Sustainable effectiveness of kinetic chain stretching on active hip flexion movement and muscle activation for hamstring tightness: A preliminary investigation
Abstract
BACKGROUND:
Conventional hamstring (HAM) stretching therapeutic effects are not substantiable in neuromusculoskeletal conditions with HAM tightness or shortness. We developed a kinetic chain stabilization exercise to provide a more sustainable effectiveness in adults with HAM tightness. However, its therapeutic effects and underlying motor mechanisms remain unknown.
OBJECTIVE:
To compare the effects of traditional active HAM stretching (AHS) and kinetic chain stretching (KCS) on electromyographic (EMG) amplitude and hip flexion range of motion (ROM) in participants with HAM tightness.
METHODS:
In this randomized controlled trial, 18 participants (mean age: 25.01
RESULTS:
Compared to AHS, KCS led to greater increase in the hip flexion ROM and EMG activation amplitudes in the left and right EO and left IO. Post-test hip flexion ROM data in both the groups were higher than the pre-test data.
CONCLUSION:
KCS produced more sustainable effectiveness in hip flexion movement and EMG motor control patterns in participants with HAM tightness than AHS.
1.Introduction
Hamstring tightness or contracture is a prevalent neuromusculoskeletal condition affecting essential daily movements such as sitting, standing, and walking in conditions like hemiparetic stroke, spastic diplegia, and Parkinsonism [1, 2, 3]. This issue often leads to functional and structural impairments within the lumbo-pelvic-hip kinetic chain, potentially resulting in back pain and hamstring strains [4, 5, 6]. Functional or structural impairments in the lumbo-pelvic-hip complex may not resolve until adequate hamstring length is restored [7, 8]. Failing to address this concern can lead to heightened hamstring tension and stiffness, thereby contributing to complications within the lumbo-pelvic-hip complex [9, 10, 11]. Prolonged shortening of the hamstrings prompts them to become relatively stiffer compared to lumbar spine tissue, resulting in a posterior pelvic tilt and lumbar spine flexion, causing an anterior shearing force. This, in turn, may lead to posterior derangement of lumbar intervertebral discs and spinal structures, commonly observed in the L4-5 vertebrae [12, 13]. Such posterior derangement of the lumbar intervertebral discs causes discogenic low back pain (LBP) and spondylolisthesis [14, 15].
Contemporary therapeutic techniques including proprioceptive neuromuscular facilitation (PNF) hold-relax and contract-relax techniques, static stretching, and dynamic stretching have been used to address hamstring tightness or shortness. However, these techniques have yielded inconsistent outcomes [16, 17, 18, 19]. Some studies attribute the increased muscle extensibility after single stretching sessions or short-term traditional active hamstring stretching (AHS) programs to modified sensations [20, 21, 22, 23]. Weppler et al. suggested that modifications to the AHS may be necessary to address these factors [24]. Recently, the lumbo-pelvic-hip kinetic chain concept emphasizes the interconnectedness of body segments during movement and stabilization [25]. Kinetic Chain Stretching (KCS) has been introduced as a critical component of intra-abdominal pressure management and dynamic spinal stabilization prior to movement [26]. The underlying mechanism of KCS is that weaknesses or dysfunctions in core and gluteus muscles can lead adjacent muscles like the hamstrings to become overactive or compensatory [27]. Therefore, KCS practice emphasizes core stabilization before hamstring stretching to effectively address these compensatory mechanisms and improve overall movement efficiency [25, 27]. This technique focuses on enhancing hip flexion and knee extension coordination, alongside motor control within core (diaphragm, internal oblique [IO], external oblique [EO]) and hip muscles during walking and running [28, 29, 30].
Despite the potentially important clinical contributions of the KCS to hamstring shortness, the effectiveness of hamstring stretching exercises with or without core stabilization in improving hamstring flexibility remains unknown. Thus, the aim of this study was to compare therapeutic effects of the KCS and AHS on hamstring muscle activity, hip joint angle, and satisfaction levels. Our hypothesis suggests a difference in hamstring flexibility and core chain muscle activation, as assessed through maximal active hip joint flexion angles, between AHS and AHS with KCS among individuals with hamstring tightness.
2.Method
2.1Participants
We enrolled 18 male participants with hamstring tightness (mean age, 25.01
2.2Procedures
The research produced a sequence of random numbers using a computer generated simple random number table to ensure unbiased and unpredictable allocation. Eighteen participants were recruited and assigned to either group AHS or KCS based on odd or even numbers. The allocation procedure was carried out by a researcher. A standardized experimental protocol was meticulously maintained, encompassing uniform verbal instructions, consistent testing intervals, and sequence uniformity. This uniformity was upheld during both the pre-test phase involving assessments of AROM and electromyography (EMG), as well as the subsequent intervention (AHS or KCS), followed by the post-test phase. The ASLR test was administered while participants were supine. Following proper positioning, participants were instructed to achieve complete muscle relaxation. Prior to data collection, participants underwent a preliminary familiarization session for the ASLR test to ensure standardized and consistent test execution. Upon instruction, participants gradually elevated their leg to a comfort level, under the careful guidance of the experimenter to ensure a measured and controlled motion. Utilizing diverse sensors and an electric goniometer, data were accurately captured. Preceding the intervention, each participant executed an active hip flexion AROM to establish baseline measurements. A visual representation of the study protocol is provided in Fig. 1. Simultaneously, we conducted measurements of electromyography (EMG) and kinematic data as participants performed the ASLR test. Stretching measurements were subsequently carried out by both groups as detailed below. The assessment of AROM was derived from an average of three repetitions. Each group adhered to the subsequent methodology. The intricacies of this procedure are expounded upon in the ensuing sections. This paper proceeds to elaborate on the delineated process in subsequent sections.
2.2.1Active hip flexion range of motion test
We measured the active hip flexion range of motion (ROM) using a smartphone placed on the tibial crest, 5 cm distal to the superior border of the lateral malleolus on the unilateral leg. The ASLR test in this study maintained the ankle in a neutral position (90∘) with no added dorsiflexion or plantar flexion (Fig. 2). To measure the angle change, we used the GetMyROM Pro software (version 1.0.3; Interactive Medical Products, Hampton, NH, USA). The validity intra-class coefficient was reported to be 0.96–0.97; test-retest reliability was reported to be 0.84–0.93 [34]. We used an iPhone 13 Pro (128 GB, model no. MLV93KH/A; Apple Inc., Cupertino, CA, USA) with GetMyROM Pro software, an inclinometry-based application to measure hip ROM.
Figure 1.
Study flow chart.
