Preclinical models of frailty: Focus on interventions and their translational impact: A review
Abstract
The concept of frailty refers to heterogeneity in the risk of adverse outcomes for people of the same age. It is traditionally thought of as the inability of the body to maintain homeostasis. It can help explain differences between chronological and biological age and can quantify healthspan in experimental studies. Although clinical studies have developed tools to quantify frailty over the past two decades, preclinical models of frailty have only recently been introduced. This review describes the notion of frailty and outlines two commonly used clinical approaches to quantify frailty: the frailty phenotype and the frailty index. Translation of these methodologies for use in animals is introduced and studies that use these models to evaluate interventions designed to attenuate or exacerbate frailty are discussed. These include studies involving manipulation of diet, implementation of exercise regimens and tests of pharmaceutical agents to exacerbate or attenuate frailty. Together, this body of work suggests that preclinical frailty assessment tools are a valuable new resource to quantify the impact of interventions on overall health. Future studies could deploy these models to evaluate new frailty therapies, test combinations of interventions and assess interventions to enhance the ability to resist stressors in the setting of ageing.
1Introduction
The number of people aged 60 and older is incre-asing worldwide at an unprecedented rate, so that between 2015 and 2050 the percentage of people in this age group will rise from 12 to 22%[1]. Older individuals accumulate health deficits with age, from the subcellular and cellular levels through to the organ and system levels [2]. These deficits increase an individual’s susceptibility to environmental and phy-siological stressors, which can affect their overall health [3, 4]. In other words, this can compromise healthspan, which can be defined as the portion of an individual’s life spent in generally good health [3]. Vulnerable older people pose challenges in terms of health management, as they have higher rates of adverse health outcomes than do people of the same age who do not have multiple health deficits [4–6]. This is an emerging global health challenge.
The notion that there is heterogeneity in the risk of death in people of the same age was termed “frailty” by demographers in the 1970’s [7]. In clinical practice, the term frailty refers to variability in the risk of adverse outcomes for people of the same age. Since 2001, two approaches to its measurement predominate, with frailty as either a specific syndrome [8] or as a vulnerability state arising from a range of health deficits [9]. The latter can be quantified across the life course as the age-related accumulation of health deficits [2, 10]. The ability to measure frailty provides a valuable tool to quantify underlying heterogeneity in individual health status and predict mortality risk [11]. Because frailty can increase the risk of adverse outcomes including death in older people, strategies that can delay or prevent frailty can improve the quality of life and life expectancy in older people.
Interventions including drug therapies, exercise, and dietary modifications have been proposed to att-enuate ageing, frailty and associated mortality [12–14]. These strategies have recently been investigated in various preclinical models, especially rodents as they are mammals with a relatively short lifespan [15, 16]. Rodents exhibit many deleterious changes in physical appearance, activity levels, strength, cognitive function and behaviour patterns with age [17–22]. These characteristics make them a good choice for preclinical studies designed to investigate frailty and its prevention. In addition, a number of preclinical frailty models have now been developed [15] and used in intervention studies [23]. These investigations have begun to shed light on novel interventions that may be translated to humans [23]. Here we will first review animal models of frailty and then focus on interventions tested in these models that have the potential to treat frail older adults in the future.
2Frailty Measurement
2.1Frailty assessment in humans
Efforts to quantify frailty in clinical practice have used two distinct operational approaches [8, 9]. The frailty phenotype approach was first proposed by Fried and colleagues in 2001 [8]. This approach assesses an individual’s frailty level based on the presence of five criteria: unintentional weight loss, self-reported exhaustion, low grip strength, slow walking speed, and low physical activity [8]. An individual is considered “frail” if they show poor performance in three or more of these criteria [8]. Those with poor performance in one or two criteria are deemed “prefrail” and those with good performance on all measures are considered “robust” [8].
A second common clinical method evaluates frailty with a frailty index. This approach measures the number of health deficits accumulated with increasing age [9, 24]. A frailty index score is created by measuring the number of health deficits in an individual and dividing this by the total number of health deficits considered. If a sufficiently large number of deficits is considered (e.g.>30), the score becomes independent of the precise nature of the items considered [25]. It produces a numerical score between 0 to 1, where values close to “0” indicate low frailty and higher values denote increasing levels of frailty [9]. A frailty index is created from clinical signs and symptoms that can be viewed as health deficits, although a frailty index based solely on standard laboratory tests (called the FI-Lab) has also been developed [26]. There is a sub-maximal limit to frailty, and those with scores near 0.7 cannot survive additional deficits [27].
Although the frailty phenotype and frailty index predict mortality in older individuals [8, 9, 24], there are differences in these two approaches [28]. A key difference is that the frailty phenotype assesses physical frailty only [8]. In contrast, the frailty index measures health deficits across a wide range of systems beyond just physical strength and activity [9, 24]. Still, these two approaches are widely used as clinical frailty assessment tools [29–31]. Other clinical frailty assessment tools including the Tilburg Frailty Index, the FRAIL scale, the Frailty Trait Scale, and the Groningen Frailty Indicator, are described in a recent review [32]. However, only the frailty phenotype and frailty index approaches have been reverse-translated into animal models, as discussed next.
2.2Frailty assessment in laboratory animals
A range of approaches have been used to investigate frailty in preclinical models. Some studies have focused on physical frailty and sarcopenia to develop specialized neuromuscular scoring systems for use in mice [17]. Others have used genetically manipulated animals such as interleukin-10 (IL-10tm/tm) and Cu/Zn superoxide dismutase (Sod1 KO) knockout mice [33, 34]. In addition, the frailty phenotype and frailty index approaches have been recently adapted for use in ageing animals. These tools can not only help us understand the biology of frailty, but they provide a platform for the development of new frailty interventions.
2.2.1The neuromuscular healthspan scoring system
The loss of muscle mass and strength with age is known as sarcopenia, a condition that is closely associated with physical frailty in older people [35]. Graber and colleagues developed a neuromuscular healthspan scoring system (NMHSS) to quantify age- related sarcopenia in ageing mice [17]. This scoring system evaluates muscle contractility and physical strength in individual mice; results show that force production and strength decline with age although there is considerable heterogeneity [17]. The authors suggest that the NMHSS is a sensitive method to assess physical frailty in ageing, where inter-individual variation can limit the true evaluation of neuromuscular health [17]. Although this is a useful approach, the NMHSS is time-consuming, requires specialized equipment and the evaluation of muscle contractility is invasive [17]. Therefore, simpler, less invasive approaches to frailty assessment in animals have been developed.
2.2.2Frailty assessment in animals based on the frailty phenotype
The frailty phenotype was first operationalized in 27-28 month-old male C57BL/6 mice by employing criteria similar to those used for human frailty phenotype assessment [36]. Grip strength, walking speed, physical activity and endurance are assessed in individual mice by setting a cut off value of 1.5 SD below the cohort mean for each criterion. Animals that fall below the cut-off for three of criteria are considered frail, two criteria below are considered mildly frail and fewer than two are considered robust. Comparative analysis shows that the prevalence of frailty is 9%in mice at this age, which is comparable to the prevalence in humans at similar survival ages [36].
These four physical frailty criteria (grip strength, endurance, walking speed, and physical activity) have also been used to quantify frailty in 17-month old male Fischer 344 rats [37]. Rats in the lowest 20%of performance on three or more tests are considered frail. Those in the lowest 20%on two tests are considered mildly-frail and those in the lowest 20%on fewer than two tests are non-frail [37]. The percentage of rats identified as frail (2.3%) in this study is lower than that reported by Liu and colleagues [36, 37]. However, the rats were tested at an age with more than 90%survival, while mice were tested at an age with only 50%survival, so it is not surprising that their frailty prevalence was lower [36, 37]. Thus, both tools identify animals as frail consistent with studies that use the phenotype approach in people [36, 37].
Interestingly, both of these rodent studies omitted the clinically relevant criterion of weight loss [36, 37]. It is possible that these studies did not assess weight loss because they were cross-sectional in design. However, even in a cross-sectional study it may be possible to assess this outcome by comparing an individual’s weight to mean values for mice of the same age and sex. As weight loss is a key component of the frailty phenotype in people [8, 9], other investigators have added this variable to frailty phenotype scoring in animals. For example, the Valencia score is a five-item frailty phenotype instrument for mice that uses weight loss as one component of the scale [38]. These authors show that the Valencia score identifies premature ageing as frailty and that Valencia scores predict lifespan in ageing male mice [39].
