Effects of wearable low-intensity continuous ultrasound on muscle biomechanical properties during delayed onset muscle soreness
Abstract
BACKGROUND:
Delayed onset muscle soreness (DOMS) is one of the most prevalent musculoskeletal symptoms in individuals engaged in strenuous exercise programs.
OBJECTIVE:
This study investigated the effects of wearable low-intensity continuous ultrasound on muscle biomechanical properties during DOMS.
METHODS:
Twenty volunteers were distributed into a wearable ultrasound stimulation group (WUG) (
RESULTS:
A significant decrease was found in the tone, stiffness, stress relaxation time, and creep in both groups after ultrasound stimulation (all
CONCLUSION:
The stiffness and pain caused by DOMS were alleviated using a wearable ultrasound stimulator. Furthermore, the effects of the wearable ultrasound stimulator were like those of a medical ultrasound stimulator.
1.Introduction
Delayed onset muscle soreness (DOMS) refers to momentary stiffness or pain felt in muscles after high-intensity or unusual physical exercise. It is one of the most prevalent musculoskeletal symptoms in individuals engaged in strenuous exercise programs. DOMS usually manifests 24–48 h after physical exercise and can last for several days. Stiffness, tenderness, and pain in the afflicted muscle areas are common symptoms. Many hypotheses exist on the induction of DOMS; however, microdamage and inflammation in the muscle are considered as the primary mechanisms [1, 2, 3].
DOMS is a serious ailment that can interfere with athletic performance. The discomfort and reduced range of motion caused by DOMS can hinder training and athletic activities, making it a concern for many athletes. Although DOMS is not a severe injury, it can indirectly increase the risk of subsequent injuries. When the muscles are sore and fatigued, athletes may alter their movement patterns to compensate for discomfort, which can lead to biomechanical imbalances and increase the risk of injury [2]. Additionally, continuing to train intensely while experiencing DOMS without an adequate recovery time can exacerbate muscle damage and increase the risk of serious injuries. DOMS from rehabilitation exercises can reduce patient motivation and willingness to participate in these exercises, thereby reducing the effectiveness of rehabilitation. Consequently, numerous methods for minimizing DOMS have been proposed.
Ultrasonic stimulation applied to the surface of the skin can deliver sufficient energy to deep tissues, including the musculoskeletal system. Under appropriate conditions, ultrasonic stimulation is safe for use, making ultrasound technology widely utilized in managing musculoskeletal issues. One application of ultrasound in musculoskeletal treatment is minimizing DOMS. It promotes the healing of wound tissue and fractures and reduces inflammation through both nonthermal and thermal effects [4]. In general, continuous ultrasound predominantly causes thermal effects in tissues, whereas pulsed ultrasound induces nonthermal effects. When the tissue temperature increases, pain decreases, blood flow increases, muscle spasm is reduced, and both subacute and chronic inflammation are diminished. Additionally, collagen extensibility increases, which aids in tissue flexibility and repair. Consequently, continuous ultrasound is widely used to alleviate pain, whereas pulsed ultrasound is often employed to promote tissue healing and reduce inflammation through nonthermal mechanisms.
Most continuous ultrasound is administered using stroking techniques that continuously move around the site of interest. Thus, the total ultrasound dose delivered to the site of interest tends to be insufficient to alleviate musculoskeletal pain, leading to non-positive effects. To achieve the thermal effects of ultrasound using this method, the application technique must be appropriate [5]. The treated area should be less than twice the size of the transducer. Therefore, the traveling speed of the ultrasound transducer should be appropriate. Stroke techniques can induce a musculoskeletal burden on therapists. Additionally, following the ultrasonic application procedure described above can be difficult for non-professionals or individuals. Furthermore, they may be unable to attend to other things while using them. Wearable ultrasound stimulators have been developed for this purpose [6, 7, 8].
The muscle properties during DOMS change with increasing muscle tension, relaxation time, and creep [9, 10, 11]. These properties are related to muscle or joint function, including the range of motion of the joint and recovery ability. Therefore, the muscle properties have to be evaluated to develop effective DOMS treatment methods. Several studies have evaluated muscle properties to verify the DOMS reduction methods [12, 13, 14]. However, only a few studies have investigated the effects of continuous low-intensity ultrasound on DOMS. This study aimed to determine the effects of low-intensity continuous ultrasound, using a wearable ultrasonic stimulator (WUS) at a frequency of 3 MHz on muscle properties and pain during DOMS. In addition, we compared the effects of WUS with those of conventional medical ultrasound devices.
