H2S attenuates the myocardial fibrosis in diabetic rats through modulating PKC-ERK1/2MAPK signaling pathway
Abstract
OBJECTIVE
To investigate the roles and underlying mechanism of exogenous H
METHODS:
A total of 40 SD rats were randomly divided into 4 groups: control group, STZ group, STZ
RESULTS:
Comparing to control group, the collagen deposition of myocardial matrix remarkably increased in the STZ group, and almost all the proteins that are relative to myocardial fibrosis, inflammatory and signaling pathway show an overexpression, except for PPARG and NF-
CONCLUSION:
The myocardial fibrosis in DM rats can be attenuated effectively by exogenous H
1.Introduction
Diabetic cardiomyopathy (DCM) is one of the major chronic complications of DM, in which myocardial fibrosis is considered as the main pathological change [1]. Pathogenesis of myocardial fibrosis is quite complicated, including oxidative stress, inflammatory responses and cell apoptosis. Many studies have confirmed the close correlation between the collagen remodeling in myocardial fibrosis and dysregulation of MMPs/TIMPs expressions. Besides, as a key mediator in fibrosis [2], CTGF is involved in the fibrotic processes of multiple organs, such as kidney, liver and lung, and, according to some literatures [3, 4], CTGF also participates in the myocardial fibrosis of DM [5]. It has been found that H
It has been reported in some studies that activations of TGF-
2.Material and methods
2.1Experimental animals and reagents
The Animal Ethics Committee of University of South China (Hengyang, China) has approved this experimental protocol. Forty male adult SD rats (weighing 280
2.2Preparation of models
A total of 40 male SD rats were randomly assigned to 4 groups: control group, DM group (STZ group), H
2.3Extraction of myocardial tissues
Eight weeks later, rats were executed immediately followed by thoracotomy to remove the heart. Heart was rinsed using normal saline to purge the residual blood, and the saline on the heart was dried using filter paper. In this study, only left ventricle and interventricular septum were preserved, from which tissues were extracted and then mounted in 10% formaldehyde for Masson staining. Remaining myocardial tissues were preserved in a
2.4Observing the myocardial collagen deposition via Masson staining
The mounted myocardial tissues in left ventricle of rat in 4 groups were regularly embedded using paraffin and sliced into sections followed by Masson staining. Thereafter, tissues were placed under the inverted microscope for observation, in which myocardial cells were in red and collagen fibers in blue.
2.5Detecting the protein expressions of collagen-I, collagen-III, CTGF and MMPs/TIMPs in myocardial tissues via western blotting
Proteins extracted from myocardial tissues in each group were used for quantification via BCA method. Extracted proteins, after being heated for degeneration, were prepared for SDS-PAGE, and then electronically transferred onto the PVDF membrane. After the membrane was blocked using 5% BSA for 2 h, primary antibodies of collagen-I, collagen-III, CTGF, MMPs/TIMPs (diluted at 1:400), and GAPDH (internal reference; diluted at 1:1000) were added onto the membrane for incubation at 37
2.6Detecting the protein expressions of TGF-β 1, HSP70, HSP90, NF-κ Bp65, TNF-α and PPARG in myocardial tissues via western blotting
Samples of myocardial tissues in each group, after being sufficiently grinded, were used for protein quantification. After SDS-PAGE, the proteins were electronically transferred onto a PVDF membrane which was then incubated using the primary antibodies of TGF-
2.7Detecting the protein expressions of PKCα and ERK1/2 in myocardial tissues via western blotting
Proteins extracted from myocardial tissues in each group were used for protein quantification. After SDS-PAGE, the proteins were electronically transferred onto a PVDF membrane which was then incubated using the primary antibodies of PKC
2.8Statistical analysis
SPSS 18.0 software was applied to analysis experimental data. Measurement data were presented as mean
3.Results
3.1Results of Masson staining
Masson staining results of myocardial tissues in left ventricle of rat in each group are shown in Fig. 1, in which the blue-stained substance is collagen fiber. Compared to control group, we observed that for rats in the STZ group, myocardium was in malalignment and collagen fiber deposition in matrix obviously increased, suggesting the obvious myocardial fibrosis. However, in comparison with the STZ group, we observed that myocardial arrangement was remarkably ameliorated and the blue-stained collagen fiber deposition was significantly reduced in rats in the STZ
Figure 1.
