Accuracy of a patient-specific instrumentation for coronal plane alignment of an anatomic alignment total knee arthroplasty system: A radiographic study
Abstract
BACKGROUND:
Patient-individualised anatomic alignment in total knee arthroplasty (TKA) requires exact positioning of the tibial and femoral components. Patient-specific instrumentation (PSI) may be advantageous for implantation. However, the role of PSI in the instrumentation of such knee designs has not been investigated.
OBJECTIVE:
The aim of this study was to investigate the accuracy of a PSI system designed for patient-individualised anatomic alignment.
METHODS:
Fifty-four patients from a single centre were consecutively enrolled in this study. Patient-specific femoral and tibial cutting guides were manufactured using 3D models from computed tomography (CT) scans. All patients received an anatomic TKA implant design through an extension gap first technique. Postoperative radiography was taken, and implant component alignment and leg alignment were compared to the preoperative planning.
RESULTS:
Thirty-four patients were evaluable. Mean differences between planned angles values obtained from CT scans and the measured radiographic values were small and not significantly different from zero.
CONCLUSIONS:
Implantation of an anatomic knee design that allows individual component alignment using PSI is feasible. The percentage of component misalignment in the coronal plane was remarkably low. Whether this leads to clinical benefits requires further verification.
1.Introduction
Obtaining alignment and soft-tissue balance are key to a successful total knee arthroplasty (TKA). Accurate implant coronal plane alignment along with equal flexion and extension gaps are essential to achieve this objective [1].
Conventional measured resection TKA performed with patient-specific instrumentation (PSI) aims to achieve a neutral axis alignment and is solely oriented in relation to bone landmarks [2]. A neutrally aligned leg axis is still considered the gold standard in knee arthroplasty [3]. However, other alignment philosophies that are based on the reconstruction of native anatomy have emerged. In some cases, an undercorrection of mechanical alignment has been recommended [2, 4, 5]. Among these, philosophies such as kinematic and anatomic alignment are becoming increasingly common [6]. Anatomical, personalised alignment tolerates deviations from the neutral mechanical axis while minimising the need for performing ligament releases [5].
The restoration of a patient’s native anatomy during TKA can be challenging. The surgeon must decide to what extent the patient’s tibial and femoral bone morphology, which is typically affected by osteoarthritis, can be followed. A coronal plane joint line orientation of the knee is not perpendicular to the mechanical axis but is in 3
Although PSI in measured resection TKA has not been proven to be superior to conventional instrumentation in terms of alignment [8], it has been demonstrated to be advantageous when applying surgical techniques that are based on patient-specific alignment [5, 9].
The present study assesses the first TKA implant system (4-motion, ARTIQO GmbH, Lüdinghausen, Germany) which is intended for up to 3
The aim of this study was to investigate the accuracy of the planning of the 4-motion PSI system designed for patient-individualised anatomic alignment.
Figure 1.
Visualisation of the preoperative (left) and the planned hip-knee-ankle (HKA) with the relevant pre- and postoperative angles (a). Three-dimensional simulation of the planned implant position with relevant angles, implant sizes and bone cuts (b). The knee with resected bone (c), the projection of the implant on the unresected bone (d) and the preoperative knee with the relevant angles and implant sizes are also visualised.
