Usability evaluation of an interactive leg press training robot for children with neuromuscular impairments
Abstract
BACKGROUND:
The use of robotic technology for neurorehabilitative applications has become increasingly important for adults and children with different motor impairments.
OBJECTIVE:
The aim of this study was to evaluate the technical feasibility and usability of a new interactive leg-press training robot that was developed to train leg muscle strength and control, suitable for children with neuromuscular impairments.
METHODS:
An interactive robotic training system was designed and constructed with various control strategies, actuators and force/position sensors to enable the performance of different training modes (passive, active resistance, and exergames). Five paediatric patients, aged between 7 and 16 years (one girl, age 13.0
RESULTS:
One patient could not perform the training session because of his small knee range of motion. Visual Analog Scale scores were given by the 4 patients who performed the training sessions. All the patients adjudged the training with the interactive device as satisfactory. The average SUS score given by the therapists was 61.2
CONCLUSION:
This study proposed an interactive lower limb training device for children with different neuromuscular impairments. The device is deemed feasible for paediatric rehabilitation applications, both in terms of technical feasibility and usability acceptance. Both patients and therapists provided positive feedback regarding the training with the device.
1.Introduction
The use of robotic technology for neurorehabilitation has become increasingly important for both adults and children with different motor impairments [1, 2, 3, 4]. Rehabilitation robots have been introduced in the clinical rehabilitation environment tocomplement conventional therapy and improve therapeutic outcomes, as they can enhance the quantity and the quality of the rehabilitation dose, by increasing the training duration and the number of repetitions and providing more accurate repetition trajectories [5, 6].
Several robotic systems are available on the market for paediatric neurorehabilitation [7, 8]. Generally, these systems were primarily developed for adult patients, and introduced to the paediatric field after technological adaptations [5, 9, 10]. For example, after the positive outcomes of the gait trainer Lokomat (Hocoma AG, Switzerland) in adults, a paediatric version has been developed [11]. The paediatric Lokomat is a gait trainer robotic platform, which consists of a body-weight support system, and two leg exoskeletons adaptable to the patient’s anthropometry and synchronized with a treadmill. The therapy principle of the Lokomat is based on repetitive gait movements with a variety of control strategies depending the rehabilitation phase and patient’s participation. Training with the Lokomat showed improvements in standing and walking ability in children with cerebral palsy [12, 13].
Another robotic system for paediatric rehabilitation is the gait trainer GT-1 (RehaStim, Germany) that aims to enhance gait ability by providing repetitive movements [14]. The GT-1 is an end-effector rehabilitation robot, where the patient’s feet are positioned on programmable footplates that are able to simulate various walking scenarios by generating different movement patterns. A study including 18 children with cerebral palsy showed that a 2-week training programme with the GT-1 could induce significant improvements in walking, hip kinematics, speed and step length compared to the control group [14].
The ability of the robotic system to provide different training modes depending on the patient’s recovery stage plays an important role in the efficiency of the therapy [15, 9]. Normally, rehabilitation recovery is divided into three phases: acute, subacute, and chronic [16]. In the acute or early recovery stage, passive training is often recommended, where the patient’s limb is passively guided to track a predefined trajectory through continuous position control [17, 18]. This promotes limb motor function, maintains range of motion and reduces muscle atrophy, but lacks patient’s motivation [19, 20]. In the subacute phase of recovery, where the patient is able to provide sufficient forces for the proposed training, it is recommended that the patient performs active training or active resistive training [19, 21]. During active training the robot provides resistive forces, while the patient is performing task-specific or repetitive movements [22]. This makes the exercise more challenging and increases muscle strength [20]. Some studies encouraged the combination of both passive and active training modes, and showed improvements in motor performance in children with neuromuscular impairments [23, 24]. In the chronic recovery phase, robotic systems assist and support the patient’s balance during training and record the data for assessment [15, 20].
The active participation of the patient and the interaction with the rehabilitation process is very important and has been proven to enhance the therapeutic outcomes [25, 26]. Especially in children and adolescents, the introduction of games and virtual reality into the rehabilitation therapy has a major influence on the treatment, as it motivates, challenges and makes the children interact in real time with the virtual environment, thus the duration and intensity of the training can be increased [9, 23, 27, 5]. The rehabilitation robotic system presented in this study allows interaction between the patient and the device by using real-time interactive training modes and virtual environments, thus increasing the patient’s active participation and motivation. The developed interactive leg press device can operate in three different modes: (i) passive training, (ii) active resistive training and (iii) active resistive training complemented with exergames. These interactive modes are based on real-time biofeedback provided from force and angle sensors, which allows on-line monitoring of performance data, and off-line records for further evaluation and analysis.
For both children and adults, the leg-press form of exercise has been proven to have several benefits for sport conditioning and neuromuscular rehabilitation applications [28, 29, 30, 31], and several leg-press training devices were developed and introduced to the market, such as the Allegro [32] and LegoPress [33]. These devices were designed mainly for adult patients’ rehabilitation and sport training applications, whereas the robotic system proposed in this study targets the paediatric population in term of size, training intensity and training modes.
2.Objective
The aim of this study was to evaluate the technical feasibility and usability of a new interactive leg-press training robot suitable for children with neuromuscular impairments. The usability analysis considered user acceptability for both patients and therapists. The work presented here is a preliminary clinical assessment of usability. A full clinical study is required to generate clinical evidence of efficacy of the treatment.
3.Methods
3.1Patients
The Ethics Review Board of the Canton of Zürrich in Switzerland reviewed the study protocol and decided by means of a jurisdictional inquiry that this study did not fall under the Human Research Act (Nr. Req-2018-00586). Written informed consent was obtained from the legal guardians of all the participating children prior to participation. Patients aged 14 and above provided written informed consent while younger ones provided oral agreement. The measurements were performed at a paediatric rehabilitation centre in Switzerland (University Children’s Hospital Zürrich, Rehabilitation Centre in Affoltern am Albis).
The inclusion criteria for recruitment were: (i) aged between 6 and 18 years, (ii) neuromuscular impairments affecting at least one lower-limb, (iii) ability to understand the tasks, and (iv) ability to answer the user satisfaction questionnaire. Exclusion criteria were: (i) orthopaedic surgery in the last 6 months, (ii) unhealed skin lesions in the lower limbs, and (iii) severe cognitive impairments (Table 1).
Table 1
Patients’ characteristics
Patients | Gender | Age (yrs) | Body mass (kg) | Height (cm) | Diagnosis |
---|---|---|---|---|---|
P01 | m | 12.5 | 38.0 | 144 | Bilateral spastic CP (after knee operation) |
P02 | m | 14.8 | 65.5 | 165 | Guillain-Barré-Syndrome |
P03 | m | 16.3 | 70.3 | 169 | Bilateral ataxic CP |
P04 | f | 16.9 | 54.5 | 163 | Severe traumatic brain injury |
P05 | m | 7.8 | 26.2 | 132 | Polytrauma after traffic accident with several surgeries |
Mean | 13.7 | 50.9 | 155 |
Abbreviations: CP: cerebral palsy, SD: standard deviation.