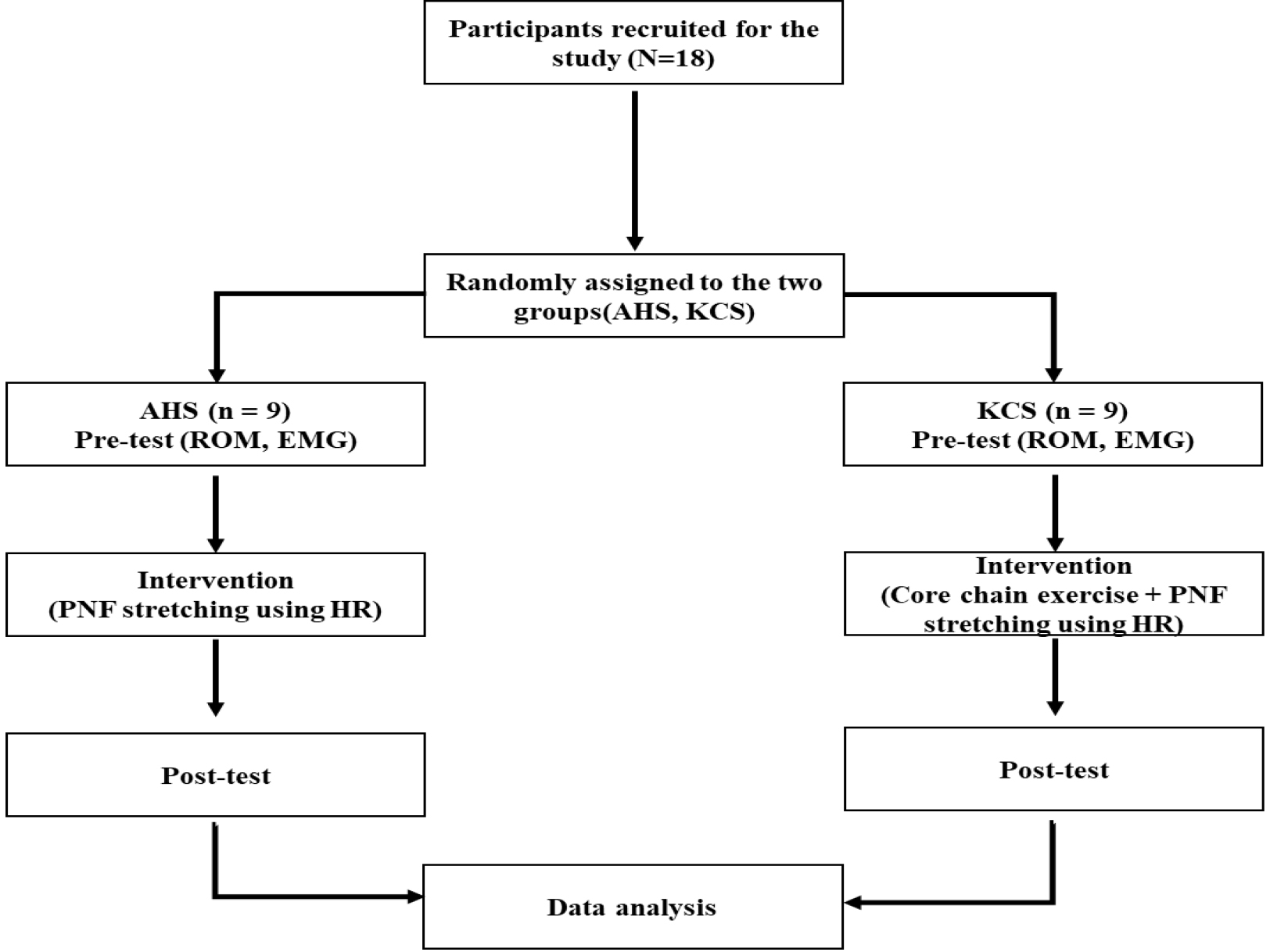
Figure 2.
Measurement of the active range of motion of the hip joint.
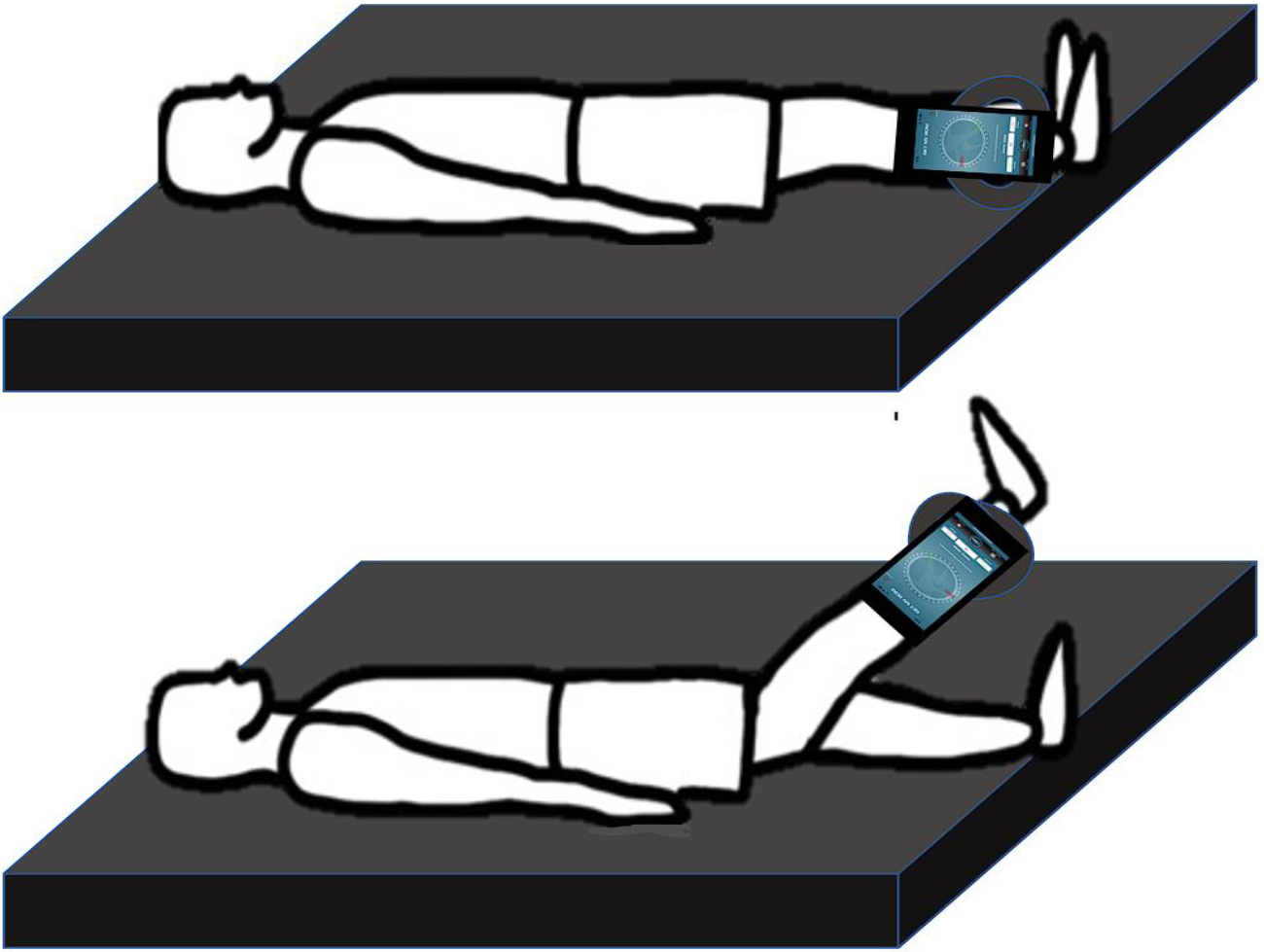
2.2.2EMG recording and data processing
Surface EMG signals were acquired using the Ultium EMG system (Noraxon Inc., Scottsdale, AZ, USA), in conjunction with the MyoResearch software package (ver. 3.18, Noraxon, Inc., Scottsdale, AZ, USA). A sampling rate of 1500 Hz was employed, with band-pass filtering ranging from 50 Hz to 450 Hz, and no application of notch filters. Root mean square calculations were conducted utilizing a 50 ms moving window, with an overall gain set at 500. Exceptional signal quality was ensured with a common mode rejection ratio exceeding 100 dB and an input impedance surpassing 100 Mohm. Participants assumed a designated position on the mat, and double electrodes were meticulously affixed in compliance with Cram’s surface EMG guidelines. Prior to electrode placement, target skin areas were appropriately prepped, involving shaving and disinfection using rubbing alcohol. Electrode pairs were strategically positioned over specific muscles: bilateral rectus abdominis (RA), bilateral external oblique (EO), bilateral internal oblique/transverse abdominis (IO/TrA), bilateral lumbar erector spinae (ES), unilateral rectus femoris (RF), and unilateral biceps femoris (BF). Detailed electrode placement procedures were previously delineated [35]. Maximal voluntary isometric contractions (MVIC) for the aforementioned muscles were conducted, involving three repetitions for each muscle. Data were subsequently analyzed through the MyoResearch software package on a notebook PC. Muscle activity was recorded over a 7-second duration, and the averaged values from all three trials were employed for subsequent analysis.
