Cleistocalyx nervosum var. paniala seed extracts exhibit sigma-1 antagonist sensitive neuroprotective effects in PC12 cells and protects C. elegans from stress via the SKN-1/NRF-2 pathway
Abstract
BACKGROUND:
Cleistocalyx nervosum var. paniala (C. nervosum) is a plant that produces berries that are popular as a health food in Thailand. In previous studies we have identified C. nervosum fruit extracts to have anti-aging and anti-stress properties in C.elegans
OBJECTIVE:
Evaluate the neuroprotective properties of C. nervosum seed extracts and investigate the mechanisms behind their neuroprotective properties.
METHODS:
PC12 cells were differentiated using NGF and treated with amyloid-β (Aβ)(25–35) creating a model of neurodegeneration, in which C. nervosum seed extracts were tested for neuroprotective properties. Cell viability was measured after 24 hours of using the MTT assay. We also measured the lifespan and “health span” of various C.elegans mutants and the wild type.
RESULTS:
C. nervosum seed extract protects PC12 cells from Aβ(25–35) toxicity, as well as potentiating NGF induced neurite outgrowth in the same cell line potentially under the control of the sigma-1 receptor. C. nervosum seed extracts have anti-aging properties in C. elegans, causing a significant increase in lifespan of Aβ expressing C. elegans and wild type worms. This appears to be regulated by SKN-1/NRF-2, possibly via the sigma-1 receptor. The DAF-16 pathway is also involved in the lifespan extension of C. elegans.
CONCLUSIONS:
C. nervousum has been often used as a functional food. We have shown its neuroprotective properties which are sensitive to sigma-1 receptor antagonism in PC12 cells, and involve the SKN-1/NRF-2 and DAF-16 pathway in C.elegans. Further research into the active compounds may lead to future drugs for preventing or treating neurodegenerative disease.
1Introduction
The world’s population has grown significantly over the past century. Furthermore, better nutrition and better access to health care has resulted in an increase in the average age of the population. Aging is a major risk factor for many neurodegenerative diseases such as Alzheimer’s (AD) and Parkinson’s disease (PD) [1]. Neurodegenerative diseases can cause a reduction in life quality not only for the patient, but also for the family and friends who are left to care for the individual. It is estimated that there are 44 million people currently living with AD worldwide, and with no cure it is expected that this figure could rise to 135 million by 2050 [2]. The cost of caring for these patients is equivalent to 1 % of the worlds gross domestic product (GDP) which can put a strain of healthcare recourses [3].
Neurodegenerative diseases like dementia and AD are characterized by the loss of neurons in the brain as well as brain atrophy and the extracellular deposition of Aβ that results in the formation plaques. There is also hyperphosphorylation of tau protein leading to neurofibril tangles [4]. All of which results in loss of efficiency in energy production within the neurons, leading to oxidative stress and a disruption in calcium ion homeostasis, which in turn lead to cell death. There are many risk factors involved in the development of AD, such as age, diet [5] and the average amount of sleep an individual obtains per night [6]. While it is not possible to control the passage of time, and not easy to sleep more, as people age, they tend to sleep less [7, 8], it is possible for people to take control of their diets. The ‘Mediterranean diet’ and other similar diets have long been touted for its healthy ageing benefits, with its low fat and high antioxidant content. There have been a small number of studies which suggest such a diet (low fat, high fruits, berries and vegetables) may reduce the risk of AD and slow the decline of cognitive performance as people age [9–11].
The sigma-1 receptor is a ligand operated chaperone protein, which is a target for neurodegenerative disease as well as cancer [12–15]. It has been shown that in studies using positron emission tomography (PET) scanning that there is a decrease in sigma-1 receptors [16], whereas in normal aging there is no sigma-1 loss, in fact, studies in primates and rodents indicate an increase in sigma-1 receptor expression [17, 18]. The sigma-1 receptor is involved in preventing mitochondrial and endoplasmic reticulum (ER) stress [15, 19–22] as well as having a role in potential role in autophagy [23] and neurite growth [15, 24–27]. These properties as well as others such as the sigma-1 receptors role in tau phosphorylation and compartmentalization [28], make it an interesting target for the treatment of AD.
Cleistocalyx nervosum var. paniala (C. nervosum) is a berry bearing plant indigenous to northern Thailand. The berries are used in Thai traditional medicine and often used as a ‘functional food’ in health drinks due to its appealing sweet and sour flavor [29]. C. nervosum has been implicated as beneficial in a wide number of disease states, including caner, immune disease and neurodegenerative diseases [30]. The berries have a distinctive dark red color, produced by anthocyanins as well as other antioxidants and phenolic compounds [31]. There have been a number of studies investigating neuroprotective properties of C. nervosum, including its ability to protect against oxidative stress, calcium ion dysregulation, glutamate toxicity and ER stress [32–35]. It has been suggested that targeting multiple pathways either by using multiple drugs or a single less specific drug could be a strategy for the treatment of many neurodegenerative diseases, particularly AD and PD that have multiple causes and many pathways involved [13]. Naturally occurring phytochemicals derived from plant extracts could well provide the synergism required to prevent or treat neurodegenerative disease [36].
Caenorhabditis elegans are a widely used model for studying ageing, stress resistance and neurological disorders [37–39]. Their short life cycle and lifespan along with the ability to generate single gene mutants and the ease of handling make them an ideal model organism for studying aging and age-related diseases [40]. It has been observed that many plants or plant products including green tea [41], Streblus asper [42], Bacopa monnieri [43], Paullinia cupanna [44], and mulberry [45] can extend the lifespan and health-span and protect against stress in C. elegans models of disease. Previously, we have shown that the fruit pulp of C. nervosum is able to extend the ‘health-span’ and lifespan of wild type and an Aβ transgenic strain of C. elegans [29].
The aim of this study was to investigate the neuroprotective effects of C. nervosum seed extracts in vitro and in vivo and identify the pathways involved. We used cell culture to measure sigma-1 receptor sensative neurite outgrowth and Aβ toxicity, and C. elegans (wild type and mutants) to investigate the mechanisms involved in neuroprotection and lifespan increasing activity.
2Materials and methods
2.1Chemicals, reagents and equipment
Chemicals were purchased from Sigma-Aldrich. Tissue culture media materials were purchased from GibThai. C. elegans culture materials were purchased from HiMedia Laboratories (Mumbai, India) and the bacterial food source E. coli OP50 was purchased from Caenorhabditis Genetics Center, (University of Minnesota, USA). For ultra-violate (UV)-A exposure of C. elegans a UV transilluminator lamp, was used SANKYO DENKI (F20T10BL).
2.2Plant collection and extraction
C. nervosum was collected from the Plant Genetic Conservation Project initiated by Her Royal Highness Princess Maha Chakri Sirindhorn in Lampang (Thailand) and the seeds were collected, ground up and dried. The powdered seeds were extracted in ethanol using Soxhlet for 2 days. The resulting extract was concentrated at 50 °C using a rotary evaporator until all the ethanol was removed leaving a dry extract. The dried extract was then dissolved in dimethyl sulfoxide (DMSO) at 10 mg/ml and stored at -20 °C until further use. From here onwards C. nervosum extract is referred to as LMKS.
2.3Cell culture
PC12 cells were purchased from Japanese Collection of Research Bioresources (Tokyo, Japan) and maintained in Roswell park memorial institute (RPMI) -1600 medium supplemented with 5 % fetal bovine serum (FBS), 10 % horse serum (HS), penicillin (50 I.U./ml) and streptomycin (50 μg/ml). The cells were incubated at 37 °C in a humidified 5 % CO2 atmosphere, with the media being replaced every 2–3 days and the cells passed approximately every 7 days. To remove large clumps of cells the cells were centrifuged at 500 g resuspended in fresh growth media and passed through a 24-gauge needle approximately 20 times; this was carried out prior to counting and plating cells.
Neuro2A expressing the sweedish mutant of the amyloid precursor protein (APP(Swe)), Neuro2A APP(Swe) cells [46], were cultured in Dulbecco’s minimum essential medium (DMEM) supplemented with 10 % FBS and incubated at 37 °C in a humidified 5 % CO2 atmosphere, with the medium being replaced every 2–3 days and the cells passed approximately every 7 days.
2.4Potentiation of neurite outgrowth in PC12 cells
For the differentiation of PC12 cells, the cells were plated on poly-d-lysine (PDL) plates (3,000 cells/cm2) and allowed to adhere overnight. The following day the medium was replaced with RPMI-1600 supplemented with 2 % HS and a submaximal concentration of nerve growth factor (NGF) (2.5–10 ng/ml). The cells were treated with LMKS (0.01–1 μg/ml) and incubated for 5–7 days with the medium and LMKS being replaced every 2–3 days. The cells were imaged using a Zeiss Axio Observer A1 Inverted Fluorescence Phase Contrast Microscope, and Image J was used to count the number of cells with neurites (defined as cellular extensions double the width of the cell) and the lengths of the neurites were measured using Neuron J (an Image J plugin) [47].
2.5Protection against beta-amyloid toxicity in differentiated PC12 cells
PC12 cells were left to adhere to precoated PDL plates (30,000 cells/cm2) overnight in RPMI-1600 supplemented with 10 % HS and 5 % FBS. Subsequently the medium was replaced with RPMI-1600 supplemented with 2 % HS and NGF (50 ng/ml). Cells were then incubated for 5–7 days to allow differentiation in a humidified 5 % CO2 atmosphere at 37 °C, with the medium being replaced every 2–3 days. Once the PC12 cells were differentiated (5–7 days after the addition of NGF), the cells were pretreated with LMKS (1–0.1 μg/ml) for 24 hours prior to the addition of 10 μM Aβ(25–35). Treated and control cells were incubated for a further 18 hours with 10 μM Aβ(25–35), before the cell survival was measured using the 3-(4,5-dimethylthiazol-2-yl)-2,5-diphenyltetrazolium bromide (MTT) assay as described previously [15, 48]. Aβ(25–35) was selected over the Aβ(1–40) and Aβ(1–42) fragments as Aβ(25–35) shows a much faster fibril formation rate than the longer amyloid peptides and retains the toxicity of the full length Aβ(1 –40/42) peptides [49].
2.6Dopamine toxicity in neuro2A APP(Swe) cells
Dopamine toxicity in Neuro2A APP(swe) was carried out as described previously [15, 46]. Briefly, Neuro2A APP(swe) cells were plated at approximately in 2.5×104 cells/cm2, and allowed to adhere overnight in DMEM containing 10 % FBS at 37 °C (humidified 5 % CO2 atmosphere). The following day the cells were treated with varying concentrations of LMKS and 150 μM dopamine. The cells were then incubated for further 18 hours and cell viability was measured using the MTT assay as described previously [15, 48].
2.7C. elegans strains used and culture conditions
The Wild type strain N2 (Bristol), daf-16 mutant (CF1038) and Aβ transgenic strain (CL2006) were obtained via the Caenorhabditis Genetics Center, (University of Minnesota, USA), and maintained in Nematode growth medium (NGM) at 15 °C unless otherwise specified. Experiments were carried out using age synchronized young adult worms.