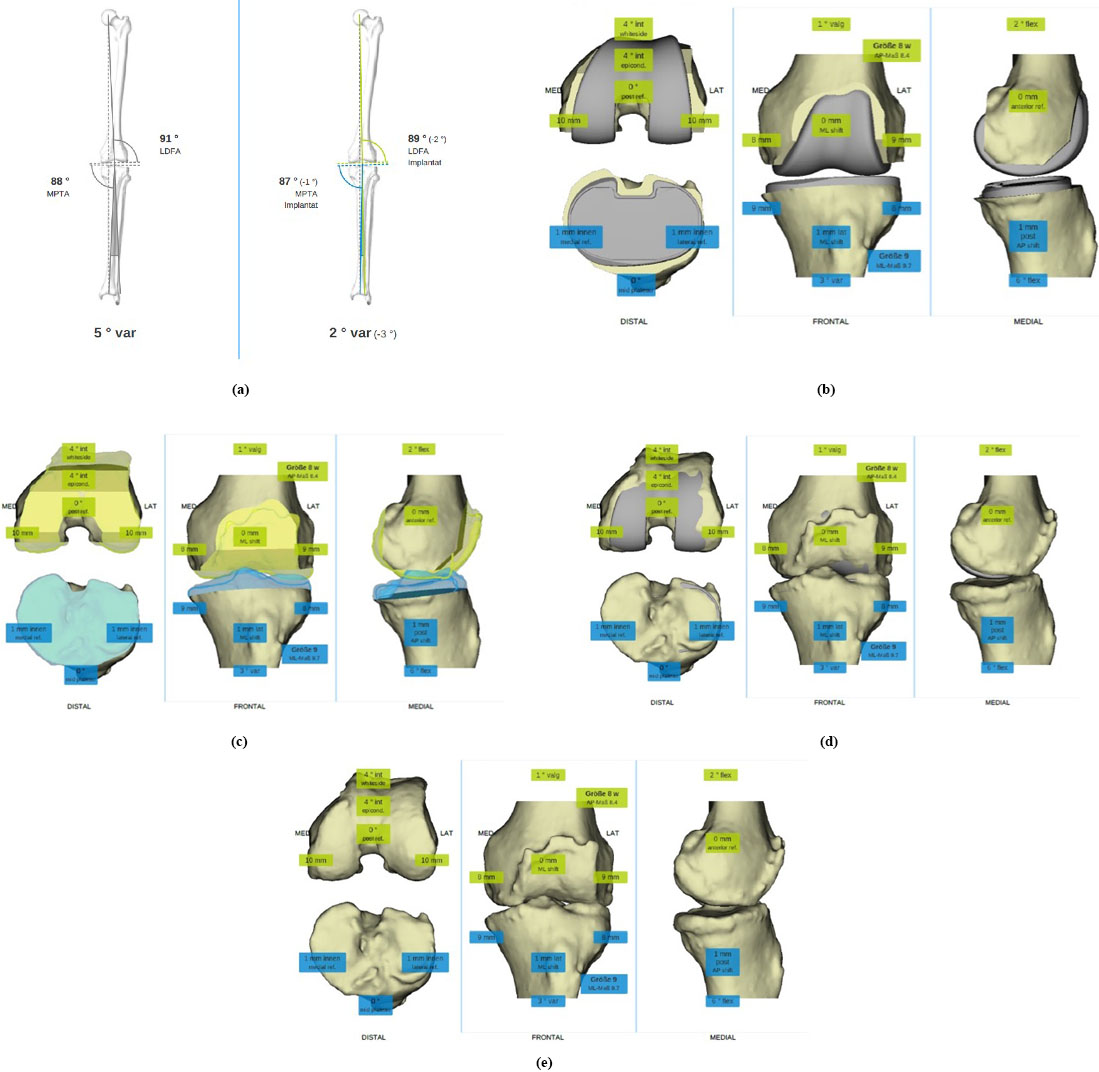
Figure 2.
Patient-specific cutting guides for tibial (a), distal femoral (b) and ventral/dorsal femoral resection. The white * marks the flange coupling to the osteophyte. The grey arrow marks the pinholes for the tensioner device.
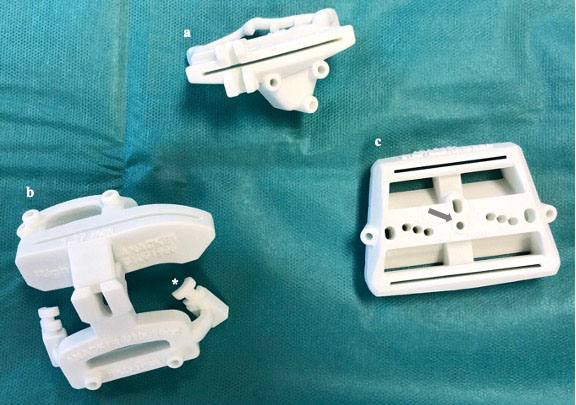
Figure 3.