Pathological changes of myocardium assessed by Masson staining (Images were obtained at
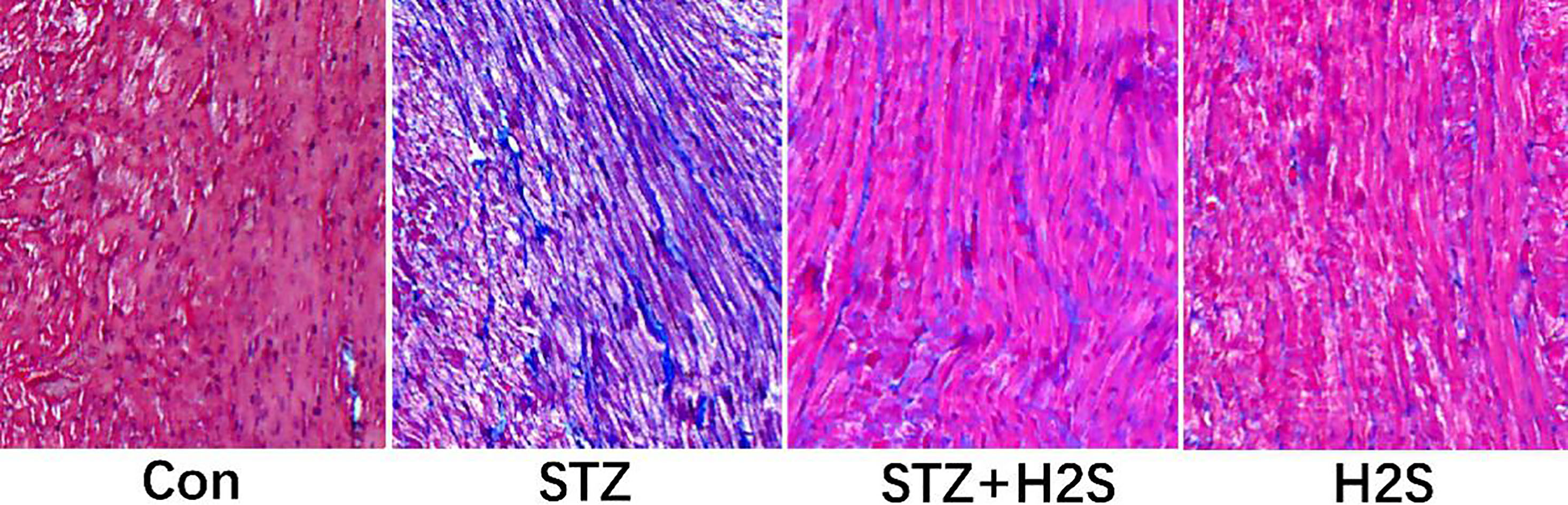
3.2Expressions of collagen-I and collagen-III in myocardial tissues of rats
As shown in Fig. 2, comparing to control group, expressions of collagen-I and collagen-III were clearly augmented (
Figure 2.