3.2Training device
3.2.1Specifications and design
The robotic system developed and evaluated in this project is an interactive leg press training device, designed for children with neuromuscular impairments to train and strengthen their lower limbs. The interactive leg press device comprises an adjustable seat module, independent footplates attached via lever mechanisms to two pneumatic linear actuators (cylinders), and a visual feedback positioned in front of the user (Fig. 1a).
Figure 1.
Training device.
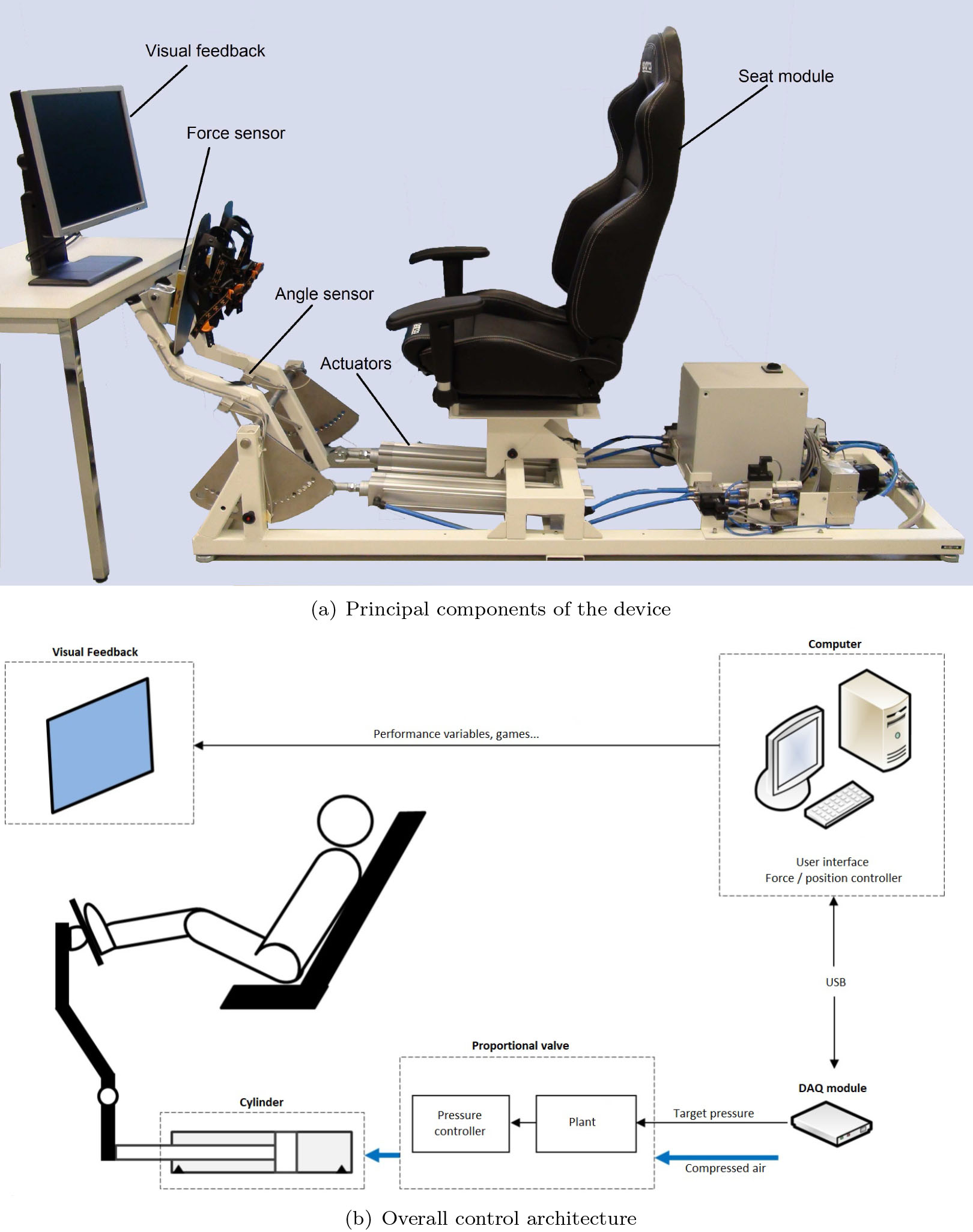
The seat module consists of a tuning car seat (Sparco S.P.A, Italy) mounted on a support mechanism which can move forwards/backwards and up/down to suit the anthropometrics of children and adolescents with different bodysizes. Prior to each training session, therapists individually adjusted the distance between the seat and the footplates for each patient. The seat provides a tilt mechanism for the back support and a mechanism to adjust the sagittal position. Standard office chair hand rails were mounted to enable the child to hold on for a safe and comfortable feeling.
The training device is based on linear pneumatic actuators (DSBC-U-63-500-PPSA-N3, Festo AG & Co, Germany) containing cylinders that use compressed air power to provide a linear movement. The actuators used for this project are double acting cylinders (push and pull directions) and can provide forces of 1682 N with compressed air of 6 bar. The idea behind using double acting cylinders is to control the movement and the force in the pedal in both directions, which is needed mainly for position control where the pedal is required to follow a trajectory (passive training). Pressure and direction of the cylinders are commanded by proportional and directional valves, which are interfaced to a data acquisition module (DAQ module) and controlled via USB cable by a systems engineering software (LabVIEW, National Instruments, Austin, US) (Fig. 1b).
To measure the forces and positions the device has two force sensors and two angle sensors. The force sensors (KD140.1kN, Transmetra GmbH, Switzerland, accuracy:
The visual feedback screen is mounted in front of the user, and displays the performance variables in real-time (forces and angles) and specific training scenarios (e.g. games, (Fig. 2)). Children were able to visualize their dynamic behaviour and asked to adapt their volitional performance to follow a target signal. This task requires and trains coordination and adequate levels of leg strength.
For safety, the device has an emergency stop button and an adjustable mechanical brake, which keeps the pedals within the desired maximal range of motion. Additionally, to ensure patient safety, therapists were able to define a maximal force value, where the device stops the actuators if this value is reached.
Figure 2.
Patient 5 playing the Space Shooter exergame.