2.3Interventions
2.3.1Traditional active hamstring stretching using hold-relax
PNF stretching employs the hold-relax technique to mitigate disruptions in the neural activity of linked muscles, autogenic inhibition, and myotatic reflexes [36]. The hold-relax technique involves lengthening the muscle to its limit, followed by an isometric contraction lasting up to 10 seconds. After this, the limb is passively moved into the new end range. This technique is used to improve muscle flexibility [18]. In Group 1, for hamstring stretching with AHS, the subject lies supine with hips flexed at a 90-degree angle. The therapist extends the knee for a mild hamstring stretch. The subject then isometrically contracts the hamstrings by flexing the knee against the therapist’s resistance at about 50% of maximal strength, ensuring no knee movement for 8 seconds. Upon relaxation, the therapist further stretches the hamstrings to a mild or moderate sensation, maintaining this position for 30 seconds. This stretching routine is repeated three times each session [37] (Fig. 3). Afterward, a two-minute rest period was allowed before measuring the active hip flexion ROM.
Figure 3.
Traditional active hamstring stretching using hold-relax.
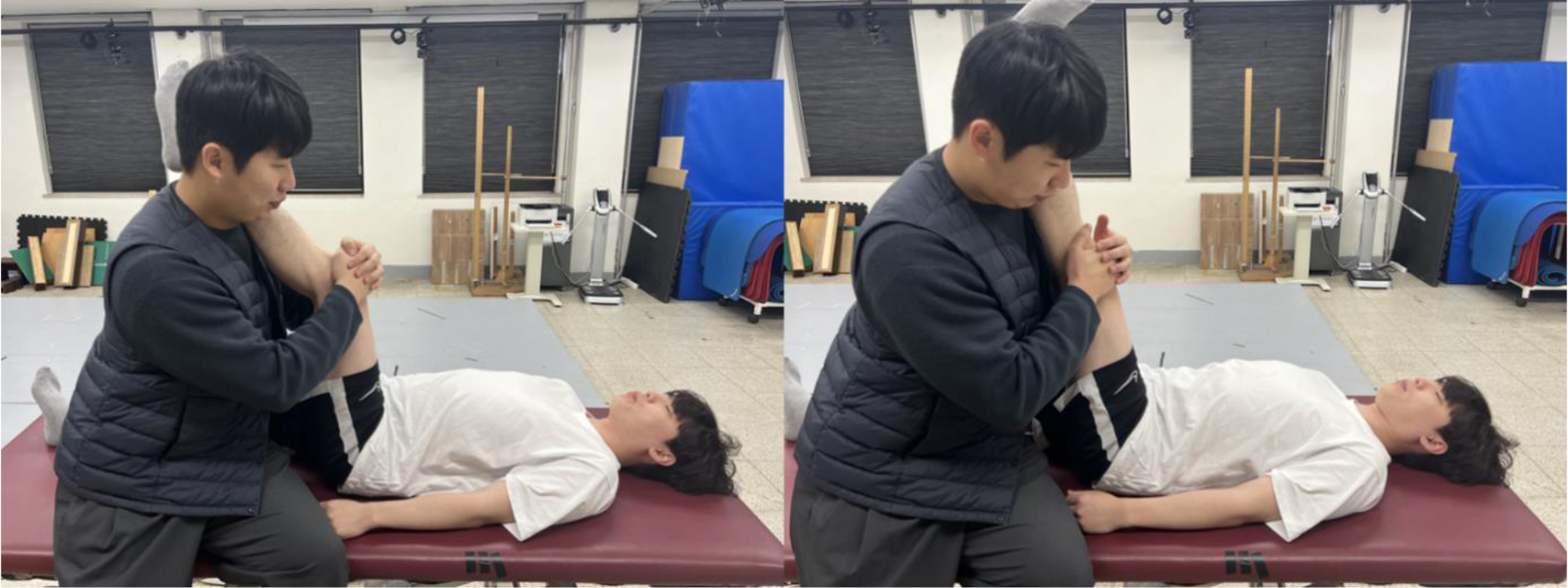
Figure 4.
Kinetic core chain (a) None-Kinetic core chain (b) Kinetic core chain.
