Effects of load increase on lower extremity kinetic and kinematic variables in the back squat exercise
Abstract
BACKGROUND:
Squats are one of the most widely used weight training methods worldwide, and the single most ubiquitous with regard to multi-joint resistance training.
OBJECTIVE:
The objective of the present study was to investigate kinematic and kinetic changes in the lower extremities as a result of load increases during a back squat exercise, and to propose an association between back squats and lower extremity injuries.
METHODS:
Eight individuals with experience of back squat training were recruited. The subjects performed back squats with loads of 25%, 50%, 100%, and 125% of their body weight. During the performance, the center of pressure (COP) sway; vertical center of mass (COM) velocity; joint moment; joint range of motion (ROM) of flexion/extension and adduction/abduction; and rotation of the ankle, knee, and hip joints were measured.
RESULTS:
The participants’ lower extremity joint ROM, vertical COM velocity, and COP variability did not change significantly with changes in weight loading. However, the moments applied to the lower extremity joints differed according to changes in barbell weight. The moments of plantar flexion (
CONCLUSION:
As the barbell load increased, posture and performance were maintained, but rotational moments of the joints differed. It is expected that the joint directions that showed significant differences in this study are likely to be vulnerable to the risk of injury when an excessive load is applied to the body. Examples include the hip adduction moment, hip external rotation moment, and ankle internal rotation moment, and apply regardless of the increase in the rotational moments of joints from load increases.
1.Introduction
Squats are one of the most widely used weight training methods worldwide, and the single most ubiquitous with regard to multi-joint resistance training [1]. Squats are generally performed by moving the weight load through eccentric flexion and concentric extension exercises of the musculoskeletal system of the lower extremities, with both feet fixed to the ground [2]. It is possible in this way to strengthen the muscles around the knee and hip joints, as well as the lower back [3] because squats activate the quadriceps, hamstrings, gluteus maximus, and soleus [4]. The most notable benefit of squat exercises is that they can be performed without any additional weight load, at any time during everyday life. Training intensity can also be easily adjusted by varying the weight of the barbell or the depth of the squat. For these reasons, squats are regularly utilized by athletes and the general population as a means of lower body strengthening or even rehabilitation [5].
The intensity of a squat exercise is determined by the squat depth and weight load [6]. The most popular and primary method of resistance training for performance improvement is the use of heavier weights [7]. Naturally, the heavier the weight, the greater the risk of injury. Barbell weight and squat depth significantly affect the extensor muscles of the hips, knees, and ankles. A study by Bryanton et al. highlighted the necessity of strengthening hip joint extensors and ankle dorsiflexors when increasing weight [8]. The risk of injury in the knee areas is particularly high when performing barbell squats [9], making strengthening and conditioning essential [10]. Squat motions must be performed correctly to reduce the risk of knee and back injuries [11]. The biomechanical elements of barbell squats are associated with high levels of knee flexion, which increases the likelihood of injury to the knee joint itself [3]. This is because the greater joint moment is a potential factor for injury [8].
Previous studies on conventional squat weight have focused on kinematic analysis of squat techniques, interpretation of moments applied to the lower extremities, and analysis of the contribution of each joint, while varying the trial set and barbell by a ratio of 1-RM, 3-RM, or weight percentage. These studies, which analyzed the load on the patellar and patellar tendons at 40, 50, 60, and 70% of 1-RM [10], revealed the extent of the contribution of the hip joint moments by using back squat load conditions of 90%, 100%, and 102% of 1-RM. This identified the importance of hip extensor moments at maximum load [12]. Appropriate positioning for injury prevention was also determined by calculating segmental moments while adjusting the stance width and foot angle [13]. However, the criteria of 1-RM or 3-RM used in these studies may have varied depending on the condition and ability of the person at the time the exercise was performed, while it is also necessary to establish a different standard for injuries.
Squats are assumed to produce equal joint torques bilaterally because a squat is a symmetrical motion on the frontal plane. However, depending on the posture, this may not be the case [14]. Several studies related to squats have demonstrated that individual factors generate greater moments in the joints of the lower extremities through inverse dynamics techniques. Prior studies related to injuries have been conducted in a variety of areas, including gait [15], jumping [16], landing [17], stair ascent [18], and squats [19, 20]. However, many studies have investigated only the voluntary moments of the lower extremities, namely the joint moments of the ankle, knee, and hip in the sagittal plane [20, 21, 22]. When a force is applied in a three-dimensional direction to the lower extremities in contact with the ground, particularly with regard to human movements, the moments on the joints also manifest in the three-axis direction. Consequently, it is difficult to obtain a complete set of information on the force exerted on the lower extremity joints by analyzing a single-axial moment or joint angle during a specific motion. Our hypothesis for this study was that the joint load on the three axes varies as the weight increases. Therefore, the objective of this study was to identify the factors that affect the lower extremities by measuring the joint moments and squat motions applied in three dimensions during a squat exercise.
