. Rigoldi , C. Celletti , L. Mainardi , N. Tenore , G. Albertini , F. Camerota . Postural analysis in time and frequency domains in patients with Ehlers-Danlos syndrome, Research in Developmental Disabilities 32: ((2011) ), 322–325. |
[22] | D. Joseph Jilk , S.A. Safavynia and L.H. Tin . Contribution of vision to postural behaviors during continuous support-surface translations, Experimental Brain Research 232: ((2014) ), 169–180. |
[23] | S. Sozzi , A. Nardone , M. Schieppati . Calibration of the Leg Muscle Responses Elicited by Predictable Perturbations of Stance and the Effect of Vision, Frontiers in Human Neuroscience 30: (10) ((2016) ), 419. |
[24] | J. Halper , M. Kjaer . Basic components of connective tissues and extracellular matrix: Elastin, fibrillin, fibulins, fibrinogen, fibronectin, laminin, tenascins and thrombospondins, Advances in Experimental Medicine and Biology 802: ((2014) ), 31–47. |
[25] | G. Percheron , G. Fayet , T. Ningler , J.M. Le Parc , Denot-Ledunois S., Leroy M., Raffestin B. and Jondeau G. Muscle strength and body composition in adult women with Marfan syndrome, Rheumatology (Oxford) 46: ((2007) ), 957–962. |
[26] | U. Granacher , A. Gollhofer . Is there an association between variables of postural control and strength in adolescents? The Journal of Strength & Conditioning Research 25: ((2011) ), 1718–1725. |
[27] | B.L. Julien , A.P. Bendrups . Reflex ankle stiffness is inversely correlated with natural body sway, Gait & Posture 44: ((2016) ), 128–130. |
[28] | V. Sakellari , A.M. Bronstein , S. Corna , C.A. Hammon , S. Jones , C.J. Wolsley . The effects of hyperventilation on postural control mechanisms, Brain 120: ((1997) ), 1659–1673. |
[29] | A. Kalron . The Romberg ratio in people with multiple sclerosis, Gait and Posture 54: ((2017) ), 209–213. |
[30] | L. Rombaut , F. Malfait , I. De Wandele , Y. Thijs , T. Palmans , A. De Paepe and P. Calder . Balance, gait, falls, and fear of falling in women with the hypermobility type of Ehlers-Danlos syndrome, Arthritis care & research (Hoboken) 63: ((2011) ), 1432–1439. |
[31] | M. Scheper , L. Rombaut , J. de Vries , I. De Wandele , M. van der Esch , B. Visser , F. Malfait , P. Calders and R. Engelber . The association between muscle strength and activity limitations in patients with the hypermobility type of Ehlers-Danlos syndrome: The impact of proprioception, Disability and Rehabilitation 39: ((2016) ), 1391–1397. |
[32] | C. Rigoldi , M. Galli , L. Mainardi , M. Crivellini , G. Albertini . Postural control in children, teenagers and adults with Down syndrome, Research in Developmental Disabilities 32: ((2011) ), 170–175. |
[33] | M. Schieppati , M. Grasso , R. Siliotto , A. Nardone . Effect of age, chronic diseases and Parkinsonism on postural control. In: Sensorimotor Impairment in the Elderly, Stelmach, George E., Hömberg, V. (Eds.), Nato Science Series D 75: ((1993) ), 355–373 . |
[34] | A. Nardone , R. Siliotto , M. Grasso , M. Schieppati . Influence of aging on leg muscle reflex responses to stance perturbation, Arch Phys Med Rehabil 76: ((1995) ), 158–165. |
[35] | C. Baeza-Velasco , G. Pailhez , A. Bulbena , A. Baghdadli . Joint hypermobility and the heritable disorders of connective tissue: Clinical and empirical evidence of links with psychiatry, General Hospital Psychiatry 37: ((2015) ), 24–30. |
[36] | J.R. Davis , A.D. Campbell , A.L. Adkin , M.G. Carpenter . The relationship between fear of falling and human postural control, Gait & Posture 29: ((2009) ), 275–279. |
[37] | G. Velvin , T. Bathen , S. Rand-Hendriksen and A.Ø. Geirda . Satisfaction with life in adults with Marfan syndrome (MFS): Associations with health-related consequences of MFS, pain, fatigue, and demographic factors, Quality of Life Research 25: ((2016) ), 1779–1790. |
[38] | D. Benninghoven , D. Hamann , Y. von Kodolitsch , M. Rybczynski , J. Lechinger , F. Schroeder , M. Vogler and E. Hober . Inpatient rehabilitation for adult patients with Marfan syndrome: An observational pilot study, Orphanet Journal of Rare Diseases 12: ((2017) ), 127. |
[39] | J. Papagiannis . Sudden death due to aortic pathology., Cardiology in the Young 27: ((2017) ), S36–S42. |
[40] | B.E. Fisher , A.D. Wu , G.J. Salem , J. Song , C.H. Lin , J. Yip , S. Cen , J. Gordon , M. Jakowec , G. Petzinger . The effect of exercise training in improving motor performance and corticomotor excitability in people with early Parkinson’s disease, Archives of Physical Medicine and Rehabilitation 89: ((2008) ), 1221–1229. |
[41] | C.A. Peacock , G.J. Sanders , K.A. Wilson , E.J. Fickes-Ryan , D.B. Corbett , K.P. von Carlowitz and A.L. Ridge . Introducing a multifaceted exercise intervention particular to older adults diagnosed with Parkinson’s disease: A preliminary study, Aging Clinical and Experimental Research 26: ((2014) ), 403–409. |
[42] | D.S. Nichols . Balance retraining after stroke using force platform biofeed-back, Physical Therapy 77: ((1997) ), 553–558. |
[43] | M. Gandolfi , E. Dimitrova , F. Nicolli , A. Modenese , A. Serina , A. Waldner , M. Tinazzi , G. Squintani , N. Smania , C. Geroin . Rehabilitation procedures in the management of gait disorders in the elderly, Minerva Medica 106: ((2015) ), 287–307. |
[44] | M. Schmid , L. Casabianca , A. Bottaro , M. Schieppati . Graded changes in balancing behavior as a function of visual acuity, Neuroscience 153: ((2008) ), 1079–1091. |
[45] | J.E. Aman , N. Elangovan , I.L. Yeh , J. Konczak . The effectiveness of proprioceptive training for improving motor function: A systematic review.Review, Frontiers in Human Neuroscience 8: . |