Investigation of the tolerability and potential health benefits of a novel butyrate generating supplement in a pilot human study
Abstract
BACKGROUND:
Butyrate is a health promoting short-chain fatty acid (SCFA) metabolite of fiber fermentation in the gut. Supplementing directly with a butyrate generator may be a dietary alternative with health benefits.
OBJECTIVE:
To evaluate the effect of tributyrin, a butyrate generator, on tolerability, gut microbiome composition, gut permeability, inflammation and metabolic markers in healthy adults at two dose levels.
METHODS:
Healthy adults (n = 29) were randomized to this single-blinded, two-arm, 28-day parallel design pilot study. Participants ingested one or two placebo capsules for 7 days followed by one or two (200 or 400 mg, respectively) ButyraGen® capsules, a novel tributyrin complex, daily for 21 days. Tolerability was assessed weekly by questionnaire. Blood and stool were collected at baseline and weekly for metabolic and inflammation markers, gut microbiome composition and SCFA concentrations, respectively. Urine was collected at baseline and end of the study for permeability assays.
RESULTS:
Twenty-four participants (n = 24, 25±8 years; 24.0±2.8 kg/m2; 66% male) completed the study. ButyraGen® was well-tolerated, with less than 10% (n = 2) reporting gastrointestinal-related discomfort. Fecal acetic (p = 0.03) and propionic (p = 0.03) acids decreased after supplementation (p = 0.03 and p = 0.03, respectively, n = 24) compared to baseline, and triglycerides increased (p = 0.02, 400 mg only, n = 11). Trends in decreased hs-CRP after 200 mg (p = 0.08) and 400 mg (p = 0.07) supplementation and decreased glucose (p = 0.10) after 200 mg supplementation was observed. No other changes in endpoints were observed.
CONCLUSIONS:
Tributyrin supplementation using ButyraGen® was safe and tolerable at the doses provided. Biological effects were observed suggesting butyrate generation and absorption in the small intestine followed by activity in the liver, though further investigation on mechanism of action is needed for confirmation.
Introduction
Dietary fibers are an important component of the diet associated with lowering risks of chronic diseases and promoting bowel health [1, 2]. The health benefits of fiber are supported by several different hypotheses. Bowel regularity and fibers’ effect on gut microbiota composition has been discussed thoroughly [3]. Certain dietary fibers serve as important substrates for gut microbes producing short-chain fatty acids (SCFAs), metabolites with varied physiological effects [4]. Butyrate is one of three major SCFAs that has received attention for its benefits on intestinal health and homeostasis, as it serves as a major fuel source for colonocytes [5, 6]. Likewise, several in vitro and in vivo studies suggest that butyrate modulates immune-inflammatory responses and intestinal barrier function [6].
Fermentation of dietary fibers by the gut microbiota provides the largest source of butyrate to humans and intestinal cells [7]. However, only 10% of women and 3% of men meet the recommended intake for dietary fiber, a statistic that has hardly changed in over 40 years [8, 9]. Considering this issue, alternative strategies have been sought to deliver fiber, and specifically microbial associated benefits, including supplementing with butyrate directly. Both rectal and oral methods of butyrate delivery have been investigated in animal and human models. Rectal enemas are a promising method of direct butyrate delivery to the distal colon, particularly in patients experiencing colonic inflammation [10]; however, this delivery method is intrusive and is accompanied by low compliance outside the clinical setting [11]. Oral administration of butyrate and/or butyrate-producing substrates, such as sodium-butyrate and tributyrin, have also been explored, potentially providing butyrate exposure more broadly in the gastrointestinal tract [12–14]. Sodium-butyrate is a butyrate salt that is readily available for absorption from the stomach. Aside from limited exposure to the intestine, tolerability is a major challenge due to its astringency, putrid smell and taste that lingers after intake [15]. In contrast, tributyrin is a short-chain triglyceride consisting of a glycerol backbone esterified to three butyrate molecules. When ingested, butyrate is liberated from the glycerol backbone via the action of pancreatic lipase, releasing free butyrate and butyrate monoglycerides available for absorption all along the small intestine and possibly proximal colon [16]. Pharmacokinetic and toxico-kinetic studies have shown that oral intake of tributyrin can sustain plasma butyrate concentrations equivalent to sodium-butyrate, indicating its promise as an oral supplement [17]. However, limited clinical studies are available on the tolerability of tributyrin and its potential health effects. Moreover, the available research is focused on pharmacological doses for therapeutic purposes vs dietary supplement levels to maintainhealth.
Therefore, the purpose of this pilot study was to evaluate oral consumption of a tributyrin-containing supplement on tolerability in a healthy population and to explore possible biological effects consistent with health. ButyraGen®, a propriety tributyrin complex, was used for supplementation. Assessments of this product focused on 1) tolerability 2) changes in gut microbiome composition and short-chain fatty acids 3) metabolic and inflammation markers and 4) markers of gut permeability.
Materials and methods
Study design and participants
This study was approved by the Institutional Review Board of Illinois Institute of Technology, Chicago, Illinois (Protocol #IRB-2023-25) and registered with ClinicalTrials.gov (NCT05601635). All study participants were provided a written informed consent before any study procedures were initiated. Healthy men and women were recruited from the greater Chicagoland area. Participants were required to meet general eligibility criteria (Supplemental Table 1). Participants with diagnosed or self-reported gastrointestinal disease or discomfort were excluded from this study.
This pilot study was a 28-day randomized, single-blinded, parallel design with two doses of the ButyraGen® supplement (Fig. 1). Study size was determined based on previous exploratory pilot studies, not based on statistical power analysis. Participants were randomly assigned to either the high dose (2 capsules, containing a total of 200 mg tributyrin) or low dose (1 capsule, containing a total of 100 mg tributyrin) regimen. Participants were blinded to a 7-day (Day -7 to Day 0) placebo lead-in followed by a 21-day (Day 0 to Day 21) active phase. Participants received the same number of capsules (one or two) throughout both phases. During the 28-day study period, participants came to the Center for Nutrition Research (CNR) at Illinois Tech on a weekly basis, with an additional mid-week visit (Day 3) during the first week of the active phase. At each study visit, participants provided self-collected fecal and urine samples. A fasting blood sample was collected at the CNR at each visit. At the beginning (Day -7) and end (Day 21) of the 28-day study period, participants also collected urine samples for a Lactulose-Mannitol Test (LMT).
Fig. 1
Study design schema. Randomized, 28-day, 2-arm (200 mg vs 400 mg) parallel design study with a 7-day placebo wash-in and 21-day active phase.