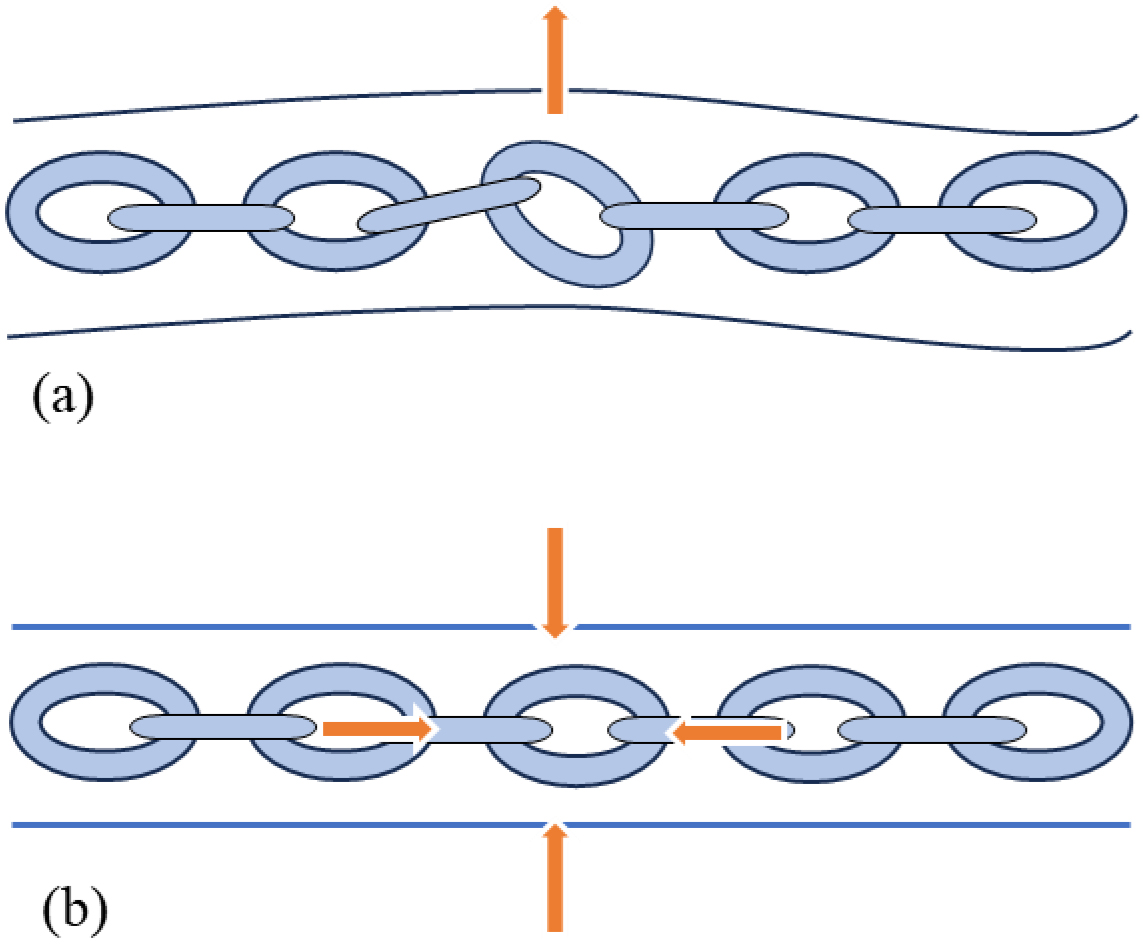
Figure 5.
Kinetic chain hamstring stretching.
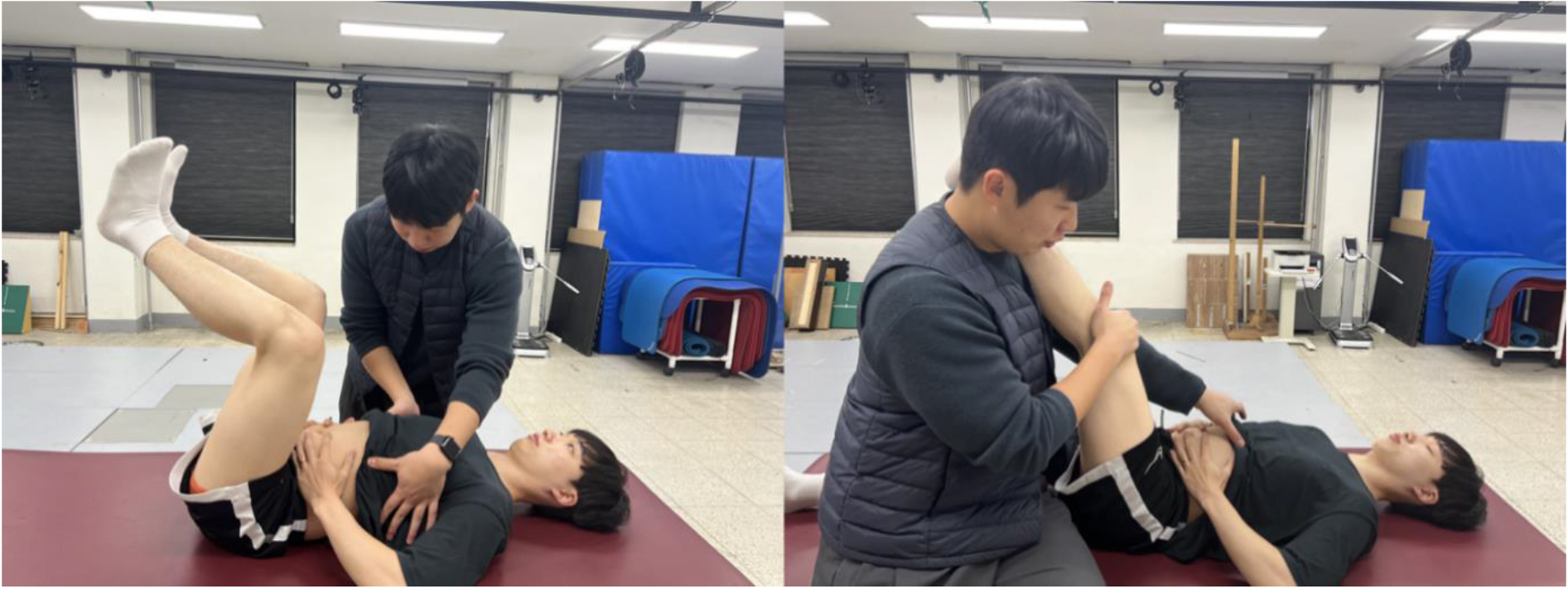
2.3.2Kinetic chain hamstring stretching
The KCS theory suggests that deep core stabilization muscles such as the diaphragm, transversus abdominis/internal oblique, multifidus, and pelvic floor, create intra-abdominal pressure (IAP) during inspiration. This is stabilized by the reflexive coactivation of external stabilizers like the rectus abdominis, external obliques, and erector spinae [30] (Fig. 4). Core stabilization forces combine with external forces to manage stabilization and movement, particularly in response to external perturbations and dynamic limb movements [29]. In Group 2, participants followed the same protocol as Group 1, incorporating AHS and core chain stability exercises. During the test, participants were instructed to maintain a neutral chest position to allow the diaphragm to descend effectively and generate IAP. This IAP eccentrically activates the transversus abdominis/internal oblique (TrA/IO), pelvic floor, multifidus, external oblique (EO), and other superficial muscles, subsequently concentrically stabilizing the spine in a cylindrical shape during dynamic movements of the extremities [27]. After mastering the technique, participants performed the ASLR three times while maintaining IAP through dynamic neuromuscular stabilization [25, 38]. For hamstring stretching with KCS, the investigator ensured neutral alignment of the thorax and rib cage, correcting any abnormalities like a flared rib cage. Participants were instructed to inhale to expand the ribcage and chest in multiple directions, creating intra-abdominal pressure through the co-activation of the diaphragm, TrA/IO, and pelvic floor muscles. The investigator focused on ensuring a symmetrical, cylindrical chest expansion by observing the anterior expansion of the xiphoid process, lateral expansion of the 10–12 ribs, and posterior expansion of the angulus costae. This process involved widening the intercostal spaces caudally without upward rib motion in the transverse plane [39] (Fig. 5). Afterward, a two-minute rest period was allowed before measuring the active hip flexion ROM.