Baumann and colleagues evaluated frailty in 14–37 month-old male C57BL/6 mice with a tool that included body weight as well as walking speed, stre-ngth, endurance and physical activity [40]. However, they used weight gain rather than weight loss as a criterion for frailty [40]. Even with weight gain as a criterion, they found that frailty could be seen as early as 17 months, its prevalence increased with age, and frailer mice had higher mortality [40]. Similar results were seen in female mice, where the frailty phenotype also predicted mortality [41]. This work used high body weight as a frailty criterion [40], unlike human frailty phenotype studies where unintentional weight loss is considered a key component of frailty [8, 9]. With respect to weight gain and its relationship to frailty, it is worth noting that some performance measures in mice may need to be adjusted for body mass prior to evaluating frailty with the frailty phenotype approach. Weight gain might also indicate the development of maladaptive organ hypertrophy and/or tumours, which could exacerbate frailty in older animals. It also would be fascinating to compare frailty in mice with low and high body weights, by measuring frailty with a frailty index instrument as outlined later in this review.
One limitation of the frailty phenotype studies in rodents discussed so far is that they used only one sex, typically males. Sex is an important factor that influences the degree of frailty observed in clinical studies [42, 43]. For instance, although women have higher frailty scores than men at any age, they live longer [42, 43]. This so-called morbidity-mortality paradox is compelling, although the underlying mechanisms are unclear. To determine whether this could be detected in animals, male and female C57BL/6 mice were assessed with a five-parameter frailty phenotype instrument [44]. They found that, although the prevalence of frailty increased with age in both sexes, it was greater in 26-month-old females than in males of the same age [44]. However, the females died earlier than the males in this study, so when frailty scores were normalized by mean lifespan there were no sex differences in frailty [44]. This may indicate differences between humans and animal models, although additional studies to explore this are certainly warranted.
The five component frailty phenotype approach has been adapted for use in companion animals [45]. These investigators explored age-associated changes and mortality in domestic dogs of both sexes [45]. The five components modelled after the human instrument include chronic undernutrition, exhaustion, low physical activity level, poor mobility, and weakness [45]. Dogs with two or more of these frailty components are considered frail while those with 1 or less are non-frail [45]. Critically, they show that higher frailty scores increase the risk of death [45].
Together, these observations show that the frailty phenotype is a flexible and potentially powerful instrument that can be used for frailty assessment and mortality prediction not only in humans but in other species including rodents and dogs. As in human work [46], preclinical studies with various modifications to the frailty phenotype tool demonstrate increased risk of adverse outcomes with higher levels of frailty.
2.2.3Experimental knockout models of frailty
The biological basis for frailty is not well understood, although mechanisms involved in the ageing process including inflammation and oxidative stress have been implicated [16]. Indeed, genetically engineered interleukin (IL)-10 (IL-10tm/tm) and Cu/Zn superoxide dismutase (Sod1KO) knockout mice [33, 34] have been proposed as frailty models. These models rely on the inflammation and oxidative stress-associated mechanisms implicated in frailty [47] to mimic aspects of age-associated frailty in people [33, 34].
In the IL-10tm/tm mouse, the anti-inflammatory cytokine IL-10 is knocked out, which results in en-hanced circulating levels of the proinflammatory cytokine IL-6 [33]. These mice exhibit muscle weakness and changes in the expression of genes associated with muscle energetics and apoptosis [33]. IL-10tm/tm knockout mice also exhibit abnormal skeletal muscle energetics and cardiovascular dysfunction [48, 49]. Thus, inflammation, cardiovascular abnormalities and skeletal muscle dysfunction contribute to the phenotype seen in IL-10tm/tm mice. Based on these data, strategies that reduce inflammation may attenuate frailty in older individuals.
An increase in oxidative stress is implicated in the development of frailty [47] so Sod1KO mice have been proposed as a frailty model [34]. These mice lack the antioxidant enzyme Cu/Zn superoxide dismutase, which reduces defence against oxidative stress [34]. Although frailty has not been quantified in these mice, they do exhibit phenotypic components of frailty including weight loss, weakness, low physical activity levels and reduced levels of endurance. Moreover, Sod1KO mice exhibit enhanced sarcopenia and have an abbreviated lifespan [34]. These observations suggest that increasing oxidative stress accelerates frailty and increases mortality. Thus, interventions that reduce oxidative damage, such as antioxidant therapies, may reduce frailty in the setting of ageing.
Although both IL-10tm/tm and Sod1KO models have been developed to investigate frailty [33, 34], there are limitations to their use as a model of frailty. First, these genetically manipulated models do not necessarily represent the natural ageing process and the multitude of mechanisms implicated in the development of frailty [16]. Second, frailty has not been formally quantified in either IL-10tm/tm or Sod1KO mice. It would be important to use either the murine frailty phenotype tool outlined above, or the frailty index approach described in the next section to quantify frailty in these animals.
2.2.4Frailty assessment based on the frailty index approach in animals
Building on more than a decade of clinical work, Howlett and colleagues first adapted the frailty index for use in naturally ageing male and female C57BL/6 mice [50]. This frailty index is based on 31 health deficits derived from open field activity data, body composition measures, hemodynamic data, and serum markers of metabolism. To determine the frailty level of each animal, each deficit is given a score of 0, 0.25, 0.5 0.75 or 1 based on the number of standard deviations (SDs) each deficit differs from mean reference values [50]. The scores for each health deficit are summed and divided by the total number of deficits (e.g. 31) to provide a frailty index score between 0 and 1, as in humans [9, 10]. This tool shows that 30-month-old mice have higher frailty index scores than 12-month old mice [50]. This initial study demonstrates that a frailty index based on deficit accumulation can be reverse-translated from humans to experimental animals [50]. However, limitations to this approach include the use of invasive procedures, time-consuming nature of the techniques used, and requirement for specialized equipment.
To address these limitations, a non-invasive clin-ical frailty index for mice was created by the same group [51]. This frailty index evaluates 31 potential clinical deficits that sample many systems including the integument, musculoskeletal, vestibulococh-lear/auditory, ocular/nasal, digestive/urogenital, and respiratory systems, as well as signs of discomfort, body temperature and body weight parameters [51]. Based on the presence of clinical deficits and their severity, a numerical score is provided for each deficit. Each deficit can be scored as 1 (severe), 0.5 (mild) or 0 (none present). The sum of these individual deficit scores is divided by the total number of deficits measured (e.g. 31) to produce a frailty index score [51]. Interestingly, the mouse clinical frailty index shows a graded increase with age as seen clinically [51]. This instrument also is flexible, as it has recently been adapted for use in other species including rats [52] and dogs [53]. Indeed, the age-associated accumulation of health deficits increases the risk of mortality in both species as well as in mice [53].
The mouse frailty index tool has been validated against data obtained in people across the life course [54]. Key features of deficit accumulation are similar in humans and mice, including the rates of deficit accumulation, submaximal limit to frailty and ability to predict mortality [54]. In addition, a murine frailty index based on laboratory data has been created [55], similar to the human FI-lab [26]. This tool is a collection of 23 standard laboratory tests related to blood pressure, blood chemistry and echocardiography. It has been used to explore mechanisms of frailty development in mice, including studies of the positive relationship between frailty and inflammation [55]. A frailty index based on laboratory plus clinical frailty data showed that female mice have higher frailty scores than males [55], similar to what is seen in people [42, 43]. Thus, quantifying deficit accumulation in mice provides a powerful new translational tool for research on ageing.
The mouse frailty index can be used to evaluate links between frailty and disease, including models of neurodegenerative disease [56]. Todorovic and colleagues used a modified 24-item clinical frailty index to assess the impact of age and sex on frailty in the 5xFAD mouse model of Alzheimer’s disease [56]. They show that frailty scores increase with age in 5xFAD mice and females have lower frailty scores than males [56]. Females also have lower frailty scores than males in the 3xTg-AD mouse model of Alzheimer’s disease [57]. This study also reported that the 3xTg-AD had higher frailty scores than wild-type controls, and that frailty increased mortality risk especially in female 3xTg-AD mice [57]. Together, these observations suggest that the frailty index can be used to investigate the impact of overall health on neurodegenerative disease expression in animal models.
The frailty index also can be used to evaluate the relationship between frailty and cardiovascular dis-ease in mouse models [58–61]. Indeed, the develop-ment of age-related cardiac hypertrophy and contractile dysfunction is graded by frailty in ageing male mice [59]. Further, these maladaptive changes in the structure and function of the ageing ventricle are proportional to, and graded by frailty index scores at the organ, cellular and molecular levels [59]. More recent work has shown that most of the relationships between cardiac ageing and heart function are graded by frailty in males, but not in females [58]. These findings are important as they suggest that frailty promotes maladaptive changes in the heart that may contribute to heart diseases in older males, while females may be resistant to these deleterious effects of frailty. Additional work in both sexes would be important.