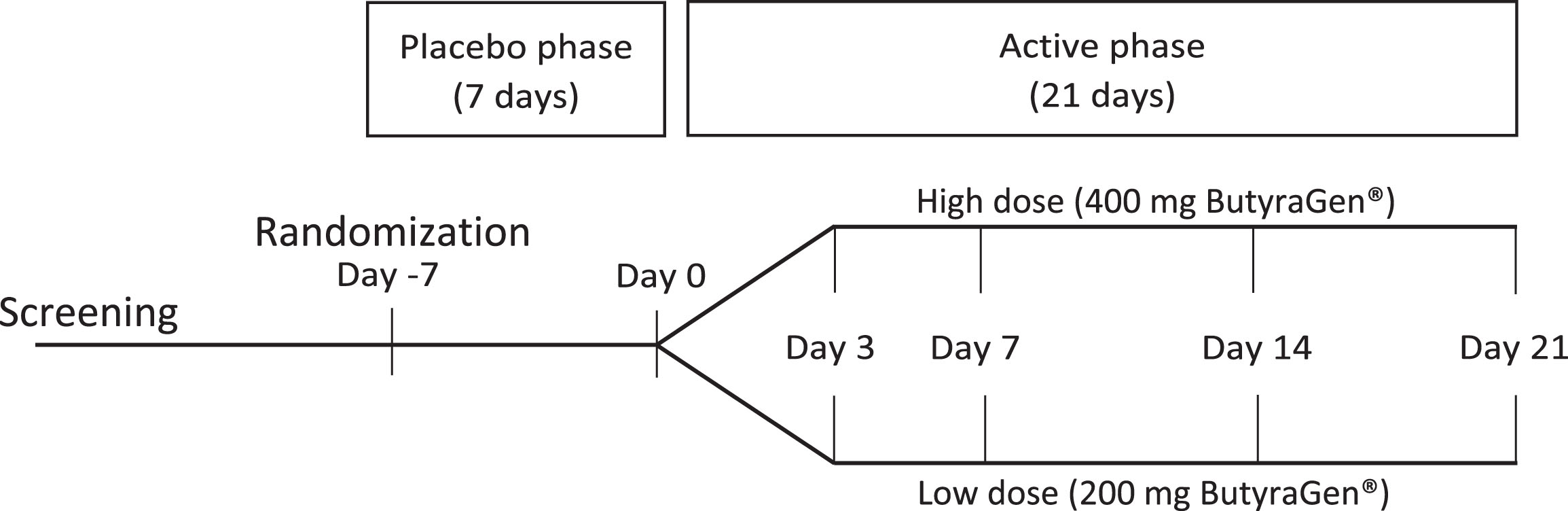
Tributyrin supplementation
ButyraGen® was the source of tributyrin for this study. ButyraGen® is a novel tributyrin complex that generates butyrate through hydrolysis of tributyrin in the small intestine. Active capsules contained 98.5–99.5% of the ButyraGen® complex powder (NutriScience Innovations, Milford, CT, USA) and 0.5–1.5% silicone dioxide. The ButyraGen® complex itself contained 50% tributyrin (primary active ingredient), acacia gum, partially hydrolyzed guar gum (Sunfiber®), rosemary extract and microcrystalline cellulose. Each capsule contained 200 mg ButyraGen®, which delivers 100 mg tributyrin. Participants randomized to the 100 mg tributyrin dose ingested 1 capsule/day and those randomized to the 200 mg tributyrin dose ingested 2 capsules/day. Placebo capsules contained equal amounts of acacia fiber, partially hydrolyzed guar gum, rosemary extract and microcrystalline cellulose as the active capsules. Maltodextrin was used to maintain capsule volume and replace the tributyrin ingredient in placebo capsules.
Study procedures
Prior to starting the 28-day trial period, eligible study participants were counseled to maintain usual dietary and physical activity patterns. Participants were also instructed on fecal and urine sample collection procedures. Participants arrived at the CNR in a fasted state (10–12 h, confirmed by finger stick) the morning of each study visit, with collected fecal and urine samples. Participant readiness was assessed by adherence to protocol compliance via dietary records and questionnaires. Anthropometrics, body composition, and vital signs were measured. Fasted blood samples were collected.
Study days that included LMT (Day -7, 21) were conducted at the CNR via instructions provided in the Genova Diagnostics Leaky Gut Test Kit. Briefly, at each LMT, participants consumed a beverage containing two sugars – lactulose and mannitol – and then collected urine specimens over the following 6 hours.
GI tract and tolerability questionnaire
The GI Tract Questionnaire was administered to participants at each study day visit. Questions were related to their overall gut health as well as tolerability of the supplement, including questions about nausea, bloating, gas/flatulence, bowel consistency, abdominal pain, and off tastes. Questions were phrased for “Yes/No” answers. If the participant answered “Yes” they categorized how often they experienced the related symptom. Options were as follows: “Much more than usual”, “Somewhat more than usual”, “Usual”, “Somewhat less than usual” and “Much less than usual”. Comment boxes were also available for participants to provide additional information.
Fecal sample collection, microbiota characterization and SCFA analysis
Participants were provided with sterilized receptacles and an insulated cooler bag for both at-home fecal and urine sample collection. Collected fecal samples were immediately stored in the insulated cooler bag with ice packs. Participants were allowed to collect fecal samples within a 3-day period leading up to each study visit, though the sample was required to be provided to the CNR within 24 hours of collection for processing. Fecal samples were aliquoted and stored at -80°C for end-of-study analysis.
DNA was extracted from stored fecal sample aliquots via Chemagic DNA Stool 360 kit. Raw data was checked using FastQC, followed by quality filtering and trimming using the algorithm bbduk. Short read taxonomic annotation was performed using the software package MetaPhlAn3(v4.0.1) and functional gene annotation with HUMAnN3(v3.5) mapping to the UniRef90 catalog (UniRefrelease 2019_01). For downstream analysis, uniRef90 relative abundance tables were regrouped into the following higher-level organizations: MetaCyc pathways, KEGG orthology and UniProt gene families.
Sample preparation and quantification methods for SCFAs analysis in fecal samples were adapted from our previously reported method [18]. In short, fecal samples were freeze-dried for 24 hours, rehydrated with 5 ml of water, centrifuged, acidified and centrifuged again. The resulting supernatant was transferred to a gas chromatography (GC) vial for direct injection. Analysis of SCFAs was performed on an Agilent 7890A GC coupled with flame ionization detection (FID) with an Agilent J&W GC Column DB-FFAP (30 m × 0.25 mm × 0.5um). Agilent OpenLab ChemStation software was used for data collection. Details of the GC-FID method are previously described [18].
Chemicals and reagents for SCFA analysis
Analytical grade standards for SCFA analysis (acetic, propionic, iso-butyric, butyric, iso-valeric, valeric, and heptanoic acids) were purchased from Millipore Sigma (St. Louis, MO, USA). Other SCFA analytical grade standards (4-methyl valeric and hexanoic acid) were purchased from Fisher Scientific (Hampton, NH, USA) and internal standard (IS) 2-ethylbutyric acid was purchased from TCI America (Portland, OR, USA). MilliQ water was obtained from Direct-Q Water Purification System (18.2 MΩ-cm at 25°C, Millipore Sigma, St. Louis, MO, USA).
Blood sample collection, metabolic and inflammatory marker analysis
Fasting blood samples were collected at each study visit at the CNR. Vacutainers coated with ethylenediaminetetraacetic acid (EDTA) were placed on ice, centrifuged for 15 minutes (4°C, 453 × g) and aliquoted for plasma. Serum Separation Tubes (SST) were used to collect serum samples. SST vacutainers were allowed to clot upright at room temperature for 30 minutes before being centrifuged for 15 minutes (4°C, 453 × g) and aliquoted. Plasma and serum samples were stored at –80°C until analysis at the end of the study.
Serum glucose, insulin, triglycerides and plasma high-sensitivity c-reactive protein (hs-CRP) were assessed using Randox Daytona Automated Clinical Analyzer (Randox) with appropriate standards and controls. Tumor necrosis factor alpha (TNF-α) and interferon-gamma (IFN-γ) were measured in plasma using Quantikine high sensitivity ELISA assay methods (cat. HS TA00e and DIF0, respectively; R&D Systems, Minneapolis, MN, USA). Zonulin was measured in plasma using an ELISA assay method (cat. EKC36091; Biomatik, Kitchener, ON, CAN). Lipopolysaccharide (LPS)-binding protein (LBP) was measured in plasma using an ancillary ELISA assay method (cat. DY008B; R&D Systems, Minneapolis, MN, USA).