2.4Statistical analysis
We conduct power analyses using the G-power software (ver. 3.1.9.7; Franz Faul, Kiel University, Kiel, Germany) and obtained a sample size of 18 from a pilot study to achieve a power of 0.95 and an effect size of 0.5 with an alpha level of 0.05. Data were presented as mean and standard deviation. We tested the normality of the variables using the Shapiro-Wilk test. All variables were normally distributed. We used the paired
3.Results
3.1Demographic characteristics
We recruited 18 participants, and each participant was randomly allocated using a random number table to either the AHS or KCS group. The independent
Table 1
Demographic characteristics of the participants (
Variables | Mean | |
---|---|---|
Age (years) | 25.01 | |
Height (cm) | 172.39 | |
Weight (kg) | 71.48 | |
Body mass index (kg/m2) | 23.96 |
a Values are presented as mean
Table 2
Active hip flexion range of motion data during straight leg raise test (
Hip flexion (∘) | AHS ( | KCS ( | ||
---|---|---|---|---|
Pre-test | 40.48 | 45.41 | 0.16 | |
Post-test | 53.46 | 61.29 |
| 0.02* |
|
|
Note. Values are expressed as the mean
3.2Active hip flexion range of motion test data
The independent
3.3Muscle activation data
The independent
Table 3
Muscle activation data (
Characteristics | AHS ( | KCS ( | ||
Lt. RA (% MVIC) | ||||
Pre-test | 3.97 | 3.67 | 0.22 | 0.83 |
Post-test | 3.78 | 5.61 | 0.17 | |
0.45 (0.66) |
| |||
Rt. RA (% MVIC) | ||||
Pre-test | 3.88 | 4.54 | 0.68 | |
Post-test | 4.84 | 5.81 | 0.57 | |
Lt. EO (% MVIC) | ||||
Pre-test | 10.61 | 9.21 | 0.61 | 0.55 |
Post-test | 9.40 | 18.61 |
| 0.01* |
1.20 (0.26) |
| |||
Rt. EO (% MVIC) | ||||
Pre-test | 8.76 | 10.95 | 0.53 | |
Post-test | 10.9 | 18.2 | 0.17 | |
Lt. IO (% MVIC) | ||||
Pre-test | 7.66 | 6.18 | 1.09 | 0.29 |
Post-test | 5.58 | 13.63 |
| 0.04* |
1.62 (0.15) |
| |||
Rt. IO (% MVIC) | ||||
Pre-test | 6.55 | 6.05 | 0.24 | 0.81 |
Post-test | 7.46 | 14.41 | 0.13 | |
Lt. ES (% MVIC) | ||||
Pre-test | 7.72 | 4.55 | 1.57 | 0.14 |
Post-test | 3.08 | 4.97 | 0.11 | |
2.53 (0.04*) | ||||
Rt. ES (% MVIC) | ||||
Pre-test | 7.20 | 5.75 | 0.91 | 0.37 |
Post-test | 4.36 | 6.16 | 0.27 | |
3.15 (0.01*) | ||||
BF (% MVIC) | ||||
Pre-test | 2.08 | 2.41 | 0.55 | |
Post-test | 1.97 | 2.24 | 0.64 | |
0.72 (0.49) | 0.98 (0.38) | |||
RF (% MVIC) | ||||
Pre-test | 32.68 | 26.87 | 0.784 | 0.44 |
Post-test | 32.01 | 23.39 | 1.167 | 0.26 |
0.41 (0.69) | 2.00 (0.08) |
Note. Values are expressed as the mean
Table 4
Overall satisfaction with the duration of the effect (
Overall satisfaction | Total ( | KCS ( | AHS ( |
| ||||
---|---|---|---|---|---|---|---|---|
N | % | N | % | N | % | |||
Strongly satisfied | 4 | 22 | 4 | 45 | 10.2 | 0.04 | ||
Satisfied | 5 | 28 | 3 | 33 | 2 | 22 | ||
Neutral | 1 | 6 | 1 | 11 | ||||
Dissatisfied | 4 | 22 | 1 | 11 | 3 | 33 | ||
Strongly dissatisfied | 4 | 22 | 4 | 45 |
Note. Strongly satisfied
3.4Post-intervention survey
We observed a statistically significant difference between the KCS and AHS groups in terms of overall satisfaction with the duration of effects (Table 4).
4.Discussion
The present study demonstrated the effects of hip flexion movement with and without kinetic chain core stabilization exercise on active hip flexion movement and muscle activation patterns in the RA, EO, IO, ES, BF, and RF in non-symptomatic participants with hamstring tightness. Our findings support our hypothesis, revealing a greater augmentation in hip flexion AROM and intricate muscle activation patterns within the KCS group, in comparison to the control group. One of the distinctive contributions of our study lies in the novelty of exploring the therapeutic effects of KCS on AROM and EMG activation in non-symptomatic individuals with hamstring tightness. The dearth of comprehensive comparative data within existing literature limits direct comparisons. Nonetheless, our research offers a new dimension to this discussion, shedding light on the potential merits of KCS.
The post-intervention assessment of hip flexion AROM illustrated a more pronounced enhancement in the KCS group compared to the AHS group. While a multitude of studies have examined the outcomes of static and traditional stretching methods, the exploration of the impact of core exercises and stretching remains relatively sparse [18, 40, 41]. Our findings are in agreement with those of previous studies [42, 43, 44]. Rad et al. documented a considerable increase in hip flexion range of motion (29.3%) following 24 sessions of core stability exercises in non-symptomatic adults, surpassing the changes observed in the non-intervention control [44]. Cho et al. reported a positive effect of 4-week core exercises on hip AROM and pain in 30 patients with chronic LBP [43]. Similarly, core stabilization training using the pilates exercise enhanced lumbar flexion (23.5%) and extension (23.86%) in patients with LBP [44]. The positive shifts observed in AROM may be intrinsically linked to the establishment of proximal core stability, furnishing a robust foundation for dynamic flexion movements within the lumbo-pelvic-hip complex. Kibler et al. observed that baseball throwing performance (e.g., speed, angular velocity, and power) remarkably improves when core stabilization is achieved prior to upper-extremity throwing [29]. Such enhanced kinetic chain movement hinges upon the quality of neuromuscular coordination, with implications for regional, global, and local anatomical and biomechanical parameters essential for lumbopelvic stability, ultimately enriching hip flexion movement [29, 38, 45].