The concept of deficit accumulation has also been used to explore the influence of frailty on heart rate and sinoatrial node dysfunction in ageing male mice [61]. This work shows that age-related sinoatrial node (SAN) dysfunction is proportional to and graded by the degree of frailty at the organ, cellular and subcellular levels [61]. These observations indicate that mice with the worst overall health had pronounced changes in atrial structure and function that create the ideal substrate for atrial arrhythmias [60]. Together, these studies strongly suggest that disease expression is modified by overall health and that individuals with high frailty scores exhibit marked signs of disease when compared to age-matched animals with low frailty scores.
3Frailty interventions
Animal models of frailty are an exciting new dev-elopment that offer the chance to systematically in-vestigate interventions that may delay or attenuate the development of frailty. These can be broadly categorized as either non-pharmacological or pharmacological interventions. Interventions such as diet management, calorie restriction (Table-1) and exercise (Table-2) represent non-pharmacological interventions, while drugs and antioxidants are considered pharmacological interventions (Tables-3 & 4). The efficacy of these interventions has been evaluated with the frailty phenotype and/or frailty index in preclinical models, as discussed in the following sections.
Table 1
Influence of diet and calorie restriction on frailty in animal models
Intervention 1 | Frailty assessment | Age | Sex | Animal | Outcomes | Reference |
Calorie restricted diet (40% fewer calories) for 13 mos. | Frailty index | 19 mos | M & F | C57BL/6 mice | Calorie restriction lowered frailty scores in males but not females. | [64] |
Calorie restricted diet (40% fewer calories) for 13 mos | Frailty index | 19 mos | M & F | DBA/2 mice | Calorie restriction reduced frailty scores in male mice with accelerated ageing. | [64] |
Calorie restriction (40% fewer calories) for 6, 12 and 18 mos. | Frailty phenotype (Valencia score) | 12–24 mos | M | Wistar rats | Dietary restriction reduced frailty scores, increased activity, and improved spatial memory. | [65] |
Calorie restriction for12 wks/or longer until ≈37–39 mos of age (40% fewer calories) | Frailty index (modified) | ≈37–39 mos | M & F | Adipose specific mTORC2 null mice | Calorie restriction enhanced longevity and improved fitness in wild-type and insulin-resistant adipose specific mTORC2 null mice of both sexes. | [66] |
High-fat diet (HFD) (35% Lard Diet) for 6.5–26 weeks. | Physiological frailty index | 6.5–26 mos | M & F | NIH Swiss mice | Both sexes gained weight but HFD-induced obesity enhanced frailty and reduced longevity in males only. Females were protected from HFD-induced effects on frailty and longevity. | [67] |
1High-fat diet (HFD).
Table 2
The impact of exercise on frailty in animal models
Treatment/regimen | Frailty assessment | Age | Sex | Animal | Outcomes | Reference |
Voluntary wheel running (4 wks) | Frailty phenotype, frailty intervention assessment value | 28–30 mos | M | C57BL/6 mice | Reversed frailty associated symptoms. Enhanced aerobic capacity, improved muscle mass and strength. | [70] |
Voluntary wheel running (3 mos of age until 14, 17, 20 or 25 mos) | Frailty phenotype (Valencia score) | 17, 20, 23 & 28 mos | M | C57BL/6J mice | Longer period of voluntary wheel running reduced detrimental changes associated with frailty. | [38] |
High-intensity interval training (10-minute uphill treadmill, three times/wks for 4 mos) | Frailty phenotype | 24 mos | M | C57BL/6 mice | Limits negative consequences of frailty. Improved physical performance and strength. Greater muscle mass, fiber cross-sectional area and mitochondrial biomass. | [68] |
High-intensity interval training (10-minute uphill treadmill, three times/wks for 2 mos) | Frailty phenotype, clinical frailty index, frailty intervention assessment value | 24 mos | F | C57BL/6J mice | Reduced frailty, enhanced physical performance, increased strength, and functional capacity (nest-building ability). | [69] |
3.1Diet and related interventions
Diet and calorie balance are important components of daily life that can be manipulated to modify lifespan in animal models [62, 63]. Therefore, studies have explored whether these interventions can affect “healthspan”, quantified as frailty, in preclinical models. These studies are summarized in Table-1.
Several investigations have explored the impact of a low-calorie diet for frailty prevention (Table-1). Kane and colleagues first reported that a calorie-restricted diet (40%fewer calories) for 13 months reduced clinical frailty index scores in older C57BL/6 mice and in DBA/2 mice, which are a model of accelerated ageing [64]. However, the benefits of dietary restriction on frailty are only seen in male mice in both strains [64]. Similar results have been reported in ageing male Wistar rats, where 40%calorie restriction reduced frailty phenotype scores, enhanced physical activity and improved spatial memory [65]. Whether female rats benefit from this was not investigated. Calorie restriction (40%fewer calories) also reduces frailty index scores and enhances longevity in wild-type mice and in insulin-resistant adipose-specific mTORC2 null mice of both sexes [66]. Thus, it seems clear that fewer calories lead to improved overall health as assessed by various frailty measures in mice and rats, although whether this differs between the sexes is controversial.
Only one study explored the influence of a dietary “stressor” on frailty in animal models [67]. These investigators showed that a high-fat diet (35%lard) increased weight in both male and female mice, but it enhanced frailty and reduced lifespan only in males [67]. Thus unlike males, females are protected from detrimental effects of a high fat diet on overall health [67]. This ability to resist a stressor may contribute to the morbidity-mortality paradox, where females have higher frailty scores than males but they live longer [42, 55].
Together, these observations suggest that calorie restriction attenuates frailty progression in ageing male rodents and calorie excess (at least in the form of fat) exacerbates frailty (Table-1). Females may be resistant to both detrimental and beneficial effects of dietary modifications, although this has not been reported in all studies and additional experimental work is required to explore this question.
3.2Exercise and frailty interventions
Age-associated sarcopenia and physical inactivity can lead to disability and death in older adults [14]. In consequence, there has been interest in the role of exercise as an intervention for frailty prevention [14]. Studies in rodent models have explored the impact of different types and intensities of exercise on the development of frailty in the setting of ageing [38, 68–70]. These trials are summarized in Table-2 and are discussed in detail below.
The first study to explore the influence of aerobic exercise on frailty in a preclinical model examined the effect of voluntary wheel running on frailty in mice assessed with the frailty phenotype [70]. They showed that even a brief (e.g. 4 weeks) trial of voluntary wheel running could reverse frailty, improve muscle mass and increase strength in 28–30-month-old male C57BL/6 mice [70]. A much longer duration of voluntary wheel running was also effective at preventing frailty in ageing male C57BL/6 mice evaluated with a modified frailty phenotype instrument called the Valencia Score [38]. One limitation of these studies is that they only used male animals; studies of voluntary exercise in females would be important.
Other studies have assessed the ability of high-intensity interval training to attenuate frailty in ageing animals of both sexes. These studies found that as little as ten minutes of high-intensity interval training three times per week for 8–16 weeks enhanced physical performance and attenuated frailty in ageing male mice [68, 69]. Although the duration of exercise was different for males (16 weeks) and females (8 weeks), high-intensity interval training attenuated frailty as quantified with the both phenotype and frailty index approaches [68, 69]. High-intensity interval training also is associated with greater muscle mass, increased fibre cross-sectional area, and more mitochondrial biomass in males, and improved nest-building activity in females [68]. These studies demonstrate that exercise can attenuate frailty in older male and female rodents (Table-2). However, studies conducted so far have used only one sex to explore effects of exercise on frailty. The impact of various exercise regimens on ageing mice of both sexes in the same study, evaluated with the same frailty tool, could be instructive.
3.3Drugs as frailty interventions
Although drugs that target fundamental ageing mechanisms have been shown to affect lifespan in experimental models [12, 71], less is known about effects on overall health. The recent development of preclinical models of frailty has now allowed investigators to determine whether these agents can affect healthspan. The results of studies testing interventions designed to attenuate frailty are summarized in Table-3. Interventions that can be considered stressors and may exacerbate frailty are summarized in Table-4.