2.Materials and methods
2.1Participants
This study was conducted on 20 healthy adults. The number of samples was calculated using the G*POWER, 3.1.9.7 program [15]. The participants were selected based on the following specific criteria: 1) chronic, nonspecific neck/shoulder pain, 2) non-traumatic neck/shoulder pain, 3) no history of cervical intervertebral disc disease, 4) no related injuries within the last three months, and 5) no adhesive capsulitis. Additionally, participants who experienced pain during the experiment were either excluded or given appropriate rest measures; those unable to continue due to COVID-19 were also excluded. The study protocol was explained to the participants, who provided written consent to participate after being assured that their personal and physical information would remain confidential. Prior to the experiment, the participants’ height was measured using an automatic BMI measuring instrument (BSM 370, Korea, 2011), and their weight was measured using a body composition analyzer (INBODY 570, Biospace, Korea, 2013).
The study was conducted in a single-blind manner with participants randomly assigned to two groups using Excel prior to the experiment. This study was approved by the Institutional Review Board (IRB) of Sunmoon University (SM-202206-029-3).
2.2Experiment procedures
The participants were randomly assigned to either the wearable ultrasound group (WUG) or the conventional medical ultrasound group (MUG). The anthropometric characteristics of the participants in each group are shown in Table 1.
Table 1
General characteristics of participants (
WUG ( | MUG ( | |||
---|---|---|---|---|
Age (years) | 22.80 | 23.50 | ||
Height (cm) | 172.09 | 166.57 | ||
Weight (kg) | 74.93 | 69.64 |
Values indicate mean
Figure 1.
Experimental procedure.
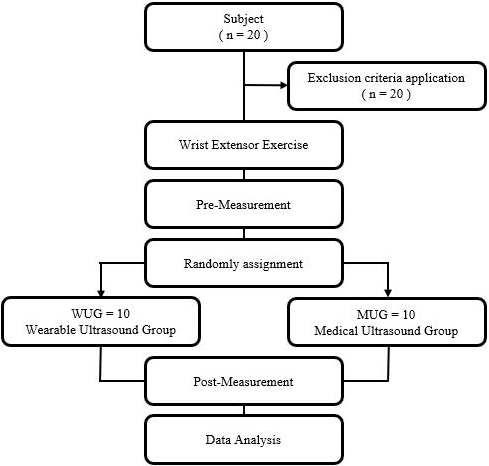
The research procedure is illustrated in Fig. 1. To induce DOMS, the participants performed wrist extensor muscle strength exercises with a dumbbell for 10 min. Subsequently, 24 h after performing the exercises, an ultrasound stimulator was applied to each participant. Muscle biomechanical properties, pain, and body temperature of the participants were evaluated before and after ultrasound stimulation.
2.3Measurement tools and methods
Muscle biomechanical properties were evaluated using a MyotonPro device (Myoton AS, Estonia), which has been shown to have excellent reliability [9, 16]. Each parameter was measured three times, and the average value was determined. The measurements were repeated if the coefficient of variance was over 3%. Mechanical impulses of 0.18 N (pre-load) and 0.40 N were applied for 15 ms to the muscle. The characteristics of interest were measured using oscillation frequency (Hz), logarithmic decrement, dynamic stiffness (N/m), mechanical stress relaxation time (ms), and creep. The inherent tension of the muscle in a passive or resting condition without any purposeful contraction, which indicates the muscle tone, is described by the oscillation frequency. Resistance to contraction or an external stimulus that deforms the initial configuration of the muscle is referred to as dynamic stiffness, and the converse is known as compliance. The recovery of the muscle’s initial form after contraction or the removal of an external force is described by a logarithmic decrement. A value of zero denotes absolute elasticity, values
Figure 2.
Measurement of muscle biomechanical properties using MyotonPro.