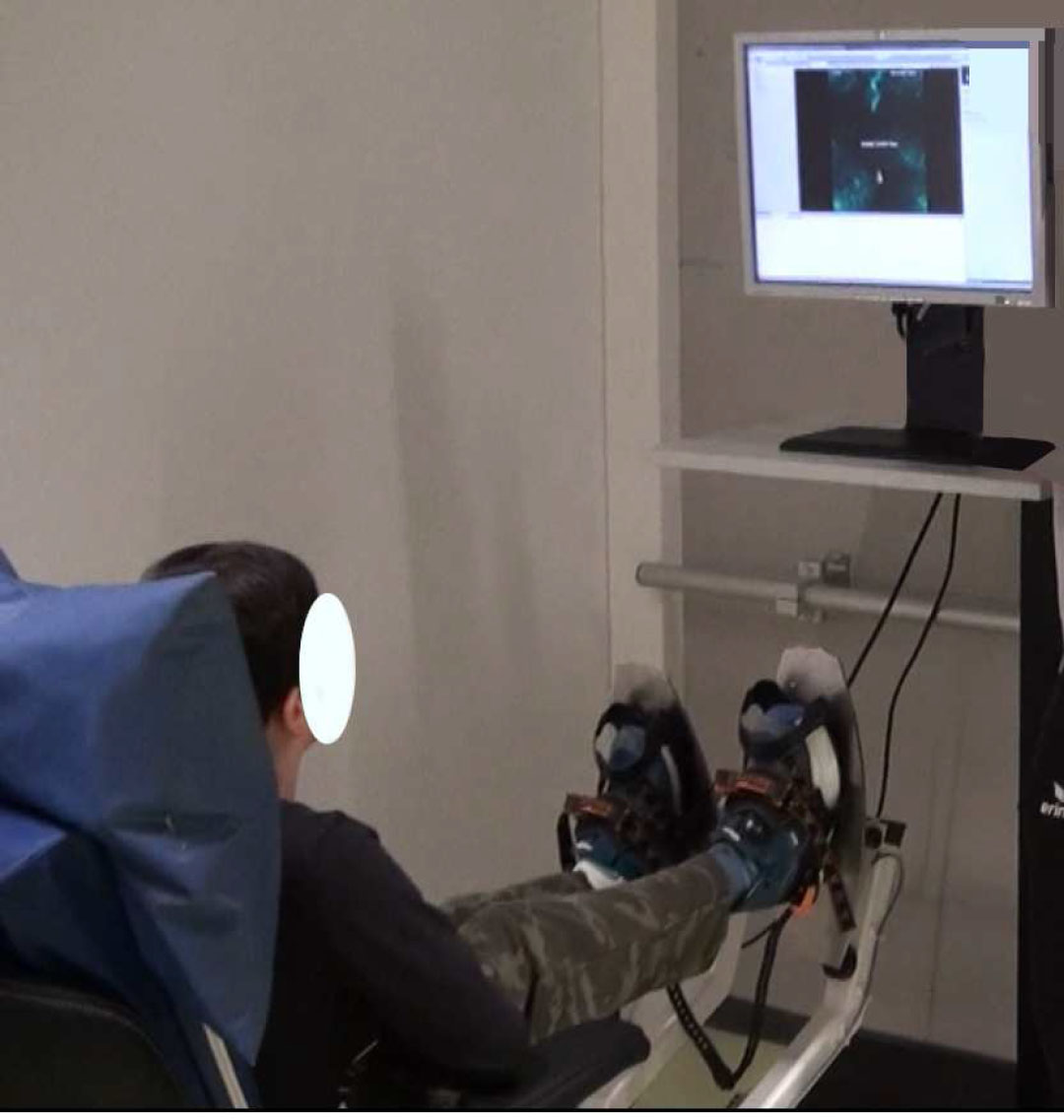
3.2.2Control architecture and training modes
The training robotic system presented in this study aims to facilitate rehabilitation exercises for children with different neuromuscular diseases. The device can operate in three different training modes:
1. Passive training: the device operates in position control mode, where it imposes a predefined trajectory on the limb, while the patient moves passively with the device. The main aim of this mode is to increase range of motion and decrease knee spasticity. It can also be used for patients to familiarize with the device. It is essential for early rehabilitation phases but lacks scenarios to increase the patient’s motivation.
2. Active resistance training: The device operates in force control mode. The robot provides resistive forces against the limb while the patient is asked to perform specific tasks. The aim of this training mode is to increase muscle strength and coordination. It is suitable for patients with advanced recovery level, and it makes the training more challenging.
3. Exergames: The device operates similarly as in active resistance, while the patient is controlling games with the legs. The aim of this training mode is to motivate the patient and increase training duration and challenges. It also distracts the patients from the movement itself (external focus of attention), which has been proven to be beneficial for motor learning [34, 35].
The principal task of the position feedback control system is to impose a precise predefined trajectory on the limb. This is implemented using a closed-loop position controller (Fig. 3). The position controller compares the predefined target angle and the actual angle measured by the angle sensors, and continuously computes and transmits a target air pressure to the proportional valve. The proportional valve has an internal pressure controller which keeps the level of air pressure in the cylinder close to the target pressure.
Figure 3.
Position/force control loop.
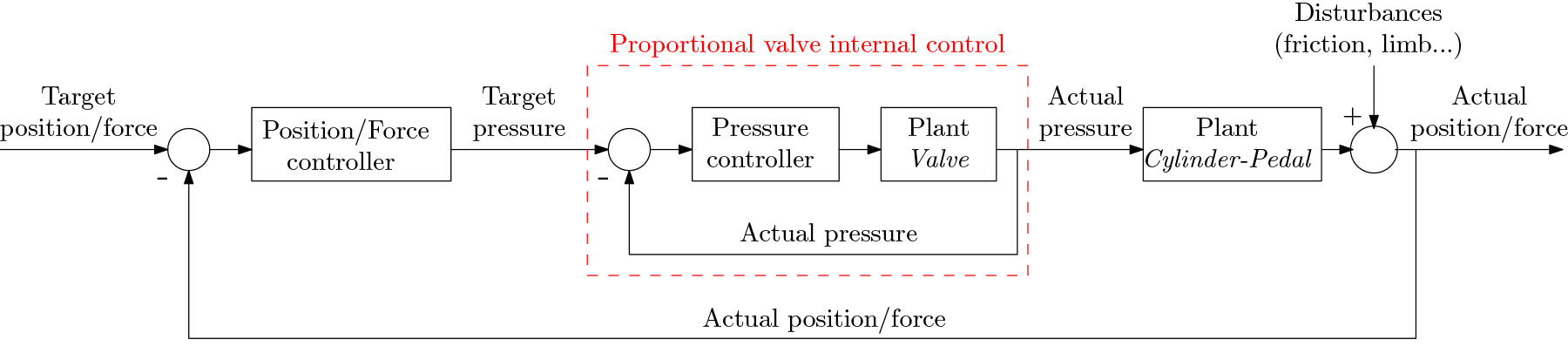
The force feedback control system aims to maintain a target force at the interaction point between the patient’s limb and the pedal (Fig. 3). Similar to the position control system, the force control system is a closed-loop controller that transmits a target air pressure to the proportional valve after comparing the target predefined force and the actual force measured by the sensors behind the footplates. The internal pressure controller supplies the cylinder with compressed air, and generates the forces on the footplates. For both position and force controllers, the left and right pedals are separately controlled, and for simplicity we describe only a single side here, while in practice the implementation is duplicated.