2.Method
2.1Participants
Eight males (age: 22.25
2.2Experimental protocol
To obtain the values of the kinematic variables, eight Oqus 500 series cameras (Qualisys Inc., Sweden) and two Amti ground reflectors (Amti Inc., USA) were used. Motion data were sampled at 100 Hz, and force plate data at 1000 Hz. A combination of plates (2.5 kg, 5 kg, 10 kg, and 20 kg) and a bar (20 kg) were used for the squats.
The participants were instructed to perform back squats. A back squat is a squat method that involves placing a barbell on the trapezius and posterior deltoid muscles [23]. After being weighed, the participants were then instructed to lift 25%, 50%, 100%, and 125% of their body weight. The participants’ weights were established as the maximum loads, based on the method described in a previous study [24]. While performing the squats the participants were instructed to maintain their gaze directly ahead and to squat as deeply as possible within their potential range. The participants were asked to squat and stand three times consecutively for each load condition; the total completion time of the back squats was not controlled. This was because a similar rhythm exists without controlling the speed of performance [25]. The participants rested for two minutes for every change in the barbell load, with the barbell loads presented in a random order.
2.3Data analysis
Analysis of the changes in kinematic and kinetic variables of the lower extremities during the performance of the back squat with increasing load was conducted on the right leg, which was the dominant leg of each of the participants. COP sway, vertical comorbidity, ROM of the joint angles, and peak joint moment during the back squat operation were analyzed. Each analysis variable was calculated when the participants performed back squats with different loads of 25%, 50%, 100%, and 125% of their body weight (BW). 150% BW and 175% BW were also predicted to analyze the effect on the joints when applying excessive loads that could not be easily measured because of the possibility of creating excessive risk or dangerous situations during the experiment. The regression equation of each variable was calculated for load increases of 50–125% to predict the change that would occur in the loads over 125%.
The phase of the back squat motion designated for analysis was set between the beginning of the downward vertical displacement of the body center from the initial standing position, and the moment when the height of the body center passed the lowest point and returned to the starting position. All variables were analyzed using the data obtained within these phases.