Table 3
Drug treatment regimens that attenuate frailty in animal models
Intervention 1 | Frailty assessment | Age | Sex | Animal | Outcomes | Reference |
Resveratrol (Antioxidant) (100 mg/kg for 6 mos) | Clinical frailty index | 24 mos | M | C57BL/6 mice | Resveratrol reduced frailty scores in male mice. | [64] |
Rapamycin (inhibitor of mTOR complex 1; 7.5–12.5μg/daily in drinking water) in HFD mice (35%Lard Diet) from 6.5 to 26 mos of age. | Physiological Frailty Index (PFI) | 6.5–26 mos | M & F | NIH Swiss mice | Rapamycin increased lifespan of female but not male mice when fed a standard diet. Rapamycin did not affect lifespan of mice fed a HFD regardless of sex, but it did reduce frailty in HFD males only. | [67] |
Diarylpropionitrile (estrogen receptor-β agonist; 3 mg/kg/day in chow). From 7 to 31 mos. | Clinical frailty index | 31 mos | F | OVX CD-1female mice | Drug attenuated frailty in female mice. Improved feeding, reduced anxiety, improved spatial learning/memory, and running performance but did not affect lifespan. | [79] |
Enalapril (angiotensin- converting enzyme inhibitor; 30 mg/kg/day in food; treated middle aged mice for 4 mos and older mice for 5–9 mos). | Clinical frailty index | 12–13 mos and 21–25 mos | M & F | C57BL/6 mice | Enalapril reduced frailty in middle-aged and older female mice and older male mice. Reduced pro-inflammatory cytokines in females and increased anti-inflammatory cytokines in males. | [82] |
Allicin (Garlic component) (4, 8, & 16 mg/kg/day) 8 mos | Clinical frailty index | 13–21 months | M | Fischer 344 rats | Allicin reduced frailty scores, attenuated age-associated bone deformation by enhancing bone formation and bone resorption, enhances bone mineral density | [83] |
Alpha-ketoglutarate (metabolite in the TCA cycle; 2%supplement w/w in chow) | Clinical frailty index | 18 months | M & F | C57BL/6 mice | Alpha-ketoglutarate reduces frailty index scores in both sexes and high frailty scores are associated with reduced lifespan | [84] |
1Physiological Frailty Index (PFI); High-fat diet (HFD); ovariectomized (OVX); tricarboxylic acid (TCA).
Table 4
Interventions that exacerbate frailty in animal models
Intervention 1 | Frailty assessment | Age | Sex | Animal | Outcomes | Reference |
Polypharmacy with simvastatin (20 mg/kg/day), metoprolol (350 mg/kg/day), omeprazole (10 mg/kg/day), acetaminophen (100 mg/kg/day), & citalopram(10 mg/kg/day) for 2–4 wks | Clinical frailty index | 24 mos | M | C57BL/6J mice | Polypharmacy resulted in a decline in mobility, balance, and strength. | [85] |
Monotherapy or polypharmacy for 9–12 mos with simvastatin, metoprolol, acetaminophen,irbesartan & citalopram(doses as above). | Clinical frailty index | 21–24 mos | M | C57BL/6J (B6) mice | Polypharmacy with increasing drug burden index exacerbated frailty. | [89] |
Single intraperitoneal injection of lipopolysaccharide (LPS; 8 mg/kg) to model pre-existing frailty. | Clinical frailty index (modified, 8 components) | 2 mos | M | C57BL/6J mice | Mice with pre-existing frailty were frailer and more susceptible to postoperative complications (e.g. cognitive dysfunction, oxidative damages and neuroinflammation). | [90] |
Diarylpropionitrile (estrogen receptor-β agonist; 3 mg/kg/day in chow from 7 to 31 mos). | Clinical frailty index | 31 mos | F | OVX CD-1 female mice | Exacerbated frailty in aged, OVX female mice. | [79] |
Sublethal whole-body irradiation 3×3 Gray (NDT 320 or X-RAD225, 225 kV; 3-times with 2 recovery days between doses | Clinical frailty index | 5-6 mos | M | C57BL/6 | Irradiation caused premature frailty, adverse cognitive changes, premature mortality and promoted early tumor development. | [94] |
1ovariectomized (OVX).
3.3.1Drug interventions that attenuate frailty in animal models
The first study to demonstrate that drug treatment could attenuate the development of frailty used the antioxidant “resveratrol” [64]. This compound was selected as it is a known longevity intervention that improves many aspects of health and increases lifespan in mice fed a high calorie diet [72]. It also enhances the activity of sirtuin 1, which mediates physiological effects of calorie restriction [73]. Kane and colleagues showed that, when 18-month-old male mice were treated with resveratrol at a dose of 100 mg/kg for 6 months, their frailty index scores were significantly lower than age-matched controls [64]. This work represents the initial “proof-of-concept” that the frailty index is responsive to pharmacological interventions.
The drug “rapamycin” inhibits mammalian/mec-hanistic target of rapamycin (mTOR), a key element in cellular metabolism that regulates processes in-volved in ageing including nutrient sensing, proteostasis, autophagy and cellular senescence [74]. Rapamycin enhances lifespan in preclinical rodent models [75–77] and improves physical strength and endurance in middle-aged mice [77]. Rapamycin treatment also attenuates the development of frailty in NIH Swiss mice challenged with a high fat diet [67]. However, rapamycin reduced frailty index scores in male but not female mice fed a high fat diet [67]. This indicates that rapamycin slows the development of frailty, although reasons for underlying sex differences in responsiveness to rapamycin in mice fed high fat diets are unclear.
Other drugs with anti-ageing properties have been evaluated for potential beneficial effects on frailty in preclinical models. Diarylpropionitrile is an estrogen receptor-β-specific agonist that has anti-aging effects including positive effects on energy metabolism and neuroprotection [78]. Based on these observations, Said and colleagues [79] proposed that it might im-prove healthspan. Indeed, chronic treatment with diarylpropionitrile (3 mg/kg/day) attenuates frailty, improves spatial learning and memory and enhances running in adult, ovariectomized CD-1 female mice [79].
Another approach is to inhibit the renin-angioten-sin system with either angiotensin converting enzyme (ACE) inhibitors or angiotensin II receptor blockers; these drugs can improve grip strength, enhance activity and reduce inflammation in rodents [80, 81]. Interestingly, chronic treatment with the ACE in-hibitor enalapril (30 mg/kg/day) reduces frailty index scores in 12-month old female C57BL/6 mice and in older mice (∼24 months of age) of both sexes [82]. These beneficial effects were attributable to sex-specific effects on inflammation, including a reduction in pro-inflammatory cytokines in female mice and an increase in anti-inflammatory cytokines in male mice [82]. This study was the first longitudinal study to assess drug treatment effects on frailty across the life course.
Yet another approach has exploited the anti-inf-lammatory and anti-osteoporotic properties of allicin, a component of garlic, as a treatment for frailty. Liu and colleagues showed that chronic administration of allicin reduced frailty index scores and protected against senile osteoporosis in ageing Fischer 344 rats [83]. This suggests that chronic administration of allicin may be beneficial in delaying the onset of both frailty and osteoporosis in older adults. A recent novel approach has investigated the impact of treatment with alpha-ketoglutarate, a key metabolite in the tricarboxylic acid (TCA) cycle [84]. This compound was selected based on its positive effects on lifespan in aging mice and other organisms. Results showed that 18-month-old male and female mice treated chronically with alpha-ketoglutarate had lower frailty index scores than mice fed standard chow [84]. Taken together, these studies show that the new frailty assessment tools are useful in detecting beneficial effects of drug treatments on overall health in preclinical models. This should facilitate exploration of novel drug treatments and the influence of combinations of interventions, such as exercise plus drug treatment and/or dietary supplements.
3.3.2Drug interventions that exacerbate frailty in animal models
While some studies have examined strategies to mitigate frailty, others have looked at the impact of stressors on frailty scores. The first study to subject mice to stressors and quantify effects on frailty looked at the ability of polypharmacy to promote frailty and other geriatric outcomes [85]. Polypharmacy is common in clinical practice and is associated with adverse health-related outcomes in older individuals [86, 87]. Huizer-Pajkos and colleagues used a polypharmacy regimen based on five commonly prescribed drugs including a lipid-lowering agent, a beta-blocker, a proton pump inhibitor, an analgesic and an antidepressant [85]. They treated 24-month-old male C57BL/6 mice with simvastatin, metoprolol, omeprazole, acetaminophen, and citalopram (2–4 weeks). Short-term exposure to polypharmacy red-uced strength and locomotion, but had no significant effect on frailty index scores [85]. As polypharmacy impaired motor function and strength, it is possible that a frailty assessment with the frailty phenotype might have reached a different conclusion. More recently the same group explored the impact of various polypharmacy regimens charac-terized by different drug burden indices (DBI) [88]. They administered different drug regimens to middle-aged (12 months) male C57BL/6J mice for up to one year [89]. Polypharmacy regimens with a high DBI as well as monotherapy with citalopram increased frailty when compared to zero and low DBI polypharmacy [89]. By contrast, deprescribing of high DBI drugs markedly reduced frailty index scores [89]. These observations indicate that chronic polypharmacy with increasing DBI increases frailty and that drug withdrawal reverses this effect. This suggests that deprescribing is a potentially useful strategy to reduce the burden of frailty in older adults.