The relevant performance signals (force, angle, pressure) are monitored and recorded during real-time feedback operations using data acquisition and signal procession software (LabView, National Instruments). Following the real-time controller tests, the signals are processed and evaluated off-line using Matlab software (Mathworks Inc., USA).
3.3Games
To offer the patient a playful training environment, two different exergames have been developed: Space Shooter and Ping-Pong. The games run on a cross-platform game engine (Unity3D, Unity Technologies ApS, USA), while the training device is set in the active resistive training mode (force controller). Both the Space Shooter and Ping-Pong games are controlled using the angle sensors, which are interfaced to an open-source micro-controller board (Arduino Uno, Arduino S.r.l., Italy). The communication between the micro-controller board and the PC is done via USB.
During the Space Shooter exergame, the patient was asked to control a spacecraft in 2D and to avoid and shoot falling stones. The position and direction of the spacecraft is defined by the difference between the right and left pedal angles, thus the player can move the spacecraft to the left by extending the left leg, and to the right by extending the right leg. The player scores 10 points for each destroyed stone. To change the difficulty, the shooting frequency and the number and speed of the falling stones can be changed in the external software configuration (Unity3D). This game focuses on improving the coordination accuracy and speed of muscle activation of the lower limbs.
The Ping-Pong exergame is adapted from the classical ping-pong arcade video game. Unlike the classical version of Ping-Pong which can be performed by two players, the exergame used for this training device is an endless game and can be played by one user: the patient is asked to control the two paddles and keep the ball bouncing between the paddles without touching the side walls. The patient controls the position of the paddles separately, where the left leg controls the left paddle and the right leg controls the right paddle. Extending the leg moves the paddle to the top, while flexing moves them down. Positive points are add to the score when the ball touches the paddles, while points are deducted when it touches the side walls. The difficulty of the game can be changed by setting the speed of the bouncing ball. Unlike the Space Shooter exergame, this game requires selectivity of right and left legs, and focuses on improving coordination and speed of the limbs.
3.4Test procedure
Usability evaluation of the training device involved both paediatric patients and therapists (Tables 1 and 2). Patients performed one training session on the therapy device under the supervision of two therapists (therapists changed for each patient). The therapists had never used the device before and received an introduction to the device prior to each session, before they were instructed to implement the different training conditions using a check-list. Each session lasted between 40 and 45 min including the calibration setup, the three training conditions (passive, active, and exergames modes), and answering the user satisfaction questionnaires.
Table 2
Therapists’ characteristics
Therapists | Gender | Age (yrs) | Profession | Working experience (yrs) | Working experience with children (yrs) |
---|---|---|---|---|---|
T01 | m | 29 | Sport scientist | 7 | 3.5 |
T02 | m | 24 | Health scientist | 0.5 | 0.5 |
T03 | m | 28 | PhD student/Physical therapist | 2.5 | 2.5 |
T04 | f | 23 | Sport therapist | 0 | 0 |
T05 | m | 45 | Scientist/Physical therapist | 23 | 8 |
T06 | f | 29 | Physical therapist | 6 | 3 |
T07 | f | 22 | Sport therapist | 0.5 | 0.5 |
T08 | f | 35 | Human movement scientist | 11 | 10 |
T09 | f | 29 | Physical therapist | 5 | 4.5 |
First, the therapist received an easy-to-understand introduction to the device and the training that the patient was going to perform. After getting agreement from the patient, and ensuring that the patient had no musculoskeletal complaints or discomfort, the patient was installed on the seat module and his/her feet were fixed on the footplates. Therapists manually adjusted the seat module position depending on the patient’s body-size and defined the maximal range of motion of the patient’s legs by placing the patient’s feet on the pedal and manually pushing the pedal to the maximal knee angle. Based on this calibration, the robotic system saved this data and automatically generated a suitable target position for each patient. Target force was determined manually on the software: therapists increased the forces applied by the footplates and asked the patients to move the pedals. Therefore therapists defined the appropriate target force for each patient. After the device software and hardware calibrations, the formal test session was conducted as follows:
1. Passive training: the patient first performed the passive training mode, where he/she was asked to sit passively on the device. Predefined trajectories (viz. sinusoidal and sawtooth signals) were imposed on the limbs. Therapeutically, this mode could be used for mobilization of the leg joints.
2. Active resistance training: the patient was asked to follow the same signals as he/she performed in passive training, with resistive forces applied by the footplates. The therapists repeated the training several times with different target signals and with different forces. This mode was designed to train strength, as well as timely coordination and adequate levels of leg strength.
3. Exergames: the patient was asked to play the exergames as described above, while the therapist could vary the forces and the game setting for difficulty. Therapeutically, this enabled training strength and coordination in a playful environment.
After performing the three training modes, the patients were asked to evaluate the training session and device with a user satisfaction questionnaire based on a smiley system and Visual Analog Scale (VAS). Additional feedback from the therapists was provided with the modified System Usability Scale (SUS) [36].
3.5Outcome measures
3.5.1User satisfaction
User satisfaction was evaluated with questionnaires for each patient and therapist. For patients, the user satisfaction evaluation considered device usability, training intensity, motivation, and general opinion. Patients answered five questions on a Visual Analog Scale (VAS), where the score was provided on a 100 mm long line. The most positive answer was always on the right side of the line (100 mm, VAS score: 100) and the most negative answer was always on the left side of the line (0 mm, VAS score: 0). The score interpretation of each question was done based on the approach of Huijgen et al. [37] where the scale was classified into three categories: not-satisfied for those with a score equal to or less than 30; average score for those with a score between 31 and 69; and satisfied for those with a score equal to or higher than 70.
Therapists provided additional feedback and answered user satisfaction questionnaires with the modified SUS. The questionnaire consists of ten questions about device usability and software manipulation, in addition to a question about the general impression about the device. The SUS score can range from 0 to 100 and gives clear and quantified idea about the usability and improvements needed [36]. Based on the literature, a score of 68 is considered as the average. Linked to the SUS, therapists were asked to provide any additional notes or comments. The results obtained from patient/therapist user satisfaction and additional comments are intended be taken into consideration in further device improvements.
To avoid bias in both SUS and VAS scoring, therapists were asked to answer the SUS questionnaire individually. The questions were formulated following the literature and using unbiased wording, structure and styling [38]. Regarding the patient satisfaction questionnaire (VAS), therapists were asked to help the children to understand and communicate the questions in a clear, unbiased and neutral manner.