The COP sway was calculated as the standard deviation of the COP trajectory during the task. The ROM of the joint angles and vertical COM velocity were analyzed for kinematic verification. The variables of the lower extremity joints were obtained in the frontal, transverse, and sagittal planes. The definitions of the rotation axes of the joints in each plane are shown in Fig. 1. The joint moments analyzed in this study were the peak joint moments during each phase. Joint moments were analyzed along the anterior-posterior axis in the frontal plane, medial-lateral axis in the sagittal plane, and vertical axis in the transverse plane. Two joint moments were analyzed at each axis: extension and flexion in the sagittal plane (abduction and adduction in the frontal plane/internal and external rotation in the transverse plane).
Figure 1.
Effect of load increase on joint moments at three joint axes. Only the significant results were presented as tables, and only the significant statistical results according to the load difference in each table were indicated (unit: Nm/kg).
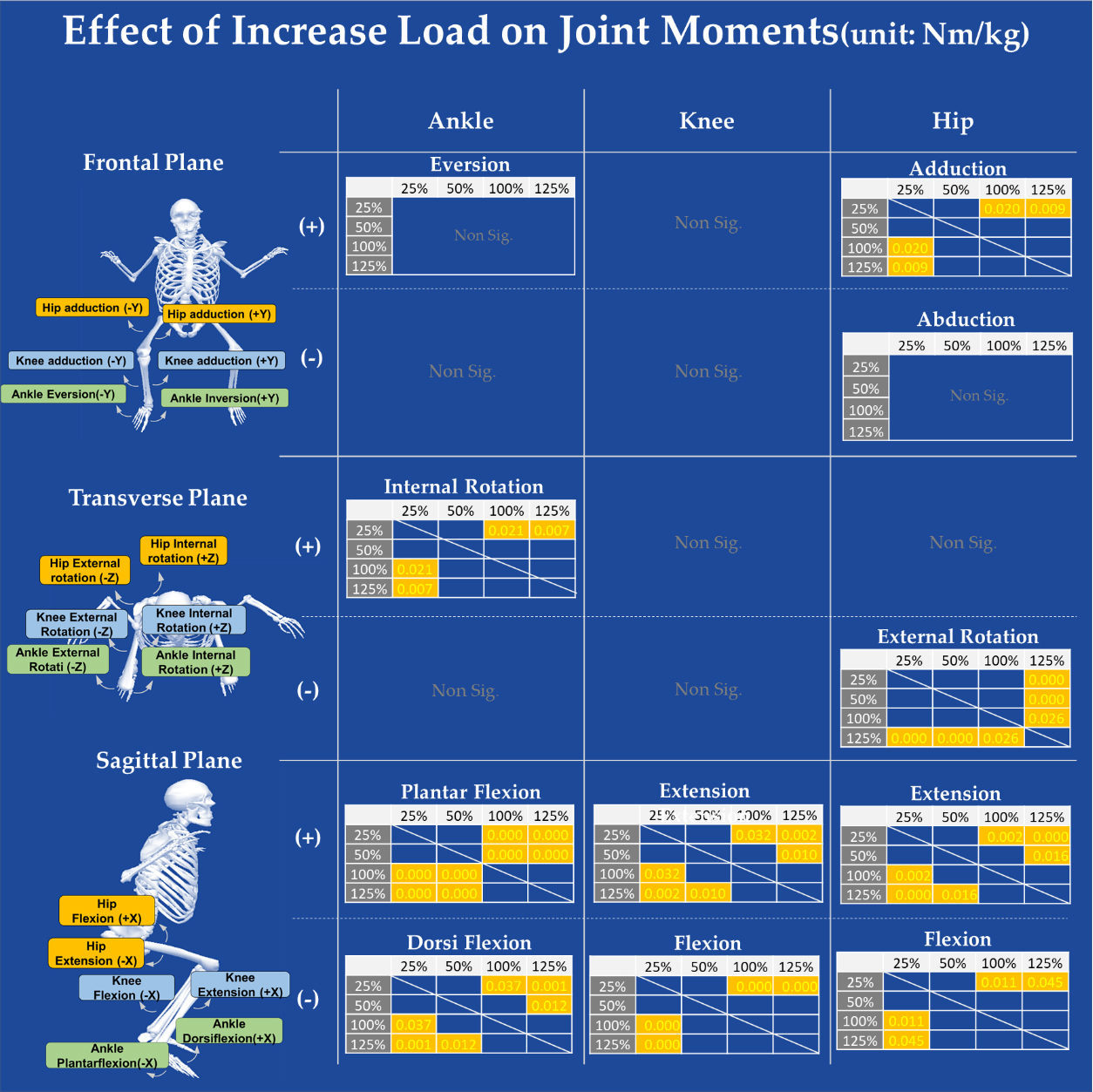
2.4Statistics
Analysis of variance (ANOVA) was performed to identify significant differences in each variable in line with load increases within the group. The Scheffe test was used for post hoc analysis, and the significance level was set at
3.Results
3.1COP and kinematic variables
The results of the analyses of the COP variability and kinematic variables in Table 1 describe the recorded back squat performance. The COP and joint ROM were analyzed to identify any changes in the movement of the lower extremities corresponding with load increase, along with the vertical COM velocity for the timing of back squat performance, and joint angles for the change in the range of movement of the lower extremity joints. None of these variables showed significant changes corresponding to increased load. These results demonstrate that there was no wavering in the movement, the timing was consistent, and the movement of the lower extremities did not differ significantly despite the increase in load.
Table 1
Kinematic and COP results of back squat
Movement | Parameter | %Body weight resistance |
|
| |||||||
---|---|---|---|---|---|---|---|---|---|---|---|
25% | 50% | 100% | 125% | ||||||||
COP sway | ML | 0.274 | 0.27 | 0.289 | 0.348 | 1.294 | 0.296 | ||||
(m) | AP | 1.651 | 1.825 | 2.269 | 2.132 | 2.492 | 0.081 | ||||
Vertical COM | Superior | 0.45 | 0.459 | 0.481 | 0.431 | 0.240 | 0.868 | ||||
velocity (m/s) | Inferior | 0.025 | 0.995 | ||||||||
Angles at | Ankle | 32.029 | 32.287 | 32.601 | 31.478 | 0.109 | 0.954 | ||||
sagittal plane | Knee | 97.837 | 98.328 | 95.162 | 92.916 | 1.221 | 0.321 | ||||
(degree) | Hip | 70.603 | 71.79 | 68.931 | 69.086 | 0.158 | 0.924 | ||||
Angles at | Ankle | 7.039 | 7.456 | 6.818 | 6.654 | 0.094 | 0.963 | ||||