2.8Lifespan assay
The C. elegans life span assay was carried out as described previously [29, 43]. Briefly a known number of worms (usually 10 age synchronized worms per treatment) were place in a 24 well plate containing M9 buffer along with E. coli OP50 and 5-Fluoro-2′-deoxyuridine (FUDR) to prevent progeny worms. The worms were treated with a range of LMKS concentrations (between 1–100 μg/ml) and the total number of live worms were counted every 24 hours. Dead worms were identified as worms which did not respond to a gentle touch from the platinum loop. The experiment was carried out in the same way for the wild type and mutant worms. Each experiment was carried out in 5 independent trials, and data is presented as mean±Standard error (SEM).
2.9Pharyngeal pumping assay
Pharyngeal pumping was assessed as described previously [37]. A known number of worms (usually 10 per treatment) were transferred to nematode growth medium (NGM) plates treated with varying concentrations of LMKS. Pharyngeal pumping was observed using a stereo microscope (Motic SMZ-171) for 30 seconds once every 24 hours. NGM plates treated with E. coli OP50 was used as the control.
2.10Florescence imaging
Reactive oxygen species (ROS) staining with H2DCFDA, Lipofuscin (autofluorescence) and Nuclear factor erythroid 2-related factor 2 (NRF2) green fluorescent protein (GFP) imaging were carried out as described previously [29, 43]. Worms were treated with varying concentrations of LMKS or OP50 as the control. For imaging the worms were washed thoroughly in M9 buffer and placed on a glass slide along with a drop of sodium azide to immobilize the worms. Images were taken with ZEISS LSM 700 confocal microscope using 10×magnification at the objective lens.
2.11Total RNA isolation and real-time (RT) Polymerase chain reaction (PCR) analysis
RNA was extracted from the worms using the TRIZOL method as described previously [29]. RNA was converted to cDNA using Accupower RT Premix (Bioneer, Korea) with oligo dT primers following the manufacturer’s instructions. SYBR Green, Green Star PCR Master Mix (Bioneer, Korea) was used for the real time PCR with the specific primes detailed in Table 1. The expression data was normalized to β-actin and then represented as up or downregulated by normalizing to the untreated control.
Table 1
Primers used in PCR analysis of gene expression
Gene name | Forward primer | Reverse primer |
act-2 | ATCGTCCTCGACTCTGGAGATG | TCACGTCCAGCCAAGTCAAG |
age-1 | ATAGAGCTCCACGGCACTTT | ATAGAGCTCCACGGCACTTT |
col-19 | CACACAAATGCTCCACCAAC | CTGGATTTCCCTTCTGTCCA |
daf-16 | TGGTGGAATTCAATCGTGAA | ATGAATATGCTGCCCTCCAG |
daf-2 | TCGAGCTCTTCCTACGGTGT | CATCTTGTCCACCACGTGTC |
dgk-1 | GTTGGGGAAGTGGTGCAAAT | GCGAGCTTGGATTGGATGAG |
egl-30 | TCAGAAAGGCGGAAGTGGAT | GGTTCTCGTTGTCACACTCG |
egl-8 | CGTATCGTTGCGCTTCTCA | AGTAGTGACACAGCGGTTG |
goa-1 | TGTTCGATGTGGGAGGTCAA | TCGTGCATTCGGTTTGTTGT |
sir-2.1 | CGGGGAAGTGCAAGAAATAA | GAGTGGCACCATCATCAAGA |
skn-1 | ATCCATTCGGTAGAGGACCA | GGCGCTACTGTCGATTTCTC |
utx-1 | GCAGAACACCAGCTCATCAG | ATCAACGCCATTCTTCTCGC |
2.12Statistical analysis
Unless otherwise stated data is presented as the mean±SEM from three independent experiments. Statistical analysis was carried out using GraphPad Prism version 8.0 for Mac. Analysis of variance (ANOVA) was used to compare the means of the treatments to the controls, Dunnett’s post hoc test was used unless otherwise stated in the results. The P values obtained are reported in the results section. P values less than 0.05 were considered significant.
2.13Data availability
Data can be made available to any who require via reasonable request to the corresponding author.
3Results
3.1In vitro Aβ toxicity
Here we used PC12 cells differentiated with 50 ng/ml NGF over 5–7 days (Fig. 1A) as the model for neuron damage by Aβ(25–35) (Fig. 1B). The treatment with 10 μM Aβ(25–35) caused damage to the cells (shown by the white arrows indicating the shrunken cells) and damage to the neurites (blue arrows indicated broken and tangled neurites). Pretreatment with 0.01–10 μg/ml LMKS appeared to have a protective effect on the cells (Fig. 1C-F) preventing cell shrinkage and damage to the neurites.
Fig. 1
Micrographs of differentiated PC12 cells exposed to Aβ(25–35). A) Control PC12 cells (differentiated in 50ng/ml NGF). B) Differentiated PC 12 cells treated with 10 μM Aβ(25–35). The image shows damaged neurites (black arrows) and shrunken cells (white arrow heads). Differentiated PC 12 cells treated with 10 μM Aβ(25–35) plus (C) LMKS (0.01 μg/ml) (D) LMKS (0.1 μg/ml) (E) LMKS (1 μg/ml) (F) LMKS (10 μg/ml).
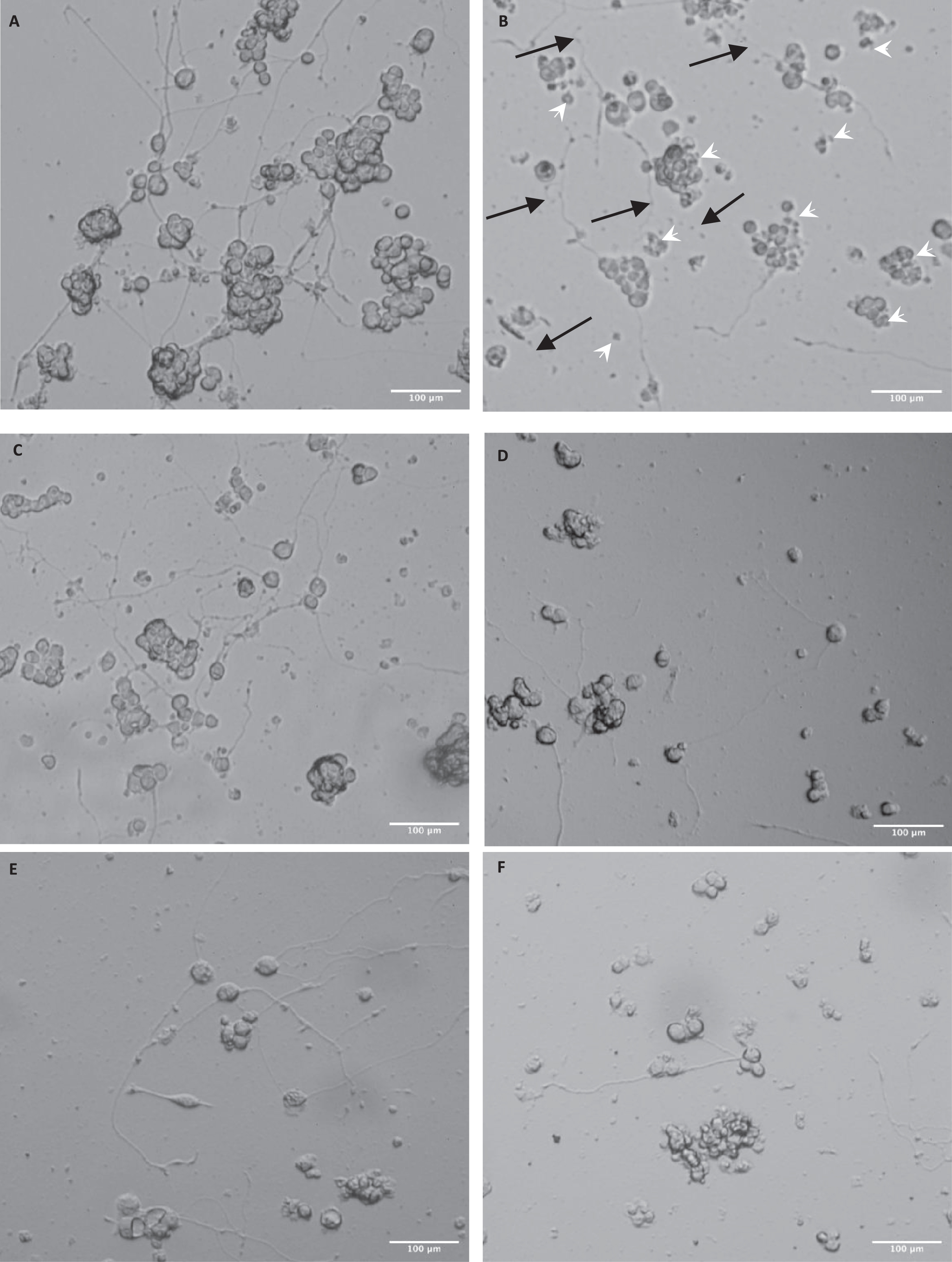
We also endeavored to quantify the effect of Aβ on the viability of NGF differentiated PC12 cells using the MTT assay (Fig. 2A). We found that Aβ reduced the metabolism of MTT by almost 60 % compared to the control (statistically significant ANOVA followed by Dunnett’s multiple comparison post hoc test P < 0.0001). Furthermore, we found that LMKS pretreatment was able to reduce they cytotoxic effect of Aβ on NGF differentiated PC12 cells with statistical significance observed at 0.1 μg/ml (ANOVA followed by Dunnett’s multiple comparison post hoc test P = 0.041)
Fig. 2
A) MTT Assay of differentiated PC12 cells treated with LMKS and/or 10 μM Aβ(25–35). §Significant difference in MTT metabolism between control and Aβ(25–35) treated PC 12 cells (ANOVA followed by Dunnett’s post hoc multiple comparison test p < 0.0001). *Significant difference in MTT metabolism between Aβ(25–35) treated PC12 cells and LMKS 0.1 μg/ml Aβ(25–35) treated PC12 cells (ANOVA followed by Dunnett’s post hoc multiple comparison test p = 0.04). B) The effect of LMKS on neurite outgrowth in PC12 cells. *Significant increase in neurite growth compared to the control ANOVA followed by Dunnett’s post hoc multiple comparison test p = 0.04).