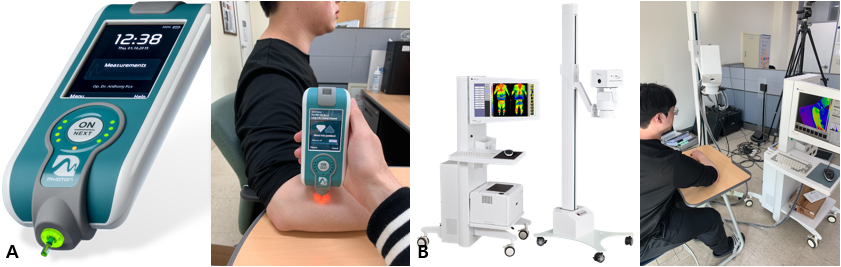
Pain was assessed using the Korean version of the short form of the McGill pain questionnaire (KSF-MPQ), which is widely used to evaluate pain quality and intensity.
2.4Ultrasound stimulation
The WUS, developed for this study, generates ultrasound at a frequency of 3 MHz with continuous waves. It comprises an ultrasound driving board (Fig. 3A), a lithium polymer battery (1,500 mAh, DTP903450, DTP, China), a stainless-steel ultrasound transducer of 25-mm diameter and 3-mm thickness, and a piezoelectric ceramic disk (Ceracomp, South Korea, Fig. 3B). The driving board of this research device consists of a voltage step-up component to boost the battery voltage, a micro process unit, and an ultrasonic driver. The stimulator generated 100 mW/cm2 of ultrasound intensity.
Figure 3.
Stimulator components. A: Ultrasound driving board, B: Ultrasound transducer.
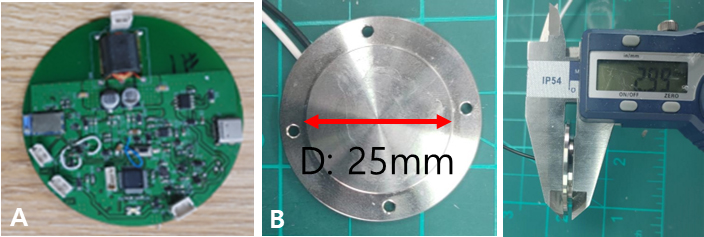
Figure 4.
Ultrasound gel pad.
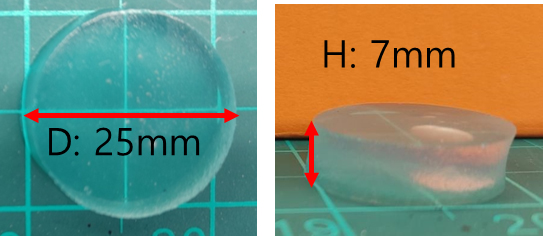
Figure 5.
Intervention program. A: Low-intensity wearable ultrasound B: Chattanooga group – Intellect Transport Ultrasound.
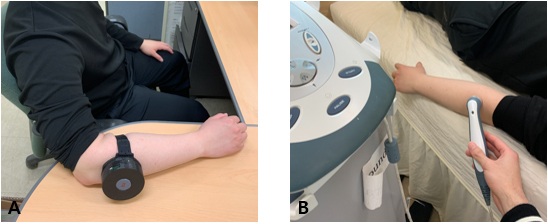
During treatment, an ultrasound gel pad (BluemTech, South Korea) with 7-mm thickness was used as the coupling material (Fig. 4). Medical ultrasound (Intelect TranSport Ultrasound 2782, Chattanooga Group, UK) used in the MUG was applied at a frequency of 3 MHz. The application period was 1 h for the WUG and 5 min for the MUG. The ultrasonic attachment was placed on the origin area of the extensor carpi radialis brevis (Fig. 5), which is commonly associated with inflammation due to the muscle’s high load during wrist disparaging exercises Ultrasound was used as a stationary technique in the WUG group and as a stroking technique in the MUG group.
For safety evaluation of WUS over a long period, we evaluated skin temperature using the IRIS-XP (Medicore device, South Korea), which measures the temperature of the area under the ultrasound transducer before and after WUS application (Fig. 6A). Deep tissue injuries were evaluated using a BSA-based ultrasound gel phantom (BSA; Institute of Health and Medical Convergence Technology, Korea). When heated above the temperature that harms living tissue, this phantom changes hue to opaque. If the phantom remains the same hue during ultrasonic stimulation in this investigation, it is considered safe. The phantom was placed under an ultrasound transducer and was subjected to ultrasound stimulation for one hour. The phantom was then observed for color change (Fig. 6B).
Figure 6.
Safety evaluation methods (a) IRIS-XP (b) BSA phantom.