The EMG muscle activity data demonstrated greater improvement in the bilateral EO (90.66%) and left IO (93.40%) during KCS than during AHS. These results were similar to those of previous studies [25, 26, 27, 38]. Park et al. reported a more “normalized core-pelvic-hip EMG muscle pattern” when facilitating underactive deep core stabilization, which provided a stable basis for active hip movement [27]. Choi et al. reported substantial improvements in IO (168.90%) and EO (94.47%) muscle activation during or after a 2-week core stabilization program, resulting in better performance in upper extremity movement function [26]. Kolar et al. observed that automatic, reflexive core stabilization occurred when active shoulder or hip flexion movements were initiated in healthy adults but was impaired in individuals with chronic LBP [38]. Similarly, Yoon and You demonstrated coordinated core muscle activation in the diaphragm and TrA/IO muscles (MVIC 11-18%) following core stabilization exercise in both the healthy and LBP groups, contributing to dynamic limb movement [25]. Such enhanced core muscle stabilization activation in the EMG data plays an important role in improving hip movement [27]. It is plausible that when the ipsilateral hip-supporting zone contacts the surface, it activates the opposite OB chain muscles [29, 38, 46]. These anterior OB chains may be counterbalanced by the posterior chain muscles to upright and stabilize all spinal segments during dynamic hip movement, as in the SLR, producing an external (negative) stabilizing force movement. Internally, the IAP force is concurrently generated by a deep diaphragm-TrA-pelvic floor-multifidus proximal core chain during inspiration, which provides a counterforce to stiffen the KCS chain [29, 38]. The simultaneous synergetic force coupling action between the internal and external KCS chains renders a crucial foundation for distal extremity muscles, such as the hamstrings to be activated in coordination with the EO and IO during active hip flexion [46, 47, 48, 49, 50, 51].
A post-intervention survey was conducted following the 4 weeks of intervention Notably, the majority of participants in the KCS group reported a sustained effectiveness in hip joint movement or hamstring flexibility through the employment of KCS. Conversely, those in the AHS group reported limited lasting effects. The current survey results are similar to those described in previous studies [52, 53, 54]. Knappstein et al. observed that after hamstring PNF stretching, ROM increases reportedly lasting for 7 minutes [52]. Similar to other studies, changes in ROM following PNF have only been observed for a maximum of 10 min following stretching [53, 54]. A single passive stretching session does not permanently deform the muscle-tendon unit because the increase in joint ROM is only momentary [55]. Alternatively, some authors have proposed that acute ROM changes result from distorted stretch perception [56]. This can be explained by the immediate effects of increased hamstring muscle elasticity and nerve tissue extensibility [40]. Moreover, core stability offers a number of advantages, as the proximal and distal segments cooperate to produce and control forces to maximize motor function. The stability and stiffness of the spine are significantly influenced by increased intra-abdominal pressure caused by the diaphragm’s contraction [29, 57]. Most importantly, during “involuntary” subconsciousness, the subcortical motor control network is selectively activated [58]. The regulation of respiration observed during the core stabilization exercise may be explained by this subcortical network [59]. This interplay highlights the importance of core stability within the realm of involuntary or automatic stability paradigms. Furthermore, this stretching paradigm contributes to a nuanced understanding of the intricate control mechanisms driving core stabilization and its role as an effective stretching method for both acute and sustained flexibility.
A few limitations of this study should be considered in the future. First, the absence of IAP measurements, which necessitate a coordinated co-activation of various muscles, remains a noteworthy omission. Further research employing noninvasive pressure sensors may shed light on this aspect. Second, the exclusive inclusion of male participants warrants consideration, highlighting cultural sensitivities. Generalizing these findings to diverse pathological populations necessitates cautious interpretation. Third, the ultrasound was not used to ensure the descending movement of the diaphragm which is associated with spinal stabilization-induced IAP via coordinated auxiliary activation of the diaphragm, transverse abdominis, multifidus, pelvic floor, internal oblique, and external oblique muscles. However, we have used manual palpation to monitor the appropriate activation of these core muscles. Fourth, we included only individuals with hamstring tightness and excluded those with hamstring shortness. Hamstring tightness is operationally defined as reaching the end range of motion, while failure to reach the end range is considered hamstring shortness [60, 61]. In the following study, it is essential to recruit a diverse group of subjects, including those with muscle shortness and hamstring tightness, to effectively evaluate the efficacy of KCS in a range of neuromuscular conditions. Nevertheless, the post-interventional survey confirmed a more effective sustainability of the KCS, with its therapeutic effects lasting longer compared to that of the control group.
5.Conclusions
This empirical investigation has unveiled significant insights into the realm of hip flexion movement, both with and without kinetic chain core stabilization exercise, within individuals experiencing hamstring tightness. The study’s outcomes furnish clinical substantiation and augment our comprehension of the biomechanical intricacies inherent in kinetic chain core stabilization exercises. These findings offer invaluable contributions to the existing body of knowledge, particularly concerning the formulation of efficacious and enduring mobility exercises tailored to individuals grappling with hamstring tightness. The empirical evidence presented herein not only underscores the potential therapeutic efficacy of kinetic chain core stabilization exercises but also address the need for further research and practical applications in this domain.
Acknowledgments
This study was supported by (1) the Korea Health Industry Development Institute, grant (No. HI18C1687000020); (2) the Brain Korea 21 PLUS Project (Grant No. 2021-51-0151); (3) the Institute of Information & Communications Technology Planning & Evaluation (IITP) funded by the Korean government (MSIT) (Grant No. 20200011290022003); (4) the National Research Foundation of Korea (Grant No. RS-2023-00221762; No. 2022RIS-005); and (5) the Institute for Project-Y Seed Grant of 2023 (Grant No. 2023-22-0277).
Conflict of interest
None to report.