3.5.2Performance parameters
The training device monitors and records quantitative performance data automatically during the training sessions. Individual performance data included forces applied on the footplates, positions of the feet and air pressure in the actuators. The data are recorded in real-time using the engineering software LabView, and exported off-line to Matlab, where analytical evaluation is carried out for each patient.
4.Results
Five paediatric patients with neuromuscular impairments and nine therapists participated in this study. The patients were aged between 7 and 16 years (one girl, age 13.0
4.1User satisfaction
VAS scores given by the four patients who performed the training sessions are presented in Table 3. All the patients adjudged the training with the interactive device to be as satisfactory (VAS of question 1
Table 3
Patients’ user satisfaction
Questions ( | Min | Max | Mean | Answers in VAS category | ||
---|---|---|---|---|---|---|
31–69 | ||||||
1. How interesting was the training with the device? | 70 | 90 | 77.5 | 0 | 0 | 4 |
2. Did you feel discomfort or pain during the training? | 10 | 100 | 72.5 | 1 | 0 | 3 |
3. Was the training intensity appropriate? | 20 | 100 | 62.5 | 1 | 1 | 2 |
4. Would you like to continue with such training? | 80 | 100 | 92.5 | 0 | 0 | 4 |
5. What is your general impression of the System? | 70 | 90 | 80 | 0 | 0 | 4 |
Abbreviations: Min: minimum, Max: maximum, SD: standard deviation. Scale: 0
4.2Performance parameters
Individual performance parameters during the three training modes were recorded. For illustration, original data records for a single patient during the three training modes are presented in Figs 4 and 5. These figures show representative data from one participant; the performance of other participants was similar.
1. Passive training: the patients were asked to sit passively while the device imposed predefined trajectories (Fig. 4).
2. Active resistance training: patients were asked to follow target position profiles, while the device was applying resistive forces on the footplates. All patients could understand the task and performed the training with no untoward events (Fig. 5).
3. Exergames: the patients played two games, while the device was operating in active resistance mode. All patients could understand the games, and high motivation and interaction could be observed.
Figure 4.
Original data record from patient 3 during passive training mode. The patient was asked to sit passively while the device was imposing predefined trajectories (position control mode).
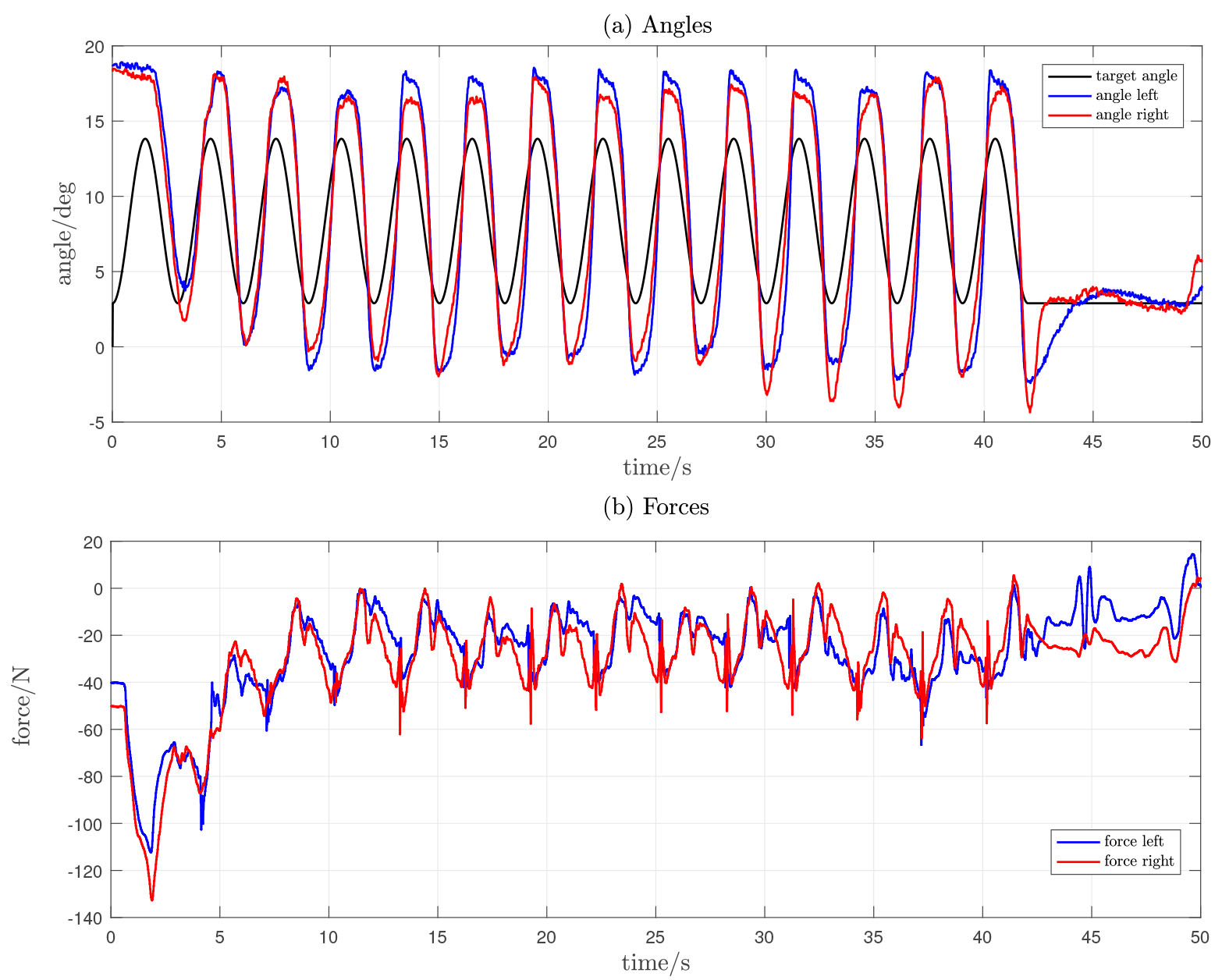
Figure 5.
Original data record from patient 3 during the active resistance training mode. The patient was asked to follow target position profiles, while the device was applying resistive forces to the footplates (force control mode).