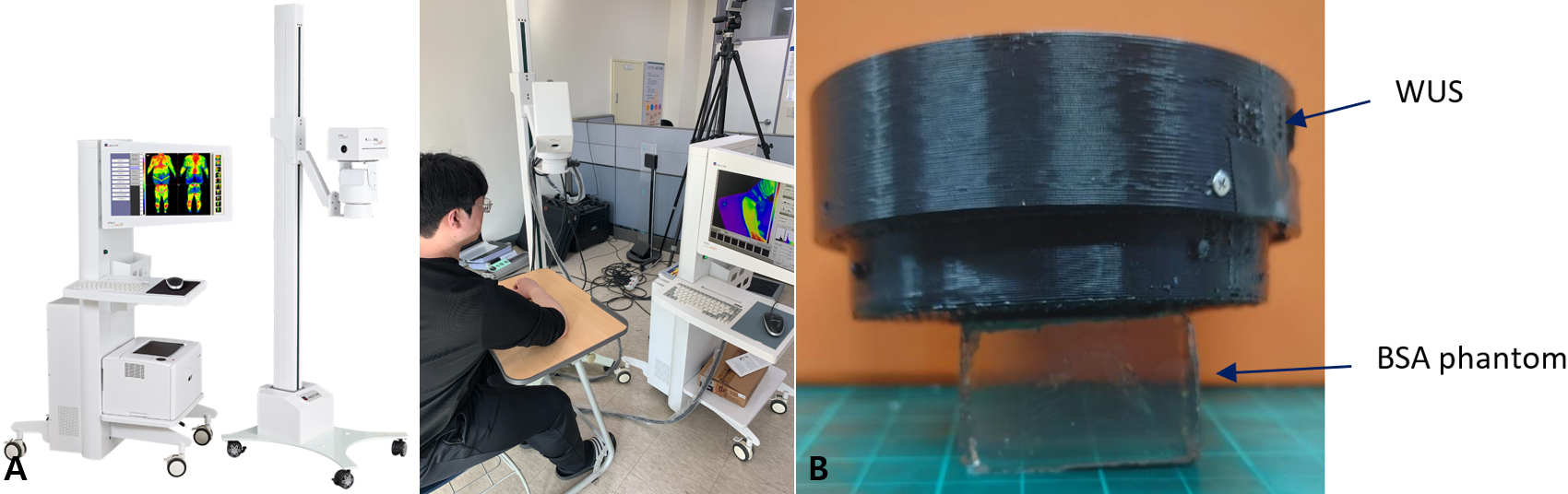
2.5Data analysis
Descriptive statistics were used to calculate the general characteristics, including the mean and standard deviation of each variable. Statistical analyses were conducted using IBM SPSS 26.0. A paired
3.Results
All measurement parameters are listed in Table 2. Following the intervention, both groups exhibited significant decreases in muscle tone (0.48 in WUG, 0.66 in MUG, all
Table 2
Comparison between and within groups
WUG | MUG | t | ||
---|---|---|---|---|
Tone (Hz) | Pre | 17.12 | 19.62 | 1.738 |
Post | 16.60 | 18.28 | ||
t | 3.182* | 2.434* | ||
Stiffness (N/m) | Pre | 336.80 | 397.20 | 1.138 |
Post | 315.70 | 356.50 | ||
t | 2.764* | 2.365* | ||
Elasticity | Pre | 1.06 | 1.01 | 0.718 |
Post | 1.03 | 0.96 | ||
t | 2.159 | 1.719* | ||
Relaxation (ms) | Pre | 16.47 | 15.47 | |
Post | 14.64 | 13.86 | ||
t | 3.409** | 4.138** | ||
Creep | Pre | 1.00 | 0.99 | 0.077 |
Post | 0.89 | 0.87 | ||
t | 2.990* | 2.245 | ||
KSF-MPQ | Pre | 9.30 | 9.00 | 0.696 |
Post | 7.30 | 6.50 | ||
t | 5.000** | 3.87** | ||
Temperature (∘C) | Pre | 30.98 | 30.15 | 1.611 |
Post | 32.90 | 31.24 | ||
t |
*p< 0.05, **p< 0.01, mean
A significant increase in skin temperature was observed for both groups (2.08 in WUG, 1.09 in MUG, all
4.Discussion
In this study, we evaluated the effects of continuous low-intensity WUS at a frequency of 3 MHz on the muscle biomechanical properties during DOMS. Additionally, we compared the effects of conventional ultrasound stimulation. Ultrasound treatment is a noninvasive and low-risk approach to relieve pain in the musculoskeletal system using thermal and mechanical energy.