Patient-specific cutting guides securely positioned and fixed to the intact osteophytes on femoral site before the femoral resection.
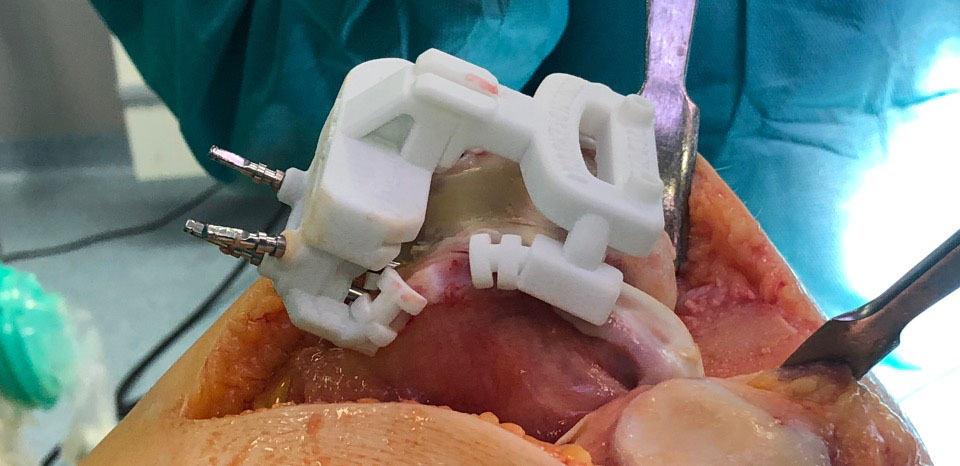
2.Methods and patients
From January to July 2019, 54 patients from a single centre were consecutively enrolled in this study. The patients’ informed consent was obtained prior to study commencement, and the local ethics review committee approved this study (Ethics Committee no. BO/44/2018). In all the patients, TKA with a fully cemented cruciate retaining 4-motion implant system was performed using PSI with patient-specific cutting guides (Medivation AG, Brugg, Switzerland). All procedures were carried out by a single senior surgeon specialised in TKA (KM). A preoperative computed tomography (CT) scan of the affected leg was obtained for each patient. A defined CT acquisition protocol was used (proximal femur and distal tibia: slice distance of 2.0 mm; distal femur and proximal tibia: slice distance of 0.5 mm). A three-dimensional (3D) model of the leg anatomy was retrieved from each CT dataset. Dedicated software was used to perform the semi-automatic segmentation step. Patient-specific cutting femoral and tibial guides were then planned and designed using the resulting 3D models. The 3D models were coupled to osteophytes and bone structures at the border of the joint surface in order to provide a stable fit to the patient’s anatomy. A planning website (Webplanning, Medivation) was used to present the resultant plan together with the designed patient-specific cutting guides to the surgeon. The website had a 3D visualisation of all bones and cutting planes so that the surgeon could adjust the planning, including the size and position of the implants (Fig. 1). The planned leg axis (between 0
Figure 4.
A laminar tensioner device (ARTIQO GmbH) is inserted, fixed to the femoral cut guide and spread by hand to assess the extension gap.