Western blotting results of collagen-I and collagen-III. Data are expressed as mean
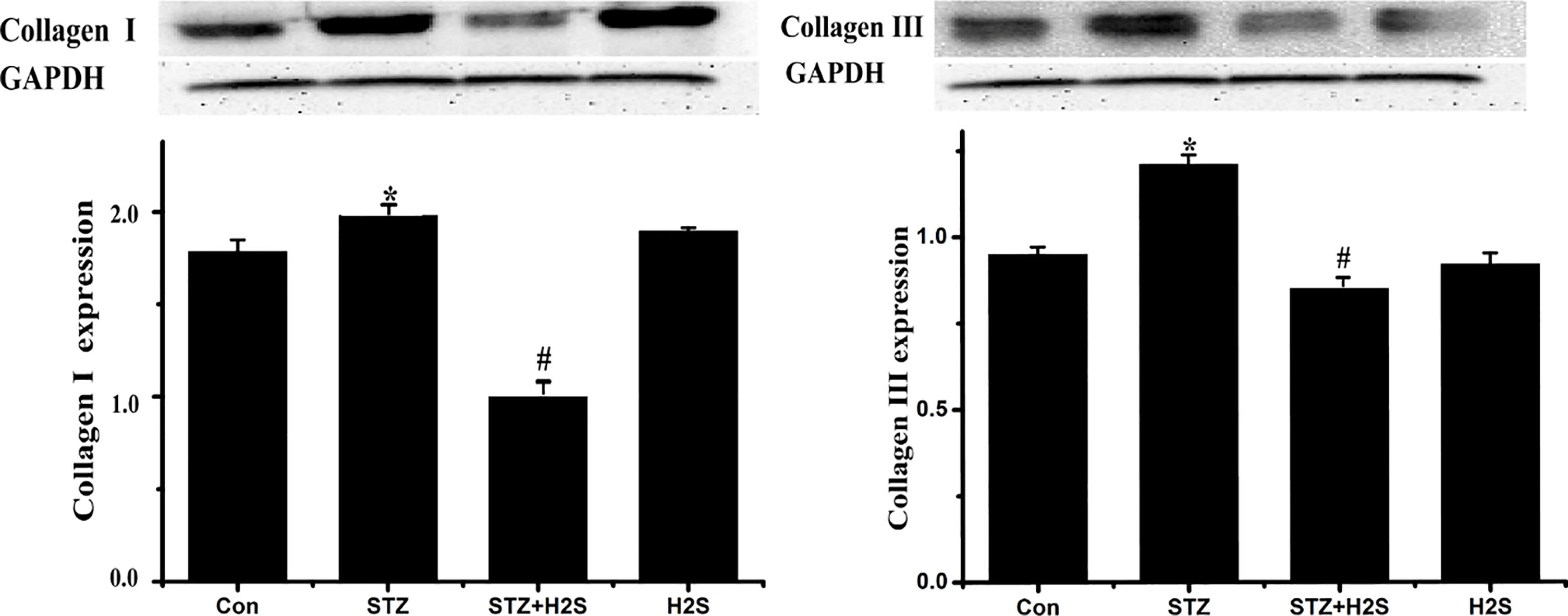
3.3Protein expressions of MMP11, TIMP2 and CTGF in myocardial tissues of rats
As shown in Fig. 3, comparing to control group, protein expressions of MMP11, TIMP2 and CTGF were significantly augmented in STZ group (
Figure 3.
Western blotting results of MMP11, TIMP2 and CTGF. Data are expressed as mean
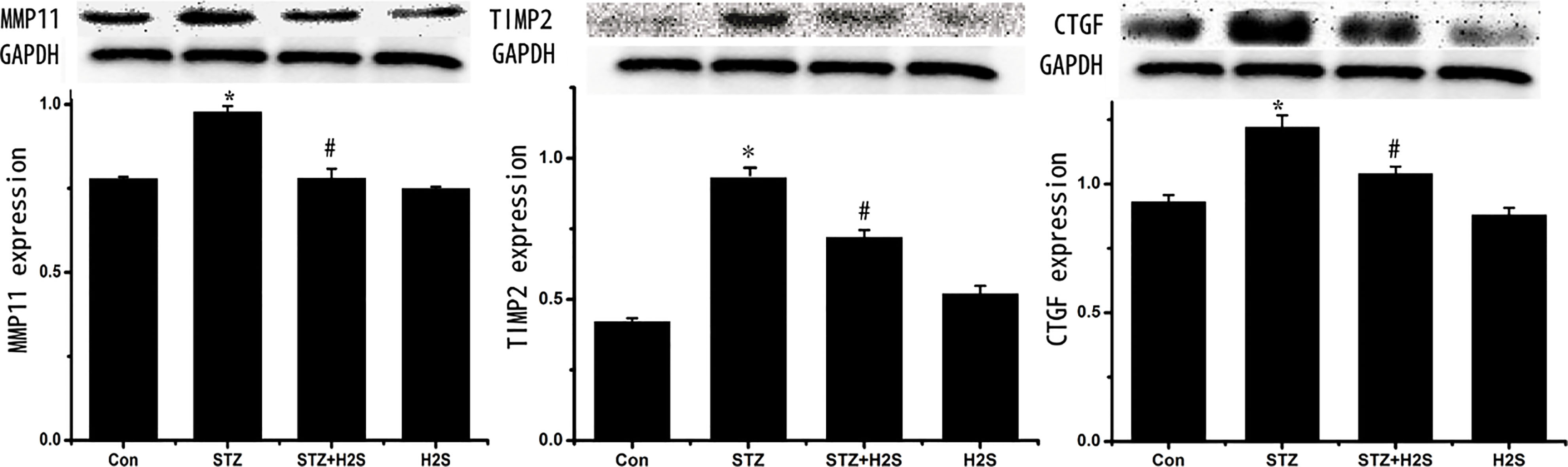
3.4Protein expressions of TGF-β 1, HSP70, HSP90, NF-κ Bp65, TNF-α and PPARG in myocardial tissues
As shown in Fig. 4, expressions of TGF-
Figure 4.