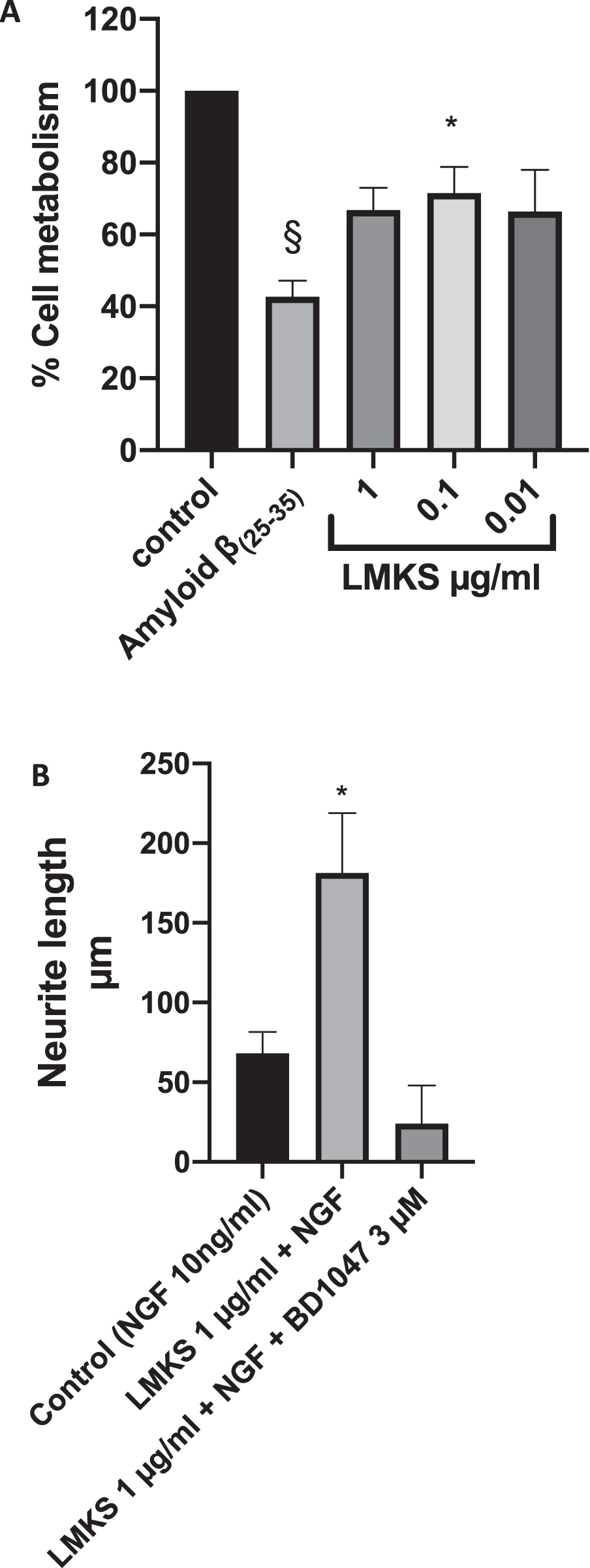
3.2Potentiation of neurite outgrowth in PC12 cells
For the potentiation of neurite outgrowth, the PC12 cells were exposed to a sub maximal dose of NGF (10 ng/ml). After seven days of treatment the average neurite length of the control cells was measured at 68.3±23.0 μm. Whereas the treatment with LMKS (1 μg/ml) along with 10 ng/ml NGF resulted in an average neurite length of 181.3±37.5, which was statistically significant (ANOVA, followed by Dunnett’s post hoc multiple comparison test P = 0.043). Potentiation of neurite outgrowth has been shown to involve the sigma-1 receptor [25–27, 50, 51]. When we introduced the sigma-1 receptor antagonist BD1047, the average neurite length was of 24.0±24.1, confirming the mechanistic role of the sigma-1 receptor (Fig. 2B).
3.3Dopamine toxicity in neuro2A APP cells
Treatment of Neuro2A cells with 150 μM dopa-mine causes ROS induced cell death, resulting in only 22±12 % cell viability measured with the MTT assay. Treatment with LMKS (0.03 –1 μg/ml) had no significant effect on the cell viability of the dopamine treated cells, with the highest being 0.03 μg/ml which had a cell viability of 28±18 % (Fig. 3).
Fig. 3
MTT assay of N2A APPswe cells treated with LMKS and/or dopamine (150 μM).
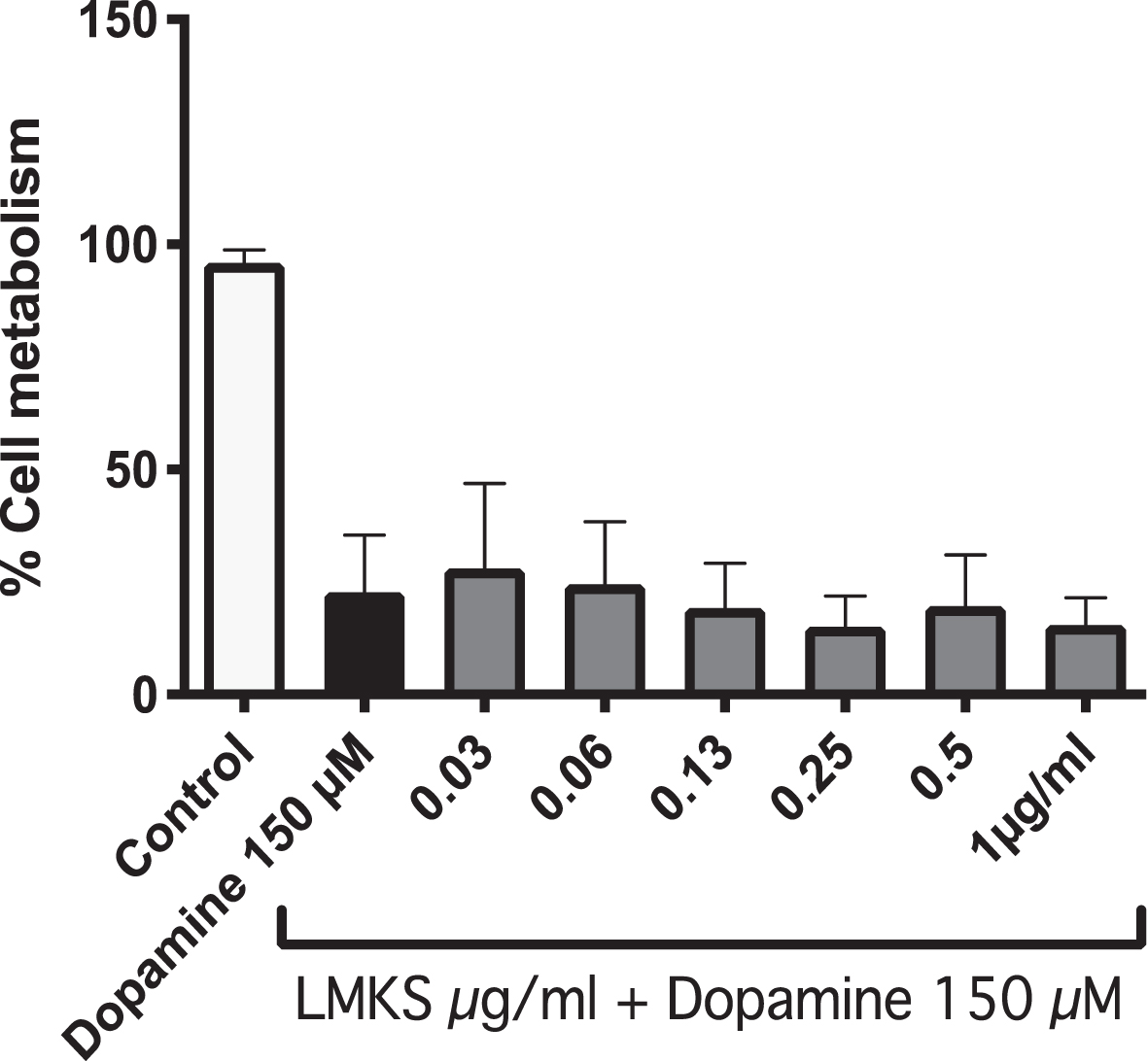
3.4LMKS effect on C. elegans lifespan: Wild type and mutants
LMKS was found to be non-toxic (Fig. 4A) at all the concentrations tested except for 100 μg/ml, which reduced the median survival time (Fig. 4B) from 17.5±1.0 days (control) to 13.5±0.5 days (LMKS 100 μg/ml), and this was statistically significant (ANOVA followed by Dunnett’s post hoc multiple comparison test P = 0.002). LMKS also affected the maximal life span with 10 and 20 μg/ml extending the maximal life span (Fig. 4C) from 25.3±0.3 days to 30±0.4 and 29.0±0.9 days respectively. These changes in maximal lifespan were statistically significant (ANOVA followed by Dunnett’s post hoc multiple comparison test P < 0.001). The maximal lifespan was also cut short by 100 μg/ml reducing the life span to 23.0±0.4 days, and this was statistically significant (ANOVA followed by Dunnett’s post hoc multiple comparisons test P < 0.05).
To confirm that the lifespan extension seen is not a result of calorie restriction in the worms, we measured the pharyngeal pumping of the worms treated with LMKS (Fig. 4D). No effects on pharyngeal pumping were observed when treated with LMKS at 10 or 20 μg/ml.
Transgenic C. elegans strains expressing Aβ have a reduced lifespan (Fig. 4E); however, LMKS treatment of the Aβ mutant (10–40 μg/ml) increased the median lifespan to 20.3±1.2 days and 19.2±1.3 days (10–20 μg/ml), respectively, from the untreated control (13.2±0.7 days) (Fig. 4F) and increased the maximal lifespan to 28.3±0.3 days (10 μg/ml), compared to 13.2±0.7 days of lifespan in untreated control (Fig. 4G).
The sole C. elegans ortholog of the Forkhead box protein O1 (FOXO) family of transcription factors is daf-16. It is responsible for activating genes involved in longevity and survival of oxidative stress [52]. We tested LMKS in the daf-16 mutant strain of C. elegans to monitor if it could extend the lifespan in C. elegans independently of daf-16 (Fig. 4H). The daf-16 mutant had a median and maximum lifespan of 10.3±0.4 and 14.3±0.3 days, respectively. LMKS at 10 and 20 μg/ml increased the median lifespan (Fig. 4I) to 14.8±0.6 and 14.8±0.4 days, respectively. LMKS 10–40 μg/ml also increased the maximum lifespan (Fig. 4J), with 10 μg/ml being the most effective (21.7±0.3 days).
Fig. 4
The effect of LMKS on the lifespan of wild type C.elegans. A) Survival curve for increasing concentrations of LMKS compared to the control. B) The median survival in days of the worms treated with LMKS (mean±SEM; n = 4). C) The maximal life span of worms treated with LMKS compared to the control (mean±SEM; n = 4). D) The effect of LMKS on pharyngeal pumping. E) Survival curve for increasing concentrations of LMKS compared to the Aβ controls. F) The median life span of Aβ expressing worms treated with LMKS (10–40 μg/ml) (mean±SEM; n = 4). G) The maximum lifespan of wild type and Aβ expressing worms treated with LMKS (10–40 μg/ml) (mean±SEM; n = 4). H) Survival curve for increasing concentrations of LMKS compared to daf-16 mutant controls. I) The median life span of daf-16 mutant worms treated with LMKS (10–40 μg/ml) (mean±SEM; n = 4). J) The maximum lifespan of daf-16 mutant worms treated with LMKS (10–40 μg/ml) (mean±SEM; n = 4). *Statistically significant change from control measured by ANOVA followed by Dunnett’s post hoc multiple comparison test. P < 0.05. **P < 0.01. ***P < 0.001.****P < 0.0001.