The relationship between frailty and the ability to tolerate a stressor has been investigated in young mice where “frailty” was induced by an injection of lipopolysaccharide (LPS; 8 mg/kg IP) [90]. This study used a modified frailty index tool based on 8 frailty components to first show that LPS increased frailty [90]. They also showed mice with pre-exi-sting LPS-induced frailty were more susceptible to postoperative complications including cognitive dysfunction, neuroinflammation and oxidative after a partial hepatic lobectomy [90]. This suggests that pre-existing frailty may reduce resilience to stressors such as surgery. On the other hand, LPS injection in young mice is not a model of frailty in the setting of natural ageing. Additional studies on links between frailty and tolerance to stressors in preclinical models of both ageing and frailty are justified.
Several other studies have looked at interventions that exacerbate frailty. One recent study showed that, although treatment with the estrogen receptor-ß agonist diarylpropionitrile attenuates frailty in young adult ovariectomized CD-1 female mice it increases frailty in older mice (17–33 months of age) [79]. The reasons for this age-specific differential effect are unclear. However, this does demonstrate the need to investigate frailty interventions across the life course and not just in younger animals [79]. Another preclinical study investigated the influence of radiation therapy on frailty. The rationale is that radiation therapy induces fatigue [91, 92] and reduces physical activity [93], which would be expected to worsen frailty. The impact of radiation on frailty index scores was investigated in male C57BL/6 mice, starting at 20–24 weeks of age [94]. This work shows that mice treated with sublethal irradiation exhibit premature frailty, premature mortality, a decline in cognitive function and early tumour development [94]. This demonstrates that the frailty index may useful to test and validate novel interventions to prevent premature ageing in cancer survivors.
3.3.3Emerging drug studies
Animal models of frailty provide an excellent platform to assess the impact of potential new the-rapies on healthspan in preclinical models. For example, cellular senescence is a process of cell ageing where cells stop dividing and secrete harmful substances including proinflammatory cytokines, chemokines and growth factors that disrupt nearby healthy tissue [95]. The newly described senolytic drugs act to kill senescent cells and suppress this so-called “senescence-associated secretory phenotype” (SASP) [95]. The impact of these agents on frailty in animal models has not yet been investigated. However, weekly treatment with a combination of senolytic drugs (e.g. 5 mg/kg dasatinib and 50 mg/kg quercetin) in the Ercc1–/Δ mouse model of human progeroid syndrome improves symptoms associated with frailty including low grip strength, poor body condition, gait disorders, kyphosis, and tremor [93]. In addition, intermittent treatment of Ercc1–/Δ mice with an inhibitor of heat shock protein 90 (17-DMAG; 10 mg/kg; 3 times per week every 3 weeks) reduces symptoms of frailty including low grip strength, poor body condition, gait disorders, kyphosis, and tremor [96]. As 17-DMAG is a potential senolytic drug [96], these findings suggest that senolytic drugs may attenuate frailty. It would be of interest to investigate this directly with one of the new preclinical frailty assessment tools.
Other anti-ageing interventions may attenuate the development of frailty. For example, the anti-diabetic drug metformin has anti-ageing effects such as reducing inflammation and oxidative stress [97]. Observational studies in animals show that long-term treatment with metformin mimics some benefits of calorie restriction (e.g. improved metabolic status and physical performance) [98]. Metformin also attenuates high fat diet-induced deleterious effects on body composition, metabolism, and motor function in mice [99]. In addition, metformin reduces anxiety-like behaviors and improves strength, behaviour, locomotion, memory, and antioxidant defence in various mouse models [100–103]. These observations suggest that metformin may attenuate frailty in ageing models and studies that investigate this would be informative.
4Summary
Frailty is an emerging area in preclinical ageing research. The ability to quantify frailty with frailty phenotype and frailty index approaches provides some powerful new translational tools for geroscientists, as highlighted in Fig. 1. These instruments already have facilitated the quantification of beneficial effects of interventions including dietary changes, exercise regimens and drug therapies in preclinical models. They have also been used to measure the ability of frail ageing animals to resist various stressors, and thus potentially to estimate resilience. There are now new opportunities to investigate novel drug therapies, repurpose existing therapies and explore the impact of combination therapies on frailty in the setting of ageing. These are exciting times in the emerging field of the biology of frailty.
Fig. 1
Preclinical models of frailty. The development of frailty phenotype and frailty index assessment tools has motivated efforts to test novel interventions that can attenuate or exacerbate frailty.
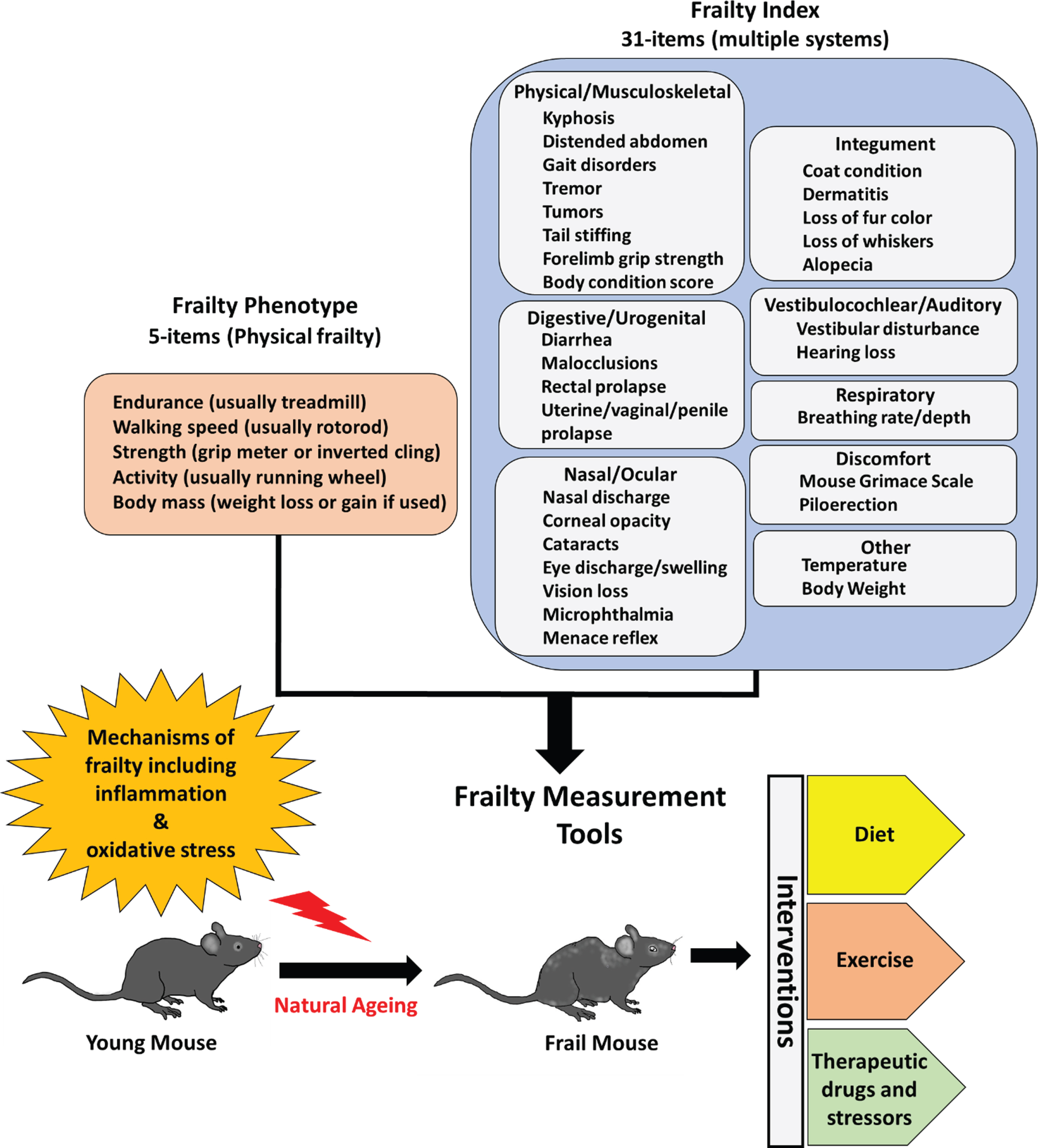
Conflict of interest
Susan E Howlett and Manish Mishra declare no conflict of interest.