Western blotting results of TGF-
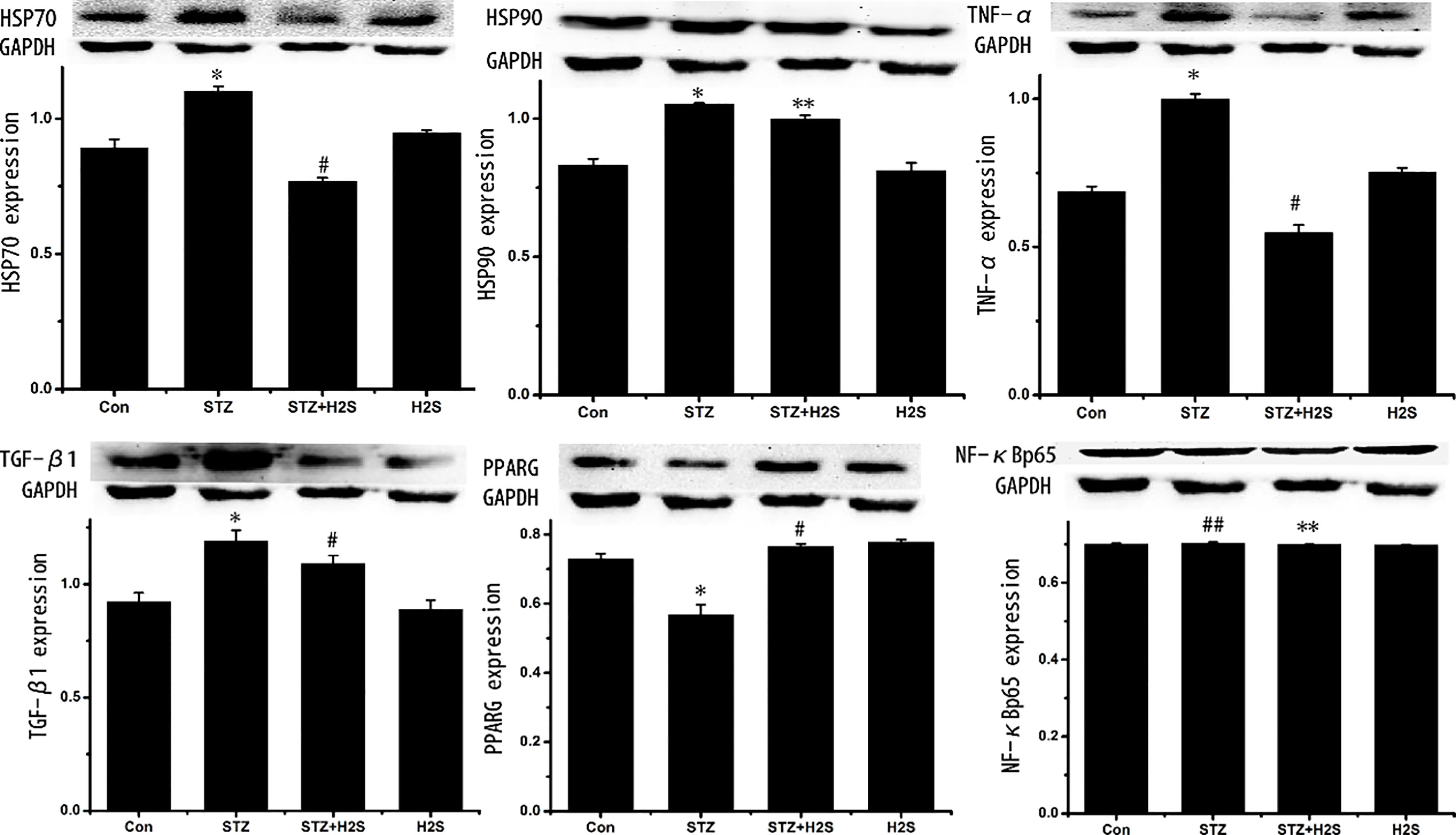
3.5Protein expressions of PKCα and ERK1/2 in myocardial tissues of rats
As shown in Fig. 5, protein expressions of PKC
Figure 5.