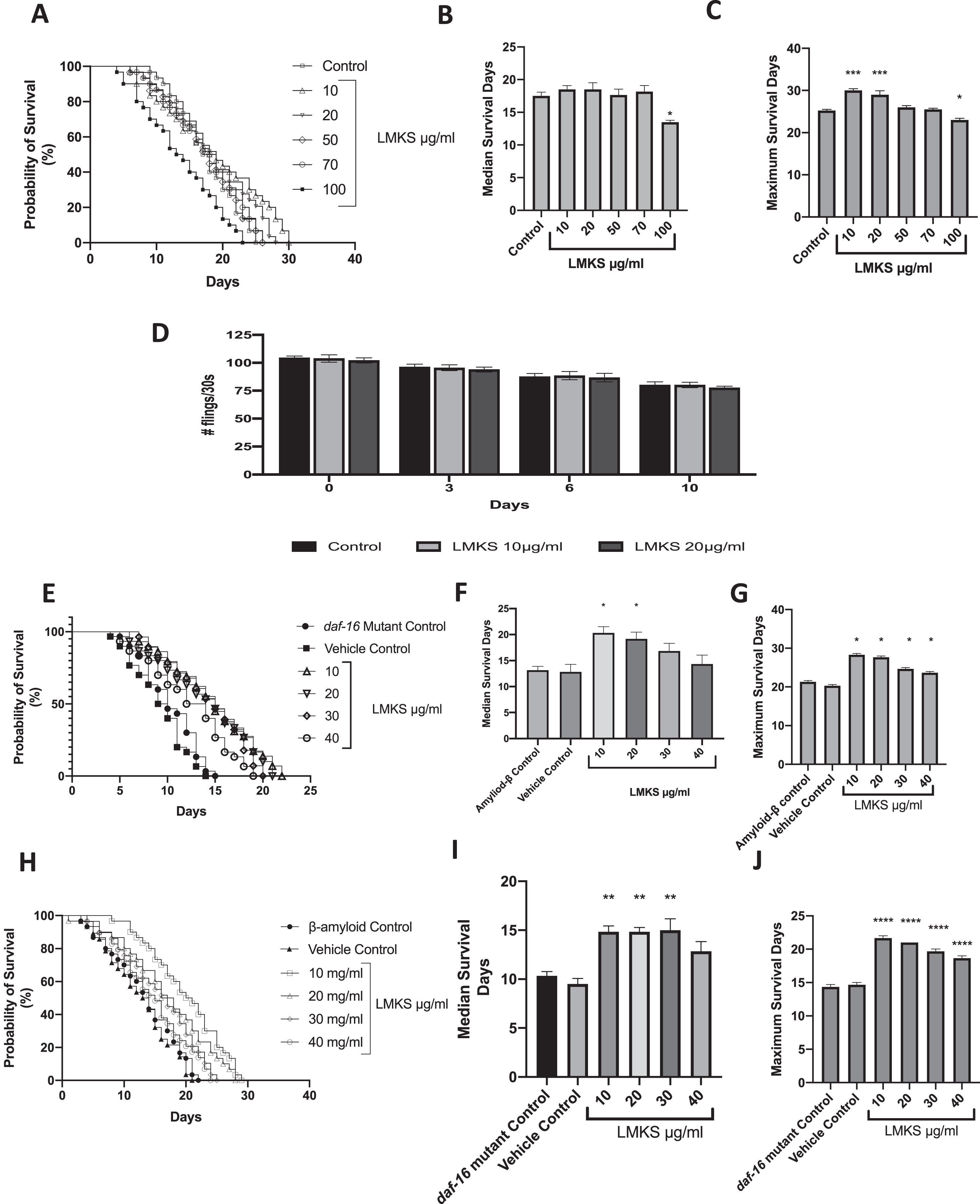
3.5LMKS effect on lipofuscin, ROS and NRF2 expression
We investigated whether LMKS affected the de-position of lipofuscin in wild type C. elegans (Fig. 5A-E). LMKS at 10 and 40 μg/ml was able to reduce the deposition of lipofuscin (Fig. 5F).
Fig. 5
Representative micrograph of lipofuscin expression in worms (A) without LMKS, (B) with 10 μg/ml LMKS, (C) 20 μg/ml LMKS, (D) 30 μg/ml LMKS, (E) 40 μg/ml LMKS, (F) Analysis of individual worms from 3 independent experiments. §Statistically significant difference compared to control (ANOVA followed by Dunnett’s post hoc multiple comparison test p = 0.01 (10 μg/ml) and p = 0.02 (20 μg/ml)).
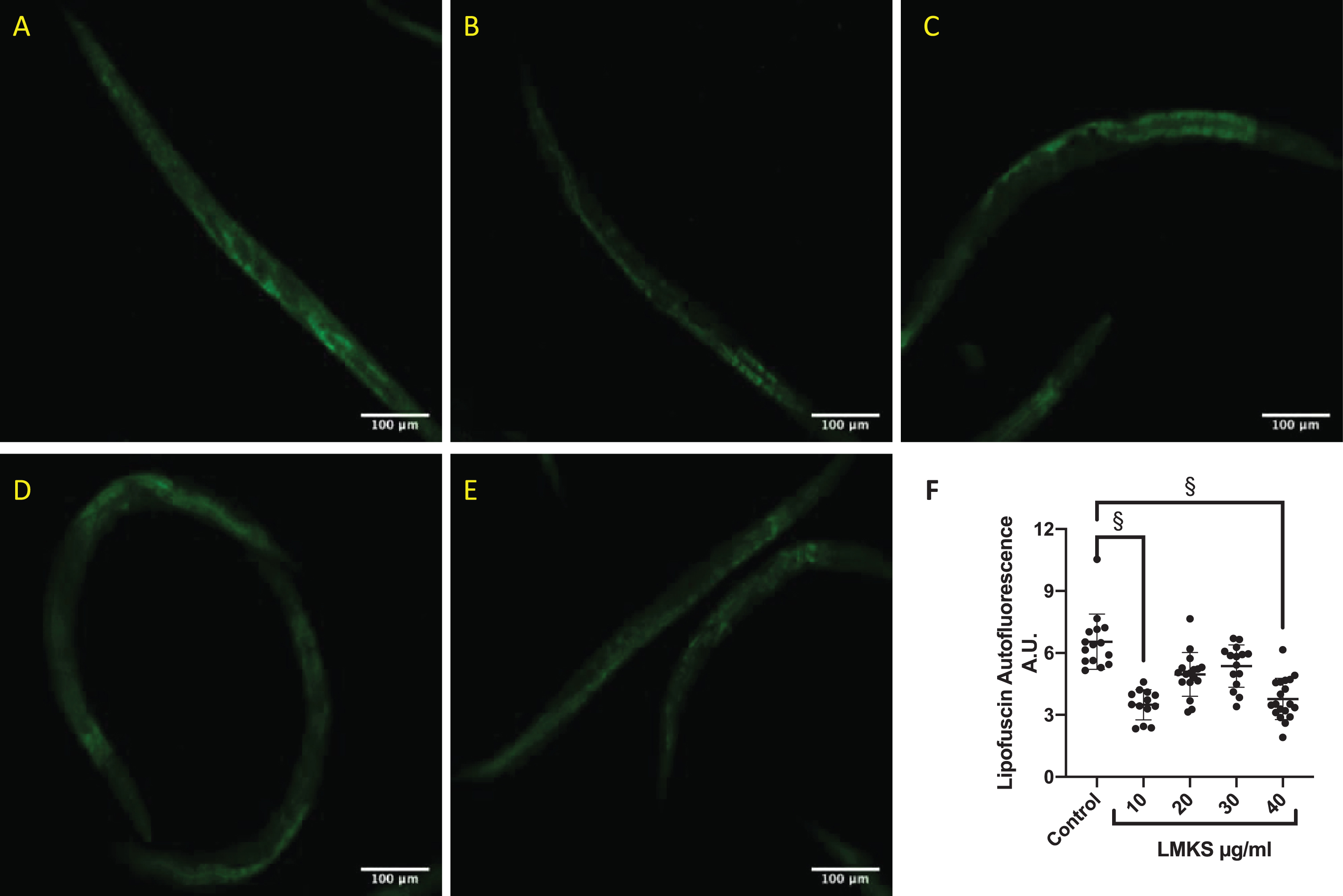
ROS damage to cells is a key component of aging and cellular damage in neurodegenerative disease. We initiated ROS expression in C. elegans using ex-posure to UV-A (Fig. 6A-B). LMKS treatment at the same time as the UV-A exposure and post UV-A exposure was able to reduce ROS expression within the worms (Fig. 6C-F). These protective and repair effects of LMKS were statistically significant (Fig. 6G) (ANOVA followed by Dunnett’s post hoc multiple comparison test P < 0.001) indicating the antioxidant potential of LMKS.
Fig. 6
ROS production in worms exposed to UV-A for 4 hours (representative micrographs). A) Control worms with no UV-A exposure. B) Worms exposed to 4 hours of UV-A. C) Worms cotreated with 10 μg/ml LMKS and exposed to 4 hours of UV-A. D) Worms cotreated with 20 μg/ml LMKS and exposed to 4 hours of UV-A. E) Worms exposed to 4 hours of UV-A and subsequently treated with 10 μg/ml LMKS. F) Worms exposed to 4 hours of UV-A and subsequently treated with 20 μg/ml LMKS. G) Analysis of the florescence of each individual worm over 3 independent experiments (mean±SD n = 3). §Significant difference compared to the control (non-UV exposed) (ANOVA followed by Dunnett’s post hoc multiple comparison test P < 0.0001). ****(Statistically significant difference compared to UV 4h ANOVA followed by Dunnett’s post hoc multiple comparison test P < 0.0001).