The stress relaxation time and creep significantly decreased in both groups after ultrasound stimulation, and no differences were observed between the groups. Stress relaxation time refers to the time required for a muscle to return to its original shape after an external force is applied that changes its form [17]. Typically, when an external force is applied to the swollen area and then removed, the muscle takes a long time to return to its original shape. In severe cases, the deformed shape retains a pitting phenomenon. After ultrasound stimulation, the stress relaxation time decreased significantly, indicating an improvement in swelling [2, 3, 18]. Additionally, creep, a measure of the structural integrity of the muscle, was evaluated, with higher integrity resulting in lower values [17]. Thus, ultrasound stimulation signifies an improvement in the compromised integrity caused by excessive infiltration of fluids (e.g., body fluids and blood) due to swelling. The changes in the biomechanical characteristics of the muscle confirmed that continuous low-intensity WUS can alleviate the symptoms of muscle damage caused by DOMS.
Muscle tone and tension significantly decreased after ultrasound stimulation, and no differences were observed between the groups. These results indicated that WUS reduced muscle stiffness, which increased after DOMS. The results were similar to those obtained using conventional ultrasound. Several studies have shown that ultrasound stimulation reduces muscle stiffness [7, 8, 19, 20]. Furthermore, other methods to alleviate DOMS, such as vibration [21], massage [22], and static stretching [23], have been shown to reduce muscle stiffness.
The pain reduction level measured using the KSF-MPQ in the WUG group showed a significant reduction (
Eccentric exercise induces microdamage to muscles and connective tissues. Subsequently, this leads to edema, swelling, and an immune response with the infiltration of inflammatory cells. This immune response generates prostaglandins (PGE2) that sensitize type 3 and type 4 nerve endings, rendering them more sensitive to mechanical, chemical, and thermal stimuli. Swelling increases intramuscular pressure, leading to continuous stimulation of sensitized pain receptors by PGE2, resulting in pain [1]. As demonstrated in this study, ultrasound appeared to reduce the characteristics of swelling, supported by changes in stress relaxation time and creep, thereby decreasing the stimulation of sensitized pain receptors and alleviating pain [25]. These findings suggest that ultrasound can relieve pain caused by eccentric exercise.
The use of WUS may potentially cause injuries such as burns to the skin and deep tissues, owing to stationary stimulation at a specific site. However, another study has shown that low-intensity continuous ultrasound with stationary contact does not cause a significant temperature increase in deeper tissues owing to the presence of blood and body fluids [26]. In this study, ultrasound was applied to the muscle region, which generally contains considerable amounts of blood and fluid. Therefore, the application of the stationary contact ultrasound to the muscles was considered safe. Nevertheless, this study evaluated potential secondary injuries to biological tissues caused by fixed-contact ultrasound by conducting thermal and phantom tests. The results showed that the skin temperature under the ultrasound transducer increased slightly, approximately 2∘C, which was not sufficient to cause damage to the skin [27]. During the study, ultrasound gel pads were used to provide adequate moisture throughout the 1-h stimulation period. This ensured better adhesion between the transducer and treatment area, maintaining a low impedance. The phantom experiment also confirmed the safety of ultrasound stimulation in deep tissues as no color changes were observed. Hence, the stationary ultrasound stimulation used in this study was considered safe and did not cause secondary damage to the DOMS.
Low-intensity continuous ultrasound was used in this study. According to the Arndt-Schultz law, low-intensity ultrasound aids in the rapid recovery of damaged muscles [28, 29]. Additionally, continuous ultrasound generates heat in deeper tissues as its primary effect. Some ultrasound-related effects have shown that pulsed ultrasound stimulation may not have a significant impact, suggesting that thermal effects might be more dominant than nonthermal effects in managing pain [20]. Therefore, the use of low-intensity continuous ultrasound in this study seems beneficial for the rapid recovery of injured muscles, and the main effect observed was likely the generation of heat in deeper tissues.