Urine sample collection and lactulose mannitol test
First-morning urine samples were collected on the morning of each study visit. Collected samples were stored in the insulated cooler bag with ice packs until provided to CNR staff. On study days that included LMT (Day -7, 21), first-morning urine samples were first aliquoted and stored via instructions provided by the Genova Diagnostics Leaky Gut Test Kit. The remaining and first-morning urine samples collected on all other study days (Day 0, 3, 7, 14) were aliquoted and stored at –80°C for end-of-study analysis.
Urine specimens for LMT study days (Day -7, 21) were collected over a 6-hour period after consuming the lactulose-mannitol beverage. All urinations during that time frame were collected in a sterilized receptacle and then added to a larger urine collection jug where all collected urine samples were consolidated. The larger urine collection jug was stored at refrigerated temperatures until the test was complete. After the final urine sample was collected, total urine volume was recorded and a homogenized urine sample was aliquoted. Collected urine samples from LMT study days were shipped to Genova Diagnostics, USA for analysis.
Statistical analysis
Statistical analysis was performed on PC-SAS 9.4 (SAS Institute). Normality distributions were analyzed and determined by the Shapiro-Wilk test (p > 0.05). Analytes that did not conform to normal distributions were log10-transformed prior to statistical analysis. Paired t-test analysis was used to determine differences between Day -7 and Day 0 to assess the effect of the placebo phase. No statistical difference was found between Day -7 and Day 0 for any analyte; therefore, Day -7 was used as the baseline. Paired t-test analysis was also used to determine significant changes in fecal SCFA content, metabolic markers (glucose, insulin and triglycerides), inflammation markers (hs-CRP TNF-α, IFN-γ, zonulin and LBP) and LMT results between Day -7 and Day 21. Statistical significance was determined based on a 2-sided comparison at the 5% significance level (p < 0.05) for assessing differences in baseline measures and all analytes. Trends were identified between 0.05≤p≤0.10. When appropriate, combined group (n = 24) analysis was used to increase statistical power.
Statistical analysis of relative abundances at the phyla level of taxonomic classification was completed with data tables as annotated by MetaPhlAn3(v4.0.1) and exported to Excel as a spreadsheet. In Excel, the Student’s t-test was used to compare relative abundance values, with significance defined as p < 0.05. Raw counts were normalized to percentages for relative abundance based on total abundance per sample. Analysis of alpha- and beta-diversity, as well as differential microbial feature analyses, correlation analyses and feature selection, were performed within the R programming environment, as described previously [19].
Results
Participant demographics and characteristics
A total of 33 participants were screened, of which 29 passed the initial screening and were enrolled in the study (Fig. 2). Five dropped out at various stages, and twenty-four participants (low-dose group, n = 11; high-dose group, n = 13) completed the study. All 24 participants were considered the evaluable data set for data analysis. Baseline demographic information is available in Table 1. In general, this was a young and healthy group without pre-existing digestive issues.
Fig. 2
CONSORT flow diagram. Evaluable data set (n = 24) following completion of the study.
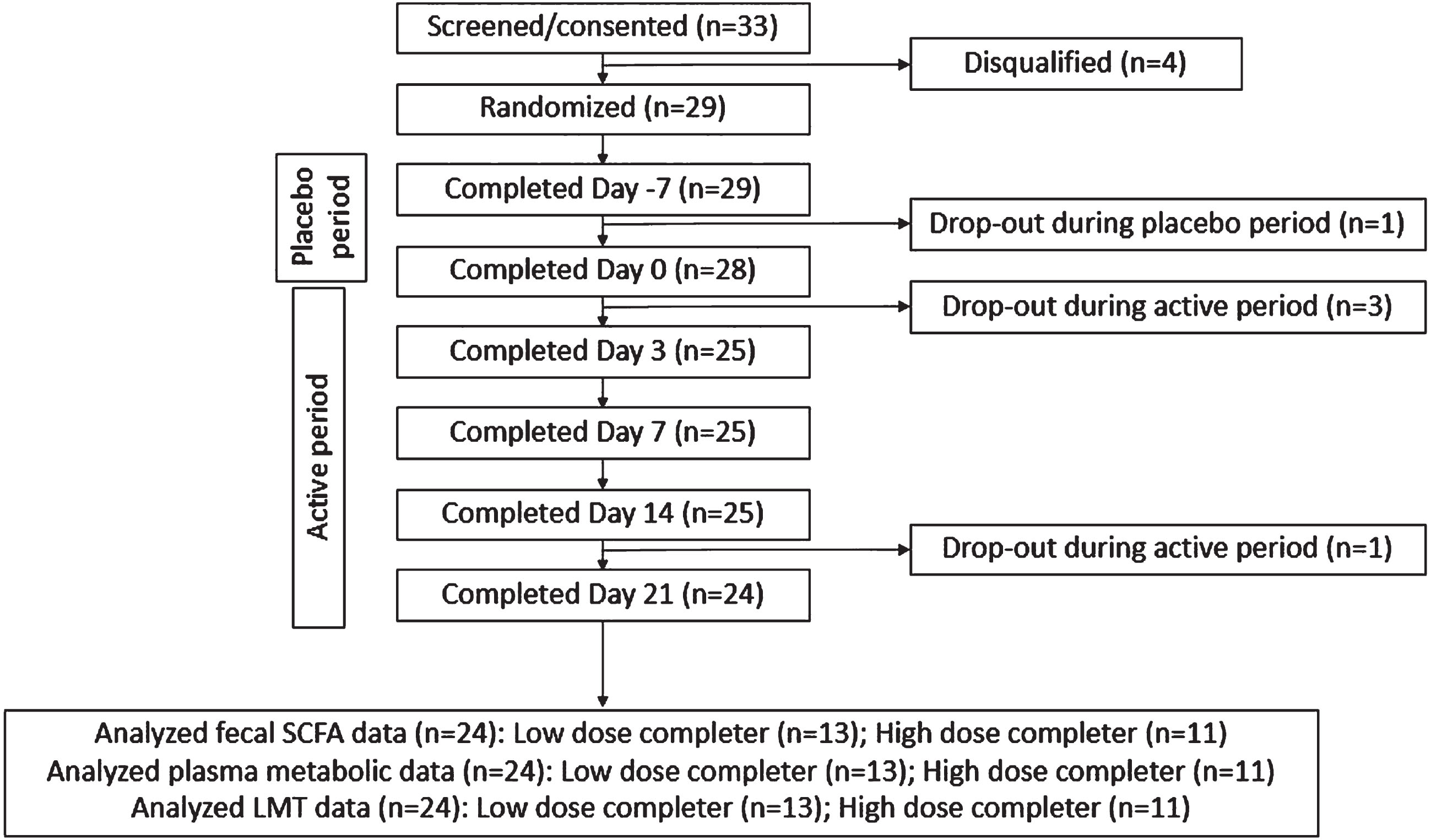
Table 1
Baseline demographics of study participants
Variable | Low dose | High dose | Combined group |
(n = 13) | (n = 11) | (n = 24) | |
Age (year)1 | 24±5 | 29±9 | 25±8 |
BMI (kg/m2)1 | 23.2±2.9 | 24.8±2.6 | 24.0±2.8 |
Female: Male (n) | 3 : 10 | 5 : 6 | 8 : 16 |
Race (Asian:Cau:His) | 9 : 4 : 0 | 5 : 5 : 1 | 14 : 9 : 1 |
1Values are unadjusted means±SD. n-number of participants; BMI-body mass index; His-Hispanic; Cau-Caucasian.