Acknowledgments
This work is supported, in part, by grants from the Canadian Institutes of Health Research (PJT 155961; PJT 162462) and the Heart and Stroke Foundation of Canada (HSFC G-19-0026260).
References
[1] | World Health Organization (2018). Ageing and health. factsheets. |
[2] | Rockwood K , Howlett SE . Age-related deficit accumulation and the diseases of ageing. Mech Ageing Dev. (2019) ;180: :107–16. |
[3] | Kaeberlein M . How healthy is the healthspan concept? Geroscience. (2018) ;40: (4):361–4. |
[4] | Clegg A , Young J , Iliffe S , Rikkert MO , Rockwood K . Frailty in elderly people. Lancet. (2013) ;381: (9868):752–62. |
[5] | Collard RM , Boter H , Schoevers RA , Oude Voshaar RC . Prevalence of frailty in community-dwelling older persons: a systematic review. J Am Geriatr Soc. (2012) ;60: (8):1487–92. |
[6] | Yang X , Lupon J , Vidan MT , Ferguson C , Gastelurrutia P , Newton PJ , et al. Impact of Frailty on Mortality and Hospitalization in Chronic Heart Failure: A Systematic Review and Meta-Analysis. J Am Heart Assoc. (2018) ;7: (23):e008251. |
[7] | Vaupel JW , Manton KG , Stallard E . The impact of heterogeneity in individual frailty on the dynamics of mortality. Demography. (1979) ;16: (3):439–54. |
[8] | Fried LP , Tangen CM , Walston J , Newman AB , Hirsch C , Gottdiener J , et al. Frailty in older adults: evidence for a phenotype. J Gerontol A Biol Sci Med Sci. (2001) ;56: (3):M146–56. |
[9] | Mitnitski AB , Mogilner AJ , Rockwood K . Accumulation of deficits as a proxy measure of aging. ScientificWorldJournal. (2001) ;1: :323–36. |
[10] | Rockwood K , Mitnitski A . Frailty defined by deficit accumulation and geriatric medicine defined by frailty. Clin Geriatr Med. (2011) ;27: (1):17–26. |
[11] | Rodriguez-Manas L , Feart C , Mann G , Vina J , Chatterji S , Chodzko-Zajko W , et al. Searching for an operational definition of frailty: a Delphi method based consensus statement: the frailty operative definition-consensus conference project. J Gerontol A Biol Sci Med Sci. (2013) ;68: (1):62–7. |
[12] | Palliyaguru DL , Moats JM , Di Germanio C , Bernier M , de Cabo R . Frailty index as a biomarker of lifespan and healthspan: Focus on pharmacological interventions. Mech Ageing Dev. (2019) ;180: :42–8. |
[13] | Hernandez Morante JJ , Gomez Martinez C , Morillas-Ruiz JM . Dietary Factors Associated with Frailty in Old Adults: A Review of Nutritional Interventions to Prevent Frailty Development. Nutrients. (2019) ;11: (1). |
[14] | Nascimento CM , Ingles M , Salvador-Pascual A , Cominetti MR , Gomez-Cabrera MC , Vina J . Sarcopenia, frailty and their prevention by exercise. Free Radic Biol Med. (2019) ;132: :42–9. |
[15] | Kane AE , Hilmer SN , Mach J , Mitchell SJ , de Cabo R , Howlett SE . Animal models of frailty: current applications in clinical research. Clin Interv Aging. (2016) ;11: :1519–29. |
[16] | Bisset ES , Howlett SE . The biology of frailty in humans and animals: Understanding frailty and promoting translation. Aging Med (Milton). (2019) ;2: (1):27–34. |
[17] | Graber TG , Ferguson-Stegall L , Kim JH , Thompson LV . C57BL/6 neuromuscular healthspan scoring system. J Gerontol A Biol Sci Med Sci. (2013) ;68: (11):1326–36. |
[18] | Justice JN , Carter CS , Beck HJ , Gioscia-Ryan RA , McQueen M , Enoka RM , et al. Battery of behavioral tests in mice that models age-associated changes in human motor function. Age (Dordr). (2014) ;36: (2):583–92. |
[19] | Brooks SV , Faulkner JA . Maximum and sustained power of extensor digitorum longus muscles from young, adult, and old mice. J Gerontol. (1991) ;46: (1):B28–33. |
[20] | Fujita T , Yamashita D , Uehara N , Inokuchi G , Hasegawa S , Otsuki N , et al. A high-fat diet delays age-related hearing loss progression in C57BL/6J mice. PLoS One. (2015) ;10: (1):e0117547. |
[21] | Barreto G , Huang TT , Giffard RG . Age-related defects in sensorimotor activity, spatial learning, and memory in C57BL/6 mice. J Neurosurg Anesthesiol. (2010) ;22: (3):214–9. |
[22] | Fahlstrom A , Yu Q , Ulfhake B . Behavioral changes in aging female C57BL/6 mice. Neurobiol Aging. (2011) ;32: (10):1868–80. |
[23] | Banga S , Heinze-Milne SD , Howlett SE . Rodent models of frailty and their application in preclinical research. Mech Ageing Dev. (2019) ;179: :1–10. |
[24] | Rockwood K , Mitnitski A . Frailty in relation to the accumulation of deficits. J Gerontol A Biol Sci Med Sci. (2007) ;62: (7):722–7. |
[25] | Rockwood K , Andrew M , Mitnitski A . A comparison of two approaches to measuring frailty in elderly people. J Gerontol A Biol Sci Med Sci. (2007) ;62: (7):738–43. |
[26] | Howlett SE , Rockwood MR , Mitnitski A , Rockwood K . Standard laboratory tests to identify older adults at increased risk of death. BMC Med. (2014) ;12: :171. |
[27] | Rockwood K , Mitnitski A . Limits to deficit accumulation in elderly people. Mech Ageing Dev. (2006) ;127: (5):494–6. |
[28] | Cesari M , Gambassi G , van Kan GA , Vellas B . The frailty phenotype and the frailty index: different instruments for different purposes. Age Ageing. (2014) ;43: (1):10–2. |
[29] | Dent E , Kowal P , Hoogendijk EO . Frailty measurement in research and clinical practice: A review. Eur J Intern Med. (2016) ;31: :3–10. |
[30] | Chang SF , Lin PL . Frail phenotype and mortality prediction: a systematic review and meta-analysis of prospective cohort studies. Int J Nurs Stud. (2015) ;52: (8):1362–74. |
[31] | Ritt M , Schwarz C , Kronawitter V , Delinic A , Bollheimer LC , Gassmann KG , et al. Analysis of Rockwood et Al’s Clinical Frailty Scale and Fried et Al’s Frailty Phenotype as Predictors of Mortality and Other Clinical Outcomes in Older Patients Who Were Admitted to a Geriatric Ward. J Nutr Health Aging. (2015) ;19: (10):1043–8. |
[32] | Dent E , Martin FC , Bergman H , Woo J , Romero-Ortuno R , Walston JD . Management of frailty: opportunities, challenges, and future directions. Lancet. (2019) ;394: (10206):1376–86. |
[33] | Walston J , Fedarko N , Yang H , Leng S , Beamer B , Espinoza S , et al. The physical and biological characterization of a frail mouse model. J Gerontol A Biol Sci Med Sci. (2008) ;63: (4):391–8. |
[34] | Deepa SS , Bhaskaran S , Espinoza S , Brooks SV , McArdle A , Jackson MJ , et al. A new mouse model of frailty: the Cu/Zn superoxide dismutase knockout mouse. Geroscience. (2017) ;39: (2):187–98. |
[35] | Wilkinson DJ , Piasecki M , Atherton PJ . The age-related loss of skeletal muscle mass and function: Measurement and physiology of muscle fibre atrophy and muscle fibre loss in humans. Ageing Res Rev. (2018) ;47: :123–32. |
[36] | Liu H , Graber TG , Ferguson-Stegall L , Thompson LV . Clinically relevant frailty index for mice. J Gerontol A Biol Sci Med Sci. (2014) ;69: (12):1485–91. |
[37] | Miller MG , Thangthaeng N , Shukitt-Hale B . A Clinically Relevant Frailty Index for Aging Rats. J Gerontol A Biol Sci Med Sci. (2017) ;72: (7):892–6. |