Western blotting results of PKC
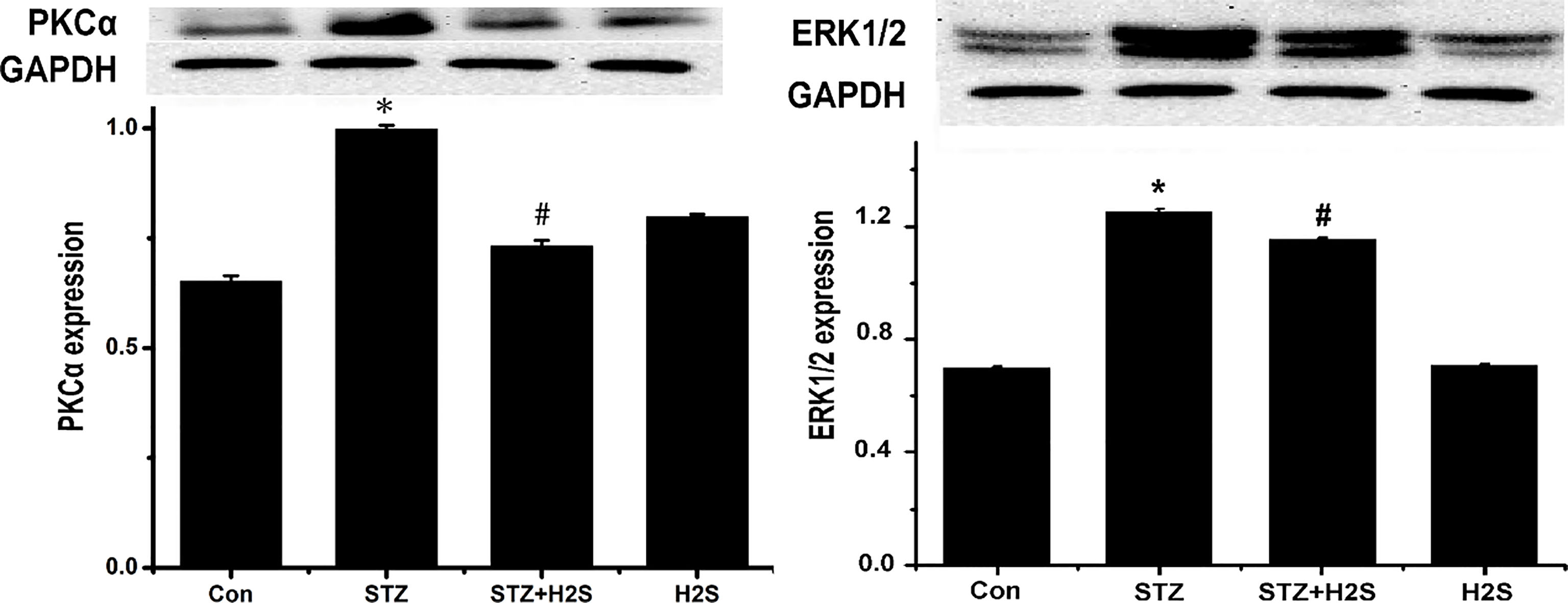
4.Discussion
DCM is a kind of cardiovascular complications of DM, and as a kind specific cardiomyopathy, it usually has the clinical manifestations in early stage, such as left ventricular hypertrophy and decrease of diastolic function [12], and its major pathological changes include hypertrophy of myocardial cells and myocardial fibrosis. However, myocardial fibrosis is generally manifested by the excessive deposition of extracellular matrix (ECM), in which dysregulation of MMPs/TIMPs expressions is closely correlated with the deposition of ECM [13]. MMPs, a kind of Ca
Myocardial fibrosis has a very complicated pathogenesis. According to the studies, inflammatory responses caused by high glucose play an important role in the myocardial fibrosis in DM, and the pro-inflammation factors induced by high glucose are critical to the initiation and progression of myocardial fibrosis [18, 19]. In these factors, TNF-
PKC-ERK1/2MAPK signal pathway is involved in the regulation mechanism of inflammatory responses and collagen remodeling in myocardial tissues in DM. PKC is a kind of phospholipid- and Ca
It has been found that H
Acknowledgments
This study was funded by the Hunan Natural Science Foundation of China (Grant no. 2017JJ3271; 2017JJ3278).