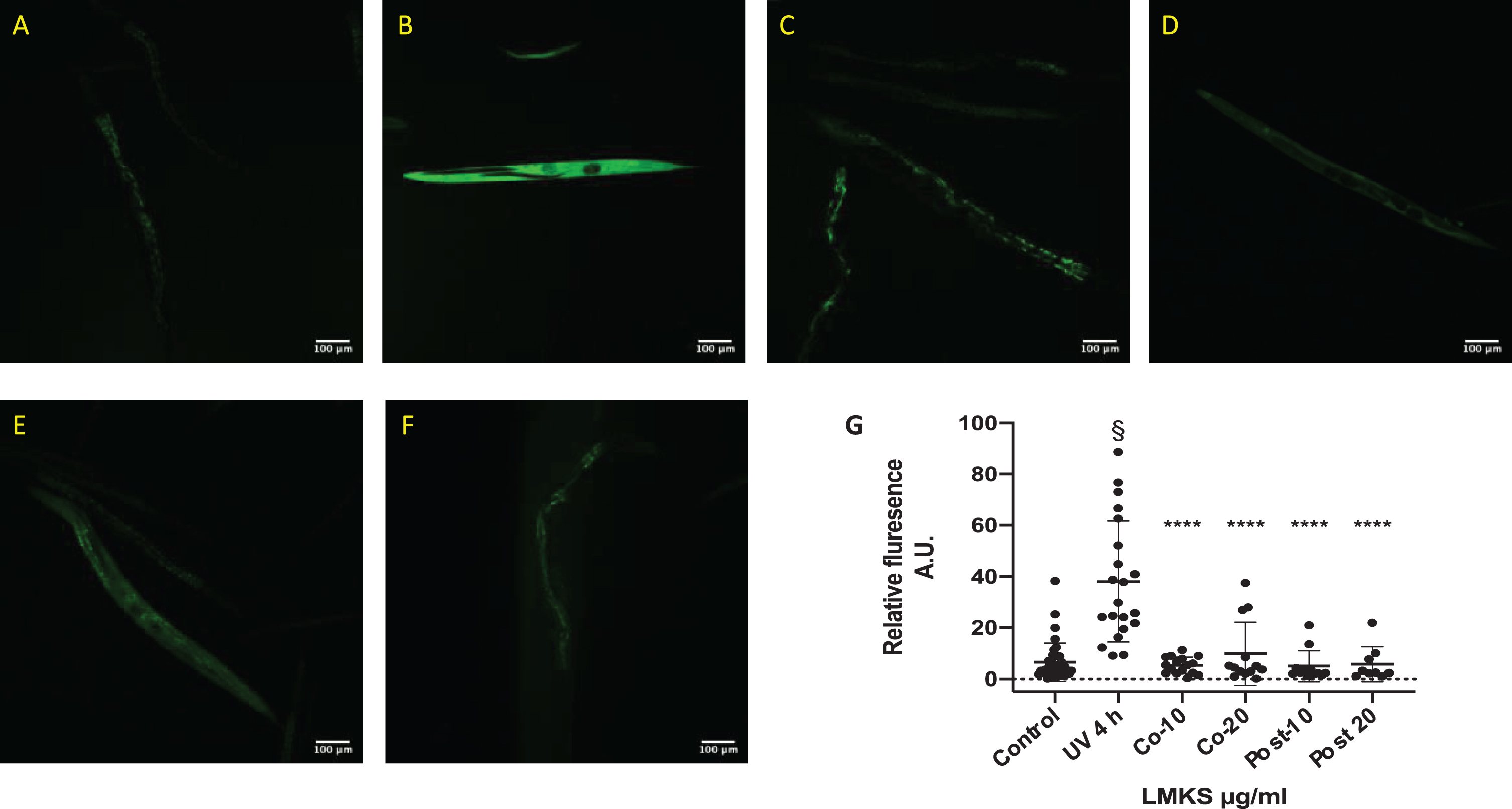
NRF2 is a mammalian transcription factor and ortholog of the C. elegans transcription factor SKN1. NRF2/SKN1 regulate the expression of antioxidant proteins in response to injury and inflammation [53, 54]. We used a transgenic strain of C. elegans (skn-1-GFP) to investigate whether LMKS treatment activated the NRF2/SKN1 pathway (Fig. 7). Exposure to UV-A for 4 hours caused a small but not statistically significant increase in GFP signal (Fig. 7A-B). However, treatment with LMKS (10 μg/ml) subsequent to UV exposure increased NFR2-GFP expression compared to the UV treated worms (Fig. 7C), although LMKS (20 μg/ml) did not (Fig. 7D) (ANOVA followed by Dunnett’s post hoc multiple comparisons test **P = 0.0095). These differences are quantified in Fig. 7E.
Fig. 7
SKN-1-GFP expressing C.elegans exposed to UV-A for 4 hours. A) Control. NRF2-GFP expressing worms with no UV-A exposure. B) NRF2-GFP worms exposed to 4 hours of UV-A. C) NRF2-GFP worms treated with 10 μg/ml LMKS after exposure to 4 hours of UV-A. D) NRF2-GFP worms treated with 20 μg/ml LMKS after exposure to 4 hours of UV-A. E) Quantification of fluorescence of the individual worms. Significant difference compared to UV 4h using ANOVA followed by Dunnett’s post hoc multiple comparisons test **P = 0.0095.
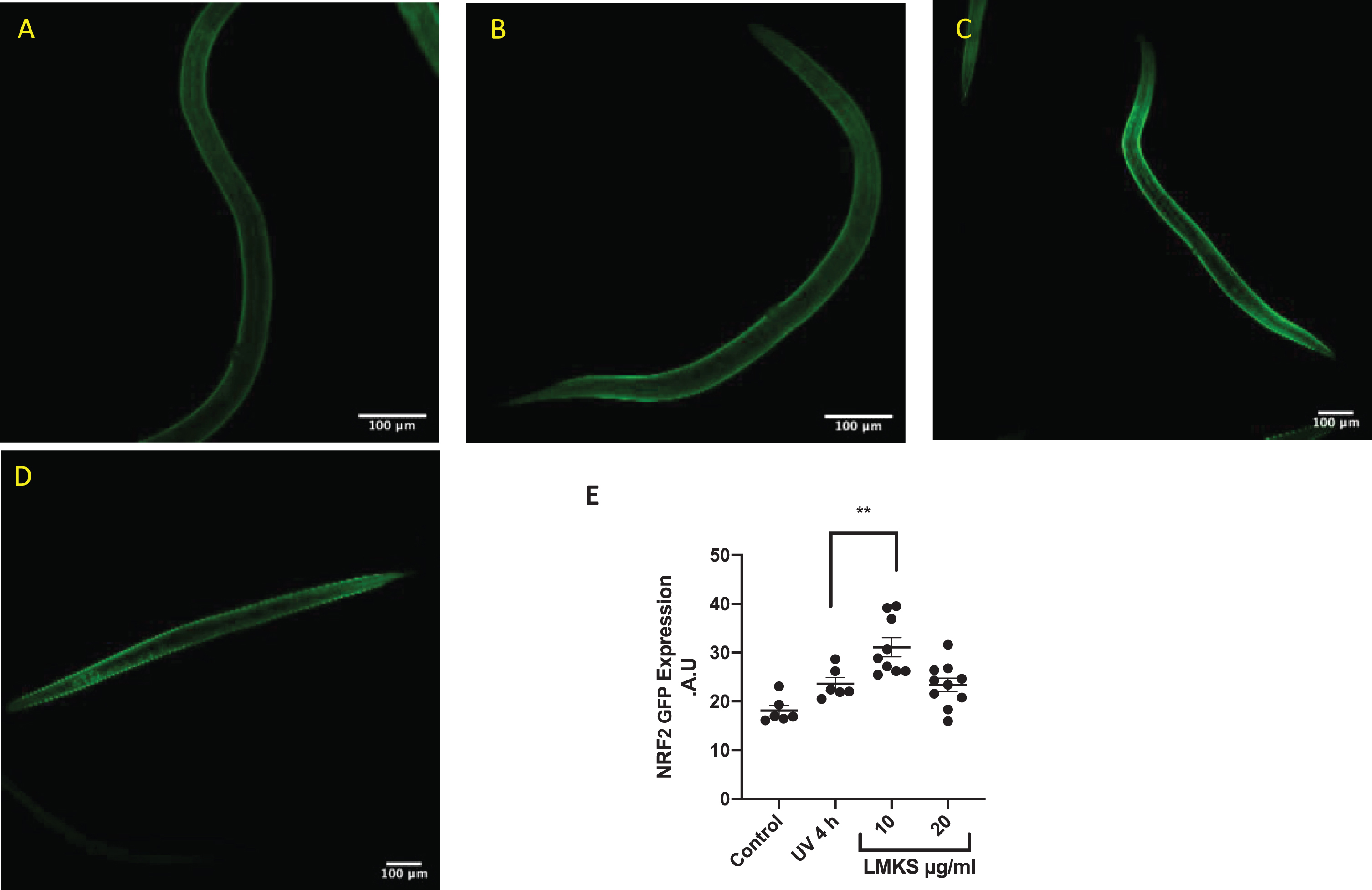
3.6LMKS effect on gene worm gene expression
We investigated the effect of LMKS on genes involved in the extension of lifespan (daf-16, daf-2, age-1 and utx-1), antioxidant mechanisms (skn-1 and sir-2.1) and genes involved in health-span (egl-8 and egl-30 col-19, dgk-1 and goa-1) (Fig. 8). We observed a small but significant down regulation of daf-2, age-1 and utx-1 (Wilcoxon one-sample t-test P < 0.05, P < 0.05, and P < 0.01 respectively). Whereas there was an aproximate 2-fold increase (LMKS 10 μg/ml) and an approximate 5-fold increase (LMKS 20 μg/ml) in daf-16 expression (Wilcoxon one-sample t-test P < 0.01 and P < 0.01 respectively). The expression of egl-8 (LMKS 10 μg/ml) sees an approximate 1-fold increase and egl-30 sees approximately a 2-fold increase in expression while col-19, dgk-1 and goa-1 all see small decreases in expression. Both skn-1 and sir-2.1 see an approximate 2-fold increase (both 10 and 20 μg/ml).
Fig. 8
Gene expression of worms exposed to LMKS (10 and 20 μg/ml) expressed as fold change compared to control. Statistically significant changes measured using one sample Wilcoxon t-test *p < 0.05 **p < 0.01 ***P < 0.001 ****P < 0.00.
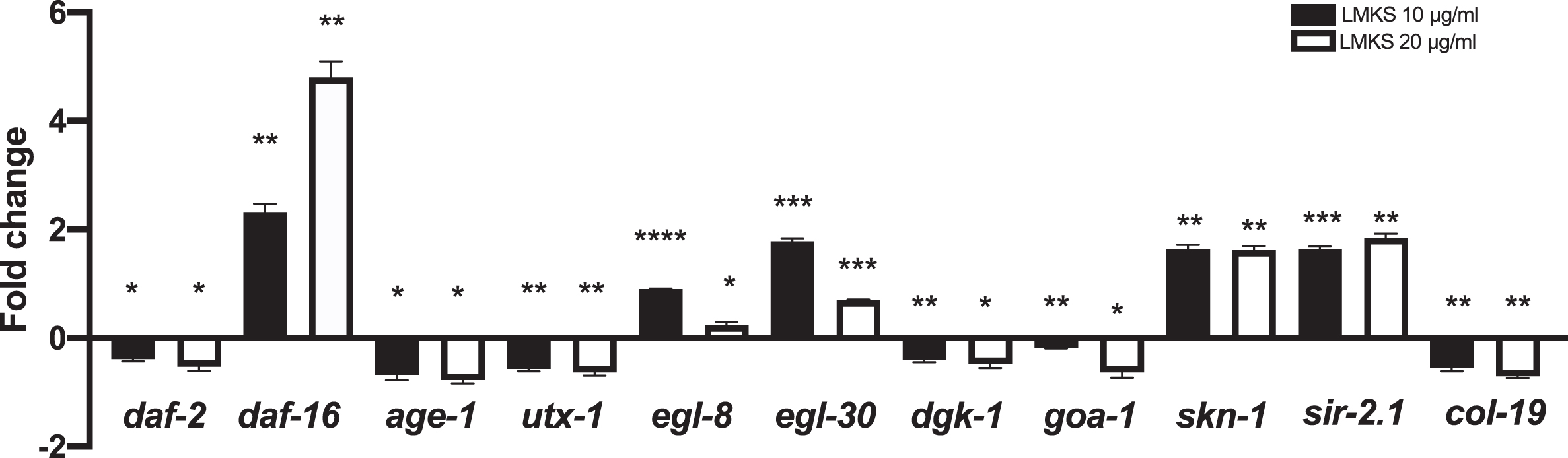
4Discussion
Oxidative stress induced activation of glial cells, neuroinflammation and mitochondrial dysfunction lead to various molecular events in brain leading to neurodegenerative disorders [55, 56]. Novel molecular peptides inhibiting the production of Aβ show promising effect in in vitro and animal model for AD [57]. However, majority of the drugs used will only reduce the symptoms of these diseases and cannot stop the process of neurodegeneration [58]. In this regard, plant-based therapies are gaining more focus in dealing with neurodegenerative disorders which can shed light on the mechanism of action also. LMKS was able to significantly extend the lifespan of transgenic Aβ strains indicating its neuroprotective potential.