GI tract questionnaire and supplement tolerability
Overall, the ButyraGen® supplement was well tolerated. Minimal disruption to normal gut activity was observed over the course of the study (Table 2). One participant (n = 1) of the 24 reported “Somewhat Unpleasant” lingering aftertastes throughout the course of the study. An additional participant (n = 2) also reported “Somewhat unpleasant” on Day 14, but did not report unpleasantness prior to or at subsequent visits (Table 2). Questionnaire results also indicated minimal disruption to normal gut activity. Few occurrences of diarrhea, constipation, or lower abdomen discomfort were reported. One participant’s baseline questionnaire was collected during their menstrual cycle, in which they reported “Much more than usual” abdominal pain and lower abdominal pain. Another participant experienced self-reported food poisoning around Day 7 of the study. The onset of the food poisoning contributed to “Much more than usual” occurrences of nausea, gastrointestinal rumblings, abdominal pain, diarrhea, stomach pain/aching and lower abdomen discomfort. On both occasions, these reports were not related to the study product.
Table 2
GI Tract questionnaire and supplement tolerability. Number of participants (n) experiencing symptoms more than usual
Study time point | ||||||
Placebo phase | Active phase | |||||
Based on the previous 7 days | Day -7 | Day 0 | Day 3 | Day 7 | Day 14 | Day 21 |
(n = 22) | (n = 21) | (n = 23) | (n = 23) | (n = 24) | (n = 21) | |
Nausea, (n) | 1b | |||||
Bloating, (n) | 1 | 2 | 1 | 2 | 1 | |
Gastrointestinal rumblings, (n) | 2 | 1 | 3b | 1 | 2 | |
Gas/flatulence, (n) | 1 | 2 | 3 | 2 | 4 | 2 |
Abdominal pain, (n) | 1a | 1b | 1 | |||
Diarrhea, (n) | 1 | 1 | 2b | 1 | 1 | |
Constipation, (n) | 1 | |||||
Indigestion, (n) | 2 | 2 | 1 | 1 | ||
Stomach pain/aching, (n) | 1 | 1 | 1b | 1 | ||
Discomfort in lower abdomen, (n) | 1a | 1 | 1 | 2b | 2 | 2 |
Lingering taste after consuming the capsule(s), (n) | 1 | 1 | 1 | 2 | 1 |
aOne participant reported menstrual cycle. bOne participant reported food poisoning.
One participant reported “Somewhat more than usual” in categories of diarrhea, bloating, gastrointestinal rumblings, gas/flatulence and lower abdomen discomfort from Day 0 to Day 21. Symptoms of bloating, gastrointestinal rumblings and gas/flatulence escalated to “Much more than usual” at Day 14, but symptoms deescalated to “Somewhat more than usual” by the following week.
Fecal SCFAs
A total of nine SCFAs (acetic, propionic, iso-butyric, butyric, iso-valeric, valeric, 4-methyl valeric, hexanoic and heptanoic acids) were identified and quantified in freeze-dried fecal samples. Acetic, propionic and butyric acids were used to compare the intervention effects due to the low abundance of all other SCFAs.
All three fecal SCFAs showed decreasing trends from baseline (Day -7) to the end of the study (Day 21) in the whole population as well as within intervention groups. A significant decrease from baseline in acetic acid (p = 0.03) and propionic acid (p = 0.03) was observed in the whole population (Fig. 3).
Fig. 3
Fecal short-chain fatty acids (SCFA). Comparison of fecal SCFAs between baseline (Day -7) and Day 21 in whole population (n = 24). Data were analyzed by paired t-test. Values are unadjusted means±SEM. *p < 0.05.
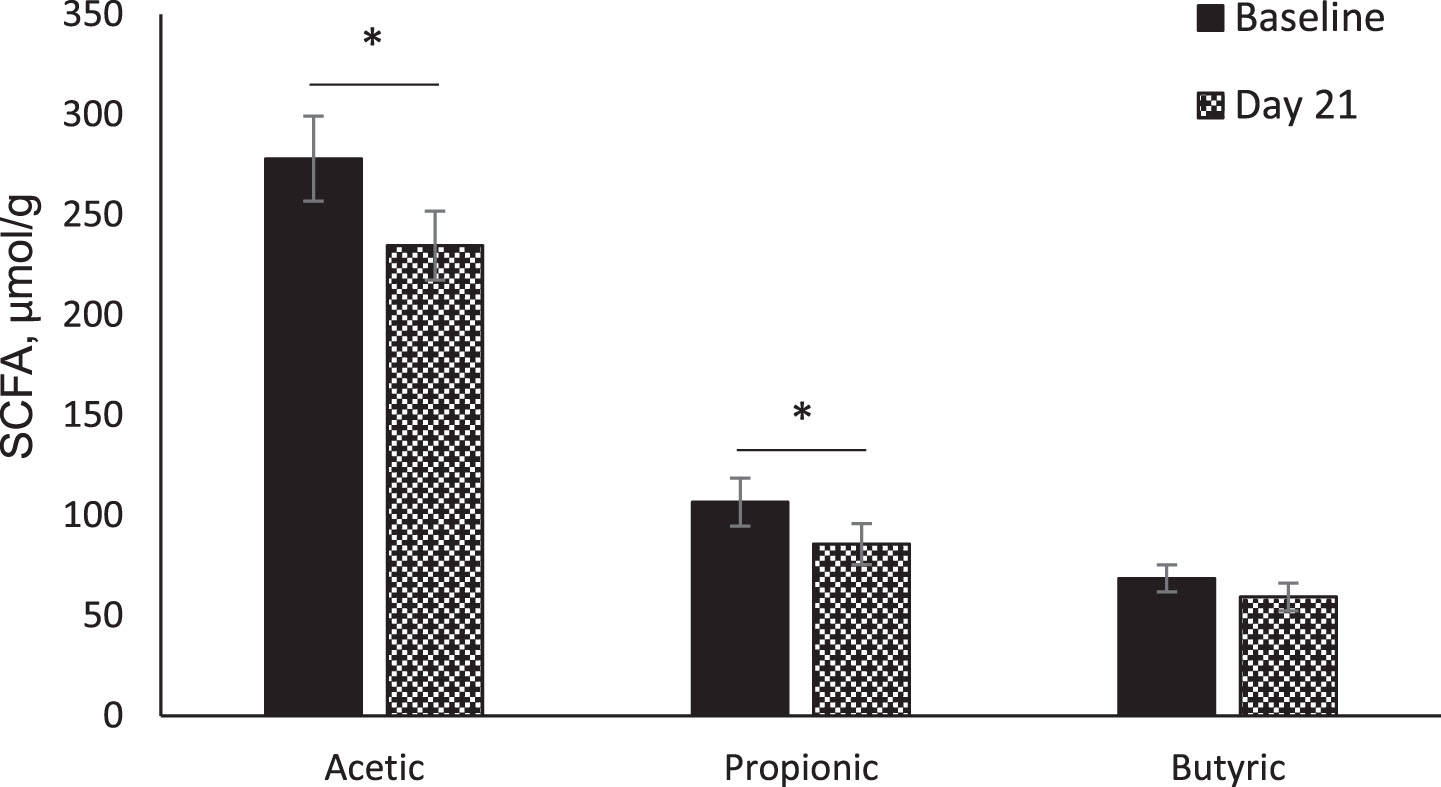
Gut microbiome composition
Within sample (α-diversity) and between samples (β-diversity) diversity was not significantly changed with ButyraGen® supplementation at either dosage comparing baseline (Day -7) to Day 21. At the phyla level, abundances of Firmicutes and Bacteroidetes or their ratio (F/B) also did not significantly change with supplementation (Table 3). Predicted pathway analysis from microbial DNA was also not significantly changed (q > 0.05, Supplemental Table 2). Other taxonomic analyses of specific genera, species, etc. did not yield any statistical effects related to the study (data not shown).