Conflict of interest
None to report.
References
[1] | Aneja A, Tang WH, Bansilal S, et al., Diabetic cardiomyopathy: insights into pathogenesis, diagnostic challenges, and therapeutic options. AM J Med. (2008) ; 121: (9): 748-757. |
[2] | Sakharova OV, Taal MW, Brenner BM. Pathogenesis of diabetic nephropathy: focus on transforming growth factor-beta and connective tissue growth factor. Curr Opin Nephrol Hy. (2001) ; 10: (6): 727-738. |
[3] | Gressner OA, Fang M, Li H, et al., Connective tissue growth factor (CTGF/CCN2) in serum is an indicator of fibrogenic progression and malignant transformation in patients with chronic hepatitis B infection. Clin Chim Acta. (2013) ; 5: (421): 126-131. |
[4] | Ponticos M, Holmes AM, Shi-wen X, et al., Pivotal role of connective tissue growth factor in lung fibrosis: MAPK-dependent transcriptional activation of type I collagen. Semin Arthritis Rheu. (2009) ; 60: (7): 2142-2155. |
[5] | Wang L, Yuan T, Du G, et al., The impact of 1,25-dihydroxyvitamin D3 on the expression of connective tissue growth factor and transforming growth factor-beta1 in the myocardium of rats with diabetes. Diabetes Res Clin Pr. (2014) ; 104: (2): 226-233. |
[6] | Xiao T, Luo J, Wu Z, et al., Effects of hydrogen sulfide on myocardial fibrosis and PI3K/AKT1-regulated autophagy in diabetic rats. Mol Med Rep. (2016) ; 13: : 1765-1773. |
[7] | Corderio MF. Beyond mitomycin: TGF – β and wound healing. Prog Reyin Eye Res. (2002) ; 21: (1): 75-89. |
[8] | Wu D, Peng F, Zhang B, et al., PKC-beta1 mediates glucose-induced Akt activation and TGF-beta1 upregulation in esangial cells. J Am Soc Nephrol. (2009) ; 20: (3): 554-66. |
[9] | Liu X., Gao S, Xu H, et al., lncRNAPCAT29; inhibits pulmonary fibrosis via the TGFβ1regulated RASAL1/ERK1/2 signal pathway. Molecular Medicine Reports. (2018) ; 7781-7788. |
[10] | Li X, Wang G, Qili M, et al., Aspirin Reduces Cardiac Interstitial Fibrosis by Inhibiting Erk1/2-Serpine2 and P-Akt Signalling Pathways. Cellular Physiology & Biochemistry. (2018) ; 45: (5): 1955-1965. |
[11] | Cheng M, Wu G, Song Y, et al. Celastrol-Induced Suppression of the MiR-21/ERK Signalling Pathway Attenuates Cardiac Fibrosis and Dysfunction. Cellular Physiology & Biochemistry International Journal of Experimental Cellular Physiology Biochemistry & Pharmacology. (2016) ; 38: (5): 1928-1938. |
[12] | Niemann M, Herrmann S, Ertl G, et al., Echocardiography in diabetic Cardiomyopathy. Herz. (2013) ; 38: (1): 42-47. |
[13] | Alameddine HS, Morgan JE. Matrix Metalloproteinases and Tissue Inhibitor of Metalloproteinases in Inflammation and Fibrosis of Skeletal Muscles. Journal of Neuromuscular Diseases. (2016) ; 3: (4): 455-473. |
[14] | Lipson KE, Wong C, Teng Y, et al., CTGF is a central mediator of tissue remodeling and fibrosis and its inhibition can reverse the process of fibrosis. Fibrogenesis & Tissue Repair. (2012) ; 5: (Suppl 1): S24. |
[15] | Igarashi A, Okochi H, Bradham DM, et al., Regulation of connective tissue growth factor gene expression in human skin fibroblasts and during wound repair. Mol Biol Cell. (1993) ; 4: (6): 637-645. |