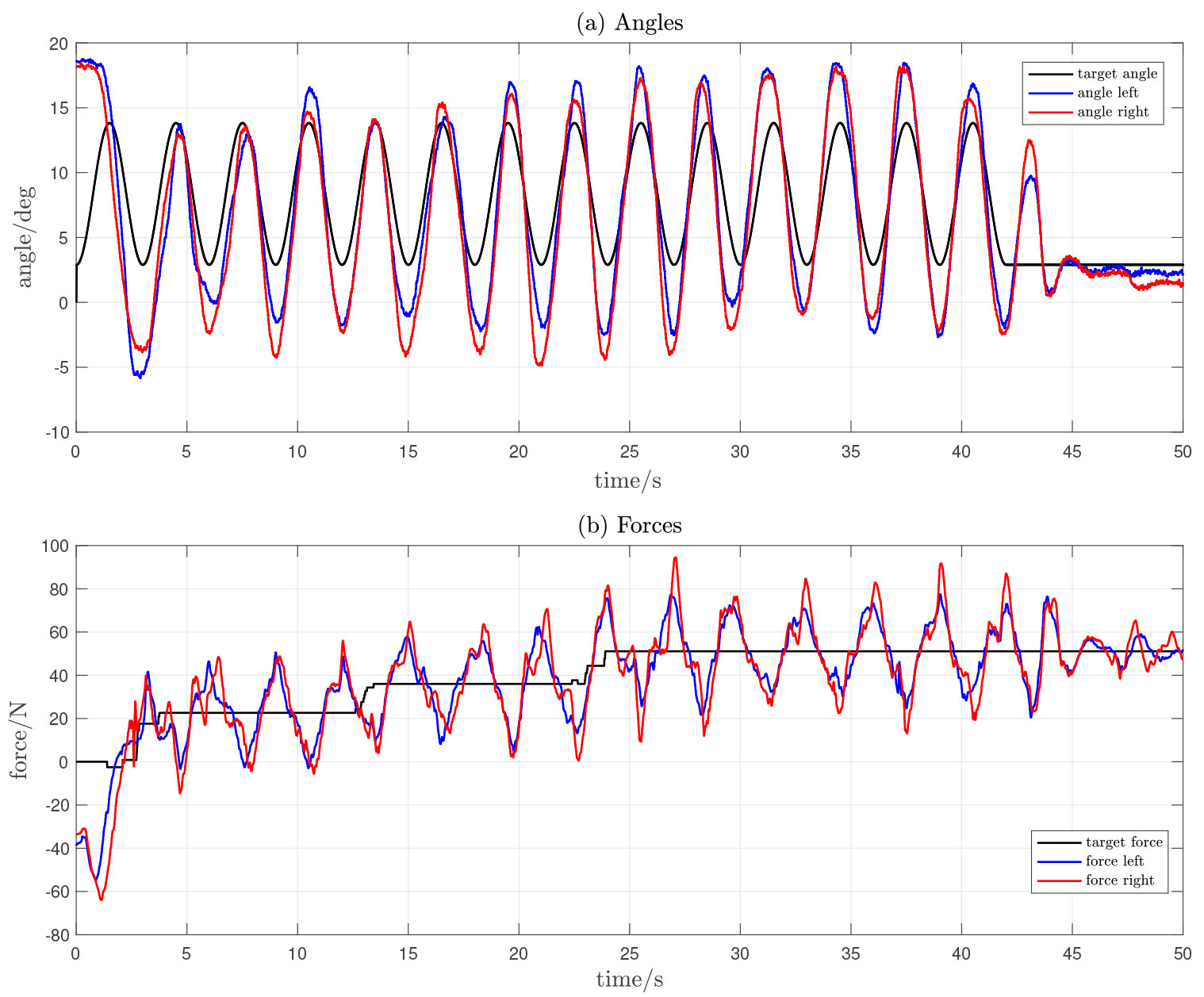
5.Discussion
The aim of this study was to evaluate the technical feasibility and usability of a new interactive leg-press training robot suitable for children with neuromuscular impairments. The usability analysis considered user acceptability for both patients and therapists. This preliminary evaluation involved five paediatric patients with different neuromuscular impairments, and nine therapists specialised in paediatric neurorehabilitation.
The main results showed that the device is feasible for paediatric rehabilitation applications, both in terms of technical implementability and usability acceptance. The developed training device is based on pneumatic actuators (cylinders), where sufficient air pressure is needed. Compressed air was supplied using a portable single-stage compressor. Employment of pneumatic cylinders could contributed to a simple pedal mechanism arrangement and compact design, and allowed the implementation of different control strategies. Force and position controller designs were done based on a simple plant model, and on proportional valve internal pressure controllers. Force and angle sensors enabled monitoring and recording the users’ dynamic behaviour and gave quantitative measures of the training. This can have a major importance for therapists to accurately plan and analyse the rehabilitation progress of their patients by monitoring the improvement in deviation between target and measured values. Despite this simple design, the controllers demonstrated accuracy and robustness for different training modes, and the actuators showed a high degree of controllability providing forces that are suitable for paediatric rehabilitation purposes.
In general, both patients and therapists were interested in and satisfied with the system. The patients’ average VAS scores and therapists’ SUS score, demonstrated that patients and the therapists rated the device and its usability as highly acceptable. Only one patient (Patient 3) rated the question “Was the training intensity appropriate?” into “not-satisfactory”, where he commented that the training duration was too short. The total test duration was 45 min including the calibration, setting changes and the three training modes. The calibration is needed only once in the first training session, and the passive training mode is needed only for familiarisation and for severely impaired patients. We believe that the duration and intensity, including active training and exergames, would be appropriate for formal therapeutic training. One patient reported pain or discomfort during the training, due to inaccuracy in the position controller strategy during the passive training mode. This patient experienced some pain on the knee level during the first training phase (passive training mode), due to a prior knee operation. Better selectivity of patients and improvements in training introduction are warranted.
Therapists assessed the device and its usability using SUS (System Usability Scale [36]) and an additional detailed feedback. The SUS score obtained from nine therapists was 61.2
The exergames employed for this study were simple two degrees of freedom games, where the patient performs in a two dimensional virtual environment while exercising. Compared to current state-of-the-art games and virtual reality scenarios [39, 40], the games developed here may appear monotone and uninteresting for paediatric patients after a certain time. However, despite the simplicity of the two exergames developed for the device, all patients showed high interest and enjoyment during training and wanted to increase the duration of the training session. Generally, the therapists praised the device interactivity, and the possibility to train the left and right legs independently.
Additional features and control strategies are needed to enable the assessment of recovery progress. In clinical and sports environments, in addition to muscle strengthening and musculoskeletal conditioning, leg-press devices can be employed as assessment tools. The one-repetition maximum test (1-RM) employs the leg-press form of exercise to evaluate the different performance parameters [41, 42]. Kirk et al. [43] used leg-press exercise for training and assessing gait function in adults with cerebral palsy, and Stravic et al. [44] employed a modified leg-press machine to assess differences in muscle power in stroke survivors. Furthermore, leg-press devices have been proved to be feasible for cardiopulmonary exercise testing and training [45, 46]. The interactive leg-press presented in this work could provide accurate quantitative measures for these kinds of test. These evaluation strategies might also be useful for paediatric neurorehabilitation recovery assessment.
Current leg-press training devices (i.e. LegoPress [33] and Allegro [32]) are designed mainly for adults’ rehabilitation and muscle strengthening and conditioning, in terms of design, actuators, and training modes. With a compact design, interactive training modes and exergames, the robotic system proposed in this study showed promise for paediatric rehabilitation applications.