However, this study has several limitations. First, because it was a one-off experiment, predicting the effects of the intervention over a longer period was difficult. Second, the study’s ability to represent various age groups was limited because only participants in their 20 s were surveyed. Third, the results of this study revealed an increase in skin temperature following ultrasound stimulation, suggesting that such an elevation could potentially produce effects similar to those of conventional heating pads or creams in managing musculoskeletal symptoms. It is inferred from these findings that the thermal stimulation on the skin surface induced by ultrasound, combined with deep tissue heating, may collectively influence the musculoskeletal system. However, this research did not verify these complex effects, and notably, it did not conduct a comparative study with traditional heating pads or creams. Future research is needed to address the issues. Nevertheless, based on the results of previous studies, ultrasound treatment has reportedly been effective in treating various diseases and symptoms in different age groups.
5.Conclusions
This study aimed to determine the ability of wearable ultrasound devices based on low-intensity ultrasound to effectively alleviate pain and muscle tension, increase body heat, and be as effective as conventional medical ultrasound devices. The results showed that low-intensity ultrasound-based wearable treatment devices had significant effects on pain, muscle tension, and body heat and could be as effective as conventional medical ultrasound devices. Thus, low-intensity ultrasound-based wearable treatment devices are viable alternatives to conventional treatment devices with the added advantage of being portable and enabling patients to move during treatment.
Acknowledgments
This research was supported by the Regional Innovation Strategy (RIS) through the National Research Foundation of Korea (NRF) funded by the Ministry of Education (MOE) in 2023 (No. 2022RIS-005) and the National Research Foundation of Korea (NRF) grant funded by the Korean government (MSIT) (No. RS-2023-00221762).
Conflict of interest
None to report.
References
[1] | Cheung K, Hume PA, Maxwell L. Delayed onset muscle soreness. Sports Medicine. (2003) ; 33: (2): 145-64. |
[2] | Nahon RL, Lopes JSS, de Magalhães Neto AM. Physical therapy interventions for the treatment of delayed onset muscle soreness (DOMS): Systematic review and meta-analysis. Physical Therapy in Sport. (2021) ; 52: : 1-12. |
[3] | Zainuddin Z, Newton M, Sacco P, Nosaka K. Effects of massage on delayed-onset muscle soreness, swelling, and recovery of muscle function. J Athl Train. (2005) ; 40: (3): 174. |
[4] | Park SK, Park SH. The effect of combined suboccipitalis release exercise and therapeutic ultrasound on mechanical properties of cervical muscles and neck disability index, headache impact test in tension-type headache patients. Journal of The Korean Society of Integrative Medicine. (2021) ; 9: (4): 271-81. |
[5] | Draper DO. Facts and misfits in ultrasound therapy: Steps to improve your treatment outcomes. Eur J Phys Rehabil Med. (2014) ; 50: (2): 206-16. |
[6] | Lewis GK Jr, Langer MD, Henderson CR Jr, Ortiz R. Design and evaluation of a wearable self-applied therapeutic ultrasound device for chronic myofascial pain. Ultrasound Med Biol. (2013) ; 39: (8): 1429-39. |
[7] | Petterson S, Plancher K, Klyve D, Draper D, Ortiz R. Low-intensity continuous ultrasound for the symptomatic treatment of upper shoulder and neck pain: a randomized, double-blind placebo-controlled clinical trial. J Pain Res. (2020) ; 1277-87. |
[8] | Uddin SMZ, Komatsu DE, Motyka T, Petterson S. Low-intensity continuous ultrasound therapie – a systematic review of current state-of-the-art and future perspectives. J Clin Med. (2021) ; 10: (12): 2698. |
[9] | Kawczyński A, Mroczek D, Andersen RE, Stefaniak T, Arendt-Nielsen L, Madeleine P. Trapezius viscoelastic properties are heterogeneously affected by eccentric exercise. J Sci Med Sport. (2018) ; 21: (8): 864-9. |