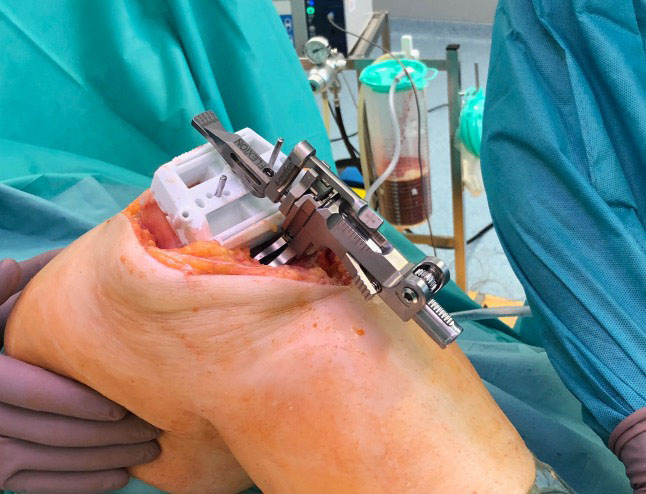
In accordance with our clinic’s standard practice, full leg weight-bearing AP radiographs of all legs were taken 3 weeks prior to surgery and at the 8-week postoperative visit (Fig. 5). All radiographs were analysed using the open-source software Ginkgo-CADx (www.ginkgo-cadx.com) by a single assessor (KM). The following angles were defined: hip-knee-ankle (HKA), which is a measure of lower limb alignment and defined as the angle between the mechanical axes of the femur and the tibia [11]; the lateral distal femoral angle (LDFA), which is the angle between the mechanical axis of the femur and the knee joint line of the femur [12]; the medial proximal tibial angle (MPTA), which is the angle between the tibial mechanical axis (drawn from the centre of the knee to the centre of the talus) and the knee joint line of the tibia [11]; physiological LDFA, which is between 85
Figure 5.
The pre- and postoperative full leg weight-bearing AP radiographs show the resulting correction of the hip-knee-ankle and the anatomically aligned implants.
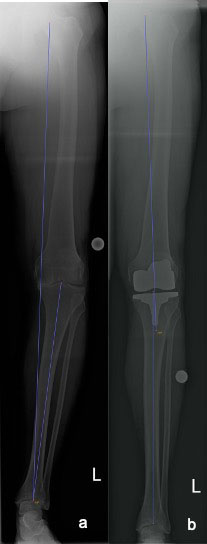
For the purpose of this study, only radiographs with a flawless quality were accepted. All of the following features had to be achieved in each radiograph: clearly visible ankle joint, clearly visible hip rotation centre, clearly visible tibia plateau (no tilting), clearly visible femoral condyles and implant (no tilting), absent twisted shooting direction and absence of artefacts [7, 13].
Two of the 54 images could not be evaluated because of slightly low image quality. In nine cases, the relevant points (especially the femoral head centre) were not exactly recognisable. In nine cases, artefacts or slight malpositioning of the leg in the X-ray images, such as slight knee flexion (more than estimated 0
The postoperative measurements of the HKA, LDFA and MPTA were subsequently compared with the preoperative planning. Malalignment was defined as a measurement that was
2.1Statistics
The reliability of the measurements was confirmed by calculating intraclass correlations to determine absolute agreement of the measurements by means of two-way mixed-effects models for individual measurements. After violation of the normality assumption was verified, descriptive statistics (mean, standard deviation, and range) were calculated. A one-sample t-test was used to assess for the presence of any deviation from the planned alignment. The proportion of differences within
3.Results
Thirty-one patients had a preoperative varus leg axis, whereas the three remaining patients had preoperative neutral alignment. No difficulties using the cutting blocks were reported by the surgeon. For three cases, a downsizing of the femoral component was required, and a recut was performed for the smaller size. The downsizing was required because the implant was too wide in the mediolateral dimension or in order to maintain size compatibility to a smaller tibial implant. In seven cases, the tibia implant was smaller than planned; recutting was not required in any of these patients. No additional soft tissue releases were necessary in any of the patients. The mean duration of surgery was 40.4
The mean preoperative HKA was
The mean preoperative and postoperative MPTAs were 85.9
Table 1
Difference between the planned angles obtained from preoperative and postoperative radiography
Angle | Signed deviation | Unsigned (absolute) deviation | |||||
---|---|---|---|---|---|---|---|
Mean | Range | Outside | Outside | Mean | Range | ||
Global – HKA | 0.4 | 0.246 | 5 (14.7%) | 16 (47.1%) | 1.8 | 0.0, 4.0 | |
Femur – LDFA | 0.317 | 5 (14.7%) | 6 (17.6%) | 1.4 | 0.1, 5.1 | ||
Tibia – MPTA | 0.1 | 0.851 | 1 (2.9%) | 8 (23.5%) | 1.3 | 0.0, 4.0 |
Abbreviations: SD, standard deviation.