References
[1] | Smith LR, Lee KS, Ward SR, Chambers HG, Lieber RL. Hamstring contractures in children with spastic cerebral palsy result from a stiffer extracellular matrix and increased in vivo sarcomere length. The Journal of Physiology. (2011) ; 589: (10): 2625-39. |
[2] | Anandhraj J, Kumaresan A. Effect of neurodynamic sliding technique on hemiplegic stroke subjects with hamstring tightness. Indian Journal of Public Health Research & Development. (2020) ; 11: (1): 251-4. |
[3] | Lumbroso D, Ziv E, Vered E, Kalichman L. The effect of kinesio tape application on hamstring and gastrocnemius muscles in healthy young adults. Journal of Bodywork and Movement Therapies. (2014) ; 18: (1): 130-8. |
[4] | Elliott MC, Zarins B, Powell JW, Kenyon CD. Hamstring muscle strains in professional football players: A 10-year review. The American Journal of Sports Medicine. (2011) ; 39: (4): 843-50. |
[5] | Witvrouw E, Mahieu N, Danneels L, McNair P. Stretching and injury prevention: An obscure relationship. Sports Medicine. (2004) ; 34: : 443-9. |
[6] | Levangie PK, Norkin CC. Joint structure and function: a comprehensive analysis: FA Davis; (2011) . |
[7] | Sahrmann S, Azevedo DC, Van Dillen L. Diagnosis and treatment of movement system impairment syndromes. Brazilian Journal of Physical Therapy. (2017) ; 21: (6): 391-9. |
[8] | Vleeming A, Schuenke M, Masi A, Carreiro J, Danneels L, Willard F. The sacroiliac joint: An overview of its anatomy, function and potential clinical implications. Journal of Anatomy. (2012) ; 221: (6): 537-67. |
[9] | Distefano LJ, Blackburn JT, Marshall SW, Padua DA. Gluteal muscle activation during common therapeutic exercises. Journal of Orthopaedic & Sports Physical Therapy. (2009) ; 39: (7): 532-40. |
[10] | Page P. Current concepts in muscle stretching for exercise and rehabilitation. International Journal of Sports Physical Therapy. (2012) ; 7: (1): 109. |
[11] | Cheatham SW, Kolber MJ, Cain M, Lee M. The effects of self-myofascial release using a foam roll or roller massager on joint range of motion, muscle recovery, and performance: A systematic review. International Journal of Sports Physical Therapy. (2015) ; 10: (6): 827. |
[12] | McKenzie R, May S. Mechanical diagnosis and therapy: the lumbar spine. Waikanae, New Zealand: Spinal Publications. (2003) . |
[13] | Croisier J-L. Factors associated with recurrent hamstring injuries. Sports Medicine. (2004) ; 34: : 681-95. |
[14] | Österman K, Schlenzka D, Poussa M, Seitsalo S, Virta L. Isthmic spondylolisthesis in symptomatic and asymptomatic subjects, epidemiology, and natural history with special reference to disk abnormality and mode of treatment. Clinical Orthopaedics and Related Research (1976–2007). (1993) ; 297: : 65-70. |
[15] | Saraste H. Long-term clinical and radiological follow-up of spondylolysis and spondylolisthesis. Journal of Pediatric Orthopedics. (1987) ; 7: (6): 631-8. |
[16] | Behm DG, Blazevich AJ, Kay AD, McHugh M. Acute effects of muscle stretching on physical performance, range of motion, and injury incidence in healthy active individuals: A systematic review. Appl Physiol Nutr Metab. (2016) ; 41: (1): 1-11. |
[17] | Decoster LC, Cleland J, Altieri C, Russell P. The effects of hamstring stretching on range of motion: A systematic literature review. Journal of Orthopaedic & Sports Physical Therapy. (2005) ; 35: (6): 377-87. |
[18] | Puentedura EJ, Huijbregts PA, Celeste S, Edwards D, In A, Landers MR, Fernandez-de-Las-Penas C. Immediate effects of quantified hamstring stretching: Hold-relax proprioceptive neuromuscular facilitation versus static stretching. Physical Therapy in Sport. (2011) ; 12: (3): 122-6. |
[19] | Westwater-Wood S, Adams N, Kerry R. The use of proprioceptive neuromuscular facilitation in physiotherapy practice. Physical Therapy Reviews. (2010) ; 15: (1): 23-8. |
[20] | Halbertsma JP, van Bolhuis AI, Göeken LN. Sport stretching: Effect on passive muscle stiffness of short hamstrings. Archives of Physical Medicine and Rehabilitation. (1996) ; 77: (7): 688-92. |
[21] | Hindle K, Whitcomb T, Briggs W, Hong J. Proprioceptive neuromuscular facilitation (PNF): Its mechanisms and effects on range of motion and muscular function. Journal of Human Kinetics. (2012) ; 31: (2012): 105-13. |
[22] | Manoel ME, Harris-Love MO, Danoff JV, Miller TA. Acute effects of static, dynamic, and proprioceptive neuromuscular facilitation stretching on muscle power in women. The Journal of Strength & Conditioning Research. (2008) ; 22: (5): 1528-34. |
[23] | Nelson RT, Bandy WD. Eccentric training and static stretching improve hamstring flexibility of high school males. Journal of Athletic Training. (2004) ; 39: (3): 254. |
[24] | Weppler CH, Magnusson SP. Increasing muscle extensibility: A matter of increasing length or modifying sensation? Physical Therapy. (2010) ; 90: (3): 438-49. |
[25] | Lee J, Kim D, Shin Y, Yi C, Jeon H, You SJH, Park C. Comparison of core stabilization techniques on ultrasound imaging of the diaphragm, and core muscle thickness and external abdominal oblique muscle electromyography activity. J Back Musculoskelet Rehabil. (2022) ; 35: (4): 839-47. |
[26] | Choi H, Park C, Hwang J, You JSH. Neuromuscular mechanisms and effects of core stabilizations on trunk and hip muscle activity during lifting movement. Journal of Mechanics in Medicine and Biology. (2021) ; 21: (09): 2140042. |
[27] | Park H, Park C. Restoration of the Broken Lumbopelvic-hip Neuromuscular Chain and Coordinated Synergistic Activation in Low Back Pain. \CJKUTF8mj한국전문물리치료학회지. (2022) ; 29: (3): 215-24. |
[28] | Liebenson C. Rehabilitation of the spine: a practitioner’s manual: Lippincott Williams & Wilkins; (2007) . |
[29] | Kibler WB, Press J, Sciascia A. The role of core stability in athletic function. Sports Medicine. (2006) ; 36: : 189-98. |
[30] | KOLÁŘ P, Neuwirth J, Šanda J, Suchanek V, Svata Z, Volejnik J, Pivec M. Analysis of diaphragm movement during tidal breathing and during its activation while breath holding using MRI synchronized with spirometry. Physiological Research. (2009) ; 58: (3). |
[31] | Gajdosik RL, Albert CR, Mitman JJ. Influence of hamstring length on the standing position and flexion range of motion of the pelvic angle, lumbar angle, and thoracic angle. Journal of Orthopaedic & Sports Physical Therapy. (1994) ; 20: (4): 213-9. |
[32] | Neto T, Jacobsohn L, Carita AI, Oliveira R. Reliability of the active-knee-extension and straight-leg-raise tests in subjects with flexibility deficits. Journal of Sport Rehabilitation. (2015) ; 24: (4). |