[10] | Ochi E, Tsuchiya Y, Nosaka K. Differences in post-exercise T2 relaxation time changes between eccentric and concentric contractions of the elbow flexors. Eur J Appl Physiol. (2016) ; 116: : 2145-54. |
[11] | Konrad A, Kasahara K, Yoshida R, Yahata K, Sato S, Murakami Y, et al. Relationship between eccentric-exercise-induced loss in muscle function to muscle soreness and tissue hardness. In: Healthcare. MDPI. (2022) . p. 96. |
[12] | Nakamura M, Ikezoe T, Tokugawa T, Ichihashi N. Acute effects of stretching on passive properties of human gastrocnemius muscle-tendon unit: Analysis of differences between hold-relax and static stretching. J Sport Rehabil. (2015) ; 24: (3): 286-92. |
[13] | Matsuo S, Suzuki S, Iwata M, Hatano G, Nosaka K. Changes in force and stiffness after static stretching of eccentrically-damaged hamstrings. Eur J Appl Physiol. (2015) ; 115: : 981-91. |
[14] | Hammons D, McCullough M. The effect of dry cupping on gastrocnemius muscle stiffness, range of motion and pain perception after delayed onset muscle soreness. Altern Ther Health Med. (2022) ; 28: (7). |
[15] | Johns LD. Nonthermal effects of therapeutic ultrasound: The frequency resonance hypothesis. J Athl Train. (2002) ; 37: (3): 293. |
[16] | Ko CY, Choi HJ, Ryu J, Kim G. Between-day reliability of MyotonPRO for the non-invasive measurement of muscle material properties in the lower extremities of patients with a chronic spinal cord injury. J Biomech. (2018) ; 73: : 6-5. |
[17] | MYOTON. MyotonPRO digital palpation device user manual. (2023) . |
[18] | Longo V, Jacobson JA, Fessell DP, Mautner K. Ultrasound findings of delaye-onset muscle soreness. Journal of Ultrasound in Medicine. (2016) ; 35: (11): 2517-21. |
[19] | Choi YH, Park YH, Park SH. Effects of ultrasound therapy on balance and gait in stroke patients with gastrocnemius muscle spasticity. World J Res Rev. (2018) ; 7: (2): 262594. |
[20] | Draper DO, Mahaffey C, Kaiser D, Eggett D, Jarmin J. Thermal ultrasound decreases tissue stiffness of trigger points in upper trapezius muscles. Physiother Theory Pract. (2010) ; 26: (3): 167-72. |
[21] | Pinto NS, Monteiro MB, Arthur AP, Paiva DN, Meyer PF, Santos-Filho SD, et al. Effectiveness of a protocol involving acute whole-body vibration exercises in an adult and health individual with delayed-onset muscle soreness observed after running: a case report. J Med Med Sci. (2011) ; 2: (1): 612-7. |
[22] | Weerapong P, Hume PA, Kolt GS. The mechanisms of massage and effects on performance, muscle recovery and injury prevention. Sports Medicine [Internet]. (2005) ; 35: (3): 235-56. Available from: doi: 10.2165/00007256-200535030-00004. |
[23] | Matsuo S, Suzuki S, Iwata M, Hatano G, Nosaka K. Changes in force and stiffness after static stretching of eccentrically-damaged hamstrings. Eur J Appl Physiol. (2015) ; 115: : 981-91. |
[24] | Aaron SE, Delgado-Diaz DC, Kostek MC. Continuous ultrasound decreases pain perception and increases pain threshold in damaged skeletal muscle. Clinical Journal of Sport Medicine. (2017) ; 27: (3): 271-7. |
[25] | Ansari NN, Ebadi S, Talebian S, Naghdi S, Mazaheri H, Olyaei G, et al. A randomized, single blind placebo controlled clinical trial on the effect of continuous ultrasound on low back pain. Electromyogr Clin Neurophysiol. (2006) ; 46: (6): 329-36. |
[26] | Rigby JH, Taggart RM, Stratton KL, Lewis GK Jr, Draper DO. Intramuscular heating characteristics of multihour low-intensity therapeutic ultrasound. J Athl Train. (2015) ; 50: (11): 1158-64. |
[27] | Lawrence JC, Bull JP. Thermal conditions which cause skin burns. Eng Med. (1976) ; 5: (3): 61-3. |
[28] | Rai S, Kaur M, Goel S, Panjwani S, Singh S. Prospective utility of therapeutic ultrasound in dentistry – Review with recent comprehensive update. Adv Biomed Res. (2012) ; 1. |
[29] | Watson T, Goh AC. Dose dependency with electro physical agents: Both the Arndt Schulz law and the Goldilocks principle provide an explanatory model. Physiotherapy [Internet]. (2015) May 1 [cited 2023 Aug 2]; 101: : e1615-6. Available from: http://www.physiotherapyjournal.com/article/S0031940615016600/fulltex. |