For all 34 knees, except the unsigned outcome variables, no major violation of the normality assumptions was observed for the differences between the target angles and measured angles. All mean differences between planned angles values obtained from preoperative and postoperative radiography were small and not significantly different from zero (Table 1). A total of 11 patients (32.4%) had TKAs that resulted in a postoperative valgus HKA (i.e., HKA
4.Discussion
Anatomic alignment, initially introduced in the 1980s by Hungerford and Krackow [15], is a technique that aims to obtain an oblique joint line (3
The primary goal of the present study was to investigate the accuracy of the planning of a PSI system designed for the alignment of the femoral and tibial implant components of a TKA that allows patient-individualised anatomic alignment.
Comparing the risk of malalignment (employing a 3
Table 2
Proportion of malaligned knees, comparison of measured resection PSI, standard instrumentation and anatomic alignment PSI
Angle | Measured resection PSI [8] | Standard instrumentation [8] | This study |
---|---|---|---|
HKA | 20.2% (15.6–25.1%) | 25.7% (21.6–30.0%) | 14.7% (5.0%–31.0%) |
Tibia Coronal Plane | 10.5% (5.9–16.1%) | 7.9% (4.9–11.5%) | 2.9% (0.1–15.3%) |
Femur Coronal Plane | 10.3% (6.2–15.3%) | 11.0% (6.5–16.3%) | 14.7% (5.0%–31.0%) |
Abbreviations: HKA: hip-knee-ankle; PSI: patient-specific instrumentation.
Preliminary evidence shows that tibial component malalignment, in both varus and valgus directions, results in increases in contact stress and pressure [17] and a higher failure rate than in a malalignment of the femoral component. Moreover, a significant increase in the failure rate results from compensation for a varus or valgus orientation of the tibial component via an alteration of the femoral component alignment [18]. Furthermore, a key criticism of the gap-balancing technique is that the alignment of the components is based on the proximal tibial cut; hence, the risk of an initial error in the alignment being carried through to all subsequent cuts occurs [19, 20]. In the present study, the rate of malpositioning of the tibial component appears to be particularly low compared with that presented in the literature, from which we infer that the instruments are particularly suited to this surgical approach.
Besides the alignment accuracy of the cut blocks in the operation, intraoperative factors, such as pin/resection accuracy and final positioning/cementing of the implant components, may also affect the final alignment and postoperative results. However, the named intraoperative factor also affects implant positioning in conventional TKA.
The limitations of the present study are its retrospective study design and the relatively small number of patients that were included. Due to the very high quality standard we applied to the radiographs, 20 patients had to be excluded. Nonetheless, we feel that this reduced the confounder of inaccurate data from the radiographs. Another major limitation of the present study is the fact that postoperative alignment measurements were based on standard radiographs rather than on CTs. However, standard radiography has a satisfactory precision (coefficient of repeatability, 0.8
5.Conclusion
The implantation of an anatomical knee design that allows individualised component alignment with the use of PSI is feasible. The accuracy of component alignment and overall alignment appears to be consistent with meta-analysed values from the literature, although the percentage of tibial component malalignment in the coronal plane was notably low.
Acknowledgments
The authors thank Peter Fennema from AMR for statistical advice and for editing a draft of this manuscript. They also thank Mario Frank from Artiqo GmbH for his help in technical issues and Tobias Schwägli (CTO Medivation AG) for providing the planning data.
Conflict of interest
None to report.