A limitation of this study is that the current version of the device does not include small knee ranges of motion. An error occurred during the calibration phase for the patient suffering from limited knee range of motion, therefore, this patient (Patient 1) could not perform the training session. Despite the promising outcomes, further changes and improvements in the hardware and software are warranted, following the evaluations of patients and therapists.
While this study has served to establish technical feasibility of the device, further developments and evaluation studies will be required to assess clinical effectiveness. This would involve the addition of software to monitor and assess patients’ progress in rehabilitation, and the necessity to conduct controlled clinical studies to evaluate effectiveness in comparison to standard therapy and to similar devices.
6.Conclusion
This study proposed an interactive lower limb training device for children with different neuromuscular impairments. The aim of this study, which was to assess technical feasibility of the new paediatric device, is deemed to have been met, both in terms of technical feasibility and user acceptance. The experimental results show quantitatively that the proposed training modes are usable and that the numerical outcomes are satisfactory.
Ethics approval and consent to participate
The Ethics Review Board of the Canton of Zürrich in Switzerland reviewed the study protocol and decided by means of a jurisdictional inquiry that this study did not fall under the Human Research Act (Nr. Req-2018-00586). Written informed consent was obtained from the legal guardians of all the participating children prior to participation. Patients aged 14 and above provided written informed consent while younger ones provided oral agreement. The measurements were performed at a paediatric rehabilitation centre in Switzerland (University Children’s Hospital Zürrich, Rehabilitation Centre in Affoltern am Albis).
Competing interests
The authors declare that they have no competing interests.
Authors’ contributions
FC, KH, TN, HVH and VM designed the study. HVH and VM recruited the participants. FC did the data acquisition. FC and KH contributed to the analysis and interpretation of the data. FC wrote the manuscript; KH, TN, HVH and VM revised it critically for important intellectual content. All authors read and approved the final manuscript.
Acknowledgments
Matthias Schindelholz and Lukas Bichsel contributed to the technical development and construction of the leg-press device.
References
[1] | Colombo R, Sanguineti V, eds. Rehabilitation robotics: technology and application. London, UK: Elsevier; (2018) . |
[2] | Michmizos KP, Krebs HI. Pediatric robotic rehabilitation: Current knowledge and future trends in treating children with sensorimotor impairments. NeuroRehabilitation. (2017) ; 41: (1): 69–76. |
[3] | Qian Z, Bi Z. Recent development of rehabilitation robots. Advances in Mechanical Engineering. (2015) ; 7: (2). |
[4] | Krebs HI, Volpe BT. Rehabilitation robotics. Handbook of Clinical Neurology. (2013) ; 110: : 283–294. |
[5] | Bayon C, Raya R. Robotic therapies for children with cerebral palsy: A systematic review. Translational Biomedicine. (2016) ; 7: (1): 1–10. Available from: http://www.transbiomedicine.com/translational-biomedicine/robotic-therapies-for-children-with-cerebral-palsy-a-systematic-review.php?aid=8788. |
[6] | Riener R. Rehabilitation robotics. Foundations and Trends in Robotics. (2012) ; 3: (1–2): 1–137. |
[7] | Lerner Z, Damiano D, Bulea T. A lower-extremity exoskeleton improves knee extension in children with crouch gait from cerebral palsy. Science Translational Medicine. (2017) ; 9: (404). |
[8] | Kim SK, Park D, Yoo B, Shim D, Choi Jo, Choi Ty, et al. Overground robot-assisted gait training for pediatric cerebral palsy. Sensors. (2021) ; 21: (6). |
[9] | Meyer-Heim A, Hedel HJAV. Robot-assisted and computer-enhanced therapies for children with cerebral palsy: Current state and clinical. Seminars in Pediatric Neurology. (2013) ; 20: (2): 139–145. |
[10] | Fasoli SE, Ladenheim B, Mast J, Krebs HI. New horizons for robot-assisted therapy in pediatrics. American Journal of Physical Medicine & Rehabilitation. (2012) ; 91: (11): 280–289. |
[11] | Meyer-Heim A, Borggraefe I, Ammann-Reiffer C, Berweck S, Sennhauser FH, Colombo G, et al. Feasibility of robotic-assisted locomotor training in children with central gait impairment. Developmental Medicine and Child Neurology. (2007) ; 49: (12): 900–906. |
[12] | Wallard L, Dietrich G, Kerlirzin Y, Bredin J. Robotic-assisted gait training improves walking abilities in diplegic children with cerebral palsy. European Journal of Paediatric Neurology. (2017) ; 21: (3): 557–564. |
[13] | Borggraefe I, Kiwull L, Schaefer JS, Koerte I, Blaschek A, Meyer-Heim A, et al. Sustainability of motor performance after robotic-assisted treadmill therapy in children: An open, non-randomized baseline-treatment study. European Journal of Physical and Rehabilitation Medicine. (2010) ; 46: (2): 125–131. |
[14] | Smania N, Bonetti P, Gandolfi M, Cosentino A, Waldner A, Hesse S, et al. Improved gait after repetitive locomotor training in children with cerebral palsy. American Journal of Physical Medicine and Rehabilitation. (2011) ; 90: (2): 137–149. |
[15] | Bayón C, Martín-Lorenzo T, Moral-Saiz B, Ramírez Ó, Pérez-Somarriba Á, Lerma-Lara S, et al. A robot-based gait training therapy for pediatric population with cerebral palsy: Goal setting, proposal and preliminary clinical implementation. Journal of NeuroEngineering and Rehabilitation. (2018) ; 15: (1): 1–15. |
[16] | Murie-Fernández M, Irimia P, Martínez-Vila E, John Meyer M, Teasell R. Rehabilitation after stroke. Neurologia. (2010) ; 25: (3): 189–169. Available from: doi: 10.1016/S2173-5808(10)70036-5. |
[17] | Bayón C, Lerma S, Ramírez O, Serrano JI, Del Castillo MD, Raya R, et al. Locomotor training through a novel robotic platform for gait rehabilitation in pediatric population: Short report. Journal of NeuroEngineering and Rehabilitation. (2016) ; 13: (1): 1–6. Available from: doi: 10.1186/s12984-016-0206-x. |
[18] | Zeng X, Zhu G, Yue L, Zhang M, Xie S. Feasibility study of SSVEP-based passive trainig on an ankle rehabilitation robot. Journal of Healthcare Engineering. (2017) ; 2017: : 1–9. |