[38] | Gomez-Cabrera MC , Garcia-Valles R , Rodriguez-Manas L , Garcia-Garcia FJ , Olaso-Gonzalez G , Salvador-Pascual A , et al. A New Frailty Score for Experimental Animals Based on the Clinical Phenotype: Inactivity as a Model of Frailty. J Gerontol A Biol Sci Med Sci. (2017) ;72: (7):885–91. |
[39] | Martinez de Toda I , Garrido A , Vida C , Gomez-Cabrera MC , Vina J , De la Fuente M . Frailty Quantified by the “Valencia Score” as a Potential Predictor of Lifespan in Mice. J Gerontol A Biol Sci Med Sci. (2018) ;73: (10):1323–9. |
[40] | Baumann CW , Kwak D , Thompson LV . Assessing onset, prevalence and survival in mice using a frailty phenotype. Aging (Albany NY). (2018) ;10: (12):4042–53. |
[41] | Kwak D , Baumann CW , Thompson LV . Identifying Characteristics of Frailty in Female Mice Using a Phenotype Assessment Tool. J Gerontol A Biol Sci Med Sci. (2020) ;75: (4):640–6. |
[42] | Gordon EH , Peel NM , Samanta M , Theou O , Howlett SE , Hubbard RE . Sex differences in frailty: A systematic review and meta-analysis. Exp Gerontol. (2017) ;89: :30–40. |
[43] | Shi J , Yang Z , Song X , Yu P , Fang X , Tang Z , et al. Sex differences in the limit to deficit accumulation in late middle-aged and older Chinese people: results from the Beijing Longitudinal Study of Aging. J Gerontol A Biol Sci Med Sci. (2014) ;69: (6):702–9. |
[44] | Baumann CW , Kwak D , Thompson LV . Sex-specific components of frailty in C57BL/6 mice. Aging (Albany NY). (2019) ;11: (14):5206–14. |
[45] | Hua J , Hoummady S , Muller C , Pouchelon JL , Blondot M , Gilbert C , et al. Assessment of frailty in aged dogs. Am J Vet Res. (2016) ;77: (12):1357–65. |
[46] | Theou O , Cann L , Blodgett J , Wallace LM , Brothers TD , Rockwood K . Modifications to the frailty phenotype criteria: Systematic review of the current literature and investigation of 262 frailty phenotypes in the Survey of Health, Ageing, and Retirement in Europe. Ageing Res Rev. (2015) ;21: :78–94. |
[47] | Kane AE , Sinclair DA . Frailty biomarkers in humans and rodents: Current approaches and future advances. Mech Ageing Dev. (2019) ;180: :117–28. |
[48] | Akki A , Yang H , Gupta A , Chacko VP , Yano T , Leppo MK , et al. Skeletal muscle ATP kinetics are impaired in frail mice. Age (Dordr). (2014) ;36: (1):21–30. |
[49] | Sikka G , Miller KL , Steppan J , Pandey D , Jung SM , Fraser CD , 3rd, et al. Interleukin 10 knockout frail mice develop cardiac and vascular dysfunction with increased age. Exp Gerontol. (2013) ;48: (2):128–35. |
[50] | Parks RJ , Fares E , Macdonald JK , Ernst MC , Sinal CJ , Rockwood K , et al. A procedure for creating a frailty index based on deficit accumulation in aging mice. J Gerontol A Biol Sci Med Sci. (2012) ;67: (3):217–27. |
[51] | Whitehead JC , Hildebrand BA , Sun M , Rockwood MR , Rose RA , Rockwood K , et al. A clinical frailty index in aging mice: comparisons with frailty index data in humans. J Gerontol A Biol Sci Med Sci. (2014) ;69: (6):621–32. |
[52] | Yorke A , Kane AE , Hancock Friesen CL , Howlett SE , O’Blenes S . Development of a Rat Clinical Frailty Index. J Gerontol A Biol Sci Med Sci. (2017) ;72: (7):897–903. |
[53] | Banzato T , Franzo G , Di Maggio R , Nicoletto E , Burti S , Cesari M , et al. A Frailty Index based on clinical data to quantify mortality risk in dogs. Sci Rep. (2019) ;9: (1):16749. |
[54] | Rockwood K , Blodgett JM , Theou O , Sun MH , Feridooni HA , Mitnitski A , et al. A Frailty Index Based On Deficit Accumulation Quantifies Mortality Risk in Humans and in Mice. Sci Rep. (2017) ;7: :43068. |
[55] | Kane AE , Keller KM , Heinze-Milne S , Grandy SA , Howlett SE . A Murine Frailty Index Based on Clinical and Laboratory Measurements: Links Between Frailty and Pro-inflammatory Cytokines Differ in a Sex-Specific Manner. J Gerontol A Biol Sci Med Sci. (2019) ;74: (3):275–82. |
[56] | Todorovic S , Loncarevic-Vasiljkovic N , Jovic M , Sok-anovic S , Kanazir S , Mladenovic Djordjevic A . Frailty index and phenotype frailty score: Sex- and age-related differences in 5XFAD transgenic mouse model of Alzheimer’s disease. Mech Ageing Dev. (2020) ;185: :111195. |
[57] | Kane AE , Shin S , Wong AA , Fertan E , Faustova NS , Howlett SE , et al. Sex Differences in Healthspan Predict Lifespan in the 3xTg-AD Mouse Model of Alzheimer’s Disease. Front Aging Neurosci. (2018) ;10: :172. |
[58] | Kane AE , Bisset ES , Keller KM , Ghimire A , Pyle WG , Howlett SE . Age, Sex and Overall Health, Measured As Frailty, Modify Myofilament Proteins in Hearts From Naturally Aging Mice. Sci Rep. (2020) ;10: (1):10052. |
[59] | Feridooni HA , Kane AE , Ayaz O , Boroumandi A , Polidovitch N , Tsushima RG , et al. The impact of age and frailty on ventricular structure and function in C57BL/6J mice. J Physiol. (2017) ;595: (12):3721–42. |
[60] | Jansen HJ , Moghtadaei M , Mackasey M , Rafferty SA , Bogachev O , Sapp JL , et al. Atrial structure, function and arrhythmogenesis in aged and frail mice. Sci Rep. (2017) ;7: :44336. |
[61] | Moghtadaei M , Jansen HJ , Mackasey M , Rafferty SA , Bogachev O , Sapp JL , et al. The impacts of age and frailty on heart rate and sinoatrial node function. J Physiol. (2016) ;594: (23):7105–26. |
[62] | Simpson SJ , Le Couteur DG , James DE , George J , Gunton JE , Solon-Biet SM , et al. The Geometric Framework for Nutrition as a tool in precision medicine. Nutr Healthy Aging. (2017) ;4: (3):217–26. |
[63] | Krittika S , Yadav P . An overview of two decades of diet restriction studies using Drosophila. Biogerontology. (2019) ;20: (6):723–40. |
[64] | Kane AE , Hilmer SN , Boyer D , Gavin K , Nines D , Howlett SE , et al. Impact of Longevity Interventions on a Validated Mouse Clinical Frailty Index. J Gerontol A Biol Sci Med Sci. (2016) ;71: (3):333–9. |
[65] | Todorovic ST , Smiljanic KR , Ruzdijic SD , Djordjevic ANM , Kanazir SD . Effects of Different Dietary Protocols on General Activity and Frailty of Male Wistar Rats During Aging. J Gerontol A Biol Sci Med Sci. (2018) ;73: (8):1036–44. |
[66] | Yu D , Tomasiewicz JL , Yang SE , Miller BR , Wakai MH , Sherman DS , et al. Calorie-Restriction-Induced Insulin Sensitivity Is Mediated by Adipose mTORC2 and Not Required for Lifespan Extension. Cell Rep. (2019) ;29: (1):236–48 e3. |
[67] | Antoch MP , Wrobel M , Kuropatwinski KK , Gitlin I , Leonova KI , Toshkov I , et al. Physiological frailty index (PFI): quantitative in-life estimate of individual biological age in mice. Aging (Albany NY). (2017) ;9: (3):615–26. |
[68] | Seldeen KL , Lasky G , Leiker MM , Pang M , Personius KE , Troen BR . High Intensity Interval Training Improves Physical Performance and Frailty in Aged Mice. J Gerontol A Biol Sci Med Sci. (2018) ;73: (4):429–37. |
[69] | Seldeen KL , Redae YZ , Thiyagarajan R , Berman RN , Leiker MM , Troen BR . High intensity interval training improves physical performance in aged female mice: A comparison of mouse frailty assessment tools. Mech Ageing Dev. (2019) ;180: :49–62. |
[70] | Graber TG , Ferguson-Stegall L , Liu H , Thompson LV . Voluntary Aerobic Exercise Reverses Frailty in Old Mice. J Gerontol A Biol Sci Med Sci. (2015) ;70: (9):1045–58. |