[33] | Yıldırım M, Ozyurek S, Tosun O, Uzer S, Gelecek N. Comparison of effects of static, proprioceptive neuromuscular facilitation and Mulligan stretching on hip flexion range of motion: A randomized controlled trial. Biology of Sport. (2016) ; 33: (1): 89-94. |
[34] | Lin NCJ, Hayward KS, D’Cruz K, Thompson E, Li X, Lannin NA. Validity and reliability of a smartphone inclinometer app for measuring passive upper limb range of motion in a stroke population. Disability and Rehabilitation. (2020) ; 42: (22): 3243-9. |
[35] | McGill SM, Marshall LW. Kettlebell swing, snatch, and bottoms-up carry: Back and hip muscle activation, motion, and low back loads. The Journal of Strength & Conditioning Research. (2012) ; 26: (1): 16-27. |
[36] | Rowlands AV, Marginson VF, Lee J. Chronic flexibility gains: Effect of isometric contraction duration during proprioceptive neuromuscular facilitation stretching techniques. Research Quarterly for Exercise and Sport. (2003) ; 74: (1): 47-51. |
[37] | Ghanbari A, Ebrahimian M, Mohamadi M, Najjar-Hasanpour A. Comparing hold relax-proprioceptive neuromuscular facilitation and static stretching techniques in management of hamstring tightness. Indian Journal of Physiotherapy and Occupational Therapy. (2013) ; 7: (1): 126. |
[38] | Frank C, Kobesova A, Kolar P. Dynamic neuromuscular stabilization & sports rehabilitation. International Journal of Sports Physical Therapy. (2013) ; 8: (1): 62. |
[39] | Cholewicki J, McGill SM. Mechanical stability of the in vivo lumbar spine: Implications for injury and chronic low back pain. Clinical Biomechanics. (1996) ; 11: (1): 1-15. |
[40] | Jeong E-D, Kim C-Y, Kim N-H, Kim H-D. Immediate effects of static and proprioceptive neuromuscular facilitation stretching of hamstring muscles on straight leg raise, craniovertebral angle, and cervical spine range of motion in neck pain patients with hamstring tightness: A prospective randomized controlled trial. Journal of Back and Musculoskeletal Rehabilitation. (2022) ; 35: (2): 429-38. |
[41] | Rubini EC, Souza AC, Mello ML, Bacurau RF, Cabral LF, Farinatti PT. Immediate effect of static and proprioceptive neuromuscular facilitation stretching on hip adductor flexibility in female ballet dancers. J Dance Med Sci. (2011) ; 15: (4): 177-81. |
[42] | Bhadauria EA, Gurudut P. Comparative effectiveness of lumbar stabilization, dynamic strengthening, and Pilates on chronic low back pain: Randomized clinical trial. Journal of Exercise Rehabilitation. (2017) ; 13: (4): 477. |
[43] | Cho HY, Kim EH, Kim J. Effects of the CORE exercise program on pain and active range of motion in patients with chronic low back pain. J Phys Ther Sci. (2014) ; 26: (8): 1237-40. |
[44] | Mohebbi Rad Y, Fadaei Chafy MR, Elmieh A. Is the novel suspension exercises superior to core stability exercises on some EMG coordinates, pain and range of motion of patients with disk herniation? Sport Sciences for Health. (2021) ; 1-11. |
[45] | Burkhart SS, Morgan CD, Kibler WB. The disabled throwing shoulder: Spectrum of pathology Part I: Pathoanatomy and biomechanics. Arthroscopy: The Journal of Arthroscopic & Related Surgery. (2003) ; 19: (4): 404-20. |
[46] | Zemková E. Strength and power-related measures in assessing core muscle performance in sport and rehabilitation. Frontiers in Physiology. (2022) ; 13: : 786. |
[47] | Hodges P, Holm AK, Holm S, Ekström L, Cresswell A, Hansson T, Thorstensson A. Intervertebral stiffness of the spine is increased by evoked contraction of transversus abdominis and the diaphragm: In vivo porcine studies. Spine. (2003) ; 28: (23): 2594-601. |
[48] | McGill S, Norman RW. Reassessment of the role of intra-abdominal pressure in spinal compression. Ergonomics. (1987) ; 30: (11): 1565-88. |
[49] | McGill S, Juker D, Kropf P. Appropriately placed surface EMG electrodes reflect deep muscle activity (psoas, quadratus lumborum, abdominal wall) in the lumbar spine. Journal of Biomechanics. (1996) ; 29: (11): 1503-7. |
[50] | Leetun DT, Ireland ML, Willson JD, Ballantyne BT, Davis IM. Core stability measures as risk factors for lower extremity injury in athletes. Medicine & Science in Sports & Exercise. (2004) ; 36: (6): 926-34. |
[51] | Willson JD, Dougherty CP, Ireland ML, Davis IM. Core stability and its relationship to lower extremity function and injury. JAAOS-Journal of the American Academy of Orthopaedic Surgeons. (2005) ; 13: (5): 316-25. |
[52] | Knappstein A, Stanley S, Whatman C. Range of motion immediately post and seven minutes post, PNF stretching. New Zealand Journal of Sports Medicine. (2004) ; 32: : 42-7. |
[53] | Behm DG, Plewe S, Grage P, Rabbani A, Beigi HT, Byrne JM, Button DC. Relative static stretch-induced impairments and dynamic stretch-induced enhancements are similar in young and middle-aged men. Applied Physiology, Nutrition, and Metabolism. (2011) ; 36: (6): 790-7. |
[54] | Whatman C, Knappstein A, Hume P. Acute changes in passive stiffness and range of motion post-stretching. Physical Therapy in Sport. (2006) ; 7: (4): 195-200. |
[55] | Opplert J, Babault N. Acute effects of dynamic stretching on muscle flexibility and performance: an analysis of the current literature. Sports Medicine. (2018) ; 48: : 299-325. |
[56] | Magnusson SP, Simonsen EB, Aagaard P, Dyhre-Poulsen P, McHugh MP, Kjaer M. Mechanical and physiological responses to stretching with and without preisometric contraction in human skeletal muscle. Archives of Physical Medicine and Rehabilitation. (1996) ; 77: (4): 373-8. |
[57] | Hodges PW, Eriksson AM, Shirley D, Gandevia SC. Intra-abdominal pressure increases stiffness of the lumbar spine. Journal of Biomechanics. (2005) ; 38: (9): 1873-80. |
[58] | Kim DH, Lee JJ, Han BS, You JH. Cortical or subcortical neural networks during dynamic neuromuscular core stabilization: A fMRI blood oxygen-level dependent (bold) analysis. Journal of Medical Imaging and Health Informatics. (2016) ; 6: (7): 1732-4. |
[59] | Kim DH, Lee JJ, You SJH. Best core stabilization exercise to facilitate subcortical neuroplasticity: A functional MRI neuroimaging study. Technology and Health Care. (2018) ; 26: (3): 401-7. |
[60] | Baker RT, Hansberger BL, Warren L, Nasypany A. A novel approach for the reversal of chronic apparent hamstring tightness: A case report. International Journal of Sports Physical Therapy. (2015) ; 10: (5): 723. |
[61] | Hansberger BL, Loutsch R, Hancock C, Bonser R, Zeigel A, Baker RT. Evaluating the relationship between clinical assessments of apparent hamstring tightness: A correlational analysis. International Journal of Sports Physical Therapy. (2019) ; 14: (2): 253. |