Here we have shown that the seed extract of C. nervosum (LMKS) is neuroprotective against Aβ toxicity in PC12 cells (Fig. 1). The Aβ(25–35) fragment is derived from the Aβ protein by proteolytic cleavage of the Aβ(1–40) fragment [59]. It lacks the N-terminal domain and has a β-sheet structure [49] The Aβ(25–35) fragment can rapidly aggregate [60] and is involved in age dependent neurotoxicity and the pathogenesis of AD [61]. The Aβ(25–35) fragment has been used previously to induce neurotoxicity in cortical cultures [62] induce AD like symptoms in rats [63] and induce cell death in cultured PC12 cells [64]. Aβ treatment causes oxidative stress in neuronal cell lines [65], and previous work from this laboratory has shown that C. nervosum has neuroprotective effects via antioxidant and ER stress preventing mechanisms [29, 34]. Previous studies have shown that Neuro2A cells expressing APP or APP Swedish variant are susceptible to dopamine toxicity as the result of ROS production leading to apoptosis [15, 46]. Neuro2A cells expressing muscarinic receptors (M2) [66] are able to undergo cholinergic dependent differentiation with neurite elongation [67], and in the right conditions express tyrosine hydroxylase for the production of dopamine and L-DOPA [68], thus making these cells a useful tool in studying drug effects on parkinsonian symptoms in AD [15, 46]. However, LMKS did not provide any protection against dopamine toxicity in APP(swe) expressing Neuro2A cells (Fig. 3). The mechanism of cell death in this model is also ROS mediated [46], which suggests that LMKS is not acting purely as an antioxidant rather it is activating an antioxidant pathway. We and others have previously shown that activation of the sigma-1 receptor is neuroprotective and can activate a ROS-dependent mechanism [15, 21, 69, 70]. Furthermore, we have shown that LMKS is able to potentiate NGF induced neurite outgrowth (Fig. 2), which itself is regulated by the sigma-1 receptor [25–27, 51]. Moreover, using a sigma-1 receptor antagonist (BD1047) prevented the potentiation of the NGF induced neurite outgrowth (Fig. 2).
Both the sigma-1 receptor and amyloid peptides are able to regulate ERK phosphorylation, which has a role in neuron cell survival [71] as well as neurite outgrowth [72, 73]. Furthermore, the sigma-1 receptor [74] and Aβ proteins [75] are involved in the phosphorylation of tau protein, and neurons with reduced sigma-1 receptor exhibit an increase in Tau hyperphosphorylation [28]. Tau functions to stabilize microtubules [74], and its hyperphosphorylation results in neurofibril tangles [76]. Also, amyloid peptides, via ERK phosphorylation, modulate the phosphorylation of tau and its subcellular compartmentalization, an event that may lead to the formation of neurofibrillary tangles and to cell death in post-mitotic neurons. If LMKS contains a compound acting as a sigma-1 receptor agonist and activates the sigma-1 receptor it is possible that it is acting to reduce Tau hyperphosphorylation. While we cannot affirm conclusively, from the data presented, that the sigma-1 receptor is involved with the LMKS neuroprotective properties, it does appear to fit some of the qualities seen with sigma-1 receptor ligands. The LMKS extract contains a wide range of compounds, and there is a likelihood that one of those may well be a sigma-1 receptor agonist especially considering the number of different compounds that the sigma-1 receptor interacts with [12].
A number of studies have implicated the sigma-1 receptor in the mediation of the NRF2 antioxidant pathway [70, 77–79], with sigma-1 receptor agonists increasing the expression of NRF2 protein. Furthermore, a recent study investigating the effects of Cyanidin-3-glucoside (C3G) (one of the major compounds found in C. nervosum) showed that C3G could protect HT-22 cells from glutamate induced oxidative stress via the upregulation of NRF2 [80]. Using an in vivo C. elegans model of skn-1-GFP transgenic strain that expresses a ortholog of NRF2 we were able to show a statistically significant increase in skn-1-GFP expression in response to LMKS treatment (at 10 μg/ml but not 20 μg/ml) when the worms were exposed to UV-A to induce oxidative stress (Fig. 7). Moreover, with the qPCR analysis of skn-1 in wild type worms, we show that LMKS induces an approximate 2-fold increase in skn-1 expression (Fig. 8), which further suggests that LMKS is able to activate oxidative stress resistance pathway via the NRF2/SKN-1 protein pathways. It is interesting that the NRF2-response does not appear to be dose dependent, with the possibility of a bell-shaped dose response curve, again another feature of sigma-1 receptor agonism [13].
Previously, we have reported that C. nervosum fruit extract could extend lifespan and health-span along with extending neuroprotection in C. elegans. The fruit pulp with its immense health benefits has been explored by the researchers. However, there has been limited research about the seeds which also has reported to have antioxidant and anti-cancer properties [81, 82]. In this regard, the seed extracts were analyzed in C. elegans for their anti-ageing and neuroprotective efficacies. LMKS was able to extend the lifespan of wild type C. elegans (Fig. 4A-D), and this was not due to calorie restriction based on the pharyngeal pumping assay. Lipofuscin is the name given to a lipid containing pigment whose accumulation is considered to be a sign of aging [83]. It is formed by the oxidation of unsaturated fatty acids and may be a sign of membrane or mitochondrial damage [84]. LMKS was also able to reduce the accumulation of lipofuscin inside the worms (Fig. 5), indicating the improvement in health span. The gene expression of col-19, egl-8, egl-30, dgk-1 and goa-1 also supported this view (Fig. 8). Interestingly, the extract was able to significantly extend the lifespan of transgenic strains expressing Aβ indicating the neuroprotective potential of the extract. Several plant extracts have been reported to exhibit neuroprotective efficiency in C. elegans [85, 86]. ROS plays a key role in enhancing neurodegeneration. In this regard, the antioxidant effect of LMKS was analyzed in wild type worms challenged with ROS by exposing to UV-A. The significant reduction in the level of ROS in the worms co-treated and post-treated with LMKS confirmed the antioxidant potential of the extract (Fig. 6). Additionally, the expression of genes that mediate the antioxidant mechanism like skn-1 and sir-2.1 also supports this view (Fig. 8). Antioxidants such as green tea could mitigate oxidative stress via sir-2.1, the mammalian ortholog of Sirtuins [87]. Recent studies suggest that different plant extracts elicit antioxidant mechanisms, which thereby impart neuroprotection to the host [86–88].
DAF-16 is known to mediate longevity and reduce the level of oxidative stress, thus its activation may be useful in the prevention of AD. In the present study, LMKS was able to upregulate the lifespan of daf-16 negative mutants, indicating that LMKS can exert its protective properties independent of the daf-16 pathway. Interestingly, the extract was also able to upregulate the expression of daf-16 along with downregulating the expression of daf-2, age-1 and utx-1 indicating that the extract works both dependently and independently of the daf-16 pathway. We have observed similar pattern in our previous study with the C. nervosum fruit pulp extract [29]. As DAF-16 and SKN-1 are known to elicit neuroprotection [86], this could explain neuroprotective activity of LMKS.
5Conclusions
LMKS is able to provide significant protection against neurodegeneration, particularly against AD as it protected the Aβ treated PC12 cells and extended the lifespan of the C. elegans transgenic strain that expresses Aβ. The neuroprotection is likely to follow an NRF2/SKN-1 antioxidant pathway, which may be under the control of the sigma-1 receptor. LMKS also provides its protection both dependently and independently of daf-16. Further studies into the constituent compounds found within LMKS could provide new lead compounds for the treatment of AD. This study highlights the importance of seed extract that could impart health benefits along with the pulp extract, thereby confirming its possible use as ‘functional food’.
Acknowledgments
JMB and MIP were supported by the Rachadapisek Sompote Fund for Postdoctoral Fellowship, Chulalongkorn University, Thailand. CI received the Chulalongkorn University’s fellowship to support foreign visiting professor. The authors are also grateful to the Plant Genetic Conservation Project initiated by Her Royal Highness Princess Maha Chakri Sirindhorn.
Funding
This work was financially supported by The Royal Thai Government’s Research Fund 2018 and by the National Research Council of Thailand.
Conflict of interest
The authors declare no conflict of interest
Author contributions
JMB, CI and TT conceived the research idea, MS carried out the collection and extraction of the herb. MIP carried out all the C. elegans work and JMB carried out the Cell culture. JMB MIP and TT drafted the manuscript. CI provided the N2A APP cell lines and edited the final draft manuscript. All authors have seen and approved the final draft of the manuscript.