Table 3
Phyla analysis and Firmicutes/Bacteroides (F/B) ratio of combined group (n= 24)
Variable | Baseline1 | Day 211 | p-value2 |
Bacteroides | 0.294 | 0.274 | 0.67 |
Firmicutes | 0.535 | 0.590 | 0.23 |
F/B Ratio | 3.204 | 6.947 | 0.23 |
1Average relative abundance. 2Student’s t-test.
Metabolic markers - glucose, insulin and triglycerides
Fasting glucose and insulin were not different between supplement dosage groups nor in the population as a whole from baseline (Day -7) to the end of the study (Day 21) (Fig. 4a, b). Serum glucose concentrations showed decreasing trends from baseline to the end of the study in the low-dose group (p = 0.10, Fig. 4a). The high-dose group showed the opposite response with an increase in glucose from baseline to the end of the study, but it was not significant. Fasting serum triglyceride concentrations at baseline (Day -7) and end of the study (Day 21) for combined groups (n = 24) and the low-dose (n = 13) and high-dose (n = 11) groups are shown in Fig. 4c. A significant increase in fasting triglycerides was observed in the high-dose group (p = 0.02), but not in the low dose group (p > 0.05).
Fig. 4
Metabolic markers. Comparison of metabolic markers between baseline (Day -7) and Day 21 in whole population, low dose (n = 13) and high dose (n = 11) groups. Data for each analyte were analyzed by paired t-test. Values are unadjusted means±SEM. (a) glucose (b) insulin (c) triglycerides (TG). *p < 0.05; #p < 0.10.
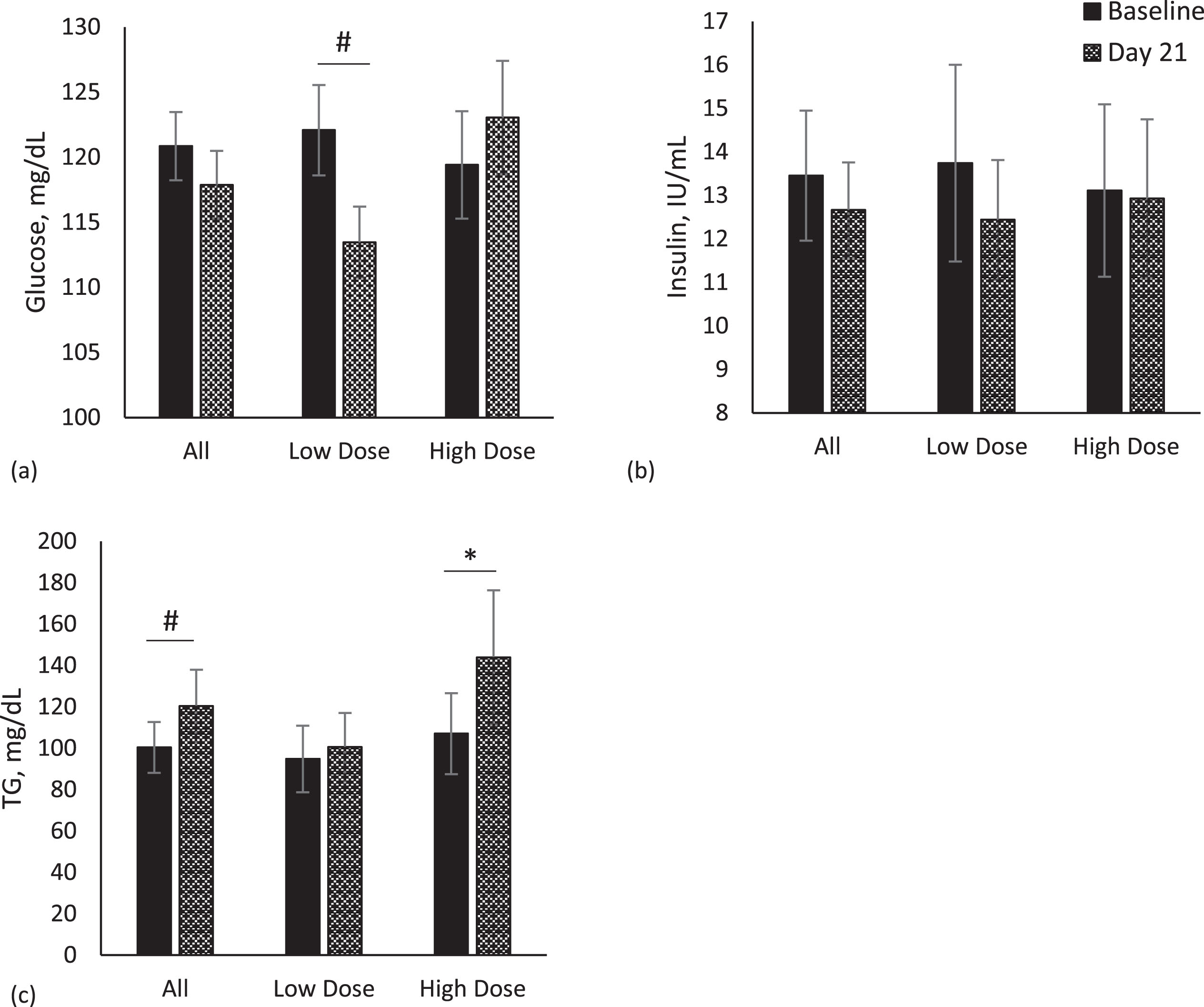
Inflammation markers- hs-CRP, TNF-α, IFN-γ, Zonulin and LBP
A marginally significant reduction in hs-CRP concentration was observed in both the low-dose (p = 0.08) and high-dose (p = 0.07) groups (Fig. 5a). No significant changes were observed for IFN-γ (Fig. 5b), TNF-α (data not shown), zonulin (data not shown) and LBP (data not shown) in either group or the population as a whole.
Fig. 5
Inflammation markers. Comparisons of inflammation markers between baseline (Day -7) and Day 21 in whole population, low dose (n = 13) and high dose (n = 11) groups. Data for each analyte were analyzed by paired t-test. Values are unadjusted means±SEM. (a) High sensitivity C-reactive protein (hs-CRP) (b) interferon gamma (IFN-γ). #p < 0.10.