References
[1] | Daines BK, Dennis DA. Gap balancing vs. measured resection technique in total knee arthroplasty. Clin Orthop Surg. (2014) ; 6: (1): 1-8. |
[2] | Bellemans J, Colyn W, Vandenneucker H, et al. The Chitranjan Ranawat award: is neutral mechanical alignment normal for all patients? The concept of constitutional varus. Clin Orthop Relat Res. (2012) ; 470: (1): 45-53. |
[3] | Thienpont E, Cornu O, Bellemans J, et al. Current opinions about coronal plane alignment in total knee arthroplasty: A survey article. Acta Orthop Belg. (2015) ; 81: (3): 471-7. |
[4] | Vanlommel L, Vanlommel J, Claes S, et al. Slight undercorrection following total knee arthroplasty results in superior clinical outcomes in varus knees. Knee Surg Sports Traumatol Arthrosc. (2013) ; 21: (10): 2325-30. |
[5] | Hommel H, Perka C, Pfitzner T. Preliminary results of a new surgical technique in total knee arthroplasty (TKA) using the native ligament tension for femoral implant positioning in varus osteoarthritis. Arch Orthop Trauma Surg. (2016) ; 136: (7): 991-7. |
[6] | Riviere C, Iranpour F, Auvinet E, et al. Alignment options for total knee arthroplasty: A systematic review. Orthop Traumatol Surg Res. (2017) ; 103: (7): 1047-56. |
[7] | Moreland JR, Bassett LW, Hanker GJ. Radiographic analysis of the axial alignment of the lower extremity. J Bone Joint Surg Am. (1987) ; 69: (5): 745-9. |
[8] | Thienpont E, Schwab PE, Fennema P. Efficacy of patient-specific instruments in total knee arthroplasty: A systematic review and meta-analysis. J Bone Joint Surg Am. (2017) ; 99: (6): 521-30. |
[9] | Woon JTK, Zeng ISL, Calliess T, et al. Outcome of kinematic alignment using patient-specific instrumentation versus mechanical alignment in TKA: A meta-analysis and subgroup analysis of randomised trials. Arch Orthop Trauma Surg. (2018) ; 138: (9): 1293-303. |
[10] | Whiteside LA. Soft tissue balancing: the knee. J Arthroplasty. (2002) ; 17: (4 Suppl 1): 23-7. |
[11] | Paley D. Normal lower limb alignment and joint orientation. In: Paley D, editor. Principles of deformity correction. Berlin, Heidelberg: Springer Berlin Heidelberg; (2002) ; pp. 1-18. |
[12] | Paley D, Herzenberg JE, Tetsworth K, et al. Deformity planning for frontal and sagittal plane corrective osteotomies. Orthop Clin North Am. (1994) ; 25: (3): 425-65. |
[13] | Marques Luís N, Varatojo R. Radiological assessment of lower limb alignment. EFORT Open Rev. (2021) ; 6: (6): 487-94. |
[14] | Thienpont E, Schwab PE, Fennema P. A systematic review and meta-analysis of patient-specific instrumentation for improving alignment of the components in total knee replacement. Bone Joint J. (2014) ; 96-B: (8): 1052-61. |
[15] | Hungerford DS, Krackow KA. Total joint arthroplasty of the knee. Clin Orthop Relat Res. (1985) ; 192: : 23-33. |
[16] | Ghosh KM, Merican AM, Iranpour-Boroujeni F, et al. Length change patterns of the extensor retinaculum and the effect of total knee replacement. J Orthop Res. (2009) ; 27: (7): 865-70. |
[17] | Innocenti B, Bellemans J, Catani F. Deviations from optimal alignment in TKA: is there a biomechanical difference between femoral or tibial component alignment? J Arthroplasty. (2016) ; 31: (1): 295-301. |
[18] | Ritter M, Davis K, Meding J, et al. The effect of alignment and BMI on failure of total knee replacement. J Bone Joint Surg Am. (2011) ; 93A: (17): 1588-96. |
[19] | Insall J, Ranawat CS, Scott WN, et al. Total condylar knee replacement: preliminary report. Clin Orthop Relat Res. (1976) ; 120: : 149-54. |
[20] | Insall JN, Binazzi R, Soudry M, et al. Total knee arthroplasty. Clin Orthop Relat Res. (1985) ; 192: : 13-22. |
[21] | Babazadeh S, Dowsey MM, Bingham RJ, et al. The long leg radiograph is a reliable method of assessing alignment when compared to computer-assisted navigation and computer tomography. Knee. (2013) ; 20: (4): 242-9. |
[22] | Lonner JH, Laird MT, Stuchin SA. Effect of rotation and knee flexion on radiographic alignment in total knee arthroplasties. Clin Orthop Relat Res. (1996) ; 331: : 102-6. |