frontal plane | Knee | 9.593 | 10.032 | 9.877 | 9.084 | 0.080 | 0.970 | ||||
(degree) | Hip | 16.474 | 17.410 | 15.266 | 16.108 | 0.183 | 0.907 | ||||
Angles at | Ankle | 13.288 | 12.517 | 12.748 | 12.399 | 0.215 | 0.885 | ||||
transverse | Knee | 23.715 | 23.443 | 20.946 | 19.916 | 1.048 | 0.387 | ||||
plane (degree) | Hip | 15.879 | 17.713 | 18.131 | 19.397 | 0.604 | 0.618 |
3.2Joint moments
A back squat is an exercise in which a person fixes both legs to the ground, holds a loaded barbell on the upper trapezius at the back of the neck, sits down, and stands up. The majority of voluntary movement of the lower extremities that occurs during sitting and standing movements takes place in the sagittal plane. As the load was increased during the back squat, significant differences were observed in all moments measured in the sagittal plane. However, among the valgus and varus moments seen on the frontal plane, significant differences were only seen in ankle adduction (
Table 2
Joint moment results of back squat
Movement plane | Joint | Moments | %Body weight resistance |
| Sig. | |||||||
---|---|---|---|---|---|---|---|---|---|---|---|---|
25% | 50% | 100% | 125% | |||||||||
Sagittal | Ankle | Plantar |
|
|
|
|
|
|
|
| 54.362 | 0.000 |
plane | Dorsi |
|
|
|
|
|
|
|
| 8.475 | 0.000 | |
(Nm/kg) | Knee | Flexion |
|
|
|
|
|
|
|
| 12.013 | 0.000 |
Extension | 1.294 |
| 1.400 |
| 1.747 |
| 1.925 |
| 8.581 | 0.000 | ||
Hip | Flexion | 0.057 |
|
|
|
|
|
|
| 5.111 | 0.006 | |
Extension |
|
|
|
|
|
|
|
| 11.053 | 0.000 | ||
Frontal | Ankle | Inversion | 2.813 | 0.057 | ||||||||
plane | Eversion |
|
|
|
|
|
|
|
| 5.612 | 0.004 | |
(Nm/kg) | Knee | Abduction | 1.764 | 0.177 | ||||||||
Adduction | 0.016 | 0.064 | 0.002 | 2.448 | 0.084 | |||||||
Hip | Abduction |
|
|
|
|
|
|
|
| 3.242 | 0.037 | |
Adduction | 0.474 |
| 0.732 |
| 0.963 |
| 1.011 |
| 5.846 | 0.003 | ||
Transverse | Ankle | External | 0.296 | 0.828 | ||||||||
plane | Internal | 0.159 |
| 0.200 |
| 0.314 |
| 0.336 |
| 7.043 | 0.001 | |
(Nm/kg) | Knee | External | 2.026 | 0.133 | ||||||||
Internal | 0.096 | 0.084 | 0.087 | 0.151 | 1.967 | 0.142 | ||||||
Hip | External |
|
|
|
|
|
|
|
| 11.070 | 0.000 | |
Internal | 0.173 | 0.269 | 0.310 | 0.222 | 0.275 | 0.843 |
Figure 2.
Time series data of ankle, knee, and hip joint moments (black line: 25% BW, blue line: 50% BW, red line: 100% BW, green line: 125% BW, dash line: event of full squat (around 57%)).
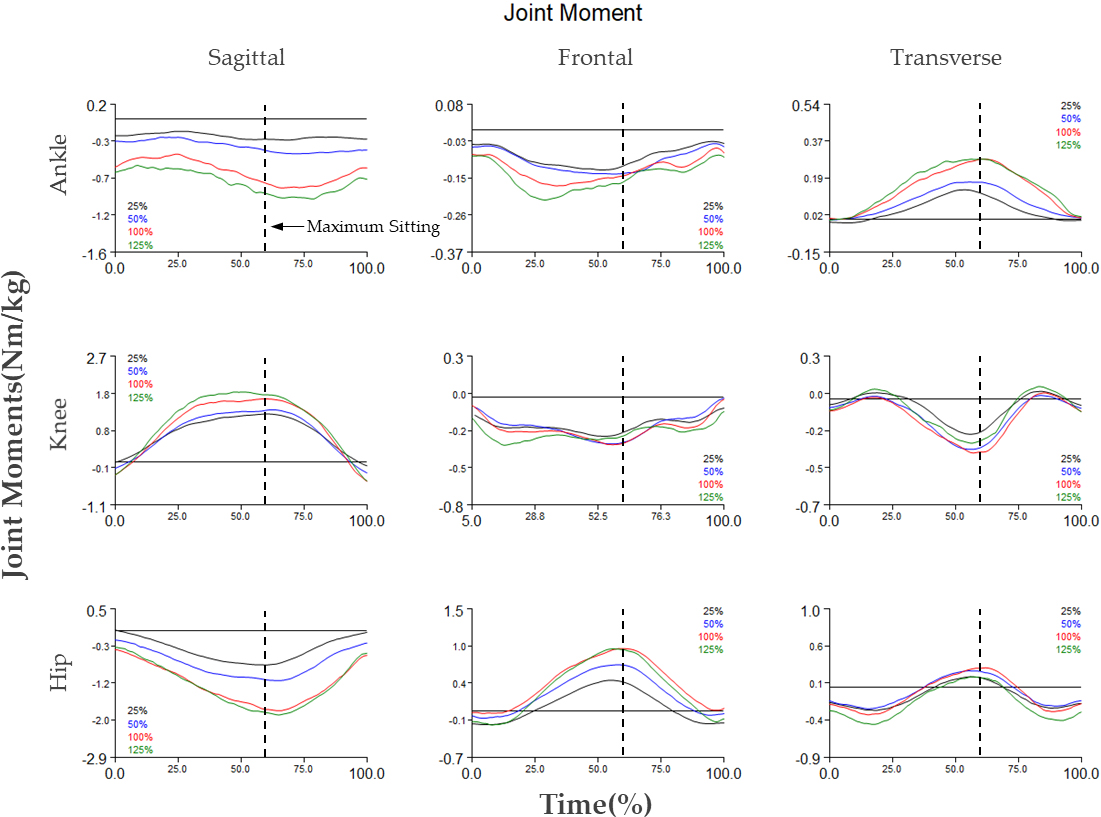
The results of the post-hoc analysis performed on the variables that showed significant differences in ANOVA are shown in Fig. 1. Most joint moments did not show differences at loads of 25% and 50%; however, most showed differences at loads of 100% or higher. Among the moments, the hip adduction moment and ankle eversion did not differ significantly in the post-hoc analysis.