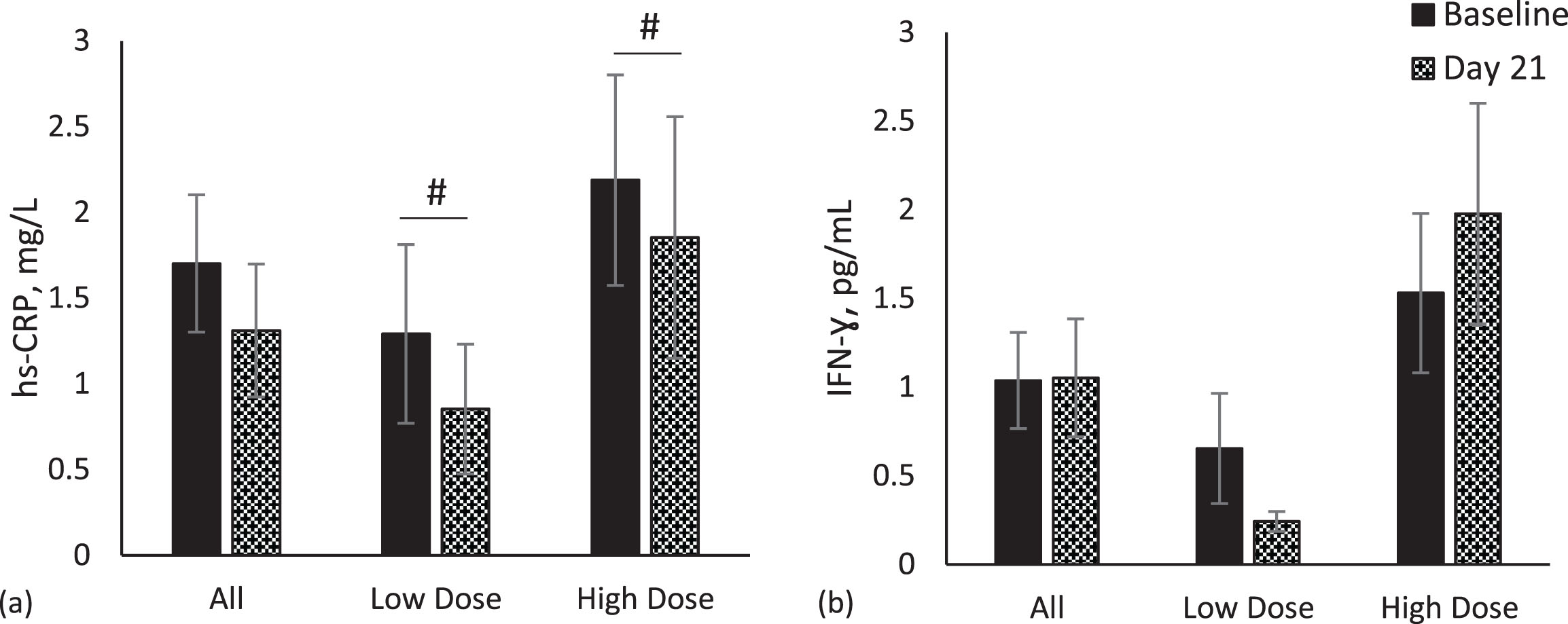
LMT
Data related to the LMT were evaluated and reported by Genova Diagnostics, Inc. Reported intestinal permeability data included lactulose percent recovery, mannitol percent recovery and lactulose to mannitol ratio. No significant differences in any of the intestinal permeability data categories were observed from baseline to the end of the study in either intervention group or the whole population.
Discussion
To our knowledge, this study reports for the first time the effects of oral tributyrin supplementation in free-living, healthy adults in a randomized controlled study. Prior research included one non-randomized/non-controlled study in three people and two other clinical trials investigated tributyrin supplementation in people with solid tumors at high pharmacological doses [16, 20, 21]. All other known tributyrin supplement studies have been conducted in animal models [17, 22–27]. In the present study, ButyraGen® was used as the source of tributyrin. The main findings of the present study were: 1) the supplement was well tolerated, 2) fecal acetic and propionic acids were decreased in the combined dose group analysis, 3) gut microbiota composition was unchanged, 4) serum triglycerides concentrations were increased after supplementation with the high dose, 5) hs-CRP, a marker of inflammation was moderately reduced in both dosage groups, and 6) glucose was moderately reduced in the low dose group.
Questionnaire-based results indicated ButyraGen® was well tolerated by all individuals and no adverse effects related to the supplement were observed. Previous tributyrin supplementation trials in humans by Conley et al. and Edelman et al. also concluded tributyrin is generally tolerable, though both studies were conducted at considerably higher dosage levels than the current study. In the study by Conley et al., an adverse event was reported at a dose of 50 mg/kg, which may not be related to the intervention, according to the investigators [16]. Regardless, a 50 mg/kg dose corresponds to 3,500 mg of tributyrin for a 70 kg individual, which is 17.5 times the highest dose in the current study. Edelman et al. reported no adverse events at 150 mg/kg dose, 3 times daily, corresponding to 31,500 mg of tributyrin for a 70 kg individual and more than 150 times the dose in the current study [20]. Outside of one participant who consistently experienced gastrointestinal-related symptoms (diarrhea, bloating, gastrointestinal rumblings, gas/flatulence, indigestion and lower abdomen) throughout the present study, there were either sporadic or no reports of changes by participants. Evaluations of taste also indicated general acceptability.
Gut microbiome analysis was conducted to determine if tributyrin supplementation in a healthy population would alter gut microbiota composition, particularly those bacteria associated with butyrate generation. Well-known butyrate-producing bacteria in the large intestine include Faecalibacterium prausnitzii and Eubacterium rectale/Roseburia spp. [11, 28]. The results of the current research showed no significant shifts in gut microbial composition, including those related to butyrate production. The results may not be unexpected given that most butyrate exposure from ingestion of tributyrin occurs in the small intestine as a result of hydrolysis by lipases, and limited butyrate likely reaches the proximal colon after oral supplementation. However, even with limited accessibility to the colon, no adverse suppressions in (endogenous) butyrate producing bacteria were evident, and the predicted metabolic pathway analysis of DNA extracted from fecal samples suggests possible bioactivity in select pathways. These include pathways related to fatty acid biosynthesis and oxidation and pyruvate fermentation (Supplemental Table 2). While these trends may represent possible butyrate supplementation effects, metatranscriptomic analysis is necessary for confirmation and was outside the scope of this study.
While there were no apparent changes in fecal butyrate, decreases in fecal acetic and propionic acid content was observed in the combined group (n = 24) after ButyraGen® supplementation. A 4-week study by Cleophas et al. investigating sodium-butyrate supplementation in lean (22.0±2.3 kg/m2, n = 9) and obese (33.2±3.6 kg/m2, n = 10) males reported similar results related to fecal SCFA content. In both groups, butyrate supplementation significantly decreased fecal acetic and propionic acid concentrations. However, fecal butyric acid was not significantly changed in the lean group, but was significantly lower in the obese group after supplementation [29]. In the current study, BMI for the combined group (n = 24) was 24.0±2.8 kg/m2. The findings of this study are concurrent with that of the work by Cleophas et al. in lean participants. The rationale for these findings, including our own, is not clear. As butyrate is the main energy source for colonocytes, its availability for measurement in stool samples may be affected by gut transit time, need by colonocytes, microbial cross-feeding and individual diet, which may vary between lean and obese individuals, adding variance and making interpretation difficult within the scope of the present study. The lack of detected changes in fecal butyrate in this study could have been due to any one of these reasons, as well as, absorption in the proximal intestine. Blood sample analysis for butyrate was explored using the GC-FID method. However, this method was not sensitive enough to detect meaningful butyrate changes in the blood. Extraction of butyrate by the intestine and the liver is significant, limiting detection and quantification in the blood [30]. Further investigation and sensitive methods for low SCFA blood analysis are necessary to understand butyrate metabolism. Changes in fecal acetic and propionic acid content may be induced by tributyrin supplementation, but further analysis is also needed to understand the mechanisms contributing to these results.