[16] | Huang P, Zhang YJ, Huang Y, et al., Effect of PKC signalling pathway and aldose reductase on expression of fibronectin induced by transforming growth factor-β1 in human mesangial cells. Zhonghua Bing Li Xue Za Zhi. (2010) ; 39: (6): 405-409. |
[17] | Gomes LR, Terra LF, Wailemann RA, et al., TGF-β1 modulates the homeostasis between MMPs and MMP inhibitors through p38 MAPK and ERK1/2 in highly invasive breast cancer cells. BMC Cancer. (2012) ; 12: : 26. |
[18] | Malfitano C, Alba-Loureiro TC, Rodrigues B, et al., Hyperglycaemia protects the heart after myocardial infarction: aspects of programmed cell survival and cell death. Eur J Heart Fail. (2010) ; 12: (7): 659-667. |
[19] | Sheng J, Shim W, Wei H, et al., Hydrogen sulfide suppresses human atrial fibroblast proliferation and transformation to myofibroblastss. J Cell Mol Med. (2013) ; 17: (10): 1345-1354. |
[20] | Yan L, Huang H, Tang QZ, et al., Breviscapine protects against cardiac hypertrophy through blocking PKC-alpha-dependent signaling. J Cell Biochem. (2010) ; 109: : 1158-71. |
[21] | Wang M, Zhang WB, Zhu JH, Fu GS, Zhou BQ. Breviscapine ameliorates hypertrophy of cardiomyocytes induced by high glucose in diabetic rats via the PKC signaling pathway. Acta Pharmacol Sin. (2009) ; 30: : 1081-91. |
[22] | Ryu JM, Lee MY, Yun SP, et al., High glucose regulates cyclin D1/E of human mesenchymal stem cells through TGF-beta1 expression via Ca2+/PKC/MAPKs and PI3K/Akt/mTOR signal pathways. J Cell Physiol. (2010) ; 224: (1): 59-70. |
[23] | Igarashi M, Wakasak H, Takahara N, et al., Glucose or diabetes activates p38MAPK via different pathways J. Chin Invest. (1999) ; 103: (2): 185-195. |
[24] | Kehat I, Molkentin JD. Extracellular Signal-Regulated Kinases 1/2 (ERK1/2) Signaling in Cardiac Hypertrophy. Annals of the New York Academy of Sciences. (2010) ; 96-102. |
[25] | He M, Xue Z, Li J, et al., Breviscapine inhibits high glucose-induced proliferation and migration of cultured vascular smooth muscle cells of rats via suppressing the ERK1/2 MAPK signaling pathway. Acta Pharmacol Sin. (2012) ; 33: (5): 606-614. |
[26] | Panchatcharam M, Miriyala S, Yang F, Leitges M, Chrzanowska-Wodnicka M, Quilliam LA, et al., Enhanced proliferation and migration of vascular smooth muscle cells in response to vascular injury under hyperglycemic conditions is controlled by beta3 integrin signaling. Int J Biochem Cell Biol. (2010) ; 42: : 965-74. |
[27] | Pan Z, Zhao W, Zhang X, Wang B, Wang J, Sun X, et al., Scutellarin alleviates interstitial fibrosis and cardiac dysfunction of infarct rats by inhibiting TGFbeta1 expression and activation of p38-MAPK and ERK1/2. Br J Pharmacol. (2011) ; 162: : 688-700. |
[28] | Xu W, Chen J, Lin J, et al., Exogenous H2S protects H9c2 cardiac cells against high glucose-induced injury and inflammation by inhibiting the activation of the NF-κB and IL-1β pathways. Int J Mol Med. (2015) ; 35: : 177-186. |
[29] | Kondo K, Bhushan S, King AL, et al., H2S Protects Against Pressure Overload Induced Heart Failure via Upregulation of Endothelial Nitric Oxide Synthase (eNOS). Circulation. (2013) ; 127: (10): 1116-1127. |
[30] | El-Seweidy MM, Sadik NA, Shaker OG. Role of sulfurous mineral water and sodium hydrosulfide as potent inhibitors of fibrosis in the heart of diabetic rats. Arch Biochem Biophys. (2011) ; 506: (1): 48-57. |