3.3Variable prediction at over load back squat
Table 3
Results of regression analysis
Parameters | Equation | r square | BW 150% prediction | BW 175% prediction | ||
---|---|---|---|---|---|---|
Moments | ||||||
Sagittal plane | Ankle | Plantar |
| 0.819 |
|
|
Dorsi |
| 0.464 |
|
| ||
Knee | Flexion |
| 0.503 |
|
| |
Extension |
0.896x | 0.461 | 2.151 | 2.599 | ||
Hip | Flexion | 0.262 | – | – | ||
Extension |
| 0.520 |
|
| ||
Frontal plane | Ankle | Inversion | 0.367 | |||
Eversion | 0.211 | |||||
Knee | Abduction | 0.159 | – | – | ||
Adduction | 0.083x | 0.066 | ||||
Hip | Abduction | 0.003 | – | – | ||
Adduction | 0.736x | 0.359 | – | – | ||
Transverse plane | Ankle | External | 0.005x | 0.001 | – | – |
Internal |
0.258x | 0.402 | 0.414 | 0.543 | ||
Knee | External | 0.121 | – | – | ||
Internal | 0.067x | 0.082 | – | – | ||
Hip | External |
| 0.458 |
|
| |
Internal | 0.075x | 0.005 | – | – | ||
COP | ||||||
COP sway | ML | 0.097x | 0.091 | – | – | |
AP | 0.755x | 0.157 | – | – | ||
COM | ||||||
Vertical COM velocity | Superior | 0.001 | – | – | ||
Inferior | 0.014x | 0.002 | – | – | ||
Joint angles | ||||||
Flexion/extension | Ankle | 0.002 | – | – | ||
Knee | 0.098 | – | – | |||
Hip | 0.008 | – | – | |||
Abduction/adduction | Ankle | 0.014 | – | – | ||
Knee | 0.002 | – | – | |||
Hip | 0.004 | – | – | |||
Rotation | Ankle | 0.004 | – | – | ||
Knee | 0.093 | – | – | |||
Hip | 4.389x | 0.058 | – | – |
The kinetic and kinematic variables analyzed in the previous section were measured at 35% to 125% of the participants’ body weights. Based on the measured data, the results were predicted for each variable at loads of 150% and 175% (Table 3). As a result, the moment variables excluding hip flexion showed r-squared values of 0.4 or higher in the sagittal plane and 0.4 or higher for ankle internal rotation and hip external rotation on the transverse plane. However, the r-squared values of regression were less than 0.4 for moments, COP, and kinematic variables of the frontal plane.
4.Discussion
Squats vary in intensity depending on the weight load and squat depth [6]. A change in exercise intensity results in a larger or smaller load being placed on the muscles and joints. Proper loads help strengthen the muscles according to the principle of progressive overload. However, attempting to use loads beyond one’s physical ability, or performing squats with improper posture may result in injuries to the lower extremity joints. Even when squats are performed with a consistent posture, the load on the joint may vary depending on the structural dynamics, posture, and movement characteristics of the joint, which can lead to injury if the varying weight loads cannot be supported.