Butyrate modulates the epithelial barrier by regulating the tight junction proteins in the intestine, but little evidence supports changes specifically in the small intestine where the action of tributyrin likely occurs [7, 27]. The LMT by Genova Diagnostics, USA evaluates non-specific intestinal permeability by measures of transcellular and paracellular permeability and compares it against a reference group. Baseline assessments of gut permeability markers via LMT indicated the study population had a generally healthy gut based on this assessment tool. No significant supplementation-related effects were observed with the intervention concurrent with other findings related to the gut in this study. Future studies in individuals with compromised intestinal integrity are warranted to understand the impact of butyrate supplementation on intestinal permeability.
Several markers of inflammation were based on the hypothesis that butyrate has anti-inflammatory properties [6, 7, 14, 25, 26]. TNF-α, IFN-γ, zonulin and LBP are known to be associated with gut inflammation. Despite a healthy group with baseline values within healthy ranges, a marginally significant lowering of hs-CRP concentrations was observed in both the low-dose and high-dose groups. hs-CRP is a widely used, non-specific marker of inflammation produced by the liver. Butyrate derived from tributyrin is likely absorbed from the small intestine into the portal vein where it is available for first-pass metabolism in the liver. Cummings et al. investigated SCFA content in regions of the large intestine as well as in portal, hepatic and peripheral blood of sudden death victims. Hepatic blood concentrations of SCFAs were 61% lower than those of the portal vein, indicating significant extraction of SCFAs by the liver [31]. As an oral supplement, small intestinal absorption of butyrate by-passes colonocyte extraction and may allow for more direct effects on the liver. Butyrate contributes to a wide variety of biological processes in the gut–liver axis. Among its beneficial properties, anti-inflammatory, metabolism regulatory and anti-oxidative effects have been documented [32]. A study by Roshanravan et al. observed significantly decreased TNF-α m-RNA in monocytes isolated from participants with type 2 diabetes supplemented with sodium butyrate followed by significantly decreased hs-CRP compared to the placebo control group. Based on the data, they proposed that butyrate inhibits NF-kappa B-related inflammatory gene activation, thereby reducing inflammation [33]. Furthermore, available data suggests that histone deacetylase (HDAC) inhibition prevents NF-kappa B activation by suppressing proteasome activity. In vitro studies suggest that butyrate and the specific HDAC inhibitor trichostatin A (TSA) reduced cellular proteasome activity similarly [34]. Results of the current study data indicates possible effects of butyrate’s HDAC activity and G-protein coupled receptor activation, albeit subtly, related to observations in hs-CRP, glucose and TG [32]. The effect of tributyrin supplementation in individuals with metabolic dysregulation is an area rich for future investigation.
Significant dose-related intervention effects on analytes measured in the present study were not observed with ButyraGen® supplementation. However, a possible low dose phenomenon was observed, particularly in IFN-γ and glucose concentrations. In both measures, the low dose group showed trends of lower IFN-γ and lower glucose with supplementation, while the high dose group showed trends of increased concentrations with supplementation. Further investigation is needed to understand the mechanisms contributing to these effects. It is possible that tributyrin, possibly as formulated in ButyraGen®, a generator of butyrate, has a hormetic effect [35]. Such an attribute would be ideal for chronic use as a dietary supplement, whereas much higher doses are effective for therapeutic and medicinal applications, as demonstrated in early clinical trials [16, 20].
This study had its strengths and its limitations. This was a 28-day study with a sequential placebo design where participants began the study in the placebo phase for 7 days before initiating the active supplementation phase for 21 days. A parallel-placebo study design may have provided more insight into the effects of ButyraGen® supplementation compared to no supplementation. The free-living conditions of the study participants may be considered a limitation due to inter-individual variability in usage, diet background, known microbiome variance, and other environmental factors influencing outcomes. However, an advantage is that we can better understand usage in real life conditions. The sample size was a limitation of this study, though this was designed to be a pilot study to inform future direction. The health status and age of our population may also be viewed as a limitation, although it was an appropriate starting point for considering tolerability and acceptance of a dietary supplement and potential biological effects for health maintenance, which surfaced for follow up research.
Few studies evaluating tributyrin supplementation in humans are available. Previous work demonstrated actions of tributyrin in people with solid tumors at pharmacological doses [16, 20]. This extremely high amount of butyrate or butyrate precursor would not be acceptable for acute or chronic daily supplement for maintenance of health. The present study demonstrated ButyraGen® is a promising tributyrin-containing complex that can be taken daily at a safe and tolerable level. This study was also informative regarding possible alternative mechanisms of butyrate generation and utilization outside of the large intestine. A proposed mechanism of action of tributyrin supplementation (as assessed using ButyraGen®) includes butyrate generation and absorption in the small intestine followed by transport to and activity in the liver, where further mechanistic evaluations are needed to unveil its downstream effects.
Acknowledgments
The clinical trial and sample analysis of blood markers and fecal SCFAs were conducted at the Center for Nutrition Research and the Department of Food Science and Nutrition, Illinois Tech. Special thank you to the volunteers participating in the study and all the staff and students helping on this project. Gut microbiome analyses of fecal samples were conducted at the Genomics and Microbiome Core Facility, RUSH University. Gut permeability analysis of urine samples were conducted by Genova Diagnostics, USA. Instrumentation for SCFA analysis was provided by Axion Analytical Labs Inc., Chicago, IL 60607, USA.
Funding
Funding for this study was provided by NutriScience Innovations (NSI). NSI provided the active and placebo capsules. NSI did not participate in any clinical aspect of the study including blinding/unblinding, randomization, or analysis of the clinical samples.
Author contributions
Conception M.S., M.L. and I.E.; performance of work M.S., S.T., A.N., B.B.-F. and I.E.; statistical analysis M.S., A.N. and B.B.-F. interpretation of data M.S., A.N., B.B.-F. and I.E.; first draft written by M.S., review and feedback provided by M.L., M.G., S.T. A.N., B.B.-F. and I.E. All authors had access to the data. All authors have read and agreed to the published version of the manuscript.
Conflict of interest
M.L. is an employee and M.G. was an employee of NSI at the time of study execution. B.B.-F. is a board member of NSI. No other authors have a COI.
Supplementary material
[1] The supplementary material is available in the electronic version of this article: https://dx.doi.org/10.3233/NHA-240005.