References
[1] | Christensen K , Doblhammer G , Rau R , Vaupel JW . Ageing populations: the challenges ahead. The Lancet. (2009) ;374: :1196–208. |
[2] | Prince M , Guerchet M , Prina M . The global impact of dementia 2013-2050, Alzheimer’s disease international, 2013. |
[3] | Guerchet M , Prina M , Prince M . Policy Brief for Heads of Government: The Global Impact of Dementia 2013–2050, Policy Brief for Heads of Government: The Global Impact of Dementia 2013–2050 Published by Alzheimer’s Disease International (ADI), London. December 2013 (2013), 1-8. |
[4] | Hamano T , Gendron TF , Causevic E , Yen SH , Lin WL , Isidoro C , DeTure M , Ko Lw , Autophagic-lysosomal perturbation enhances tau aggregation in transfectants with induced wild-type tau expression. European Journal of Neuroscience. (2008) ;27: :1119–30. |
[5] | Winiarska-Mieczan A , Baranowska-Wójcik E , Kwiecień M , Grela ER , Szwajgier D , Kwiatkowska K , Kiczorowska B . The Role of Dietary Antioxidants in the Pathogenesis of Neurodegenerative Diseases and Their Impact on Cerebral Oxidoreductive Balance. Nutrients. 2020;12. |
[6] | Brice KN , Hagen CW , Peterman JL , Figg J , Braden PN , Chumley MJ , Boehm GW . Chronic sleep restriction increases soluble hippocampal Aβ-42 and impairs cognitive performance. Physiol Behav. 2020;113128. |
[7] | Edwards BA , O’Driscoll DM , Ali A , Jordan AS , Trinder J , Malhotra A . Aging and sleep: physiology and pathophysiology, in: Seminars in respiratory and critical care medicine, NIH Public Access, 2010, pp. 618. |
[8] | Espiritu JRD . Aging-related sleep changes. Clinics in Geriatric Medicine. (2008) ;24: :1–14. |
[9] | Lourida I , Soni M , Thompson-Coon J , Purandare N , Lang IA , Ukoumunne OC , Llewellyn DJ . Mediterranean diet, cognitive function, and dementia: a systematic review. Epidemiology. (2013) ;24: :479–89. |
[10] | Petersson SD , Philippou E . Mediterranean Diet, Cognitive Function, and Dementia: A Systematic Review of the Evidence. Adv Nutr. (2016) ;7: :889–904. |
[11] | Singh VP , Bali A , Singh N , Jaggi AS . Advanced glycation end products and diabetic complications. The Korean Journal of Physiology & Pharmacology. (2014) ;18: :1–14. |
[12] | Brimson JM , Akula KK , Abbas H , Ferry DR , Kulkarni SK , Russell ST , Tisdale MJ , Tencomnao T , Safrany ST . Simple ammonium salts acting on sigma-1 receptors yield potential treatments for cancer and depression. Scientific Reports. (2020) ;10: :9251–9251. |
[13] | Brimson JM , Brimson S , Chomchoei C , Tencomnao T . Using Sigma-ligands as part of a multi-receptor approach to target diseases of the brain. Expert Opinion on Therapeutic Targets (2020). |
[14] | Brimson J , Brown C , Safrany S . Antagonists show GTP-sensitive high affinity binding to the sigma-1 receptor. British Journal of Pharmacology. (2011) ;164: :772–80. |
[15] | Brimson JM , Safrany ST , Qassam H , Tencomnao T . Dipentylammonium Binds to the Sigma-1 Receptor and Protects Against Glutamate Toxicity, Attenuates Dopamine Toxicity and Potentiates Neurite Outgrowth in Various Cultured Cell Lines. Neurotoxicity Research. (2018) ;34: :263–72. |
[16] | Mishina M , Ohyama M , Ishii K , Kitamura S , Kimura Y , Oda K-i , Kawamura K , Sasaki T , Kobayashi S , Katayama Y . Low density of sigma 1 receptors in early Alzheimer’s disease. Annals of Nuclear Medicine. (2008) ;22: :151–151. |
[17] | Kawamura K , Kimura Y , Tsukada H , Kobayashi T , Nishiyama S , Kakiuchi T , Ohba H , Harada N , Matsuno K , Ishii K . An increase of sigma1 receptors in the aged monkey brain. Neurobiology of Aging. (2003) ;24: :745–52. |
[18] | Wallace DR , Mactutus CF , Booze RM . Sigma binding sites identified by [3H] DTG are elevated in aged Fischer-344×Brown Norway (F1) rats. Synapse. (2000) ;35: :311–3. |
[19] | Villard V , Espallergues J , Keller E , Vamvakides A , Maurice T . Anti-amnesic and neuroprotective potentials of the mixed muscarinic receptor/sigma1 (σ1) ligand ANAVEX2-73, a novel aminotetrahydrofuran derivative. Journal of Psychopharmacology. (2011) ;25: :1101–17. |
[20] | Frecska E , Szabo A , Winkelman MJ , Luna LE , McKenna DJ . A possibly sigma-1 receptor mediated role of dimethyltryptamine in tissue protection, regeneration, and immunity. Journal of Neural Transmission (Vienna, Austria : 1996) (2013). |
[21] | Pal A , Fontanilla D , Gopalakrishnan A , Chae Y-K , Markley JL , Ruoho AE . The sigma-1 receptor protects against cellular oxidative stress and activates antioxidant response elements. European Journal of Pharmacology. (2012) ;682: :12–20. |
[22] | Hayashi T , Tsai SYS-Y , Mori T , Fujimoto M , Su T-P . Targeting ligand-operated chaperone sigma-1 receptors in the treatment of neuropsychiatric disorders. Expert Opinion Ther Targets. (2011) ;15: :557–77. |
[23] | Christ MG , Huesmann H , Nagel H , Kern A , Behl C . Sigma-1 receptor activation induces autophagy and increases proteostasis capacity in vitro and in vivo. Cells. (2019) ;8: :211–211. |
[24] | Ishima T , Fujita Y , Hashimoto K . Interaction of new antidepressants with sigma-1 receptor chaperones and their potentiation of neurite outgrowth in PC12 cells. European Journal of Pharmacology. (2014) ;727: :167–73. |
[25] | Nishimura T , Ishima T , Iyo M , Hashimoto K . Potentiation of nerve growth factor-induced neurite outgrowth by fluvoxamine: role of sigma-1 receptors, IP3 receptors and cellular signaling pathways. PLoS ONE. (2008) ;3: :e2558–e2558. |
[26] | Ishima T , Nishimura T , Iyo M , Hashimoto K . Potentiation of nerve growth factor-induced neurite outgrowth in PC12 cells by donepezil: role of sigma-1 receptors and IP3 receptors. Progress in Neuro-Psychopharmacology and Biological Psychiatry. (2008) ;32: :1656–9. |
[27] | Ishima T , Hashimoto K . Potentiation of nerve growth factor-induced neurite outgrowth in PC12 cells by ifenprodil: the role of sigma-1 and IP3 receptors. PLoS ONE. (2012) ;7: :e37989–e37989. |
[28] | Tsai S-YA , Pokrass MJ , Klauer NR , Nohara H , Su T-P . Sigma-1 receptor regulates Tau phosphorylation and axon extension by shaping p35 turnover via myristic acid. Proceedings of the National Academy of Sciences. (2015) ;112: :6742–47. |
[29] | Prasanth MI , Brimson JM , Chuchawankul S , Sukprasansap M , Tencomnao T . Antiaging, Stress Resistance, and Neuroprotective Efficacies of Cleistocalyx nervosum var. paniala Fruit Extracts Using Caenorhabditis elegans Model. Oxidative Medicine and Cellular Longevity. (2019) ;2019: . |
[30] | Prasanth MI , Sivamaruthi BS , Sukprasansap M , Chucha-wankul S , Tencomnao T. C . Chaiyasut Functional properties and Bioactivities of Cleistocalyx nervosum var. paniala berry plant: a review. Food Science and Technology (2020). |
[31] | Patthamakanokporn O , Puwastien P , Nitithamyong A , Sirichakwal PP . Changes of antioxidant activity and total phenolic compounds during storage of selected fruits. Journal of Food Composition and Analysis. (2008) ;21: :241–8. |
[32] | Charoensin S , Taya S , Wongpornchai S , Wongpoomchai R . Assessment of genotoxicity and antigenotoxicity of an aqueous extract of Cleistocalyx nervosum var. paniala in in vitro and in vivo models. Interdisciplinary Toxicology. (2012) ;5: :201–6. |
[33] | Manosroi J , Chankhampan C , Kumguan K , Manosroi W , Manosroi A . In vitro anti-aging activities of extracts from leaves of Ma Kiang (Cleistocalyx nervosum var. paniala). Pharmaceutical Biology. (2015) ;53: :862–9. |
[34] | Sukprasansap M , Chanvorachote P , Tencomnao T . Cleistocalyx nervosum var. paniala berry fruit protects neurotoxicity against endoplasmic reticulum stress-induced apoptosis. Food and Chemical Toxicology. (2017) ;103: :279–88. |
[35] | Taya S , Punvittayagul C , Inboot W , Fukushima S , Wongpoomchai R . Cleistocalyx nervosum extract ameliorates chemical-induced oxidative stress in early stages of rat hepatocarcinogenesis. Asian Pac J Cancer Prev. (2014) ;15: :2825–30. |
[36] | Khan A , Jahan S , Imtiyaz Z , Alshahrani S , Antar Makeen H , Mohammed Alshehri B , Kumar A , Arafah A , Rehman MU . Neuroprotection: Targeting Multiple Pathways by Naturally Occurring Phytochemicals. Biomedicines. (2020) ;8: :284. |
[37] | Prasanth MI , Santoshram GS , Bhaskar JP , Balamurugan K . Ultraviolet-A triggers photoaging in model nematode Caenorhabditis elegans in a DAF-16 dependent pathway. Age. (2016) ;38: :1–13. |
[38] | Sharika R , Subbaiah P , Balamurugan K . Studies on reproductive stress caused by candidate Gram positive and Gram negative bacteria using model organism. Caenorhabditis elegans, Gene. (2018) ;649: :113–26. |
[39] | Yang J , Huang X-B , Wan Q-L , Ding A-J , Yang Z-L , Qiu M-H , Sun H-Y , Qi S-H , Luo H-R . Otophylloside B protects against Aβ toxicity in Caenorhabditis elegans models of Alzhei-mer’s disease. Natural Products and Bioprospecting. (2017) ;7: :207–14. |
[40] | Qian H , Xu X , Niklason LE . PCH-2 regulates Caenorhabditis elegans lifespan. Aging (Albany NY). (2015) ;7: :1. |
[41] | Deusing DJ , Winter S , Kler A , Kriesl E , Bonnländer B , Wenzel U , Fitzenberger E . A catechin-enriched green tea extract prevents glucose-induced survival reduction in Caenorhabditis elegans through sir-2. 1 and uba-1 dependent hormesis. Fitoterapia. (2015) ;102: :163–70. |
[42] | Prasansuklab A , Meemon K , Sobhon P , Tencomnao T . Ethanolic extract of Streblus asper leaves protects against glutamate-induced toxicity in HT22 hippocampal neuronal cells and extends lifespan of Caenorhabditis elegans. BMC Complementary and Alternative Medicine. (2017) ;17: :1–14. |
[43] | Brimson JM , Prasanth MI , Plaingam W , Tencomnao T . Bacopa monnieri (L.) wettst. Extract protects against glutamate toxicity and increases the longevity of Caenorhabditis elegans. Journal of Traditional and Complementary Medicine. (2020) ;10: :460–70. |
[44] | Boasquívis PF , Silva GMM , Paiva FA , Cavalcanti RM , Nunez CV , de Paula Oliveira R . Guarana (Paullinia cupana) extract protects Caenorhabditis elegans models for Alzheimer disease and Huntington disease through activation of antioxidant and protein degradation pathways. Oxidative Medicine and Cellular Longevity. (2018) ;2018: . |
[45] | Yan F , Chen Y , Azat R , Zheng X . Mulberry anthocyanin extract ameliorates oxidative damage in HepG2 cells and prolongs the lifespan of Caenorhabditis elegans through MAPK and Nrf2 pathways. Oxidative Medicine and Cellular Longevity. (2017) ;2017: . |