The experimental task of this study was to perform squats as deep as possible in the participants’ own rhythms under all load conditions. Kinematic analysis showed no significant differences in COP sway, joint angles, or COM velocity despite the increased load (Table 1). These results confirm that the back squat performance of the study’s participants was relatively consistent, regardless of the extent of the load increase. The results of the kinematic experiment were consistent with those of a previous study that reported a similar rhythm of movements, even when the speed of squats was not controlled [25].
In this study, it was possible to calculate and estimate the moments applied to the lower extremity joints according to each load condition, while largely excluding the influence of posture because the participants performed back squat movements correctly without breaking posture, regardless of the load conditions applied. The fact that the participants’ posture did not collapse implies that the moment arm factor could be excluded from the calculation of the moment. As shown in the joint moment results (Fig. 2), significant changes in moments occurred in all lower extremity joints (ankle, knee, and hip) in the sagittal plane as load increased (Table 2). These results closely matched with previous research findings [12]. This is partly consistent with other previous studies that showed that the increase in joint torque is caused by the inertia of the barbell load and extension of the hip joint angle. The results of this study also demonstrated an increase in flexion and extension moments in the sagittal plane, but did not record extension of the hip joint angle. These differences may be attributed to the fact that the current study used 125% of the participants’ weight as the maximum load and caused no excessive load. Participants in the previous studies cited performed squats at a maximum load of up to 102% of 1-RM based on the standard of 1-RM. No differences were recorded in the joint ROM or COP variability of the participants; the only differences found were in joint moments. This study confirmed that the joint moments varied according to the load applied, even in the same posture. According to the pattern of joint moment occurrence in the sagittal plane, the largest knee and hip moments occurred when the squat was at its deepest, or when the COM was near its lowest position. By contrast, in the case of ankle moments, the heavier the load, the larger the moments that occurred during ascent. This pattern suggests the need for greater ankle torque in order to maintain posture during the latter motions of the squat when the load increases.
The squat is undeniably a multi-joint exercise, with many studies having shown that it is an exercise with a high contribution from the hips, ankles, and knees. In support of these existing studies [12], the flexion, extension, and external rotation moments of the hip joint were shown to increase as the weight increased. In the knee joint, the flexion/extension moment increased significantly with an increase in weight, which can be a major factor in injury. This result is consistent with the findings of Gullett et al. [3]. Although the flexion/extension moments showed significant differences in all joints, the adduction/abduction (frontal plane) and internal/external (transverse plane) moments showed significant differences only in the ankle and hip joints. In the case of the ankles, there were significant differences in the eversion and internal rotation moments. Examination of the occurrence pattern of the moment during a squat revealed that the internal rotation of the ankle on the transverse plane increased during the descent, and decreased during the ascent of a squat as the load increased. The ankle eversion moment on the frontal plane was at its maximum before reaching the lowest squat position when using a light load condition. However, the maximum moment occurred during the ascent as the load increased. From these results, it can be concluded that as the load increases, the use of the internal rotator muscle increases significantly during the descent, with eversion-related muscles being primarily used during the ascent. Although squat motions are known to require strengthening of the ankle dorsiflexors [8], rotators and adductors also play an essential role in maintaining ankle stability when the load increases. Ankles are especially susceptible to injury because they are the final area that receives the load at the bottom of the human body and has a wide range of motion [26, 27]. Therefore, improving the stability of the directionality of all joints, as well as the flexion and extension of the ankles is crucial to avoiding injury.
Excessive increases in hip adduction [28] and hip internal rotation [29] during hip joint movement can cause knee pain. The results of this study showed a significant difference in hip adduction and abduction during squat movements as the load increased. The hip adduction moment during a squat using 100% or 125% body weight load was more than twice the moment recorded during a squat using a 25% weight load. However, the post-hoc test of the abduction moment showed no significant differences. These results were similar to those of previous studies that showed excessive hip adduction as a cause of knee injuries. Therefore, the results of this experiment can be interpreted as follows: if the muscle strength required to withstand the occurring joint moments is insufficient, performing squats using more than 100% of one’s body weight can result in knee injuries. Prior research has shown that an increase in the internal moment could be associated with injury; however, the results of this experiment showed no differences in the internal moments, but did show an increase in the external moments. The load increase of squats can be said not to affect the hip internal moment, which is one of the factors that causes knee injuries, but rather to affect the increase in the opposite load. Among the hip rotation moments, the maximum external moment occurred when the squat was at its deepest, and the maximum internal moment occurred at the beginning and end of the squat. Therefore, training the rotators of the gluteus muscles, which are required at the deepest squat position, is deemed necessary to prevent the excessive internal moment identified in a previous study.