References
[1] | Reynolds A , Mann J , Cummings J , Winter N , Mete E , Te Morenga L . Carbohydrate quality and human health: a series of systematic reviews and meta-analyses. The Lancet. (2019) ;393: (10170):434–45. |
[2] | Veronese N , Solmi M , Caruso MG , Giannelli G , Osella AR , Evangelou E , et al. Dietary fiber and health outcomes: an umbrella review of systematic reviews and meta-analyses. Am J Clin Nutr. (2018) ;107: (3):436–44. |
[3] | Kuo SM . The Interplay Between Fiber and the Intestinal Microbiome in the Inflammatory Response. Advances in Nutrition. (2013) ;4: (1):16–28. |
[4] | Slavin J . Fiber and prebiotics: mechanisms and health benefits. Nutrients. (2013) ;5: (4):1417–35. |
[5] | Roediger WE . Utilization of nutrients by isolated epithelial cells of the rat colon. Gastroenterology. (1982) ;83: (2):424–9. |
[6] | Salvi PS , Cowles RA . Butyrate and the Intestinal Epithelium: Modulation of Proliferation and Inflammation in Homeostasis and Disease. Cells. (2021) ;10: (7):1775. |
[7] | Hamer HM , Jonkers D , Venema K , Vanhoutvin S , Troost FJ , Brummer RJ . Review article: the role of butyrate on colonic function. Aliment Pharmacol Ther. (2008) ;27: (2):104–19. |
[8] | 1980 DGA. US Department of Agriculture and US Department of Health and Human Services. 1980 Feb;(1st Edition). |
[9] | Dietary Guidelines for Americans, 2020-2025. US Department of Agriculture and US Department of Health and Human Services. 2020 Dec;(9th Edition). |
[10] | Scheppach W , Sommer H , Kirchner T , Paganelli GM , Bartram P , Christl S , et al. Effect of butyrate enemas on the colonic mucosa in distal ulcerative colitis. Gastroenterology. (1992) ;103: (1):51–6. |
[11] | Pryde SE , Duncan SH , Hold GL , Stewart CS , Flint HJ . The microbiology of butyrate formation in the human colon. FEMS Microbiol Lett. (2002) ;217: (2):133–9. |
[12] | Roda A . A new oral formulation for the release of sodium butyrate in the ileo-cecal region and colon. World J Gastroenterol. (2007) ;13: (7):1079. |
[13] | Vernia P , Monteleone G , Grandinetti G , Villotti G , Di Giulio E , Frieri G , et al. Combined oral sodium butyrate and mesalazine treatment compared to oral mesalazine alone in ulcerative colitis: randomized, double-blind, placebo-controlled pilot study. Dig Dis Sci. (2000) ;45: (5):976–81. |
[14] | Mattace Raso G , Simeoli R , Russo R , Iacono A , Santoro A , Paciello O , et al. Effects of sodium butyrate and its synthetic amide derivative on liver inflammation and glucose tolerance in an animal model of steatosis induced by high fat diet. PLoS One. (2013) ;8: (7):e68626. |
[15] | Canani RB , Costanzo M Di , Leone L , Pedata M , Meli R , Calignano A . Potential beneficial effects of butyrate in intestinal and extraintestinal diseases. World J Gastroenterol. (2011) ;17: (12):1519–28. |
[16] | Conley BA , Egorin MJ , Tait N , Rosen DM , Sausville EA , Dover G , et al. Phase I study of the orally administered butyrate prodrug, tributyrin, in patients with solid tumors. Clin Cancer Res. (1998) ;4: (3):629–34. |
[17] | Egorin MJ , Yuan ZM , Sentz DL , Plaisance K , Eiseman JL . Plasma pharmacokinetics of butyrate after intravenous administration of sodium butyrate or oral administration of tributyrin or sodium butyrate to mice and rats. Cancer Chemother Pharmacol. (1999) ;43: (6):445–53. |
[18] | Smith M , Polite L , Christy A , Edirisinghe I , Burton-Freeman B , Sandhu A . An Improved Validated Method for the Determination of Short-Chain Fatty Acids in Human Fecal Samples by Gas Chromatography with Flame Ionization Detection (GC-FID). Metabolites. (2023) ;13: (11). |
[19] | Garrett-Bakelman FE , Darshi M , Green SJ , Gur RC , Lin L , Macias BR , et al. The NASA Twins Study: A multidimensional analysis of a year-long human spaceflight. Science. (2019) ;364: (6436). |
[20] | Edelman MJ , Bauer K , Khanwani S , Tait N , Trepel J , Karp J , et al. Clinical and pharmacologic study of tributyrin: an oral butyrate prodrug. Cancer Chemother Pharmacol. (2003) ;51: (5):439–44. |
[21] | Titlow M . Tributyrin compositions and methods therefor. United States patent; US11,141,442 B2, 2021. |
[22] | Miyoshi M , Sakaki H , Usami M , Iizuka N , Shuno K , Aoyama M , et al. Oral administration of tributyrin increases concentration of butyrate in the portal vein and prevents lipopolysaccharide-induced liver injury in rats. Clin Nutr. (2011) ;30: (2):252–8. |
[23] | Chen G , Zhuo R , Ding H , Yang K , Xue J , Zhang S , et al. Effects of dietary tributyrin and physterol ester supplementation on growth performance, intestinal morphology, microbiota and metabolites in weaned piglets. J Appl Microbiol. (2022) ;132: (3):2293–305. |
[24] | Li Z , Wang X , Wang W , An R , Wang Y , Ren Q , et al. Benefits of tributyrin on growth performance, gastrointestinal tract development, ruminal bacteria and volatile fatty acid formation of weaned Small-Tailed Han lambs. Anim Nutr. (2023) ;15: :187–96. |
[25] | Vinolo MAR , Rodrigues HG , Festuccia WT , Crisma AR , Alves VS , Martins AR , et al. Tributyrin attenuates obesity-associated inflammation and insulin resistance in high-fat-fed mice. Am J Physiol Endocrinol Metab. (2012) ;303: (2):E272–82. |
[26] | Sato FT , Yap YA , Crisma AR , Portovedo M , Murata GM , Hirabara SM , et al. Tributyrin Attenuates Metabolic and Inflammatory Changes Associated with Obesity through a GPR109A-Dependent Mechanism. Cells. (2020) ;9: (9). |
[27] | Yang N , Lan T , Han Y , Zhao H , Wang C , Xu Z , et al. Tributyrin alleviates gut microbiota dysbiosis to repair intestinal damage in antibiotic-treated mice. PLoS One. (2023) ;18: (7):e0289364. |
[28] | Liu H , Wang J , He T , Becker S , Zhang G , Li D , et al. Butyrate: A Double-Edged Sword for Health? Adv Nutr. (2018) ;9: (1):21–9. |
[29] | Cleophas MCP , Ratter JM , Bekkering S , Quintin J , Schraa K , Stroes ES , et al. Effects of oral butyrate supplementation on inflammatory potential of circulating peripheral blood mononuclear cells in healthy and obese males. Sci Rep. (2019) ;9: (1):775. |
[30] | Cummings JH , Englyst HN . Fermentation in the human large intestine and the available substrates. Am J Clin Nutr. (1987) ;45: (5):1243–55. |
[31] | Cummings JH , Pomare EW , Branch WJ , Naylor CP , Macfarlane GT . Short chain fatty acids in human large intestine, portal, hepatic and venous blood. Gut. (1987) ;28: (10):1221–7. |
[32] | Amiri P , Arefhosseini S , Bakhshimoghaddam F , Jamshidi Gurvan H , Hosseini SA . Mechanistic insights into the pleiotropic effects of butyrate as a potential therapeutic agent on NAFLD management: A systematic review. Front Nutr. (2022) ;9: :1037696. |
[33] | Roshanravan N , Mahdavi R , Alizadeh E , Ghavami A , Rahbar Saadat Y , Mesri Alamdari N , et al. The effects of sodium butyrate and inulin supplementation on angiotensin signaling pathway via promotion of Akkermansia muciniphila abundance in type 2 diabetes; A randomized, double-blind, placebo-controlled trial. J Cardiovasc Thorac Res. (2017) ;9: (4):183–90. |
[34] | Place RF , Noonan EJ , Giardina C . HDAC inhibition prevents NF-κB activation by suppressing proteasome activity: Down-regulation of proteasome subunit expression stabilizes IκBα. Biochem Pharmacol. (2005) ;70: (3):394–406. |
[35] | Calabrese EJ , Mattson MP . How does hormesis impact biology, toxicology, and medicine? NPJ Aging Mech Dis. (2017) ;3: :13. |