[46] | Cagnin M , Ozzano M , Bellio N , Fiorentino I , Follo C , Isidoro C . Dopamine induces apoptosis in APPswe-expressing Neuro2A cells following Pepstatin-sensitive proteolysis of APP in acid compartments. Brain Research. (2012) ;1471: :102–17. |
[47] | Meijering E , Jacob M , Sarria JC , Steiner P , Hirling H , Unser M . Design and validation of a tool for neurite tracing and analysis in fluorescence microscopy images. Cytometry Part A: the journal of the International Society for Analytical Cytology. (2004) ;58: :167–76. |
[48] | Brimson JM , Brimson SJ , Brimson CA , Rakkhitawatthana V , Tencomnao T . Rhinacanthus nasutus Extracts Prevent Glutamate and Amyloid-β Neurotoxicity in HT-22 Mouse Hippocampal Cells: Possible Active Compounds Include Lupeol, Stigmasterol and β-Sitosterol. International Journal of Molecular Sciences. (2012) ;13: :5074–97. |
[49] | Naldi M , Fiori J , Pistolozzi M , Drake AF , Bertucci C , Wu R , Mlynarczyk K , Filipek S , De Simone A , Andrisano V . Amyloid β-peptide 25–35 self-assembly and its inhibition: a model undecapeptide system to gain atomistic and secondary structure details of the Alzheimer’s disease process and treatment. ACS Chemical Neuroscience. (2012) ;3: :952–62. |
[50] | Oda T , Kume T , Katsuki H , Niidome T , Sugimoto H , Akaike A . Donepezil potentiates nerve growth factor-induced neurite outgrowth in PC12 cells. Journal of Pharmacological Sciences. (2007) ;104: :349–54. |
[51] | Takebayashi M , Hayashi T , Su T-P . Nerve growth factor-induced neurite sprouting in PC12 cells involves σ-1 receptors: implications for antidepressants. Journal of Pharmaco-logy and Experimental Therapeutics. (2002) ;303: :1227–37. |
[52] | Murphy CT , McCarroll SA , Bargmann CI , Fraser A , Kamath RS , Ahringer J , Li H , Kenyon C . Genes that act downstream of DAF-16 to influence the lifespan of Caenorhabditis elegans. Nature. (2003) ;424: :277–83. |
[53] | Blackwell TK , Steinbaugh MJ , Hourihan JM , Ewald CY , Isik M . SKN-1/Nrf, stress responses, and aging in Caenorhabditis elegans. Free Radical Biology andMedicine. 2015;290-301. |
[54] | Yu J , Yang H , Fang B , Zhang Z , Wang Y , Dai Y . mfat-1 transgene protects cultured adult neural stem cells against cobalt chloride-mediated hypoxic injury by activating Nrf2/ARE pathways. Journal of Neuroscience Research. (2018) ;96: :87–102. |
[55] | Janda E , Isidoro C , Carresi C , Mollace V . Defective autophagy in Parkinson’s disease: role of oxidative stress. Molecular Neurobiology. (2012) ;46: :639–61. |
[56] | Janda E , Lascala A , Carresi C , Parafati M , Aprigliano S , Russo V , Savoia C , Ziviani E , Musolino V , Morani F . Parkinsonian toxin-induced oxidative stress inhibits basal autophagy in astrocytes via NQO2/quinone oxidoreductase Implications for neuroprotection. Autophagy. (2015) ;11: :1063–80. |
[57] | Resende R , Ferreira-Marques M , Moreira P , Coimbra JR , Baptista SJ , Isidoro C , Salvador JA , Dinis TC , Pereira CF , Santos AE . New BACE1 Chimeric Peptide Inhibitors Selectively PreventAβPPβCleavageDecreasingAmyloid-β Production and Accumulation in Alzheimer’s Disease Models. Journal of Alzheimer’s Disease. 2020;1-21. |
[58] | Dinda B , Dinda M , Kulsi G , Chakraborty A , Dinda S . Therapeutic potentials of plant iridoids in Alzheimer’s and Parkinson’s diseases: A review. European Journal of Medicinal Chemistry. (2019) ;169: :185–99. |
[59] | Castellani RJ , Zhu X , Lee H-G , Smith MA , Perry G . Molecular pathogenesis of Alzheimer’s disease: reductionist versus expansionist approaches. International Journal of Molecular Sciences. (2009) ;10: :1386–406. |
[60] | Millucci L , Ghezzi L , Bernardini G , Santucci A . Conformations and biological activities of amyloid beta peptide 25-35. Current Protein and Peptide Science. (2010) ;11: :54–67. |
[61] | Millucci L , Raggiaschi R , Franceschini D , Terstappen G , Santucci A . Rapid aggregation and assembly in aqueous solution of Aβ (25–35) peptide. Journal of Biosciences. (2009) ;34: :293–303. |
[62] | Chang CY , Lin TY , Lu CW , Huang SK , Wang YC , Chou SSP , Wang SJ . Hesperidin inhibits glutamate release and exerts neuroprotection against excitotoxicity induced by kainic acid in the hippocampus of rats. NeuroToxicology. (2015) ;50: :157–69. |
[63] | Zuo H , Liu X , Wang D , Li Y , Xu X , Peng R , Song T . RKIP-Mediated NF-κB Signaling is involved in ELF-MF-mediated improvement in AD rat. International Journal of Medical Sciences. (2018) ;15: :1658. |
[64] | Wei H , Leeds PR , Qian Y , Wei W , Chen R-w , Chuang D-M . β-Amyloid peptide-induced death of PC 12 cells and cerebellar granule cell neurons is inhibited by long-term lithium treatment. European Journal of Pharmacology. (2000) ;392: :117–23. |
[65] | Butterfield DA , Pocernich CB . The glutamatergic system and Alzheimer’s disease. CNS Drugs. (2003) ;17: :641–52. |
[66] | Edwards A , Gillard M , Merler E . Characterization of muscarinic receptors: type M2 (subtype B) on neuro-2A neuroblastoma cells. Journal of Receptor Research. (1989) ;9: :259–70. |
[67] | Kojima N , Kurosawa N , Nishi T , Hanai N , Tsuji S . Induction of cholinergic differentiation with neurite sprouting by de novo biosynthesis and expression of GD3 and b-series gangliosides in Neuro2a cells. Journal of Biological Chemistry. (1994) ;269: :30451–6. |
[68] | Akahoshi E , Yoshimura S , Uruno S , Ishihara-Sugano M . Effect of dioxins on regulation of tyrosine hydroxylase gene expression by aryl hydrocarbon receptor: a neurotoxicology study. Environmental Health. (2009) ;8: :24–24. |
[69] | Prasansuklab A , Tencomnao T . Amyloidosis in Alzheimer’s disease: The toxicity of amyloid beta (A β), mechanisms of its accumulation and implications of medicinal plants for therapy. Evidence-Based Complementary and Alternative Medicine. (2013) ;2013: :1–30. |
[70] | Wang Y , Zhao C-S . Sigma-1 receptor activation ameliorates LPS-induced NO production and ROS formation through the Nrf2/HO-1 signaling pathway in cultured astrocytes. Neuroscience Letters. (2019) ;711: :134387. |
[71] | Zhao J , Mysona BA , Wang J , Gonsalvez GB , Smith SB , Bollinger KE . Sigma 1 receptor regulates ERK activation and promotes survival of optic nerve head astrocytes. PLoS ONE. (2017) ;12: :e0184421. |
[72] | Liao K-K , Wu M-J , Chen P-Y , Huang S-W , Chiu S-J , Ho C-T , Yen J-H . Curcuminoids promote neurite outgrowth in PC12 cells through MAPK/ERK-and PKC-dependent pathways. Journal of Agricultural and Food Chemistry. (2012) ;60: :433–43. |
[73] | Perron JC , Bixby JL . Distinct neurite outgrowth signaling pathways converge on ERK activation. Molecular and Cellular Neuroscience. (1999) ;13: :362–78. |
[74] | Cleveland DW , Hwo S-Y , Kirschner MW . Purification of tau, a microtubule-associated protein that induces assembly of microtubules from purified tubulin. Journal of Molecular Biology. (1977) ;116: :207–25. |
[75] | Nizzari M , Barbieri F , Gentile MT , Passarella D , Caorsi C , Diaspro A , Taglialatela M , Pagano A , Colucci-D’Amato L , Florio T . Amyloid-β protein precursor regulates phosphorylation and cellular compartmentalization of microtubule associated protein tau. Journal of Alzheimer’s Disease. (2012) ;29: :211–27. |
[76] | Mohandas E , Rajmohan V , Raghunath B . Neurobiology of Alzheimer’s disease. Indian Journal of Psychiatry. (2009) ;51: :55. |
[77] | Wang J , Shanmugam A , Markand S , Zorrilla E , Ganapathy V , Smith SB . Sigma 1 receptor regulates the oxidative stress response in primary retinal Müller glial cells via NRF2 signaling and system xc–, the Na+-independent glutamate–cystine exchanger. Free Radical Biology and Medicine. (2015) ;86: :25–36. |
[78] | Wang J , Zhao J , Cui X , Mysona B , Navneet S , Saul A , Ahuja M , Lambert N , Gazaryan IG , Thomas B . The molecular chaperone sigma 1 receptor mediates rescue of retinal cone photoreceptor cells via modulation of NRF2. Free Radical Biology and Medicine. (2019) ;134: :604–16. |
[79] | Weng T-Y , Hung DT , Su T-P , Tsai S-YA . Loss of Sigma-1 receptor chaperone promotes astrocytosis and enhances the Nrf2 antioxidant defense. Oxidative Medicine and Cellular Longevity. (2017) ;2017: . |
[80] | Sukprasansap M , Chanvorachote P , Tencomnao T . Cyanidin-3-glucoside activates Nrf2-antioxidant response element and protects against glutamate-induced oxidative and endoplasmic reticulum stress in HT22 hippocampal neuronal cells. BMC Complementary Medicine and Therapies. (2020) ;20: :1–12. |
[81] | Inboot W , Taya S , Chailungka A , Meepowpan P , Wongpoomchai R . Genotoxicity and antigenotoxicity of the methanol extract of Cleistocalyx nervosum var. paniala seed using a Salmonella mutation assay and rat liver micronucleus tests. Molecular & Cellular Toxicology. (2012) ;8: :19–24. |
[82] | Maisuthisakul P , Suttajit M , Pongsawatmanit R . Assessment of phenolic content and free radical-scavenging capacity of some Thai indigenous plants. Food Chemistry. (2007) ;100: :1409–18. |
[83] | Yin D . Biochemical basis of lipofuscin, ceroid, and age pigment-like fluorophores. Free Radical Biology and Medicine. (1996) ;21: :871–88. |
[84] | Brunk UT , Terman A . The mitochondrial-lysosomal axis theory of aging: accumulation of damaged mitochondria as a result of imperfect autophagocytosis. European Journal of Biochemistry. (2002) ;269: :1996–2002. |
[85] | Link P , Wink M . Isoliquiritigenin exerts antioxidant activity in Caenorhabditis elegans via insulin-like signaling pathway and SKN-1. Phytomedicine. (2019) ;55: :119–24. |
[86] | Pandey S , Phulara SC , Mishra SK , Bajpai R , Kumar A , Niranjan A , Lehri A , Upreti DK , Chauhan PS . Betula utilis extract prolongs life expectancy, protects against amyloid-β toxicity and reduces Alpha Synuclien in Caenorhabditis elegans via DAF-16 and SKN-1. Comparative Biochemistry and Physiology Part C: Toxicology & Pharmacology. (2020) ;228: :108647. |
[87] | Lin C , Chen Y , Lin Y , Wang X , Hu L , Cao Y , Chen Y . Antistress and anti-aging activities of Caenorhabditis elegans were enhanced by Momordica saponin extract. European Journal of Nutrition. 2020;1-14. |
[88] | Wang L , Pu Z , Li M , Wang K , Deng L , Chen W . Antioxidative and antiapoptosis: Neuroprotective effects of dauricine in Alzheimer’s disease models. Life Sciences. (2020) ;243: :117237. |