The r-squared value was larger in the sagittal plane than in the front and transverse planes. This indicates that as the squat load increased, the flexion and extension moments were more likely to increase. As the squat operation is a movement mainly composed of flexion and extension, the role of the flexor and extension muscles also increases as the load increases [8]. If sufficient muscle strength is present, the movement can be successfully performed; however, it is also believed that it can effectively counter the moments that occur. Ultimately, this study aimed to secure reference data on the risk of joint injuries during back squat motions resulting from estimated weight increases, as demonstrated through the analysis of the results shown in Table 3. It was possible to identify the moments applied to each joint of the lower extremities when performing back squats with 150% and 175% body weight loads (Table 3). Linear predictions were made according to the increase in the moments of the ankle, knee, and hip joints in the sagittal plane. In addition to the moments occurring in the sagittal plane, sufficient regression equations were produced to predict the ankle adduction and hip external rotation moments. The ankle adduction moment was estimated to be 0.414 Nm/kg at 150% of body weight and 0.543 Nm/kg at 175%, whereas the hip external rotation moment was estimated to be
5.Conclusion
This study aimed to identify changes in the load on the lower extremity joints corresponding with changes in barbell weight during back squats. No significant differences were found in the participants’ movements of the lower extremities, variations in body center, or levels of balance maintenance despite the changes in weight load. However, the moments applied to the lower extremity joints were shown to differ with the changes in barbell weight. First, flexion/extension moments occurred in all joints in the sagittal plane, in the hips, knees, and ankles. Ankle internal rotation, hip adduction, and hip external rotation all showed differences in joint moments depending on the directions of adduction, abduction, and rotation. These results suggest that ankle and hip moments may occur to maintain a stable and consistent posture while withstanding increased weight load. This further signifies that resistance training for individual muscles that increase the internal and external rotation of the ankle and hip joints should be performed in parallel to ensure a stable and gradual weighted-back squat.
In addition, after calculating the applied joint moments when lifting the barbell at 25%, 50%, 100%, and 125% of the participants’ body weights, the estimated moments for additional weight loads (150% and 175%) were predicted using regression analysis. The load on the lower extremity joints becomes considerably greater when exercising with a barbell heavier than 150% of body weight; therefore, caution is recommended. However, because the joint moments at 150% and 175% loads that were not tested in this study were predicted as a linear function, changes in motion that occur when the actual load is increased were not considered. The results presented in the study also have limitations in that they cannot be applied to people who cannot lift high weights equating to more than 150% of their weight. Explaining the direct relationship between the results of the moments presented in this study and injury is a further limitation of this study. Future research involving additional musculoskeletal simulation studies should be conducted to explain the correlation between joint moments and injuries. It is hoped that this data can be used as a reference point for injury prevention in the future, by allowing people who wish to increase the intensity of their workouts to accurately gauge the demands placed on each lower extremity while increasing the weight load of back squats.
Acknowledgments
The authors have no acknowledgments.
Conflict of interest
The authors declare that they have no conflict of interest.
References
[1] | Larsen S, Kristiansen E, Nygaard Falch H, Estifanos Haugen M, Fimland MS, van den Tillaar R. Effects of barbell load on kinematics, kinetics, and myoelectric activity in back squats. Sports Biomechanics. (2022) ; 1-15. |
[2] | Lim Y-t, Lee J-w, Park J-s, Lee S-y, Kwon M-s. Effects of kinesio taping position of shank and gender on the range of motion and moment of ankle joint during deep squat. The Korean Journal of Physical Education. (2018) ; 57: (1): 471-80. |
[3] | Gullett JC, Tillman MD, Gutierrez GM, Chow JW. A biomechanical comparison of back and front squats in healthy trained individuals. The Journal of Strength & Conditioning Research. (2009) ; 23: (1): 284-92. |
[4] | Baechle TR, Earle RW. Essentials of strength training and conditioning: Human kinetics; (2008) . |