[71] | Piskovatska V , Strilbytska O , Koliada A , Vaiserman A , Lushchak O . Health Benefits of Anti-aging Drugs. Subcell Biochem. (2019) ;91: :339–92. |
[72] | Baur JA , Pearson KJ , Price NL , Jamieson HA , Lerin C , Kalra A , et al. Resveratrol improves health and survival of mice on a high-calorie diet. Nature. (2006) ;444: (7117):337–42. |
[73] | Guarente L , Picard F . Calorie restriction–the SIR2 connection. Cell. (2005) ;120: (4):473–82. |
[74] | Papadopoli D , Boulay K , Kazak L , Pollak M , Mallette F , Topisirovic I , et al. mTOR as a central regulator of lifespan and aging. F1000Res. 2019;8. |
[75] | Harrison DE , Strong R , Sharp ZD , Nelson JF , Astle CM , Flurkey K , et al. Rapamycin fed late in life extends lifespan in genetically heterogeneous mice. Nature. 460. (2009) ;460: (7253):392–5. |
[76] | Zhang Y , Bokov A , Gelfond J , Soto V , Ikeno Y , Hubbard G , et al. Rapamycin extends life and health in C57BL/6 mice. J Gerontol A Biol Sci Med Sci. (2014) ;69: (2):119–30. |
[77] | Bitto A , Ito TK , Pineda VV , LeTexier NJ , Huang HZ , Sutlief E , et al. Transient rapamycin treatment can increase lifespan and healthspan in middle-aged mice. Elife. 2016;5. |
[78] | Rettberg JR , Yao J , Brinton RD . Estrogen: a master regulator of bioenergetic systems in the brain and body. Front Neuroendocrinol. (2014) ;35: (1):8–30. |
[79] | Said SA , Isedowo R , Guerin C , Nar NN , Lillie L , Bukovac S , et al. Effects of long-term dietary administration of estrogen receptor-beta agonist diarylpropionitrile on ovariectomized female ICR (CD-1) mice. Geroscience. (2018) ;40: (4):393–403. |
[80] | Carter CS , Giovannini S , Seo DO , DuPree J , Morgan D , Chung HY , et al. Differential effects of enalapril and losartan on body composition and indices of muscle quality in aged male Fischer 344 x Brown Norway rats. Age (Dordr). (2011) ;33: (2):167–83. |
[81] | Lin CH , Yang H , Xue QL , Chuang YF , Roy CN , Abadir P , et al. Losartan improves measures of activity, inflammation, and oxidative stress in older mice. Exp Gerontol. (2014) ;58: :174–8. |
[82] | Keller K , Kane A , Heinze-Milne S , Grandy SA , Howlett SE . Chronic Treatment With the ACE Inhibitor Enalapril Attenuates the Development of Frailty and Differentially Modifies Pro- and Anti-inflammatory Cytokines in Aging Male and Female C57BL/6 Mice. J Gerontol A Biol Sci Med Sci. (2019) ;74: (8):1149–57. |
[83] | Liu Y , You M , Shen J , Xu Y , Li L , Wang D , et al. Allicin Reversed the Process of Frailty in Aging Male Fischer 344 Rats With Osteoporosis. J Gerontol A Biol Sci Med Sci. (2020) ;75: (5):821–5. |
[84] | Asadi Shahmirzadi A , Edgar D , Liao CY , Hsu YM , Lucanic M , Asadi Shahmirzadi A , et al. Alpha-Ketoglutarate, an Endogenous Metabolite, Extends Lifespan and Compresses Morbidity in Aging Mice. Cell Metab. (2020) ;32: (3):447–56 e6. |
[85] | Huizer-Pajkos A , Kane AE , Howlett SE , Mach J , Mitchell SJ , de Cabo R , et al. Adverse Geriatric Outcomes Secondary to Polypharmacy in a Mouse Model: The Influence of Aging. J Gerontol A Biol Sci Med Sci. (2016) ;71: (5):571–7. |
[86] | Payne RA , Avery AJ , Duerden M , Saunders CL , Simpson CR , Abel GA . Prevalence of polypharmacy in a Scottish primary care population. Eur J Clin Pharmacol. (2014) ;70: (5):575–81. |
[87] | Gnjidic D , Hilmer SN , Blyth FM , Naganathan V , Waite L , Seibel MJ , et al. Polypharmacy cutoff and outcomes: five or more medicines were used to identify community-dwelling older men at risk of different adverse outcomes. J Clin Epidemiol. (2012) ;65: (9):989–95. |
[88] | Hilmer SN , Mager DE , Simonsick EM , Cao Y , Ling SM , Windham BG , et al. A drug burden index to define the functional burden of medications in older people. Arch Intern Med. (2007) ;167: (8):781–7. |
[89] | Mach J , Gemikonakli G , Logan C , Vander Wyk B , Allore H , Ekambareshwar S , et al. Chronic polypharmacy with increasing Drug Burden Index (DBI) exacerbates frailty and impairs physical function, with effects attenuated by deprescribing, in aged mice. J Gerontol A Biol Sci Med Sci. 2020. |
[90] | Tang Y , Wang X , Zhang S , Duan S , Qing W , Chen G , et al. Pre-existing weakness is critical for the occurrence of postoperative cognitive dysfunction in mice of the same age. PLoS One. (2017) ;12: (8):e0182471. |
[91] | Renner M , Feng R , Springer D , Chen MK , Ntamack A , Espina A , et al. A murine model of peripheral irradiation-induced fatigue. Behav Brain Res. (2016) ;307: :218–26. |
[92] | Wolff BS , Renner MA , Springer DA , Saligan LN . AMouse Model of Fatigue Induced by Peripheral Irradiation. J Vis 1139 Exp. 2017(121). |
[93] | Zhu Y , Tchkonia T , Pirtskhalava T , Gower AC , Ding H , Giorgadze N , et al. The Achilles’ heel of senescent cells: from transcriptome to senolytic drugs. Aging Cell. (2015) ;14: (4):644–58. |
[94] | Fielder E , Weigand M , Agneessens J , Griffin B , Parker C , Miwa S , et al. Sublethal whole-body irradiation causes progressive premature frailty in mice. Mech Ageing Dev. (2019) ;180: :63–9. |
[95] | Khosla S , Farr JN , Tchkonia T , Kirkland JL . The role of cellular senescence in ageing and endocrine disease. Nat Rev Endocrinol. (2020) ;16: (5):263–75. |
[96] | Fuhrmann-Stroissnigg H , Ling YY , Zhao J , McGowan SJ , Zhu Y , Brooks RW , et al. Identification of HSP90 inhibitors as a novel class of senolytics. Nat Commun. (2017) ;8: (1):422. |
[97] | Espinoza SE , Jiwani R , Wang J , Wang CP . Review of Interventions for the Frailty Syndrome and the Role of Metformin as a Potential Pharmacologic Agent for Frailty Prevention. Clin Ther. (2019) ;41: (3):376–86. |
[98] | Martin-Montalvo A , Mercken EM , Mitchell SJ , Palacios HH , Mote PL , Scheibye-Knudsen M , et al. Metformin improves healthspan and lifespan in mice. Nat Commun. (2013) ;4: :2192. |
[99] | Allard JS , Perez EJ , Fukui K , Carpenter P , Ingram DK , de Cabo R . Prolonged metformin treatment leads to reduced transcription of Nrf2 and neurotrophic factors without cognitive impairment in older C57BL/6J mice. Behav Brain Res. (2016) ;301: :1–9. |
[100] | Alfaras I , Mitchell SJ , Mora H , Lugo DR , Warren A , Navas-Enamorado I , et al. Health benefits of late-onset metformin treatment every other week in mice. NPJ Aging Mech Dis. (2017) ;3: :16. |
[101] | Fatemi I , Heydari S , Kaeidi A , Shamsizadeh A , Hakimizadeh E , Khaluoi A , et al. Metformin ameliorates the age-related changes of d-galactose administration in ovariectomized mice. Fundam Clin Pharmacol. (2018) ;32: (4):392–9. |
[102] | Fatemi I , Khaluoi A , Kaeidi A , Shamsizadeh A , Heydari S , Allahtavakoli MA . Protective effect of metformin on D-galactose-induced aging model in mice. Iran J Basic Med Sci. (2018) ;21: (1):19–25. |
[103] | Zakeri M , Fatemi I , Kaeidi A , Zakeri MA , Hakimizadeh E , Hassanipour M , et al. Pro-neurocognitive and anti-sarcopenic benefits of one-year metformin therapy in ovariectomized aged mice. Clin Exp Pharmacol Physiol. (2019) ;46: (12):1133–40. |