[19] | Eiammanussakul T, Sangveraphunsiri V. A lower limb rehabilitation robot in sitting position with a review of training activities. Journal of Healthcare Engineering. (2018) ; 2018: : 1–18. |
[20] | Meng W, Liu Q, Zhou Z, Ai Q, Sheng B, Shane S. Recent development of mechanisms and control strategies for robot-assisted lower limb rehabilitation. Mechatronics. (2015) ; 31: : 132–145. |
[21] | Saglia JA, Tsagarakis NG, Dai JS, Caldwell DG. A high-performance redundantly actuated parallel mechanism for ankle rehabilitation. International Journal of Robotics Research. (2009) ; 28: (9): 1216–1227. |
[22] | Chrif F, Nef T, Lungarella M, Dravid R, Hunt KJ. Control design for a lower-limb paediatric therapy device using linear motor technology. Biomedical Signal Processing and Control. (2017) ; 38: : 119–127. |
[23] | Chen K, Xiong B, Ren Y, Dvorkin AY, Gaebler-Spira D, Sisung CE, et al. Ankle passive and active movement training in children with acute brain injury using a wearable robot. Journal of Rehabilitation Medicine. (2018) ; 50: (1): 30–36. |
[24] | Wu YN, Hwang M, Ren Y, Gaebler-Spira D, Zhang LQ. Combined passive stretching and active movement rehabilitation of lower-limb impairments in children with cerebral palsy using a portable robot. Neurorehabilitation and Neural Repair. (2014) ; 25: (4): 378–385. |
[25] | Huang X, Naghdy F, Naghdy G, Du H, Todd C. The combined effects of adaptive control and virtual reality on robot-assisted fine hand motion rehabilitation in chronic stroke patients: A case study. Journal of Stroke and Cerebrovascular Diseases. (2018) ; 27: (1): 221–228. |
[26] | Park DS, Lee DG, Lee K, Lee GC. Effects of virtual reality training using Xbox Kinect on motor function in stroke survivors: A preliminary study. Journal of Stroke and Cerebrovascular Diseases. (2017) ; 26: (10): 2313–2319. |
[27] | Gerber CN, Kunz B, Van Hedel HJA. Preparing a neuropediatric upper limb exergame rehabilitation system for home-use: A feasibility study. Journal of NeuroEngineering and Rehabilitation. (2016) ; 13: (1): 1–12. |
[28] | Padulo J, Migliaccio GM, Ardigò LP, Leban B, Cosso M, Samozino P. Lower limb force, velocity, power capabilities during leg press and squat movements. International Journal of Sports Medicine. (2017) ; 38: (14): 1083–1089. |
[29] | Orssatto LBdR, de Moura BM, Sakugawa RL, Radaelli R, Diefenthaeler F. Leg press exercise can reduce functional hamstring:quadriceps ratio in the elderly. Journal of Bodywork and Movement Therapies. (2018) ; 22: (3): 592–597. |
[30] | Fry AC, Irwin CC, Nicoll JX, Ferebee DE. Muscular strength and power in 3- to 7-year-old children. Pediatric Exercise Science. (2015) aug; 27: (3): 345–354. Available from: doi: 10.1123/pes.2014-0152. |
[31] | Ioakimidis P, Gerodimos V, Kellis E, Alexandris N, Kellis S. Combined effects of age and maturation on maximum isometric leg press strength in young basketball players. The Journal of Sports Medicine and Physical Fitness. (2004) dec; 44: (4): 389–97. Available from: http://www.ncbi.nlm.nih.gov/pubmed/15758851. |
[32] | Pfeifer R, Iida F, Lungarella M. Cognition from the bottom up: On biological inspiration, body morphology, and soft materials. Trends in Cognitive Sciences. (2014) ; 18: (8): 404–413. |
[33] | Olivier J, Jeanneret M, Bouri M, Bleuler H. The LegoPress: a rehabilitation, performance assessment and training device mechanical design and control. In: Auvray M, Duriez C, eds. Haptics: Neuroscience, Devices, Modeling, and Applications. Berlin, Germany: Springer; (2014) . pp. 198–205. |
[34] | Wulf G, Lewthwaite R. Optimizing performance through intrinsic motivation and attention for learning: The OPTIMAL theory of motor learning. Psychonomic Bulletin and Review. (2016) ; 23: (5): 1382–1414. |
[35] | Wulf G, Shea C, Lewthwaite R. Motor skill learning and performance: A review of influential factors. Medical Education. (2010) ; 44: (1): 75–84. |
[36] | Bangor A, Staff T, Kortum P, Miller J, Staff T. Determining what individual SUS scores mean: Adding an adjective rating scale. Journal of Usability Studies. (2009) ; 4: (3): 114–123. |
[37] | Huijgen BC, Vollenbroek-Hutten MM, Zampolini M, Opisso E, Bernabeu M, Van Nieuwenhoven J, et al. Feasibility of a home-based telerehabilitation system compared to usual care: Arm/hand function in patients with stroke, traumatic brain injury and multiple sclerosis. Journal of Telemedicine and Telecare. (2008) ; 14: (5): 249–256. |
[38] | Brooke J. SUS: A retrospective. Journal of Usability Studies. (2013) ; 8: (2): 29–40. |
[39] | Chanpimol S, Seamon B, Hernandez H, Harris-love M, Blackman MR. Virtual reality and serious games in neurorehabilitation of children and adults: Prevention, plasticity and participation. Pediatric Physical Therapy. (2017) ; 29: : 23–36. |
[40] | Ravi DK, Kumar N, Singhi P. Effectiveness of virtual reality rehabilitation for children and adolescents with cerebral palsy: An updated evidence-based systematic review. Physiotherapy. (2017) ; 103: (3): 245–258. |
[41] | Rivière J, Rossi J, Jimenez-Reyes P, Morin JB, Samozino P. Where does the one-repetition maximum exist on the force-velocity relationship in squat? International Journal of Sports Medicine. (2017) ; 38: : 1035–1043. |
[42] | Ferraresi C, Baldissera V, Perez SEA, Júnior EM, Bagnato VS, Parizotto NA. One-repetition maximum test and isokinetic leg extension and flexion: Correlations and predicted values. Isokinetics and Exercise Science. (2013) ; 21: (1): 69–76. |
[43] | Kirk H, Geertsen SS, Lorentzen J, Krarup KB, Bandholm T, Nielsen JB. Explosive resistance training increases rate of force development in ankle dorsiflexors and gait function in adults with Cerebral Palsy. Journal of Strength and Conditioning Research. (2016) ; 30: (10): 2749–2760. |
[44] | Stavric VA, McNair PJ. Optimizing muscle power after stroke: A cross-sectional study. Journal of NeuroEngineering and Rehabilitation. (2012) ; 9: (1): 1–8. |
[45] | Chrif F, Nef T, Hunt KJ. Investigation of cardiopulmonary exercise testing using a dynamic leg press and comparison with a cycle ergometer. BMC Sports Science, Medicine & Rehabilitation. (2018) ; 10: : 5. |
[46] | Chrif F, Nef T, Hunt KJ. Technical feasibility of constant-load and high-intensity interval training for cardiopulmonary conditioning using a re-engineered dynamic leg press. (2019) ; 1: : 26. |