[5] | Dunn B, Klein K, Kroll B, McLaughun T, O’Shea P, Wathen D. Coaches roundtable: The squat and its application to athletic performance. Strength Cond J. (1984) ; 6: (68): 10-22. |
[6] | Caterisano A, Moss RE, Pellinger TK, Woodruff K, Lewis VC, Booth W, et al. The effect of back squat depth on the EMG activity of 4 superficial hip and thigh muscles. The Journal of Strength & Conditioning Research. (2002) ; 16: (3): 428-32. |
[7] | Comfort P, Jones PA, Smith LC, Herrington L. Joint kinetics and kinematics during common lower limb rehabilitation exercises. Journal of Athletic Training. (2015) ; 50: (10): 1011-8. |
[8] | Bryanton MA, Kennedy MD, Carey JP, Chiu LZ. Effect of squat depth and barbell load on relative muscular effort in squatting. The Journal of Strength & Conditioning Research. (2012) ; 26: (10): 2820-8. |
[9] | Sinclair J, Bentley I, Kudiersky N, Atkins S, Vincent H. Effects of four different relative loads on knee joint kinetics during the barbell back squat. JMEST. (2015) ; 2: : 286-90. |
[10] | Chiu LZ. Sitting back in the squat. Strength & Conditioning Journal. (2009) ; 31: (6): 25-7. |
[11] | Fry AC, Smith JC, Schilling BK. Effect of knee position on hip and knee torques during the barbell squat. The Journal of Strength & Conditioning Research. (2003) ; 17: (4): 629-33. |
[12] | Larsen S, Kristiansen E, van den Tillaar R. New insights about the sticking region in back squats: an analysis of kinematics, kinetics, and myoelectric activity. Frontiers in Sports and Active Living. (2021) ; 151. |
[13] | Lorenzetti S, Ostermann M, Zeidler F, Zimmer P, Jentsch L, List R, et al. How to squat? Effects of various stance widths, foot placement angles and level of experience on knee, hip and trunk motion and loading. BMC Sports Science, Medicine and Rehabilitation. (2018) ; 10: (1): 1-11. |
[14] | Flanagan SP, Salem GJ. Bilateral differences in the net joint torques during the squat exercise. Journal of Strength and Conditioning Research. (2007) ; 21: (4): 1220. |
[15] | Willems T, Witvrouw E, Delbaere K, De Cock A, De Clercq D. Relationship between gait biomechanics and inversion sprains: A prospective study of risk factors. Gait & Posture. (2005) ; 21: (4): 379-87. |
[16] | Goerger BM, Marshall SW, Beutler AI, Blackburn JT, Wilckens JH, Padua DA. Anterior cruciate ligament injury alters preinjury lower extremity biomechanics in the injured and uninjured leg: The JUMP-ACL study. British Journal of Sports Medicine. (2015) ; 49: (3): 188-95. |
[17] | Simpson JD, Stewart EM, Rendos NK, Cosio-Lima L, Wilson SJ, Macias DM, et al. Anticipating ankle inversion perturbations during a single-leg drop landing alters ankle joint and impact kinetics. Human Movement Science. (2019) ; 66: : 22-30. |
[18] | Atkins LT, Reid J, Zink D. The Effects of Increased Forward Trunk Lean during Stair Ascent on Hip Adduction and Internal Rotation in Asymptomatic Females. Gait & Posture. (2022) . |
[19] | Li X, Adrien N, Baker JS, Mei Q, Gu Y. Novice female exercisers exhibited different biomechanical loading profiles during full-squat and half-squat practice. Biology. (2021) ; 10: (11): 1184. |
[20] | Wallace DA, Salem GJ, Salinas R, Powers CM. Patellofemoral joint kinetics while squatting with and without an external load. Journal of Orthopaedic & Sports Physical Therapy. (2002) ; 32: (4): 141-8. |
[21] | Hwang S, Kim Y, Kim Y. Lower extremity joint kinetics and lumbar curvature during squat and stoop lifting. BMC Musculoskeletal Disorders. (2009) ; 10: (1): 1-10. |
[22] | Flanagan SP, Salem GJ. The validity of summing lower extremity individual joint kinetic measures. Journal of Applied Biomechanics. (2005) ; 21: (2): 181-8. |
[23] | Lee SW, Moon YJ, Eun S-D. The kinematic differences and distribution of joint Loads according to squat type. Korean Journal of Sport Science. (2011) ; 22: (1): 1674-84. |
[24] | Kim Y, Eh K, Jang S. The effect of squat exercise on cardio-respiratory and stress hormone. Journal of Sport and Leisure Studies. (2001) ; 16: : 297-305. |
[25] | Escamilla RF. Knee biomechanics of the dynamic squat exercise. Medicine & Science in Sports & Exercise. (2001) ; 33: (1): 127-41. |
[26] | Almeida SA, Williams KM, Shaffer RA, Brodine SK. Epidemiological patterns of musculoskeletal injuries and physical training. Medicine and Science in sports and Exercise. (1999) ; 31: (8): 1176-82. |
[27] | Garrick JG. The frequency of injury, mechanism of injury, and epidemiology of ankle sprains. The American Journal of Sports Medicine. (1977) ; 5: (6): 241-2. |
[28] | Willson JD, Davis IS. Lower extremity strength and mechanics during jumping in women with patellofemoral pain. Journal of Sport Rehabilitation. (2009) ; 18: (1): 76-90. |
[29] | Nakagawa TH, Moriya ÉT, Maciel CD, Serrão FV. Trunk, pelvis, hip, and knee kinematics, hip strength, and gluteal muscle activation during a single-leg squat in males and females with and without patellofemoral pain syndrome. Journal of Orthopaedic & Sports Physical Therapy. (2012) ; 42: (6): 491-501. |